- 1Department of Biology, Stanford University, Stanford, CA, United States
- 2Institute of Earth Surface Dynamics, University of Lausanne, Lausanne, Switzerland
- 3Department of Biosciences, University of Milan, Milan, Italy
- 4Betty and Gordon Moore Center for Science, Conservation International, Arlington, VA, United States
- 5Department of Biological Sciences, Northern Arizona University, Flagstaff, AZ, United States
- 6Departamento de Ecologia, Universidade de São Paulo, São Paulo, Brazil
- 7Stanford Woods Institute for the Environment, Stanford University, Stanford, CA, United States
Mutualistic interactions are regulated by plant and animal traits, including animal body size and population density. In seed dispersal networks, frugivore body size determines the interaction outcome, and species population density determines interaction probability through encounter rates. To date, most studies examining the relative role of body size and population density in seed dispersal networks have examined animal guilds encompassing a narrow range of body sizes (e.g., birds only). Given non-random, body-size dependent defaunation, understanding the relative role of these traits is important to predict and, ideally, mitigate the effects of defaunation. We analyzed a hyper-diverse seed dispersal network composed of birds and mammals that cover a wide range of body sizes and population densities in the Brazilian Pantanal. Animal density per se did not significantly explain interaction patterns. Instead, population biomass, which represents the combination of body size and population density, was the most important predictor for most interaction network metrics. Population biomass was strongly correlated with body size, but not with density. Thus, larger frugivore species dispersed more plant species and were involved in more unique pairwise interactions than smaller species. Moreover, species with larger population biomass had the strongest influence (i.e., as indicated by measures of centrality) on other species in the network and were more generalist, interacting with a broader set of species, compared to species with lower population biomass. We posit that the increased abundance of small-sized frugivores resulting from the pervasive defaunation of large vertebrates would not compensate for the loss-of-function of the latter and the inherent disruption of seed dispersal networks.
Introduction
The Anthropocene defaunation resulting from global environmental change constitutes a driver of further biodiversity change (Dirzo et al., 2014). Large vertebrate species are more frequently affected by anthropogenic change because they are more intensively overexploited and more sensitive to disturbance than small vertebrates (Peres, 1990; Young et al., 2016). Large frugivores are potentially more important for seed dispersal networks than smaller frugivores in connecting different groups of species (Vidal et al., 2014; Guimarães, 2020), and their disappearance can lead to changes in plant communities due to disruptions in animal and plant trait-matching (Schleuning et al., 2015; Donoso et al., 2020).
Vertebrate population density decline leads to local extinctions of plant-animal interactions, including seed dispersal, even before species extinctions take place (Janzen, 1974; Redford, 1992; Säterberg et al., 2013; Valiente-Banuet et al., 2015). Extinction of interactions may be differentially affected by traits and population characteristics of the vanishing vertebrates. For example, large mammal defaunation and its associated changes in plant-animal interactions can cascade to long-term changes in plant communities, changing the structure and diversity of regeneration understories (Dirzo and Miranda, 1990; Villar et al., 2020; Villar and Medici, 2021; Souza et al., 2022), and reducing tropical forest aboveground biomass and carbon stocks (Peres et al., 2016). However, large frugivore defaunation can also lead to ecological release of smaller frugivores that could, potentially, compensate for the lack of large vertebrates (Jansen et al., 2012; but see McConkey and Drake, 2015). If seed dispersal interaction patterns are structured by animal population density, then one would expect an ecological release to compensate for such functional roles. On the other hand, if interactions are more structured by animal body size, then compensation is unlikely. Understanding to what extent smaller-sized species may compensate for the loss of larger ones is critical to predict the effects of differential defaunation (sensu Dirzo et al., 2014) on seed dispersal networks.
Vertebrate body size regulates seed dispersal interactions mainly through energy requirements, movement, and trait-matching. Larger-sized animals generally require more fruits than smaller animals to fulfill their energy requirements (Martin, 1985), which leads to foraging on a wider number of plant species, or consuming more fruits per plant (Fleming, 1991; Palacio et al., 2016). Furthermore, large-sized frugivores can consume a wider range of plant species due to their ability of swallowing both large and small-sized seeds (Jordano, 2000; Burns, 2013). For example, small-gaped birds may only be able to disperse small seeds (e.g., Galetti et al., 2013). Moreover, larger animals can forage across larger distances, having access to a wider range of fruit species (Jordano et al., 2007). In turn, animal density is expected to affect the frequency of interactions, such that more abundant species can interact more frequently and with more plant species (Vázquez et al., 2009, 2007). Furthermore, seed dispersal interactions may also be modulated by a combination of animal population density and body size (hereafter, population biomass). Populations with high biomass may represent very abundant species with small body size, non-abundant species with large body size, or abundant species with large body size. In non-hunted sites within the Brazilian Amazon, frugivore species with highest body size had stronger contributions to local population biomass, and declines in frugivore population biomass were caused by a disproportionate impact on larger-bodied frugivores, with possible consequences on seed dispersal and forest aboveground biomass (Peres et al., 2016).
Isolating the role of species density from body size in determining dispersal interaction outcomes requires observation of seed dispersal in a community that shows a natural range of density and traits, including size. However, interaction patterns have been mostly studied in communities with a low range in body size, such as bats or birds (Rezende et al., 2007; Laurindo et al., 2020). There are three potential explanations for the lack of studies in systems with a broader range in body size: (1) studies mostly take place in areas subjected to human disturbances that truncate the natural variation in body size within a community (Vidal et al., 2013); (2) a methodological focus on specific functional guilds (such as birds and bats) due to researchers’ expertise or preference (Vidal et al., 2013; but see Timóteo et al., 2018); and (3) to record seed dispersal interactions from a more diverse functional guild, a suite of different methods may be required (e.g., Quintero et al., 2022).
To address this knowledge gap, we analyzed a hyper-diverse tropical seed dispersal network that comprises a frugivore assemblage of birds and mammals with a wide range of body size and population density (Supplementary Table 1) to assess the role of animal population density, body size and biomass in determining seed dispersal interaction patterns. In particular, we asked whether and to what extent animal body size, population density or population biomass, explain the role of species in this interaction network.
Methods
Study Site and Data Collection
Data used in this study were collected in two non-defaunated sites within the Brazilian Pantanal, the largest wetland ecosystem in the world: Rio Negro (19°34′15″S, 56°14′43″W) and Barranco Alto farms (19°34′40″S, 56°09′08″W), totaling 18,500 ha. The vegetation consists of seasonally flooded savannas, semi-deciduous and gallery forests (Prance and Schaller, 1982).
Donatti et al. (2011) used various methods to detect seed dispersers and seed dispersal, interactions mediated by the vertebrate frugivore assemblage at both sites. These methods included plant focal observations to record bird seed dispersal, camera trapping below fruiting trees to record mammal and bird seed dispersal and analysis of mammalian scats (Donatti et al., 2011). Vertebrate occurrence was surveyed independently from interaction observations, using line-transects, as described in Peres (2000), that totaled 196 km (see Donatti, 2011 for a detailed description). Density estimates were obtained for ten mammal and six bird species (Supplementary Table 1) by dividing the number of animals from a particular species observed by the surveyed area. For each animal species, the total area surveyed was computed by multiplying the distance surveyed by the average distance of animals of that particular species from the transect (Sutherland, 1996). Animal species body size was obtained from Fonseca et al. (1996), Mata et al. (2006), and Van Perlo (2015), and species population biomass was calculated as a product of each species body size and its local density. As we only had density estimates for 16 animal species, we used a subset of the original network presented in Donatti et al. (2011) when analyzing the relationship between animal traits and network metrics. However, we calculated network metrics based on the full seed dispersal network (see section “Data Analysis”).
Data Analysis
To assess the relative importance of animal population density, body size and biomass in structuring seed dispersal networks, we first calculated species-level metrics based on the entire Donatti et al. (2011) network comprising 46 animal species of birds, mammals, reptile and fish (excluding only the exotic feral pig Sus scrofa), and 46 plant species. We computed the following species-level interaction metrics: interaction richness (i.e., the number of plant species each frugivore consumed), interaction records (i.e., interaction events, or the total number of feeding records for each animal species), interaction diversity (i.e., the diversity of plant species consumed by each animal species), closeness centrality (i.e., how connected – through direct or indirect pathways – each animal species is to other species in the network), c-score (i.e., how evenly distributed are the interactions of a given species across all modules in the network, in which a module is a semi-independent cohesive group of interacting species) and z-score (i.e., a standardized measure of the number of interactions each species has within its own module). For these computations we used the bipartite package (Dormann, 2011) in R version 4.1.1 (R Core Team, 2021).
We then used these species-level interaction metrics as response variables. We used log-transformed population density, body size and biomass as explanatory variables. We applied log-transformation to predictor variables due to data skewness and ecologically meaningful outliers. First, we assessed the correlations between predictor variables using Pearson’s correlation coefficient. Population density and species body size (r = −0.32, t = −1.29, df = 14, p-value = 0.2), and population density and population biomass (r = 0.35, t = 1.41, df = 14, p-value = 0.1) are not significantly correlated. Population biomass and species body size are correlated (r = 0.74, t = 4.15, df = 14, p-value < 0.001), but these two predictor variables were not simultaneously included in any model. Because metrics were calculated using the full network in Donatti et al. (2011), species roles were investigated in the context of the entire sampled seed dispersal interacting community.
We used generalized linear models (GLMs) with Negative Binomial error distribution for interaction richness and interaction records due to overdispersion, and Gamma error distribution for interaction diversity and z-score (continuous, non-negative, and positively skewed data), and Gaussian error distribution for closeness centrality and c-score. We built models with increasing complexity, from null models with only the intercept, to models testing the interaction between body size and density. Models were fitted using R version 4.1.1 (R Core Team, 2021), and selected based on the ΔAICc < 2.0 threshold.
Results
Within the studied seed dispersal network, frugivore species showed a wide range of population densities (0.1–36 individuals/km2), body sizes (0.07–240 kg), and population biomasses (0.1–337 kg/km2) (Figure 1). The set of 16 bird and mammal species included in this study dispersed 31 plant species (Figure 2) and, notably, large-bodied species such as the tapir (Tapirus terrestris) and the white-lipped peccary (Tayassu pecari) showed more frequent interactions than small-bodied species. Indeed, according to our models, body size and population biomass were better predictors of seed dispersal interaction patterns than population density (Supplementary Figure 1) in this non-defaunated frugivore community (Figure 3). However, relatively smaller-sized species, such as the toco toucan (Ramphastos toco) also interacted with a high number of plant species.
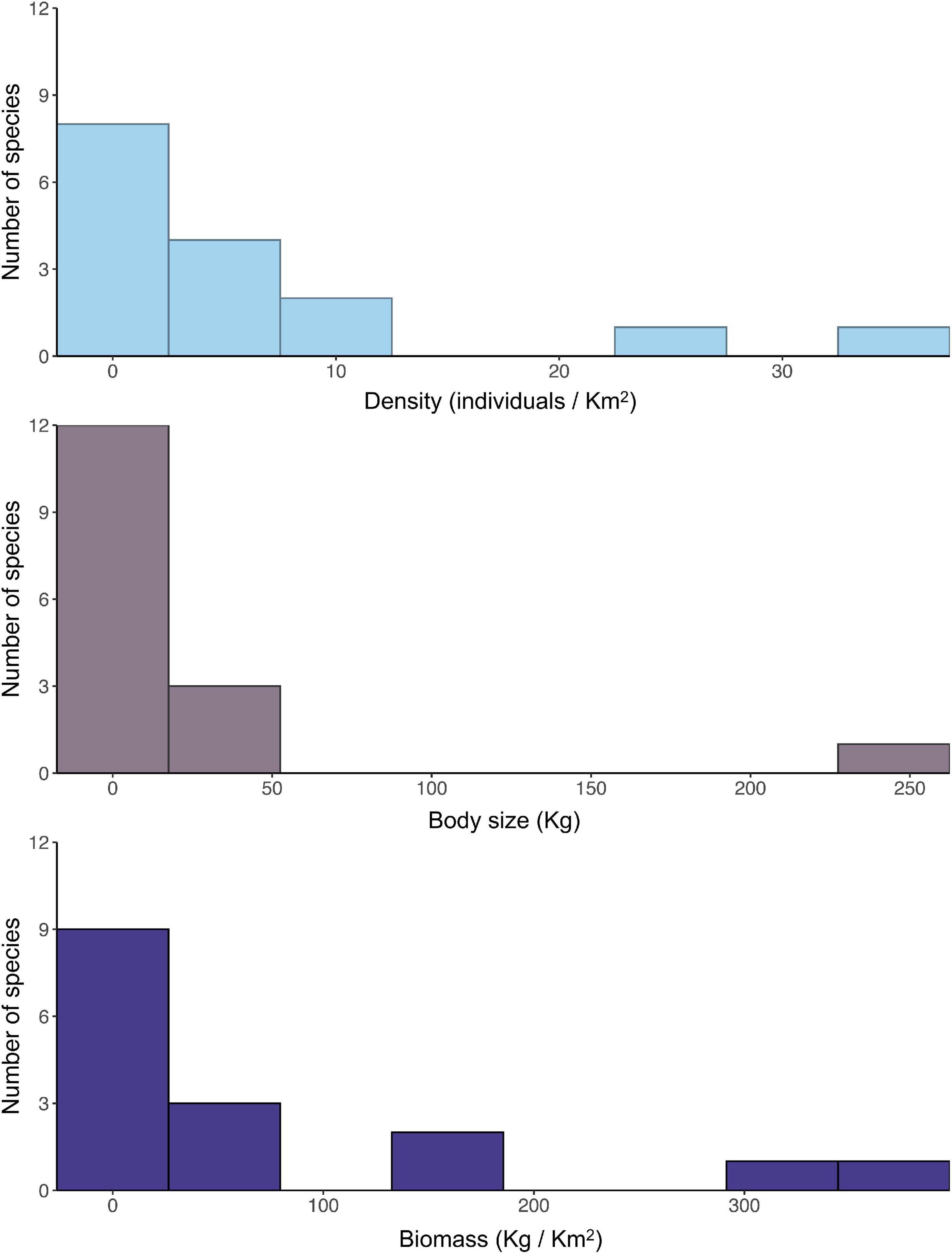
Figure 1. Frequency distribution of studied frugivore population density, species body size and population biomass of frugivores from the Brazilian Pantanal, including ten mammal and six bird species.
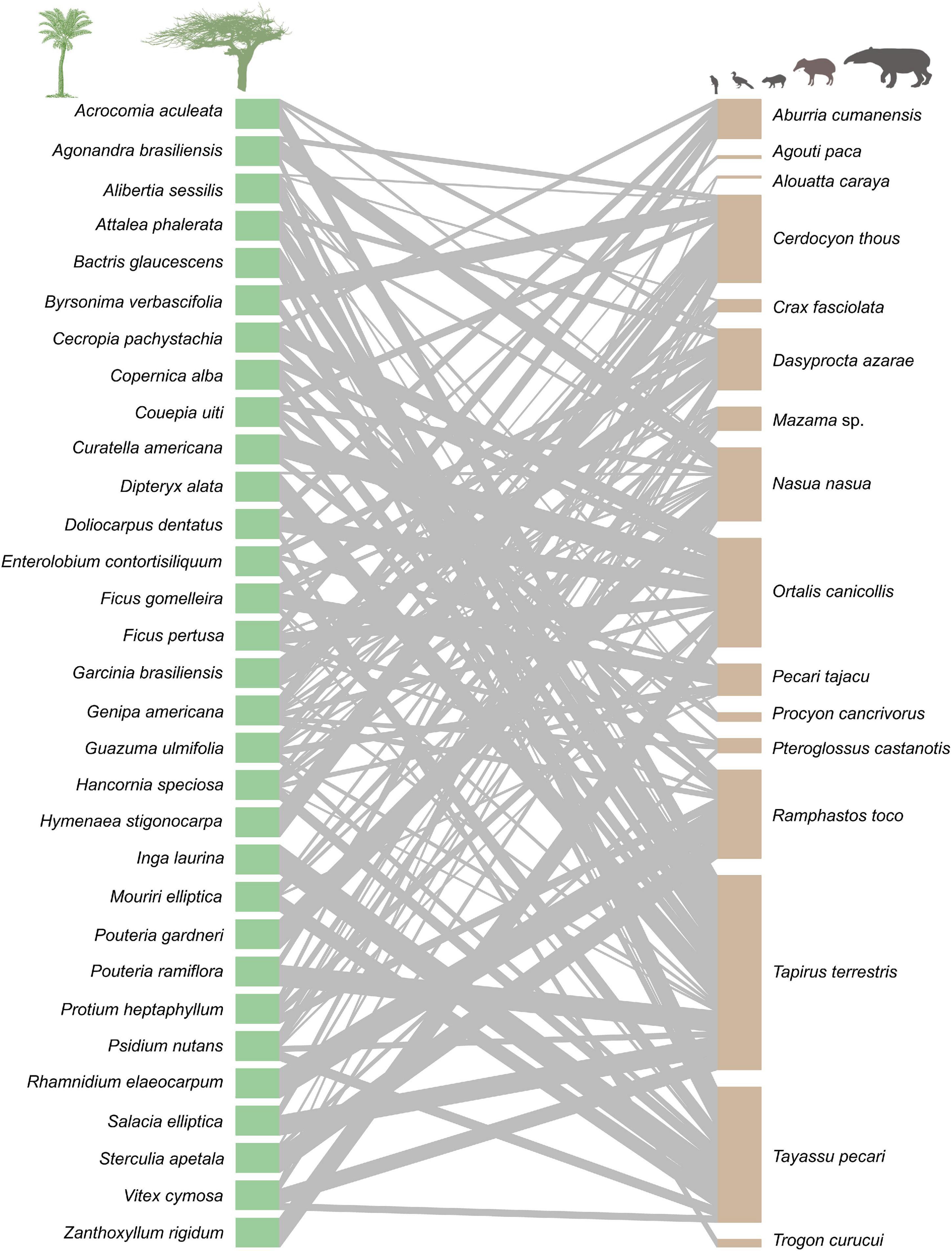
Figure 2. Seed dispersal network at two nearby sites in the Brazilian Pantanal. Each green rectangle represents one plant species, and each brown rectangle represents an animal species. Gray lines represent links between species, meaning that fruits from the plant species on the left were consumed by the linked animal species on the right, and the width of the lines indicates the strength of the interaction. This network includes bird and mammal species for which population density was estimated. Species are sorted in alphabetic order.
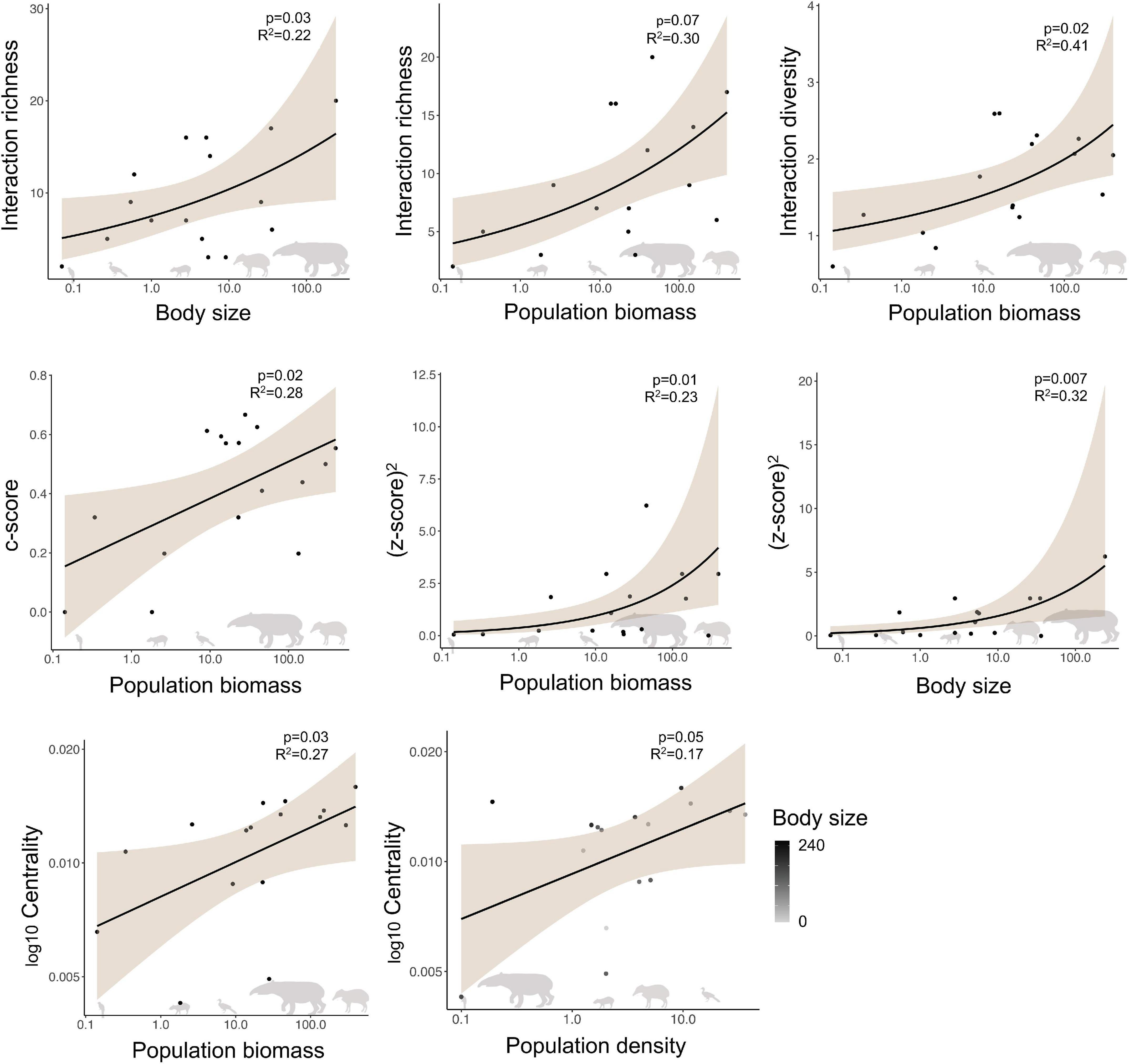
Figure 3. Relationship for significant predictors of the structure of a seed dispersal network in the Brazilian Pantanal, according to generalized linear models. Shaded areas depict 95% confidence intervals. Body size is measured in kg, population biomass is measured in kg/km2, and population density is measured by individuals/km2.
Body size (GLM, p = 0.03, z = 2.154, n = 16, R2 = 0.227; AICc = 101.58) and population biomass (p = 0.007, z = 2.674, n = 16, R2 = 0.303; AICc = 99.93) were equally good predictors of interaction richness (ΔAICc = 1.65; Table 1; Supplementary Table 2), although population biomass explained a slightly larger proportion of the variance in the response variable. Population biomass was the best predictor of interaction diversity (GLM, p = 0.02, t = 0.05, n = 16, R2 = 0.415) and the c-score (GLM, p = 0.02, t = 0.04, n = 16, R2 = 0.264), that represents the participation of each species in a network. A model with only population biomass (LM, p = 0.03, t = 2.29, n = 16, R2 = 0.221) and a model that included both body size and density (LM, p = 0.03, n = 16, R2 = 0.309) equally predicted closeness centrality, that is how connected each species is to other species in the network. Thus, as population biomass is a function of body size and density, these results support the notion that total biomass is a key factor structuring the role of frugivorous species in the network. Finally, population biomass (GLM, p = 0.01, t = 0.31, n = 16, R2 = 0.238) and body size (GLM, p = 0.01, t = 0.32, n = 16, R2 = 0.326; AICc = 40.56) were equally good predictors of the z-score.
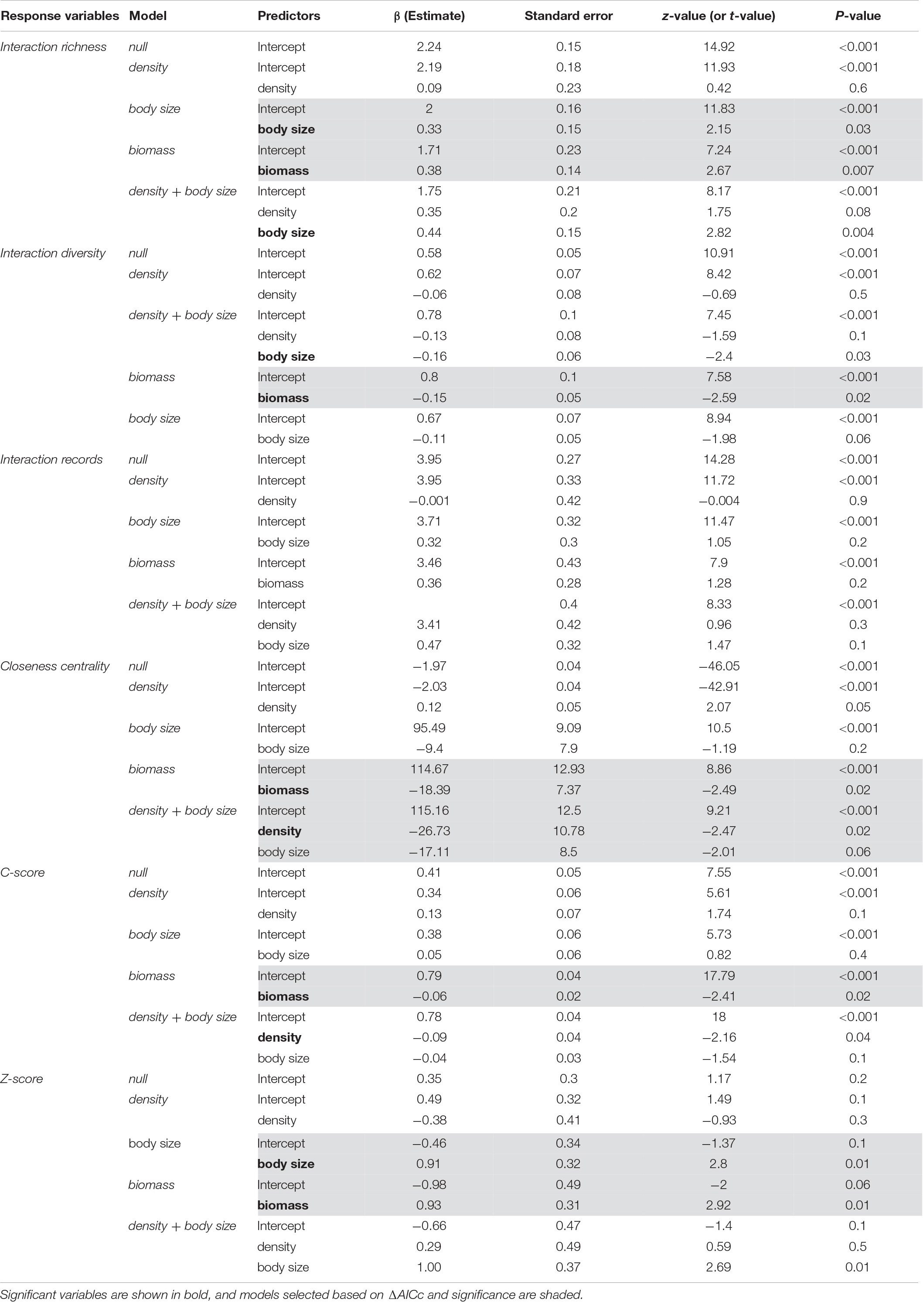
Table 1. Summary of generalized linear models for predicting the effect of population density, body size, and population biomass on seed dispersal network patterns.
Discussion
Recent studies highlight the role of frugivores in ecosystem functioning, but little is known about whether and how differences in animals body size and population density explain the structure of seed dispersal networks and compensate for the defaunation of large vertebrates. In a non-defaunated landscape from the Brazilian Pantanal we examined a frugivory community that comprises a wide range of population densities and body sizes across 16 native vertebrate species. We found that frugivore population density itself is not a good predictor of seed dispersal patterns and seed dispersal network structure. In contrast, both body size, and population biomass (which is a combination of density and body size) were found to significantly influence the role of frugivores in the seed dispersal network (Figure 2). In this same community, there is a strong association between species body size and population biomass, whereas the correlation between species density and population biomass is weak. More broadly, our results indicate that large frugivores are more important for the structure of this seed dispersal network, even if these species are not abundant. In general, these species consumed more fruit species (interaction richness), consumed more fruit species in a higher frequency (interaction diversity), were more central in the interaction network (closeness centrality) and were more generalist (c- and z-score). However, besides this general pattern, smaller-sized frugivores, such as the Chaco chachalaca (Ortalis canicollis) were found amongst the top-three frugivores for each of the metrics described above.
Other studies have pointed to the importance of functional traits, such as body size (e.g., Vidal et al., 2013) or neutral-based processes, such as species density (Krishna et al., 2008; Vázquez et al., 2009; Laurindo et al., 2020) in structuring species interactions. In the context of defaunation, where large-sized, and often rarer animals, tend to be extirpated in higher frequencies (Dirzo et al., 2014; Bogoni et al., 2020), and smaller-sized animals tend to become more abundant (Peres, 1990), addressing this size-biased defaunation may provide relevant insights into the potential compensatory effect of smaller animals in seed dispersal networks. In the studied non-defaunated seed dispersal network, there are many large-seeded plant species which could not be dispersed by smaller frugivores if larger frugivores were extirpated (Donatti et al., 2007). Elsewhere, studies have found that compensation may also be unlikely due to frugivore and seed size matching (Donoso et al., 2017) and when seed dispersal compensation occurs, it may not translate into plant recruitment being comparable to before defaunation levels (Culot et al., 2017). The fact that density was not as important as body size or biomass in structuring interactions implies a low potential for compensatory effects. This low possibility for compensating the loss-of-function arises as abundant animals per se were not found to be as important to the seed dispersal patterns as species with high population biomass and high body size. In other words, even if numerous, small frugivores will not be enough to replace the functions once accomplished by larger frugivores. This finding is of conservation significance as the lack of potential for compensatory effects in defaunated communities could cause lower dispersal and regeneration of several plant species. Examining the consistency of this finding across multiple seed dispersal networks (in conserved and differentially defaunated sites) is an aspect that warrants further research.
Out of the top three most important frugivores in each interaction pattern, only the Chaco chachalaca bird is a Least Concern species according to the IUCN Red List. All other species are listed as Near Threatened, Vulnerable, or Critically Endangered. For example, population declines of large mammals, such as IUCN Vulnerable, specifically species that show larger population biomass such as peccaries (Tayassu pecari) and lowland tapir (Tapirus terrestris), are unlikely to be compensated for in their seed dispersal roles by abundant, but smaller animals, such as blue-crowned trogons (Trogon curucui). On the other hand, it would be important to examine if other types of compensatory effects might occur where some of these large mammals experience population declines (e.g., Williams et al., 2021). Many of them are also seed predators and herbivores (e.g., Dracxler and Kissling, 2021; Mittelman et al., 2021) and some plant species may be released from these pressures, with subsequent cascading effects on the overall plant community (Martínez-Ramos et al., 2016). In addition to that, seed dispersal effectiveness is a complex process that depends on quantity and quality of dispersal and includes many interaction steps, such as the number of seeds dispersed, number of seeds dispersed per visit, gut passage effect on germination, and deposition site suitability, among others (Schupp et al., 2010). The interaction network depicted in this study only considers the quantity component of seed dispersal effectiveness. Future studies that examine compensatory effects in seed dispersal networks should consider the wide range of quality components of seed dispersal effectiveness to better assess the potential for functional replacements. However, our results support the notion that smaller seed dispersers cannot compensate the loss of larger-bodied vertebrate seed dispersers.
Given the low support for compensatory effects in this seed dispersal network, we posit that conservation actions should target areas where a wide range of frugivore functional traits is extant and promote such species’ reintroductions where they have been extirpated. Clearly, the relative role of species density and body size in structuring interaction networks continues to be a fundamental research agenda in ecology (Guimarães, 2020). The results we uncovered in this work suggest that similar studies, comparing defaunated and non-defaunated contexts considering a wide-range of disperser densities and body sizes, may provide further insights on the consequences of the omnipresent size-biased defaunation that characterizes the Anthropocene.
Data Availability Statement
The original contributions presented in the study are included in the article/Supplementary Material, further inquiries can be directed to the corresponding author.
Ethics Statement
Ethical review and approval was not required for the animal study because we used data previously collected and available.
Author Contributions
LG, PRG, and RD conceptualized this study. CD conducted the fieldwork and participated in former discussions. LG and GL conducted the analyses and wrote the first draft. All authors contributed to the writing of the article and approved the submitted version.
Funding
This work was supported by the Gabilan Stanford Graduate Fellowship (LG), Dirzo Lab’s unrestricted funds (RD), GL was supported by the Swiss National Science Foundation (PZ00P3_202127), CD fieldwork and research was supported by FAPESP (2004/00810-3 and 2008/10154-7), Earthwatch Institute and Conservation International. PRG received funding from CNPq (307134/2017-2), FAPESP (2018/14809-0), and the Royal Society, London (CHL/R1/180156).
Conflict of Interest
The authors declare that the research was conducted in the absence of any commercial or financial relationships that could be construed as a potential conflict of interest.
Publisher’s Note
All claims expressed in this article are solely those of the authors and do not necessarily represent those of their affiliated organizations, or those of the publisher, the editors and the reviewers. Any product that may be evaluated in this article, or claim that may be made by its manufacturer, is not guaranteed or endorsed by the publisher.
Acknowledgments
Members of the Dirzo Lab, and attendees at the 2020 Species Interactions Workshop (Stanford University/University of California, Santa Cruz) provided discussion and feedback that helped develop this manuscript.
Supplementary Material
The Supplementary Material for this article can be found online at: https://www.frontiersin.org/articles/10.3389/fevo.2022.794723/full#supplementary-material
References
Bogoni, J. A., Peres, C. A., and Ferraz, K. M. P. (2020). Effects of mammal defaunation on natural ecosystem services and human well being throughout the entire Neotropical realm. Ecosyst. Serv. 45:101173. doi: 10.1016/j.ecoser.2020.101173
Burns, K. C. (2013). What causes size coupling in fruit-frugivore interaction webs? Ecology 94, 295–300. doi: 10.1890/12-1161.1
Culot, L., Bello, C., Batista, J. L. F., Do Couto, H. T. Z., and Galetti, M. (2017). Synergistic effects of seed disperser and predator loss on recruitment success and long-term consequences for carbon stocks in tropical rainforests. Sci. Rep. 7:82224. doi: 10.1038/s41598-017-08222-4
Dirzo, R., and Miranda, A. (1990). Contemporary neotropical defaunation and forest structure, function, and diversity-a sequel to john terborgh. Conserv. Biol. 4, 444–447. doi: 10.1111/j.1523-1739.1990.tb00320.x
Dirzo, R., Young, H. S., Galetti, M., Ceballos, G., Isaac, N. J. B., and Collen, B. (2014). Defaunation in the anthropocene. Science 345, 401–406. doi: 10.1126/science.1251817
Donatti, C. I. (2011). Ecological studies on seed dispersal networks: insights from a diverse tropical ecosystem. Stanford: Stanford University.
Donatti, C. I., Guimarães, P. R., Galetti, M., Pizo, M. A., Marquitti, F. M. D., and Dirzo, R. (2011). Analysis of a hyper-diverse seed dispersal network: modularity and underlying mechanisms. Ecol. Lett. 14, 773–781. doi: 10.1111/j.1461-0248.2011.01639.x
Donatti, C., Galetti, M., Pizo, M. A., Guimarães, P. R., and Jordano, P. (2007). Living in the land of ghosts: fruit traits and the importance of large mammals as seed dispersers in the Pantanal, Brazil. in: Seed Dispersal: Theory and Its Application in a Changing World. Wallingford: CABI, 104–123. doi: 10.1079/9781845931650.0104
Donoso, I., Schleuning, M., García, D., and Fründ, J. (2017). Defaunation effects on plant recruitment depend on size matching and size trade-offs in seed-dispersal networks. Proc. R. Soc. B Biol. Sci. 284:20162664. doi: 10.1098/rspb.2016.2664
Donoso, I., Sorensen, M. C., Blendinger, P. G., Kissling, W. D., Neuschulz, E. L., Mueller, T., et al. (2020). Downsizing of animal communities triggers stronger functional than structural decay in seed-dispersal networks. Nat. Commun. 11, 1–8. doi: 10.1038/s41467-020-15438-y
Dracxler, C., and Kissling, W. D. (2021). The mutualism–antagonism continuum in Neotropical palm–frugivore interactions: from interaction outcomes to ecosystem dynamics. Biol. Rev 31:809. doi: 10.1111/brv.12809
Fleming, T. H. (1991). The relationship between body size, diet, and habitat use in frugivorous bats, genus Carollia (Phyllostomidae). J. Mammal. 72, 493–501. doi: 10.2307/1382132
Fonseca, G. A. B., Herrmann, G., Leite, Y. L. R., Mittermeier, R. A., Rylands, A. B., and Patton, J. L. (1996). Lista Anotada dos Mamíferos do Brasil. Occasional Papers Conservation International, 4.
Galetti, M., Guevara, R., Cortes, M. C., Fadini, R., Von Matter, S., Leite, A. B., et al. (2013). Functional extinction of birds drives rapid evolutionary changes in seed size. Science 340, 1086–1090. doi: 10.1126/science.1233774
Guimarães, P. R. (2020). The structure of ecological networks across levels of organization. Annu. Rev. Ecol. Evol. Syst. 51, 433–460. doi: 10.1146/annurev-ecolsys-012220-120819
Jansen, P. A., Hirsch, B. T., Emsens, W.-J., Zamora-Gutierrez, V., Wikelski, M., and Kays, R. (2012). Thieving rodents as substitute dispersers of megafaunal seeds. Proc. Natl. Acad. Sci. USA 109, 12610–12615. doi: 10.1073/pnas.1205184109
Jordano, P. (2000). “Fruits and frugivory,” in Seeds: The Ecology of Regeneration in Plant Communities, ed. M. Fenner (Wallingfords: CABI Publ), 125–166. doi: 10.1079/9780851994321.0125
Jordano, P., Garcia, C., Godoy, J. A., and Garcia-Castano, J. L. (2007). Differential contribution of frugivores to complex seed dispersal patterns. Proc. Natl. Acad. Sci. 104, 3278–3282. doi: 10.1073/pnas.0606793104
Krishna, A., Guimarães, P. R., Jordano, P., and Bascompte, J. (2008). A neutral-niche theory of nestedness in mutualistic networks. Oikos 117, 1609–1618. doi: 10.1111/j.1600-0706.2008.16540.x
Laurindo, R., de, S., Vizentin-Bugoni, J., Tavares, D. C., Mancini, M. C. S., Mello, R., et al. (2020). Drivers of bat roles in Neotropical seed dispersal networks: abundance is more important than functional traits. Oecologia 193, 189–198. doi: 10.1007/s00442-020-04662-4
Martin, T. E. (1985). Selection of second-growth woodlands by frugivorous migrating birds in Panama: an effect of fruit size and plant density? J. Trop. Ecol. 1, 157–170. doi: 10.1017/S0266467400000213
Martínez-Ramos, M., Ortiz-Rodríguez, I. A., Piñero, D., Dirzo, R., and Sarukhán, J. (2016). Anthropogenic disturbances jeopardize biodiversity conservation within tropical rainforest reserves. Proc. Natl. Acad. Sci. 113, 5323–5328. doi: 10.1073/pnas.1602893113
Mata, J. R., Rodriguez, F. E., and Maurice, R. (2006). A Field Guide to the Birds of South America: Non-Passerines: From Rheas to Woodpeckers. Harper Collins.
McConkey, K. R., and Drake, D. R. (2015). Low redundancy in seed dispersal within an island frugivore community. AoB Plants 7:lv088. doi: 10.1093/aobpla/plv088
Mittelman, P., Dracxler, C. M., Santos Coutinho, P. R. O., and Pires, A. S. (2021). Sowing forests: a synthesis of seed dispersal and predation by agoutis and their influence on plant communities. Biol. Rev. 96, 2425–2445. doi: 10.1111/brv.12761
Palacio, R. D., Valderrama-Ardila, C., and Kattan, G. H. (2016). Generalist species have a central role in a highly diverse plant-frugivore network. Biotropica 48, 349–355. doi: 10.1111/btp.12290
Peres, C. A. (1990). Effects of hunting on western Amazonian primate communities. Biol. Conserv. 54, 47–59. doi: 10.1016/0006-3207(90)90041-M
Peres, C. A. (2000). Effects of subsistence hunting on vertebrate community structure in amazonian forests. Conserv. Biol. 14, 240–253. doi: 10.1046/j.1523-1739.2000.98485.x
Peres, C. A., Emilio, T., Schietti, J., Desmoulière, S. J. M., and Levi, T. (2016). Dispersal limitation induces long-term biomass collapse in overhunted Amazonian forests. Proc. Natl. Acad. Sci. 113, 892–897. doi: 10.1073/pnas.1516525113
Prance, G. T., and Schaller, G. B. (1982). Preliminary study of some vegetation types of the pantanal, mato grosso, brazil. Brittonia 34, 228–251. doi: 10.2307/2806383
Quintero, E., Isla, J., and Jordano, P. (2022). Methodological overview and data-merging approaches in the study of plant–frugivore interactions. Oikos 2022, 1–18. doi: 10.1111/oik.08379
R Core Team (2021). R: A language and environment for statistical computing. Vienna: R Foundation for Statistical Computing.
Rezende, E. L., Lavabre, J. E., Guimarães, P. R., Jordano, P., and Bascompte, J. (2007). Non-random coextinctions in phylogenetically structured mutualistic networks. Nature 448, 925–928. doi: 10.1038/nature05956
Säterberg, T., Sellman, S., and Ebenman, B. (2013). High frequency of functional extinctions in ecological networks. Nature 499, 468–470. doi: 10.1038/nature12277
Schleuning, M., Fründ, J., and García, D. (2015). Predicting ecosystem functions from biodiversity and mutualistic networks: An extension of trait-based concepts to plant-animal interactions. Ecography 38, 380–392. doi: 10.1111/ecog.00983
Schupp, E. W., Jordano, P., and Gomez, J. M. (2010). Seed dispersal effectiveness revisited: a conceptual review. New Phytol. 188, 333–353. doi: 10.1111/j.1469-8137.2010.03402.x
Souza, Y., Villar, N., Zipparro, V., Nazareth, S., and Galetti, M. (2022). Large mammalian herbivores modulate plant growth form diversity in a tropical rainforest. J. Ecol. 2022, 1–15. doi: 10.1111/1365-2745.13846
Sutherland, W. J. (1996). Ecological Census Techniques: a Handbook. Cambridge: Cambridge University Press.
Timóteo, S., Correia, M., Rodríguez-Echeverría, S., Freitas, H., and Heleno, R. (2018). Multilayer networks reveal the spatial structure of seed-dispersal interactions across the Great Rift landscapes. Nat. Commun. 9, 1–11. doi: 10.1038/s41467-017-02658-y
Valiente-Banuet, A., Aizen, M. A., Alcántara, J. M., Arroyo, J., Cocucci, A., Galetti, M., et al. (2015). Beyond species loss: the extinction of ecological interactions in a changing world. Funct. Ecol. 29, 299–307. doi: 10.1111/1365-2435.12356
Van Perlo, B. (2015). Collins Field Guide – Birds of South America: Passerines, from Sapayoas to Finches. Harper Collins, 2015.
Vázquez, D. P., Chacoff, N. P., and Cagnolo, L. (2009). Evaluating multiple determinants of the structure of plant-animal mutualistic networks. Ecology 90, 2039–2046. doi: 10.1890/08-1837.1
Vázquez, D. P., Melián, C. J., Williams, N. M., Blüthgen, N., Krasnov, B. R., and Poulin, R. (2007). Species abundance and asymmetric interaction strength in ecological networks. Oikos 116, 1120–1127. doi: 10.1111/j.2007.0030-1299.15828.x
Vidal, M. M., Hasui, E., Pizo, M. A., Tamashiro, J. Y., Silva, W. R., and Guimarães, P. R. (2014). Frugivores at higher risk of extinction are the key elements of a mutualistic network. Ecology 95, 3440–3447. doi: 10.1890/13-1584.1
Vidal, M. M., Pires, M. M., and Guimarães, P. R. (2013). Large vertebrates as the missing components of seed-dispersal networks. Biol. Conserv. 163, 42–48. doi: 10.1016/j.biocon.2013.03.025
Villar, N., and Medici, E. P. (2021). Large wild herbivores slow down the rapid decline of plant diversity in a tropical forest biodiversity hotspot. J. Appl. Ecol. 58, 2361–2370. doi: 10.1111/1365-2664.14054
Villar, N., Siqueira, T., Zipparro, V., Farah, F., Schmaedecke, G., Hortenci, L., et al. (2020). The cryptic regulation of diversity by functionally complementary large tropical forest herbivores. J. Ecol. 108, 279–290. doi: 10.1111/1365-2745.13257
Williams, P. J., Ong, R. C., Brodie, J. F., and Luskin, M. S. (2021). Fungi and insects compensate for lost vertebrate seed predation in an experimentally defaunated tropical forest. Nat. Commun. 12:1650. doi: 10.1038/s41467-021-21978-8
Keywords: frugivory, Pantanal, plant-animal interaction, seed dispersal, mutualism
Citation: Genes L, Losapio G, Donatti CI, Guimarães PR Jr and Dirzo R (2022) Frugivore Population Biomass, but Not Density, Affect Seed Dispersal Interactions in a Hyper-Diverse Frugivory Network. Front. Ecol. Evol. 10:794723. doi: 10.3389/fevo.2022.794723
Received: 14 October 2021; Accepted: 21 March 2022;
Published: 04 May 2022.
Edited by:
Isabel Donoso, Spanish National Research Council (CSIC), SpainReviewed by:
Jörg Albrecht, Senckenberg Nature Research Society, GermanyGiuseppe Donati, Oxford Brookes University, United Kingdom
Copyright © 2022 Genes, Losapio, Donatti, Guimarães and Dirzo. This is an open-access article distributed under the terms of the Creative Commons Attribution License (CC BY). The use, distribution or reproduction in other forums is permitted, provided the original author(s) and the copyright owner(s) are credited and that the original publication in this journal is cited, in accordance with accepted academic practice. No use, distribution or reproduction is permitted which does not comply with these terms.
*Correspondence: Luísa Genes, lgenes@stanford.edu