- 1Global Change Research Group, Mediterranean Institute for Advanced Studies Mediterranean Institute for Advanced Studies (IMEDEA) (CSIC-UIB), Esporles, Spain
- 2Senckenberg Biodiversity and Climate Research Centre, Frankfurt am Main, Germany
- 3Department of BioSciences, Rice University, Houston, TX, United States
- 4Department of Ecology, Evolution, and Organismal Biology, Iowa State University, Ames, IA, United States
As the sole opportunity for most plants to move, seed dispersal influences the biodiversity and functioning of plant communities. Global change drivers have the potential to disrupt seed dispersal processes, affecting plant communities and ecosystem functions. Even though much information is available on the effects of seed dispersal disruption (SDD), we still lack a comprehensive understanding of its main causes at a global scale, as well as the potential knowledge gaps derived from research biases. Here we present a systematic review of biotic and abiotic SDDs to ascertain the global change drivers addressed, dispersal modes impacted, plant processes affected, and spatial focus of existing research on this topic up-to-date. Although there are many modes of dispersal and global change drivers in temperate and tropical ecosystems worldwide, research efforts have predominantly addressed the effect of alien species for biotic seed dispersal in temperate systems and oceanic islands as well as how defaunation of bird or mammal dispersers has affected seed removal in the Neotropics. SDD studies were also biased toward forest ecosystems, with few in shrublands or grasslands. Finally, the effects of climate change, ecological consequences at the whole community level, and evolutionary changes were largely unrepresented in SDD studies. These trends are likely due to a combination of true geographic and ecological patterns in seed dispersal and global change and bias in research focus. We conclude that increased research investment in the less-studied systems and a better understanding of potential synergies and feedback between multiple global change drivers will be important to forecast the threats to plant biodiversity and those ecosystem functions derived from seed dispersal in the Anthropocene.
Introduction
The movement of seeds away from the mother plant allows them to colonize specific microsites and new areas, reduces sibling competition and attack by natural enemies (e.g., herbivores, pathogens), and determines the potential area of recruitment, acting as a template for the subsequent stages of plant growth as well as the plant spatial patterns (Howe and Smallwood, 1982; Howe and Miriti, 2004; Jordano, 2014; Traveset et al., 2014; Rogers et al., 2021a). The dispersal vectors can be both biotic (i.e., transported in the digestive tracts, fur, plumage or feet of animals) or abiotic (i.e., transported by wind, water, or gravity). Besides moving seeds across the landscape, animals that ingest fruits and pass viable seeds through their digestive tracts can further play an important role in plant establishment as they can modify the rate of seed germination and seedling growth (Traveset and Verdú, 2002; Rogers et al., 2021b). Biotic and abiotic movement of seeds supports plant communities, the biodiversity they contain, and the ecosystem services they provide, including fruit, wood and non-timber products, in addition to enhanced carbon sequestration, at no cost to humans (Leverkus and Castro, 2017; Egerer et al., 2018; Chagas et al., 2021).
Animals play an important role as seed dispersers for more than half of the plant species around the globe (Levey et al., 2002; Dennis et al., 2007; Rogers et al., 2021a), thus contributing to numerous ecosystem functions. Birds, mammals, lizards, fish and invertebrates are common dispersers, although their relative importance varies across habitat types (Fleming and John Kress, 2011; Albert et al., 2015; Correa et al., 2015; Gómez et al., 2019; Anjos et al., 2020; Araujo et al., 2021; Rogers et al., 2021a). Within studies involving avian frugivores, most research has focused on those species dispersing fleshy-fruited plants, but many birds can serve as dispersers including waterbirds which move many wetland species (Green et al., 2016, 2021). Among mammals, key dispersers include frugivores like primates and bats which disperse seeds primarily through endozoochory (Fuzessy et al., 2018), herbivores like deer and sheep which disperse non-fleshy fruits both through ectozoochory and endozoochory (Albert et al., 2015), and rodents which typically disperse seeds through scatter-hoarding (Gómez et al., 2019).
Multiple drivers of global change have been documented to cause pervasive disruptions in the seed dispersal process (SDD, hereafter), with poorly understood consequences for the functioning of communities and ecosystems. In the case of animal seed dispersal, plants are susceptible to dispersal failure when the animal populations on which they depend decline or even become extinct (Traveset and Richardson, 2006; Traveset et al., 2012; Pérez-Méndez et al., 2016; Rogers et al., 2017; Tucker et al., 2021). Habitat loss, land use change, overhunting, and invasive species are key threats underlying animal seed disperser population declines, extinctions, and range reductions (McConkey et al., 2012; Dirzo et al., 2014). The disruption of plant-seed disperser interactions have shown to have serious consequences for plants, which can cascade to affect other species, as well as the whole community and ecosystem (reviewed in Rogers et al., 2021a). Widespread rapid changes in seed disperser assemblages—which likely translate into changes in the seed dispersal process—have occurred since prehistoric times, when humans started colonizing new regions, strongly modifying landscapes and defaunating ecosystems by hunting mainly large-body animals, leading to many megafaunal extinctions in the Pleistocene (Janzen and Martin, 1982; Malhi et al., 2016; Galetti et al., 2018). Megafauna frugivores and herbivores are still the most susceptible seed dispersers to defaunation in tropical forests (Young et al., 2016) and globally (Ripple et al., 2015; Atwood et al., 2020). Islands mostly lack megafauna, but the pattern of greater threat to larger-bodied animals holds true on islands as well, where large-bodied birds, bats, and lizards are those suffering the largest declines (Traveset et al., 2012; Pérez-Méndez et al., 2016; Kingston et al., 2021). It is important to recognize that small-bodied bird and mammal dispersers tend to be less threatened although they also have experienced declines (Davidson et al., 2009; Rosenberg et al., 2019), which could cause imperceptible but pervasive impacts on seed dispersal (Rogers et al., 2021a).
Seeds dispersed by abiotic vectors may also be disrupted by human activities (Zhang et al., 2011; Teller et al., 2015). For instance, the construction of dams influences the connectivity of riparian plant communities along rivers by affecting seed dispersal distances of hydrochorous species and strongly altering community composition (Jansson et al., 2000; Merritt and Wohl, 2006). In general, landscape structure can have strong effects on the distance that seeds travel (San-José et al., 2019) and, thus, any kind of disturbance (e.g., habitat loss, fragmentation, defaunation, or modification by a non-native species) is likely to change the patterns of seed movement and recruitment, as well as the genetic structure of plant populations. Wind-dispersed seeds, for instance, can travel much further in open landscapes than in dense forests, owing to differences in the shape of the wind profile (San-José et al., 2019). Seed dispersal disruptions (SDDs), in fact, might either increase or decrease dispersal distance depending both on the dispersal mode of the species, the global change driver, and the specific context in which the disruption occurs.
Despite the large amount of information dealing with the effects of SDD, biases in scientific research can limit our understanding of the actual main patterns of SDD at a global scale. Moreover, most studies focus on just one global change driver, when it is widely accepted that such drivers act synergistically on many processes (Tylianakis et al., 2008; González-Varo et al., 2013; Maciel et al., 2021). A previous study by McConkey et al. (2012) pointed out that such synergistic interactions between different types of disturbances could exacerbate the negative impacts on seed dispersal, although they were rarely examined (but see Portela and Dirzo, 2020). Since then, there has been a number of studies that have simultaneously evaluated the effects of more than one driver on SDD, but they remain scarce (e.g., Pejchar, 2015; Rotllan-Puig and Traveset, 2016; Brocardo et al., 2018; de Assis Bomfim et al., 2018; Qie et al., 2019; Kiel et al., 2020; Bello et al., 2021; Marone and Pol, 2021; Sales et al., 2021). Assessing regional trends in research could thus help in understanding the main patterns in the drivers and synergisms between processes that could operate at different scales.
In this study, we review and synthesize the most up-to-date evidence of different drivers of global change causing SDD. Using a comprehensive dataset of 184 studies encompassing all biogeographical regions, our goal is to: (1) identify the realms and ecosystems in which SDD are more frequently documented. Given that a higher proportion of animal-dispersed species is found in tropical areas than at higher latitudes, and given also the high rates of habitat loss in the tropics, we might expect more studies on SDD in the tropics; (2) assess which are the most commonly studied drivers behind SDD, and (3) in the case of animal-seed dispersed species, evaluate what disperser taxa are the most often studied. In addition, we aim to (4) identify general mechanisms by which the different drivers of global change interfere with the seed dispersal process. For this, we evaluate which are the response variables most often measured in SDD studies. Here we move beyond previous reviews by (i) considering both abiotic and biotic SDD, (ii) quantifying research trends accounting not only for ecological consequences but also for evolutionary disruptions and (iii) capturing the latest studies addressing potential synergistic effects among drivers. We conclude by pointing out research gaps and frontiers in the study of global change that can help mitigate SDD and thus avoid their cascading impacts.
Materials and Methods
We performed a thorough literature search using Web of Science (WOS)1 and included all records from 1980 (oldest record found) until May 2021. We used the following search string “seed dispers*” AND “disrupt*” OR “seed dispers*” AND “failure*” resulting in a total of 884 records published in English-language journals. We first read titles and abstracts to exclude papers which were clearly not relevant, and then inspected 489 full texts searching for evidence of SDD. Out of these scientific publications, we developed a final database which retained a total of 184 original papers reporting at least one driver causing SDD from unique case studies (i.e., we exclude review papers). PRISMA guidelines were followed for this systematic revision (Page et al., 2021, see Supplementary Figure 1). Although most of the scientific publications were based on empirical data, we also considered theoretical studies aiming at modeling the effects of human impacts on seed dispersal (see e.g., Jones et al., 2017). We realize that the resulting database is not fully comprehensive since there are some articles not captured by our search terms (e.g., Donoso et al., 2020). However, we believe this compilation represents a largely unbiased sampling of the literature which allows us to identify patterns in seed dispersal research. As noted above, our search was mostly limited to English language publications, except for two studies published in Portuguese and one in Spanish that appeared in our search because their abstract was published in English. Therefore, research from regions where publications are commonly in other languages are likely under-represented in our database. Because we aim to provide a broad understanding of SDD, we focused on both anthropogenic and non-anthropogenic drivers, which were classified according to the following eight categories: (i) non-native species; (ii) climate change; (iii) defaunation; (iv) habitat loss/degradation; (v) fragmentation; (vi) other anthropogenic disturbances; (vii) non-anthropogenic abiotic factors; and (viii) non-anthropogenic biotic factors. The criteria used to classify each driver within each category is shown in Table 1.
In addition, we extracted the following information. First, the bioregion was assigned according to the 11 broad realms identified by Holt et al. (2013), with the exception of oceanic islands that were treated as a separate region (following Fricke and Svenning, 2020). Second, the ecosystem in which the disruption was reported was categorized according to the IUCN Habitats Classification Scheme (Version 3.1) into: forest, savanna, shrubland, grassland, wetland, desert and artificial habitat (i.e., agricultural, gardens, pastures and urban areas). We established two additional categories to consider a few cases in which we were unable to assign one of the above-mentioned-categories. These categories corresponded to agroforests (e.g., agricultural crops such as coffee or cacao under canopy of shade trees) and others, in which we included gypsum soils and dunes. Third, in those studies focused on biotic seed dispersal, we identified the seed dispersers under study, which were assigned according to the following eight functional groups: bats, birds, fishes, invertebrates (mainly ants, beetles and gastropods), primates, reptiles, rodents, and other mammals (e.g., carnivores, herbivores, marsupials). Mammals were classified into four functional groups given their heterogeneity in terms of functional traits and handling behavior (e.g., flying vs. non-fly ability; diurnal vs. nocturnal habits, frugivory vs. scatter-hoarding) compared to other taxa. Lastly, to explore the general mechanisms by which the different drivers interfered with the seed dispersal process, we identified the response variables that were assessed in each publication. To homogenize the broad terminology used among studies, we established 10 categories trying to represent the main stages of the seed dispersal cycle (Wang and Smith, 2002; Carlo and Yang, 2011; see classification in Table 2). This information was further interpreted based on the seed dispersal effectiveness framework proposed by Schupp (1993), and re-classified into four main categories to identify whether SDD interfered with the seed dispersal process by causing: (i) community changes, (ii) disruptions in the quantity component of seed dispersal, (iii) disruptions in the quality component of seed dispersal, or/and (iv) by triggering evolutionary changes (see Table 2).
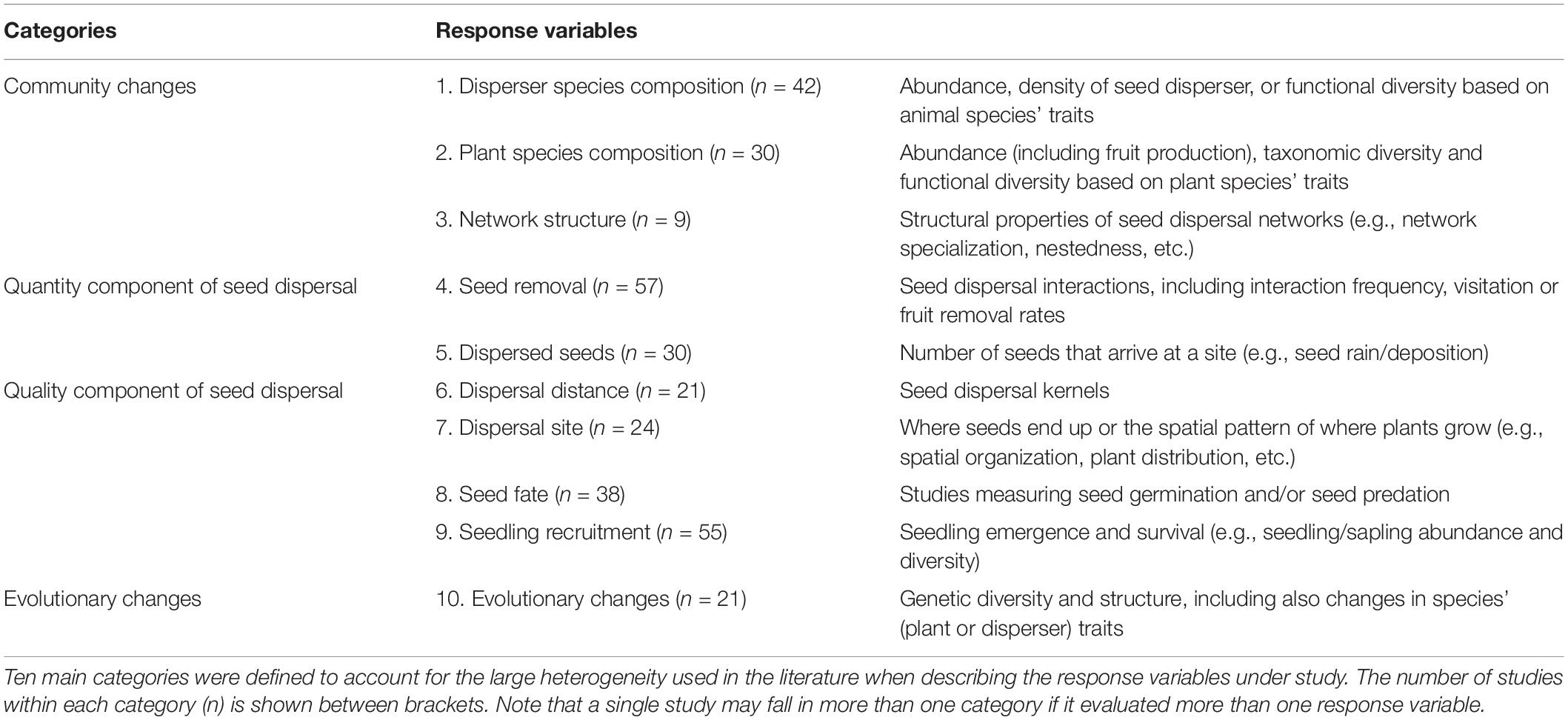
Table 2. Categorization of the main mechanisms by which the different drivers of global change interfere with the seed dispersal process.
We completed our dataset by including the following information: (i) in the case of abiotic dispersal, the main seed dispersal vector (e.g., gravity, wind, water), (ii) whether SDD occurred on an island, and if so, the type of island (i.e., continental vs. oceanic); (iii) if the study considered SDD at a species- or at community- level; and (iv) whether the main results were based on empirical data, simulations, or the combination of these two approaches.
To identify general trends in the study of SDD, we estimated the percentage of scientific publications (out of the 184 studies) reporting each category per variable in our dataset. However, for some of the studies we identified more than one case per variable; i.e., the authors considered more than one bioregion, ecosystem, driver, disperser or response variable within the same study. Thus, percentages can add up to >100% because each paper could contain more than one category per variable.
Results
Overall, we found studies addressing SDD in all biogeographical regions (Figure 1A) but they were unevenly distributed worldwide. Disruptions have been more frequently documented in the Neotropics, with almost a quarter of the publications reporting SDD in this realm (Figure 1B). Studies in the Paleartic are also highly represented (21%), followed by almost 15% of the studies carried out in the Nearctic. For the rest of bioregions, including the oceanic islands, disruptions in the seed dispersal process are reported in less than 10% of the publications.
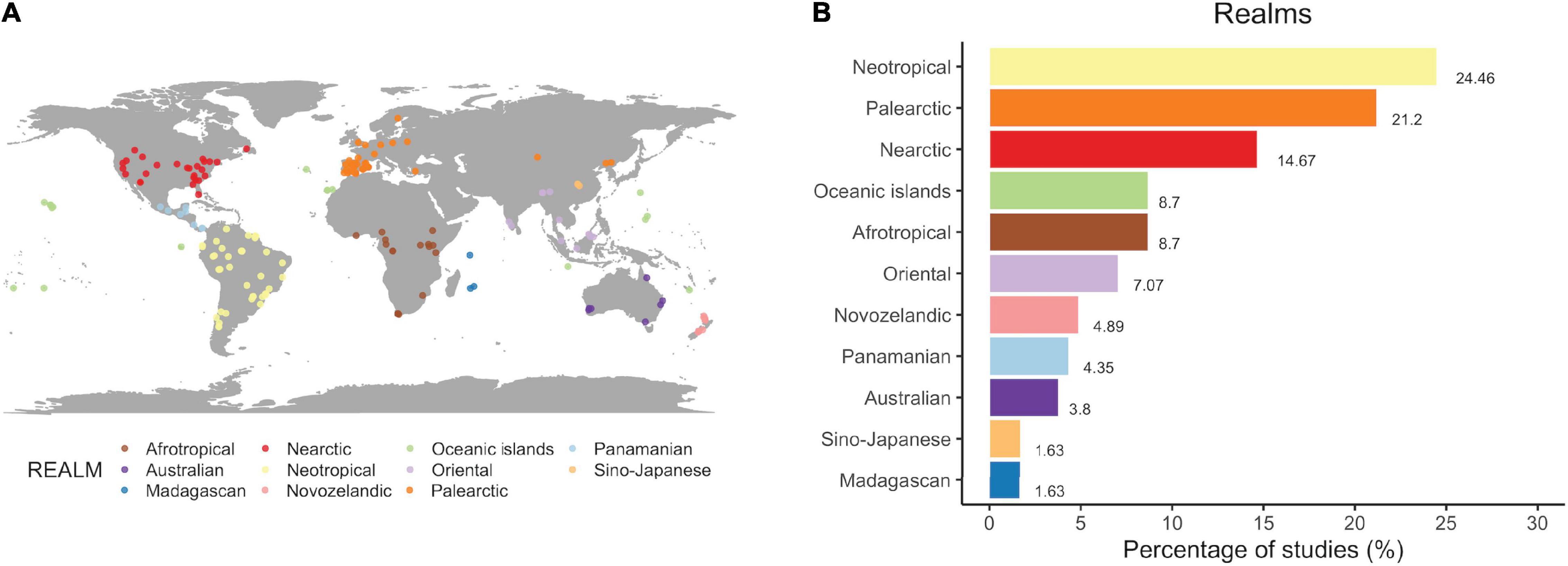
Figure 1. The global extent of seed dispersal disruptions. (A) Map of the study locations, with each point representing an individual case (N = 186 realms identified in 184 articles). (B) Bar chart representing the percentage of studies addressing seed dispersal disruptions in each of the biogeographical regions.
Regarding the main drivers of SDD, the introduction of non-native species is the anthropogenic factor most frequently studied, with almost 30% of the publications, followed by defaunation (25%), habitat loss (∼22%) and fragmentation (∼21%) (Figure 2). On the contrary, the effect of non-anthropogenic abiotic and biotic factors, as well as the impact of climate change on SDD have been rarely documented, with < 8% of the publications in all three cases. Only about one fourth (26%) of the publications reported more than one driver of SDD.
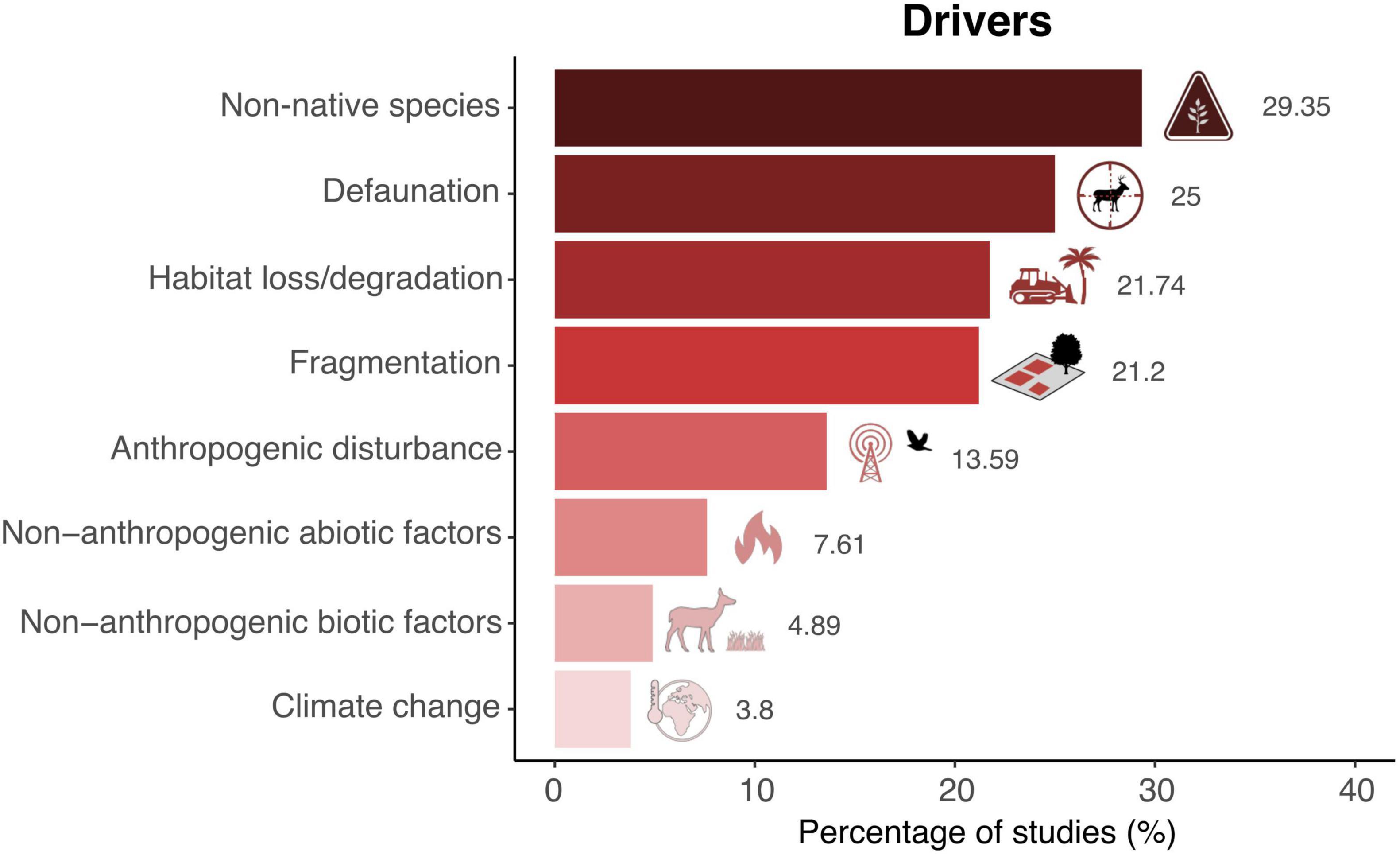
Figure 2. Main causes of seed dispersal disruptions. Shown are the percentages of studies reporting each anthropogenic or non-anthropogenic impact as a driver of SDD. Note that the total sum of percentages exceeds 100% because some studies reported more than one driver (N = 237 records identified in the 184 original articles).
Most SDD studies (75%) have been documented in forest ecosystems. By contrast, only 16 and 4% of the studies have been performed in shrublands and grasslands, respectively (Figure 3A). Among the functional groups assessed, disruptions involving birds were addressed in 45.6% of the studies, followed by mammals such as herbivores and carnivores (19%), rodents (16%), and primates (14%) (Figure 3B). While we detected that seed dispersal failure in interactions involving invertebrates occurred in almost 12% of the studies, only 4 and 1% of the publications considered bats and fishes, respectively.
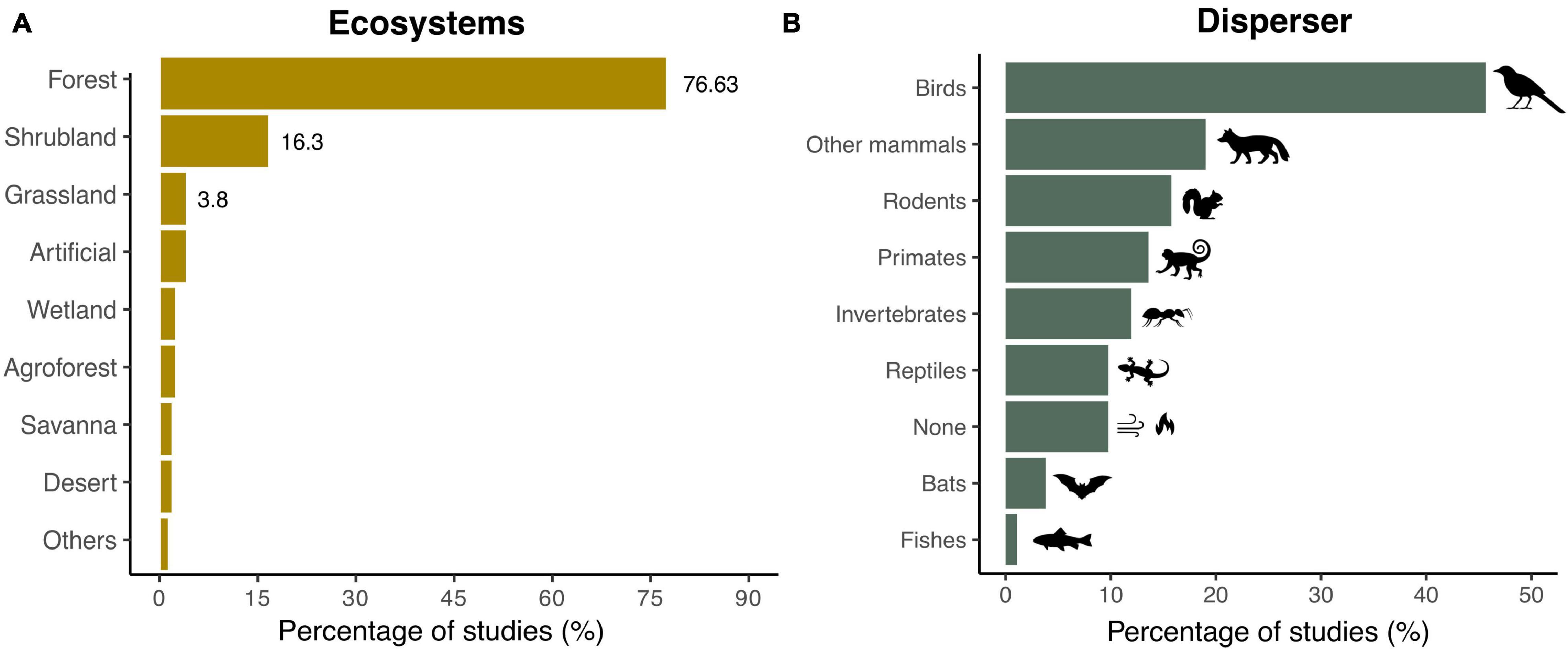
Figure 3. Ecosystems and dispersers documented in SDD studies. Shown are the percentage of studies reporting SDD (A) in each type of ecosystem (N = 196 records) and (B) for each seed disperser functional group (N = 237 records). Note that the scale of the x-axes differs between (A,B).
The response variables most frequently measured in SDD studies were seed removal and seedling recruitment, reported in ∼30% of the publications in each case (Figure 4). Overall, however, the quality component of SDD has received more attention than the quantitative component. Impacts on plant and disperser species composition were also often documented, while only a few studies (<5%) evaluated community changes in terms of network structure. Finally, a small fraction of the studies (11%) has assessed whether there are evolutionary changes involved in SDD, either from the plant or the disperser’s perspective.
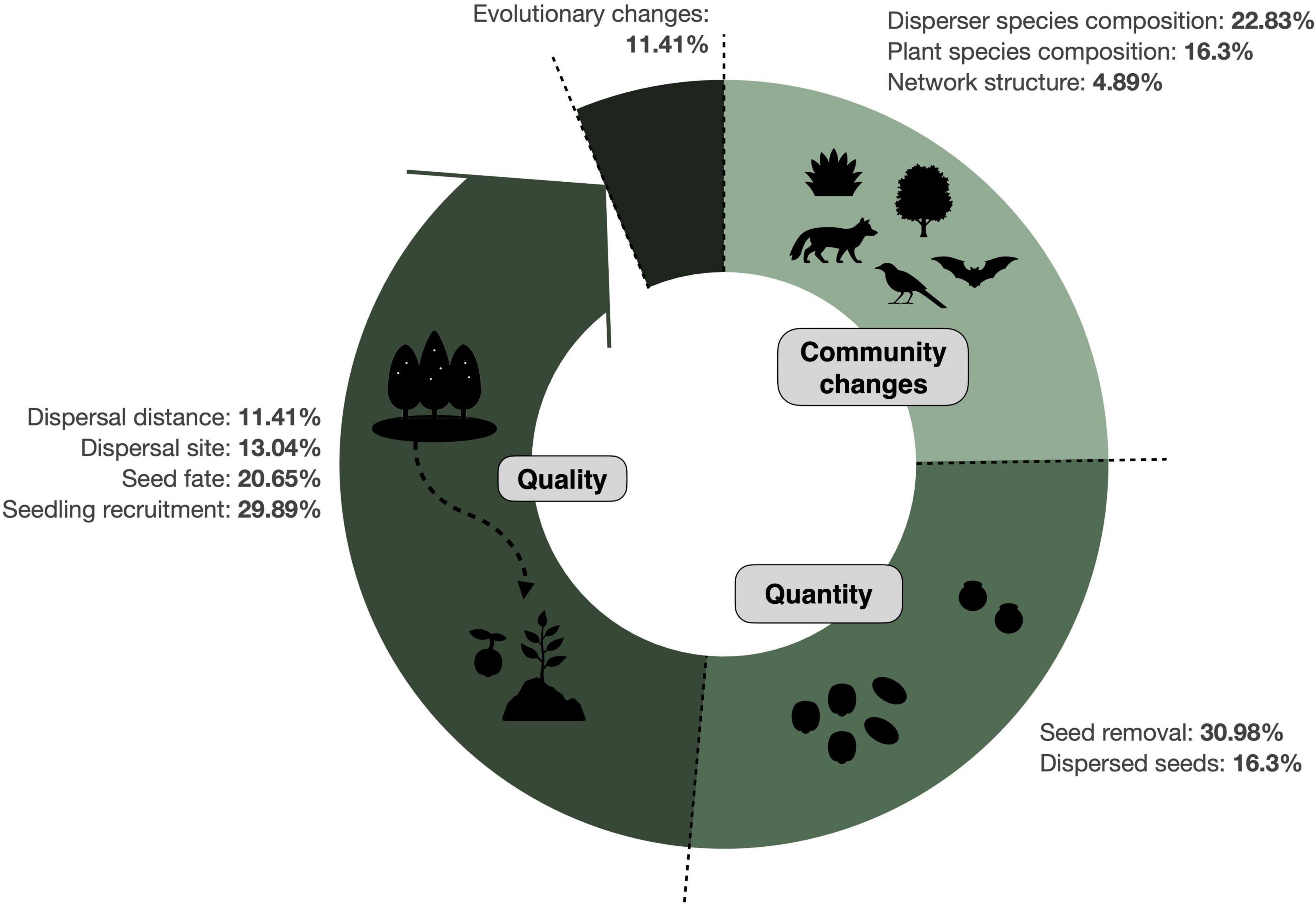
Figure 4. Response variables measured in SDD studies. Doughnut chart illustrates how SDD occurred along the seed dispersal process (represented in the direction that happens in nature) by causing community changes, disruptions in the quantity and in the quality components of seed dispersal, as well as evolutionary changes. Shown are the percentage of studies evaluating disruptions for each individual response variable (N = 327 records). See Table 2 for further details on the definition of these categories.
Although most of the studies reported biotic SDD, abiotically dispersed species have been the focus of ∼ 19% of the publications (see Supplementary Figure 2). In addition, regardless of the bioregion, SDD has been especially reported in mainland areas (∼70%), in studies carried out at a species-level (70%), and on empirical studies (87%) (see Supplementary Figure 2).
Discussion
Our review identified broad trends and research biases on SDD. The studies documented disruptions in biotically and abiotically dispersed plants, on every continent and many oceanic and continental islands, on every major biome, and involving all major groups of animal dispersers in the case of animal-dispersed plants. About two-thirds of studies have focused on the initial stages of the seed dispersal cycle—seed removal and seedling recruitment—while far fewer have dealt with the longer-term patterns demonstrated through plant community composition and evolutionary changes. Overall, about 70% of the studies focused on individual plant species, and only 30% on plant communities. We foresee that given the widespread nature of SDD and its impacts on the early life history stages in plant populations, there will be increasingly more evidence for changes in plant community composition and evolution.
The trends we identified in SDD research result from a combination of geographic and ecological patterns in seed dispersal and in global change, as well as bias in research focus. For example, islands have experienced more alien species invasions and native species extinctions than mainlands (Sax and Gaines, 2008; Turvey and Fritz, 2011; Blackburn et al., 2019). As such, this global change driver dominates island studies, with mainly birds and reptiles involved in such disruptions (see e.g., Traveset and Riera, 2005; Rumeu et al., 2011; Calviño-Cancela et al., 2012; Rogers et al., 2017; Fricke et al., 2018). Similarly, we found that SDD in the Neotropics is more frequently documented than in other regions. This could be due to Neotropical systems being more disrupted or to more research effort in this realm where biotic seed dispersal is prevalent (Jordano, 2014; Rogers et al., 2021a). The latter seemed to be the most likely reason given the following research evidence up-to-date. First, literature about global biodiversity sampling biases and biodiversity-ecosystem studies have found disproportionally poor tropical sampling overall (Clarke et al., 2017; Hughes et al., 2021). Second, results derived from the IPBES Global Report regarding the temporal trend of each main driver showed that neotropical systems were not under more threat than other high biodiversity regions (Balvanera et al., 2019). In contrast to the Neotropic, the Afrotropic and Oriental regions are understudied, especially given the commonness of biotic dispersal and the frequency of threatened species in these regions (Dirzo et al., 2014; Sridhara et al., 2016; Rogers et al., 2021a). This biogeographic bias mirrors the bias found in research on forest fragmentation and biological invasions, which has been attributed primarily to limited financial resources and political instability (Deikumah et al., 2014; Bellard and Jeschke, 2016; Chong et al., 2021). On the other hand, many studies on abiotic SDD have been carried out in the Palearctic and Nearctic (e.g., De hert et al., 2013; Labatore et al., 2017), probably because abiotic dispersal is more prevalent at higher latitudes (Kling and Ackerly, 2020; Rogers et al., 2021a) and because a good fraction of researchers investigating seed dispersal have home institutions in the temperate realm.
Although forests cover ∼30% of the world’s terrestrial landmass (FAO and UNEP, 2020), they are by far the most common ecosystem studied with respect to SDD. This likely reflects the increased prevalence of animal-mediated dispersal in forest systems, as well as a bias toward studying biodiversity, and seed dispersal in particular, in forest systems compared to, for example, grassland systems (Hughes et al., 2021). Indeed, these results might also reflect the large body of scientific research into endozoochory by frugivores addressing how fragmentation could affect seed dispersal in multiple types of forests (e.g., Herrera and Garcia, 2010; Deikumah et al., 2014; Emer et al., 2018; Lander et al., 2019). However, grasslands cover around 40% of the Earth’s surface (Bardgett et al., 2021). In grasslands, as well as in arid and wetland systems, many plants also benefit from dispersal by grazing herbivores and ants (Janzen, 1984; Wills and Landis, 2018; Anjos et al., 2020; Green et al., 2021; Hyvarinen et al., 2021). The dearth of research in non-forest ecosystems could be related to a higher proportion of abiotically (e.g., wind) dispersed species, assuming this kind of dispersal might be less limited than biotically dispersed species. Alternatively, it could also be related to the availability of suitable sites to conduct studies. In this sense, research activities could be facilitated in forest ecosystems, which usually fall within or nearby protected areas. Non-forested ecosystems, however, could be more complicated to sample, which can thus limit our global understanding of SDD.
Non-native species and defaunation were the most frequently studied global change drivers of SDD. Research efforts addressing the impact of alien species focused either on the introduction of non-native dispersers to a novel system or the loss of native dispersers caused by the introduction of non-native predators (e.g., Pejchar, 2015; Rogers et al., 2017). Biotic and abiotic seed dispersal have also been acknowledged as ecological processes that are vulnerable to climate change (Mokany et al., 2014; Kling and Ackerly, 2020). However, to date climate change has received much less attention. Given that seed dispersal is critical for species to shift ranges with climate changes (Dawson et al., 2011; Corlett and Westcott, 2013; González-Varo et al., 2021; Fricke et al., 2022), the relative lack of theoretical or empirical studies on this topic suggests SDD disruption due to climate change is an area requiring additional focus. We posit that advancing knowledge in this regard is particularly urgent given the omnipresent impact of climate change, which operates at a higher (i.e., global) scale and could thus worsen the consequences of any other driver of SDD (McConkey et al., 2012; Zhou et al., 2013; Maciel et al., 2021). For instance, one of the latest studies showed that the loss of seed dispersal interactions may impair the potential of a palm species to track climate change, especially in combination with fragmentation and forest loss (Sales et al., 2021). The handful of SDD studies found in our literature review mainly derived from empirical data concerning extreme climatic events (e.g., Zhou et al., 2013; Standish et al., 2018) or the combination of empirical data with simulations based on future climate projections and species distribution models (see e.g., Bello et al., 2021; Sales et al., 2021). More research following such an approach would thus be a promising way to provide insights into SDD under climate change, synergistic effects among drivers, and to guide management interventions for conservation and restoration.
Animal seed dispersal has been the focus of most SDD studies, although abiotic dispersal has also faced major disruptions due to habitat fragmentation, transformation, and destruction, as well as climate change. Among animal dispersers, birds and non-flying mammals are the most frequently studied groups, probably due to research bias on endozoochorous vertebrate dispersers, whereas fishes and bats are rarely studied. Only very recently, a few studies have demonstrated how overfishing may negatively affect seed dispersal, suggesting potential functional homogenization in floodplain ecosystems (Costa-Pereira et al., 2018; Araujo et al., 2021). Similarly, only a few studies showed dispersal limitation for bat-dispersed plants due to habitat loss and defaunation (Henry and Jouard, 2007; Valiente-Banuet and Verdú, 2013). This lack of SDD studies likely reflects the scant research attention of seed dispersers with nocturnal habits (Mello et al., 2011; Seltzer et al., 2013), as well as the limited knowledge on the impact of disturbances on bat’s role as seed dispersers, especially in the Old World (see Aziz et al., 2021 and references therein). These results might be particularly worrisome given the non-redundant resource use and unique ecological role of bats compared to other seed dispersers (Fleming, 1987). For abiotic dispersal, on the other hand, most available information deals with wind-dispersed species that are affected by habitat loss and fragmentation (e.g., Alados et al., 2010; Bagchi et al., 2011), non-anthropogenic abiotic factors such as wildfires (e.g., Ziegenhagen and Miller, 2009; Legras et al., 2010) or how the dispersal of hydrochorous plant species is interfered with dam constructions (e.g., Merritt and Wohl, 2006).
We found that existing research addresses processes at the earliest life stages of plants to quantify outcomes of dispersal disruption on plant populations, by studying processes such as seed removal and seedling recruitment. Few studies track the effects of dispersal long enough to determine the true cost to fitness, and relatively few explore the community-level consequences of SDD. The seed dispersal mutualism can be challenging to study either observationally or experimentally, because seeds can move long distances, few seeds successfully establish and survive to reproduce, and the influence of dispersal on seed fate can take years to discern (Beckman et al., 2020). As a result, few studies comprehensively measure the impacts of SDD. Some of the best examples of population and community-level effects come from places that have experienced widespread defaunation, such as the Canary Islands (Pérez-Méndez et al., 2016), New Zealand (Bombaci et al., 2021), the Mascarenes (Albert et al., 2020, 2021), and Guam (Wandrag et al., 2017). Furthermore, few studies are able to capture evolutionary changes as a result of SDD (Galetti et al., 2013; Carvalho et al., 2016; Traveset et al., 2019). To capture the cumulative ecological and evolutionary effects of SDD on plant species and communities, research designs that match the spatial or temporal scales at which these processes operate are necessary. These may include long-term monitoring at study sites impacted by disruption to monitor change from historical baselines (Harrison et al., 2013), experimental disruption of seed dispersal and comparisons to appropriate controls (Wandrag et al., 2017; Albert et al., 2020), and observations across gradients of SDD through remote sensing or biodiversity monitoring networks (Terborgh et al., 2008).
Future Perspectives
Seed dispersal is a critical mechanism by which plants respond to environmental change (Nathan et al., 2008). This systematic review captures the latest studies addressing SDD and quantified research gaps in terms of, not only global change drivers, but also different realms, habitats, seed dispersers and response variables less often recorded. It also highlights the potential synergistic effects among drives in both biotic and abiotic seed dispersal process, which could be impacted by one or various global change drivers. Yet, feedbacks between different processes are still rarely investigated and represent an important frontier for forecasting the threats to plant biodiversity and ecosystem services in the Anthropocene. We caution that, as we enter a period of increasing environmental change due to climate change, the accrued impacts of SDD may only now manifest. Ecosystem tipping points may occur as the pace of environmental change exceeds plants’ capacity to respond to these changes (Lenton, 2011). This prioritizes research to understand how seed dispersal function changes in human-modified environments that are impacted by multiple global change drivers. Quantifying the interactions among these drivers would be critical to avoid overestimating or underestimating threats to seed dispersal. A potential way forward to improve the predictability of multiple anthropogenic stressors could be the application of recently developed modeling frameworks based on the distribution of driver effects across targets and ecological scales (Simmons et al., 2021).
We further see the potential for significant advances in understanding the ecosystem services that seed dispersers provide as agents of biotic connectivity (e.g., enabling plant species to track climate change, González-Varo et al., 2021) and for their role in enhancing carbon storage in regenerating or intact forests (Bello et al., 2015). Although benefits of seed dispersal are well-studied and evidence for SDD is widespread, less research effort has focused on building an evidence base for the effective use of habitat corridors (Levey, 2005) or protected areas (Bombaci et al., 2021) to facilitate plant connectivity via seed dispersal. In addition, studying how dispersal mode influences which plant species experience SDD will help forecast biodiversity and ecosystem services. We anticipate that species that are abiotically dispersed or where humans commonly intentionally or unintentionally facilitate dispersal will be winners, to the detriment of many other plant species.
Data Availability Statement
The dataset generated for this study is based on the published literature and is available in Zenodo with the identifier https://doi.org/10.5281/zenodo.5794532.
Author Contributions
ID, EF, SH-P, HR, and AT assembled the data from published literature. ID prepared the figures and took the lead in writing the manuscript with substantial contributions from AT, EF, and HR and comments provided by SH-P. All authors contributed to the article and approved the submitted version.
Funding
This work was supported by the Balearic Government to ID and SH-P, and the Spanish Ministry of Science to AT (project CGL2017-88122-P and PID2020-114324GB-C21).
Conflict of Interest
The authors declare that the research was conducted in the absence of any commercial or financial relationships that could be construed as a potential conflict of interest.
Publisher’s Note
All claims expressed in this article are solely those of the authors and do not necessarily represent those of their affiliated organizations, or those of the publisher, the editors and the reviewers. Any product that may be evaluated in this article, or claim that may be made by its manufacturer, is not guaranteed or endorsed by the publisher.
Supplementary Material
The Supplementary Material for this article can be found online at: https://www.frontiersin.org/articles/10.3389/fevo.2022.794481/full#supplementary-material
Footnotes
References
Alados, C. L., Navarro, T., Komac, B., Pascual, V., and Rietkerk, M. (2010). Dispersal abilities and spatial patterns in fragmented landscapes: spatial organization with fragmentation. Biol. J. Linn. Soc. 100, 935–947. doi: 10.1111/j.1095-8312.2010.01465.x
Albert, A., Auffret, A. G., Cosyns, E., Cousins, S. A. O., D’hondt, B., Eichberg, C., et al. (2015). Seed dispersal by ungulates as an ecological filter: a trait-based meta-analysis. Oikos 124, 1109–1120. doi: 10.1111/oik.02512
Albert, S., Flores, O., and Strasberg, D. (2020). Collapse of dispersal trait diversity across a long-term chronosequence reveals a strong negative impact of frugivore extinctions on forest resilience. J. Ecol. 108, 1386–1397. doi: 10.1111/1365-2745.13359
Albert, S., Flores, O., Baider, C., Florens, F. V., and Strasberg, D. (2021). Differing severity of frugivore loss contrasts the fate of native forests on the land of the Dodo (Mascarene archipelago). Biol. Conserv. 257:109131. doi: 10.1016/j.biocon.2021.109131
Anjos, D. V., Leal, L. C., Jordano, P., and Del-Claro, K. (2020). Ants as diaspore removers of non-myrmecochorous plants: a meta-analysis. Oikos 129, 775–786. doi: 10.1111/oik.06940
Araujo, J. M., Correa, S. B., Penha, J., Anderson, J., and Traveset, A. (2021). Implications of overfishing of frugivorous fishes for cryptic function loss in a Neotropical floodplain. J. Appl. Ecol. 58, 1499–1510. doi: 10.1111/1365-2664.13891
Atwood, T. B., Valentine, S. A., Hammill, E., McCauley, D. J., Madin, E. M. P., Beard, K. H., et al. (2020). Herbivores at the highest risk of extinction among mammals, birds, and reptiles. Sci. Adv. 6:eabb8458. doi: 10.1126/sciadv.abb8458
Aziz, S. A., McConkey, K. R., Tanalgo, K., Sritongchuay, T., Low, M. R., Yong, J. Y., et al. (2021). The critical importance of Old World fruit bats for healthy ecosystems and economies. Front. Ecol. Evol. 9:641411. doi: 10.3389/fevo.2021.641411
Bagchi, R., Philipson, C. D., Slade, E. M., Hector, A., Phillips, S., Villanueva, J. F., et al. (2011). Impacts of logging on density-dependent predation of dipterocarp seeds in a South East Asian rainforest. Philos. Trans. R. Soc. B Biol. Sci. 366, 3246–3255. doi: 10.1098/rstb.2011.0034
Balvanera, P., Pfaff, A., Viña, A., Garcia Frapolli, E., Hussain, S. A., and Merino, L. (2019). “IPBES global assessment report on biodiversity and ecosystem services. Chapter 2.1 status and trends – drivers of change,” in Global Assessment Report of the Intergovernmental Science-Policy Platform on Biodiversity and Ecosystem Services, eds L. Eric and M. Jayalaxshmi (Bonn: IPBES Secretariat). doi: 10.5281/zenodo.3831881
Bardgett, R. D., Bullock, J. M., Lavorel, S., Manning, P., Schaffner, U., Ostle, N., et al. (2021). Combatting global grassland degradation. Nat. Rev. Earth Environ. 2, 720–735. doi: 10.1038/s43017-021-00207-2
Beckman, N. G., Aslan, C. E., Rogers, H. S., Kogan, O., Bronstein, J. L., Bullock, J. M., et al. (2020). Advancing an interdisciplinary framework to study seed dispersal ecology. AoB Plants 12, 1–18. doi: 10.1093/AOBPLA/PLZ048
Bellard, C., and Jeschke, J. M. (2016). A spatial mismatch between invader impacts and research publications. Conserv. Biol. 30, 230–232. doi: 10.1111/cobi.12611
Bello, C., Cintra, A. L. P., Barreto, E., Vancine, M. H., Sobral-Souza, T., Graham, C. H., et al. (2021). Environmental niche and functional role similarity between invasive and native palms in the Atlantic Forest. Biol. Invasions 23, 741–754. doi: 10.1007/s10530-020-02400-8
Bello, C., Galetti, M., Pizo, M. A., Magnago, L. F. S., Rocha, M. F., Lima, R. A. F., et al. (2015). Defaunation affects carbon storage in tropical forests. Sci. Adv. 1:e1501105. doi: 10.1126/sciadv.1501105
Blackburn, T. M., Bellard, C., and Ricciardi, A. (2019). Alien versus native species as drivers of recent extinctions. Front. Ecol. Environ. 17:203–207. doi: 10.1002/fee.2020
Bombaci, S. P., Innes, J., Kelly, D., Flaherty, V., and Pejchar, L. (2021). Excluding mammalian predators increases bird densities and seed dispersal in fenced ecosanctuaries. Ecology 102, 1–9. doi: 10.1002/ecy.3340
Brocardo, C. R., Pedrosa, F., and Galetti, M. (2018). Forest fragmentation and selective logging affect the seed survival and recruitment of a relictual conifer. For. Ecol. Manag. 408, 87–93. doi: 10.1016/j.foreco.2017.09.046
Calviño-Cancela, M., Escudero, M., Rodríguez-Pérez, J., Cano, E., Vargas, P., Velo-Antón, G., et al. (2012). The role of seed dispersal, pollination and historical effects on genetic patterns of an insular plant that has lost its only seed disperser: genetic patterns in a disrupted mutualism. J. Biogeogr. 39, 1996–2006. doi: 10.1111/j.1365-2699.2012.02732.x
Carlo, T. A., and Yang, S. (2011). Network models of frugivory and seed dispersal: challenges and opportunities. Acta Oecol. 37, 619–624. doi: 10.1016/j.actao.2011.08.001
Carvalho, C. S., Galetti, M., Colevatti, R. G., and Jordano, P. (2016). Defaunation leads to microevolutionary changes in a tropical palm. Sci. Rep. 6:31957. doi: 10.1038/srep31957
Chagas, G., Salk, C. F., Vidal, E. J., Souza, S. E. X. F., and Brancalion, P. H. S. (2021). Exploiting fruits of a threatened palm to trigger restoration of Brazil’s Atlantic Forest. Restor. Ecol. 29, 1–10. doi: 10.1111/rec.13294
Chong, K. Y., Corlett, R. T., Nuñez, M. A., Chiu, J. H., Courchamp, F., Dawson, W., et al. (2021). Are terrestrial biological invasions different in the tropics? Annu. Rev. Ecol. Evol. Syst. 52, 291–314. doi: 10.1146/annurev-ecolsys-012021-095454
Clarke, D. A., York, P. H., Rasheed, M. A., and Northfield, T. D. (2017). Does biodiversity–ecosystem function literature neglect tropical ecosystems? Trends Ecol. Evol. 32, 320–323. doi: 10.1016/j.tree.2017.02.012
Corlett, R. T., and Westcott, D. A. (2013). Will plant movements keep up with climate change? Trends Ecol. Evol. 28, 482–488. doi: 10.1016/j.tree.2013.04.003
Correa, S. B., Costa-Pereira, R., Fleming, T., Goulding, M., and Anderson, J. T. (2015). Neotropical fish-fruit interactions: eco-evolutionary dynamics and conservation. Biol. Rev. 90, 1263–1278. doi: 10.1111/brv.12153
Costa-Pereira, R., Lucas, C., Crossa, M., Anderson, J. T., Albuquerque, B. W., Dary, E. P., et al. (2018). Defaunation shadow on mutualistic interactions. Proc. Natl. Acad. Sci. U.S.A. 115, E2673–E2675. doi: 10.1073/pnas.1801106115
Davidson, A. D., Hamilton, M. J., Boyer, A. G., Brown, J. H., and Ceballos, G. (2009). Multiple ecological pathways to extinction in mammals. Proc. Natl. Acad. Sci. U.S.A. 106, 10702–10705. doi: 10.1073/pnas.0901956106
Dawson, T. P., Jackson, S. T., House, J. I., Prentice, I. C., and Mace, G. M. (2011). Beyond predictions: biodiversity conservation in a changing climate. Science 332, 53–58. doi: 10.1126/science.1200303
de Assis Bomfim, J., Guimarães, P. R., Peres, C. A., Carvalho, G., and Cazetta, E. (2018). Local extinctions of obligate frugivores and patch size reduction disrupt the structure of seed dispersal networks. Ecography 41, 1899–1909. doi: 10.1111/ecog.03592
De hert, K., Jacquemyn, H., Provoost, S., and Honnay, O. (2013). Absence of recruitment limitation in restored dune slacks suggests that manual seed introduction can be a successful practice for restoring orchid populations: seed introduction as restoration practice in orchids. Restor. Ecol. 21, 159–162. doi: 10.1111/j.1526-100X.2012.00925.x
Deikumah, J. P., Mcalpine, C. A., and Maron, M. (2014). Biogeographical and taxonomic biases in tropical forest fragmentation research. Conserv. Biol. 28, 1522–1531. doi: 10.1111/cobi.12348
Dennis, A. J., and Cooperative Research Centre for Tropical Rainforest Ecology and Management, and Csiro (Australia), (eds). (2007). Seed Dispersal: Theory and Its Application in a Changing World. Cambridge, MA: CABI.
Dirzo, R., Young, H. S., Galetti, M., Ceballos, G., Isaac, N. J. B., and Collen, B. (2014). Defaunation in the Anthropocene. Science 345, 401–406. doi: 10.1126/science.1251817
Donoso, I., Sorensen, M. C., Blendinger, P. G., Kissling, W. D., Neuschulz, E. L., Mueller, T., et al. (2020). Downsizing of animal communities triggers stronger functional than structural decay in seed-dispersal networks. Nat. Commun. 11:1582. doi: 10.1038/s41467-020-15438-y
Egerer, M. H., Fricke, E. C., and Rogers, H. S. (2018). Seed dispersal as an ecosystem service: frugivore loss leads to decline of a socially valued plant, Capsicum frutescens. Ecol. Appl. 28, 655–667. doi: 10.1002/eap.1667
Emer, C., Galetti, M., Pizo, M. A., Guimaraes, Jr P. R., Moraes, S., Piratelli, A., et al. (2018). Seed-dispersal interactions in fragmented landscapes-a metanetwork approach. Ecol. Lett. 21, 484–493. doi: 10.1111/ele.12909
FAO and UNEP (2020). The State of the World’s Forests 2020. Rome: FAO and UNEP. doi: 10.4060/ca8642en
Fleming, T. H., and John Kress, W. (2011). A brief history of fruits and frugivores. Acta Oecol. 37, 521–530. doi: 10.1016/j.actao.2011.01.016
Fricke, E. C., and Svenning, J. C. (2020). Accelerating homogenization of the global plant–frugivore meta-network. Nature 585, 74–78. doi: 10.1038/s41586-020-2640-y
Fricke, E. C., Ordonez, A., Rogers, H. S., and Svenning, J. C. (2022). The effects of defaunation on plants’ capacity to track climate change. Science 375, 210–214. doi: 10.1126/science.abk3510
Fricke, E. C., Tewksbury, J. J., and Rogers, H. S. (2018). Defaunation leads to interaction deficits, not interaction compensation, in an island seed dispersal network. Glob. Change Biol. 24, e190–e200. doi: 10.1111/gcb.13832
Fuzessy, L. F., Janson, C., and Silveira, F. A. (2018). Effects of seed size and frugivory degree on dispersal by Neotropical frugivores. Acta Oecol. 93, 41–47. doi: 10.1016/j.actao.2018.10.004
Galetti, M., Guevara, R., Cortes, M. C., Fadini, R., Von Matter, S., Leite, A. B., et al. (2013). Functional extinction of birds drives rapid evolutionary changes in seed size. Science 340, 1086–1090. doi: 10.1126/science.1233774
Galetti, M., Moleón, M., Jordano, P., Pires, M. M., Guimarães, P. R., Pape, T., et al. (2018). Ecological and evolutionary legacy of megafauna extinctions. Biol. Rev. 93, 845–862. doi: 10.1111/brv.12374
Gómez, J. M., Schupp, E. W., and Jordano, P. (2019). Synzoochory: the ecological and evolutionary relevance of a dual interaction. Biol. Rev. 94, 874–902. doi: 10.1111/brv.12481
González-Varo, J. P., Biesmeijer, J. C., Bommarco, R., Potts, S. G., Schweiger, O., Smith, H. G., et al. (2013). Combined effects of global change pressures on animal-mediated pollination. Trends Ecol. Evol. 28, 524–530. doi: 10.1016/j.tree.2013.05.008
González-Varo, J. P., Rumeu, B., Albrecht, J., Arroyo, J. M., Bueno, R. S., Burgos, T., et al. (2021). Limited potential for bird migration to disperse plants to cooler latitudes. Nature 595, 75–79. doi: 10.1038/s41586-021-03665-2
Green, A. J., Baltzinger, C., and Lovas-Kiss, Á (2021). Plant dispersal syndromes are unreliable, especially for predicting zoochory and long-distance dispersal. Oikos doi: 10.1111/oik.08327
Green, A. J., Soons, M., Brochet, A.-L., and Kleyheeg, E. (2016). “Chapter 6. Dispersal of plants by waterbirds,” in Why Birds Matter: Avian Ecological Function and Ecosystem Services, eds Ç.H. Sekercioglu, D. G. Wenny, and C. J. Whelan (Chicago, IL: University of Chicago Press), 147–195. doi: 10.7208/chicago/9780226382777.001.0001
Herrera, J. M., and Garcia, D. (2010). Effects of forest fragmentation on seed dispersal and seedling establishment in ornithochorous trees. Conserv. Biol., 24, 1089–1098. doi: 10.1111/j.1523-1739.2010.01459.x
Harrison, R. D., Tan, S., Plotkin, J. B., Slik, F., Detto, M., Brenes, T., et al. (2013). Consequences of defaunation for a tropical tree community. Ecol. Lett. 16, 687–694. doi: 10.1111/ele.12102
Henry, M., and Jouard, S. (2007). Effect of bat exclusion on patterns of seed rain in tropical rain forest in French Guiana. Biotropica 39, 510–518. doi: 10.1111/j.1744-7429.2007.00286.x
Holt, B. G., Lessard, J.-P., Borregaard, M. K., Fritz, S. A., Araújo, M. B., Dimitrov, D., et al. (2013). An update of wallace’s zoogeographic regions of the world. Science 339, 74–78. doi: 10.1126/science.1228282
Howe, H. F., and Miriti, M. N. (2004). When seed dispersal matters. BioScience 54, 651–660. doi: 10.1641/0006-3568(2004)054[0651:wsdm]2.0.co;2
Howe, H. F., and Smallwood, J. (1982). Ecology of seed dispersal. Annu. Rev. Ecol. Syst. 13, 201–228. doi: 10.1146/annurev.es.13.110182.001221
Hughes, A. C., Orr, M. C., Ma, K., Costello, M. J., Waller, J., Provoost, P., et al. (2021). Sampling biases shape our view of the natural world. Ecography 44, 1259–1269. doi: 10.1111/ecog.05926
Hyvarinen, O., Te Beest, M., le Roux, E., Kerley, G., de Groot, E., Vinita, R., et al. (2021). Megaherbivore impacts on ecosystem and Earth system functioning: the current state of the science. Ecography 44, 1–16. doi: 10.1111/ecog.05703
Jansson, R., Nilsson, C., and Renöfält, B. (2000). Fragmentation of riparian floras in rivers with multiple dams. Ecology 81, 899–903.
Janzen, D. H. (1984). Dispersal of small seeds by big herbivores: foliage is the fruit. Am. Nat. 123, 338–353. doi: 10.1086/284208
Janzen, D. H., and Martin, P. S. (1982). Neotropical anachronisms: the fruits the gomphotheres ate. Science 215, 19–27. doi: 10.1126/science.215.4528.19
Jones, L. R., Duke-Sylvester, S. M., Leberg, P. L., and Johnson, D. M. (2017). Closing the gaps for animal seed dispersal: separating the effects of habitat loss on dispersal distances and seed aggregation. Ecol. Evol. 7, 5410–5425. doi: 10.1002/ece3.3113
Jordano, P. (2014). “Fruits and frugivory,” in Seeds: The Ecology of Regeneration in Plant Communities, ed. R. S. Gallagher (Wallingford: CABI), 18–61. doi: 10.1079/9781780641836.0018
Kiel, N. G., Griffiths, G. R., and McGee, G. G. (2020). Can disruption of an ant–plant mutualism explain a lack of recovery of forest herbs in post-agricultural forests of New York? Northeast. Nat. 27:215. doi: 10.1656/045.027.0204
Kingston, T., Florens, F. B. V., and Vincenot, C. E. (2021). Crumbling Island keystones: threat diversity and intensification on Islands push large island fruit bats to the brink. EcoEvoRxiv [Preprint]. doi: 10.32942/osf.io/ehjcn
Kling, M. M., and Ackerly, D. D. (2020). Global wind patterns and the vulnerability of wind-dispersed species to climate change. Nat. Clim. Change 10, 868–875. doi: 10.1038/s41558-020-0848-3
Labatore, A. C., Spiering, D. J., Potts, D. L., and Warren, R. J. (2017). Canopy trees in an urban landscape – viable forests or long-lived gardens? Urban Ecosyst. 20, 393–401. doi: 10.1007/s11252-016-0601-x
Lander, T. A., Harris, S. A., Cremona, P. J., and Boshier, D. H. (2019). Impact of habitat loss and fragmentation on reproduction, dispersal and species persistence for an endangered Chilean tree. Conserv. Genet. 20, 973–985. doi: 10.1007/s10592-019-01187-z
Legras, E. C., Vander Wall, S. B., and Board, D. I. (2010). The role of germination microsite in the establishment of sugar pine and Jeffrey pine seedlings. For. Ecol. Manag. 260, 806–813. doi: 10.1016/j.foreco.2010.05.039
Lenton, T. M. (2011). Early warning of climate tipping points. Nat. Clim. Change 1, 201–209. doi: 10.1038/nclimate1143
Leverkus, A. B., and Castro, J. (2017). An ecosystem services approach to the ecological effects of salvage logging: valuation of seed dispersal. Ecol. Appl. 27, 1057–1063. doi: 10.1002/eap.1539
Levey, D. J. (2005). Effects of landscape corridors on seed dispersal by birds. Science 309, 146–148. doi: 10.1126/science.1111479
Levey, D. J., Silva, W. R., and Galetti, M. (eds). (2002). Seed Dispersal and Frugivory: Ecology, Evolution, and Conservation. New York, NY: CABI Pub.
Maciel, E. A., Martins, V. F., de Paula, M. D., Huth, A., Guilherme, F. A. G., Fischer, R., et al. (2021). Defaunation and changes in climate and fire frequency have synergistic effects on aboveground biomass loss in the brazilian savanna. Ecol. Model. 454:109628. doi: 10.1016/j.ecolmodel.2021.109628
Malhi, Y., Doughty, C. E., Galetti, M., Smith, F. A., Svenning, J.-C., and Terborgh, J. W. (2016). Megafauna and ecosystem function from the Pleistocene to the Anthropocene. Proc. Natl. Acad. Sci. U.S.A. 113, 838–846. doi: 10.1073/pnas.1502540113
Marone, L., and Pol, R. G. (2021). Continuous grazing disrupts desert grass-soil seed bank composition under variable rainfall. Plant Ecol. 222, 247–259. doi: 10.1007/s11258-020-01102-4
McConkey, K. R., Prasad, S., Corlett, R. T., Campos-Arceiz, A., Brodie, J. F., Rogers, H., et al. (2012). Seed dispersal in changing landscapes. Biol. Conserv. 146, 1–13. doi: 10.1016/j.biocon.2011.09.018
Mello, M. A. R., Marquitti, F. M. D., Guimarães, P. R., Kalko, E. K. V., Jordano, P., and de Aguiar, M. A. M. (2011). The missing part of seed dispersal networks: structure and robustness of bat-fruit interactions. PLoS One 6:e0017395. doi: 10.1371/journal.pone.0017395
Merritt, D. M., and Wohl, E. E. (2006). Plant dispersal along rivers fragmented by dams. River Res. Appl. 22, 1–26. doi: 10.1002/rra.890
Mokany, K., Prasad, S., and Westcott, D. A. (2014). Loss of frugivore seed dispersal services under climate change. Nat. Commun. 5:3971. doi: 10.1038/ncomms4971
Nathan, R., Schurr, F. M., Spiegel, O., Steinitz, O., Trakhtenbrot, A., and Tsoar, A. (2008). Mechanisms of long-distance seed dispersal. Trends Ecol. Evol. 23, 638–647. doi: 10.1016/j.tree.2008.08.003
Page, M. J., McKenzie, J. E., Bossuyt, P. M., Boutron, I., Hoffmann, T. C., Mulrow, C. D., et al. (2021). The PRISMA 2020 statement: an updated guideline for reporting systematic reviews. BMJ 372:n71. doi: 10.1136/bmj.n71
Pejchar, L. (2015). Introduced birds incompletely replace seed dispersal by a native frugivore. AoB Plants 7:lv072. doi: 10.1093/aobpla/plv072
Pérez-Méndez, N., Jordano, P., García, C., and Valido, A. (2016). The signatures of Anthropocene defaunation: cascading effects of the seed dispersal collapse. Sci. Rep. 6:24820. doi: 10.1038/srep24820
Portela, R. C. Q., and Dirzo, R. (2020). Forest fragmentation and defaunation drive an unusual ecological cascade: predation release, monkey population outburst and plant demographic collapse. Biol. Conserv. 252:108852. doi: 10.1016/j.biocon.2020.108852
Qie, L., Elsy, A. D., Stumvoll, A., Kwasnicka, M., Peel, A. L., Sullivan, J. A., et al. (2019). Impending regeneration failure of the IUCN vulnerable borneo ironwood (Eusideroxylon zwageri). Trop. Conserv. Sci. 12:194008291882335. doi: 10.1177/1940082918823353
Ripple, W. J., Newsome, T. M., Wolf, C., Dirzo, R., Everatt, K. T., Galetti, M., et al. (2015). Collapse of the world’s largest herbivores. Sci. Adv. 1:e1400103. doi: 10.1126/sciadv.1400103
Rogers, H. S., Buhle, E. R., HilleRisLambers, J., Fricke, E. C., Miller, R. H., and Tewksbury, J. J. (2017). Effects of an invasive predator cascade to plants via mutualism disruption. Nat. Commun. 8:14557. doi: 10.1038/ncomms14557
Rogers, H. S., Donoso, I., Traveset, A., and Fricke, and F. C. (2021a). Cascading impacts of seed disperser loss on plant communities and ecosystems. Annu. Rev. Ecol. Evol. Syst. 52, 641–666 doi: 10.1146/annurev-ecolsys-012221-111742
Rogers, H. S., Cavazos, B. R., Gawel, A. M., Karnish, A. T., Ray, C. A., Rose, E., et al. (2021b). Frugivore gut passage increases seed germination: an updated meta-analysis. bioRxiv [Preprint]. doi: 10.1101/2021.10.12.462022
Rosenberg, K. V., Dokter, A. M., Blancher, P. J., Sauer, J. R., Smith, A. C., Smith, P. A., et al. (2019). Decline of the North American avifauna. Science 366, 120–124. doi: 10.1126/science.aaw1313
Rotllan-Puig, X., and Traveset, A. (2016). Declining relict plants: climate effect or seed dispersal disruption? A landscape-scale approach. Basic Appl. Ecol. 17, 81–91. doi: 10.1016/j.baae.2015.08.003
Rumeu, B., Elias, R. B., Padilla, D. P., Melo, C., and Nogales, M. (2011). Differential seed dispersal systems of endemic junipers in two oceanic Macaronesian archipelagos: the influence of biogeographic and biological characteristics. Plant Ecol. 212, 911–921. doi: 10.1007/s11258-010-9875-x
Sales, L. P., Rodrigues, L., and Masiero, R. (2021). Climate change drives spatial mismatch and threatens the biotic interactions of the Brazil nut. Glob. Ecol. Biogeogr. 30, 117–127. doi: 10.1111/geb.13200
San-José, M., Arroyo-Rodríguez, V., Jordano, P., Meave, J. A., and Martínez-Ramos, M. (2019). The scale of landscape effect on seed dispersal depends on both response variables and landscape predictor. Landsc. Ecol. 34, 1069–1080. doi: 10.1007/s10980-019-00821-y
Sax, D. F., and Gaines, S. D. (2008). Species invasions and extinction: The future of native biodiversity on islands. Proc. Natl. Acad. Sci. U.S.A. 105, 11490–11497. doi: 10.1073/pnas.0802290105
Schupp, E. W. (1993). Quantity, quality and the effectiveness of seed dispersal by animals. Vegetatio 107, 15–29. doi: 10.1007/BF00052209
Seltzer, C. E., Ndangalasi, H. J., and Cordeiro, N. J. (2013). Seed dispersal in the dark: shedding light on the role of fruit bats in Africa. Biotropica 45, 450–456. doi: 10.1111/btp.12029
Simmons, B. I., Blyth, P. S. A., Blanchard, J. L., Clegg, T., Delmas, E., Garnier, A., et al. (2021). Refocusing multiple stressor research around the targets and scales of ecological impacts. Nat. Ecol. Evol. 5, 1478–1489. doi: 10.1038/s41559-021-01547-4
Sridhara, S., McConkey, K., Prasad, S., and Corlett, R. T. (2016). “Frugivory and seed dispersal by large herbivores of Asia,” in The Ecology of Large Herbivores in South and Southeast Asia Ecological Studies, eds F. S. Ahrestani and M. Sankaran (Dordrecht: Springer Netherlands), 121–150. doi: 10.1007/978-94-017-7570-0_5
Standish, R. J., Gove, A. D., Daws, M. I., and Renton, M. (2018). Nestedness patterns reveal impacts of reduced rainfall on seedling establishment in restored jarrah forest. Forest Ecol. Manag. 427, 242–249. doi: 10.1016/j.foreco.2018.06.006
Teller, B. J., Miller, A. D., and Shea, K. (2015). Conservation of passively dispersed organisms in the context of habitat degradation and destruction. J. Appl. Ecol. 52, 514–521. doi: 10.1111/1365-2664.12379
Terborgh, J., Nuñez-Iturri, G., Pitman, N. C. A., Valverde, F. H. C., Alvarez, P., Swamy, V., et al. (2008). Tree recruitment in an empty forest. Ecology 89, 1757–1768. doi: 10.1890/07-0479.1
Traveset, A., and Richardson, D. (2006). Biological invasions as disruptors of plant reproductive mutualisms. Trends Ecol. Evol. 21, 208–216. doi: 10.1016/j.tree.2006.01.006
Traveset, A., and Riera, N. (2005). Disruption of a plant-lizard seed dispersal system and its ecological effects on a threatened endemic plant in the Balearic Islands. Conserv. Biol. 19, 421–431. doi: 10.1111/j.1523-1739.2005.00019.x
Traveset, A., and Verdú, M. (2002). “A meta-analysis of the effect of gut treatment on seed germination,” in Proceedings of the Seed Dispersal and Frugivory: Ecology, Evolution and Conservation. Third International Symposium-Workshop on Frugivores and Seed Dispersal, São Pedro, Brazil, 6-11 August 2000, (Wallingford: CABI), 339–350. doi: 10.1079/9780851995250.0339
Traveset, A., Escribano-Avila, G., Gómez, J. M., and Valido, A. (2019). Conflicting selection on Cneorum tricoccon (Rutaceae) seed size caused by native and alien seed dispersers. Evolution 73, 2204–2215. doi: 10.1111/evo.13852
Traveset, A., González-Varo, J. P., and Valido, A. (2012). Long-term demographic consequences of a seed dispersal disruption. Proc. R. Soc. B Biol. Sci. 279, 3298–3303. doi: 10.1098/rspb.2012.0535
Traveset, A., Heleno, R., and Nogales, M. (2014). “The ecology of seed dispersal,” in Seeds: The Ecology of Regeneration in Plant Communities, ed. R. S. Gallagher (Wallingford: CABI), 62–93. doi: 10.1079/9781780641836.0062
Tucker, M. A., Busana, M., Huijbregts, M. A. J., and Ford, A. T. (2021). Human-induced reduction in mammalian movements impacts seed dispersal in the tropics. Ecography 44, 897–906. doi: 10.1111/ecog.05210
Turvey, S. T., and Fritz, S. A. (2011). The ghosts of mammals past: biological and geographical patterns of global mammalian extinction across the holocene. Philos. Trans. R. Soc. B Biol. Sci. 366, 2564–2576. doi: 10.1098/rstb.2011.0020
Tylianakis, J. M., Didham, R. K., Bascompte, J., and Wardle, D. A. (2008). Global change and species interactions in terrestrial ecosystems. Ecol. Lett. 11, 1351–1363. doi: 10.1111/j.1461-0248.2008.01250.x
Valiente-Banuet, A., and Verdú, M. (2013). Human impacts on multiple ecological networks act synergistically to drive ecosystem collapse. Front. Ecol. Environ. 11:408–413. doi: 10.1890/130002
Wandrag, E. M., Dunham, A. E., Duncan, R. P., and Rogers, H. S. (2017). Seed dispersal increases local species richness and reduces spatial turnover of tropical tree seedlings. Proc. Natl. Acad. Sci. U.S.A. 114, 10689–10694. doi: 10.1073/pnas.1709584114
Wang, B. C., and Smith, T. B. (2002). Closing the seed dispersal loop. Trends Ecol. Evol. 17, 379–386. doi: 10.1016/S0169-5347(02)02541-7
Wills, B. D., and Landis, D. A. (2018). The role of ants in north temperate grasslands: a review. Oecologia 186, 323–338. doi: 10.1007/s00442-017-4007-0
Young, H. S., McCauley, D. J., Galetti, M., and Dirzo, R. (2016). Patterns, causes, and consequences of anthropocene defaunation. Annu. Rev. Ecol. Evol. Syst. 47, 333–358. doi: 10.1146/annurev-ecolsys-112414-054142
Zhang, R., Jongejans, E., and Shea, K. (2011). Warming increases the spread of an invasive thistle. PLoS One 6:e21725. doi: 10.1371/journal.pone.0021725
Zhou, Y., Newman, C., Chen, J., Xie, Z., and Macdonald, D. W. (2013). Anomalous, extreme weather disrupts obligate seed dispersal mutualism: snow in a subtropical forest ecosystem. Glob. Change Biol. 19, 2867–2877. doi: 10.1111/gcb.12245
Keywords: dispersal failure, global change, anthropogenic disturbance, non-native species, climate change, defaunation, habitat loss, fragmentation
Citation: Donoso I, Fricke EC, Hervías-Parejo S, Rogers HS and Traveset A (2022) Drivers of Ecological and Evolutionary Disruptions in the Seed Dispersal Process: Research Trends and Biases. Front. Ecol. Evol. 10:794481. doi: 10.3389/fevo.2022.794481
Received: 13 October 2021; Accepted: 18 January 2022;
Published: 22 February 2022.
Edited by:
Robert Ptacnik, Wasser Cluster Lunz, AustriaReviewed by:
Francisco E. Fonturbel, Pontificia Universidad Católica de Valparaíso, ChileKim McConkey, National Institute of Advanced Studies, India
Copyright © 2022 Donoso, Fricke, Hervías-Parejo, Rogers and Traveset. This is an open-access article distributed under the terms of the Creative Commons Attribution License (CC BY). The use, distribution or reproduction in other forums is permitted, provided the original author(s) and the copyright owner(s) are credited and that the original publication in this journal is cited, in accordance with accepted academic practice. No use, distribution or reproduction is permitted which does not comply with these terms.
*Correspondence: Isabel Donoso, isa.donoso@imedea.uib-csic.es, idonoso002@gmail.com