- 1Harte Research Institute, Texas A&M University-Corpus Christi, Corpus Christi, TX, United States
- 2Department of Life Sciences, Texas A&M University-Corpus Christi, Corpus Christi, TX, United States
Restoration of shellfish reefs has increased exponentially over the past two decades, due in part to increased awareness of widespread oyster habitat loss. Large-scale, acute disturbances such as hurricanes have the potential to influence restoration outcomes, but because storm occurrence is unpredictable with respect to restoration timelines, the responses of restored habitats are not well understood. We quantified the ecological dynamics of a newly constructed Crassostrea virginica oyster reef and nearby reference reef in a Texas estuary immediately after Hurricane Harvey, a major category 4 storm. Biophysical structure (e.g., oyster density, shell height, sediment grain size), and community composition (abundance of reef-associated epifauna, and nearby infauna) were measured for 18 months. A sharp decrease in salinity and temporary deposition of fine sediments within the first 3 months corresponded with increases in oyster and epifaunal recruitment on the restored reef, although densities were generally below those measured on restored reefs without hurricanes. Criteria for oyster reef restoration success were met within 12–18 months post-storm. Infaunal densities decreased but returned to pre-storm densities within 2 months, but bivalves were delayed, returning to pre-storm levels after 9 months. A lack of historical baseline data on the newly restored reef limited our ability to assess the magnitude of reef recovery to pre-disturbance levels or separate the direct effects of the hurricane from the dynamics of early recruitment and growth. Results provide important information about restored and natural oyster reef dynamics after large-scale disturbance and can help inform effective management and conservation measures.
Introduction
High-quality estuarine habitats are essential for supporting reproduction, growth, and persistence of dense aggregations of estuarine fauna (Boesch and Turner, 1984). Reefs formed by the oyster Crassostrea virginica are a critical habitat of estuarine ecosystems in the United States. Gulf of Mexico and Atlantic coasts that provide important ecosystem benefits (Breitburg et al., 1995; Harding and Mann, 2001; Gutierrez et al., 2003; Grabowski et al., 2012; Beseres Pollack et al., 2013). Oyster reef restoration has increased exponentially over the past two decades, due in part to increased awareness of widespread oyster habitat loss (Rothschild et al., 1994; Kirby, 2004; Jackson, 2008; Beck et al., 2011; zu Ermgassen et al., 2012). Because the frequency and intensity of extreme climatic events has increased in many regions (Wetz and Yoskowitz, 2013), restored habitats are being increasingly confronted by large-scale, acute disturbances that have the potential to influence restoration outcomes.
Disturbance is a central organizing principle in community ecology (Dayton, 1971; Levin, 1984; Sousa, 1984), with the timing, magnitude and duration of the disturbance influencing response and recovery dynamics. Whereas small-scale disturbances at intermediate frequencies may have beneficial effects on ecological systems (Connell, 1978), larger-scale disturbances can negatively affect community composition and slow recovery rates. Resilient systems can return to pre-disturbance or near pre-disturbance conditions within a reasonable time frame following a disturbance (Holling, 1973). However, because the occurrence of large-scale disturbances is unpredictable with respect to construction of restored habitats and monitoring timelines, the responses of restored habitats are not well understood.
Hurricanes and tropical storms are relatively common large-scale disturbances along the United States. Gulf and Atlantic coasts, and are often accompanied by coastal flooding, erosion, and altered salinities that influence the distribution and abundance of estuarine organisms (Paerl et al., 2001; Patrick et al., 2020; Walker et al., 2021). Resulting periods of prolonged low salinity can lead to increases in oyster mortality and reductions in oyster reef structure (e.g., oyster size, density; Munroe et al., 2013; Du et al., 2021). Sedimentation and loss of cultch may also contribute to high oyster mortality (Berrigan, 1990; Perret et al., 1999). Alterations to structural habitat complexity can have significant impacts on biodiversity (Airoldi et al., 2008) and ecosystem dynamics within oyster reef ecosystems (Jackson et al., 2001). Physical disturbance can also influence the composition of adjacent soft sediment infaunal communities (Dernie et al., 2003). Recovery of natural oyster populations after hurricanes can vary from shorter (12 months; Livingston et al., 1999) to longer (∼10 year; Munroe et al., 2013) time scales, but information from restored oyster habitats is lacking.
Hurricane Harvey made landfall as a Category 4 storm near Rockport, Texas, United States on 26 August 2017, accompanied by heavy rainfall, storm surges 2–3 m above sea level and catastrophic coastal flooding (Blake and Zelinsky, 2018). The goal of this research is to evaluate dynamics on a newly constructed Crassostrea virginica oyster reef and natural reference reef after the hurricane. Biophysical structure (e.g., oyster density, shell height, sediment grain size), and community composition (abundance of reef-associated epifauna, and nearby infauna) were measured for 18 months. Results were compared with those from prior reef restorations without hurricanes to identify potential differences in early recruitment and growth and help inform management and conservation decisions.
Methods
St. Charles Bay, Texas, United States, is a relatively shallow (less than 2 m) secondary bay within the Mission-Aransas Estuary in the northwestern Gulf of Mexico (Longley, 1994; Figure 1). The estuary is microtidal with low mixing efficiency (< 0.05) and long residence times (∼360 days; Solis and Powell, 1999), with an average annual rainfall of 81 cm y–1 (1941–1999; Tolan, 2007). The surrounding watershed is approximately 530 km2 and is relatively undeveloped (Asquith et al., 1997). Pre-construction samples were collected in May 2017 (macrobenthic infauna) and July 2017 (macrobenthic infauna and sediment grain size). On 28–29 July 2017, ∼1.83 ha of oyster reef complex (∼610 linear m) was constructed in St. Charles Bay (N 28° 9′ 14″ W 96° 58′ 20″) using reclaimed oyster shells. Shells were deployed by barge as a series of seven rectangular mounds 40 m long × 10 m wide × 0.33 m high, oriented parallel to the shoreline along the 1-m depth contour.
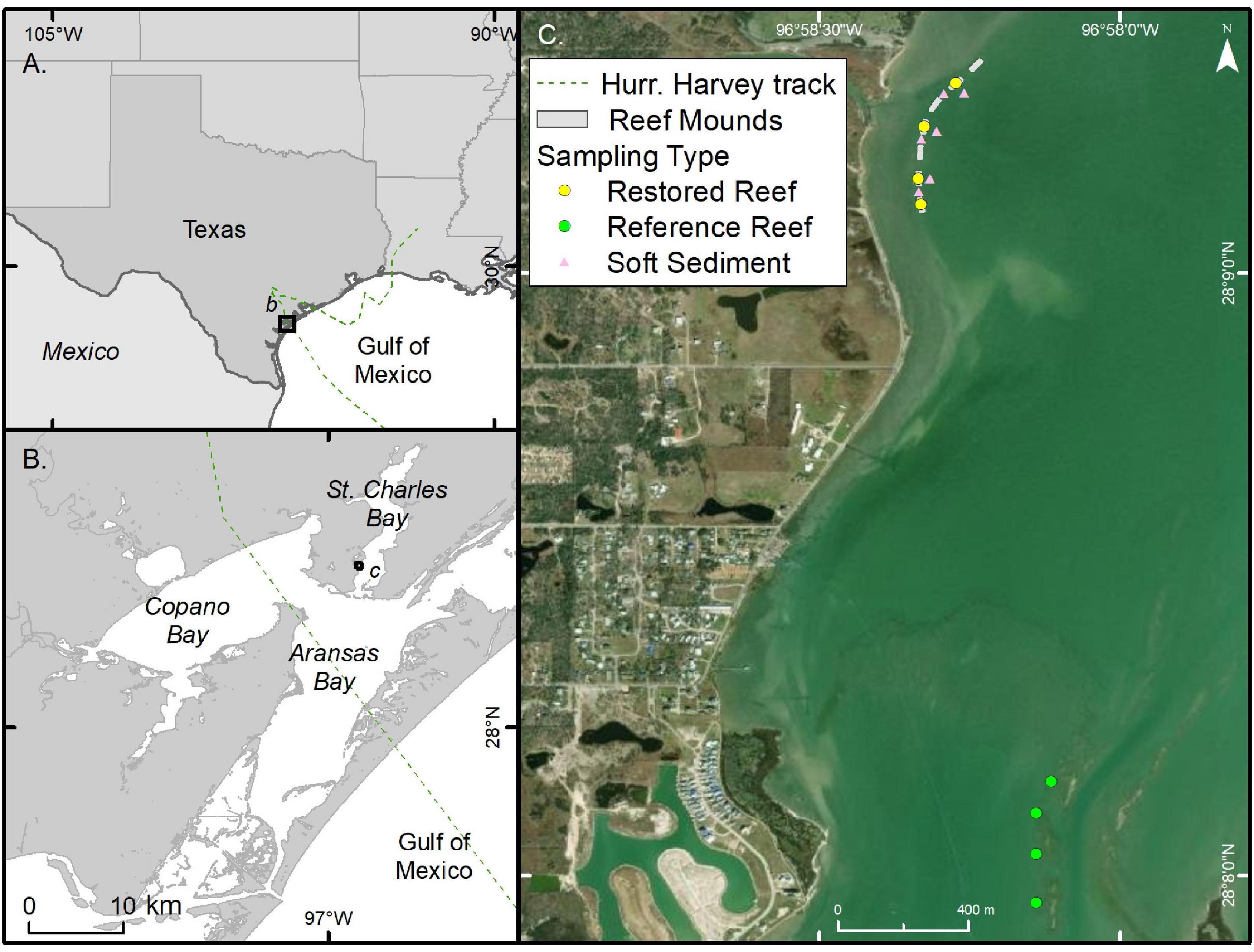
Figure 1. Map of the study area, including Texas coastline and Gulf of Mexico (A), the Mission-Aransas Estuary and St. Charles Bay, TX (B), and sampling locations (C). The Hurricane Harvey track is shown as a dashed line.
Sampling trays of dimensions 45 × 30 cm (0.135 m2) were deployed at four sites within the restored reef and nearby reference reef habitat on 7 and 8 August 2017. Restored reef trays were filled with reclaimed oyster shells from the restored reef, and reference reef trays were filled with cultch from the natural reef. Monitoring of the reef was conducted monthly from September 2017 (3 weeks after the hurricane) to November 2017 and then quarterly to February 2019. During each sampling event, one haphazardly selected sampling tray was removed from each site, and all resident epifauna (> 1 mm) were collected. Oysters were enumerated, and shell heights of 20 live oysters from each tray were measured. Macrobenthic infauna (≥ 0.5 mm) and sediment grain size samples were collected using replicate 35.4 cm2 cylindrical cores to a depth of 10 cm at three adjacent sites (< 5 m from the restored reef) and three distant sites (30 m from the restored reef). The distant and adjacent sites were combined in the results because there were no differences in infauna densities and grain size between the two site types. Temperature and salinity were measured every sampling date using a YSI Pro DSS multiparameter sonde (YSI Incorporated, Yellow Springs, OH, United States). Sediment grain size was analyzed in the laboratory following the methods of Folk (1966). Data management and analysis were performed using SAS 9.4 (SAS Institute Inc., 2013).
Results
Heavy rains over the bay and watershed (∼39 cm; Blake and Zelinsky, 2018) during Hurricane Harvey (26 August 2017) were associated with a sharp decrease in salinities from 24.4 ± 0.2 [mean ± standard error (SE)] on 8 August 2017 to 6.0 (no replication) on 8 September 2017 (Figure 2). Salinities slowly increased to pre-disturbance levels 9 months after the storm, in May 2018. Salinities decreased a second time to 7.5 ± 0.2 in November 2018 following an extended period of high rainfall in September 2018 (∼51 cm; National Estuarine Research Reserve System, 2021). Mean temperature displayed expected seasonal patterns, ranging from 10.3 ± 0.1°C in November 2018 to 29.8 ± 0.2°C in August 2017.
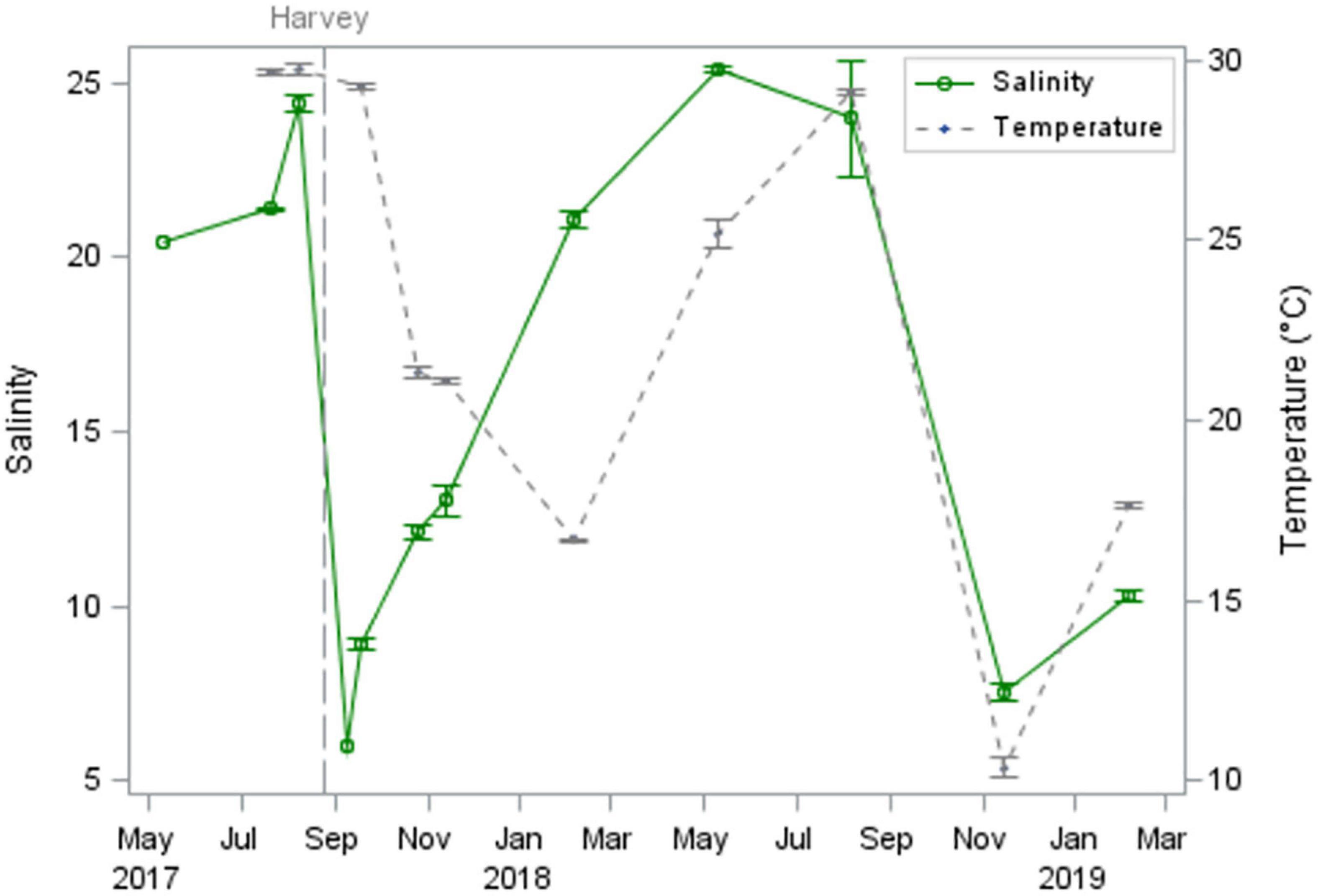
Figure 2. Mean salinity and temperature in St. Charles Bay, TX, measured from May 2017-February 2019. Hurricane Harvey indicated by vertical dashed line.
In the 2 months following the hurricane, spat (≤ 25 mm) densities were relatively high on the restored reef (188 ± 72 m–2 in September 2017 and 174 ± 53 m–2 in October 2017) compared to the reference reef (0 ± 0 m–2 in September 2017 and 49.4 ± 20.4 m–2 in October 2017), which was instead dominated by post-spat size classes, including market size oysters (≥ 76 mm) (Figure 3 and Supplementary Figure 1). Spat densities were low during winter 2017/18 (restored: 26 m–2, reference: 4 m–2). Two relatively strong recruitment pulses occurred at the restored reef in May 2018 (441 ± 207 m–2) and November 2018 (337 ± 166 m–2). Densities of larger, post-spat size classes (≥ 26 mm) increased sharply in the 3 months immediately following the hurricane, from 0 m–2 in September 2017 to 591 ± 43 m–2 in November 2017, before reaching a peak of 1,092 ± 89 m–2 in May 2018 and remaining elevated for the remainder of the study. Densities of oysters on the reference reef were lower and less variable (113–475 m–2) throughout the study period (Figure 3). Oysters on the restored reef grew rapidly during the first 3 months after the hurricane, with average growth rates of 0.29–0.41 mm d–1 from August to September, and September to October 2017 (Supplementary Figure 2). Mean oyster size on the reference reef decreased sharply in the 2 months following the hurricane from 62 ± 2 mm in September 2017 to 42 ± 6 mm in October 2017 (Figure 3). Market size oysters were present on the reference reef throughout the study period and were first observed on the restored reef in May 2018 (Supplementary Figure 1).
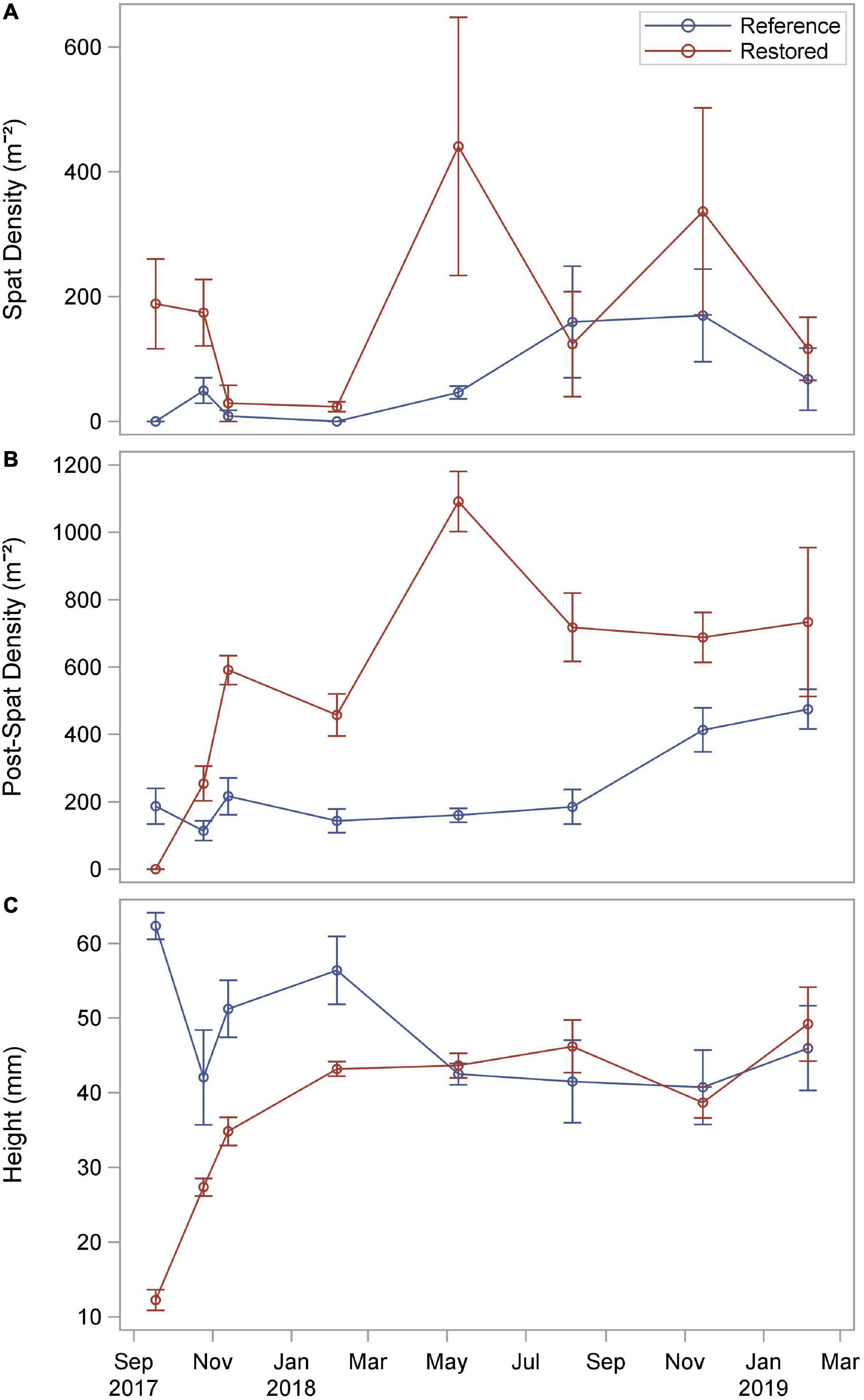
Figure 3. Mean density of oyster spat (≤ 25 mm; A) and larger size classes (≥ 26 mm; B), and oyster size (shell height; C) on the restored and reference reefs from September 2017-February 2019.
Resident epifauna density on the restored reef increased for the first 3 months after the hurricane, from 357 ± 65 m–2 in September 2017 to 635 ± 16 m–2 in November 2017 (Supplementary Figure 3). Epifauna density on the reference reef decreased during this period from 804 ± 163 m–2 to 594 ± 143 m–2. After 6 months, epifauna densities steadily increased on both the restored and reference reefs to a high of 1,983 ± 652 m–2 (restored) and 1,104 ± 215 m–2 (reference) at the end of the study period. Epifauna densities were dominated by the porcelain crab Petrolisthes sp., and the mud crabs Eurypanopeus depressus and Panopeus herbstii (Supplementary Table 1).
In the month immediately following the hurricane, deposition of fine sediments decreased the proportion of sand in the study area from 95% in July 2017 to 89% in September 2017 and increased the proportion of silt and clay from 4% in July 2017 to 10% in September 2017 (Figure 4). Sediment grain size distribution returned to pre-storm levels within 3 months (by November 2017). Infaunal densities (dominated by the polychaetes Mediomastus spp. and Streblospio benedicti, and the tanaid Leptochelia rapax) decreased in the month following the hurricane, from 7,154 ± 687 m–2 in July 2017, to 2,994 ± 343 m–2 in September 2017 (Figure 4 and Supplementary Table 2). Most notably, bivalves (mainly Mulinia lateralis and Mactra fragilis) decreased from 47 ± 26 m–2 in July 2017 to 0 m–2 from September 2017 and remained absent for 6 months following the hurricane until after February 2018. Bivalve densities increased to above pre-storm levels after 9 months (May 2018, 173 ± 52 m–2) and remained present for the remainder of the study (32–126 m–2).
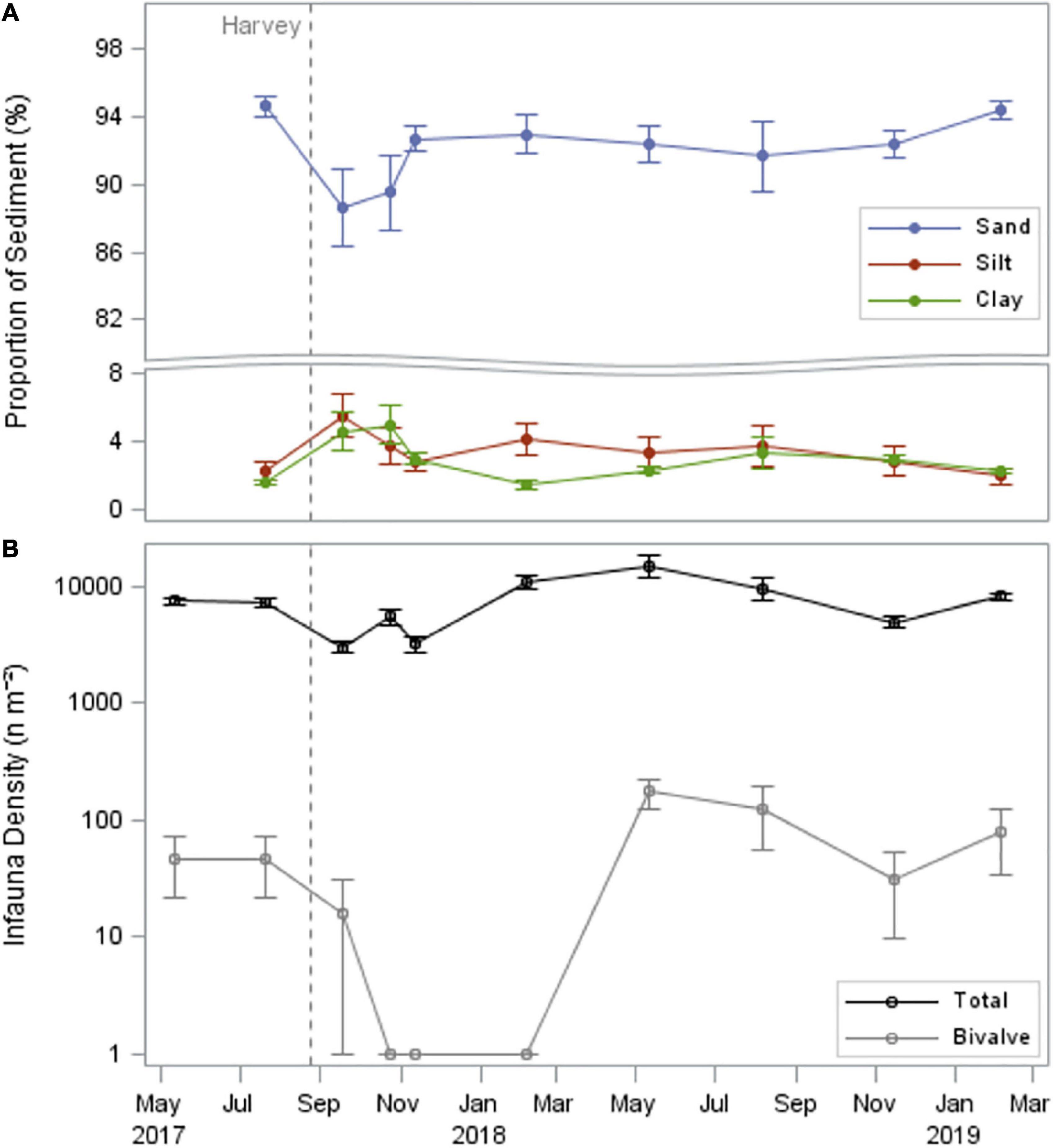
Figure 4. Sediment grain size distribution (%; A; note axis break), and mean density of infauna and bivalves (n m– 2; B; note log-scale). Hurricane Harvey indicated by vertical dashed line.
Discussion
Hurricane-induced effects on estuarine systems often vary and depend on various storm attributes, including hurricane category and rainfall (Mallin and Corbett, 2006). Storms with prolonged rainfall and flooding tend to have greater effects on estuarine ecosystems (Paerl et al., 2001). A sharp decrease in salinity and temporary deposition of fine sediments within the first 3 months after the hurricane corresponded with increases in oyster and epifaunal recruitment on the restored reef. Salinity returned to pre-storm levels within 9 months and sediment composition within 3 months. Because the reef was constructed just weeks before passage of Hurricane Harvey, we did not have historical baseline data to assess the magnitude of fauna recovery or separate the direct effects of the hurricane from the changes due to early recruitment and growth. Regardless, results provide useful information about dynamics of restored and natural oyster reefs after large-scale disturbance that can help assess future recovery and inform conservation and management strategies.
The observed increase in oyster recruitment, density and size on the restored reef during the first 3 months after the storm likely relates to the condition of the shell substrate. Clean shells, like those provided by the newly restored reef, have been shown to support significantly higher oyster recruitment compared to freshly resurfaced buried shells (Hanke et al., 2021) and biofouled shells on natural reefs that obscure the surface to recruitment (Harding et al., 2012). However, spat densities within the first 3 months were below those previously reported for restored reefs (without hurricanes) in Texas estuaries (high of 188 n m–2, current study; 617–1,556 n m–2, George et al., 2015; 231–260 n m–2, Graham et al., 2016; 2,500 n m–2, De Santiago et al., 2019), indicating that oyster recruitment was diminished. Post-settlement survival on the reference reef may have also been reduced through competition (e.g., for space, resources) with existing resident species (Osman et al., 1989), compared to the newly restored reef. A second strong salinity reduction in November 2018 was accompanied by another recruitment pulse on the restored reef but only minor changes in oyster size and density of larger size classes. Relatively lower temperatures in November 2018 (∼10°C vs. August 2017: ∼30°C) may have contributed to this muted response, as acute low salinity is most detrimental to oyster growth and survival at high water temperatures (La Peyre et al., 2013; Rybovich et al., 2016; Marshall et al., 2021). Bivalves in the Mission-Aransas Estuary have been shown to demonstrate temporary reductions in recruitment and density in response to acute summertime flood events, with recovery driven by strong recruitment the following spring (Beseres Pollack et al., 2011).
The restored reef provided immediate habitat benefits to reef resident epifauna. However, epifaunal density within the first 3 months was below that reported for previously restored reefs (without hurricanes) within 2 km of the study area in the current study (∼630 n m–2, current study; ∼1,200 n m–2, George et al., 2015; ∼2,500 n m–2, Rezek et al., 2017) elsewhere in the Mission-Aransas Estuary (∼1,200 n m–2, Blomberg et al., 2018) and in the Lavaca-Colorado Estuary, Texas (∼1,500 n m–2, De Santiago et al., 2019), indicating that initial recruitment rates were constrained. Nevertheless, epifaunal densities on the restored reef 12–18 months after the hurricane met or exceeded those from previously restored reefs (without hurricanes) in the Mission-Aransas Estuary (∼1,900 n m–2 vs. ∼1,000 n m–2, Graham et al., 2016; 2,000 n m–2, Rezek et al., 2017) and Lavaca-Colorado Estuary, Texas (∼1,500 n m–2, De Santiago et al., 2019), indicating the capacity for similar habitat provision within a short period of time. Increases in epifaunal density on the restored reef were likely facilitated by the enhanced biophysical structure and habitat complexity (Tolley and Volety, 2005; Humphries et al., 2011; George et al., 2015; Humphries and La Peyre, 2015). The high vertical relief of the restored reef likely minimized sediment deposition and reef burial and positively influenced survival, abundance, and size of oysters and reef fauna (Lenihan and Peterson, 1998; Lenihan, 1999; Lenihan et al., 1999; Taylor and Bushek, 2008; Powers et al., 2009; Schulte et al., 2009; Lipcius et al., 2015; Lipcius and Burke, 2018). The muted response of epifauna to the second salinity reduction 15 months after the hurricane may be related to presence of later successional stages (Cranfield et al., 2004).
An immediate and strong decline in infaunal densities coincided with a sharp decrease in salinity after the hurricane. Based on abundance of infauna, recovery from the effects of the hurricane appears rapid, consistent with previous studies (Boesch et al., 1976; Mallin et al., 1999), with a return to pre-storm densities occurring within 2 months and then stabilizing after 6 months. Bivalve recovery was delayed, with strong recruitment and return to pre-storm densities not occurring until the following spring. Infaunal bivalves, and Mulinia lateralis in particular, have highly variable life cycles that correspond with changes in salinity, with recovery facilitated by their ability to colonize disturbed areas and grow rapidly (Calabrese, 1969; Boesch et al., 1976; Montagna and Kalke, 1995). Results corroborate previous work demonstrating sensitivity of benthic infauna to hurricane-induced changes in salinity, with post-storm recovery driven by recruitment of mollusks (Patrick et al., 2020). A lack of synergy among multiple stressors (e.g., salinity, temperature; Côté et al., 2016; Hewitt et al., 2016; Carrier-Belleau et al., 2021) during the second salinity drop in November 2018 may have averted significant reductions in infauna and bivalves.
Conclusion
Despite experiencing a large-scale hurricane disturbance and acute decrease in salinity, changes in physical and biological complexity on a newly restored oyster reef were generally limited to 1–3 months, although oyster and epifaunal densities were generally below those measured on restored reefs without hurricanes. The high vertical relief of the restored reef likely minimized sediment deposition and facilitated survival of oysters and reef fauna (Lipcius et al., 2015 and references therein). Criteria for oyster reef restoration, including increases in physical (e.g., oyster shell height and density) and biological complexity (e.g., faunal abundance and biomass), were met within 12–18 months (Coen and Luckenbach, 2000; Peterson et al., 2003; Powers et al., 2009). Because the reef was constructed just weeks before passage of Hurricane Harvey, pre-disturbance data were not available to assess the magnitude of reef recovery to pre-disturbance levels or separate the direct effects of the hurricane from the dynamics of early recruitment and growth. Nevertheless, increasing our understanding of the dynamics of restored and natural oyster reefs after large-scale disturbance can help in assessing recovery and informing management and conservation decisions.
Data Availability Statement
The datasets presented in this study can be found in online repositories. The names of the repository/repositories and accession number(s) can be found below: Martinez et al. (2021, https://doi.org/10.7266/4M7E26BH).
Ethics Statement
The animal study was reviewed and approved by the Institutional Animal Care and Use Committee of Texas A&M University-Corpus Christi (Protocol Number 10–17).
Author Contributions
MM, TP, and NB conducted the field sampling. MM processed samples in the laboratory. MM and TP analyzed and visualized the data. JB wrote the first draft of this manuscript and obtained the funding. JB and TP revised the manuscript. All authors conceived and designed the study.
Funding
This project was made possible with funding from the National Fish and Wildlife Foundation and the Building Conservation Trust Program of the Coastal Conservation Association. Partial support for this publication was made possible by the National Oceanic and Atmospheric Administration, Office of Education Educational Partnership Program award NA16SEC4810009. Its contents were solely the responsibility of the award recipient and do not necessarily represent the official views of the United States Department of Commerce, National Oceanic and Atmospheric Administration.
Conflict of Interest
The authors declare that the research was conducted in the absence of any commercial or financial relationships that could be construed as a potential conflict of interest.
Publisher’s Note
All claims expressed in this article are solely those of the authors and do not necessarily represent those of their affiliated organizations, or those of the publisher, the editors and the reviewers. Any product that may be evaluated in this article, or claim that may be made by its manufacturer, is not guaranteed or endorsed by the publisher.
Acknowledgments
We would like to thank members of the Coastal Conservation and Restoration Ecology Lab at the Harte Research Institute of Gulf of Mexico Studies, Texas A&M University-Corpus Christi for their hard work on this project. We thank Romuald Lipcius and two reviewers for their helpful remarks that served to improve the quality of this manuscript. We are also grateful to Paul Montagna and Kim Withers for providing useful comments and suggestions on an earlier version of this manuscript.
Supplementary Material
The Supplementary Material for this article can be found online at: https://www.frontiersin.org/articles/10.3389/fevo.2022.791739/full#supplementary-material
References
Airoldi, L., Balata, D., and Beck, M. W. (2008). The Gray Zone: relationships between habitat loss and marine diversity and their applications in conservation. J. Exp. Mar. Biol. Ecol. 366, 8–15. doi: 10.1016/j.jembe.2008.07.034
Asquith, W. H., Mosier, J. G., and Bush, P. W. (1997). Status, Trends, and Changes in Freshwater Inflows to Bay Systems in the CCBNEP Study Area. Corpus Christi: Corpus Christi Bay National Estuary Program.
Beck, M. W., Brumbaugh, R. D., Airoldi, L., Carranza, A., Coen, L. D., Crawford, C., et al. (2011). Oyster Reefs at Risk and Recommendations for Conservation, Restoration, and Management. Bioscience 61, 107–116. doi: 10.1525/bio.2011.61.2.5
Berrigan, M. E. (1990). Biological and economical assessment of an oyster resource development project in Apalachicola Bay. Florida. J. Shellfish Res. 9, 149–158.
Beseres Pollack, J., Kim, H.-C., Morgan, E. K., and Montagna, P. A. (2011). Role of flood disturbance in natural oyster (Crassostrea virginica) population maintenance in an estuary in South Texas. USA. Estuar. Coast. 34, 187–197. doi: 10.1007/s12237-010-9338-6
Beseres Pollack, J., Yoskowitz, D., Kim, H.-C., and Montagna, P. A. (2013). Role and value of nitrogen regulation provided by oysters (Crassostrea virginica) in the Mission-Aransas Estuary. PLoS One 8:e65314. doi: 10.1371/journal.pone.0065314
Blake, R. S., and Zelinsky, D. A. (2018). Hurricane Harvey. NOAA National Hurricane Center Tropical Cyclone Report. AL092017. Available Online at https://www.nhc.noaa.gov/data/tcr/AL092017_Harvey.pdf (accessed September 22, 2021).
Blomberg, B. N., Palmer, T. A., Montagna, P. A., and Beseres Pollack, J. (2018). Habitat assessment of a restored oyster reef in South Texas. Ecol. Eng. 122, 48–61. doi: 10.1016/j.ecoleng.2018.07.012
Boesch, D. F., Diaz, R. J., and Virnstein, R. W. (1976). Effects of Tropical Storm Agnes on soft-bottom macrobenthic communities of the James and York estuaries and the lower Chesapeake Bay. Ches. Sci. 17, 246–259.
Boesch, D. F., and Turner, R. E. (1984). Dependence of fishery species on salt marshes: the role of food and refuge. Estuaries 7, 460–468.
Breitburg, D. L., Palmer, M. A., and Loher, T. (1995). Larval distributions and the spatial patterns of settlement of an oyster reef fish: responses to flow and structure. Mar. Ecol. Prog. Ser. 125, 45–60. doi: 10.3354/meps125045
Calabrese, A. (1969). Individual and combined effects of salinity and temperature on embryos and larvae of the coot clam, Mulina lateralis (Say). Biol. Bull. 137, 417–428.
Carrier-Belleau, C., Drolet, D., McKindsey, C. W., and Archambault, P. (2021). Environmental stressors, complex interactions and marine benthic communities’ responses. Sci. Rep. 11:4194. doi: 10.1038/s41598-021-83533-1
Coen, L. D., and Luckenbach, M. W. (2000). Developing success criteria and goals for evaluating oyster reef restoration: ecological function or resource exploitation? Ecol. Eng. 15, 323–343. doi: 10.1016/S0925-8574(00)00084-7
Côté, I., Darling, E., and Brown, C. (2016). Interactions among ecosystem stressors and their importance in conservation. Proc. R. Soc. Lond. B Biol. Sci. 283:20152592. doi: 10.1098/rspb.2015.2592
Cranfield, H. J., Rowden, A. A., Smith, D. J., Gordon, D. P., and Michael, K. P. (2004). Macrofaunal assemblages of benthic habitat of different complexity and the proposition of a model of biogenic reef habitat regeneration in Foveaux Straight, New Zealand. J. Sea Res. 52, 109–125. doi: 10.1016/j.seares.2003.12.003
Dayton, P. K. (1971). Competition, disturbance and community organization: the provision and subsequent utilization of space in a rocky intertidal community. Ecol. Monogr. 41, 351–389. doi: 10.2307/1948498
Dernie, K. M., Kaiser, M. J., and Warwick, R. (2003). Recovery rates of benthic communities following physical disturbance. J. Anim. Ecol. 72, 1043–1056. doi: 10.1046/j.1365-2656.2003.00775.x
De Santiago, K., Palmer, T. A., Dumesnil, M., and Pollack, J. B. (2019). Rapid development of a restored oyster reef facilitates habitat provision for estuarine fauna. Restor. Ecol. 27, 870–880. doi: 10.1111/rec.12921
Du, J., Park, K., Jensen, C., Dellapenna, T. M., Zhang, W. G., and Shi, Y. (2021). Massive oyster kill in Galveston Bay caused by prolonged low-salinity exposure after Hurricane Harvey. Sci. Total Environ. 774:145132. doi: 10.1016/j.scitotenv.2021.145132
George, L. M., De Santiago, K., Palmer, T. A., and Beseres Pollack, J. (2015). Oyster reef restoration: effect of alternative substrates on oyster recruitment and nekton habitat use. J. Coast. Conserv. 19, 2111–2116. doi: 10.1007/s11852-014-0351-y
Grabowski, J. H., Brumbaugh, R. D., Conrad, R. F., Keeler, A. G., Opaluch, J. J., Peterson, C., et al. (2012). Economic valuation of ecosystem services provided by oyster reefs. Bioscience 62, 900–909. doi: 10.1525/bio.2012.62.10.10
Graham, P., Palmer, T. A., and Beseres Pollack, J. (2016). Oyster reef restoration: substrate suitability may depend on specific restoration goals. Restor. Ecol. 25, 459–470. doi: 10.1111/rec.12449
Gutierrez, J. L., Jones, C. G., Strayer, D. L., and Iribarne, O. O. (2003). Mollusks as ecosystem engineers: the role of shell production in aquatic habitats. Oikos 101, 79–90. doi: 10.1034/j.1600-0706.2003.12322.x
Hanke, M. H., Bobby, N., and Sanchez, R. (2021). Can relic shells be an effective settlement substrate for oyster reef restoration? Restor. Ecol. 29:e13371. doi: 10.1111/rec.13371
Harding, J. M., and Mann, R. (2001). Oyster reefs as fish habitat: opportunistic use of restored reefs by transient fishes. J. Shellfish Res. 3, 951–959.
Harding, J. M., Southworth, M. J., Mann, R., and Wesson, J. A. (2012). Comparison of Crassostrea virginica Gmelin (eastern oyster) recruitment on constructed reefs and adjacent natural oyster bars over decadal time scales. Northeast. Nat. 19, 627–646.
Hewitt, J. E., Ellis, J. I., and Thrush, S. F. (2016). Multiple stressors, nonlinear effects and the implications of climate change impacts on marine coastal ecosystems. Glob. Chang Biol. 22, 2665–2675. doi: 10.1111/gcb.13176
Holling, C. S. (1973). Resilience and stability of ecological systems. Annu. Rev. Ecol. System. 4, 1–23.
Humphries, A. T., La Peyre, M. K., Kimball, M. E., and Rozas, L. P. (2011). Testing the effect of habitat structure and complexity on nekton assemblages using experimental oyster reefs. J. Exp. Mar. Bio. Ecol. 409, 172–179. doi: 10.1016/j.jembe.2011.08.017
Humphries, A. T., and La Peyre, M. K. (2015). Oyster reef restoration supports increased nekton biomass and potential commercial fishery value. PeerJ 3:e1111. doi: 10.7717/peerj.1111
Jackson, J. B. C., Kirby, M. X., Berger, W. H., Bjorndal, K. A., Botsford, L. W., Bourque, B. J., et al. (2001). Historical overfishing and the recent collapse of coastal ecosystems. Science 293, 629–638. doi: 10.1126/science.1059199
Jackson, J. B. C. (2008). Ecological extinction and evolution in the brave new ocean. Proc. Natl. Acad. Sci. U S A. 105, 11458–11465. doi: 10.1073/pnas.0802812105
Kirby, M. X. (2004). Fishing down the coast: historical expansion and collapse of oyster fisheries along continental margins. Proc. Natl. Acad. Sci. U S A. 101, 13096–13099. doi: 10.1073/pnas.0405150101
La Peyre, M. K., Eberline, B. S., Soniat, T. M., and La Peyre, J. F. (2013). Differences in extreme low salinity timing and duration differentially affect eastern oyster (Crassostrea virginica) size class growth and mortality in Breton Sound. LA. Estuar. Coast. Shelf Sci. 135, 146–157. doi: 10.1016/j.ecss.2013.10.001
Lenihan, H. S. (1999). Physical–biological coupling on oyster reefs: how habitat structure influences individual performance. Ecol. Monogr. 69, 251–275.
Lenihan, H. S., Micheli, F., Shelton, S. W., and Peterson, C. H. (1999). The influence of multiple environmental stressors on susceptibility to parasites: an experimental determination with oysters. Limnol. Oceanogr. 44, 910–924.
Lenihan, H. S., and Peterson, C. H. (1998). How habitat degradation through fishery disturbance enhances impacts of hypoxia on oyster reefs. Ecol. Appl. 11, 128–140.
Levin, L. A. (1984). Life history and dispersal patterns in a dense infaunal polychaete assemblage: community structure and response to disturbance. Ecology 65, 1185–1200.
Lipcius, R. N., and Burke, R. P. (2018). Successful recruitment, survival and long-term persistence of eastern oyster and hooked mussel on a subtidal, artificial restoration reef system in Chesapeake Bay. PLoS One 13:e0204329. doi: 10.1371/journal.pone.0204329
Lipcius, R., Burke, R., McCulloch, D., Schreiber, S., Schulte, D., Seitz, R., et al. (2015). Overcoming restoration paradigms: value of the historical record and metapopulation dynamics in native oyster restoration. Front. Mar. Sci. 2:65. doi: 10.3389/fmars.2015.00065
Livingston, R. J., Howell, R. L., Niu, X., Lewis, F. G., and Woodsum, G. C. (1999). Recovery of oyster reefs in a Gulf estuary following disturbance by two hurricanes. Bull. Mar. Sci. 64, 465–483.
Longley, W. L. (1994). Freshwater Inflows to Texas Bays and Estuaries: Ecological Relationships and Methods for Determination of Needs. Texas: Water Development Board and Texas Parks and Wildlife Department.
Mallin, M. A., Posey, M. H., Shank, G. C., McIver, M. R., Ensign, S. H., and Alphin, T. D. (1999). Hurricane effects on water quality and benthos in the Cape Fear watershed: natural and anthropogenic impacts. Ecol. Appl. 9, 350–362.
Mallin, M. A., and Corbett, C. A. (2006). How hurricane attributes determine the extent of environmental effects: multiple hurricanes and different coastal systems. Estuaries Coast. 29, 1046–1061. doi: 10.1007/BF02798667
Marshall, D. A., Casas, S. M., Walton, W. C., Rikard, F. S., Palmer, T. A., Breaux, N., et al. (2021). Divergence in salinity tolerance of northern Gulf of Mexico eastern oysters under field and laboratory exposure. Conserv. Physiol. 9:coab065. doi: 10.1093/conphys/coab065
Martinez, M. J., Palmer, T.A., Breaux, N., and Beseres Pollack, J. (2021). Monitoring of Oyster and Epifauna Populations at a Constructed Oyster Reef (Big Tree Reef), St Charles Bay, Texas from 2017 to 2019. Christi, TX: Texas A&M University-Corpus Google Scholar
Montagna, P. A., and Kalke, R. D. (1995). Ecology of infaunal Mollusca in south Texas estuaries.Am. Malacol. Bull. 15, 307–326.
Munroe, D., Tabatabai, A., Burt, I., Bushek, D., Powell, E. N., and Wilkin, J. (2013). Oyster mortality in Delaware Bay: impacts and recovery from Hurricane Irene and Tropical Storm Lee. Estuar. Coast. Shelf Sci. 135, 209–219. doi: 10.1016/j.ecss.2013.10.011
National Estuarine Research Reserve System (2021). System-wide Monitoring Program. Data accessed from the NOAA NERRS Centralized Data Management. Available Online at: http://www.nerrsdata.org [accessed on Dec 10, 2021]
Osman, R. W., Whitlatch, R. B., and Zajac, R. N. (1989). Effects of resident species on recruitment into a community: larval settlement versus post-settlement mortality in the oyster Crassostrea virginica. Mar. Ecol. Prog. Ser. 54, 61–73. doi: 10.3354/meps054061
Paerl, H. W., Bales, J. D., Ausley, L. W., Buzzelli, C. P., Crowder, L. B., Eby, L. A., et al. (2001). Ecosystem impacts of three sequential hurricanes (Dennis, Floyd, and Irene) on the United States’ largest lagoonal estuary, Pamlico Sound, NC. Proc. Natl. Acad. Sci. U S A. 98, 5655–5660. doi: 10.1073/pnas.101097398
Patrick, C. J., Yeager, L., Armitage, A. R., Carvallo, F., Congdon, V. M., Dunton, K. H., et al. (2020). A system level analysis of coastal ecosystem responses to hurricane impacts. Estuaries Coast. 43, 943–959. doi: 10.1007/s12237-019-00690-3
Perret, W. S., Dugas, R., Roussel, J., Wilson, C., and Supan, J. (1999). “Oyster habitat restoration: a response to Hurricane Andrew,” in Oyster Reef Habitat Restoration: A Synopsis and Synthesis of Approaches, eds M. Luckenbach, R. Mann, and J. Wesson (Virginia: Institute of Marine Science Press), 93–100.
Peterson, C., Grabowski, J., and Powers, S. (2003). Estimated enhancement of fish production resulting from restoring oyster reef habitat: quantitative valuation. Mar. Ecol. Prog. Ser. 264, 249–264. doi: 10.3354/meps264249
Powers, S. P., Peterson, C. H., Grabowski, J. H., and Lenihan, H. S. (2009). Success of constructed oyster reefs in no-harvest sanctuaries: implications for restoration. Mar. Ecol. Prog. Ser. 389, 159–170. doi: 10.3354/meps08164
Rezek, R. J., Lebreton, B., Roark, E. B., Palmer, T. A., and Beseres Pollack, J. (2017). How does a restored oyster reef develop? An assessment based on stable isotopes and community metrics. Mar. Biol. 164:54. doi: 10.1007/s00227-017-3084-2
Rothschild, B. J., Ault, J. S., Goulletquer, P., and Heral, M. (1994). Decline of the Chesapeake Bay oyster population: a century of habitat destruction and overfishing. Mar. Ecol. Prog. Ser. 111, 29–39. doi: 10.3354/meps111029
Rybovich, M., La Peyre, M. K., Hall, S. G., and La Peyre, J. F. (2016). Increased temperatures combined with lowered salinities differentially impact oyster size class growth and mortality. J. Shellfish Res. 35, 101–113. doi: 10.2983/035.035.0112
Schulte, D. M., Burke, R. P., and Lipcius, R. N. (2009). Unprecedented restoration of a native oyster metapopulation. Science 325, 1124–1128.
Solis, R. S., and Powell, G. L. (1999). “Hydrography, mixing characteristics, and residence times of Gulf of Mexico estuaries,” in Biogeochemistry of Gulf of Mexico Estuaries, eds T. S. Bianchi, J. R. Pennock, and R. R. Twilley (New York, NY: John Wiley & Sons), 29–61.
Sousa, W. P. (1984). The role of disturbance in natural communities. Ann. Rev. Ecol. Syst. 15, 353–392.
Taylor, J., and Bushek, D. (2008). Intertidal oyster reefs can persist and function in a temperate North American Atlantic estuary. Mar. Ecol. Prog. Ser. 361, 301–306.
Tolan, J. M. (2007). El Nino-Southern Oscillation impacts translated to the watershed scale: estuarine salinity patterns along the Texas Gulf Coast, 1982 to 2004. Estuar. Coast. Shelf Sci. 72, 247–260. doi: 10.1016/j.ecss.2006.10.018
Tolley, S. G., and Volety, A. K. (2005). The role of oysters in habitat use of oyster reefs by resident fishes and decapod crustaceans. J. Shellfish Res. 24, 1007–1012. doi: 10.2983/0730-8000200524
Walker, L. M., Montagna, P. A., Hu, X., and Wetz, M. S. (2021). Timescales and Magnitude of Water Quality Change in Three Texas Estuaries Induced by Passage of Hurricane Harvey. Estuar. Coast. 44, 960–971. doi: 10.1007/s12237-020-00846-6
Wetz, M. S., and Yoskowitz, D. W. (2013). An ‘extreme’ future for estuaries? Mar. Pollut. Bull. 69, 7–18. doi: 10.1016/j.marpolbul.2013.01.020
Keywords: Crassostrea virginica, extreme climate event, Gulf of Mexico, habitat, salinity, Texas
Citation: Martinez MJ, Palmer TA, Breaux NJ and Beseres Pollack J (2022) Dynamics of Restored and Natural Oyster Reefs After a Hurricane. Front. Ecol. Evol. 10:791739. doi: 10.3389/fevo.2022.791739
Received: 08 October 2021; Accepted: 04 January 2022;
Published: 26 January 2022.
Edited by:
Romuald Lipcius, College of William & Mary, United StatesReviewed by:
Benny K. K. Chan, Academia Sinica, TaiwanGulnihal Ozbay, Delaware State University, United States
Copyright © 2022 Martinez, Palmer, Breaux and Beseres Pollack. This is an open-access article distributed under the terms of the Creative Commons Attribution License (CC BY). The use, distribution or reproduction in other forums is permitted, provided the original author(s) and the copyright owner(s) are credited and that the original publication in this journal is cited, in accordance with accepted academic practice. No use, distribution or reproduction is permitted which does not comply with these terms.
*Correspondence: Jennifer Beseres Pollack, Jennifer.pollack@tamucc.edu
†Present address: Meghan J. Martinez, California State Coastal Conservancy, Oakland, CA, United States