- 1Department of Evolutionary Neuroethology, Max Planck Institute for Chemical Ecology, Jena, Germany
- 2Max Planck Center Next Generation Insect Chemical Ecology, Jena, Germany
- 3Department of Plant Protection Biology, Swedish University of Agricultural Sciences, Alnarp, Sweden
- 4Max Planck Center Next Generation Insect Chemical Ecology, Alnarp, Sweden
- 5Department of Biology, Lund University, Lund, Sweden
- 6Max Planck Center Next Generation Insect Chemical Ecology, Lund, Sweden
- 7Disease Vector Group, Chemical Ecology, Department of Plant Protection Biology, Swedish University of Agricultural Sciences, Alnarp, Sweden
- 8Department of Molecular Sciences, Swedish University of Agricultural Sciences, Uppsala, Sweden
The planet is presently undergoing dramatic changes caused by human activities. We are living in the era of the Anthropocene, where our activities directly affect all living organisms on Earth. Insects constitute a major part of the world’s biodiversity and currently, we see dwindling insect biomass but also outbreaks of certain populations. Most insects rely on chemical communication to locate food, mates, and suitable oviposition sites, but also to avoid enemies and detrimental microbes. Emissions of, e.g., CO2, NOx, and ozone can all affect the chemical communication channel, as can a rising temperature. Here, we present a review of the present state of the art in the context of anthropogenic impact on insect chemical communication. We concentrate on present knowledge regarding fruit flies, mosquitoes, moths, and bark beetles, as well as presenting our views on future developments and needs in this emerging field of research. We include insights from chemical, physiological, ethological, and ecological directions and we briefly present a new international research project, the Max Planck Centre for Next Generation Insect Chemical Ecology (nGICE), launched to further increase our understanding of the impact of human activities on insect olfaction and chemical communication.
Introduction
Insects comprise a significant part of the world’s biodiversity while affecting human life and health in both positive and negative directions. Human activities, at the same time, have been shown to have a strong detrimental effect on many insect populations [Seibold et al., 2019, but see also Crossley et al. (2020) and van Klink et al. (2020), which both found significant human effects to be either limited to terrestrial insect communities or to be absent]. As insects are, to a very large extent, odour-driven creatures, it is of critical importance to investigate how their olfactory-driven behaviours will be affected by the challenges imposed in the Anthropocene era. Climatic changes and atmospheric pollution have already begun to affect natural environmental odours, and concomitantly insect odour-mediated behaviours associated with the utilisation of their essential resources. Such altered insect behaviour may change ecological interactions, alter biodiversity, and hence have significant effects on natural communities. The abilities of insects, together with their microbiomes, to spread geographically and adapt to new niches are likewise affected by ongoing global, regional, and local changes. Additionally, in an era in which sustainable development is a necessity, altered insect behaviour presents new challenges (and opportunities) for agriculture and forestry, affecting crop protection and ecosystem services, as well as for human health as a result of vector-transmitted diseases, including malaria, dengue, and hundreds of arbovirus-induced encephalitic illnesses worldwide. Each of these aspects are dependent on insect olfaction, and therefore sensitive to variations in the natural odour space.
In the coming decades, we will face rising temperatures with risk for increasing frequency of weather extremes, e.g., droughts, fires, and floods, as well as increasing levels of ambient CO2 and pollutants, including ozone and nitric oxides (NOx). Recent findings suggest that pollutants interfere with insects on different levels (Figure 1). They can, e.g., oxidise odours emitted from plants, and in doing so, can drastically reduce effective pollination (Fuentes et al., 2013; Farre-Armengol et al., 2016; Cook et al., 2020). Intraspecific insect communication via sex and aggregation pheromones are also affected by air pollutants, potentially diminishing insect reproductive success, as well as impacting on the ability of natural enemies to locate their prey [reviewed in McFrederick et al. (2008)]. Moreover, ozone directly affects the production and emission of volatiles from plants through oxidative stress, both qualitatively and quantitatively, in combination with other stressors, both biotic and abiotic (e.g., warm temperatures and drought) (Blande et al., 2014). Rising temperatures also have direct effects on both chemical and physiological processes involved in chemical communication. However, the extent of the effects of all of these Anthropogenic factors on odour landscapes and their detection by insects remains to a large degree elusive.
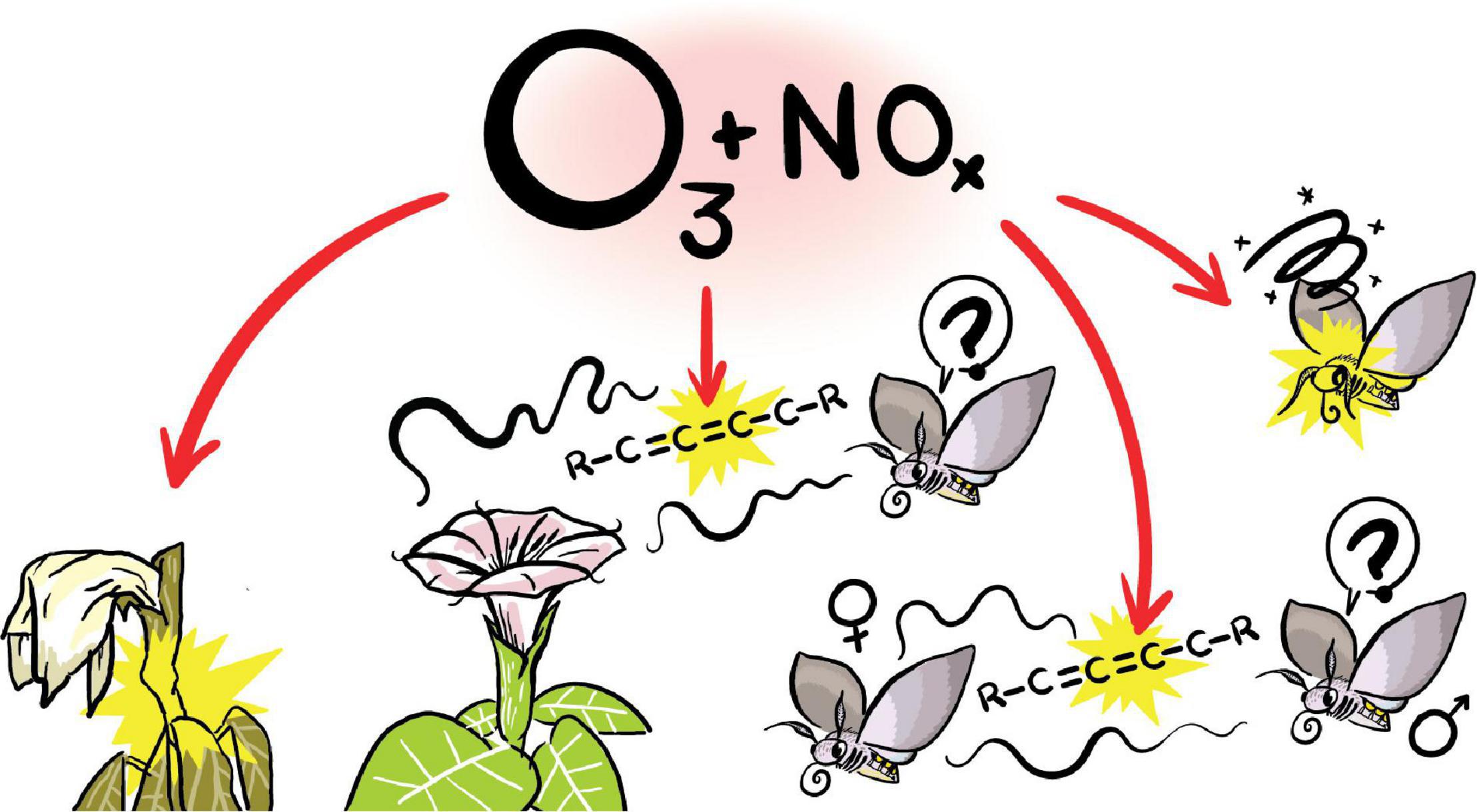
Figure 1. Potential effects of pollutants like ozone and nitric oxides on plant and insects. Pollutants can cause direct oxidative physiological stress in plants and insects and can degrade volatiles involved in plant-insect and insect-insect communication. (Figure courtesy of Diego Galagovsky; Sci-flies.com.)
Since 2020, a German-Swedish collaboration in the form of a Max Planck Center (nGICE) between the Max Planck Institute for Chemical Ecology in Jena, Lund University, and the Swedish University of Agricultural Sciences in Alnarp, focuses on elucidating the effects of the above-mentioned factors on insect chemical communication using mosquitoes, fruit flies, bark beetles, and moths as model organisms. Here, we aim to review the present state-of-the-art in this quite young field of research.
The Chemistry
Following the industrial revolution—apart from increasing levels of CO2—there has been a dramatic increase in the tropospheric load of atmospheric pollutants, including the oxidant species nitric oxides (NOx) and ozone (Olivier et al., 1998; Hauglustaine and Brasseur, 2001). Ozone, for example, has increased from approximately 10 ppbV or less in preindustrial times (Hauglustaine and Brasseur, 2001) to current averages in North America of 20–45 ppbV (Vingarzan, 2004), with spikes as high as 120 ppbV during summertime ozone events (Fiore et al., 2002; Vingarzan, 2004). Both, NOx and ozone are highly reactive oxidants that can react with the carbon-carbon double bonds commonly found in volatiles emitted from food sources (Atkinson and Arey, 2003; Baker et al., 2004) and pheromones (Arndt, 1995). As these volatiles differ in their chemical structures, and thus react differently from each other with a given oxidant, oxidant pollution leads to both decreases in some key compounds and changes in the relative concentrations of individual compounds in a blend (McFrederick et al., 2008; Lusebrink et al., 2015; Farre-Armengol et al., 2016). Moreover, when oxidants react with a volatile, these induce a series of reactions that can lead to the production of secondary compounds. Secondary compounds may themselves be long-lived volatiles, many of which are compounds that share little similarity to the parent volatiles, e.g., formaldehyde, acetone, and carbon monoxide (Lee et al., 2006; McFrederick et al., 2008). As blend composition has been shown to be crucial both in foraging insects (Bruce et al., 2005) and in insects following pheromone plumes (Linn and Roelofs, 1985; Linn et al., 1987), selective oxidation by pollutants may potentially affect both insect feeding, mating, and oviposition behaviour.
Apart from the increase of atmospheric pollutants, global warming is perhaps the most noticeable characteristic of the Anthropocene. As discussed for atmospheric pollutants, increasing temperature could have severe effects on blend composition and, hence, on odour-guided behaviour in insects: the amount of each compound in the blend is a function of its volatility. With increasing temperature, highly volatile compounds might evaporate faster than those of low volatility, resulting in a novel blend with which the insect has not evolved. While the effects of pollutants on flower location (Farre-Armengol et al., 2016; Cook et al., 2020) and mating (Arndt, 1995) have been investigated, the effect of temperature on insect olfactory-driven behaviour are less well-studied, and have until recently, mainly focussed on temperature-related behavioural changes of males following pheromone plumes (see below).
How Does Exposure to Increased Levels of CO2, NOx, Ozone, and Increased Temperatures Affect Chemosensory Gene Family Expression?
Human-derived emissions of pollutants into the atmosphere, as well as altered temperatures might not only affect insect behaviour by changing the odour profiles of food or oviposition sites, but also function via direct effects on the insect’s olfactory system. Electroantennogram experiments with bumblebees, as well as parasitoid wasps revealed changes in sensitivity after ozone exposure to a number of synthetic compounds (Vanderplanck et al., 2021). While it has been shown for plants (Sharma and Davis, 1994) and insects (Deska, 2020) that ozone and other oxidants induce the expression of stress-related genes, and by that the production of antioxidants, the direct effects of pollutants on genes expressed in the insect olfactory system are not well-understood. The same holds true for the effects of increasing temperature. Like pollutants, increased temperatures can induce the expression of stress-related genes in insects (Chen et al., 2019), which may have direct effects on the olfactory system. However, most insects have developed species-specific temperature preferences that match the temperature range to which they are physiologically best adapted. As a result, global warming could open up new habitats for heat-tolerant species, while relatives that prefer lower temperatures might disappear locally.
To better understand potential effects at the molecular level of olfaction, future studies should target antennal transcriptomes of insects exposed or reared under various ecologically relevant CO2, NOx, and ozone levels with a focus on target genes in the antennae, e.g., odorant receptors (OR), gustatory receptors (GR; which include the CO2 receptors), and ionotropic receptors (IR), as well as odorant binding proteins (OBP). New techniques, such as the Nanostring nCounter method (Nanostring Technologies Inc., Washington, United States), which represents a robust, sensitive, and highly accurate assay that enables high-throughput analysis to profile gene expression levels (Ammeux et al., 2016), might allow a good overview regarding which genes become differentially expressed when the insect is facing pollution or heat stress.
Next Generation Studies of Olfactory Receptors
Insect olfactory systems have evolved to allow insects to detect and discriminate amongst an enormous variety of odorants. Different insect species have converged on a common strategy in which odorant identity is detected through combinations of different families of olfactory receptors.
Odorant receptors are the primary olfactory receptors involved in resource discrimination by insects, i.e., their ability to assess the quality of a suitable resource by the odours emitted. Insect ORs form a unique class of odorant-gated ion channels, normally consisting of two types of transmembrane proteins forming a heteromeric tetramer complex consisting of a conserved co-receptor (Orco) subunit and a highly divergent OR subunit that contains the odorant-binding site and confers chemical sensitivity to the heteromeric receptor complex (Sato et al., 2008; Wicher et al., 2008). Most insect species express one Orco and a distinct complement of tuning ORs (Missbach et al., 2014), ranging from four in the damselfly (Ioannidis et al., 2017) to more than 350 in some ant species (McKenzie et al., 2016).
Determining the structure of ORs has for many years been the “Holy Grail” in the chemosensory field. Lack of structural information about ORs has limited our mechanistic insight into the function of ORs at the molecular level. With recent advances in methods to investigate structure and function of membrane-bound proteins, such as chemoreceptors, using synchrotron light, this limitation is beginning to ease. The recent advances in structural biology have led to the structural determination of more than 200 transmembrane proteins since 2010 (Erlandson et al., 2018). Most of these structures have been determined using canonical X-ray crystallography following protein purification and stabilisation. Rapid method development within cryo-EM has dramatically increased the number and the quality of membrane protein structures generated, and consequently, improved the deductive power generated by the structural data.
The only two structures of an OR protein involved in the insect olfactory transduction chain have been determined using cryo-EM. In the first case, the homo-tetramer structure of Orco from the parasitic fig wasp Apocrypta bakeri was determined at 3.5 Å resolution, giving the first insight into the structure-function of this receptor family (Butterwick et al., 2018). In the second case, the native homo-tetramer structure of the odorant receptor MhOR5 from the jumping bristletail Machilis hrabei, a basal insect group that lacks Orco, was determined at 3.5 Å resolution (Del Marmol et al., 2021). This recent breakthrough on OR and Orco structure function relations may be the starting point for the next generation of studies of insect ORs, allowing for comparative studies of ORs from different species, either in their non-ligated form, or in complex with their specific odorants, giving structural insights into OR specificity and how this is gained structurally and chemically by different ORs.
The structural information obtained for ORs can be utilised to address the specificity and sensitivity of ORs for both deepening our understanding of how odorants interact with insect ORs, as well as to discover and design novel ligands that either can be used to study insect behaviour in response to specific odorants, or used in biotechnological applications. Why could this knowledge be relevant to understand the human impact on insect olfaction? As mentioned above, oxidant pollutants can degrade, e.g., flower volatiles (Farre-Armengol et al., 2016; Cook et al., 2020), and by that change those odour ligands, which insects, since evolutionary times, use to locate and identify their nectar sources since evolutionary times. A better understanding of OR-ligand interactions will, hence, tell us whether an oxidised ligand still will activate the same set of olfactory sensory receptor neurons or not. The OR structures can be determined in complex with identified oxidised and non-oxidised ligands, in order to thoroughly describe the receptor-ligand interactions, and to identify ORs that are affected by anthropogenic conditions and to identify “hot-spots”–if any–in the ligand binding domains of the ORs that are correlated with anthropogenic conditions. This could potentially allow us to predict how severe the anthropogenic effects on a given insect species might become.
Do Anthropogenic Pollutants Have an Impact on Olfactory Neurons in the Brain?
What is known regarding the effects of human-derived pollutants, such as ozone, NOx, and CO2, on the neuronal circuitry of the insect brain? As mentioned above, a recent study in bumblebees (Bombus terrestris) and fig wasps (Blastophaga psenes) showed that chronic exposure (i.e., 60–180 min) of these insects to ozone significantly affected their antennal responses to odours present in floral scents (Vanderplanck et al., 2021), suggesting that ozone exposure has a direct impact on the olfactory neural circuitry. Interestingly, the observed effects were odour- and concentration-dependent in a manner that antennal responses to some odours, such as linalool oxides, were not impaired by ozone exposure. Furthermore, the species studied were affected differently. This finding indicates that ozone pollution alters odour detection in different ways and that distinct molecular and/or neuronal mechanisms might account for the effects resulting in certain species, not surprisingly, being more vulnerable to pollutants than others.
In addition to odour detection, effects of pollutants on olfactory learning and memory abilities have been analysed in honeybees (Leonard et al., 2019). Honeybees exposed to ozone and traffic-related air pollution displayed strongly reduced olfactory learning performances and memory formation following appetitive conditioning, while mortality was not affected. These findings suggest that the odour recognition process, which enables an animal to locate, identify and discriminate an odour source, is directly impaired by human-derived emissions of pollutants.
Whether the olfactory circuits in the brain are impacted by exposure to pollutants is so far unknown and can only be speculated upon. We already know that the olfactory system of insects can adapt to altered environmental conditions, such as increased levels of CO2, and can exhibit activity-dependent plasticity (Devaud et al., 2001; Berdnik et al., 2006; Sachse et al., 2007). Hence, it seems plausible that olfactory neurons may be affected by the exposure to other anthropogenic factors. While the underlying mechanisms remain elusive for the insect brain, several studies have elucidated the consequences of exposure to air pollution in the brain of mammals (Bello-Medina et al., 2019; Morris et al., 2021). Studies in mice and rats have revealed that the cellular mechanisms in neurons and glia cells exhibit strong and long-term perturbations following exposure to diverse pollutants during early development (Morris et al., 2021). The crucial mechanisms that have been identified can be broadly categorised into an imbalance of neurotransmitter release, which is linked to neuroinflammation, and oxidative stress, which causes the loss of synaptic plasticity, to name one factor (Bello-Medina et al., 2019; Morris et al., 2021). The consequences are dramatic and lead to an impaired development of the nervous system, which, in turn, leads to brain injury and neurodegeneration during adult life. Similar mechanisms may also occur in the insect brain. Hence, investigating the effects of anthropogenic pollutants on the neuronal circuitry of the insect brain, with regard to anatomical and functional features of individual olfactory neurons to determine the consequences for their survival and reproduction, is highly relevant and timely as these behaviours depend heavily on olfaction.
Plant-Insect Interactions
Effects on Pollination
As mentioned above, we are currently facing a dramatic decline in insect biomass (Hallmann et al., 2017; Seibold et al., 2019), which is thought to depend both on habitat loss and the increased use of pesticides, which in turn, might have severe effects on plants that rely on insect pollinators. However, recent studies have revealed that, in the Anthropocene, plants can suffer from decreased pollination service, even when insects are present. Whether a plant is pollinated depends heavily on the area in which the plant grows, as plants in urban areas are less well-pollinated compared to similar plants in other landscapes (Bennett et al., 2020). Such anthropogenic effects also exist over short distances. When wildflower meadows are provided along streets, pollinating insects avoid the flowers close to the street, while already 5–7 m away from the traffic, many more insects are observed (Phillips et al., 2021). Not only do insect numbers decline due to anthropogenic factors, but the remaining insects are hampered in their odour-guided behaviour close to human activities.
Pollination and floral location for nectar feeding are essential to the life cycles of many insects. Oxidative pollutants in the environment can change floral odour plumes and affect their behaviour, resulting in decreased energetic feeding habits and pollination efficiency. We recently showed that increased levels of ozone can change the composition of a floral plume and disrupt the innate attraction of naïve tobacco hawkmoths Manduca sexta to the odour of one of their most preferred flowers, that of tobacco, Nicotiana alata. The gas chromatographic–mass spectrometric (GC-MS) analysis of ozone-exposed and non-exposed flower odours revealed that some components from the original flower blend were strongly reduced, or almost absent, after ozone exposure, while novel components were identified in the ozone-exposed flower emissions (Cook et al., 2020). Ozone has also been shown to degrade the floral scent of Brassica nigra resulting in reduced pollination by bumble bees (Farre-Armengol et al., 2016). Chemical analysis of the B. nigra floral odour revealed that plant-produced volatiles, e.g., monoterpenes, anisaldehyde, phenol, and p-cymene, were reduced, while breakdown products, e.g., benzaldehyde, were slightly increased, as the distance from the ozonated flowers increased. At 4.5 m distance, foraging bumblebees were no longer attracted to the Brassica flowers. Pollutants, other than ozone and NOx, are also implicated in reducing ecosystem services during the Anthropocene. Riffell et al. (2014) showed that moths had difficulties in locating flowers against a background of car-related pollutants, e.g., toluene and xylene, as these activate a similar subset of olfactory sensory neurons as the original floral odour and, therefore, impair the ability of the moth to discriminate among flowers at the neuronal level. These results call for more studies on other insects, to determine whether these are generalisable effects.
There is, however, some light at the end of the tunnel. While the above-mentioned hawkmoths were not attracted by ozonated flower odours, moths were attracted to visually conspicuous flowers emitting the ozonated odour blend. When moths were allowed to nectar feed on these flowers, they associated the changed odour blend with the reward, and subsequently followed odour plumes regardless of ozonation. Although pollutants might reduce the innate olfactory attraction of pollinators, insects that search for flowers do not always rely on olfaction alone, but also use other modalities such as vision, and can thus be able to find their host plants. To properly understand the mechanisms underlying how plant-insect interactions might be affected by anthropogenic pollutants, we need to first assess the different levels at which these pollutants could interfere.
1. Oxidative stress responses of plants after exposure to, e.g., ozone, could result in changed emissions of volatile organic compounds (VOCs).
2. Ozone could chemically react with these VOCs, i.e., further change the odour blend that the insect experiences.
3. Whether the insect is affected by these changes depends on how narrowly tuned its olfactory system is to the blends of its host plants.
4. Insects might cope with these changes by exhibiting adaptive behaviours, e.g., learning.
However, regardless where pollutants would interfere with pollinator-plant interactions, they could potentially result in global reduction in pollination. As 80% of our food crops are dependent on active insect pollination (Klein et al., 2007), further investigations are needed.
Effects on Oviposition Site Seeking and Selection
The anthropogenic effect on plant-insect interactions might not be restricted to pollination and nectar feeding. Females of M. sexta oviposit on the plants from which these moths also nectar-feed (Smith et al., 2018). Pollutants could thus, apart from interrupting pollination, lead to a changed oviposition preference in this and other species. Oviposition decisions are often crucial for progeny performance and survival, and the location and selection of egg-laying sites by gravid insects rely strongly on plant cues emanating from suitable local environments. For herbivorous insects, plant secondary metabolites, volatile as well as non-volatile, are important for host-plant identification and assessment of plant quality during oviposition. The plants may produce these secondary compounds constitutively or these can be induced by biotic and/or abiotic stressors. These compounds are considered to be plant defence compounds, which in general function as repellents and deterrents (Bohinc et al., 2012), but for some species they may act as attractants and stimulants (Steinbauer et al., 2004; Liu et al., 2020). The insect response and valence of the compounds depend on their identity and concentration present in individual plants (Bruce et al., 2005).
Rising temperatures, as well as increasing ozone and CO2-levels, can change plant phytochemistry and affect the profiles of both primary and secondary metabolites. Thus, these abiotic factors can affect the nutritional value of plants and, by this, influence insect oviposition and feeding patterns of the emerging larvae (Zavala et al., 2017; Hamann et al., 2021), with, e.g., leaf beetle larvae facing higher mortality when growing on Eucalyptus plants, which were exposed to increased levels of CO2 (Lawler et al., 1996). Furthermore, such factors can affect the production of constitutive and induced defence compounds within the plant by changing the allocation of resources between the different biosynthetic pathways (Jamieson et al., 2017; Zavala et al., 2017). This can have direct consequences for the insects, as well as their associated microbial communities, which are crucial for the success of many insect species. Altered emission of volatile compounds can influence the perceived suitability of the plant as a source of food and shelter by affecting herbivore-host plant attraction, as well as the efficacy of natural enemies of the herbivore (Bruce et al., 2005). Understanding the anthropogenic effects on plant cues and oviposition behaviours is important, as these factors can have direct effects on the future production of agricultural and silvicultural products, as well as on human health.
Conifer-feeding bark beetles (Coleoptera, Curculionidae, Scolytinae) are keystone species of forest ecosystems, contributing to the decomposition of dead wood and nutrient recycling. At high population levels, however, some species have the ability to attack and kill trees in large areas of healthy forest (Edmonds and Eglitis, 1989; Raffa et al., 2016). Beetles attack trees and, after mating, the females oviposit under the bark and the emerging larvae develop, well-protected, while feeding on the phloem of the tree and on specific symbiotic ophiostomatoid fungi deposited by the ovipositing female. These fungi are believed to provide nutrients to bark beetle offspring, promoting their successful development (Kandasamy et al., 2021). Short-range laboratory bioassays have shown that females of the Eurasian spruce bark beetle Ips typographus are more attracted to a mixture of the male-produced aggregation pheromone and fungal volatiles compared to the pheromone alone (Kandasamy et al., 2021). Hence, in the natural environment, successful establishment of fungi inside the male-constructed mating galleries in the tree, may influence the females’ choice of mates as well as oviposition sites, because the females excavate a tunnel for oviposition, extending from this gallery after mating has taken place (Kandasamy et al., 2019; Kandasamy et al., 2021). Evidence is accumulating that the success of bark beetles, to a significant extent, is attributed to their association with these fungi, including species in the genera Grosmannia, Endoconidiophora, and Ophiostoma (Kandasamy et al., 2016). Apart from contributing to beetle nutrition as described above, these fungi metabolise host tree defence chemicals, and may also accelerate tree death (Horntvedt et al., 1983; Kandasamy et al., 2016; Kandasamy et al., 2019; Kandasamy et al., 2021). The distribution of the fungi varies over time and through space, which is thought to be due to different climatic needs and temperature optima for growth, as well as local adaptations to phloem moisture levels and tree chemistry (Solheim, 1991; Six and Bentz, 2007; Giordano et al., 2013; Kandasamy et al., 2016). Increasing temperatures and more frequent severe weather events, such as drought or heavy precipitation, will affect all of these factors. In addition, temperature and host tree chemistry, such as resistance markers, also have direct effects on the beetles, including their development and chemosensory-mediated host choice (e.g., Schiebe et al., 2012). One can therefore expect that the increasing temperatures in the Anthropocene will affect the beetle-fungal-tree interaction, with effects being dependent on the climatic zone. In turn, this may impose additional challenges for pest control; for instance if more virulent fungi (such as Endoconidiophora polonica and Grosmannia penicillata; Krokene and Solheim, 1998; Zhao et al., 2019) are favoured by the new climatic conditions, the chances that trees will survive an attack may become reduced. Also, an altered fungal community may impose novel selection pressures on the bark beetles, and local adaptations to new fungal communities may evolve.
The different bark beetle-associated fungi release different volatile blends. In addition to increasing short-range attraction to the aggregation pheromone in females, laboratory bioassays have shown that I. typographus prefers a volatile mixture of host- and fungal compounds from several (but not all) of its fungal associates over host volatiles alone, and the beetle also uses olfaction to discriminate among the different fungi (Kandasamy et al., 2019; Kandasamy et al., 2021; Tanin et al., 2021). Hence, olfactory cues are likely crucial for maintaining the symbiotic relationship across beetle generations (Kandasamy et al., 2019; Kandasamy et al., 2021). Thus, in addition to the expected effects of increasing temperatures on fungal communities and hence fungal “semiochemical diversity,” elevated levels of atmospheric pollutants (e.g., ozone) may have consequences for the symbiosis, including the selection of feeding and oviposition sites. For instance, increased ozone concentration may have direct negative effects on fungal growth and survival, degrade the fungal volatiles that may change beetle preferences (Blande et al., 2010; Blande et al., 2014), and/or affect the beetle olfactory system directly. Pollutants, together with rising temperatures, are thus likely to change the interactions among beetles, fungi, and trees, with unknown evolutionary, ecological, and economic outcomes.
Apart from its effect on the nutritional value of plants (Zavala et al., 2017; Hamann et al., 2021), an increased CO2-level can affect the production of constitutive and induced defence compounds within the plant by changing the allocation of resources between different biosynthetic pathways (Jamieson et al., 2017; Zavala et al., 2017). Numerous studies in herbivorous insects, with emphasis on lepidopteran species, show how increased CO2-levels affect insect performance (Hamann et al., 2021). For example, increased CO2-levels can affect the performance of different moth species (Barbehenn et al., 2004; Li et al., 2017). While it is still unclear whether the effects of increased CO2 on insect performance are direct (Pincebourde et al., 2017), it is well-established that herbivorous insects are indirectly affected by changes in phytochemistry in the host plants under increased CO2-levels (Robinson et al., 2012; Hamann et al., 2021). However, there are few studies on preference and especially oviposition decisions in which both the plant and the insect have been reared under elevated CO2-levels in the laboratory followed by a bioassay performed under the same conditions as those of rearing (Hamann et al., 2021). Thus, there is a need for more studies investigating the effect on oviposition under conditions that resemble future climate conditions.
Spodoptera moths can potentially provide important knowledge about how climate change affects oviposition decisions under elevated CO2-levels. Several of these species are range-expanding or invasive and are forecasted to further increase their range due to climate change. A striking example here is the recent expansion of the Fall armyworm, Spodoptera frugiperda (Zacarias, 2020). Anthropogenic-driven changes are expected to have a large impact on host plant choice in these species, and thus on their potential as devastating crop pests. Studies on oviposition in Spodoptera littoralis have shown that this species has an innate preference hierarchy among potential host plants that can be modified through experience by both intra- and trans-generational plasticity (Thoming et al., 2013; Proffit et al., 2015; Rosvik et al., 2020). Differences in plant-derived olfactory secondary metabolites are important for plant identification, assessment of plant quality (Zakir et al., 2013; Conchou et al., 2017), and for the experience-based plasticity (Lhomme et al., 2018). Therefore, S. littoralis, provides an excellent model species to investigate how increased CO2-levels may affect host plant preference and decisions during oviposition. In addition to increased CO2-levels, other factors of the climate change, e.g., the variation in nutrient levels and rainfall, can also affect plant suitability and hence the array of leaves of different age and quality available to insects (Gleadow et al., 1998; Gherlenda et al., 2016).
Oviposition-site selection by mosquitoes can be directly influenced by the presence of plants and their associated volatiles in conjunction with aquatic habitats (Wondwosen et al., 2016; Wondwosen et al., 2017; Wondwosen et al., 2018). While mosquitoes are not generally considered herbivores, both larvae and adults feed on plant-derived and plant-associated food sources, e.g., pollen (Ye-Ebiyo et al., 2000; Asmare et al., 2017) and microbes, as well as nectar (Foster, 1995), respectively. As with other insects, plant-associated volatiles can indicate the suitability of the oviposition site as a larval habitat (Wondwosen et al., 2016; Wondwosen et al., 2017; Wondwosen et al., 2018). Alterations in the perception of the volatile profiles at these potential oviposition sites with rising CO2-levels may indirectly change the context of the plant-derived cues, as has been previously demonstrated with host odours (Majeed et al., 2014). Increasing ozone-levels also have the potential to directly change the composition of plant-derived volatile emissions through selective oxidation. While mosquitoes can tolerate a degree of variation in the composition of plant volatiles associated with oviposition sites, there are limits, outside of which these blends lose their attraction (Wondwosen et al., 2021). By altering the oviposition odour landscape of the mosquito in response to these anthropogenic factors, we risk creating a much less predictable landscape in which to identify the probable larval habitats of mosquito disease vectors and employ effective control measures.
Host Range Expansions and Host Shifts
As the distribution of species changes in response to a changing climate and insects spread into new territories, different species and biotypes will come into conjunction. Such range-expanding insects, as well as insects that are introduced into new environments by human activities, may encounter a very different community of species to which they must adapt, in order to persistently colonise a new region. This can lead to large ecological consequences and result in changes in host utilisation and preferences, and as a consequence, to host shifts. One striking example of range-expansion and change in utilisation of host plants over evolutionary time is the fruit fly, Drosophila melanogaster. The ancestral home of the fruit fly is found in miombo and mopane forests in Zambia and Zimbabwe. During the 10,000 years since the fly left these forests as a human commensal (Sprengelmeyer et al., 2020), it has managed to colonise almost every corner of the planet. An important factor in the spread has been host plant shifts. While wild flies in the African Urheimat feed and breed on marula fruit (Mansourian et al., 2018), flies in the diaspora utilise a wide range of resources.
Understanding the mechanisms by which host shifts occur under novel selection pressures can aid in the prediction of future preference changes as a result of anthropogenic pressures. It is important to explore these and other factors, along with the generalisability of factors, resulting in an increasing frequency of host shift in our various model systems. One example of such a host shift can be found in the ongoing conjunction of ranges of the two Culex pipiens biotypes and the resulting hybrids (Ruybal et al., 2016; Figueroa et al., 2020). Other examples can be seen in bark beetles, in which climate change has facilitated range expansions and intensified outbreaks, both of which may lead to the colonisation of novel hosts, including previously allopatric/naïve tree species or less preferred sympatric trees within the beetles’ historical range (Cudmore et al., 2010; Raffa et al., 2013). Recent human-aided spread of insects and range-expansion in moth species of the genus Spodoptera, is yet another example that can provide new knowledge about host shifts connected to the introduction to new environments and climates. These species are expected to further expand their range due to climate change and will then encounter new habitats with novel potential host plants (Zacarias, 2020). However, little is known about the effects on host plant choice when colonising new areas with a different host plant assembly. Knowledge about mechanisms regulating host shifts can be essential for designing future control strategies.
The Anthropocene is witnessing host range expansions in mosquitoes, in which the risk of disease transmission previously posed by minor disease vectors increases along with the preference for biting humans. The house mosquito, Cx. pipiens, is synanthropic, with a wide distribution in temperate regions (Vinogradova, 2000; Brugman et al., 2018), and occurs as two behaviourally and physiologically different biotypes, pipiens and molestus. Biotype pipiens is anautogenous (requires a blood meal), eurygamous (mates in open spaces), heterodynamic (diapauses in winter), ornithophilic (prefers to feed on birds), and less synanthropic than molestus, which is autogenous, stenogamous (mates in enclosed spaces), homodynamic, mammophilic, and strongly synanthropic (Harbach et al., 1984; Harbach et al., 1985; Clements, 1999; Gomes et al., 2013). These differences in ecological, behavioural, and physiological traits have a strong impact on the vectorial capacities of the biotypes.
As the Anthropocene progresses, the increasing temperatures and intensification of urbanisation in temperate regions are affecting the life history traits and distribution of Cx. pipiens (Ruybal et al., 2016; Figueroa et al., 2020). The effects, however, are complex and variable, likely due to behavioural and physiological differences in the biotypes, along with the variability within biotypes demonstrated across geographical areas (Ruybal et al., 2016). While needing more investigation, the regions of sympatry between pipiens and molestus are likely to increase as the distribution and ecological traits of the biotypes change with the landscape and the climate, increasing the prevalence of hybrid Cx. pipiens (Osorio et al., 2014). Hybrid offspring, which occur in areas where the biotypes are sympatric, are opportunistic blood feeders, demonstrating that host preference in Cx. pipiens is heritable (Fritz et al., 2015). New and ongoing introgression among the biotypes and hybrids, due to the maintenance and expansion of the current Cx. pipiens distributions (Brugman et al., 2018), will likely result in increasing mosquito-human interactions. As this creates excellent conditions for these mosquitoes to increase their capacity as bridge vectors of arboviruses from the natural hosts, birds, to the accidental and dead-end hosts, both humans and domestic mammals (Chevillon et al., 1995; Fonseca et al., 2004; Kilpatrick et al., 2007), we can expect the mosquito-borne disease burden of, e.g., West Nile virus, in the temperate areas to rise in the coming decades, if left unchecked.
Another example in which increasing temperature may foster range expansions that eventually may lead to host shifts is found in conifer-feeding bark beetles of the Scolytinae subfamily (Kurz et al., 2008; Raffa et al., 2013; Biedermann et al., 2019). Bark beetles have repeatedly been shown to successfully reproduce in trees with which they do not share an evolutionary history. This is likely, in part, attributed to the fact that the naïve hosts lack coevolved defences, offering little resistance against novel insect invaders and their microbial associates (Cudmore et al., 2010; Raffa et al., 2013). In North America, for example, climate change has facilitated the spread of mountain pine beetle (Dendroctonus ponderosae) from British Columbia (where it primarily colonises lodgepole pine, Pinus contorta, and ponderosa pine, Pinus ponderosa) over the geoclimatic barrier of the Rocky Mountains, into Alberta, where it now infests a naïve host tree, the jack pine (Pinus banksiana), threatening the entire Canadian boreal forest (Sambaraju and Goodsman, 2021). Additionally, the warmer climate has resulted in more frequent attacks of this beetle on high-elevation whitebark pine (Pinus albicaulis), a keystone species of subalpine forests that previously was only occasionally attacked (Logan et al., 2010; Bentz et al., 2015; Raffa et al., 2016; Sambaraju and Goodsman, 2021).
Abilities to utilise non-native host tree material have also been shown in the Eurasian species I. typographus. Multi-choice laboratory bioassays using semi-natural bark diets demonstrated that I. typographus has similar tunnelling preferences for the bark of its historical host Norway spruce (Picea abies) as compared to the two naïve North American spruce species, white spruce (Picea glauca) and black spruce (Picea mariana) (Tanin et al., 2021). Additionally, beetles preferred bark media colonised by symbiotic fungi over bark media without fungi, while not showing a stronger preference for their own fungal associates over the fungi associated with the North American spruce beetle Dendroctonus rufipennis (Tanin et al., 2021). Although it remains unknown whether I. typographus is able to infest these non-native trees in nature, the existing pre-adaptations, or preferences, may contribute to the evolution of a host shift should I. typographus become introduced into North America. Then, associations with novel fungal communities could evolve, if these fungi increase beetle fitness in the new host trees (Tanin et al., 2021).
Climate change has also resulted in increased frequency, severity, and duration of bark beetle population outbreaks, causing unprecedented landscape-scale forest destruction. Intensified I. typographus outbreaks will increase the risk of Norway spruce populations becoming locally or regionally extinct, which is likely to result in more frequent attacks on other sympatric conifer species. Indeed, I. typographus has been observed to attack Scots pine (Pinus sylvestris) in its native range in Europe (Jankowiak and Hilszczanski, 2005; Andersson and Hansson, personal observations), but with unknown effects on reproductive success and offspring development. It remains unclear whether these attacks are spill-over effects from local population outbreaks or whether pine trees are also utilised during endemic population densities. Exploring the capacity of bark beetles, such as I. typographus, to utilise different host trees, and the associated fitness consequences for the beetles and their fungal symbionts, is an important avenue for further research and future forest protection.
Another possible mechanism that can drive host shifts is how Anthropocene-driven changes affect host plant characters within an assembly of plants in a habitat. Elevated temperatures and changes in the concentration of atmospheric gases can have large effects on plant chemistry. The magnitude of the effect of CO2 on plant primary and secondary metabolites in response to anthropogenic changes may differ between plant species and influence insect feeding and oviposition preference (Zavala et al., 2017). For example, the defences of C4-plants is predicted to be less affected than for C3-plants, and this can change herbivory patterns between the two plant types (Barbehenn et al., 2004). Such differential changes in plant suitability and preference can lead to host plant shifts in herbivorous insects and have large effects on plant-insect interactions and the communities of both plants and of insects (Robinson et al., 2012; Hamann et al., 2021). Thus, host shifts may occur among already available host plants, as these may respond differently under increased CO2-levels. These changes may affect both the innate preference and preference shifts through feeding experience-driven phenotypic plasticity. For example, S. littoralis larval feeding preference may change under increased CO2-levels (Agrell et al., 2006), which could lead to changes in feeding patterns between host plants. Increased CO2-levels also have the potential to change adult behaviour, as larval feeding can induce phenotypic changes on host plant preference in the adult (Thoming et al., 2013; Lhomme et al., 2018). Thus, a change in host plant chemistry that changes both the larval and adult preference hierarchy between potential host plant species can be an important factor that facilitates host plant shifts within an environment.
Insect-Insect Interactions
Effects on Pheromone Communication
Increased temperatures, emission of air pollutants, and globalisation may dramatically affect insect-insect communication systems. Many insects find and choose mates by means of more or less species-specific sex pheromones, or in the case of bark beetles, by using aggregation pheromones, which are also used for mate finding. Closely related species often use chemically similar pheromone components, but in specific combinations or in specific relative amounts. While the sender has to produce a species-specific signal, the receiver needs to be able to detect it and to discriminate it from allospecific signals. The evolution of chemical communication is thus the delicate coevolution of signals with the matching olfactory receptors and neural circuits, tuned to detect and interpret chemical signals of potential mating partners. The required specific fit of signal and receiver bears the problem that even minute chemical modifications of the pheromones may potentially interrupt sexual communication. In bark beetles, the commonly used aggregation pheromone components cis- and trans-verbenol are oxidised by microbes or by autoxidation into verbenone, a compound that inhibits pheromone attraction in several species, especially during the later attack phases (e.g., Brand et al., 1976; Leufven et al., 1984; Schlyter et al., 1989). In the case of moths, probably the best studied insect order when it comes to pheromone communication, long-chain fatty acyl derivatives with double bonds in specific positions and with specific geometry are typical pheromone components. The dependence on double bonds makes pheromone communication in both bark beetles and moths vulnerable to effects from oxidising pollutants, e.g., ozone or NOx. To our knowledge, so far only one study has shown that a pheromone, in this case the well-known D. melanogaster aggregation pheromone cis-vaccenyl acetate (cVA), becomes degraded at increasing ozone levels (Arndt, 1995). It will, however, be necessary to repeat similar analyses with different kinds of pheromones and a variety of ozone and/or NOx levels to learn whether pheromones in general might be affected by changes in ozone and/or NOx levels during the Anthropocene. Depending on double bond configuration and functional groups, pheromones might differ in their vulnerability to oxidation. Some might become significantly degraded by increased levels of pollutants that we are already facing, while others might be more stable and, hence, not affected by the levels of pollutants which are expected during the coming century. As pheromones and mating assays in, e.g., many moth, bark beetle, and fly species are well-established, future experiments should clarify whether any of the expected chemical degradations will result in reproductive problems.
How changes in global temperature might affect sexual communication in animals was reviewed by Groot and Zizzari (2019). Relevant information is scarce and our understanding is consequently limited. Following Groot and Zizzari (2019), effects of extreme temperatures on pheromone communication may be divided into effects on pheromone biosynthesis, effects on pheromone decay, and impairment of pheromone reception/perception. Females of the potato tuber worm moth, Phtorimaea operculella, modified the ratio of their pheromone components when exposed to high temperature (35°C) (Ono, 1993, 1994), while in Helicoverpa zea, females produced less of the major sex pheromone component (Raina, 2003). In three species of Drosophila, heat stress altered mating and courtship behaviours, which was attributed to changes in the composition of male cuticular hydrocarbons involved in chemical communication between the sexes (Markow and Toolson, 1990). Temperature effects on the emission of chemical signals, possibly also altering the ratio between pheromone components, have been addressed above. Importantly, such temperature-related effects may have implications for pheromone-based insect control strategies, as temperature may also influence release rates and longevity of synthetic pheromone dispensers.
The pheromone response window of male moths is narrowly tuned to female pheromone production and release [see, e.g., Linn and Roelofs (1989) for a review], but males usually respond to a wider range of pheromone component ratios than the typical females in the population release (Löfstedt, 1990). An elegant series of experiments on the Oriental fruit moth Grapholita molesta (Baker et al., 1981; Linn and Roelofs, 1983) showed that maximal pheromone response was dependent on presence of all pheromone components and that males exhibited maximal levels of upwind flight and source contact to a narrow range and doses of the geometric isomers of the pheromone components. The level of response dropped drastically with slight changes away from the female-produced ratio of (Z)- to (E)-8-dodecenyl acetate. A comparative study was performed with the pink bollworm Pectinophora gossypiella to assess the generality of these findings (Linn and Roelofs, 1985). Bollworm females produce a two-component pheromone mixture (61:39) of (Z,Z)- and (Z,E)-7,11-hexadecadienyl acetates (Haynes et al., 1984). This species exhibited a much lower response specificity, responding to a wider ratio of component ratios and release rates. It turned out that the difference was due to effects of temperature on the response windows (Linn et al., 1988). Whereas the original study on G. molesta was carried out at 20°C the P. gossypiella experiments had been carried out at 26°C. When tested at the higher temperature, the response specificity of G. molesta was relaxed. Although the blend-dose combination eliciting the highest response remained the same, the range of combinations eliciting peak attraction was expanded. Baker et al. (1989) showed that the temperature effect on male response specificity at the receptor level is associated with differential rates of adaptation to the individual pheromone components. What this means under natural conditions remains an open question, as it has been shown in many species of moths that changes in temperature may shift the daily period of their mating activity.
Finally, climate-induced changes in the distribution and phenology of species have effects on the olfactory landscape of the individual species. Species-specific pheromones may have evolved by selection for efficient mate-finding and reproductive isolation from species with overlapping distributions. Fast changes in the distribution of many insects and the resulting potential interference with pheromone communication of novel sympatric species may give rise to both character displacement and character release, and provide us with opportunities to study selection on communication systems in real time under natural conditions.
Host Preference in Mosquitoes
Of the 3,500 described mosquito species, only a fraction have evolved to specialise in biting humans and are competent to transmit human diseases (Clements, 1999). Climate and human activity affect mosquito-human interactions, an effect that can be traced back to the onset of sedentary human settlement at the dawn of agriculture, ca. 10.000 years ago (White et al., 2011; Rose et al., 2020). Climatic change within the Anthropocene has strengthened this interaction and is predicted to severely alter disease transmission (Colon-Gonzalez et al., 2021) due to decreasing biodiversity and increasing preference for human hosts as a result of evolutionary pressure (Hoberg and Brooks, 2015). Current anthropogenic effects on mosquito-human interactions are predominantly caused by greenhouse gas emissions and urbanisation (Rocklov and Dubrow, 2020). The resulting changes in temperature and humidity create urban heat islands and may alter the onset, duration, and intensity of seasons, which may be beneficial for mosquito-borne disease (Ligsay et al., 2021). In contrast, indirect effects of anthropogenic activity may negatively affect the ability of mosquitoes to locate resources, such as their human hosts, by interfering with the quality and quantity of relevant bioactive volatile compounds.
Regional changes in temperature profiles have already, and are predicted to continue to, alter the composition and distribution of mosquito species, as a result of the temperature tolerances of different mosquito populations and species (e.g., Cunze et al., 2016; Rose et al., 2020). While temperature and other climatic factors, including humidity and precipitation, may alter mosquito distribution, human activity appears to be the main driver for mosquito-borne disease (Franklinos et al., 2019; Rocklov and Dubrow, 2020). Early human activity modified the landscape, dividing mosquito populations and creating separate ecological niches, which was further strengthened through a change in climate, especially intense dry seasons, that drove changes in host preference in Aedes aegypti (Rose et al., 2020) and likely other anthropophilic mosquito species (White et al., 2011). Current trends in urbanisation, i.e., 68% of the global population will live in cities by 2050 (UN Department of Economic and Social Affairs, 2018), are creating urban heat islands, cityscapes with increased land temperatures, reduced water sources, and limited vegetation compared with surrounding rural areas. Strategies to mitigate the effect of urban heat islands, provide patches with vegetation and water that intensify the association of mosquitoes with humans, thus increasing the urban health burden (e.g., Ligsay et al., 2021). Species that are adapted to urban areas and have a tolerance for high temperatures, such as Ae. aegypti and Anopheles stephensi, are currently thriving in cities and broadening their distribution, as well as increasing the association with humans and the risk of disease transmission (Reinhold et al., 2018; Sinka et al., 2020). Urbanisation may further modify mosquito-human interactions by directly affecting the ability of mosquitoes to detect and locate their human hosts.
Locating a human host for a blood meal is dependent on olfaction (Takken and Knols, 1999). The molecular and physiological mechanisms regulating the activation and attraction of mosquitoes to a human host, has received substantial attention, identifying CO2 in exhaled breath and salient human body odorants as essential for these behaviours (Takken and Knols, 1999; Lu et al., 2007; Carey et al., 2010; Majeed et al., 2014; Majeed et al., 2016; Majeed et al., 2017; Ghaninia et al., 2019; Omondi et al., 2019). Factors affecting the olfactory system have the potential to alter the host-seeking behaviour, and thus change the dynamics of disease transmission (Cohuet et al., 2010). Transient increase in the background levels of CO2, approximate to those predicted for 2050 and 2100 (600 ppm and 1200 ppm, respectively), disorient host-seeking Culex, but not Aedes or Anopheles, mosquitoes (Turner et al., 2011; Majeed et al., 2014). Many Culex species have evolved a preference for feeding on birds, which emit low levels of CO2 that are detected by the acutely sensitive peripheral olfactory system. Elevated CO2 levels adapt the olfactory sensory neurons sensitive to CO2 in Culex females and prevent the activation of host seeking as well as the subsequent gating of attraction to host odours (Majeed et al., 2017). Given the predicted changes in atmospheric CO2-levels over the next several decades, it is likely that mosquito-host interactions, which have evolved over the last 30–24 million years under CO2 levels similar to current ambient atmospheric conditions, will be disrupted (Tang et al., 2018).
Mosquitoes are attracted to a blend of salient volatile compounds released by humans (Takken and Knols, 1999; Omondi et al., 2019), many of which are derived from the oxidation of sapienic acid and squalene in sebaceous glands of the skin (Zhao et al., 2019). A number of these compounds, including aliphatic aldehydes, short chain carboxylic acids, and ketones, are prominent ozonolysis products (Tang et al., 2016; Liu et al., 2021). Rising levels of tropospheric ozone increase the production of these compounds, which has the potential to increase the level of human attractiveness to mosquitoes, a phenomenon still to be investigated. Previous studies have shown that alterations to the blend of salient human odours by increasing aliphatic aldehydes increases the attraction of host-seeking Anopheles and Culex females to human odour (Leal et al., 2017; Debebe et al., 2020). The potential increase in mosquito-human interactions under elevated ozone levels currently being experienced in highly polluted cities, and predicted to include many more regions around the globe in coming decades, will likely have significant consequences for mosquito-borne disease transmission.
How the Anthropocene and its attendant climatic changes will impact vector borne disease is not clear, but it is certain that there will be significant effects. Many of the factors described above that influence the mosquito-human association interact with each other, and will likely regulate the future vectorial capacity of disease vector mosquitoes (Messina et al., 2015; Franklinos et al., 2019; Rocklov and Dubrow, 2020). Current modelling studies on the future risk of mosquito borne disease often omit global changes that are not directly linked to climate change, limiting their predictive power (Franklinos et al., 2019). Connecting vectorial capacity models with the broader effects of urbanisation, greenhouse gasses, and pollutants on vector ecology has the potential to provide more accurate predictions of future disease transmission.
nGICE
The Max Planck Center (MPC) “Next Generation Insect Chemical Ecology (nGICE)” was initiated in 2020. A MPC is a collaboration between a German Max Planck institute and strong collaborators in other countries. The funding and status is given after a highly competitive, international review process. Within nGICE, the three partners (the Max Planck Institute for Chemical Ecology, Lund University, and the Swedish University of Agricultural Sciences) will use complementary knowledge, skills, and equipment to address the effects of global change on all levels of insect chemical ecology through a novel approach. The three partners complement each other with expertise in different insect models; herbivores (bark beetles and moths), blood feeders (mosquitoes), and the best-established insect model, the fruit fly. This will allow us to cover individual insect interactions, as well as the evolutionary, ecological, and mechanical aspects in a forward-looking perspective. By studying these model systems using state-of-the-art technology, we specifically concentrate on:
1. anthropogenic impacts on olfaction;
2. adaptations and plasticity; and
3. host preference and shift.
A second dimension of nGICE is an ambition to train the next generation insect chemical ecologists. By concentrating most of the funding on hiring doctoral and postdoctoral researchers, we aim to provide a full technological tool box as well as a solid theoretical foundation to these young scientists, to prepare them as future leaders in the field.
Conclusion
Insects are an integral part of our everyday lives, providing many indispensable ecosystem services while also competing with us for resources and spreading disease. As we constantly modify our environment and cause the emission of different gases, we change the balance of the chemical communication in the ecosystem. Flowers and insects start smelling differently, trees are weakened, geographical barriers are moved and broken. All of these processes mean that we are facing a new future in our human-insect interaction. Some ecosystem services might already be broken or diminished, e.g., when it comes to pollination. Geographical areas unused to the presence of disease vectors face new challenges when new species invade. Forestry and agriculture face similar challenges. We thus need to adapt to the new conditions. Hopefully, the ongoing political processes to ameliorate the fallout of human activities will have some effect, but still we are facing severe changes in the environment. This means that we need to increase our understanding of how the by far most speciose group of organisms, the insects, are affected by the changes. What is clear from the present review is that we are just at the beginning of a scientific journey to understand these effects and that the questions faced are highly multi-faceted. No factor works in isolation.
Author Contributions
All authors listed have made a substantial, direct, and intellectual contribution to the work, and approved it for publication.
Conflict of Interest
The authors declare that the research was conducted in the absence of any commercial or financial relationships that could be construed as a potential conflict of interest.
Publisher’s Note
All claims expressed in this article are solely those of the authors and do not necessarily represent those of their affiliated organizations, or those of the publisher, the editors and the reviewers. Any product that may be evaluated in this article, or claim that may be made by its manufacturer, is not guaranteed or endorsed by the publisher.
References
Agrell, J., Anderson, P., Oleszek, W., Stochmal, A., and Agrell, C. (2006). Elevated CO2 levels and herbivore damage alter host plant preferences. Oikos 112, 63–72. doi: 10.1111/j.0030-1299.2006.13614.x
Ammeux, N., Housden, B. E., Georgiadis, A., Hu, Y. H., and Perrimon, N. (2016). Mapping signaling pathway cross-talk in Drosophila cells. Proc. Natl. Acad. Sci. U.S.A. 113, 9940–9945. doi: 10.1073/pnas.1610432113
Arndt, U. (1995). Air pollutants and pheromones - a problem. Chemosphere 30, 1023–1031. doi: 10.1016/0045-6535(95)00013-x
Asmare, Y., Hopkins, R. J., Tekie, H., Hill, S. R., and Ignell, R. (2017). Grass pollen affects survival and development of larval Anopheles arabiensis (Diptera: Culicidae). J. Insect Sci. 17:93. doi: 10.1093/jisesa/iex067
Atkinson, R., and Arey, J. (2003). Gas-phase tropospheric chemistry of biogenic volatile organic compounds: a review. Atmos. Environ. 37, S197–S219. doi: 10.1016/s1352-2310(03)00391-1
Baker, J., Walker, H. L., and Cai, X. M. (2004). A study of the dispersion and transport of reactive pollutants in and above street canyons - a large eddy simulation. Atmos. Environ. 38, 6883–6892. doi: 10.1016/j.atmosenv.2004.08.051
Baker, T. C., Hansson, B. S., Löfstedt, C., and Lofqvist, J. (1989). Adaptation of male moth antennal neurons in a pheromone plume is associated with cessation of pheromone-mediated flight. Chem. Senses 14, 439–448. doi: 10.1093/chemse/14.3.439
Baker, T. C., Meyer, W., and Roelofs, W. L. (1981). Sex-pheromone dosage and blend specificity of response by oriental fruit moth males. Entomol. Exp. Appl. 30, 269–279. doi: 10.1111/j.1570-7458.1981.tb03110.x
Barbehenn, R. V., Karowe, D. N., and Spickard, A. (2004). Effects of elevated atmospheric CO2 on the nutritional ecology of C-3 and C-4 grass-feeding caterpillars. Oecologia 140, 86–95. doi: 10.1007/s00442-004-1572-9
Bello-Medina, P. C., Prado-Alcala, R. A., and Rivas-Arancibia, S. (2019). Effect of ozone exposure on dendritic spines of CA1 pyramidal neurons of the dorsal hippocampus and on object-place recognition memory in rats. Neuroscience 402, 1–10. doi: 10.1016/j.neuroscience.2019.01.018
Bennett, J. M., Steets, J. A., Burns, J. H., Burkle, L. A., Vamosi, J. C., Wolowski, M., et al. (2020). Land use and pollinator dependency drives global patterns of pollen limitation in the Anthropocene. Nat. Commun. 11:3999. doi: 10.1038/s41467-020-17751-y
Bentz, B. J., Boone, C., and Raffa, K. F. (2015). Tree response and mountain pine beetle attack preference, reproduction and emergence timing in mixed whitebark and lodgepole pine stands. Agric. For. Entomol. 17, 421–432. doi: 10.1111/afe.12124
Berdnik, D., Chihara, T., Couto, A., and Luo, L. Q. (2006). Wiring stability of the adult Drosophila olfactory circuit after lesion. J. Neurosci. 26, 3367–3376. doi: 10.1523/jneurosci.4941-05.2006
Biedermann, P. H. W., Muller, J., Gregoire, J. C., Gruppe, A., Hagge, J., Hammerbacher, A., et al. (2019). Bark beetle population dynamics in the anthropocene: challenges and solutions. Trends Ecol. Evol. 34, 914–924. doi: 10.1016/j.tree.2019.06.002
Blande, J. D., Holopainen, J. K., and Li, T. (2010). Air pollution impedes plant-to-plant communication by volatiles. Ecol. Lett. 13, 1172–1181. doi: 10.1111/j.1461-0248.2010.01510.x
Blande, J. D., Holopainen, J. K., and Niinemets, U. (2014). Plant volatiles in polluted atmospheres: stress responses and signal degradation. Plant Cell Environ. 37, 1892–1904. doi: 10.1111/pce.12352
Bohinc, T., Ban, S. G., Ban, D., and Trdan, S. (2012). Glucosinolates in plant protection strategies: a review. Arch. Biol. Sci. 64, 821–828. doi: 10.2298/ABS1203821B
Brand, J. M., Bracke, J. W., Britton, L. N., Markovetz, A. J., and Barras, S. J. (1976). Bark beetle pheromones: production of verbenone by a mycangial fungus of Dendroctonus frontalis. J. Chem. Ecol. 2, 195–199. doi: 10.1007/bf00987742
Bruce, T. J. A., Wadhams, L. J., and Woodcock, C. M. (2005). Insect host location: a volatile situation. Trends Plant Sci. 10, 269–274. doi: 10.1016/j.tplants.2005.04.003
Brugman, V. A., Hernandez-Triana, L. M., Medlock, J. M., Fooks, A. R., Carpenter, S., and Johnson, N. (2018). The role of Culex pipiens L. (Diptera: Culicidae) in virus transmission in Europe. Int. J. Environ. Res. Public Health 15:389. doi: 10.3390/ijerph15020389
Butterwick, J. A., Del Marmol, J., Kim, K. H., Kahlson, M. A., Rogow, J. A., Walz, T., et al. (2018). Cryo-EM structure of the insect olfactory receptor Orco. Nature 560:447. doi: 10.1038/s41586-018-0420-8
Carey, A. F., Wang, G. R., Su, C. Y., Zwiebel, L. J., and Carlson, J. R. (2010). Odorant reception in the malaria mosquito Anopheles gambiae. Nature 464, U66–U77. doi: 10.1038/nature08834
Chen, K. K., Tang, T., Song, Q. S., Wang, Z. Y., He, K. L., Liu, X., et al. (2019). Transcription analysis of the stress and immune response genes to temperature stress in Ostrinia furnacalis. Front. Physiol. 10:1289. doi: 10.3389/fphys.2019.01289
Chevillon, C., Eritja, R., Pasteur, N., and Raymond, M. (1995). Commensalism, adaptation, and gene flow - mosquitos of the Culex pipiens complex in different habitats. Genet. Res. 66, 147–157. doi: 10.1017/s0016672300034492
Clements, A. N. (1999). The Biology Of Mosquitoes. Sensory Reception And Behaviour. Wallingford: CABI Publishing, 2.
Cohuet, A., Harris, C., Robert, V., and Fontenille, D. (2010). Evolutionary forces on Anopheles: what makes a malaria vector? Trends Parasitol. 26, 130–136. doi: 10.1016/j.pt.2009.12.001
Colon-Gonzalez, F. J., Sewe, M. O., Tompkins, A. M., Sjodin, H., Casallas, A., Rocklov, J., et al. (2021). Projecting the risk of mosquito-borne diseases in a warmer and more populated world: a multi-model, multi-scenario intercomparison modelling study. Lancet Planet. Health 5, E404–E414. doi: 10.1016/S2542-5196(21)00132-7
Conchou, L., Anderson, P., and Birgersson, G. (2017). Host plant species differentiation in a polyphagous moth: olfaction is enough. J. Chem. Ecol. 43, 794–805. doi: 10.1007/s10886-017-0876-2
Cook, B., Haverkamp, A., Hansson, B. S., Roulston, T., Lerdau, M., and Knaden, M. (2020). Pollination in the Anthropocene: a moth can learn ozone-altered floral blends. J. Chem. Ecol. 46, 987–996. doi: 10.1007/s10886-020-01211-4
Crossley, M. S., Meier, A. R., Baldwin, E. M., Berry, L. L., Crenshaw, L. C., Hartman, G. L., et al. (2020). No net insect abundance and diversity declines across US Long Term Ecological Research sites. Nat. Ecol. Evol. 4, 1368–1376. doi: 10.1038/s41559-020-1269-4
Cudmore, T. J., Bjorklund, N., Carroll, A. L., and Lindgren, B. S. (2010). Climate change and range expansion of an aggressive bark beetle: evidence of higher beetle reproduction in naive host tree populations. J. Appl. Ecol. 47, 1036–1043. doi: 10.1111/j.1365-2664.2010.01848.x
Cunze, S., Koch, L. K., Kochmann, J., and Klimpel, S. (2016). Aedes albopictus and Aedes japonicus - two invasive mosquito species with different temperature niches in Europe. Parasit. Vectors 9:573. doi: 10.1186/s13071-016-1853-2
Debebe, Y., Hill, S. R., Birgersson, G., Tekie, H., and Ignell, R. (2020). Plasmodium falciparum gametocyte-induced volatiles enhance attraction of Anopheles mosquitoes in the field. Malar. J. 19:327 doi: 10.1186/s12936-020-03378-3
Del Marmol, J., Yedlin, M. A., and Ruta, V. (2021). The structural basis of odorant recognition in insect olfactory receptors. Nature 597:126. doi: 10.1038/s41586-021-03794-8
Deska, M. (2020). Activity of antioxidant enzymes under induced oxidative stress. J. Ecol. Eng. 21, 42–51. doi: 10.12911/22998993/125454
Devaud, J. M., Acebes, A., and Ferrus, A. (2001). Odor exposure causes central adaptation and morphological changes in selected olfactory glomeruli in Drosophila. J. Neurosci. 21, 6274–6282. doi: 10.1523/JNEUROSCI.21-16-06274.2001
Edmonds, R. L., and Eglitis, A. (1989). The role of the douglas-fir beetle and wood borers in the decomposition of and nutrient release from douglas-fir logs. Can. J. For. Res. 19, 853–859. doi: 10.1139/x89-130
Erlandson, S. C., Mcmahon, C., and Kruse, A. C. (2018). Structural basis for G protein-coupled receptor signaling. Ann. Rev. Biophys. 47, 1–18. doi: 10.1146/annurev-biophys-070317-032931
Farre-Armengol, G., Penuelas, J., Li, T., Yli-Pirila, P., Filella, I., Llusia, J., et al. (2016). Ozone degrades floral scent and reduces pollinator attraction to flowers. New Phytol. 209, 152–160. doi: 10.1111/nph.13620
Figueroa, D. P., Scott, S., Gonzalez, C. R., Bizama, G., Flores-Mara, R., Bustamante, R., et al. (2020). Estimating the climate change consequences on the potential distribution of Culex pipiens L. 1758, to assess the risk of West Nile virus establishment in Chile. Gayana 84, 46–53.
Fiore, A. M., Jacob, D. J., Bey, I., Yantosca, R. M., Field, B. D., Fusco, A. C., et al. (2002). Background ozone over the United States in summer: origin, trend, and contribution to pollution episodes. J. Geophys. Res. Atmosp. 107:982. doi: 10.1029/2001jd000982
Fonseca, D. M., Keyghobadi, N., Malcolm, C. A., Mehmet, C., Schaffner, F., Mogi, M., et al. (2004). Emerging vectors in the Culex pipiens complex. Science 303, 1535–1538. doi: 10.1126/science.1094247
Foster, W. A. (1995). Mosquito sugar feeding and reproductive energetics. Annu. Rev. Entomol. 40, 443–474. doi: 10.1146/annurev.en.40.010195.002303
Franklinos, L. H. V., Jones, K. E., Redding, D. W., and Abubakar, I. (2019). The effect of global change on mosquito-borne disease. Lancet Infect. Dis. 19, E302–E312. doi: 10.1016/s1473-3099(19)30161-6
Fritz, M. L., Walker, E. D., Miller, J. R., Severson, D. W., and Dworkin, I. (2015). Divergent host preferences of above- and below-ground Culex pipiens mosquitoes and their hybrid offspring. Med. Vet. Entomol. 29, 115–123. doi: 10.1111/mve.12096
Fuentes, J. D., Roulston, T. H., and Zenker, J. Z. (2013). Ozone impedes the ability of a herbivore to find ist host. Environ. Res. Lett. 8:014048.
Ghaninia, M., Majeed, S., Dekker, T., Hill, S. R., and Ignell, R. (2019). Hold your breath - Differential behavioral and sensory acuity of mosquitoes to acetone and carbon dioxide. PLos One 14:e0226815. doi: 10.1371/journal.pone.0226815
Gherlenda, A. N., Esveld, J. L., Hall, A. A. G., Duursma, R. A., and Riegler, M. (2016). Boom and bust: rapid feedback responses between insect outbreak dynamics and canopy leaf area impacted by rainfall and CO2. Glob. Change Biol. 22, 3632–3641. doi: 10.1111/gcb.13334
Giordano, L., Garbelotto, M., Nicolotti, G., and Gonthier, P. (2013). Characterization of fungal communities associated with the bark beetle Ips typographus varies depending on detection method, location, and beetle population levels. Mycol. Prog. 12, 127–140. doi: 10.1007/s11557-012-0822-1
Gleadow, R. M., Foley, W. J., and Woodrow, I. E. (1998). Enhanced CO2 alters the relationship between photosynthesis and defence in cyanogenic Eucalyptus cladocalyx F. Muell. Plant Cell Environ. 21, 12–22. doi: 10.1046/j.1365-3040.1998.00258.x
Gomes, B., Sousa, C. A., Vicente, J. L., Pinho, L., Calderon, I., Arez, E., et al. (2013). Feeding patterns of molestus and pipiens forms of Culex pipiens (Diptera: Culicidae) in a region of high hybridization. Parasit. Vectors 6:93. doi: 10.1186/1756-3305-6-93
Groot, A. T., and Zizzari, Z. V. (2019). Does climate warming influence sexual chemical signaling? Anim. Biol. 69, 83–93. doi: 10.1163/15707563-20191103
Hallmann, C. A., Sorg, M., Jongejans, E., Siepel, H., Hofland, N., Schwan, H., et al. (2017). More than 75 percent decline over 27 years in total flying insect biomass in protected areas. PLos One 12:e0185809. doi: 10.1371/journal.pone.0185809
Hamann, E., Blevins, C., Franks, S. J., Jameel, M. I., and Anderson, J. T. (2021). Climate change alters plant-herbivore interactions. New Phytol. 229, 1894–1910. doi: 10.1111/nph.17036
Harbach, R. E., Dahl, C., and White, G. B. (1985). Culex pipiens Linnaeus (Diptera, Culicidae) - concepts, type designation, and description. Proc. Entomol. Soc. Washington 87, 1–24.
Harbach, R. E., Harrison, B. A., and Gad, A. M. (1984). Culex molestus Forskal (Diptera, Culicidae) - Neotype designation, description, variation, and taxonomic status. Proc. Entomol. Soc. Washington 86, 521–542.
Hauglustaine, D. A., and Brasseur, G. P. (2001). Evolution of tropospheric ozone under anthropogenic activities and associated radiative forcing of climate. J. Geophys. Res. 106, 32337–32360. doi: 10.1029/2001jd900175
Haynes, K. F., Gaston, L. K., Pope, M. M., and Baker, T. C. (1984). Potential for evolution of resistance to pheromones - interindividual and interpopulational variation in chemical communication system of pink-bollworm moth. J. Chem. Ecol. 10, 1551–1565. doi: 10.1007/bf00988424
Hoberg, E. P., and Brooks, D. R. (2015). Evolution in action: climate change, biodiversity dynamics and emerging infectious disease. Philos. Trans. R. Soc. B Biol. Sci. 370:20130553. doi: 10.1098/rstb.2013.0553
Horntvedt, R. C. E., Solheim, H., and Wang, S. (1983). Artificial inoculation with Ips typographus-associated blue stain fungi can kill healthy Norway spruce trees. Skogforsk Meddelelser 38:34.
Ioannidis, P., Simao, F. A., Waterhouse, R. M., Manni, M., Seppey, M., Robertson, H. M., et al. (2017). Genomic features of the damselfly Calopteryx splendens representing a sister clade to most insect orders. Genome Biol. Evol. 9, 415–430. doi: 10.1093/gbe/evx006
Jamieson, M. A., Burkle, L. A., Manson, J. S., Runyon, J. B., Trowbridge, A. M., and Zientek, J. (2017). Global change effects on plant-insect interactions: the role of phytochemistry. Curr. Opin. Insect Sci. 23, 70–80. doi: 10.1016/j.cois.2017.07.009
Jankowiak, R., and Hilszczanski, J. (2005). Ophiostomatoid, fungi associated with Ips typographus (L.) on Picea abies (L.) H. Karst. and Pinus sylvestris L. in north-eastern Poland. Acta Soc. Bot. Poloniae 74, 345–350.
Kandasamy, D., Gershenzon, J., and Hammerbacher, A. (2016). Volatile organic compounds emitted by fungal associates of conifer bark beetles and their potential in bark beetle control. J. Chem. Ecol. 42, 952–969. doi: 10.1007/s10886-016-0768-x
Kandasamy, D., Gershenzon, J., Andersson, M. N., and Hammerbacher, A. (2019). Volatile organic compounds influence the interaction of the Eurasian spruce bark beetle (Ips typographus) with its fungal symbionts. ISME J. 13, 1788–1800. doi: 10.1038/s41396-019-0390-3
Kandasamy, D., Zaman, R., Nakamura, Y., Zhao, T., Hartmann, H., Andersson, M. N., et al. (2021). Bark beetles locate fungal symbionts by detecting volatile fungal metabolites of host tree resin monoterpenes. bioRxiv [Preprint]. 2021.2007.2003.450988, doi: 10.1101/2021.07.03.450988
Kilpatrick, A. M., Kramer, L. D., Jones, M. J., Marra, P. P., Daszak, P., and Fonseca, D. M. (2007). Genetic influences on mosquito feeding behavior and the emergence of zoonotic pathogens. Am. J. Trop. Med. Hygiene 77, 667–671. doi: 10.4269/ajtmh.2007.77.667
Klein, A. M., Vaissiere, B. E., Cane, J. H., Steffan-Dewenter, I., Cunningham, S. A., Kremen, C., et al. (2007). Importance of pollinators in changing landscapes for world crops. Proc. R. Soc. B Biol. Sci. 274, 303–313. doi: 10.1098/rspb.2006.3721
Krokene, P., and Solheim, H. (1998). Assessing the virulence of four bark beetle-associated bluestain fungi using Norway spruce seedlings. Plant Pathol. 47, 537–540. doi: 10.1046/j.1365-3059.1998.00268.x
Kurz, W. A., Dymond, C. C., Stinson, G., Rampley, G. J., Neilson, E. T., Carroll, A. L., et al. (2008). Mountain pine beetle and forest carbon feedback to climate change. Nature 452, 987–990. doi: 10.1038/nature06777
Lawler, I. R., Foley, W. J., Woodrow, I. E., and Cork, S. J. (1996). The effects of elevated co2 atmospheres on the nutritional quality of eucalyptus foliage and its interaction with soil nutrient and light availability. Oecologia 109, 59–68. doi: 10.1007/s004420050058
Leal, H. M., Hwang, J. K., Tan, K. M., and Leal, W. S. (2017). Attraction of Culex mosquitoes to aldehydes from human emanations. Sci. Rep. 7:17965. doi: 10.1038/s41598-017-18406-7
Lee, J. D., Lewis, A. C., Monks, P. S., Jacob, M., Hamilton, J. F., Hopkins, J. R., et al. (2006). Ozone photochemistry and elevated isoprene during the UK heatwave of August 2003. Atmos. Environ. 40, 7598–7613. doi: 10.1016/j.atmosenv.2006.06.057
Leonard, R. J., Pettit, T. J., Irga, P., Mcarthur, C., and Hochuli, D. F. (2019). Acute exposure to urban air pollution impairs olfactory learning and memory in honeybees. Ecotoxicology 28, 1056–1062. doi: 10.1007/s10646-019-02081-7
Leufven, A., Bergstrom, G., and Falsen, E. (1984). Interconversion of verbenols and verbenone by identified yeasts isolated from the spruce bark beete Ips typographus. J. Chem. Ecol. 10, 1349–1361. doi: 10.1007/bf00988116
Lhomme, P., Carrasco, D., Larsson, M., Hansson, B., and Anderson, P. (2018). A context-dependent induction of natal habitat preference in a generalist herbivorous insect. Behav. Ecol. 29, 360–367. doi: 10.1093/beheco/arx173
Li, N., Li, Y. H., Zhang, S. Z., Fan, Y. L., and Liu, T. X. (2017). Effect of elevated CO2 concentration and temperature on antioxidant capabilities of multiple generations of Bemisia tabaci MEAM1 (Hemiptera: Aleyrodidae). J. Insect Physiol. 103, 91–97. doi: 10.1016/j.jinsphys.2017.10.009
Ligsay, A., Telle, O., and Paul, R. (2021). Challenges to mitigating the urban health burden of mosquito-borne diseases in the face of climate change. Int. J. Environ. Res. Public Health 18:5035. doi: 10.3390/ijerph18095035
Linn, C. E., and Roelofs, W. L. (1983). Effect of varying proportions of the alcohol component on sex-pheromone blend discrimination in male oriental fuit moths. Physiol. Entomol. 8, 291–306. doi: 10.1111/j.1365-3032.1983.tb00361.x
Linn, C. E., and Roelofs, W. L. (1985). Response specificity of male pink-bollworm moths to different blends and dosages of sex-pheromone. J. Chem. Ecol. 11, 1583–1590. doi: 10.1007/bf01012203
Linn, C. E., and Roelofs, W. L. (1989). Response specificity of male moths to multicomponent pheromones. Chem. Senses 14, 421–437. doi: 10.1093/chemse/14.3.421
Linn, C. E., Campbell, M. G., and Roelofs, W. L. (1987). Pheromone components and active spaces - what do moths smell and where do they smell it. Science 237, 650–652. doi: 10.1126/science.237.4815.650
Linn, C. E., Campbell, M. G., and Roelofs, W. L. (1988). Temperature modulation of behavioral thresholds controlling male moth sex-pheromone response specificity. Physiol. Entomol. 13, 59–67. doi: 10.1111/j.1365-3032.1988.tb00909.x
Liu, X. L., Zhang, J., Yan, Q., Miao, C. L., Han, W. K., Hou, W., et al. (2020). The molecular basis of host selection in a crucifer-specialized moth. Curr. Biol. 30, 4476–4482. doi: 10.1016/j.cub.2020.08.047
Liu, Y. J., Misztal, P. K., Arata, C., Weschler, C. J., Nazaroff, W. W., and Goldstein, A. H. (2021). Observing ozone chemistry in an occupied residence. Proc. Natl. Acad. Sci. U.S.A. 118:e2018140118. doi: 10.1073/pnas.2018140118
Löfstedt, C. (1990). Population variation and genetic control of pheromone communication systems in moths. Entomol. Exp. Appl. 54, 199–218.
Logan, J. A., Macfarlane, W. W., and Willcox, L. (2010). Whitebark pine vulnerability to climate-driven mountain pine beetle disturbance in the Greater Yellowstone Ecosystem. Ecol. Appl. 20, 895–902. doi: 10.1890/09-0655.1
Lu, T., Qiu, Y. T., Wang, G., Kwon, J. Y., Rutzler, M., Kwon, H. W., et al. (2007). Odor coding in the maxillary palp of the malaria vector mosquito Anopheles gambiae. Curr. Biol. 17, 1533–1544. doi: 10.1016/j.cub.2007.07.062
Lusebrink, I., Girling, R. D., Farthing, E., Newman, T. A., Jackson, C. W., and Poppy, G. M. (2015). The effects of diesel exhaust pollution on floral volatiles and the consequences for honey bee olfaction. J. Chem. Ecol. 41, 904–912. doi: 10.1007/s10886-015-0624-4
Majeed, S., Hill, S. R., and Ignell, R. (2014). Impact of elevated CO2 background levels on the host-seeking behaviour of Aedes aegypti. J. Exp. Biol. 217, 598–604. doi: 10.1242/jeb.092718
Majeed, S., Hill, S. R., Birgersson, G., and Ignell, R. (2016). Detection and perception of generic host volatiles by mosquitoes modulate host preference: context dependence of (R)-1-octen-3-ol. R. Soc. Open Sci. 3:160467. doi: 10.1098/rsos.160467
Majeed, S., Hill, S. R., Dekker, T., and Ignell, R. (2017). Detection and perception of generic host volatiles by mosquitoes: responses to CO2 constrains host-seeking behaviour. R. Soc. Open Sci. 4:170189 doi: 10.1098/rsos.170189
Mansourian, S., Enjin, A., Jirle, E. V., Ramesh, V., Rehermann, G., Becher, P. G., et al. (2018). Wild African Drosophila melanogaster are seasonal specialists on marula fruit. Curr. Biol. 28:3960. doi: 10.1016/j.cub.2018.10.033
Markow, T. A., and Toolson, E. C. (1990). “Temperature effects on epicuticular hydrocarbons and sexual isolation in Drosophila mojavensis,” in Ecological and Evolutionary Genetics of Drosophila Plenum, eds J. S. F. Barker, W. T. Starmer, and R. J. MacIntyre (New York, NY: Springer), 315–331. doi: 10.1007/978-1-4684-8768-8_21
McFrederick, Q. S., Kathilankal, J. C., and Fuentes, J. D. (2008). Air pollution modifies floral scent trails. Atmos. Environ. 42, 2336–2348. doi: 10.1016/j.atmosenv.2007.12.033
McKenzie, S. K., Fetter-Pruneda, I., Ruta, V., and Kronauer, D. J. C. (2016). Transcriptomics and neuroanatomy of the clonal raider ant implicate an expanded clade of odorant receptors in chemical communication. Proc. Natl. Acad. Sci. U.S.A. 113, 14091–14096. doi: 10.1073/pnas.1610800113
Messina, J. P., Brady, O. J., Pigott, D. M., Golding, N., Kraemer, M. U. G., Scott, T. W., et al. (2015). The many projected futures of dengue. Nat. Rev. Microbiol. 13, 230–239. doi: 10.1038/nrmicro3430
Missbach, C., Dweck, H. K. M., Vogel, H., Vilcinskas, A., Stensmyr, M. C., Hansson, B. S., et al. (2014). Evolution of insect olfactory receptors. Elife 3:e02115. doi: 10.7554/eLife.02115
Morris, R. H., Counsell, S. J., Mcgonnell, I. M., and Thornton, C. (2021). Early life exposure to air pollution impacts neuronal and glial cell function leading to impaired neurodevelopment. Bioessays 43:2000288. doi: 10.1002/bies.202000288
Olivier, J. G. J., Bouwman, A. F., Hoek, K. W. V. D., and Berdowski, J. J. M. (1998). Global air emission inventories for Anthropogenic sources of NOx, NH3 and N2O in 1990. Environ. Pollut. 102, 135–148. doi: 10.1016/b978-0-08-043201-4.50024-1
Omondi, W. P., Owino, E. A., Odongo, D., Mwangangi, J. M., Torto, B., and Tchouassi, D. P. (2019). Differential response to plant- and human-derived odorants in field surveillance of the dengue vector, Aedes aegypti. Acta Trop. 200:105163. doi: 10.1016/j.actatropica.2019.105163
Ono, T. (1993). Effect of rearing temperature on pheromone component ratio in potato tuberworm moth. Phthorimaea operculella (Lepidoptera, Gelechiidae). J. Chem. Ecol. 19, 71–81. doi: 10.1007/bf00987472
Ono, T. (1994). Effect of temperature on biosynthesis of sex-pheromone components in potato tuberworm moth Phthorimaea operculella (Lepidoptera. Gelechiidae). J. Chem. Ecol. 20, 2733–2741. doi: 10.1007/bf02036204
Osorio, H. C., Ze-Ze, L., Amaro, F., Nunes, A., and Alves, M. J. (2014). Sympatric occurrence of Culex pipiens (Diptera. Culicidae) biotypes pipiens, molestus and their hybrids in Portugal, Western Europe: feeding patterns and habitat determinants. Med. Vet. Entomol. 28, 103–109. doi: 10.1111/mve.12020
Phillips, B. B., Bullock, J. M., Gaston, K. J., Hudson-Edwards, K. A., Bamford, M., Cruse, D., et al. (2021). Impacts of multiple pollutants on pollinator activity in road verges. J. Appl. Ecol. 58, 1017–1029. doi: 10.1111/1365-2664.13844
Pincebourde, S., Van Baaren, J., Rasmann, S., Rasmont, P., Rodet, G., Martinet, B., et al. (2017). “Plant-insect interactions in a changing world,” in Insect-Plant Interactions in a Crop Protection Perspective, eds N. Sauvion, D. Thiery, and P. A. Calatayud (Amsterdam: Elsevier), 289–332. doi: 10.1016/bs.abr.2016.09.009
Proffit, M., Khallaf, M. A., Carrasco, D., Larsson, M. C., and Anderson, P. (2015). ‘Do you remember the first time?’. Host plant preference in a moth is modulated by experiences during larval feeding and adult mating. Ecol. Lett. 18, 365–374. doi: 10.1111/ele.12419
Raffa, K. F., Andersson, M. N., and Schlyter, F. (2016). “Host selection by bark beetles: Playing the odds in a high-stakes game,” in Pine Bark Beetles, eds C. Tittiger and G. J. Blomquist (Cambridge, MA: Academic Press), 1–74. doi: 10.1016/bs.aiip.2016.02.001
Raffa, K. F., Powell, E. N., and Townsend, P. A. (2013). Temperature-driven range expansion of an irruptive insect heightened by weakly coevolved plant defenses. Proc. Natl. Acad. Sci. U.S.A. 110, 2193–2198. doi: 10.1073/pnas.1216666110
Raina, A. K. (2003). Pheromone production in corn earworm: effect of temperature and humidity. Southwest. Entomol. 28, 115–120.
Reinhold, J. M., Lazzari, C. R., and Lahondere, C. (2018). Effects of the environmental temperature on Aedes aegypti and Aedes albopictus mosquitoes: a review. Insects 9:158. doi: 10.3390/insects9040158
Riffell, J. A., Shlizerman, E., Sanders, E., Abrell, L., Medina, B., Hinterwirth, A. J., et al. (2014). Flower discrimination by pollinators in a dynamic chemical environment. Science 344, 1515–1518. doi: 10.1126/science.1251041
Robinson, E. A., Ryan, G. D., and Newman, J. A. (2012). A meta-analytical review of the effects of elevated CO2 on plant-arthropod interactions highlights the importance of interacting environmental and biological variables. New Phytol. 194, 321–336. doi: 10.1111/j.1469-8137.2012.04074.x
Rocklov, J., and Dubrow, R. (2020). Climate change: an enduring challenge for vector-borne disease prevention and control. Nat. Immunol. 21, 479–483. doi: 10.1038/s41590-020-0648-y
Rose, N. H., Sylla, M., Badolo, A., Lutomiah, J., Ayala, D., Aribodor, O. B., et al. (2020). Climate and urbanization drive mosquito preference for humans. Curr. Biol. 30:3570. doi: 10.1016/j.cub.2020.06.092
Rosvik, A., Lhomme, P., Khallaf, M. A., and Anderson, P. (2020). Plant-induced transgenerational plasticity affecting performance but not preference in a polyphagous moth. Front. Ecol. Evol. 8:598395. doi: 10.3389/fevo.2020.598395
Ruybal, J. E., Kramer, L. D., and Kilpatrick, A. M. (2016). Geographic variation in the response of Culex pipiens life history traits to temperature. Parasit. Vectors 9:116. doi: 10.1186/s13071-016-1402-z
Sachse, S., Rueckert, E., Keller, A., Okada, R., Tanaka, N. K., Ito, K., et al. (2007). Activity-dependent plasticity in an olfactory circuit. Neuron 56, 838–850. doi: 10.1016/j.neuron.2007.10.035
Sambaraju, K. R., and Goodsman, D. W. (2021). Mountain pine beetle: an example of a climate-driven eruptive insect impacting conifer forest ecosystems. CAB Rev. 16, 1–18.
Sato, K., Pellegrino, M., Nakagawa, T., Nakagawa, T., Vosshall, L. B., and Touhara, K. (2008). Insect olfactory receptors are heteromeric ligand-gated ion channels. Nature 452, 1002–U1009. doi: 10.1038/nature06850
Schiebe, C., Hammerbacher, A., Birgersson, G., Witzell, J., Brodelius, P. E., Gershenzon, J., et al. (2012). Inducibility of chemical defenses in Norway spruce bark is correlated with unsuccessful mass attacks by the spruce bark beetle. Oecologia 170, 183–198. doi: 10.1007/s00442-012-2298-8
Schlyter, F., Birgersson, G., and Leufven, A. (1989). Inhibition of attraction to aggregation pheromone by verbenone and ipsenol - density regulation mechanisms in bark beetle. Ips typographus. J. Chem. Ecol. 15, 2263–2277. doi: 10.1007/bf01014114
Seibold, S., Gossner, M. M., Simons, N. K., Bluthgen, N., Muller, J., Ambarli, D., et al. (2019). Arthropod decline in grasslands and forests is associated with landscape-level drivers. Nature 574:671. doi: 10.1038/s41586-019-1684-3
Sharma, Y. K., and Davis, K. R. (1994). Ozone-induced expression of stress-related genes in Arabidopis thaliana. Plant Physiol. 105, 1089–1096. doi: 10.1104/pp.105.4.1089
Sinka, M. E., Pironon, S., Massey, N. C., Longbottom, J., Hemingway, J., Moyes, C. L., et al. (2020). A new malaria vector in Africa: predicting the expansion range of Anopheles stephensi and identifying the urban populations at risk. Proc. Natl. Acad. Sci. U.S.A. 117, 24900–24908. doi: 10.1073/pnas.2003976117
Six, D. L., and Bentz, B. J. (2007). Temperature determines symbiont abundance in a multipartite bark beetle-fungus ectosymbiosis. Microb. Ecol. 54, 112–118. doi: 10.1007/s00248-006-9178-x
Smith, G. P., Johnson, C. A., Davidowitz, G., and Bronstein, J. L. (2018). Linkages between nectaring and oviposition preferences of Manduca sexta on two co-blooming Datura species in the Sonoran Desert. Ecol. Entomol. 43, 85–92. doi: 10.1111/een.12475
Solheim, H. (1991). Oxygen deficiency and spruce resin inhibition of growth of blue stain fungi associated with Ips typographus. Mycol. Res. 95, 1387–1392. doi: 10.1016/s0953-7562(09)80390-0
Sprengelmeyer, Q. D., Mansourian, S., Lange, J. D., Matute, D. R., Cooper, B. S., Jirle, E. V., et al. (2020). Recurrent collection of Drosophila melanogaster from wild African environments and genomic insights into species history. Mol. Biol. Evol. 37, 627–638. doi: 10.1093/molbev/msz271
Steinbauer, M. J., Schiestl, F. P., and Davies, N. W. (2004). Monoterpenes and epicuticular waxes help female autumn gum moth differentiate between waxy and glossy Eucalyptus and leaves of different ages. J. Chem. Ecol. 30, 1117–1142. doi: 10.1023/b:joec.0000030267.75347.c1
Takken, W., and Knols, B. G. J. (1999). Odor-mediated behavior of Afrotropical malaria mosquitoes. Annu. Rev. Entomol. 44, 131–157. doi: 10.1146/annurev.ento.44.1.131
Tang, C. F., Davis, K. E., Delmer, C., Yang, D., and Wills, M. A. (2018). Elevated atmospheric CO2 promoted speciation in mosquitoes (Diptera, Culicidae). Commun. Biol. 1, 1–8. doi: 10.1038/s42003-018-0191-7
Tang, X. C., Misztal, P. K., Nazaroff, W. W., and Goldstein, A. H. (2016). Volatile organic compound emissions from humans indoors. Environ. Sci. Technol. 50, 12686–12694. doi: 10.1021/acs.est.6b04415
Tanin, S. M., Kandasamy, D., and Krokene, P. (2021). Fungal interactions and host tree preferences in the spruce bark beetle Ips typographus. Front. Microbiol. 12:695167. doi: 10.3389/fmicb.2021.695167
Thoming, G., Larsson, M. C., Hansson, B. S., and Anderson, P. (2013). Comparison of plant preference hierarchies of male and female moths and the impact of larval rearing hosts. Ecology 94, 1744–1752. doi: 10.1890/12-0907.1
Turner, S. L., Li, N., Guda, T., Githure, J., Carde, R. T., and Ray, A. (2011). Ultra-prolonged activation of CO2-sensing neurons disorients mosquitoes. Nature 474, U87–U114. doi: 10.1038/nature10081
UN Department of Economic and Social Affairs. (2018). Revision of World Urbanization Prospects. Available online at: https://www.un.org/development/desa/publications/2018
van Klink, R., Bowler, D. E., Gongalsky, K. B., Swengel, A. B., Gentile, A., and Chase, J. M. (2020). Meta-analysis reveals declines in terrestrial but increases in freshwater insect abundances. Science 368, 417–420. doi: 10.1126/science.aax9931
Vanderplanck, M., Lapeyre, B., Brondani, M., Opsommer, M., Dufay, M., Hossaert-Mckey, M., et al. (2021). Ozone pollution alters olfaction and behavior of pollinators. Antioxidants 10:636. doi: 10.3390/antiox10050636
Vingarzan, R. (2004). A review of surface ozone background levels and trends. Atmos. Environ. 38, 3431–3442. doi: 10.1016/j.atmosenv.2004.03.030
Vinogradova, A. N. (2000). Culex Pipiens Pipiens Mosquitoes:Taxonomy, Distribution, Ecology, Physiology, Genetics, Applied Importance And Control. Sofia: Pensoft Publishers, 222.
White, B. J., Collins, F. H., and Besansky, N. J. (2011). “Evolution of Anopheles gambiae in relation to humans and malaria,” in Annual Review of Ecology, Evolution, and Systematics, Vol. 42, eds D. J. Futuyma, H. B. Shaffer, and D. Simberloff (Fort Worth, TX: BRIT Press), 111–132. doi: 10.1146/annurev-ecolsys-102710-145028
Wicher, D., Schafer, R., Bauernfeind, R., Stensmyr, M. C., Heller, R., Heinemann, S. H., et al. (2008). Drosophila odorant receptors are both ligand-gated and cyclic-nucleotide-activated cation channels. Nature 452, 1007–U1010. doi: 10.1038/nature06861
Wondwosen, B., Birgersson, G., Seyoum, E., Tekie, H., Torto, B., Fillinger, U., et al. (2016). Rice volatiles lure gravid malaria mosquitoes, Anopheles arabiensis. Sci. Rep. 6:37930. doi: 10.1038/srep37930
Wondwosen, B., Birgersson, G., Tekie, H., Torto, B., Ignell, R., and Hill, S. R. (2018). Sweet attraction: sugarcane pollen-associated volatiles attract gravid Anopheles arabiensis. Malar. J. 17:90. doi: 10.1186/s12936-018-2245-1
Wondwosen, B., Dawit, M., Debebe, Y., Tekie, H., Hill, S. R., and Ignell, R. (2021). Development of a chimeric odour blend for attracting gravid malaria vectors. Malar. J. 20:262. doi: 10.1186/s12936-021-03797-w
Wondwosen, B., Hill, S. R., Birgersson, G., Seyoum, E., Tekie, H., and Ignell, R. (2017). A(maize)ing attraction: gravid Anopheles arabiensis are attracted and oviposit in response to maize pollen odours. Malar. J. 16:39. doi: 10.1186/s12936-016-1656-0
Ye-Ebiyo, Y., Pollack, R. J., and Spielman, A. (2000). Enhanced development in nature of larval Anopheles arabiensis mosquitoes feeding on maize pollen. Am. J. Trop. Med. Hygiene 63, 90–93. doi: 10.4269/ajtmh.2000.63.90
Zacarias, D. A. (2020). Global bioclimatic suitability for the fall armyworm. Spodoptera frugiperda (Lepidoptera: Noctuidae), and potential co-occurrence with major host crops under climate change scenarios. Clim. Change 161, 555–566. doi: 10.1007/s10584-020-02722-5
Zakir, A., Bengtsson, M., Sadek, M. M., Hansson, B. S., Witzgall, P., and Anderson, P. (2013). Specific response to herbivore-induced de novo synthesized plant volatiles provides reliable information for host plant selection in a moth. J. Exp. Biol. 216, 3257–3263. doi: 10.1242/jeb.083188
Zavala, J. A., Gog, L., and Giacometti, R. (2017). Anthropogenic increase in carbon dioxide modifies plant-insect interactions. Ann. Appl. Biol. 170, 68–77. doi: 10.1111/aab.12319
Zhao, T., Kandasamy, D., Krokene, P., Chen, J. Y., Gershenzon, J., and Hammerbacher, A. (2019). Fungal associates of the tree-killing bark beetle. Ips typographus, vary in virulence, ability to degrade conifer phenolics and influence bark beetle tunneling behavior. Fungal Ecol. 38, 71–79. doi: 10.1016/j.funeco.2018.06.003
Keywords: insect, pollutants, ozone, nitric oxides, global warming
Citation: Knaden M, Anderson P, Andersson MN, Hill SR, Sachse S, Sandgren M, Stensmyr MC, Löfstedt C, Ignell R and Hansson BS (2022) Human Impacts on Insect Chemical Communication in the Anthropocene. Front. Ecol. Evol. 10:791345. doi: 10.3389/fevo.2022.791345
Received: 08 October 2021; Accepted: 23 February 2022;
Published: 22 March 2022.
Edited by:
Tao Li, Sichuan University, ChinaReviewed by:
Martin James Steinbauer, La Trobe University, AustraliaNicolas Montagné, Sorbonne Universités, France
Copyright © 2022 Knaden, Anderson, Andersson, Hill, Sachse, Sandgren, Stensmyr, Löfstedt, Ignell and Hansson. This is an open-access article distributed under the terms of the Creative Commons Attribution License (CC BY). The use, distribution or reproduction in other forums is permitted, provided the original author(s) and the copyright owner(s) are credited and that the original publication in this journal is cited, in accordance with accepted academic practice. No use, distribution or reproduction is permitted which does not comply with these terms.
*Correspondence: Bill S. Hansson, aGFuc3NvbkBpY2UubXBnLmRl
†These authors have contributed equally to this work