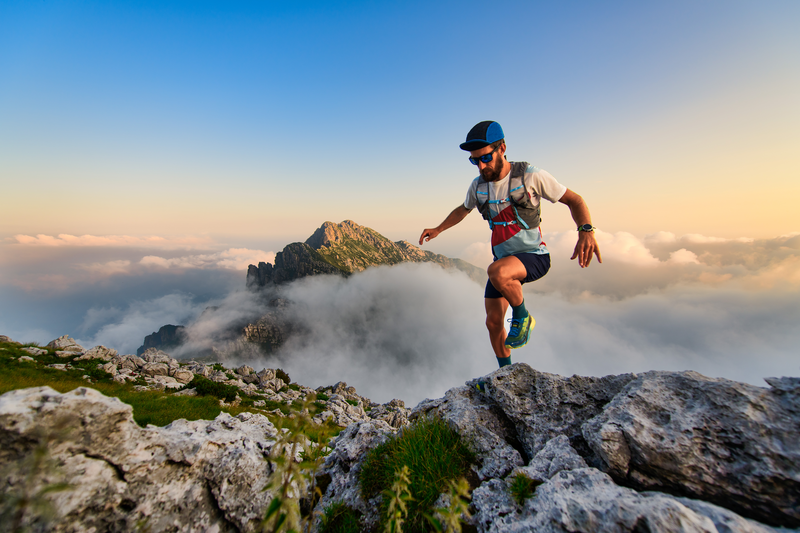
94% of researchers rate our articles as excellent or good
Learn more about the work of our research integrity team to safeguard the quality of each article we publish.
Find out more
ORIGINAL RESEARCH article
Front. Ecol. Evol. , 17 February 2022
Sec. Biogeography and Macroecology
Volume 10 - 2022 | https://doi.org/10.3389/fevo.2022.788184
This article is part of the Research Topic The Role of Rivers in the Origins, Evolution, Adaptation, and Distribution of Biodiversity View all 13 articles
Abiotic and biotic factors drive compositional differences among local species assemblages. Determining the influence of different drivers on beta diversity patterns can provide insights into processes governing community organization. Examining beta diversity patterns along taxonomic, phylogenetic and functional dimensions enables a nuanced understanding of underlying processes that govern community assembly and dynamics. The dynamic and complex riparian habitats in the Himalaya, and the hyper-diverse riverine bird community offer a fascinating setup to examine the role of environmental factors in influencing community structuring. Using a large dataset on river bird communities from field census across multiple drainages in the Indian Himalaya, we aimed at discerning processes that structure these communities through an understanding of pair-wise dissimilarities in species composition across sites. We determined the relative contributions of turnover and nestedness in taxonomic, phylogenetic, and functional beta diversity patterns in the Eastern and Western Himalaya that differ in species richness. Generalized Dissimilarity Modeling was used to examine the relative contributions of climatic, geographic, and anthropogenic factors toward explaining different metrics of beta diversity. The riverine bird communities in the drier and seasonal Western Himalaya were poorer in species richness, more phylogenetically and functionally clustered than that in the Eastern Himalaya. The contribution of the turnover component to the overall beta diversity was higher than the nestedness component in river bird communities, particularly in the Eastern Himalaya. Habitat and climatic factors differentially influenced the beta diversity patterns in both Eastern and Western Himalaya, with river width consistently explaining a large variation in beta diversity in the east and the west. The results show that environmental filtering plays a crucial role in structuring riverine bird communities in the Himalayan headwaters, highlighting the need to ameliorate the threats posed by the slew of hydroelectric projects and forest loss in the region.
Understanding patterns of community assembly and identifying underlying ecological processes across geographic gradients is a predominant theme in theoretical and applied ecology (Diamond, 1975; Vellend, 2010). Species diversity is shaped by current and historical conditions that include a range of environmental and geographical factors (Mittelbach, 2012). Traditionally, variation in species diversity has been examined at local (α-diversity) and regional (γ-diversity) scales; however, in the last three decades, variation in species diversity among sites (β-diversity) has also gained considerable attention (Harrison et al., 1992; Lennon et al., 2001; Soininen et al., 2007; Baselga, 2010; Legendre, 2014). Beta diversity is a measure of turnover in species diversity among sites, habitats, or along gradients (Whittaker, 1960). Beta diversity measures can be classified into variance-based and diversity partitioning-based metrics (Koleff et al., 2003; Legendre, 2014; Matthews et al., 2019). Availability of species-site matrix (as in the case of our study) enables the estimation of variance-based beta diversity metric (Matthews et al., 2019), which further allows us to determine the relative influence of “nestedness” and “turnover” components on compositional differences (Baselga, 2010).
Determining the processes that drive the variation in community compositional across sites is a fundamental challenge (Ricklefs, 2004). Past (e.g., historical climate and geographic barriers) and contemporary niche-based factors (e.g., climate, habitat complexity, and biotic interactions) and other factors (e.g., geographic distance, geometric constraints, and land-use change) may influence beta diversity patterns (Nekola and White, 1999; Ricklefs, 2004; Trøjelsgaard et al., 2015; Newbold et al., 2016; Barnagaud et al., 2017). The predictors that influence beta diversity patterns are categorized under niche-based processes or neutral processes (Hubbell, 2001; Chase and Myers, 2011). Deterministic niche-based processes emphasize variation in environmental gradients, where species are assembled through environmental filtering or biotic interactions, while neutral processes highlight the roles of dispersal limitation and genetic drift (Hubbell, 2001; Chase and Myers, 2011). For example, compositional differences in communities could be caused by geographic barriers that may prevent the dispersal of species, the absence of specific environmental factors (e.g., microhabitats) that are preferred by certain species, or the presence of superior competitors/predators. For instance, human induced land-use changes may filter species, especially habitat specialists, resulting in compositional changes across natural and human-modified landscapes (McKinney, 2006; Newbold et al., 2016; Barnagaud et al., 2017). Hence, identifying the drivers of beta diversity patterns can help explain the roles of historical processes (Fitzpatrick et al., 2013) and current environmental and anthropogenic activities (Capinha et al., 2015).
Studies on beta diversity often focus on variation in taxonomic composition across communities. These assessments assume that all species in an assemblage are similar in terms of the role they play in ecosystems. When they do so, they neglect the role of functionality in assemblage structure and ecosystem functioning (Villéger et al., 2008). Furthermore, the importance of examining functional and phylogenetic beta diversity is increasingly being acknowledged (McGill et al., 2006; Anderson et al., 2011; Branco et al., 2020; Qian et al., 2020). Functional traits may be responsible for the species’ ecological role, interactions among species, and the interaction among species and its environment (Petchey and Gaston, 2006; Davies et al., 2007). Functional beta diversity measures are based on quantifying differences between species with respect to morphological, physiological, and behavioral traits that drive functional diversity. Phylogenetic measures explicitly integrate information on the evolutionary history of the species in a community and allow for incorporating information on trait conservatism (Webb et al., 2002; Cadotte et al., 2009). Complementary information provided by these measures enable a more comprehensive understanding of the ecological and evolutionary processes driving diversity patterns (Graham and Fine, 2008; Cavender-Bares et al., 2009).
The global plight of rivers has generated significant interest in documenting biodiversity patterns and determining the effects of environmental change on riverine biodiversity (Dudgeon et al., 2006). High-energy river systems in mountainous landscapes, with wide altitudinal ranges, complex topography, and geo-morphological dynamism provide diverse habitats into which species have proliferated (Ormerod et al., 1994; Townsend and Hildrew, 1994; Villéger et al., 2013; Branco et al., 2020). However, our understanding of beta diversity patterns in these riverine systems is relatively poor (Ormerod et al., 1994; Jacobsen et al., 1997). Although riverine habitats comprise a relatively small proportion of mountainous landscapes, they provide unique niches for avian species from surrounding forested areas (Palmer and Bennett, 2006; Sullivan et al., 2007; Sinha et al., 2019b). Many riverine birds specialize in aquatic and riparian resources and depend on these linear habitats for their survival (Buckton and Ormerod, 2002).
The Himalayan mountain range has among the highest diversity of birds in the world with species richness peaking in the Eastern Himalaya (henceforth, E Himalaya). Past climatic factors (such as extensive snow cover) and increasingly drier and seasonal environment in the Western Himalaya (henceforth, W Himalaya) are thought to be among the important factors driving differences in bird diversity between W and E Himalaya. Among riverine birds too, E Himalaya has the highest diversity of river birds globally (Buckton and Ormerod, 2002; Srinivasan et al., 2014). The W Himalaya is comparatively species poor (Sinha, 2021). The differences in species richness and environment offer an excellent opportunity to determine the role of environmental filtering (vis-à-vis other factors) in driving beta diversity patterns. While studies in the W and Central Himalaya have examined species-habitat relationships (Manel et al., 1999; Buckton and Ormerod, 2008; Sinha et al., 2019a,b), studies from the E Himalaya remain restricted to few studies providing species-specific information (Menzies et al., 2021). Hence, our understanding of the drivers of regional community composition and beta diversity patterns of river bird assemblages remains limited (Manel et al., 2000; Buckton and Ormerod, 2008).
Previous studies on avian assemblages in the Himalaya have emphasized the roles of temperature seasonality in shaping bird communities in the E and W Himalaya (Srinivasan et al., 2019). Birds are susceptible to changes in habitat structure due to human land use at both local and landscape scales (Tscharntke et al., 2005; Larsen et al., 2010). Variation in river topography related variables like river width, depth and flow influence community assembly processes through different pathways (Royan, 2015). Regulation of river flow is the most damaging impact on riverine systems resulting from anthropogenic activities (Dynesius and Nilsson, 1994), which is particularly evident in the developing countries of the Indian subcontinent (Grumbine and Pandit, 2013). With approximately 300 dams across 90% of the Indian Himalayan valleys, severe impacts on biodiversity, including species extinctions, are to be expected (Pandit and Grumbine, 2012).
In this study, we present data on river bird communities from field censuses across multiple drainages in the Himalaya. We assessed different aspects of avian diversity in the E and W Himalaya to (1) compare patterns in taxonomic, functional, and phylogenetic beta diversity; (2) determine the relative contributions of turnover and nestedness to beta diversity; and (3) examine the relative contributions of climatic, geographic, and anthropogenic factors on beta diversity. Given that W Himalaya has a distinctly drier climate, we expect its riverine bird communities to be more clustered than those in the E Himalaya, owing to the more substantial role of environmental filtering. Given the higher diversity of riverine birds in the E Himalaya when compared to the W Himalaya, we expect a more significant role of turnover (compared to nestedness) in the E Himalaya indicating the role of biotic processes in structuring riverine bird communities. If climate-, habitat-, and human-related factors influence beta diversity patterns, it would indicate the role of environmental filtering in structuring riverine bird communities. Whereas if geographic distance influences beta diversity patterns, it would indicate the role of dispersal limitation in structuring river bird communities.
The Himalaya spread over 3,200 km across seven countries, representing the tallest and youngest mountain range in the world. Western Himalaya extends from Pakistan to north-west India (west of Nepal), while E Himalaya extends from eastern Nepal to northern Myanmar (Rodgers et al., 2000). It has high biodiversity value as recognized by its four biodiversity hotspots, 60 ecoregions and 330 important bird areas (Myers et al., 2000). Riverine areas in the Himalaya harbor the highest diversity of specialist riverine birds in the world, among which several species are of conservation concern (Buckton, 1998; Sinha et al., 2019a; Menzies et al., 2021).
We conducted field surveys in the states of Arunachal Pradesh and Uttarakhand in the Indian part of the E and W Himalaya, respectively (Figure 1). Arunachal Pradesh is part of the Eastern Himalaya Biodiversity Hotspot, sharing its borders with Myanmar, China, and Bhutan (Mittermeier et al., 2011). It is drained by many rivers, such as the Noa Dehing, Kamlang, Lohit, Dibang, Siang, Subansiri, and Kameng which are important headwaters to the river Brahmaputra. In the west, Uttarakhand shares international borders with Nepal and China and is drained by torrential snow-melt rivers such as the Bhagirathi, Alaknanda, Mandakini, Pindar, Kosi, and Kali, several of which are headwaters to the Ganges. In the areas sampled in the E Himalaya, the vegetation is dominated by tropical and subtropical broadleaved evergreen forests. In the W Himalaya, the vegetation in riparian areas consists of conifers at higher elevations and subtropical vegetation at the foothills (Gaur et al., 2019).
Figure 1. Map showing the study area in the Indian Himalaya. Blue rectangles show the location of political states in the east and west where fieldwork was conducted and circles in red show locations of river stretches sampled. Eighty-one river reaches were sampled in the E Himalaya (Arunachal Pradesh) and 53 in the W Himalaya (Uttarakhand).
Following Buckton (1998), we sampled river-dependent birds by walking along river banks and recording the number of individuals of different bird species detected. In the E Himalaya, we sampled 81 sites of variable lengths (average length = 1,285 m; range = 300 m–2 km; total effort = 101.3 km) across seven river drainages encompassing a wide elevational gradient of 60–2,000 m asl (Figure 1). In the W Himalaya, we sampled 53 river reaches of equal length (500 m; total effort = 26.5 km) in the Bhagirathi (main river and six first-order streams) and Amrut Ganga basins, important headstreams of the Upper Ganges, between an elevation gradient of 330–3,100 m asl. Surveys were carried out post-monsoon (mainly covering winter months) when several of the river bird species migrate to lower altitudes. Conducting surveys on foot during the monsoons is not feasible or safe since the rivers swell and prevent crossing for long stretches. Sampling was conducted between September to late January (2016–2018) in the W Himalaya and between late August to early March (2017–2019) in the E Himalaya. All species’ nomenclature follows the Clements Checklist by the Cornell Lab of Ornithology (Clements, 2007). For more details on the field survey design, please see Menzies et al. (2021) for the E Himalaya and Sinha et al. (2019a) for the W Himalaya.
We built a phylogenetic tree of 39 bird species recorded in our field surveys by pruning the global bird phylogenetic tree obtained from www.birdtree.org (Jetz et al., 2012). The original tree in Jetz et al. (2012) was assimilated from genetic data of 6,693 species of extant birds. The backbone of the tree was constructed using 15 genes (19 loci) of 151 key species and time-calibrated with 10 well-known fossils (Jetz et al., 2012). A total of 10,000 trees were sub-sampled for our target species using a pseudo-posterior distribution1 to obtain 100 trees, which were used to prepare a consensus tree used in further analysis. Packages “ape” (Paradis et al., 2004) and “phytools” (Revell, 2012) were used to construct a phylogenetic tree of the species recorded in the field.
We obtained data on the trophic level, foraging niche, and morphometry from Supplementary Dataset 1 in Pigot et al. (2020). Birds were classified into four trophic guilds (herbivore, carnivore, omnivore, and scavenger) and 10 foraging niches (e.g., aquatic dive, aquatic ground, aquatic perch, ground) (Pigot et al., 2020). For morphological trait data, we used the principal component scores reported for the overall body traits (comprising data on body mass, beak, wing, tail, and tarsus). For overall body traits, we used principal component scores of the first eight axes, which explained 99.9% of the variation among 9,963 bird species. Please see Pigot et al. (2020) for additional details on the functional trait data.
Global patterns in species richness of river specialist birds highlight the role of net primary productivity (NPP) and temperature in shaping species richness patterns (Buckton and Ormerod, 2002). We downloaded net primary productivity data from the Terra Net primary productivity database from MODIS.2 We obtained temperature seasonality (bio4) and annual precipitation (bio12) data from WorldClim (Fick and Hijmans, 2017). The recently developed human modification raster layer from Kennedy et al. (2019) and the forest cover data from Global Forest Watch3 and its extracted values using a 500 m buffer along the surveyed river reaches were used in this study. We kept the buffer radius short as the life history parameters of the focal bird species are strictly tied to the riparian habitat (Buckton and Ormerod, 2008). We recorded elevation and river width at survey locations during fieldwork.
To understand beta diversity patterns in river bird communities, we calculated taxonomic, phylogenetic, and functional diversity values between each pair of sites (81 sites in the east and 53 sites in the west). For this analysis, we did not use abundance-weighted diversity measures to control for variable sampling length of river reaches in the field. We used species richness values of each site to calculate taxonomic diversity and used Sorensen’s dissimilarity matrix as implemented by the function “vegdist” in package “vegan” to calculate taxonomic beta diversity (Oksanen et al., 2019).
For phylogenetic and functional diversity, we used two dispersion metrics—mean pair-wise distance (MPD) and mean nearest taxon distance (MNTD)—which are calculated using the variation among traits or tips in a phylogeny, thus aiding in understanding the role of deterministic processes. MPD calculates the pair-wise distance between each species (including conspecifics) in the community and is independent of the species richness (Webb et al., 2002). MNTD calculates the distance to the closest non-conspecific relative and is hence dependent on the number of species in a community (Webb et al., 2002). While MPD is based on the overall structure of the tree, MNTD is based on the architecture of the tree near its terminals. Different drivers may influence basal and terminal architecture of the trees differentially, thus necessitating the use of the two measures. For functional diversity, we created a tree using the principal component scores for the different species associated with the overall phenotype (described above) from the global bird-trait dataset (Pigot et al., 2020); we did this using the UPGMA clustering method in package “phangorn” (Schliep, 2011).
MPD and MNTD were calculated for the phylogenetic and functional diversity of species assemblages in the E and W Himalaya separately. To examine the changes in phylogenetic and functional MPD and MNTD, we calculated the cophenetic distance using the function “cophenetic” in package “vegan” (Oksanen et al., 2019) and created a site-wise dissimilarity measure using phylogenetic and functional tree. MPD and MNTD were calculated as Bray-Curtis distance using functions “comdist” and “comdistnt,” respectively, in package “picante” (Kembel et al., 2010). Additionally, we calculated the standardized effect sizes (SES) of MPD and MNTD for both the phylogenetic and functional diversity using established methods (Webb et al., 2002). We compared the observed estimates with the null distribution (n = 1,000 randomizations) while controlling for species richness. SES are informative as they make measures such as MNTD, which are sensitive to species richness, comparable. Negative values of SES indicate clustering (species are more closely related) in the community while positive values indicate overdispersion (species are more distantly related) (Webb et al., 2002; Kembel et al., 2010). We used a Mann-Whitney U-test to compare the different diversity measures across the E and the W Himalaya.
Total beta diversity was partitioned into its two constituent components—dissimilarity due to turnover (βturn) and nestedness (βnest) using the function “beta.pair” from the package “betapart” (Baselga and Orme, 2012). While turnover is the proportion of dissimilarity between a pair of sites due to the replacement of species, nestedness is the proportion of dissimilarity owing to species in a site being a nested subset of the other due to the addition or removal of species.
We used a non-linear regression-based method of Generalized Dissimilarity Modeling (GDM) (Ferrier et al., 2007) to model the taxonomic, phylogenetic, and functional beta diversity. Pair-wise dissimilarity in community composition across sites was modeled as a function of environmental covariates and geographic distance; this was done separately for the E and the W Himalaya to identify local and regional drivers of beta diversity for the three components. GDM is an extension of matrix regression where different covariates are fitted using splines. GDM explicitly accounts for the curvilinear relationship, depicting the dissimilarity of species between sites as ecological distance and identifying a subset of significant environmental predictors. This approach enables plotting predicted community turnover rates as a function of each variable (Ferrier et al., 2007). We used a combination of environmental (precipitation, temperature seasonality, primary productivity, forest cover), geographic (elevation, geographic distance, river width), and human disturbance variables to model beta diversity measures. Since the length of the sampling unit varied across different sites in the E Himalaya, we used sampling length as a predictor for the E Himalayan dataset. All spatial analyses were carried out in package “raster” (Hijmans, 2015). We calculated the variance explained by each predictor variable using the function “gdm.varImp” in “gdm” (Ferrier et al., 2007) package. All statistical analyses was carried out in software R ver. 3.6 (R Core Team, 2019).
We recorded 3,897 individuals of 39 species of birds belonging to 15 families during combined field surveys. We encountered 2,996 individual birds consisting of 36 bird species in the E Himalaya and 901 individual birds from 17 species in the west (Table 1). Muscicapidae (old world flycatchers) and Alcedinidae (kingfishers) were the most represented families in both the east and the west. Plumbeous Redstart (Phoenicurus fuliginosus) was the most encountered species in W Himalaya and the second most encountered species in E Himalaya. Ibisbill (Ibidoryncha struthersii) was the least encountered bird in W Himalaya, whereas Black-backed Forktail (Enicurus immaculatus) and White-throated Dipper (Cinclus cinclus) were the least encountered species in E Himalaya (for species list see Table 1). We recorded four species of conservation concern, including the Critically Endangered White-bellied Heron (Ardea insignis), Endangered Black-bellied Tern (Sterna acuticauda), and the Near Threatened River Lapwing (Vanellus duvaucelii) and Great Thick-knee (Esacus recurvirostris). There was some evidence phylogenetic clustering for the W Himalaya sites as their median values were beyond −1.96 units from the null expectation (Figure 2).
Table 1. List of bird species recorded during field surveys in E and W Himalaya with their family, IUCN conservation status and trophic niche as classified by Pigot et al. (2020).
Figure 2. Standardized effect size (SES) for mean pair-wise phylogenetic distance (MPD) and mean nearest taxon distance (MNTD) for phylogenetic and functional beta diversity in the W and E Himalaya.
We did not find differences in taxonomic and MNTD metrics for the phylogenetic and functional components (p > 0.05). However, we found greater clustering in phylogenetic (W = 2,229,371; P < 0.001) and functional (W = 2,230,439; P < 0.001) MPD for the W Himalaya than that for the E Himalaya (Figure 2). Beta diversity was highest for the taxonomic component compared to that for phylogenetic and functional components (Figure 3). MPD was higher than MNTD for both the phylogenetic and functional components of beta diversity (Figure 3).
Figure 3. Box-whisker plots showing pair-wise taxonomic, phylogenetic and functional β-total (Sørensen’s pairwise dissimilarity) in river bird communities in the E and the W Himalaya based on field surveys in winter. TD, Taxonomic Diversity; FD, Functional Diversity; PD, Phylogenetic Diversity; MPD, Mean Pair-wise Distance; MNTD, Mean nearest taxon distance.
The relative contribution of turnover and nestedness to the overall beta diversity varied across the different components and also between the E and W Himalaya. In the E Himalaya, there was a greater contribution of turnover to overall beta diversity as compared to that by nestedness for the taxonomic (W = 10,556,567, P < 0.001), phylogenetic (W = 7,699,831, P < 0.001), and functional (W = 8,994,152, P < 0.001) components (Figure 3). In the W Himalaya, there was greater contribution of turnover to overall beta diversity only for the taxonomic component (W = 581,821, P < 0.001). The overall turnover (β-total) was similar across E and W Himalaya for the taxonomic component (P > 0.05). However, the overall turnover was higher for the E Himalaya than that for W Himalaya for phylogenetic (W = 1,698,385; P = 0.023) and functional (W = 1,756,820; P < 0.001) components (Figure 4).
Figure 4. Box-whisker plots showing beta diversity components (β-turnover, β-nestedness, β-total) of the river bird community in the E and W Himalaya.
The GDM explained 64–90% of the variation in different beta diversity measures, thus explaining beta diversity patterns in river bird communities in the Indian Himalaya (Figure 5). Overall, GDM explained maximum variance for the functional components of beta diversity followed by the taxonomic and phylogenetic components; comparatively, a higher proportion of variance was explained for the W Himalayan communities than that for the east (Figure 5). Maximum variance was explained for β-FDMPD (93%) followed by β-FDMNTD (82%) and β-TD (81.8%) in the west.
Figure 5. Variance partitioning of different predictor variables across beta diversity measures (taxonomic, phylogenetic, and functional) for river birds at different scales.
Results of spatial GDM showed that the habitat and environmental variables, rather than geographical distance, influenced the observed beta diversity patterns in river bird communities in both the east and the west (Figure 5). River width explained a bulk of the variation in beta diversity for most measures across all three components (Figure 5). The relationship between river width was non-linear and beta diversity increased exponentially with increasing river width, particularly in the E Himalaya and reached an asymptote in the W Himalaya (Supplementary Figures 1–6). While forest cover and elevation explained variation in beta diversity patterns in the east, rainfall was an important variable influencing beta diversity patterns in the west (Figure 5). Temperature seasonality, net primary productivity, and human modification showed limited roles in driving the beta diversity patterns (Figure 5). The relationships between the different predictors and beta diversity measures were non-linear and varied across the different measures and the E and W Himalaya regions (Supplementary Figures 1–6).
In this study, we found that river bird communities exhibited phylogenetic and functional clustering with a greater signal of clustering in the W Himalaya. The turnover component had a larger contribution in explaining the variation in beta diversity patterns than did the nestedness component, especially in the E Himalaya. Predictors that explained the variation of beta diversity varied across the different components (taxonomic, phylogenetic, and functional) and between the E and the W Himalaya. Climatic and habitat factors, rather than geographic distance explained the variation in overall compositional (taxonomic, phylogenetic, and functional) dissimilarity in riverine bird communities in the Indian Himalaya. Our results demonstrate the key role of environmental filtering in structuring riverine bird communities in the Himalaya. Given the strong influence of habitat features in influencing diversity patterns, ongoing threats to riverine habitats posed by hydroelectric projects and forest loss in the Himalaya can impact the riverine bird communities in this hotspot negatively.
A significant proportion of the variation in beta diversity patterns in riverine bird communities was best explained by habitat features such as river width, elevation, forest cover, and climatic factors such as precipitation. River width was a consistent driver of all measures of beta diversity (Figure 5). In the E Himalaya, beta diversity for all three measures increased with river width, with a sharp rise in dissimilarity across river stretches wider than 150 m (Supplementary Figures 1–3). Larger river width is associated with river stretches at lower elevations with a higher discharge; they are also characterized by variable flow rates offered lotic to lentic habitats, which provide niches to a diverse array of riverine birds preferring slow- or medium-flowing river sections. The bank substrates are also variable (rocky or sandy), particularly in the lower sections of the rivers. Bouldery and pebbly bank substrates offer important microhabitats for birds such as dippers and redstarts, while the lower elevation sandy banks are preferred by wagtails, pratincoles, and lapwings (Sinha et al., 2019a). Since several riverine bird species show a preference for specific micro-habitats, there is likely to be higher species turnover as rivers progress from narrow headstreams to wider river stretches. Narrower river stretches support prey-base for a small group of specialist birds (e.g., redstarts and forktails) that predominantly have an insectivorous diet (Buckton and Ormerod, 2008; Sinha et al., 2019a). In comparison, wider river stretches offer greater river depths associated with larger prey such as fishes, amphibians, and mollusks, thus attracting other waterbirds such as kingfishers, cormorants, mergansers, gulls, and sandpipers to visit these river stretches opportunistically (for species list see Table 1).
Interestingly, the relative influence of other drivers varied across the E and W Himalaya. Forest cover and elevation explained significant variation for the E Himalayan birds. Along with the river channel and flood plain, the riparian forests are also recognized as an integral part of the riverscape (Weins, 2002). Distributions of many riverine bird species are positively or negatively associated with tree cover (Sullivan et al., 2007; Vaughan et al., 2007; Sullivan and Vierling, 2012; Sinha et al., 2019a). Here, beta diversity increased with increasing forest cover, indicating a higher homogenization in bird composition in river stretches with lower forest cover (Supplementary Figures 1–3). Forests in the Himalaya are being lost at an alarming rate (Srivastava et al., 2002; Gaur et al., 2019; Sheth et al., 2020), which could potentially result in lower species turnover in riverine bird communities with cascading effects on overall diversity patterns in the long-term.
Elevation is an important driver of riverine bird diversity (Manel et al., 2000). Interestingly, at elevations above 300 m in the E Himalaya, the beta diversity patterns were consistent and showed a relatively lower species turnover at elevations below 300 m. In the E Himalaya, the lowermost elevations are characterized by higher anthropogenic disturbances, resulting threats such as lower forest cover, and boulder and sand mining (Srivastava et al., 2002; Menzies et al., 2021), which likely contribute to the lower species turnover in the lowland elevations. Anthropogenic activities negatively impact specialist bird species resulting in the persistence of generalist species that are able to withstand habitat changes (Sinha et al., 2019a) likely resulting in lower turnover in the more modified, low elevation riparian habitats. Additionally, lower elevation avian communities were dominated by large-body sized species (e.g., cormorants, mergansers, pratincoles, waders) which prefer wider river stretches and habitat features, like sand bars and river islands. These could be uniform across sites in the lower elevation which may have resulted in lower turnover. These aspects need to be explored in greater detail in future.
Climatic factors such as precipitation and temperature are related to the variation in beta diversity patterns (Naka et al., 2020; Wayman et al., 2021). We found that precipitation was an important factor in explaining the variation in beta diversity patterns in the W Himalaya. The W Himalaya is more seasonal and drier than the E Himalaya (Price et al., 2011). There was a lower species turnover in drier habitats as compared to wetter habitats (precipitation lower than ∼1,400 mm). E Himalaya is consistently wetter across the entire elevation gradient; therefore, precipitation does not play a significant role in influencing the beta diversity patterns (Supplementary Figures 1–3). Similar to forest birds, the W Himalayan riverine bird assemblage is a nested subset of the E Himalaya assemblage, which is more species-rich (Buckton and Ormerod, 2002; Price et al., 2011; Srinivasan et al., 2014). This indicates that species that were able to pass through the environmental filter imposed by a drier and variable climate in the W Himalaya were able to successfully colonize, despite the harsh climate. However, this pattern is also likely to be a consequence of past climatic influences. During the Pleistocene glaciation, the W Himalayan region was under ice, while evergreen forests persisted in the E Himalayan region (Owen et al., 2002; Srinivasan et al., 2014). Thus, the past and present seasonality and variability in climate may have led to a greater clustering in riverine communities in the W Himalaya than that in the E Himalaya.
We found a greater contribution of the turnover component in taxonomic and functional beta diversity, especially in the E Himalaya. Higher turnover is a consequence of biotic interactions (e.g., competition), and greater nestedness is a consequence of local or regional extinctions, particularly in unfavorable and variable environments (Schemske et al., 2009; Soininen et al., 2018). As discussed earlier, the E Himalaya is more species-rich than the W Himalaya (Buckton and Ormerod, 2002); therefore, it is more likely that biotic interactions have an influence on turnover of species communities in the E Himalaya. Moreover, past (Pleistocene glaciation) and present (variable precipitation) climatic conditions likely cause similar influence of nestedness and turnover in the W Himalaya.
There was a relatively higher turnover in taxonomic diversity than that in phylogenetic and functional diversity, which indicates that species are replaced by their close relatives with similar traits across sites. Himalayan rivers are characterized by high species richness consisting of multiple species within a lineage of riverine birds (e.g., three species of redstarts, five species of forktails, five species of kingfishers) that differ in their microhabitat requirements (Buckton and Ormerod, 2008; Sinha et al., 2019a). Riverine ecosystems are extremely dynamic because of high natural disturbances offering diverse microhabitats (Ward et al., 2002). Changing microhabitats and the associated replacement of species by their close relatives play a functionally similar role that likely results in the pattern observed in this study.
We found that the different beta diversity measures were related to similar predictors within a region (i.e., E or W Himalaya). However, the relative contribution of the different predictors in explaining the variation in beta diversity differed across the different measures (Figure 5), which has been reported elsewhere (Wayman et al., 2021). Interestingly, precipitation explained a large proportion of variation in taxonomic component of beta diversity in the W Himalaya (Figure 5), but a lower proportion of variation in phylogenetic and functional component. This result could be a likely outcome of closely related species (which are likely to be functionally similar) replacing each other along a gradient of precipitation. Moreover, the proportion of variation explained by forest cover across the different components was similar; this is a likely outcome of certain groups disappearing along the forest cover gradient and needs to be examined in greater detail in the future.
Geographical distance is an important driver of beta diversity patterns in birds (Wayman et al., 2021). However, we did not find a significant influence of geographical distance on beta diversity patterns in the E or the W Himalaya. The influence of distance on beta diversity patterns is explained by dispersal limitation (Myers et al., 2013; Weinstein et al., 2014). Birds are unlikely to be dispersal limited at the scale at which the analysis has been carried out in the E and the W Himalaya. Although we found limited evidence of the influence of habitat modification on beta diversity patterns in the W but not in the E Himalaya, past studies have documented birds being sensitive to anthropogenic influences (Sinha et al., 2019a; Abreu et al., 2020; Menzies et al., 2021). Fine-scale information on human disturbance may be able to provide additional insights on human impacts.
This study was conducted primarily in the non-breeding season. Given that some riverine species exhibit altitudinal migration, beta diversity patterns and the influence of underlying drivers may vary. It will be important to conduct a similar study during the breeding season. However, conducting studies during the breeding season might be difficult due to inclement weather and associated flooding of the river. Nevertheless, the non-breeding season is also associated with a greater diversity of birds in the region as riverine stretches are being used by several other bird species that are dependent on riparian resources; hence, the non-breeding season is an important time for evaluating community organization. Given the high rates of human-driven modification of the Himalayan rivers, a systematic Himalaya wide survey across all drainages is required to determine the role of anthropogenic impacts on riverine systems.
Another potential shortcoming of the study could be variable sampling effort, particularly in the Eastern Himalaya. However, we used sampling length as a predictor in the variance partitioning analysis and demonstrate that very little variation in the beta diversity in E Himalaya is explained by the sampling length. Additionally, analysis of turnover in taxonomic beta diversity using a novel method (Zou and Axmacher, 2020) that helps control for variable sampling effort did not reveal any differences in beta diversity patterns (Supplementary Figure 7). Given this it is likely that variable sampling length will unlikely influence the outcome of this study.
This study provides evidence for the role of environmental filtering in structuring animal communities. This is the first study to examine turnover in riverine bird diversity in the Himalaya, the most species rich region for riverine birds and among the most threatened riverine habitats in the world. Globally, there are few studies that have examined the relative roles of different drivers on the different beta diversity measures of riverine birds and this study fills that knowledge gap. This study highlights the strong role of environment in driving beta diversity patterns. The wide climatic (precipitation), geographic (elevation), and habitat (river width, forest cover) gradient of the Himalayan mountain range contributes to the riverine bird diversity in the region. More than 160 dams have been proposed in the E Himalaya (Vagholikar and Das, 2010), which are likely to permanently alter the riverine systems with significant negative impacts on riverine bird communities as reported elsewhere (Abreu et al., 2020). In the Bhagirathi basin, the construction of the Tehri dam has converted a 75 km stretch of lotic habitat into a lentic habitat (Gaur et al., 2019). The riverine forests in the region also experience threats from severe habitat loss, destructive fishing methods, and sand and boulder mining, which can also be expected to impact riverine bird communities (Manel et al., 2000; Srivastava et al., 2002; Menzies et al., 2021). There is an urgent need to systematically determine the relative impacts of the different threats to riverine bird communities. Additionally, greater variability in precipitation patterns and river flows due to climate change (Prudhomme et al., 2014) will negatively impact the riverine bird communities (Royan et al., 2015), particularly in the drier and more variable W Himalaya.
The raw data supporting the conclusions of this article will be made available by the authors, without undue reservation.
Ethical review and approval was not required for the animal study because this was a purely observational study that did not involve the handling or collection of animals. The data collected in one region was approved by the Ethics Committee of the Nature Conservation Foundation.
RN and AS conceived the study. RM, MR, AS, and RN collected field data. NC ran the analysis with inputs from AS, RN, RM, and MR. AS, RN, NC, and RM wrote the manuscript with inputs from MR. All authors contributed to manuscript revision, read, and approved the submitted version.
The field work in E Himalaya was carried out with a Rufford Small Grants for Nature Conservation (23932-1) awarded to MR, Ravi Sankaran Inlaks Fellowship awarded to RM, Wildlife Conservation Trust Small Grant awarded to MR and RM, and in W Himalaya was carried out with the National Mission on Himalayan Studies fellowship to AS.
The authors declare that the research was conducted in the absence of any commercial or financial relationships that could be construed as a potential conflict of interest.
All claims expressed in this article are solely those of the authors and do not necessarily represent those of their affiliated organizations, or those of the publisher, the editors and the reviewers. Any product that may be evaluated in this article, or claim that may be made by its manufacturer, is not guaranteed or endorsed by the publisher.
RM, MR, and RN thank the Arunachal Pradesh and Assam Forest Departments for providing necessary permits to conduct the study in the E Himalaya. We thank to Rufford Small Grants, Ravi Sankaran Inlaks Foundation, and Wildlife Conservation Trust for funding the research in Arunachal Pradesh and Assam. Goutam Narayan, Nandita Hazarika, Neelam Dutta, Sartaj Ghuman, Abhishek Harihar, Kulbhushan Suryawanshi, Gopi Sundar, and Aparajita Datta provided valuable support during the study. AS thanks the National Mission on Himalayan Studies (MOEFCC and GBPNIHESD, Almora) for funding field studies in the W Himalaya and K. Ramesh at the Wildlife Institute of India for facilitating the same. AS also thanks the Director, Dean, and S. Sathyakumar of Wildlife Institute of India, Hima Nair, Jaswant Singh, Kuwar Singh, Manish Bisht, and others who assisted with fieldwork.
The Supplementary Material for this article can be found online at: https://www.frontiersin.org/articles/10.3389/fevo.2022.788184/full#supplementary-material
Abreu, T. L. S., Berg, S. B., de Faria, I. P., Gomes, L. P., Marinho-Filho, J. S., and Colli, G. R. (2020). River dams and the stability of bird communities: a hierarchical Bayesian analysis in a tropical hydroelectric power plant. J. Appl. Ecol. 57, 1124–1136. doi: 10.1111/1365-2664.13607
Anderson, M. J., Crist, T. O., Chase, J. M., Vellend, M., Inouye, B. D., Freestone, A. L., et al. (2011). Navigating the multiple meanings of β diversity: a roadmap for the practicing ecologist. Ecol. Lett. 14, 19–28. doi: 10.1111/j.1461-0248.2010.01552.x
Barnagaud, J.-Y., Kissling, W. D., Tsirogiannis, C., Fisikopoulos, V., Villéger, S., Sekercioglu, C. H., et al. (2017). Biogeographical, environmental and anthropogenic determinants of global patterns in bird taxonomic and trait turnover. Glob. Ecol. Biogeogr. 26, 1190–1200. doi: 10.1111/geb.12629
Baselga, A. (2010). Partitioning the turnover and nestedness components of beta diversity. Glob. Ecol. Biogeogr. 19, 134–143. doi: 10.1111/j.1466-8238.2009.00490.x
Baselga, A., and Orme, C. D. L. (2012). betapart: an R package for the study of beta diversity. Methods Ecol. Evol. 3, 808–812. doi: 10.1111/j.2041-210X.2012.00224.x
Branco, C. C. Z., Bispo, P. C., Peres, C. K., Tonetto, A. F., Krupek, R. A., Barfield, M., et al. (2020). Partitioning multiple facets of beta diversity in a tropical stream macroalgal metacommunity. J. Biogeogr. 47, 1765–1780. doi: 10.1111/jbi.13879
Buckton, S. T. (1998). Spatio-Temporal Patterns in the Distribution and Ecology of Himalayan River Birds. Ph.D. thesis. Wales: University of Wales.
Buckton, S. T., and Ormerod, S. J. (2002). Global patterns of diversity among the specialist birds of riverine landscapes. Freshw. Biol. 47, 695–709. doi: 10.1046/j.13652427.2002.00891.x
Buckton, S. T., and Ormerod, S. J. (2008). Niche segregation of Himalayan river birds. J. Field Ornithol. 79, 176–185. doi: 10.1111/j.1557-9263.2008.00160.x
Cadotte, M. W., Cavender-Bares, J., Tilman, D., and Oakley, T. H. (2009). Using phylogenetic, functional and trait diversity to understand patterns of plant community productivity. PLoS One 4:e5695. doi: 10.1371/journal.pone.0005695
Capinha, C., Essl, F., Seebens, H., Moser, D., and Pereira, H. M. (2015). The dispersal of alien species redefines biogeography in the Anthropocene. Science 348, 1248–1251.
Cavender-Bares, J., Kozak, K. H., Fine, P. V. A., and Kembel, S. W. (2009). The merging of community ecology and phylogenetic biology. Ecol. Lett. 12, 693–715. doi: 10.1111/j.1461-0248.2009.01314.x
Chase, J. M., and Myers, J. A. (2011). Disentangling the importance of ecological niches from stochastic processes across scales. Philos. Trans. R. Soc Biol. Sci. 366, 2351–2363. doi: 10.1098/rstb.2011.0063
Clements, J. F. (2007). The Clements Checklist of Birds of the World, 6th Edn. Ithaca, NY: Cornell University Press.
Davies, T. J., Meiri, S., Barraclough, T. G., and Gittleman, J. L. (2007). Species co-existence and character divergence across carnivores. Ecol. Lett. 10, 146–152. doi: 10.1111/j.1461-0248.2006.01005.x
Diamond, J. M. (1975). The island dilemma: lessons of modern biogeographic studies for the design of natural reserves. Biol. Conserv. 7, 129–146. doi: 10.1016/0006-3207(75)90052-X
Dudgeon, D., Arthington, A. H., Gessner, M. O., Kawabata, Z.-I., Knowler, D. J., Lévêque, C., et al. (2006). Freshwater biodiversity: importance, threats, status and conservation challenges. Biol. Rev. 81, 163–182. doi: 10.1017/S1464793105006950
Dynesius, M., and Nilsson, C. (1994). Fragmentation and flow regulation of river systems in the northern third of the world. Science 266, 753–762. doi: 10.1126/science.266.5186.753
Ferrier, S., Manion, G., Elith, J., and Richardson, K. (2007). Using generalized dissimilarity modelling to analyse and predict patterns of beta diversity in regional biodiversity assessment. Divers. Distrib. 13, 252–264. doi: 10.1111/j.1472-4642.2007.00341.x
Fick, S. E., and Hijmans, R. J. (2017). WorldClim 2: new 1-km spatial resolution climate surfaces for global land areas. Int. J. Climatol. 37, 4302–4315. doi: 10.1002/joc.5086
Fitzpatrick, M. C., Sanders, N. J., Normand, S., Svenning, J.-C., Ferrier, S., Gove, A. D., et al. (2013). Environmental and historical imprints on beta diversity: insights from variation in rates of species turnover along gradients. Proc. Biol. Sci. 280:20131201. doi: 10.1098/rspb.2013.1201
Gaur, T., Sinha, A., Adhikari, B. S., and Ramesh, K. (2019). Dynamics of landscape change in a mountainous river basin: a case study of the Bhagirathi River, Western Himalaya. Appl. Ecol. Environ. Res. 17, 8271–8289. doi: 10.15666/aeer/1704_82718289
Graham, C. H., and Fine, P. V. A. (2008). Phylogenetic beta diversity: linking ecological and evolutionary processes across space in time. Ecol. Lett. 11, 1265–1277. doi: 10.1111/j.1461-0248.2008.01256.x
Grumbine, R. E., and Pandit, M. K. (2013). Threats from India’s Himalaya dams. Science 339, 36–37. doi: 10.1126/science.1227211
Harrison, S., Ross, S. J., and Lawton, J. H. (1992). Beta diversity on geographic gradients in Britain. J. Anim. Ecol. 61, 151–158. doi: 10.2307/5518
Hijmans, R. J. (2015). raster: Geographic Data Analysis and Modeling. R package version 3.4-13. R Package. Available online at: https://CRAN.R-project.org/package=raster (accessed May 1, 2020).
Hubbell, S. P. (2001). The Unified Neutral Theory of Biodiversity and Biogeography. Princeton, NJ: Princeton University Press.
Jacobsen, D., Schultz, R., and Encalada, A. (1997). Structure and diversity of stream invertebrate assemblages: the influence of temperature with altitude and latitude. Freshw. Biol. 38, 247–261. doi: 10.1046/j.1365-2427.1997.00210.x
Jetz, W., Thomas, G. H., Joy, J. B., Hartmann, K., and Mooers, A. O. (2012). The global diversity of birds in space and time. Nature 491, 444–448. doi: 10.1038/nature11631
Kembel, S. W., Cowan, P. D., Helmus, M. R., Cornwell, W. K., Morlon, H., Ackerly, D. D., et al. (2010). Picante: R tools for integrating phylogenies and ecology. Bioinformatics 26, 1463–1464.
Kennedy, C. M., Oakleaf, J. R., Theobald, D. M., Baruch-Mordo, S., and Kiesecker, J. (2019). Managing the middle: a shift in conservation priorities based on the global human modification gradient. Glob. Change Biol. 25, 811–826. doi: 10.1111/gcb.14549
Koleff, P., Gaston, K. J., and Lennon, J. J. (2003). Measuring beta diversity for presence–absence data. J. Anim. Ecol. 72, 367–382. doi: 10.1046/j.1365-2656.2003.00710.x
Larsen, S., Sorace, A., and Mancini, L. (2010). Riparian bird communities as indicators of human impacts along Mediterranean streams. Environ. Manage. 45, 261–273. doi: 10.1007/s00267-009-9419-0
Legendre, P. (2014). Interpreting the replacement and richness difference components of beta diversity. Glob. Ecol. Biogeogr. 23, 1324–1334. doi: 10.1111/geb.12207
Lennon, J. J., Koleff, P., GreenwooD, J. J. D., and Gaston, K. J. (2001). The geographical structure of british bird distributions: diversity, spatial turnover and scale. J. Anim. Ecol. 70, 966–979. doi: 10.1046/j.0021-8790.2001.00563.x
Manel, S., Buckton, S. T., and Ormerod, S. J. (2000). Testing large-scale hypotheses using surveys: the effects of land use on the habitats, invertebrates and birds of Himalayan rivers. J. Appl. Ecol. 37, 756–770. doi: 10.1046/j.1365-2664.2000.00537.x
Manel, S., Dias, J. M., Buckton, S. T., and Ormerod, S. J. (1999). Alternative methods for predicting species distribution: an illustration with Himalayan river birds. J. Appl. Ecol. 36, 734–747. doi: 10.1046/j.1365-2664.1999.00440.x
Matthews, T. J., Aspin, T. W. H., Ulrich, W., Baselga, A., Kubota, Y., Proios, K., et al. (2019). Can additive beta diversity be reliably partitioned into nestedness and turnover components? Glob. Ecol. Biogeogr. 28, 1146–1154. doi: 10.1111/geb.12921
McGill, B. J., Enquist, B. J., Weiher, E., and Westoby, M. (2006). Rebuilding community ecology from functional traits. Trends Ecol. Evol. 21, 178–185. doi: 10.1016/j.tree.2006.02.002
McKinney, M. L. (2006). Urbanization as a major cause of biotic homogenization. Biol. Conserv. 127, 247–260. doi: 10.1016/j.biocon.2005.09.005
Menzies, R. K., Rao, M., and Naniwadekar, R. (2021). Assessing the status of the Critically Endangered White-bellied Heron Ardea insignis in north-east India. Bird Conserv. Int. 31, 255–267. doi: 10.1017/S0959270920000301
Mittermeier, R. A., Turner, W. R., Larsen, F. W., Brooks, T. M., and Gascon, C. (2011). “Global biodiversity conservation: the critical role of hotspots,” in Biodiversity Hotspots: Distribution and Protection of Conservation Priority Areas, eds F. E. Zachos and J. C. Habel (Berlin: Springer), 3–22. doi: 10.1007/978-3-642-20992-5_1
Myers, J. A., Chase, J. M., Jiménez, I., Jørgensen, P. M., Araujo-Murakami, A., Paniagua-Zambrana, N., et al. (2013). Beta-diversity in temperate and tropical forests reflects dissimilar mechanisms of community assembly. Ecol. Lett. 16, 151–157. doi: 10.1111/ele.12021
Myers, N., Mittermeier, R. A., Mittermeier, C. G., da Fonseca, G. A. B., and Kent, J. (2000). Biodiversity hotspots for conservation priorities. Nature 403, 853–858. doi: 10.1038/35002501
Naka, L. N., Laranjeiras, T. O., Lima, G. R., Plaskievicz, A., Pinto, F., and Gonçalves-Souza, T. (2020). Climate as a major driver of avian diversity in riparian amazonian habitats along an environmental gradient. J. Biogeogr. 47, 2328–2340. doi: 10.1111/jbi.13957
Nekola, J. C., and White, P. S. (1999). The distance decay of similarity in biogeography and ecology. J. Biogeogr. 26, 867–878. doi: 10.1046/j.1365-2699.1999.00305.x
Newbold, T., Hudson, L. N., Hill, S. L. L., Contu, S., Gray, C. L., Scharlemann, J. P. W., et al. (2016). Global patterns of terrestrial assemblage turnover within and among land uses. Ecography 39, 1151–1163. doi: 10.1111/ecog.01932
Oksanen, J., Blanchet, F. G., Friendly, M., Kindt, R., Legendre, P., McGlinn, D., et al. (2019). vegan: Community Ecology Package. Available online at: https://CRAN.R-project.org/package=vegan (accessed May 1, 2020).
Ormerod, S. J., Rundle, S. D., Wilkinson, S. M., Daly, G. P., Dale, K. M., and Juttner, I. (1994). Altitudinal trends in the diatoms, bryophytes, macroinvertebrates and fish of a Nepalese river system. Freshw. Biol. 32, 309–322. doi: 10.1111/j.1365-2427.1994.tb01128.x
Owen, L. A., Finkel, R. C., and Caffee, M. W. (2002). A note on the extent of glaciation throughout the Himalaya during the global Last Glacial Maximum. Quat. Sci. Rev. 21, 147–157. doi: 10.1016/S0277-3791(01)00104-4
Palmer, G. C., and Bennett, A. F. (2006). Riparian zones provide for distinct bird assemblages in forest mosaics of south-east Australia. Biol. Conserv. 130, 447–457. doi: 10.1016/j.biocon.2006.01.006
Pandit, M. K., and Grumbine, R. E. (2012). Potential effects of ongoing and proposed hydropower development on terrestrial biological diversity in the Indian Himalaya. Conserv. Biol. 26, 1061–1071. doi: 10.1111/j.1523-1739.2012.01918.x
Paradis, E., Claude, J., and Strimmer, K. (2004). APE: analyses of phylogenetics and evolution in R language. Bioinformatics 20, 289–290. doi: 10.1093/bioinformatics/btg412
Petchey, O. L., and Gaston, K. J. (2006). Functional diversity: back to basics and looking forward. Ecol. Lett. 9, 741–758. doi: 10.1111/j.1461-0248.2006.00924.x
Pigot, A. L., Sheard, C., Miller, E. T., Bregman, T. P., Freeman, B. G., Roll, U., et al. (2020). Macroevolutionary convergence connects morphological form to ecological function in birds. Nat. Ecol. Evol. 4, 230–239. doi: 10.1038/s41559-019-1070-4
Price, T. D., Mohan, D., Tietze, D. T., Hooper, D. M., Orme, C. D. L., and Rasmussen, P. C. (2011). Determinants of northerly range limits along the Himalayan bird diversity gradient. Am. Nat. 178, S97–S108. doi: 10.1086/661926
Prudhomme, C., Giuntoli, I., Robinson, E. L., Clark, D. B., Arnell, N. W., Dankers, R., et al. (2014). Hydrological droughts in the 21st century, hotspots and uncertainties from a global multimodel ensemble experiment. Proc. Nat. Acad. Sci. U.S.A. 111, 3262–3267. doi: 10.1073/pnas.1222473110
Qian, H., Jin, Y., Leprieur, F., Wang, X., and Deng, T. (2020). Geographic patterns and environmental correlates of taxonomic and phylogenetic beta diversity for large-scale angiosperm assemblages in China. Ecography 43, 1706–1716. doi: 10.1111/ecog.05190
R Core Team (2019). R: A Language and Environment for Statistical Computing. Vienna: R Foundation for Statistical Computing.
Revell, L. J. (2012). phytools: an R package for phylogenetic comparative biology (and other things). Methods Ecol. Evol. 3, 217–223. doi: 10.1111/j.2041-210X.2011.00169.x
Ricklefs, R. E. (2004). A comprehensive framework for global patterns in biodiversity. Ecol. Lett. 7, 1–15. doi: 10.1046/j.1461-0248.2003.00554.x
Rodgers, W. A., Panwar, H. S., and Mathur, V. B. (2000). Wildlife Protected Area Network in India: A Review: Executive Summary. Dehradun: Wildlife Institute of India.
Royan, A. (2015). The Influence of River Flow on the Distribution and Community Organisation of River Birds. Ph.D. thesis. Birmingham: University of Birmingham.
Royan, A., Prudhomme, C., Hannah, D. M., Reynolds, S. J., Noble, D. G., and Sadler, J. P. (2015). Climate-induced changes in river flow regimes will alter future bird distributions. Ecosphere 6, 1–10. doi: 10.1890/ES14-00245.1
Schemske, D. W., Mittelbach, G. G., Cornell, H. V., Sobel, J. M., and Roy, K. (2009). Is there a latitudinal gradient in the importance of biotic interactions? Annu. Rev. Ecol. Evol. Syst. 40, 245–269. doi: 10.1146/annurev.ecolsys.39.110707.173430
Schliep, K. P. (2011). phangorn: phylogenetic analysis in R. Bioinformatics 27, 592–593. doi: 10.1093/bioinformatics/btq706
Sheth, C., Datta, A., and Parashuram, D. (2020). Persistent loss of biologically-rich tropical forests in the Indian Eastern Himalaya. Silva Fenn. 54, 10373. doi: 10.1101/827360
Sinha, A. (2021). Patterns of Distribution and Multi-Scale Habitat Correlates of Riverine Birds in the Upper Ganges, Western Himalaya. Ph.D. thesis. Gujarat: Saurashtra University.
Sinha, A., Hariharan, H., Adhikari, B. S., and Krishnamurthy, R. (2019b). Bird diversity along riverine areas in the Bhagirathi Valley, Uttarakhand, India. Biodivers. Data J. 7:e31588. doi: 10.3897/BDJ.7.e31588
Sinha, A., Chatterjee, N., Ormerod, S. J., Adhikari, B. S., and Krishnamurthy, R. (2019a). River birds as potential indicators of local- and catchment-scale influences on Himalayan river ecosystems. Ecosyst. People 15, 90–101. doi: 10.1080/26395916.2019.1591508
Soininen, J., Heino, J., and Wang, J. (2018). A meta-analysis of nestedness and turnover components of beta diversity across organisms and ecosystems. Glob. Ecol. Biogeogr. 27, 96–109. doi: 10.1111/geb.12660
Soininen, J., Lennon, J. J., and Hillebrand, H. (2007). A multivariate analysis of beta diversity across organisms and environments. Ecology 88, 2830–2838. doi: 10.1890/06-1730.1
Srinivasan, U., Elsen, P. R., and Wilcove, D. S. (2019). Annual temperature variation influences the vulnerability of montane bird communities to land-use change. Ecography 42, 2084–2094. doi: 10.1111/ecog.04611
Srinivasan, U., Tamma, K., and Ramakrishnan, U. (2014). Past climate and species ecology drive nested species richness patterns along an east-west axis in the Himalaya. Glob. Ecol. Biogeogr. 23, 52–60. doi: 10.1111/geb.12082
Srivastava, S., Singh, T. P., Singh, H., Kushwaha, S. P. S., and Roy, P. S. (2002). Assessment of large-scale deforestation in Sonitpur district of Assam. Curr. Sci. 82, 1479–1484.
Sullivan, S. M. P., and Vierling, K. T. (2012). Exploring the influences of multiscale environmental factors on the American dipper Cinclus mexicanus. Ecography 35, 624–636.
Sullivan, S. M. P., Watzin, M. C., and Keeton, W. S. (2007). A riverscape perspective on habitat associations among riverine bird assemblages in the Lake Champlain Basin, USA. Landsc. Ecol. 22, 1169–1186. doi: 10.1007/s10980-007-9097-3
Townsend, C. R., and Hildrew, A. G. (1994). Species traits in relation to a habitat templet for river systems. Freshw. Biol. 31, 265–275. doi: 10.1111/j.1365-2427.1994.tb01740.x
Trøjelsgaard, K., Jordano, P., Carstensen, D. W., and Olesen, J. M. (2015). Geographical variation in mutualistic networks: similarity, turnover and partner fidelity. Proc. Biol. Sci. 282:20142925. doi: 10.1098/rspb.2014.2925
Tscharntke, T., Klein, A.-M., Kruess, A., Steffan-Dewenter, I., and Thies, C. (2005). Landscape perspectives on agricultural intensification and biodiversity – ecosystem service management. Ecol. Lett. 8, 857–874. doi: 10.1111/j.1461-0248.2005.00782.x
Vagholikar, N., and Das, P. J. (2010). Damming the Northeast. Kalpavriksh, Aaranyak and ActionaAid India. Pune: Kalpavriksh.
Vaughan, I. P., Noble, D. G., and Ormerod, S. J. (2007). Combining surveys of river habitats and river birds to appraise riverine hydromorphology. Freshw. Biol. 52, 2270–2284. doi: 10.1111/j.1365-2427.2007.01837.x
Vellend, M. (2010). Conceptual synthesis in community ecology. Q. Rev. Biol. 85, 183–206. doi: 10.1086/652373
Villéger, S., Grenouillet, G., and Brosse, S. (2013). Decomposing functional β-diversity reveals that low functional β-diversity is driven by low functional turnover in European fish assemblages. Glob. Ecol. Biogeogr. 22, 671–681. doi: 10.1111/geb.12021
Villéger, S., Mason, N. W. H., and Mouillot, D. (2008). New multidimensional functional diversity indices for a multifaceted framework in functional ecology. Ecology 89, 2290–2301. doi: 10.1890/07-1206.1
Ward, J. V., Tockner, K., Arscott, D. B., and Claret, C. (2002). Riverine landscape diversity. Freshw. Biol. 47, 517–539. doi: 10.1046/j.1365-2427.2002.00893.x
Wayman, J. P., Sadler, J. P., Pugh, T. A. M., Martin, T. E., Tobias, J. A., and Matthews, T. J. (2021). Identifying the drivers of spatial taxonomic and functional beta-diversity of British breeding birds. Front. Ecol. Evol. 9:620062. doi: 10.3389/fevo.2021.620062
Webb, C. O., Ackerly, D. D., McPeek, M. A., and Donoghue, M. J. (2002). Phylogenies and community ecology. Annu. Rev. Ecol. Evol. Syst. 33, 475–505. doi: 10.1146/annurev.ecolsys.33.010802.150448
Weins, J. A. (2002). Riverine landscapes: taking landscape ecology into the water. Freshw. Biol. 47, 501–515. doi: 10.1046/j.1365-2427.2002.00887.x
Weinstein, B. G., Tinoco, B., Parra, J. L., Brown, L. M., McGuire, J. A., Stiles, F. G., et al. (2014). Taxonomic, phylogenetic, and trait beta diversity in South American Hummingbirds. Am. Nat. 184, 211–224. doi: 10.1086/676991
Whittaker, R. H. (1960). Vegetation of the Siskiyou Mountains, Oregon and California. Ecol. Monogr. 30, 279–338. doi: 10.2307/1943563
Keywords: beta diversity (β), Brahmaputra, forest cover, Ganges, generalized dissimilarity modeling (GDM), nestedness, river width, species turnover
Citation: Sinha A, Menzies RK, Chatterjee N, Rao M and Naniwadekar R (2022) Drivers of Taxonomic, Phylogenetic, and Functional Beta Diversity of Himalayan Riverine Birds. Front. Ecol. Evol. 10:788184. doi: 10.3389/fevo.2022.788184
Received: 01 October 2021; Accepted: 10 January 2022;
Published: 17 February 2022.
Edited by:
Luciano N. Naka, Federal University of Pernambuco, BrazilReviewed by:
Reginaldo Augusto Farias De Gusmão, Federal Rural University of Pernambuco, BrazilCopyright © 2022 Sinha, Menzies, Chatterjee, Rao and Naniwadekar. This is an open-access article distributed under the terms of the Creative Commons Attribution License (CC BY). The use, distribution or reproduction in other forums is permitted, provided the original author(s) and the copyright owner(s) are credited and that the original publication in this journal is cited, in accordance with accepted academic practice. No use, distribution or reproduction is permitted which does not comply with these terms.
*Correspondence: Rohit Naniwadekar, cm9oaXRAbmNmLWluZGlhLm9yZw==
†These authors have contributed equally to this work
Disclaimer: All claims expressed in this article are solely those of the authors and do not necessarily represent those of their affiliated organizations, or those of the publisher, the editors and the reviewers. Any product that may be evaluated in this article or claim that may be made by its manufacturer is not guaranteed or endorsed by the publisher.
Research integrity at Frontiers
Learn more about the work of our research integrity team to safeguard the quality of each article we publish.