- 1The Laboratory for Wood Anatomy and Dendrochronology, Department of Geology, Lund University, Lund, Sweden
- 2Natural Resources Institute Finland, Rovaniemi, Finland
- 3Quaternary Sciences, Department of Geology, Lund University, Lund, Sweden
Our overall understanding of long-term climate dynamics is largely based on proxy data generated from archives such as ice cores, ocean sediments, tree rings, speleothems, and corals, whereas reconstructions of long-term changes in vegetation and associated climate during the Holocene are largely based on paleoecological records from peat and lake sequences, primarily pollen and plant macrofossil data. However, since no proxy can provide a complete picture of the past, it is important to integrate different types of data, and to use methods that can support the paleoecological and paleoclimatic interpretations. Here we review how tree-ring data and dendrochronological approaches can be integrated with stratigraphic records to provide complementary paleoecological and paleoclimatic information. The review includes multiproxy studies in which dendrochronological data have been either compared or integrated with stratigraphic records, mainly pollen records, with the aim to contribute to a better understanding of long-term ecosystem and climate dynamics. We mainly focus on studies from northwest Europe in which tree-ring data and at least one type of paleoecological proxy record from the same site or area has been either compared or integrated. We find that integration of dendrochronological data and paleoecological records from peat and lake sequences is a powerful but underutilized approach to reconstruct long-term ecological and climatic changes. One likely reason for its limited use is the contrasting character of the two categories of data, including their different time resolution and occurrence, making them difficult to integrate. For example, subfossil wood providing annual dendrochronological data usually only occurs sporadically in peat and lake sediments, and the presence/absence of the trees are normally expected to be recorded in the pollen data with multi-decadal or coarser resolution. Therefore, we also discuss methods to compare and integrate dendrochronological and stratigraphic records, as well as the relevant paleoecological and paleoclimatic information provided by dendrochronology, pollen, and peat stratigraphy, with the aim to facilitate new multi-proxy initiatives that will contribute to a better understanding of long-term ecosystem and climate dynamics and thereby a firmer basis for future nature conservation initiatives.
Introduction
To distinguish natural long-term climate variability and ecosystem dynamics from anthropogenic effects, reconstructions of paleoecological and paleoclimatic conditions based on both qualitative and quantitative proxy records from the time before industrialization are required (Jansen et al., 2007; Marcott et al., 2013). To obtain more robust and detailed long-term climate and ecosystem reconstructions, improvements of the paleoecological and paleoclimatic methodologies in combination with precise chronological techniques are constantly being made (Wanner et al., 2008; Marcott et al., 2013). Over recent decades, we have thereby increased our knowledge of climate and ecosystem dynamics over the course of the Holocene.
Proxy data generated from natural archives such as ice cores, ocean sediments, tree rings, and speleothems are essential for our understanding of climate dynamics during the Holocene. Many archives consist of organic material, which under oxygen-poor (i.e., usually water saturated) or dry conditions can be preserved for thousands of years, and contain paleoecological records in the form of pollen and other microfossils. In such contexts, often in water-saturated wetlands, lake sediments or deserts, wood remains referred to as subfossil trees can also be found. In the boreal zone, the most common sites to find subfossil trees suitable for dendrochronological studies are peatlands (Leuschner et al., 2002; Eckstein et al., 2009; Edvardsson et al., 2016a), lakes (Eronen et al., 2002; Grudd et al., 2002; Gunnarson, 2008; Helama et al., 2008) and ancient river terraces (Becker, 1983; Friedrich et al., 2004). Dead and decaying trees are also found either as standing or downed boles from dry forest soils and mountainous terrains, and these materials can be referred to as subfossil trees; however, the preservation of stemwood in such conditions may be considerably limited in comparison to water-logged depositional environments (Helama et al., 2020). Dendrochronology is recognized as an accurate dating method, but the growth patterns of the trees also reflect environmental and climate changes that have occurred during the trees’ lifetime (Fritts, 2012; Schweingruber, 2012; Cook and Kairiukstis, 2013). Over recent decades, several multi-proxy reconstructions of climate dynamics during last two millennia have been presented, many of which have included annually resolved tree-ring data (Moberg et al., 2005; D’Arrigo et al., 2006; Mann et al., 2008; Birks et al., 2014; Stoffel et al., 2015). To reach even further back in time, dendrochronological studies using subfossil trees have proved to be important, allowing precise and long-term studies of climate dynamics and environmental changes during the Holocene (Eronen et al., 2002; Leuschner et al., 2002; Helama et al., 2008; Edvardsson et al., 2016a). But records from subfossil trees are not always straightforward to develop or interpret in terms of past climate, especially since deposition, preservation and patterns of tree growth may have changed over time.
In Quaternary sciences, the research fields of paleoclimatology and paleoecology have developed significantly since the early twentieth century. The research disciplines were improved significantly by pollen analysis, which in many aspects was developed by von Post (1918, 1924, 1930). In combination with studies of peat stratigraphy, several abrupt changes associated with rapid climatic shifts could be detected (Granlund, 1932). Another important breakthrough was the introduction of radiocarbon dating (Libby et al., 1949) which allowed for much improved age control on the proxy records. Ever since, climate reconstructions based on radiocarbon dated stratigraphic records from lakes and peatlands have become common in the paleoclimatic literature (Langdon and Barber, 2005; Mauquoy et al., 2008), and are often based on proxies such as peat humification, pollen, and plant macrofossils (Swindles et al., 2013). Despite this, studies that make use of the combined information, which dendrochronology and classical paleoecological proxies provide, are still rare.
In this review, we primarily focus on articles presenting state-of-the-art studies integrating tree-ring based analyses with stratigraphic proxy data. Among the stratigraphic proxies, we will mainly focus on pollen-based climate or vegetation reconstructions, but also include studies based plant macrofossils, peat humification, charcoal fragments, and testate amoebae. Records developed from these proxies provide valuable complementary information about vegetation, land-use and other aspects of landscape and environmental change, as well as climate dynamics. However, inherent differences in properties, methodological issues, and time resolution between dendrochronological and peat- or sediment-based datasets make it difficult to bring these together. This review offers an opportunity to discuss how dendrochronology (tree-ring analysis) and stratigraphic proxy data can be integrated to better characterize long-term environmental and climatic changes, and to address the pros and cons of these approaches.
Materials and Methods
Literature Studies
For this review, we focus on available scientific literature on paleoclimatic or paleoecological studies integrating dendrochronology and stratigraphic proxy data such as pollen as a minimum, but preferably also including macrofossil analysis and further analysis of the stratigraphic records (Figure 1). To delimit the study, our review primarily focuses on studies from northwestern Europe. Studies of paleoecology and paleoclimatology have a long tradition in this geographic region, and many modern paleoecological research techniques providing insights to the potential of the field have been applied and developed by researchers active in for example Fennoscandia (Sernander, 1908; von Post, 1918; Iversen, 1941).
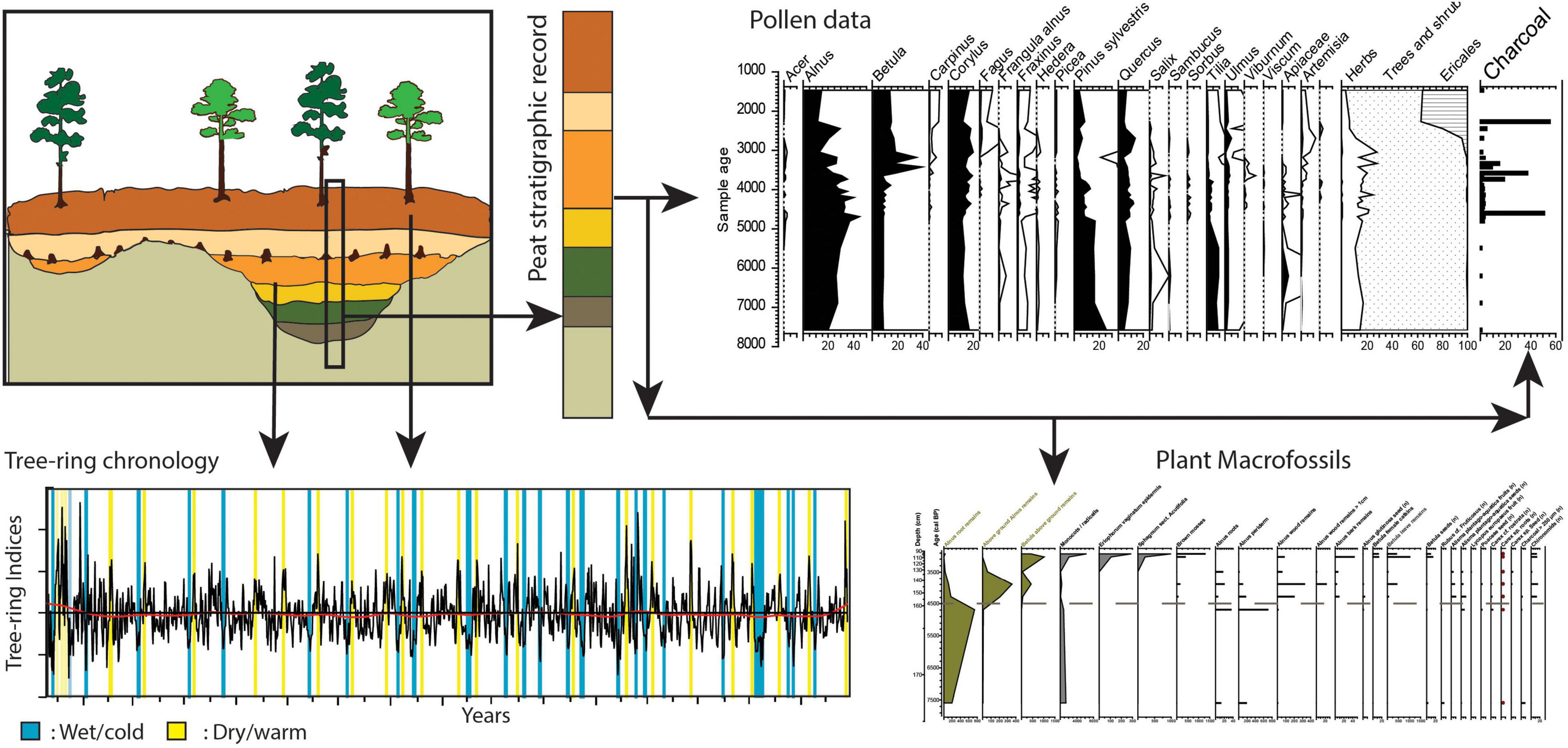
Figure 1. Many different proxy records can be obtained from peatlands. From the trees growing on the peat surface as well as subfossil trees preserved in the peat it is possible to create annually resolved tree-ring chronologies. The stratigraphy in the peatland can also be studied, including the composition of mosses and the degree of degradation of the peat. Furthermore, it is possible to analyze changes in the pollen concentrations and composition in the peat as well as if plant macrofossils or charcoal can be observed at different levels in the stratigraphic record.
We searched for relevant publications in electronic databases using various combinations of Boolean search terms to ensure a thorough assessment of the available literature. The databases used were Google Scholar1 and Web of Science.2 We used the following search terms: “dendrochronology” or “tree-ring analysis,” “pollen,” “pollen analysis,” “macro fossil,” “macrofossil,” “stratigraphy,” and “peat.” We also obtained papers directly from colleagues and through reference lists from published studies including major review articles and books on climate and paleoecology during the Holocene.
From our literature search we identified 38 studies which we highlight and discuss in this review (Figure 2 and Table 1). They are primarily multi-proxy studies with focus on dendrochronology using subfossil wood material from sites in northwest Europe. Multiproxy studies such as Jalkanen et al. (2008), Edvardsson et al. (2019), and Theuerkauf et al. (2019), primarily focusing on the last century, were excluded from the review but are still valuable as a basis for describing and understanding the various methods presented and discussed.
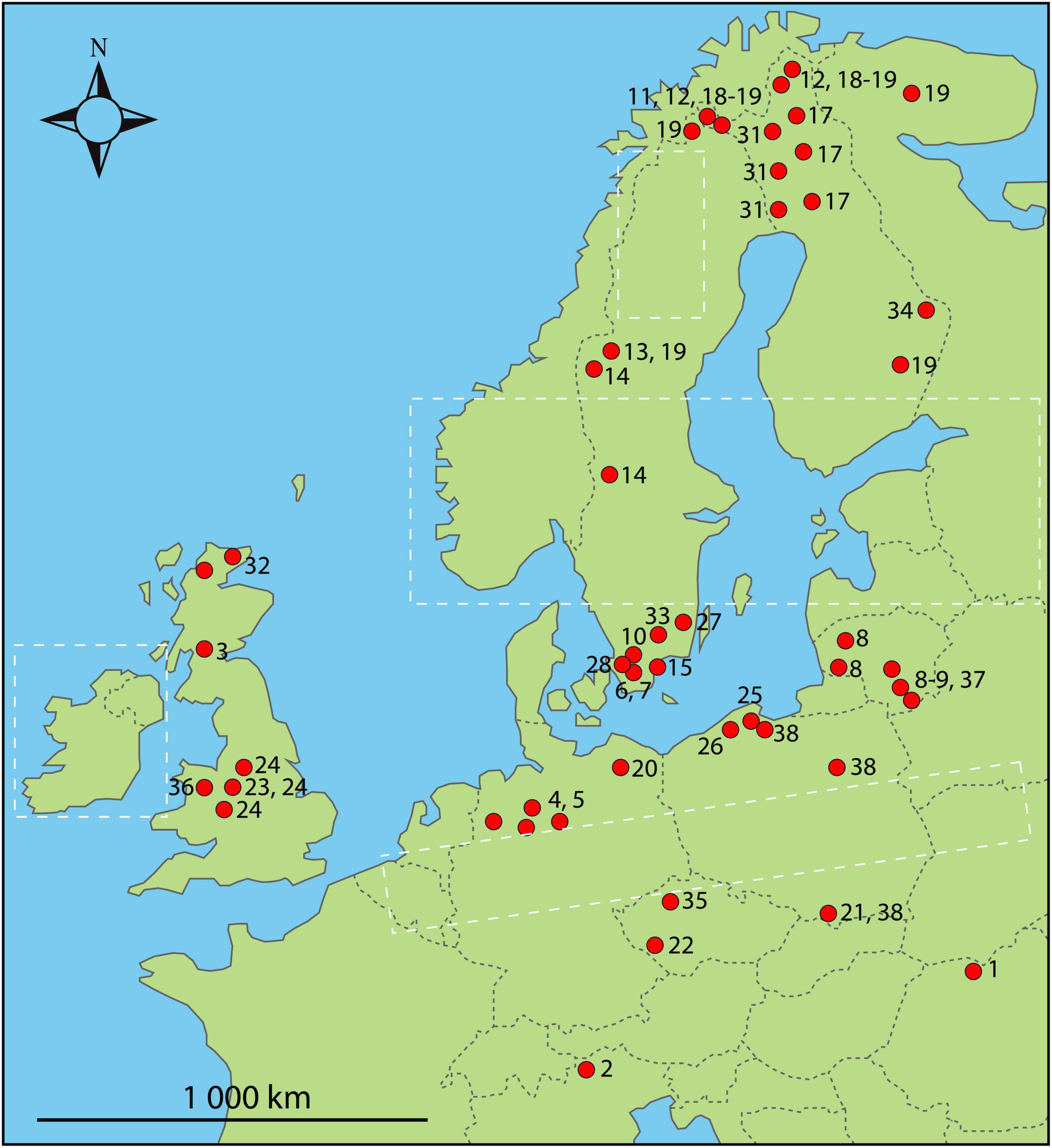
Figure 2. Map showing location of the main sites presented in the articles reviewed. The numbers represent the articles presented in Table 1. The white boxes show underutilized areas from which either dendrochronological and paleobotanical studies have been performed without being combined, or there are good opportunities for further studies.
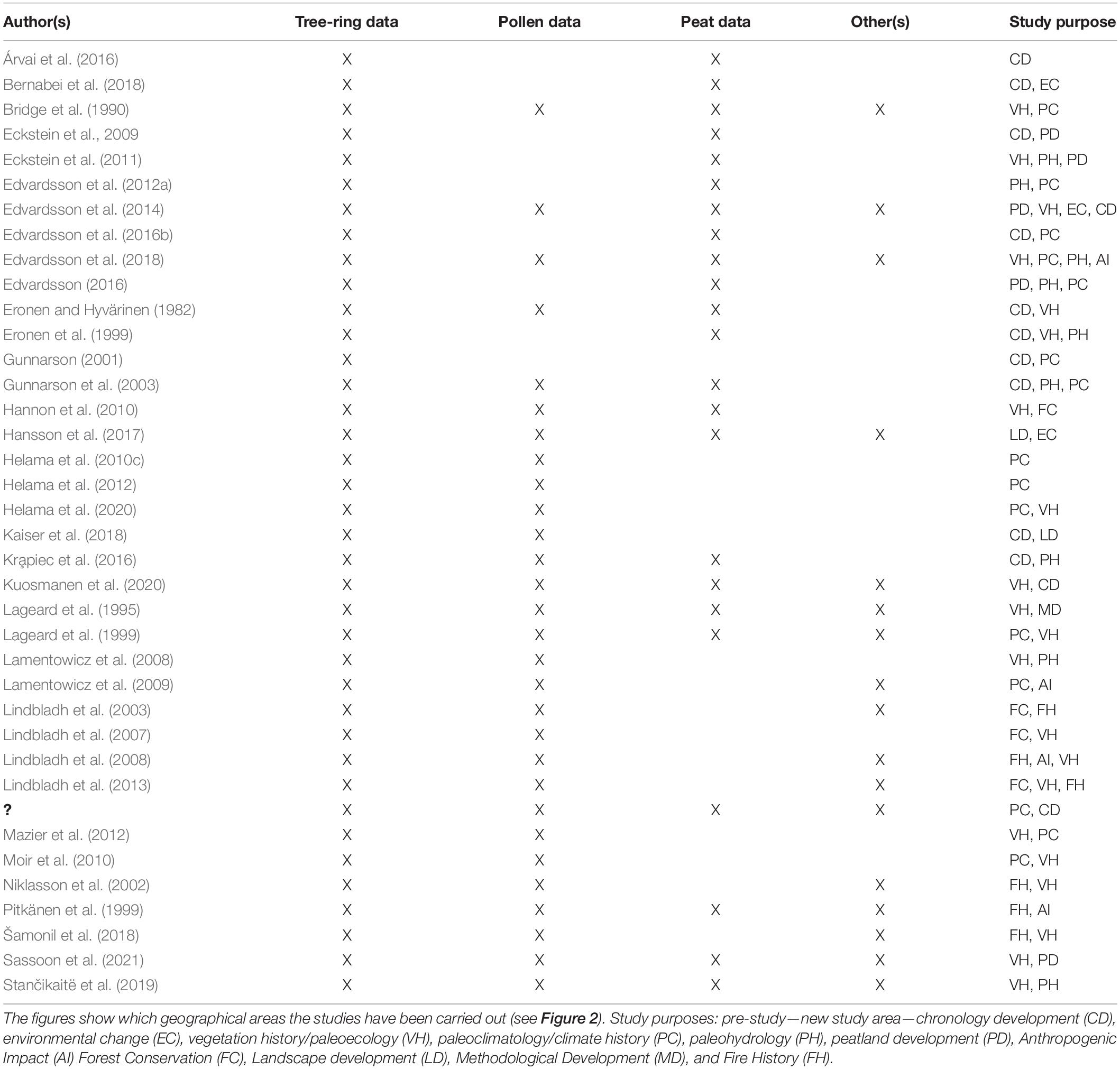
Table 1. Multi-proxy studies including tree-ring data (dendrochronology) in combination with stratigraphic proxy data including pollen data, peat stratigraphic data (e.g., content and degree of humification), and other(s) stratigraphic proxy data (e.g., plant macrofossil, charcoal, and testate amoebae).
Comparison Between Records With Different Precision and Time Resolution
The various proxy records in the reviewed literature (Table 1) have different time resolution (annual to centennial) and margins of errors (0 year up to centuries), which means that it is usually not possible to merge tree-ring and stratigraphic records without e.g., smoothing the age scale on the tree-ring record from annual to decadal. The reviewed records also cover different periods, ranging from recent centuries to the Holocene (Figure 3). Since tree-ring chronologies and stratigraphic proxy records are of significance to both paleoclimatologists and archeologists, the literature contains a mixture of records that quote ages as both calibrated years before present (cal. BP i.e., before 1950 CE) and calendar years (CE/BCE). Consequently, in this review when we refer to dendrochronologically dated tree-ring ages, we quote the age in CE/BCE, whereas the calibrated radiocarbon dates (cal. BP) are given as alternative in brackets, but where an event is known by a BP age, we quote the cal. BP age (calibrated years before 1950 CE) and refer to an approximate BCE age in brackets.
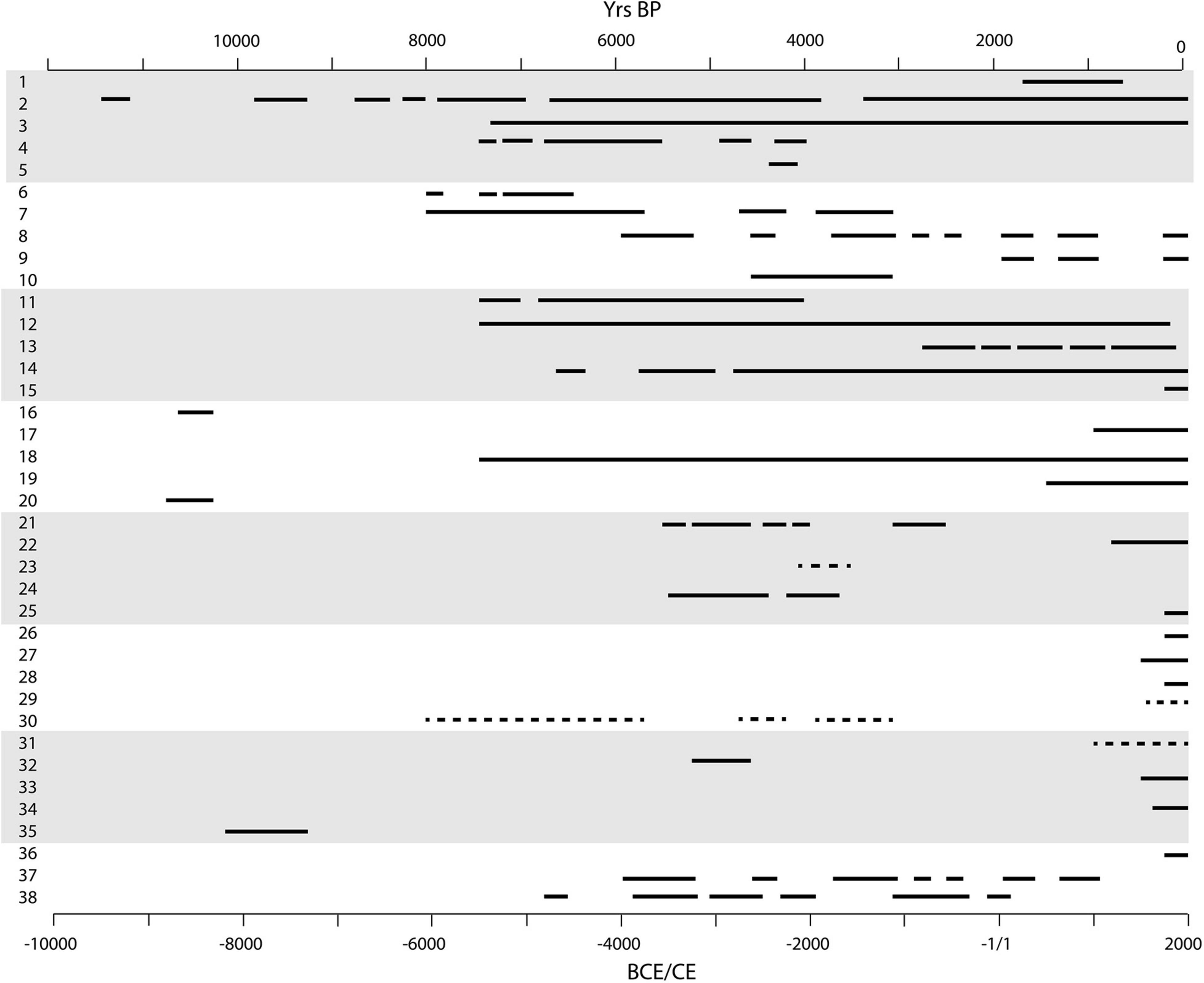
Figure 3. The black lines show the main periods covered by the tree-ring data presented in the 38 reviewed studies (see Table 1). The dashed lines show periods tree-ring data used, but not presented in the studies covers. For most of the studies the paleobotanical data covers longer, sometimes significantly longer, periods. The figures correspond to those in Table 1 and Figure 2.
Results and Discussion
Dendrochronological Data Types
Tree-ring based paleoenvironmental proxy records can be roughly divided into two categories according to the type of tree-ring data used and analyzed: (i) chronological and (ii) growth comparisons. The first category (“dates as data”) consist of records of sample replication, i.e., the number of overlapping trees from which a tree-ring chronology has been developed (Figures 4A,B). In this form, the dendrochronological data is used to quantify the number of dated tree-ring samples per annum, which can be used to illustrate depositional histories of subfossil trees through time (Gunnarson et al., 2003; Helama et al., 2005, 2010a, 2017a, 2020; Edvardsson et al., 2016a). Such histories can in turn be linked to past climatic and environmental changes that affected the density of tree populations, or their presence/absence, as well as deposition and preservation of trees. Dendrochronological data therefore may be used as records indicative of such changes in the past. The processes leading to changes in the rate of tree deposition and those affecting the preservation potential of trees (i.e., taphonomy) are not universal but vary between sites and regions and, as a consequence, the depositional histories of subfossil trees are linked to a range of climatic and environmental variables, especially to changes in moisture (Gunnarson, 2001, 2008; Gunnarson et al., 2003; Edvardsson et al., 2016a; Helama et al., 2017a) and temperature (Helama et al., 2004, 2005, 2010a). Divergent courses of depositional histories may be obtained for different sedimentary settings in nearby sites, as was evident for the accumulation records of pine in peatland and lake sites in southern Finland (Helama et al., 2017a). Tree deposition records usually show long-term (centennial to millennial) changes in moisture and temperature, but they can also be connected to more abrupt changes, leading to phases of tree establishment and/or die-off (Leuschner et al., 2002; Edvardsson et al., 2012a; Figures 4A,B). In addition to purely temporal comparisons, depositional histories over a larger region or within a range of tree-ring sites may be used for reconstructing the tree population occurrence/disappearance across the sites. Near ecotones, such as altitudinal and latitudinal timberlines, such tree-ring data may be highly indicative of past changes in the location of the treeline and/or timberline (Eronen et al., 1999; Helama et al., 2004, 2010a, 2020), the advances and retreats of which are typically temperature-driven (Harsch et al., 2009). Both short- and long-term changes in depositional histories inferred from dendrochronological data have been compared to records of multiple other proxy types. Compared to studies where subfossil tree remains are radiocarbon (14C) dated (Eronen, 1979; Kullman, 2002), dendrochronological records benefit from the dating precision/accuracy of tree-ring cross-dating (Fritts, 2012; Schweingruber, 2012; Cook and Kairiukstis, 2013) making it possible to juxtapose the lifespans of the studied trees exactly and thus more reliably indicate the past density fluctuations of the tree populations (Helama et al., 2005, 2010a).
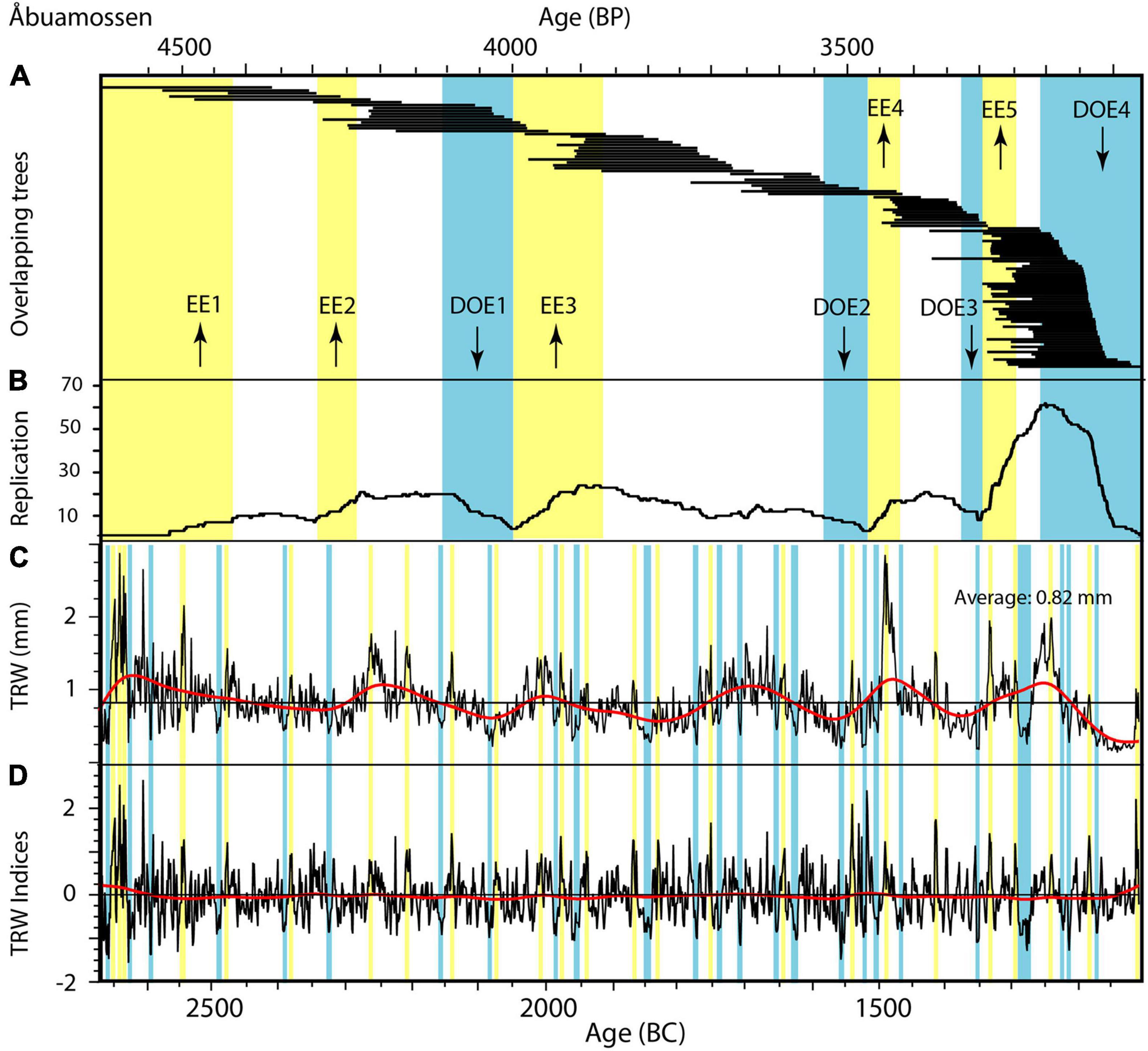
Figure 4. Example showing a tree-replication record and a tree-ring width chronology. The example is based on the 1561-year tree-ring chronology from Åbuamossen (Edvardsson, 2013). (A) The horizontal black lines represent individual trees sorted by ending years. Five tree-establishment (EE1–5) and four dying-off (DOE1–4) events are shown in yellow and blue, respectively. (B) Sample replication, number of overlapping samples. (C) Averaged tree-ring width (TRW) chronology (mm). (D) Averaged and standardized TRW chronology (dimensionless indices). The smooth red curves are 20-year low-pass filter splines highlighting the low-frequency patterns of variability. The blue fields are periods of 3 years in a row or longer with depressed growth (TRW indices < 1 SD), whereas the yellow fields show corresponding periods with strong growth (TRW indices > 1 SD). The replication record show population changes, often on a decadal resolution whereas the tree-ring width data show the year-by-year growth variability of the trees.
In the second category of tree-ring based paleoenvironmental studies, tree-ring chronologies provide data in the form of past tree-growth variability, in the form of tree-ring width, maximum late wood density, or other growth-related parameters. These should preferably be linked to a specific climatic variable such as temperature or precipitation. But before such a link is established, the tree-ring width data series usually go through a process named standardization (Cook, 1985; Biondi and Qeadan, 2008; Helama et al., 2017b), also referred to as detrending or indexing. The aim of the detrending is to minimize the influence of trends related to the age and geometry of single trees in the tree-ring data and thereby amplify climate or environment related signals in the data series (Cook and Kairiukstis, 2013). The standardization also means that the raw tree-ring data with millimeter scale is transformed to dimensionless tree-ring indices (Figures 4C,D). However, to obtain the best possible correlation between climate-related parameters and the tree-ring data, parameters other than tree-ring width are sometimes used. Examples of such parameters can be maximum latewood density (Briffa et al., 1988; Matskovsky and Helama, 2014), blue intensity (Björklund et al., 2015), and stable isotopes (McCarroll and Loader, 2004). Furthermore, it is possible to study scars caused by damages to the trees (Stoffel and Bollschweiler, 2008; Edvardsson et al., 2021), wood anatomical changes (von Arx et al., 2016), and trace elements (Hoffmann et al., 1994) that are detected in the annual rings for environmental and climatological studies. The benefit of all such types of tree-ring data is not only the dating precision/accuracy of dendrochronological cross-dating but also the annual resolution inherent to tree-ring archives (Fritts, 2012; Schweingruber, 2012; Cook and Kairiukstis, 2013; Figures 4C,D). Due simply to dendrochronological cross-dating, where the tree-ring variability is rigorously synchronized among all specimens in the chronology, tree rings allow for the development of paleoclimatic records with a high temporal veracity of each reconstructed event. In Fennoscandia, the construction of millennia-long subfossil tree-ring chronologies (Eronen et al., 2002; Grudd et al., 2002; Linderholm and Gunnarson, 2005; Helama et al., 2008) has indeed enabled tree-ring based paleoclimatic reconstructions comparable in length to other proxy records and have thus made it possible to compare dendrochronology (i.e., tree-ring based) and other proxy records over similar timescales (Figure 5).
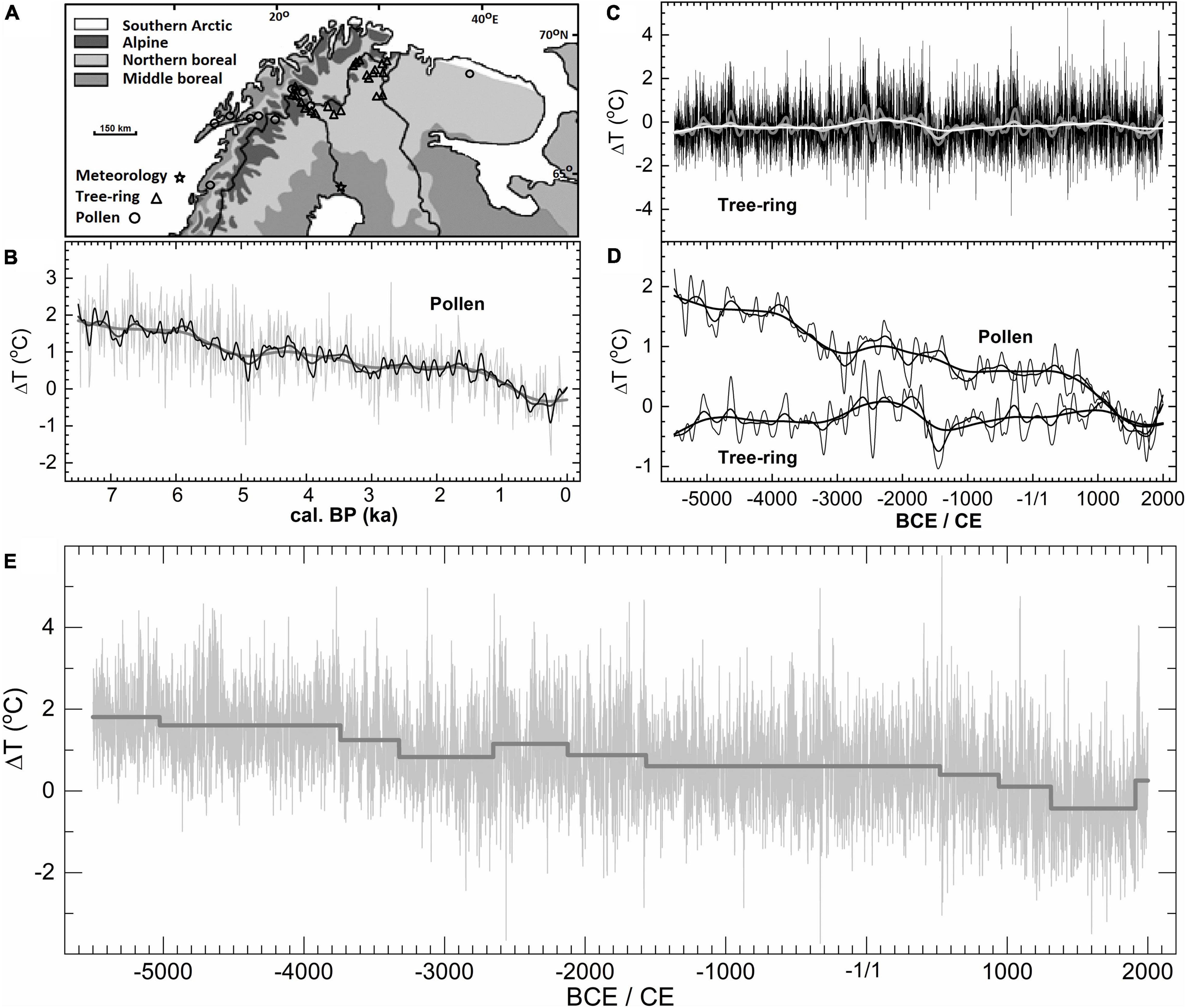
Figure 5. (A) A map of northern Fennoscandia with sites and proxy records used for the proxy-fusions (Helama et al., 2010c, 2012). Locations of the meteorological station and the lakes used for tree-ring studies (one triangle may indicate multiple nearby lakes) and pollen analysis, with approximate major vegetation zones. (B) Reconstructed July temperatures based on the initial data of pollen (C) and tree-ring data, and (D) the low-frequency comparison of pollen and tree-ring proxy records, shown as raw values and as filtered to exhibit the timescales of centennial, multi-centennial, and millennial components of the variability. (E) Annually resolved July temperature estimates produced by the proxy-fusion (Helama et al., 2012) shown with the temperature regimes and shifts in the reconstructed values. All temperature records (ΔT) are shown relative to the reference period AD 1950–2000.
Historical Overview—Studies of Environmental Changes and Climate Dynamics Based on Subfossil Trees
For close to two centuries, researchers have studied layers of subfossil tree remains discovered in peatlands (Dau, 1829; Steenstrup, 1842; Sernander, 1890; Birks, 2008; Birks and Seppä, 2010; Nielsen and Helama, 2012), but it was not until the late 1960s that serious attempts to develop tree-ring chronologies from such peatland trees were initiated (Pilcher et al., 1977). During the 1970s and 1980s, several multi-millennial tree-ring chronologies were developed from subfossil oak excavated from peatlands in Ireland and Germany (Pilcher et al., 1977, 1984; Leuschner and Delorme, 1984). Apart from being valuable for dating of archeological material, the chronologies can also allowed for detailed paleoclimatological reconstructions and enable construction of radiocarbon calibration curves (Pearson, 1986; Reimer et al., 2020). In Scandinavia, bog oak chronologies with the potential to cover the last 8,000 years are under construction (Edvardsson et al., 2016a, unpublished data).
Oak was the tree species initially used for multi-millennial chronologies from north European peatlands, but the interest in pines later increased, as they proved to have the potential to extend further back than the oak chronologies in some regions (Pilcher et al., 1995; Friedrich et al., 2004). In northwest Germany, extensive work on peatland pines have been performed (Leuschner et al., 2007; Eckstein et al., 2009, 2011), with overlapping chronologies reaching back to 6703 BCE (Achterberg et al., 2018). In Scandinavia and the Baltic countries, pine represents the most common tree species used for dendrochronological studies based on subfossil trees (Pukienë, 1997; Gunnarson, 1999; Helama et al., 2008; Edvardsson et al., 2012a, 2016b). In central and southern Sweden, peatland pines have been used to develop several multi-millennial tree-ring chronologies (Gunnarson, 1999, 2008; Edvardsson et al., 2012a,b; Edvardsson, 2016). In northern Finland, subfossil pines from peatlands and lacustrine sediments have been used for decades for dendrochronological studies (Eronen et al., 1999). Tree-ring analyses of this material yielded a continuous more than 7,600-year long (from 5634 BCE to present) tree-ring chronology (Eronen et al., 2002; Helama et al., 2008). In northern Sweden, nearly as long tree-ring chronology constructed from subfossil pine covers the period 5407 BCE to 1997 CE (Grudd et al., 2002). The suitability of subfossil pines for long-term climate and paleohydrology reconstructions has now been shown in a range of studies (Pilcher et al., 1995; Grudd et al., 2000; Lageard et al., 2000; Boswijk and Whitehouse, 2002; Leuschner et al., 2007; Eckstein et al., 2009; Moir et al., 2010; Edvardsson et al., 2012a; Helama et al., 2017a).
Depositional Environment—Subfossil Trees
Subfossil wood usable for dendrochronological studies is found in both lakes (Eronen et al., 2002; Grudd et al., 2002; Gunnarson, 2008; Helama et al., 2008) and peatlands (Leuschner et al., 2002; Eckstein et al., 2009; Edvardsson et al., 2016a). Depositional processes and preservation conditions are, however, different between the two environments. Wood retrieved from lake sediments primarily represents trees growing at, or within a short distance of, the lake shore that have fallen into the lake. There, tree trunks are either immediately incorporated into previously deposited sediments or gradually embedded through subsequent sedimentation, and constantly oxygen-poor conditions in the lake environment imply a high preservation potential. However, because the tree rings reflect growing conditions in a different environment (on land) and may have formed prior to the surrounding sediments, it is usually difficult to directly relate tree-ring data to changes in lake sediment stratigraphy. The stratigraphic position of wood found in peatlands is, on the other hand, generally indicative of the peatland surface at which the trees were growing, although fallen tree trunks may partly be embedded in peat that was recently formed when the trees died (Edvardsson et al., 2012a, 2014). Consequently, it may be feasible to directly relate tree-ring data to peat-stratigraphic changes. Wood found in peat may, however, also represent trees growing on drier ground in marginal areas of the peatland that either have fallen into the adjacent peatland or become embedded in peat during phases of rapid peatland expansion (Edvardsson et al., 2014). Moreover, peatland wood may experience a gradual change to anaerobic conditions until it is completely buried and no longer affected by changes in groundwater level, which implies a generally lower preservation potential than for wood buried in lakes (Edvardsson et al., 2016a).
An additional challenge when integrating tree-ring data from lake wood with sediment stratigraphy is that Holocene lake sediments often are relatively homogenous, which means that sediment stratigraphy alone provides limited paleoenvironmental and paleoclimatic information. Late-glacial sediments are, by contrast, usually characterized by distinct stratigraphic changes that can be used to infer changes in environmental and climatic conditions, but such sediments are not expected to contain wood from trees as they were deposited in essentially tree-less environments. Still, lakes constitute valuable archives for subfossil wood (Eronen et al., 2002; Grudd et al., 2002; Linderholm and Gunnarson, 2005; Helama et al., 2008), and there are examples of studies successfully integrating dendrochronological data obtained from lake wood with proxy records from the same sediment (Helama et al., 2012).
Linking Peat Stratigraphic Records and Tree Horizons
Largely, peat consists of accumulated litter from the plants that once formed the vegetation at the peatland surface and have become preserved because of predominantly wet and anaerobic conditions, but remains of other organisms also occur as a minor component. Consequently, peat deposits essentially constitute accumulations of plant macrofossils, but they also contain other macro- and microscopic remains of, e.g., charcoal fragments, pollen, and testate amoebae, which makes them excellent for multi-proxy paleoenvironmental and paleoclimatic studies (Chambers et al., 2012). In addition, peat deposits may contain large pieces of wood, sometimes referred to as megafossils, either as scattered finds or distinct horizons rich in tree stumps and trunks. It was noted already in pioneering peat studies in Denmark (Dau, 1829; Steenstrup, 1842) that peat layers containing remains of subfossil wood were generally darker in color and more strongly decomposed compared to other parts of the peat stratigraphy. These differences were ascribed to climatic changes with strongly decomposed peat rich in wood reflecting drier but possibly also warmer conditions. Such climatic inferences were later extended to Scandinavian peat stratigraphies displaying alternating layers with different degrees of decomposition but lacking wood horizons. They were also increasingly based on changes in peat type, i.e., changes in the composition of peat-forming plants identified through plant macrofossil analysis. This resulted in the development of the Blytt-Sernander scheme for postglacial climatic changes (Blytt, 1881; Sernander, 1908; Birks, 2008; Birks and Seppä, 2010). Later, peat horizons characterized by an abrupt transition from well-decomposed to well-preserved Sphagnum peat were frequently observed during extensive peat-stratigraphic studies in southern Sweden, and these were named recurrence surfaces by Granlund (1932) and proposed to represent regionally synchronous and rapid changes toward wetter conditions.
Paleoenvironmental and paleoclimatic studies based on peat largely rely on documentation of peat-forming vegetation and degree of peat decomposition, but this peat-stratigraphic information is usually complemented by proxy records of peat decomposition and bog-surface wetness with high resolution based on analysis of, for example, organic matter bulk density, light transmission/absorption, C/N ratio, plant macrofossils, and testate amoebae (Chambers et al., 2012). Obviously, such records are necessary to integrate dendrochronological data from subfossil wood with peat-stratigraphic records and thereby accurately place tree establishment and degeneration phases into the context of long-term environmental and climatic changes (Edvardsson et al., 2014, 2016a).
Dating and Dating Constraints of Stratigraphic Proxy Records From Lakes and Peatlands
Proxy records from lakes and peatlands are usually dated by radiocarbon dating. Peat can often be radiocarbon dated directly, although care should be taken to avoid dating roots, which can penetrate underlying layers and thus lead to too young ages. Dates for lake sediment are preferably based on terrestrial macrofossils, as dates of bulk sediment or aquatic organisms are often affected by older carbon, which can come both from long residence times of carbon in the lake system (reservoir effect) and from for example carbonate rich bedrock (hard water effect). Young lake sediments (up to ca. 150 years) can be dated using the isotope 210Pb (Appleby and Oldfield, 1983). Once a number of radiometric dates have been obtained from a sediment or peat sequence, they are calibrated and combined into an age-depth model, so that the age of samples taken at other depths can be estimated. The uncertainty on such interpolated ages depends on the number of dates in the core, their individual uncertainties and the shape of the age-depth relationship and is often in the range of centuries (Telford et al., 2004). Although this can be improved by using a high number of dates and Bayesian approaches to age-depth modeling, the maximum precision obtainable is still measured in decades rather than years.
The temporal resolution and accuracy thus differ greatly between dendrochronological data and sedimentary records, often hampering direct comparisons and data integration. Annually laminated (varved) lake sediments, however, provide a possibility to study sedimentary paleoecological proxies, such as geochemistry, isotopes, and microfossils at annual or seasonal resolution comparable to that of tree rings. However, the dating control obtained by varve counting is, unlike in dendrochronological data, usually affected by increasingly large dating uncertainties toward the past (Theuerkauf et al., 2019). This is partly because varves form in soft lake sediments and are therefore less well defined and more easily disturbed than tree rings, and therefore have higher counting uncertainties; and partly because varve formation is highly site specific and influenced by many factors impacting the lake. The most critical drawback for downcore uncertainties in varve dating is the lack of cross-dating (between the sediment cores) which is routinely applied in dendrochronological studies between the tree samples (Fritts, 2012; Cook and Kairiukstis, 2013). Thus, the varves can usually not be correlated regionally between sites, the way tree-ring chronologies can. However, combining varve and tree ring data may provide a way to overcome these problems.
Pollen Analysis—Overview and Comparison to Tree-Ring Records
Pollen analysis is a well-established scientific method used to provide estimates for the quantitative occurrence of various plant species over time in the past (Hicks, 2001). In most environments, enormous quantitates of pollen are spread and deposited, and they are preserved in lakes and peatlands, making them one of the most widely available paleoecological proxies, both in terms of number of sites and the temporal extent of the records. By comparing the pollen composition in stratigraphic records with observations of present pollen-vegetation and climate relationships it is possible to reconstruct the terrestrial vegetation of the past, as well as use the method to reveal past ecological and climatic changes (Bradshaw and Lindbladh, 2005). Usually, large basins contain pollen from extensive areas, and thereby showing large-scale regional vegetation changes (Sugita, 1994, 2007a), whereas pollen records from small basins to a higher degree represent local conditions (Jacobson and Bradshaw, 1981; Sugita, 2007b; Overballe-Petersen and Bradshaw, 2011).
Models for the quantitative relationship between pollen deposition at a site and the surrounding vegetation developed gradually since the 1960s with the calculation of pollen correction factors for different tree species (Davis, 1963; Andersen, 1970). But quantification of past vegetation is complicated by the non-linear relationship between vegetation and pollen percentages known as the “Fagerlind effect” (Fagerlind, 1952) as well as by the differential dispersal of pollen types from the source plants to the pollen sites (Tauber, 1965). But in the 1980s, the Extended R-value model that mathematically solves the Fagerlind effect was developed (Parsons and Prentice, 1981; Prentice and Parsons, 1983) and this was combined with physical models of pollen dispersal- and deposition (Prentice, 1985), resulting in a clearer definition of the pollen source area of different basin types (Sugita, 1994). Many studies on calibrating, testing and applying these models, as well as using the models for quantitative vegetation reconstruction at regional and local scales have been performed (Sugita, 2007a,b; Hellman et al., 2008; Nielsen and Odgaard, 2010; Mehl and Hjelle, 2015; Fredh et al., 2016).
A simultaneous, but only partly related model development has taken place in the field of pollen/climate relationships, where different statistical techniques have been applied to obtain pollen-based climate reconstructions, as recently reviewed by Chevalier et al. (2020). Much of the pioneering work again took place in Scandinavia, where interpretation of pollen assemblages in terms of climate started with von Post (1918). At first, interpretations were qualitative, but Iversen (1941) presented the first quantitative pollen-based temperature reconstructions for the Holocene. Later the use of large calibration datasets of surface pollen spectra and climate data developed, along with multivariate, and lately Bayesian statistical methods to apply them to fossil data for reconstructions of past climate variability, also facilitated by the increasing availability of pollen data through open-access databases (Chevalier et al., 2020). As with vegetation reconstruction, the scale of climatic information that can be obtained from a pollen record depends on the pollen source area of the site, local conditions, and by long distance transported pollen. Human impact can also affect the relationship between vegetation and climate, and potentially lead to biases which are hard to quantify (Chevalier et al., 2020). In substantially impacted landscapes, pollen-based climate reconstructions should be evaluated carefully by comparison to other proxies, such as dendrochronological data.
A major difference between sedimentary-based pollen data and dendrochronological data is obviously their temporal resolution. The relatively low resolution of non-varved sedimentary data combined with dating uncertainties as discussed in section “Dating and Dating Constraints of Stratigraphic Proxy Records From Lakes and Peatlands,” usually makes the comparison of pollen data and the relatively short (typically at most ∼ 100–150 years in length) instrumental climate records impossible. Therefore, pollen and climate data are not usually related on temporal but on spatial scales (Barnekow et al., 2007). Thus, the transfer functions used to transform pollen data into estimates of past climate parameters are typically constructed using a calibration set of spatially collected surface-sediment samples, expected to represent the modern pollen spectra in comparable sedimentary environments (Seppä and Birks, 2001; Seppä et al., 2004). As an example, the calibration dataset used by Bjune et al. (2009) was based on more than three hundred Nordic lakes sampled along contemporary climate gradients (Seppä and Birks, 2001). Although the transfer functions, calibrated and evaluated on spatial scales (Seppä and Birks, 2001; Seppä and Bennett, 2003; Seppä et al., 2004), they are applied backward in time and thus over temporal scales. By contrast, in dendroclimatology, the calibration, verification, and implementation of the transfer function are all applied over temporal scales (Cook and Kairiukstis, 2013).
In an overall comparison between pollen and dendrochronological records, it is obvious that more records covering the Holocene have been constructed from pollen than tree rings, and that pollen records from more geographical areas therefore are accessible. It is simply more realistic to generate long records covering the main part of the Holocene from pollen compared to subfossil trees, and to find sites with sufficient amounts of pollen to reconstruct long records are by far more common than places with subfossil wood. Both pollen and tree-ring records might be affected by humans, an important factor to consider when analyzing both types of data. Both methods can be considered as time consuming, but if multi-millennia-long datasets are to be constructed, tree-ring data is usually the most problematic and sometimes close to impossible. At the same time, the precision of the dates, the annual time resolution and the annual variations of the data series are superior once a chronology has been constructed. The differences between the pollen and tree-ring records mean that many of the studies reviewed that include both proxy records present significantly longer and more coherent data series from pollen than from the tree rings (Pitkänen et al., 1999; Šamonil et al., 2018; Stančikaitë et al., 2019).
Other Stratigraphic Proxies and How They Can Complement Dendrochronology
Plant macrofossils, e.g., seeds, fruits, leaves, and fragments of wood and bark, are a common source of paleoecological and paleoclimatic information in lake-sediment and peat studies (Birks, 2007). In contrast to pollen, macrofossils are usually not transported long distances due to their larger size. Plant macrofossils thereby provide more reliable information on local conditions, but little information on the regional landscape. In addition, they can often be identified with a higher taxonomic precision compared to pollen (Birks, 2007). On the other hand, these advantages are counteracted by the fact that plant macrofossils are less common and more sporadically present in lake sediment and peat sequences. Plant macrofossils are therefore often used in combination with pollen (Birks and Birks, 2000). In lake sediments, plant macrofossils are derived from both limnic and terrestrial plants, whereas in peat they are mainly derived from plants growing on the peatland but may also have been transported by wind over short distances from the surrounding landscape (Birks, 2007). Wood fragments from trees and shrubs encountered during macrofossil analysis usually contain too few annual rings to be used for dendrochronological studies but can be helpful when reconstructing vegetation composition based on finds of subfossil trees and pollen data (Edvardsson et al., 2014).
Charcoal can also be detected in stratigraphic records and can provide concrete evidence of fire frequency and intensity at local to regional scale (Patterson and Backman, 1988). Charcoal records are often used to supplement fire reconstructions based on historical documents or tree-ring data (Whitlock and Larsen, 2002). For example, Edvardsson et al. (2015) dated a fire scar in pine trees growing at the peatland Kerëplis in Lithuania to 1949 CE. Charcoal particles that most likely originate from the same fire could be detected in the upper part of the peat stratigraphy and could thus be dated using dendrochronology. Precise dating of fire scars in trees in combination with charcoal analysis of lake-sediment and peat sequences thereby has a great potential for detailed reconstructions of historical fires in a region (Pitkänen et al., 1999; Stivrins et al., 2019).
Not only plant remains can be preserved in stratigraphic records, also parts of animals can be detected and studied. Insects are extremely diverse and present in a wide range of habitats and have therefore become an essential contributor in many paleoecological, environmental, and archeological studies (Buckland et al., 2018). Paleoentomology is the study of prehistoric insects including beetles, and their diversity in combination with a long evolutionary history as well as the potential to be preserved in stratigraphic records provides an excellent tool for environmental and climatic reconstructions. Apart from a few studies by, for example, Boswijk and Whitehouse (2002) and Kuosmanen et al. (2020), few attempts have been made to integrate dendrochronological data with paleoecological reconstructions based on paleoentomological analyses. However, this approach is considered to have great potential for studies with conservation purposes (Lindbladh et al., 2013).
In many stratigraphic records from aquatic to moist habitats such as lakes, rivers and wetlands, testate amoebae (also referred to as rhizopods, thecamoebians and arcellaceans) can be detected (Charman, 2001; Mitchell et al., 2008). Testate amoebae is a group of single-celled, shelled organisms and useful indicators of moisture conditions (Charman, 2001). Stratigraphic studies of testate amoebae often include analyses of pollen and macrofossils (Mitchell et al., 2008), but a few attempts have been made to also include tree-ring data from subfossil trees. Like pollen and macrofossil records, stratigraphic testate amoebae records are limited by chronological inaccuracies. Despite this, it was possible to combine data from living peatland pines with the recent testate amoebae record to develop a local hydrological reconstruction for the Čepkeliai peatland in Lithuania (Edvardsson et al., 2019). This should encourage more multiproxy studies where not only pollen, plant macrofossils and testate amoebae are used but studies that also include subfossil trees.
Multiproxy Studies
Multiproxy Fusion Studies for Paleoclimatic Reconstructions
There are studies where subfossils of both spruce (Picea abies) and birch (Betula) have been explored for paleoclimatic purposes (Kullman, 1995, 1996; Holtmeier and Broll, 2006), but when it comes to studies over long periods of time based on subfossil trees, Scots pine (Pinus sylvestris L.) is by far the most investigated tree species, both in northern Finland (Eronen et al., 1999, 2002; Helama et al., 2002, 2004, 2005, 2008, 2010a,b,c, 2019, 2020) and Sweden (Sonesson, 1974; Grudd et al., 2002; Gunnarson et al., 2003). A large proportion of these trees have been found in lakes with oxygen-poor sediments and have grown on mineral (riparian) soils or on thick layers of organogenic riparian substrate. Dendroclimatic studies on living pine trees in similar environments in northern Fennoscandia show that temperature most often is a significantly growth limiting factor (Helama et al., 2002, 2012; Linderholm et al., 2015). The subfossil pine material is therefore assumed to be suitable for dendroclimatic studies aiming to reconstruct temperature variability of the past.
During the late 1970s (Eronen, 1979) and early 1980s, studies on the Holocene history of the pine forests of northern Fennoscandia were performed using radiocarbon dated pine trees from northwestern Finnish Lapland and northern Norway (Eronen and Hyvärinen, 1982). Eronen and Hyvärinen (1982) used pollen records from lake sediments and radiocarbon dated subfossil pine trees. The study showed that the tree and pollen records agreed outlining the history of the pine forests of northern Fennoscandia, and showed that pine spread around 7500–7000 cal. BP (∼5550–5050 BCE) and that the extent of the forest was greatest between 7,000 and 4000 cal. BP (∼5050–2050 BCE; Eronen and Hyvärinen, 1982). Since then, many dendrochronological studies using radiocarbon and dendrochronologically dated subfossil pine materials from northern Finland have been conducted, and the materials have constantly been complemented and improved (Eronen et al., 1999, 2002; Helama et al., 2008, 2015, 2019, 2020).
Topical to this review, Helama et al. (2010c, 2012) compared (and integrated) July temperature reconstructions derived independently from pollen and tree-ring width data as previously published for northern Fennoscandia (Bjune et al., 2009; Helama et al., 2009, 2010b). These studies were based on two paleobotanical proxies, namely spectra of fossil pollen and tree rings of subfossil pine trees from the same region (Helama et al., 2010c, 2012). A summary pollen-based July temperature reconstruction was produced by stacking the individual pollen-based reconstructions from 11 sites representing northern Fennoscandia, expressed as sample-specific deviations from the pollen-inferred present-day July temperature at each site (Bjune et al., 2009; Seppä et al., 2009; Birks et al., 2010). The initial tree-ring dataset of Eronen et al. (2002) was processed to remove biological trends from the raw data series using an approach similar to the regional-curve-standardization (Briffa et al., 1996; Helama et al., 2017b), with an exception that the curvature of the growth trend was adjusted for past changes in the pine population density in the study region (Helama et al., 2010a,b). The rationale behind these comparisons was the hypothesis that the two types of proxy data could be connected to climate variability in a timescale-dependent fashion and, accordingly, their climatic signals could be fused in similar manner making use of the low- and high-frequencies from pollen-stratigraphic and tree-ring data, respectively. First, the two independent reconstructions were correlated over the past millennium i.e., since 750 CE (Helama et al., 2010c). It was shown that that the pollen and tree-ring based reconstructions exhibited similar low-frequency (centennial and longer scales) temperature variability, revealed using timescale-dependent filtering techniques. That is, the two proxies did not only show in what direction conditions for various species are changing, it was also demonstrated when changes had happened and at what rate. The same method was used to surmount obvious geochronological discrepancies (see above sections for the discussion on differing dating and resolution issues), thus enabling reconciliation of the proxies where the proxy-fusion was achieved as a sum of the timescale-dependent components of the original reconstructions (for details, see Helama et al., 2010c, 2012). Reconstructions based both on pollen and tree-ring data have been performed, and the results have been correlated and validated against the instrumentally observed temperature data available for the region over the 1802–2002 CE period (instrumental data from Holopainen and Vesajoki, 2001; Klingbjer and Moberg, 2003; Holopainen, 2006). Over longer intervals, the proxy-fusions exhibited the climatic reversals of the “Medieval Climate Anomaly” (during the tenth to thirteenth centuries CE) and the “Little Ice Age” (during the 14th to nineteenth centuries CE), as well as the twentieth century warming, with a decadal temperature amplitude of 2.5°C between the warmest and coolest decades of the study period (750–1998 CE) (Helama et al., 2010c).
Second, the pollen and tree-ring based reconstructions of July temperature variability were compared over the longest possible time interval, that is over the past 7,500 years (Helama et al., 2012). A new, enlarged spatial pollen-climate calibration dataset was used to develop a robust calibration-function model to transform fossil pollen spectra into temperature estimates. Also, this comparison highlighted overall climatic variability from inter-annual to millennial timescales. Two major discrepancies between the two proxies concerned the highest and lowest frequencies of the reconstructed temperatures: the absence of temperature estimates of the shortest term in the pollen-based proxy and, on the other hand, a lack of a long-term temperature trend in the tree-ring based temperatures over the past 7,500 years. Despite the discrepancies, the low-frequency (i.e., long-term) temperatures variations showed similarities, most well expressed as positive temperature excursion between 3000 and 2000 BCE (which they termed “mid-Holocene warmth”) and as a negative anomaly over much of the past millennium (“Little Ice Age”) (Figure 5). Like a previous study (Helama et al., 2010c), the combined i.e., fused temperature record (representing low-frequency signal from pollen data and high-frequency signal from tree-ring data) correlated positively and significantly with the instrumentally observed temperatures with verification statistics indicating real skill in the reconstruction (Helama et al., 2012). On much longer scales, the final fused temperature reconstruction showed a cooling of 0.26°C per millennium and a cooling of 2.0°C since 5500 BCE. Annual resolution of the record allowed the years 1934 and 1937 to be evaluated among the warmest, and the years 1903 and 1910 are among the coldest summers in the context of the late and mid Holocene temperatures. Moreover, the average reconstructed mid and late Holocene climate was found to remain 0.85°C warmer than the twentieth century CE.
These studies indicated that a method of proxy fusion could be successfully used to integrate the proxy-specific reconstructions, as exemplified for past changes in summer temperature in the pollen-stratigraphic and dendroclimatic data from northern Fennoscandia. To do so, the proxies should exhibit similar temporal coverage, be similar in their climatic response, and exhibit coherence at low frequencies (given that the sedimentary pollen data lacks the high-frequency amplitudes). Although the studies from northern Finland proved that both temperature reconstructions were similar in their climate response (Helama et al., 2010c) and that calibration in time, performed on the fused reconstructions, compared reasonably with instrumentally recorded July temperature, it is important to be aware that this connection may not apply everywhere. In the adjacent areas in southern Scandinavia, the conditions appear quite different in comparison to the North as the temperature is not as strongly controlling the radial tree growth of the trees in these more temperate site conditions.
Another study from northern Finland using climate reconstructions from dendrochronological data, which showed the potential to improve the possibilities for quantitative interpretations of pollen data was performed by Mazier et al. (2012). The pollen productivity of trees varies from year to year, depending on climatic conditions (Andersen, 1974), and in northern Fennoscandia is often strongly dependent on summer temperature (Huusko and Hicks, 2009). By combining absolute pollen productivity estimates for pine for warm and cold years, respectively, from pollen trap data (Mazier et al., 2012), with a summer temperature reconstruction based on tree growth parameters obtained from tree rings (Young et al., 2012), it was thereby possible to obtain a more detailed reconstruction of variations in pine tree biomass over the last 1,000 years from high resolution pollen data from a peat core (Mazier et al., 2012). The relative pollen productivity of different species, used in the landscape reconstruction algorithm may also vary depending on climate, although the relationship is still poorly understood (Broström et al., 2008). If this relationship can be quantified, the potential for using independent climate data from tree rings to improve vegetation reconstructions could be even greater, although this of course requires that relative pollen production and tree growth are limited largely by the same climatic variables in the region of study. For northern Fennoscandia, this is likely to be the case in terms of summer temperature, but in other regions the relationship may be more complicated.
Multi-proxy studies covering recent decades show that there still is additional potential to unlock. Once such example is a study from North Germany by Theuerkauf et al. (2019) in which high-resolution pollen data from a varved lake sediment and tree-ring data were used for dating and paleoclimatic interpretation. Theuerkauf et al. (2019) noted that peaks in annual beech (Fagus sylvatica) pollen deposition correlated with minima in beech tree-ring width, a relationship that was used as a link between their varved lake sediments and tree-ring record. The study mainly focused on the period 1980–2017 CE but sets an example how pollen data from a varved sediment record and local tree-ring data can be integrated.
Multiproxy Paleohydrology Studies
A study from southern Finland showed that the replication curves of pine tree-ring chronologies from peatland and lake sites may demonstrate divergent courses of depositional histories (Helama et al., 2017a). This comparison demonstrated that the accumulation of peatland (subfossil) pines was high, but that of lake site subfossil trees low during the Medieval Climate Anomaly, whereas the accumulation of peatland pines was found to decline toward the Little Ice Age during which that of lake site pines increased. The results were confirmed by comparisons of the water level reconstructions based on subfossils of the chironomid midges and cladoceran water fleas (Luoto, 2009; Nevalainen et al., 2011; Nevalainen and Luoto, 2012). Generally, these microfossil-based reconstructions of late Holocene water depth variations showed a lowered water table during the late part of the first millennium CE, coinciding with peatland tree accumulation. Moreover, rising water tables not later than the twelfth century CE were indicated, consistent with the increasing lake site tree accumulation. These results from differing site conditions of subfossil pine accumulation were taken to demonstrate the relative roles of recruitment and preservation potentials, and thus of paleoecological and taphonomic processes, in controlling the tree accumulation in peatland and lake environments, respectively (Helama et al., 2017a). Comparisons between tree-ring and subfossil animal remain (Cladocera, midges, mayfly, and caddisfly mandibles) based reconstructions, of hydroclimatic conditions were also carried out in nearby sites in eastern Finland (Luoto and Helama, 2010; Luoto et al., 2013; Nevalainen et al., 2013). These comparisons showed that the two types of reconstructions could be used for interpretations of multiple paleoclimatic (seasonal) variables such as precipitation, stream flow, lake water depth, the length of the ice-free season and water temperatures. For example, Luoto and Helama (2010) showed that the Medieval Climate Anomaly was characterized by warm and dry summer conditions, whereas their stream flow reconstruction portrayed simultaneously increased values, implying enhanced spring floods after snowy winters. During the Little Ice Age, wetter climate conditions were reconstructed, however, with the stream flow indicating less snowy winters. Overall, their results were explained by the low-frequency changes in the North Atlantic Oscillation producing contrasting seasonal climate regimes over similarly long timescales in the region (Luoto and Helama, 2010).
Comparative Multiproxy Studies
Both climate and vegetation differ significantly between northern Fennoscandia and the area around the southern Baltic Sea. Which species that can be used as well as the type of paleoecological or climatic information that can be obtained therefore differ greatly between south and north. When it comes to dendrochronological studies, tree species such as pedunculate oak (Quercus robur L.) and beech (Fagus sylvatica L.) occur in addition to the previously mentioned Scots pine. Subfossil oaks have been studied in the reviewed literature (Christensen, 2007; Edvardsson et al., 2014), but not to the same extent as in Germany (Leuschner et al., 2002), Netherlands (Sass-Klaassen and Hanraets, 2006), and Ireland (Brown and Baillie, 2012). The most dominant tree species in the subfossil archives is once again Scots pine (Edvardsson et al., 2012a,b, 2014; Edvardsson, 2016). However, in addition to the geographical location, also the stratigraphic context differs between the subfossil pines from southern and northern sites. The subfossil pine material available in southern Fennoscandia that is a couple of thousand years or older comes almost exclusively from peatlands (Edvardsson et al., 2012a,b; Edvardsson, 2016), and similar availability has been noted in the Baltic states (Pukienë, 1997; Edvardsson et al., 2016b, 2018). Moreover, there are examples with pine materials from submerged landscapes in the Baltic Sea (Hansson et al., 2017; Edvardsson et al., 2021). Furthermore, the correlation between temperature records and tree-ring width in these regions are not as strong as in northern Fennoscandia (Helama et al., 2010c; Edvardsson et al., 2015). Pollen based climate reconstruction from this region may also be hampered by the strong human influence on vegetation composition during the late Holocene. In the multiproxy studies from southern Sweden and the Baltic region, the tree-ring and stratigraphic records can therefore not be integrated in similar manner as described in northern Finland (Helama et al., 2010c, 2012). Instead, records from different proxies have almost exclusively been used for comparative studies in which the data series have been placed next to each other to identify any common patterns (Edvardsson et al., 2012a, 2014). Most often, a similar procedure has been performed in studies from Germany (Eckstein et al., 2009, 2011) and Lithuania (Edvardsson et al., 2016b, 2018; Stančikaitë et al., 2019). In its simplicity, this way of comparing data series can be effective in identifying common patterns in the data types. In Edvardsson et al. (2016b), for example, total tree replication was compared to long-term pollen-based summer temperature deviations (Heikkilä and Seppä, 2010), a water-table reconstruction based on testate amoebae assemblages (Sillasoo et al., 2007), and a lake-level reconstruction from ostracods (Sohar and Kalm, 2008). When combined, the records provide a more detailed story of the transition from the relatively warm mid Holocene to the colder and more changeable late Holocene. More remarkable is that the temperature and water-level changes recorded in the stratigraphic archives during the last millennium appear limited, while several major changes were detected in the tree-replication record. For example, complete absence of peatland trees was noted during cold episodes such as the Little Ice Age and the Migration Period, whereas rapid tree colonization events were recorded during relatively warm episodes such as the Roman and Medieval Warm Periods (Edvardsson et al., 2016b).
Underutilized Geographic Areas
As highlighted in Figure 2, there are several geographic regions with potential for new or improved As highlighted in Figure 2, there are several geographic regions with potential for new or improved in depth paleoecological and paleoclimatic studies combining dendrochronology and paleobotanical datasets. A long tradition of vegetation and climate studies based on e.g., pollen and plant macrofossils from lake sediments (Barnekow, 1999; Rundgren and Beerling, 1999; Bigler et al., 2006) and peat records (Kokfelt et al., 2010; Gałka et al., 2017) from the Abisko region, northern Sweden, have resulted in extensive vegetation and climate records covering substantial parts of the Holocene. In parallel, the 7,400-year tree-ring chronology from Torneträsk (Grudd et al., 2002) as well as chronologies from the Scandinavian mountains (Linderholm et al., 2015) have been developed. These types of datasets should be able to be combined for more detailed studies than what has been done so far. In a similar way, it should be possible to combine and continue working on dendrochronological (Edvardsson et al., 2012b) and peat stratigraphic records (Kylander et al., 2013) from southern Sweden. Furthermore, the similar landscape with lakes and peat bogs found in Estonia, Latvia, and western Russia should make it possible to find subfossil trees to construct tree-ring chronologies to compare with the proxy data already existing in these regions. In Denmark, there is an oak material (Christensen, 2007; Edvardsson et al., 2016a) that might well be linked to other local proxy archives (Bradshaw et al., 2005). Belarus, the Netherlands and Ireland are other examples that can be considered as regions with coexisting archives and/or potential for new proxy data for paleoecological or paleoclimatological studies (Figure 2).
Conclusion
This review aimed to convince our readers that the benefits of combining, or even better integrating, proxy records generated from tree rings and stratigraphic sequences can result in improved paleoecological and paleoclimatic reconstructions. We also hope the review can inspire to new research initiatives aiming to overcome the difficulties with the proxy integration, and thereby contribute to improved long-term ecosystem and climate reconstructions as well as a firmer basis for future nature conservation initiatives.
The studies reviewed (Table 1) and the examples discussed illustrate the great benefits of the integrated use of tree-ring data and paleoecological records from lakes and peatlands. Several examples show that adding paleoecological records to the tree-ring records can result in substantially longer and more continuous time series, as well as more detailed and robust paleoecological and paleoclimatic interpretations. In parallel, the tree-ring records can generate improved time resolution and dating accuracy to the paleoecological records (Figure 6). But since there are major differences between dendrochronology and the other techniques discussed, comparison or integration must be done with caution. Moreover, the knowledge of how difficult it can be to construct multi-millennium long tree-ring chronologies for such studies is hard to circumvent. However, we have identified areas where there are long tree-ring chronologies completed or under construction that could be integrated into future studies (Figure 2).
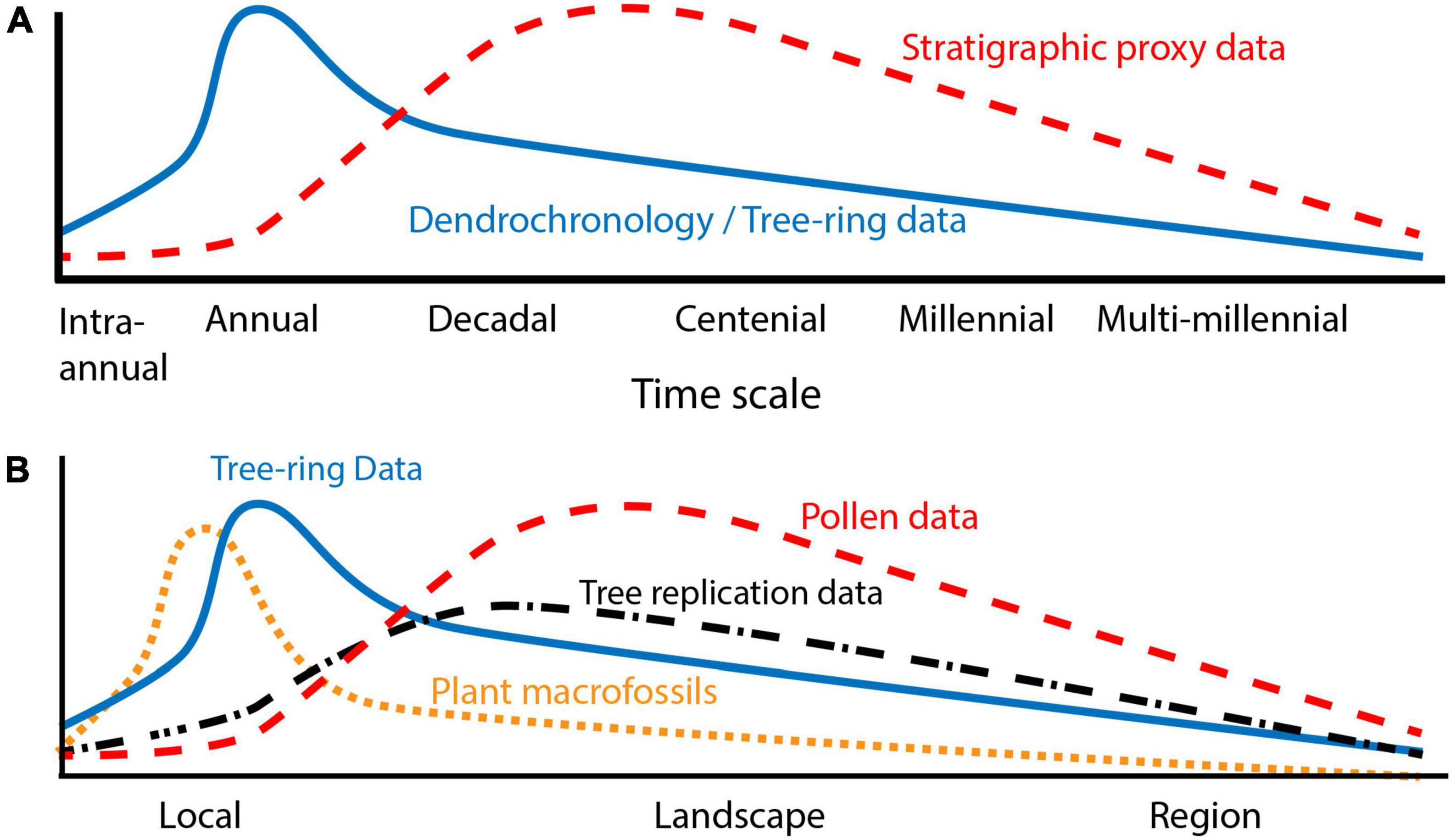
Figure 6. (A) A schematic presentation of temporal scales associated with tree-ring data (blue line) and stratigraphic proxy data (red dashed line). (B) The proxy data discussed, namely tree-ring data (blue line), tree replication data (black line with dashes and dots), pollen data (red dashed line), and plant macrofossils (orange dotted line), also differ in the spatial scales at which they best represent paleoecological and paleoclimatic information. The advantages of integrating several types of proxies are that both time scale and geographical coverage can be improved.
One of the most prominent differences between dendrochronology and other methods reviewed is that dendrochronology can be considered both as a dating technique and as an annually resolved proxy for paleoecological and climatic reconstructions, whereas the other proxies discussed generally rely on independent dating, e.g., with radiocarbon, and have varying time resolution depending on local peat or sediment accumulation rates. On the other hand, proxies extracted from a common stratigraphic sequence automatically have a comparable time resolution and time scale. It is therefore relatively easy to directly compare such data series. Moreover, stratigraphic records can often offer uninterrupted multi-millennial paleobotanical, and paleoenvironmental records for studies of vegetation and climate dynamics. In comparison, the relatively short life cycle of the trees growing in the boreal zone makes it practically impossible to create continuous records based on tree-ring data in a similar manner. Consequently, tree-ring data from hundreds of trees per millennium are often needed when long tree-ring chronologies are to be constructed (Figure 4A). This makes the work of constructing tree-ring chronologies like those from northern Fennoscandia (Grudd et al., 2002; Helama et al., 2008) a process that can last for decades. Although there are pros and cons to all the methods covered by this review (Table 2), the examples discussed show that there are ways to integrate them to be able to benefit from the advantage of combining different data and proxies.
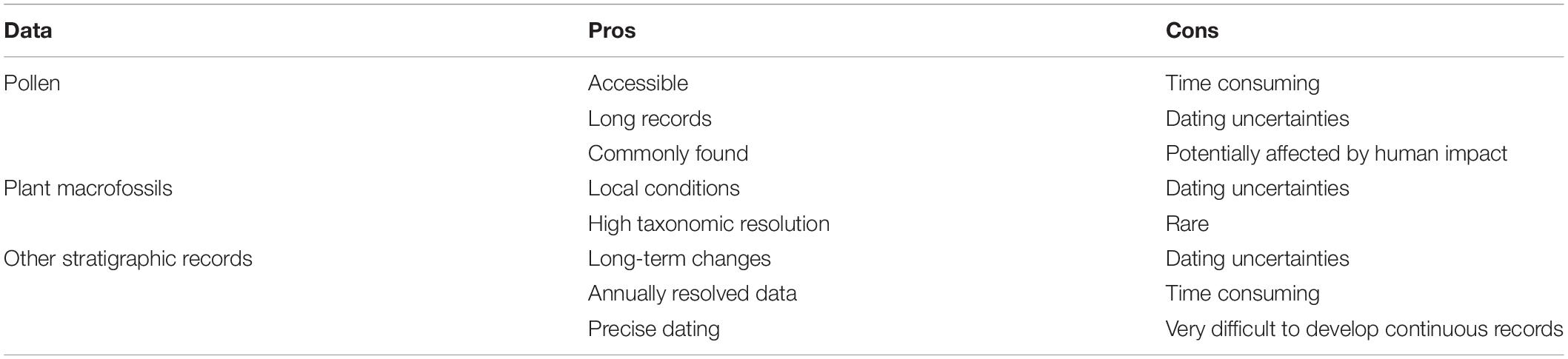
Table 2. Pros and cons of different proxy data for the assessment of paleoecological and paleoclimatic reconstructions.
There are several multi-proxy studies covering recent decades, such as Theuerkauf et al. (2019) discussed in section “Multiproxy Studies,” or articles by Jalkanen et al. (2008) and Edvardsson et al. (2019), that set examples how paleoecological sediment records and local tree-ring data can be integrated. Studies like these shows that there still is additional potential to unlock from the paleoecological records. It will of course be a challenge to perform similar studies over several millennia, but it would be worth the efforts if stratigraphic proxy records could be developed with the same dating accuracy as tree-ring archives offer.
Author Contributions
JE designed the review and wrote the manuscript. SH helped design the research, conducted the dendrochronology and pollen interface, and helped write the manuscript. MR helped to design the research, provided the sections in the text about stratigraphic analysis and comments on writing. AN helped to design the research, provided the sections in the text about pollen analysis and comments on writing. All authors contributed to the article and approved the submitted version.
Funding
JE was supported by the Swedish Research council (grant: 2016-04726) and FORMAS (grant: 2020-00936). SH was supported by the Academy of Finland.
Conflict of Interest
The authors declare that the research was conducted in the absence of any commercial or financial relationships that could be construed as a potential conflict of interest.
Publisher’s Note
All claims expressed in this article are solely those of the authors and do not necessarily represent those of their affiliated organizations, or those of the publisher, the editors and the reviewers. Any product that may be evaluated in this article, or claim that may be made by its manufacturer, is not guaranteed or endorsed by the publisher.
Acknowledgments
We would like to thank Matts Lindbladh (SLU) and two reviewers for valuable input that improved the article.
Footnotes
References
Achterberg, I. E. M., Eckstein, J., Birkholz, B., Bauerochse, A., and Leuschner, H. H. (2018). Dendrochronologically dated pine stumps document phase-wise bog expansion at a northwest German site between ca. 6700 and ca. 3400 BC. Clim. Past 14, 85–100.
Andersen, S. T. (1970). The Relative Pollen Productivity and Pollen Representation of North European Trees, and Correction Factors for Tree Pollen Spectra. DGU II. Række, 96. Copenhagen: Danmarks Geologiske Undersøgelser.
Andersen, S. T. (1974). Wind conditions and pollen deposition in a mixed deciduous forest. II. Seasonal and annual pollen deposition 1967–1972. Grana 14, 64–77.
Appleby, P. G., and Oldfield, F. (1983). The assesment of 210Pb data from sites with varying sediment accumulation rates. Hydrobiologia 103, 29–35. doi: 10.1007/978-94-009-7290-2_5
Árvai, M., Popa, I., Mîndrescu, M., Nagy, B., and Kern, Z. (2016). Dendrochronology and radiocarbon dating of subfossil conifer logs from a peat bog, Maramures̨; Mts, Romania. Quat. Int. 415, 6–14.
Barnekow, L. (1999). Holocene tree-line dynamics and inferred climatic changes in the Abisko area, northern Sweden, based on macrofossil and pollen records. Holocene 9, 253–265.
Barnekow, L., Loader, N. J., Hicks, S., Froyd, C. A., and Goslar, T. (2007). Strong correlation between summer temperature and pollen accumulation rates for Pinus sylvestris, Picea abies and Betula spp. in a high-resolution record from northern Sweden. J. Quat. Sci. 22, 653–658. doi: 10.1002/jqs.1096
Becker, B. (1983). The long-term radiocarbon trend of the absolute German oak tree-ring chronology, 2800 to 800 BC. Radiocarbon 25, 197–203.
Bernabei, M., Bontadi, J., and Nicolussi, K. (2018). Observations on Holocene subfossil tree remains from high-elevation sites in the Italian Alps. Holocene 28, 2017–2027. doi: 10.1177/0959683618798149
Bigler, C., Barnekow, L., Heinrichs, M. L., and Hall, R. I. (2006). Holocene environmental history of Lake Vuolep Njakajaure (Abisko National Park, northern Sweden) reconstructed using biological proxy indicators. Veg. Hist. Archaeobot. 15, 309–320.
Biondi, F., and Qeadan, F. (2008). A theory-driven approach to tree-ring standardization: defining the biological trend from expected basal area increment. Tree Ring Res. 64, 81–96.
Birks, H. H. (2007). “Plant macrofossil introduction,” in Encyclopedia of Quaternary Science, Vol. 3, eds S. A. Elias and B. V. Elsevier (Oxford: Oxford), 2266–2288. doi: 10.1111/j.1469-8137.1995.tb04325.x
Birks, H. H., and Birks, H. J. B. (2000). Future uses of pollen analysis must include plant macrofossils. J. Biogeogr. 2000, 31–35. doi: 10.1046/j.1365-2699.2000.00375.x
Birks, H. J., and Seppä, H. (2010). Late-Quaternary palaeoclimatic research in Fennoscandia – A historical review. Boreas 39, 655–673. doi: 10.1111/j.1502-3885.2010.00160.x
Birks, H. J. B. (2008). “Holocene climate research – progress, paradigms, and problems,” in Natural Climate Variability and Global Warming: a Holocene Perspective, eds R. W. Battarbee and H. Binney (Hoboken, NJ: Wiley-Blackwell), 7–57.
Birks, H. J. B., Heiri, O., Seppä, H., and Bjune, A. E. (2010). Strengths and weaknesses of quantitative climate reconstructions based on late-Quaternary biological proxies. Open Ecol. J. 3, 68–110.
Birks, J., Battarbee, R., Mackay, A., and Oldfield, F. (2014). Reconstructing Holocene climates from pollen and plant macrofossils in Global change in the Holocene. Milton Park: Routledge, 358–373.
Björklund, J., Gunnarson, B. E., Seftigen, K., Zhang, P., and Linderholm, H. W. (2015). Using adjusted blue intensity data to attain high-quality summer temperature information: a case study from Central Scandinavia. Holocene 25, 547–556. doi: 10.1177/0959683614562434
Bjune, A. E., Seppä, H., and Birks, H. J. B. (2009). Quantitative summer-temperature reconstructions for the last 2000 years based on pollen-stratigraphical data from northern Fennoscandia. J. Paleolimnol. 41, 43–56. doi: 10.1007/s10933-008-9254-y
Blytt, A. (1881). Die Theorie der wechselnden kontinentalen und insularen Klimate. Engl. Bot. Jahrb. 2, 1–50.
Boswijk, G., and Whitehouse, N. J. (2002). Pinus and Prostomis: a dendrochronological and palaeoentomological study of a mid-Holocene woodland in eastern England. Holocene 12, 585–596.
Bradshaw, E. G., Rasmussen, P., and Odgaard, B. V. (2005). Mid-to late-Holocene land-use change and lake development at Dallund S0, Denmark: synthesis of multiproxy data, linking land and lake. Holocene 15, 1152–1162.
Bradshaw, R. H. W., and Lindbladh, M. (2005). Regional spread and stand-scale establishment of Fagus sylvatica and Picea abies in Scandinavia. Ecology 86, 1679–1686.
Bridge, M. C., Haggart, B. A., and Lowe, J. J. (1990). The history and palaeoclimatic significance of subfossil remains of Pinus sylvestris in blanket peats from Scotland. J. Ecol. 1990, 77–99. doi: 10.2307/2261038
Briffa, K. R., Jones, P. D., and Schweingruber, F. H. (1988). Summer temperature patterns over Europe: a reconstruction from 1750 AD based on maximum latewood density indices of conifers. Quat. Res. 30, 36–52. doi: 10.1016/0033-5894(88)90086-5
Briffa, K. R., Jones, P. D., Schweingruber, F. H., Karlen, W., and Shiyatov, S. G. (1996). “Tree-ring variables as proxy-climate indicators: problems with low-frequency signals,” in Climate Variations and Forcing Mechanisms of the Last 2000 Years, eds P. D. Jones, R. S. Bradley, and J. Jouzel (Berlin: Springer), 9–41.
Broström, A., Nielsen, A. B., Gaillard, M. J., Hjelle, K., Mazier, F., Binney, H., et al. (2008). Pollen productivity estimates of key European plant taxa for quantitative reconstruction of past vegetation: a review. Veg. Hist. Archaeob. 17, 461–478. doi: 10.1007/s00334-008-0148-8
Brown, D. M., and Baillie, M. G. L. (2012). Confirming the existence of gaps and depletions in the Irish oak tree-ring record. Dendrochronologia 30, 85–91. doi: 10.1016/j.dendro.2010.09.003
Buckland, P. I., Buckland, P. C., and Olsson, F. (2018). “Paleoentomology: insects and other arthropods in environmental archaeology,” in Encyclopedia of global archaeology, ed. C. Smith (Cham: Springer).
Chambers, F. M., Booth, R. K., De Vleeschouwer, F., Lamenowicz, M., Le Roux, G., Mauquoy, D., et al. (2012). Development of proxy-climate indicators from peats. Quat. Int. 268, 21–33.
Charman, D. J. (2001). Biostratigraphic and palaeoenvironmental applications of testate amoebae. Quat. Sci. Rev. 20, 1753–1764. doi: 10.1016/s0277-3791(01)00036-1
Chevalier, M., Davis, B. A., Heiri, O., Seppä, H., Chase, B. M., Gajewski, K., et al. (2020). Pollen-based climate reconstruction techniques for late Quaternary studies. Earth Sci. Rev. 2020:103384.
Cook, E. R. (1985). A time series analysis approach to tree ring standardization Doctoral dissertation. Tucson: University of Arizona.
Cook, E. R., and Kairiukstis, L. A. (eds) (2013). Methods of dendrochronology: applications in the environmental sciences. New York, NY: Springer Science & Business Media.
D’Arrigo, R., Wilson, R., and Jacoby, G. (2006). On the long-term context for late twentieth century warming. J. Geophys. Res.: Atmospheres 111, D3.
Dau, J. H. C. (1829). Allerunterthänigster Bericht an die Königliche Dänische Rentekammerüber die Torfmoore Seelands. Glydendahl und Hinnichs. Munich: Bavarian State Library.
Davis, M. B. (1963). On the theory of pollen analysis. Am. J. Sci. 261, 897–912. doi: 10.2475/ajs.261.10.897
Eckstein, J., Leuschner, H. H., and Bauerochse, A. (2011). Mid-Holocene pine woodland phases and mire development–significance of dendroecological data from subfossil trees from northwest Germany. J. Veg. Sci. 22, 781–794.
Eckstein, J., Leuschner, H. H., Bauerochse, A., and Sass-Klaassen, U. (2009). Subfossil bog-pine horizons document climate and ecosystem changes during the Mid-Holocene. Dendrochronologia 27, 129–146.
Edvardsson, J. (2013). Holocene climate change and peatland dynamics in southern Sweden based on tree-ring analysis of subfossil wood from peat deposits Doctoral dissertation. Sweden: Lund University.
Edvardsson, J. (2016). Mid-to Late Holocene climate transition and moisture dynamics inferred from South Swedish tree-ring data. J. Quat. Sci. 31, 256–264.
Edvardsson, J., Baužienë, I., Lamentowicz, M., Šimanauskienë, R., Tamkevičiûtë, M., Taminskas, J., et al. (2019). A multi-proxy reconstruction of moisture dynamics in a peatland ecosystem: a case study from Čepkeliai. Lithuania. Ecol. Indic. 106:105484.
Edvardsson, J., Leuschner, H. H., Linderson, H., Linderholm, H. W., and Hammarlund, D. (2012b). South Swedish bog pines as indicators of Mid-Holocene climate variability. Dendrochronologia 30, 93–103.
Edvardsson, J., Linderson, H., Rundgren, M., and Hammarlund, D. (2012a). Holocene peatland development and hydrological variability inferred from bog-pine dendrochronology and peat stratigraphy–a case study from southern Sweden. J. Quat. Sci. 27, 553–563. doi: 10.1002/jqs.2543
Edvardsson, J., Stoffel, M., Corona, C., Bragazza, L., Leuschner, H. H., Charman, D. J., et al. (2016a). Subfossil peatland trees as proxies for Holocene palaeohydrology and palaeoclimate. Earth Sci. Rev. 163, 118–140. doi: 10.1016/j.earscirev.2016.10.005
Edvardsson, J., Corona, C., Mažeika, J., Pukienë, R., and Stoffel, M. (2016b). Recent advances in long-term climate and moisture reconstructions from the Baltic region: Exploring the potential for a new multi-millennial tree-ring chronology. Quat. Sci. Rev. 131, 118–126.
Edvardsson, J., Magnell, O., Hansson, A., Linderson, H., Sjöström, A., and Nilsson, B. (2021). Early Holocene bark-stripping damages as an indicator of large herbivores: Evidence from a submerged Mesolithic landscape in the Haväng area, southern Baltic basin. Holocene 2021:09596836211033213.
Edvardsson, J., Poska, A., Van der Putten, N., Rundgren, M., Linderson, H., and Hammarlund, D. (2014). Late-Holocene expansion of a south Swedish peatland and its impact on marginal ecosystems: Evidence from dendrochronology, peat stratigraphy and palaeobotanical data. Holocene 24, 466–476. doi: 10.1177/0959683613520255
Edvardsson, J., Rimkus, E., Corona, C., Šimanauskiene, R., Kažys, J., and Stoffel, M. (2015). Exploring the impact of regional climate and local hydrology on Pinus sylvestris L. growth variability – A comparison between pine populations growing on peat soils and mineral soils in Lithuania. Plant Soil 392, 345–356. doi: 10.1007/s11104-015-2466-9
Edvardsson, J., Stančikaitë, M., Miras, Y., Corona, C., Gryguc, G., Gedminienë, L., et al. (2018). Late-Holocene vegetation dynamics in response to a changing climate and anthropogenic influences–Insights from stratigraphic records and subfossil trees from southeast Lithuania. Quat. Sci. Rev. 185, 91–101. doi: 10.1016/j.quascirev.2018.02.006
Eronen, M. (1979). The retreat of pine forest in Finnish Lapland since the Holocene climatic optimum: a general discussion with radiocarbon evidence from subfossil pines. Fennia-Internat. J. Geogr. 157, 93–114.
Eronen, M., and Hyvärinen, H. (1982). Subfossil pine dates and pollen diagrams from northern Fennoscandia. Geologiska Föreningen i Stockholm Förhandlingar 103, 437–445.
Eronen, M., Hyvärinen, H., and Zetterberg, P. (1999). Holocene humidity changes in northern Finnish Lapland inferred from lake sediments and submerged Scots pines dated by tree-rings. Holocene 9, 569–580. doi: 10.1191/095968399677209885
Eronen, M., Zetterberg, P., Briffa, K. R., Lindholm, M., Meriläinen, J., and Timonen, M. (2002). The supra-long Scots pine tree-ring record for Finnish Lapland: Part 1, chronology construction and initial inferences. Holocene 12, 673–680.
Fredh, D., Mazier, F., Bragée, P., Lagerås, P., Rundgren, M., Hammarlund, D., et al. (2016). The effect of local land-use changes on floristic diversity during the past 1000 years in southern Sweden. Holocene 27, 694–711. doi: 10.1177/0959683616670464
Friedrich, M., Remmele, S., Kromer, B., Hofmann, J., Spurk, M., Kaiser, K. F., et al. (2004). The 12,460-year Hohenheim oak and pine tree-ring chronology from central Europe—a unique annual record for radiocarbon calibration and paleoenvironment reconstructions. Radiocarbon 46, 1111–1122.
Gałka, M., Szal, M., Watson, E. J., Gallego-Sala, A., Amesbury, M. J., Charman, D. J., et al. (2017). Vegetation succession, carbon accumulation and hydrological change in subarctic peatlands, Abisko, Northern Sweden. Permaf. Perigl. Proc. 28, 589–604.
Granlund, E. (1932). De svenska högmossarnas geologi. Stockholm: Sveriges Geologiska Undersökning Ser. C.
Grudd, H., Briffa, K. R., Gunnarson, B. E., and Linderholm, H. W. (2000). Swedish tree rings provide new evidence in support of a major, widespread environmental disruption in 1628 BC. Geophys. Res. Lett. 27, 2957–2960.
Grudd, H., Briffa, K. R., Karlén, W., Bartholin, T. S., Jones, P. D., and Kromer, B. (2002). A 7400-year tree-ring chronology in northern Swedish Lapland: natural climatic variability expressed on annual to millennial timescales. Holocene 12, 657–665. doi: 10.1191/0959683602hl578rp
Gunnarson, B. E. (1999). A 200-year tree-ring chronology of pine from a raised bog in Sweden: implication for climate change? Geografiska Annaler: Series A, Phys. Geogr. 81, 421–430. doi: 10.1111/j.0435-3676.1999.00071.x
Gunnarson, B. E. (2001). Lake level changes indicated by dendrochronology on subfossil pine, Jämtland, central Scandinavian Mountains, Sweden. Arctic, Antarctic, Alpine Res. 33, 274–281. doi: 10.2307/1552234
Gunnarson, B. E. (2008). Temporal distribution pattern of subfossil pines in central Sweden: perspective on Holocene humidity fluctuations. Holocene 18, 569–577.
Gunnarson, B. E., Borgmark, A., and Wastegård, S. (2003). Holocene humidity fluctuations in Sweden inferred from dendrochronology and peat stratigraphy. Boreas 32, 347–360.
Hannon, G., Niklasson, M., Brunet, J., Eliasson, P., and Lindbladh, M. (2010). How long has the hotspot been hot? Past stand-scale structures at Siggaboda Nature Reserve in southern Sweden. Biodivers. Conserv. 19, 2167–2187.
Hansson, A., Björck, S., Linderson, H., Rundgren, M., Nilsson, B., Sjöström, A., et al. (2017). Early Holocene landscape development and Baltic Sea history based on high-resolution bathymetry and lagoonal sediments in the Hanö bay, southern Sweden. In Under the sea: Archaeology and palaeolandscapes of the continental shelf. Cham: Springer, 197–209.
Harsch, M. A., Hulme, P. E., McGlone, M. S., and Duncan, R. P. (2009). Are treelines advancing? A global meta-analysis of treeline. Ecol. Lett. 12, 1040–1049. doi: 10.1111/j.1461-0248.2009.01355.x
Heikkilä, M., and Seppä, H. (2010). Holocene climate dynamics in Latvia, eastern Baltic region: a pollen-based summer temperature reconstruction and regional comparison. Boreas 39:705e719. doi: 10.1111/j.1502-3885.2010.00164.x
Helama, S., Arppe, L., Timonen, M., Mielikäinen, K., and Oinonen, M. (2015). Age-related trends in subfossil tree-ring δ13C data. Chem. Geol. 416, 28–35.
Helama, S., Eronen, M., and Timonen, M. (2010a). “Dendroécologie des bois fossiles dans le nord de la Laponie,” in La Dendroécologie: Principes, méthodes et applications, eds S. Payette and L. Filion (Québec: Presses de l’Université Laval), 709–730.
Helama, S., Macias Fauria, M., Mielikäinen, K., Timonen, M., and Eronen, M. (2010b). Sub-Milankovitch solar forcing of past climates: mid and late Holocene perspectives. Geol. Soc. Am. Bull. 122, 1981–1988. doi: 10.1130/b30088.1
Helama, S., Kuoppamaa, M., and Sutinen, R. (2020). Subaerially preserved remains of pine stemwood as indicators of late Holocene timberline fluctuations in Fennoscandia, with comparisons of tree-ring and 14C dated depositional histories of subfossil trees from dry and wet sites. Rev. Palaeobot. Palynol. 2020:104223.
Helama, S., Lindholm, M., Timonen, M., and Eronen, M. (2004). Dendrochronologically dated changes in the limit of pine in northernmost Finland during the past 7.5 millennia. Boreas 33, 250–259.
Helama, S., Lindholm, M., Timonen, M., and Eronen, M. (2005). Mid- and late Holocene tree population density changes in northern Fennoscandia derived by a new method using megafossil pines and their tree-ring series. J. Quat. Sci. 20, 567–575. doi: 10.1002/jqs.929
Helama, S., Lindholm, M., Timonen, M., Meriläinen, J., and Eronen, M. (2002). The supra-long Scots pine tree-ring record for Finnish Lapland: Part 2, interannual to centennial variability in summer temperatures for 7500 years. Holocene 12, 681–687.
Helama, S., Luoto, T. P., Nevalainen, L., and Edvardsson, J. (2017a). Rereading a tree-ring database to illustrate depositional histories of subfossil trees. Palaeontol. Electronica 20, 1–12.
Helama, S., Melvin, T. M., and Briffa, K. R. (2017b). Regional curve standardization: State of the art. Holocene 27, 172–177.
Helama, S., Mielikäinen, K., Timonen, M., and Eronen, M. (2008). Finnish supra-long tree-ring chronology extended to 5634 BC. Norw. J. Geogr. 62, 271–277.
Helama, S., Saranpää, P., Pearson, C. L., Arppe, L., Holopainen, J., Mäkinen, H., et al. (2019). Frost rings in 1627 BC and AD 536 in subfossil pinewood from Finnish Lapland. Quat. Sci. Rev. 204, 208–215.
Helama, S., Seppä, H., Birks, H. J. B., and Bjune, A. E. (2010c). Reconciling pollen-stratigraphical and tree-ring evidence for high-and low-frequency temperature variability in the past millennium. Quat. Sci. Rev. 29, 3905–3918.
Helama, S., Seppä, H., Bjune, A. E., and Birks, H. J. B. (2012). Fusing pollen-stratigraphic and dendroclimatic proxy data to reconstruct summer temperature variability during the past 7.5 ka in subarctic Fennoscandia. J. Paleolimnol. 48, 275–286. doi: 10.1007/s10933-012-9598-1
Helama, S., Timonen, M., Holopainen, J., Ogurtsov, M. G., Mielikäinen, K., Eronen, M., et al. (2009). Summer temperature variations in Lapland during the Medieval Warm Period and the Little Ice Age relative to natural instability of thermohaline circulation on multi-decadal and multi-centennial scales. J. Quat. Sci. 24, 450–456. doi: 10.1002/jqs.1291
Hellman, S., Gaillard, M.-J., Broström, A., and Sugita, S. (2008). The REVEALS model, a new tool to estimate past regional plant abundance from pollen data in large lakes: validation in southern Sweden. J. Quat. Sci. 23, 21–42.
Hicks, S. (2001). The use of annual arboreal pollen deposition values for delimiting tree-lines in the landscape and exploring models of pollen dispersal. Rev. Palaeobot. Palynol. 117, 1–29. doi: 10.1016/s0034-6667(01)00074-4
Hoffmann, E., Lüdke, C., Scholze, H., and Stephanowitz, H. (1994). Analytical investigations of tree rings by laser ablation ICP-MS. Fresenius J. Analy. Chem. 350, 253–259.
Holopainen, J. (2006). Reconstructions of Past Climates from Documentary and Natural Sources in Finland since the 18th Century. Doctoral dissertation, Department of Geology. Finland: University of Helsinki.
Holopainen, J., and Vesajoki, H. (2001). Varhainen lämpötilahavaintosarja Torniosta vuosilta 1737–1749. Terra 113, 196–201.
Holtmeier, F. K., and Broll, G. (2006). Radiocarbon-dated peat and wood remains from the Finnish Subarctic: Evidence of treeline and landscape history. Holocene 16, 743–751.
Huusko, A., and Hicks, S. (2009). Conifer pollen abundance provides a proxy for summer temperature: evidence from the latitudinal forest limit in Finland. J. Quat. Sci. 4, 522–528.
Iversen, J. (1941). Land occupation in Denmark’s Stone Age. Danmarks Geologiske Undersogelse II 66, 1–68.
Jacobson, G. L., and Bradshaw, R. H. (1981). The Selection of Sites for Paleovegetational Studies1. Quat. Res. 16, 80–96.
Jalkanen, R., Hicks, S., Aalto, T., and Salminen, H. (2008). Past pollen production reconstructed from needle production in Pinus sylvestris at the northern timberline: a tool for evaluating palaeoclimate reconstructions. Silva Fennica 42, 499–513.
Jansen, R. K., Cai, Z., Raubeson, L. A., Daniell, H., Depamphilis, C. W., Leebens-Mack, J., et al. (2007). Analysis of 81 genes from 64 plastid genomes resolves relationships in angiosperms and identifies genome-scale evolutionary patterns. Proc. Natl. Acad. Sci. 104, 19369–19374. doi: 10.1073/pnas.0709121104
Kaiser, K., Oldorff, S., Breitbach, C., Kappler, C., Theuerkauf, M., Scharnweber, T., et al. (2018). A submerged pine forest from the early Holocene in the Mecklenburg Lake District, northern Germany. Boreas 47, 910–925.
Klingbjer, P., and Moberg, A. (2003). A composite monthly temperature record from Tornedalen in northern Sweden, 1802e2002. Int. J. Climatol. 23, 1465–1494. doi: 10.1002/joc.946
Kokfelt, U., Reuss, N., Struyf, E., Sonesson, M., Rundgren, M., Skog, G., et al. (2010). Wetland development, permafrost history and nutrient cycling inferred from late Holocene peat and lake sediment records in subarctic Sweden. J. Paleolimnol. 44, 327–342. doi: 10.1007/s10933-010-9406-8
Kra̧piec, M., Margielewski, W., Korzeń, K., Szychowska-Kra̧piec, E., Nalepka, D., and Ľajczak, A. (2016). Late Holocene palaeoclimate variability: The significance of bog pine dendrochronology related to peat stratigraphy. The Puścizna Wielka raised bog case study (Orawa–Nowy Targ Basin, Polish Inner Carpathians). Quat. Sci. Rev. 148, 192–208. doi: 10.1016/j.quascirev.2016.07.022
Kullman, L. (1995). New and firm evidence for Mid-Holocene appearance of Picea abies in the Scandes Mountains, Sweden. J. Ecol. 1995, 439–447. doi: 10.2307/2261597
Kullman, L. (1996). Norway spruce present in the Scandes Mountains, Sweden at 8000 BP: new light on Holocene tree spread. Glob. Ecol. Biogeogr. Lett. 1996, 94–101.
Kullman, L. (2002). Boreal tree taxa in the central Scandes during the Late-Glacial: implications for Late-Quaternary forest history. J. Biogeogr. 29, 1117–1124. doi: 10.1046/j.1365-2699.2002.00743.x
Kuosmanen, N., Čada, V., Halsall, K., Chiverrell, R. C., Schafstall, N., Kuneš, P., et al. (2020). Integration of dendrochronological and palaeoecological disturbance reconstructions in temperate mountain forests. For. Ecol. Manage. 475:118413.
Kylander, M. E., Bindler, R., Cortizas, A. M., Gallagher, K., Mörth, C. M., and Rauch, S. (2013). A novel geochemical approach to paleorecords of dust deposition and effective humidity: 8500 years of peat accumulation at Store Mosse (the “Great Bog”), Sweden. Quat. Sci. Rev. 69, 69–82. doi: 10.1016/j.quascirev.2013.02.010
Lageard, J. G., Chambers, F. M., and Thomas, P. A. (1999). Climatic significance of the marginalization of Scots pine (Pinus sylvestris L.) c. 2500 BC at White Moss, south Cheshire. UK. Holocene 9, 321–331.
Lageard, J. G. A., Chambers, F. M., and Thomas, P. A. (1995). Recording and reconstruction of wood macrofossils in three-dimensions. J. Archaeol. Sci. 22, 561–567. doi: 10.1006/jasc.1995.0053
Lageard, J. G. A., Thomas, P. A., and Chambers, F. M. (2000). Using fire scars and growth release in subfossil Scots pine to reconstruct prehistoric fires. Palaeogeogr. Palaeoecol. 164, 87–99.
Lamentowicz, M., Cedro, A., Gałka, M., Goslar, T., Miotk-Szpiganowicz, G., Mitchell, E. A., et al. (2008). Last millennium palaeoenvironmental changes from a Baltic bog (Poland) inferred from stable isotopes, pollen, plant macrofossils and testate amoebae. Palaeogeogr. Palaeoecol. 265, 93–106. doi: 10.1016/j.palaeo.2008.04.023
Lamentowicz, M., Milecka, K., Gazka, M., Cedro, A., Pawlyta, J., Piotrowska, N., et al. (2009). Climate and human induced hydrological change since AD 800 in an ombrotrophic mire in Pomerania (N Poland) tracked by testate amoebae, macro-fossils, pollen and tree rings of pine. Boreas 38, 214–229. doi: 10.1111/j.1502-3885.2007.00004.x
Langdon, P. G., and Barber, K. E. (2005). The climate of Scotland over the last 5000 years inferred from multiproxy peatland records: inter-site correlations and regional variability. J. Quat. Sci. 20, 549–566. doi: 10.1002/jqs.934
Leuschner, H. H., Bauerochse, A., and Metzler, A. (2007). Environmental change, bog history and human impact around 2900 BC in NW Germany–preliminary results from a dendroecological study of a sub-fossil pine woodland at Campemoor, Dümmer Basin. Veg. Hist. Archaeobot. 16, 183–195. doi: 10.1007/s00334-006-0084-4
Leuschner, H. H., and Delorme, A. (1984). Extension of the Goettingen oak-tree-ring chronology for North-and South-Germany back to 4008 BC Forstarchiv. Germany.
Leuschner, H. H., Sass-Klaassen, U., Jansma, E., Baillie, M. G. L., and Spurk, M. (2002). Subfossil European bog oaks: population dynamics and long-term growth depressions as indicators of changes in the Holocene hydro-regime and climate. Holocene 12, 695–706. doi: 10.1191/0959683602hl584rp
Libby, W. F., Anderson, E. C., and Arnold, J. R. (1949). Age Determination by Radiocarbon Content: World-wide Assay of Natural Radiocarbons. Science 109, 227–228. doi: 10.1126/science.109.2827.227
Lindbladh, M., Brunet, J., Hannon, G., Niklasson, M., Eliasson, P., Eriksson, G., et al. (2007). Forest history as a basis for ecosystem restoration – a multi-disciplinary case-study in a south Swedish temperate landscape. Restor. Ecol. 15, 284–295. doi: 10.1111/j.1526-100x.2007.00211.x
Lindbladh, M., Fraver, S., Edvardsson, J., and Felton, A. (2013). Past forest composition, structures and processes–How paleoecology can contribute to forest conservation. Biol. Conserv. 168, 116–127.
Lindbladh, M., Niklasson, M., Karlsson, M., Björkman, L., and Churski, M. (2008). Close anthropogenic control of Fagus establishment and expansion in a Swedish protected landscape – implications for forest history and conservation. J. Biogeogr. 35, 682–697.
Lindbladh, M., Niklasson, M., and Nilsson, S. G. (2003). Long-time record of fire and open canopy in a high biodiversity forest in southeast Sweden. Biol. Conserv. 114, 231–243. doi: 10.1016/s0006-3207(03)00043-0
Linderholm, H. W., Björklund, J., Seftigen, K., Gunnarson, B. E., and Fuentes, M. (2015). Fennoscandia revisited: a spatially improved tree-ring reconstruction of summer temperatures for the last 900 years. Clim. Dynam. 45, 933–947.
Linderholm, H. W., and Gunnarson, B. E. (2005). Summer temperature variability in central Scandinavia during the last 3600 years. Geografiska Annaler. Series A. Physical Geography 87, 231–241. doi: 10.1111/j.0435-3676.2005.00255.x
Luoto, T. P. (2009). A Finnish chironomid- and chaoborid-based inference model for reconstructing past lake levels. Quat. Sci. Rev. 28, 1481–1489. doi: 10.1016/j.quascirev.2009.01.015
Luoto, T. P., and Helama, S. (2010). Palaeoclimatological and palaeolimnological records from fossil midges and tree-rings: the role of the North Atlantic Oscillation in eastern Finland through the Medieval Climate Anomaly and Little Ice Age. Quat. Sci. Rev. 29, 2411–2423.
Luoto, T. P., Helama, S., and Nevalainen, L. (2013). Stream flow intensity of the Saavanjoki River, eastern Finland, during the past 1500 years reflected by mayfly and caddisfly mandibles in adjacent lake sediments. J. Hydrol. 476, 147–153. doi: 10.1016/j.jhydrol.2012.10.029
Margielewski, W. Krąpiec, M., Kupryjanowicz, M. Fiłoc, M., Buczek, K. Stachowicz-Rybka, R., et al. (2022). Bog pine dendrochronology related to peat stratigraphy: palaeoenvironmental changes reflected in peatland deposits since the Late Glacial (case study of the Imszar raised bog, Northeastern Poland). Quat. Int. 613, 61–80. doi: 10.1016/j.quaint.2021.11.007
Mann, M. E., Zhang, Z., Hughes, M. K., Bradley, R. S., Miller, S. K., Rutherford, S., et al. (2008). Proxy-based reconstructions of hemispheric and global surface temperature variations over the past two millennia. Proc. Natl. Acad. Sci. 105, 13252–13257. doi: 10.1073/pnas.0805721105
Marcott, S. A., Shakun, J. D., Clark, P. U., and Mix, A. C. (2013). A reconstruction of regional and global temperature for the past 11,300 years. Science 339, 1198–1201. doi: 10.1126/science.1228026
Matskovsky, V. V., and Helama, S. (2014). Testing long-term summer temperature reconstruction based on maximum density chronologies obtained by reanalysis of tree-ring data sets from northernmost Sweden and Finland. Clim. Past 10, 1473–1487. doi: 10.5194/cp-10-1473-2014
Mauquoy, D., Yeloff, D., Van Geel, B., Charman, D. J., and Blundell, A. (2008). Two decadally resolved records from north-west European peat bogs show rapid climate changes associated with solar variability during the mid–late Holocene. J. Quat. Sci. 23, 745–763. doi: 10.1002/jqs.1158
Mazier, F., Nielsen, A. B., Broström, A., Sugita, S., and Hicks, S. (2012). Signals of tree volume and temperature in a high-resolution record of pollen accumulation rates in northern Finland. J. Quat. Sci. 27, 564–574. doi: 10.1002/jqs.2549
McCarroll, D., and Loader, N. J. (2004). Stable isotopes in tree rings. Quat. Sci. Rev. 23, 771–801.
Mehl, I. K., and Hjelle, K. L. (2015). From pollen percentage to regional vegetation cover — A new insight into cultural landscape development in western Norway. Rev. Palaeobot. Palynol. 217, 45–60.
Mitchell, E. A., Charman, D. J., and Warner, B. G. (2008). Testate amoebae analysis in ecological and paleoecological studies of wetlands: past, present and future. Biodivers. Conserv. 17, 2115–2137. doi: 10.1007/s10531-007-9221-3
Moberg, A., Sonechkin, D. M., Holmgren, K., Datsenko, N. M., and Karlén, W. (2005). Highly variable Northern Hemisphere temperatures reconstructed from low-and high-resolution proxy data. Nature 433, 613–617. doi: 10.1038/nature03265
Moir, A. K., Leroy, S. A. G., Brown, D., and Collins, P. E. F. (2010). Dendrochronological evidence for a lower water-table on peatland around 3200–3000 BC from subfossil pine in northern Scotland. Holocene 20, 931–942. doi: 10.1177/0959683610365935
Nevalainen, L., Helama, S., and Luoto, T. P. (2013). Hydroclimatic variations over the last millennium in eastern Finland disentangled by fossil Cladocera. Palaeogeogr. Palaeoecol. 378, 13–21. doi: 10.1016/j.palaeo.2013.03.016
Nevalainen, L., and Luoto, T. P. (2012). Intralake training set of fossil Cladocera for paleohydrological inferences: evidence for multicentennial drought during the Medieval Climate Anomaly. Ecohydrology 5, 834–840. doi: 10.1002/eco.275
Nevalainen, L., Sarmaja-Korjonen, K., and Luoto, T. P. (2011). Sedimentary Cladocera as indicators of past water-level changes in shallow northern lakes. Quat. Res. 75, 430–437.
Nielsen, A. B., and Odgaard, B. V. (2010). Quantitative landscape dynamics in Denmark through the last three millennia based on the Landscape Reconstruction Algorithm approach. Veg. Hist. Archaeobot. 19, 375–387. doi: 10.1007/s00334-010-0249-z
Nielsen, J. K., and Helama, S. (2012). Christian Theodor Vaupell, a Danish 19th century naturalist and a pioneering developer of the Quaternary geoscience. Hist. Geo- Space Sci. 3, 143–150. doi: 10.5194/hgss-1-1-2012
Niklasson, M., Lindbladh, M., and Björkman, L. (2002). A long-term record of Quercus decline, logging and fire history in a southern Swedish Fagus-Picea forest. J. Veg. Sci. 13, 765–774. doi: 10.1658/1100-9233(2002)013[0765:alroqd]2.0.co;2
Overballe-Petersen, M. V., and Bradshaw, R. H. W. (2011). The selection of small forest hollows for pollen analysis in boreal and temperate forest regions. Palynology 35, 146–153. doi: 10.1080/01916122.2011.558173
Parsons, R. W., and Prentice, I. C. (1981). Statistical approaches to R-values and pollen-vegetation relationship. Rev. Palaeobot. Palynol. 32, 127–152. doi: 10.1016/0034-6667(81)90001-4
Patterson, W. A. III, and Backman, A. E. (1988). Fire and disease history of forests. Vegetation History. Netherlands: Springer, 603–632.
Pearson, G. (1986). Precise calendrical dating of known growth-period samples using a ‘curve fitting’ technique. Radiocarbon 28, 292–299. doi: 10.1017/s0033822200007396
Pilcher, J. R., Baillie, M. G., Schmidt, B., and Becker, B. (1984). A 7,272-year tree-ring chronology for western Europe. Nature 312, 150–152. doi: 10.1038/312150a0
Pilcher, J. R., Baillie, M. G. L., Brown, D. M., McCormac, F. G., MacSweeney, P. B., and McLawrence, A. S. (1995). Dendrochronology of subfossil pine in the north of Ireland. J. Ecol. 1995, 665–671. doi: 10.2307/2261634
Pilcher, J. R., Hillam, J., Baillie, M. G. L., and Pearson, G. W. (1977). A long sub-fossil oak tree-ring chronology from the north of Ireland. New Phytol. 1977, 713–729. doi: 10.1111/j.1469-8137.1977.tb02255.x
Pitkänen, A., Lehtonen, H., and Huttunen, P. (1999). Comparison of sedimentary microscopic charcoal particle records in a small lake with dendrochronological data: evidence for the local origin of microscopic charcoal produced by forest fires of low intensity in eastern Finland. Holocene 9, 559–567. doi: 10.1191/095968399670319510
Prentice, I. C. (1985). Pollen Representation, Source Area, and Basin Size: Toward a Unified Theory of Pollen Analysis. Quat. Res. 23, 76–86. doi: 10.1016/0033-5894(85)90073-0
Prentice, I. C., and Parsons, R. W. (1983). Maximum Likelihood Linear Calibration of Pollen Spectra in Terms of Forest Composition. Biometrics 39, 1051–1057. doi: 10.2307/2531338
Pukienë, R. (1997). Pinewood growth dynamics in Užpelkiø Tyrelis oligotrophic bog during the Subatlantic period, Summary of doctoral diss. doctoral dissertation. Vilnius: Institute of Botany and Vilnius University.
Reimer, P. J., Austin, W. E., Bard, E., Bayliss, A., Blackwell, P. G., Ramsey, C. B., et al. (2020). The IntCal20 Northern Hemisphere radiocarbon age calibration curve (0–55 cal kBP). Radiocarbon 62, 725–757. doi: 10.1017/rdc.2020.41
Rundgren, M., and Beerling, D. (1999). A Holocene CO2 record from the stomatal index of subfossil Salix herbacea L. leaves from northern Sweden. Holocene 9, 509–513. doi: 10.1191/095968399677717287
Šamonil, P., Moravcová, A., Pokornı, P., Žáčková, P., Kašpar, J., Vašíčková, I., et al. (2018). The disturbance regime of an Early Holocene swamp forest in the Czech Republic, as revealed by dendroecological, pollen and macrofossil data. Palaeogeogr. Palaeoecol. 507, 81–96. doi: 10.1016/j.palaeo.2018.07.001
Sass-Klaassen, U., and Hanraets, E. (2006). Woodlands of the past — the excavation of wetland woods at Zwolle-Stadshagen (the Netherlands): growth pattern and population dynamics of oak and ash. Neth. J. Geosci. 85, 61–71. doi: 10.1017/s0016774600021429
Sassoon, D., Fletcher, W. J., Hotchkiss, A., Owen, F., and Feng, L. (2021). Scots pine (Pinus sylvestris) dynamics in the Welsh Marches during the mid to late-Holocene. Holocene 2021:0959683621994654.
Schweingruber, F. H. (2012). Tree rings: basics and applications of dendrochronology. New York, NY: Springer Science & Business Media.
Seppä, H., and Bennett, K. D. (2003). Quaternary pollen analysis: recent progress in palaeoecology and palaeoclimatology. Progr. Phys. Geogr. 27, 548–579. doi: 10.1191/0309133303pp394oa
Seppä, H., and Birks, H. J. B. (2001). July mean temperature and annual precipitation trends during the Holocene in the Fennoscandian tree-line area: pollen-based reconstructions. Holocene 11, 527–539. doi: 10.1191/095968301680223486
Seppä, H., Birks, H. J. B., Odland, A., Poska, A., and Veski, S. (2004). A modern pollen-climate calibration set from northern Europe: developing and testing a tool for palaeoclimatological reconstructions. J. Biogeogr. 31, 251–267. doi: 10.1111/j.1365-2699.2004.00923.x
Seppä, H., Bjune, A. E., Telford, R. J., Birks, H. J. B., and Veski, S. (2009). Last nine-thousand years of temperature variability in Northern Europe. Clim. Past. 5, 523–535. doi: 10.5194/cp-5-523-2009
Sernander, R. (1890). Omförekomsten af subfossila stubbar på svenska insjöars botten. Bot. Notiser 1890, 10–20.
Sernander, R. (1908). On the evidence of post-glacial changes of climate furnished by the peat mosses of northern Europe. Geologiska Föreningens i Stockholm Förhandlingar 30, 365–378.
Sillasoo, U., Mauquoy, D., Blundell, A., Charman, D., Blaauw, M., Daniell, J. R. G., et al. (2007). Peat multi-proxy data from Männikjärve bog as indicators of late Holocene climate changes in Estonia. Boreas 36, 20–37.
Sohar, K., and Kalm, V. (2008). A 12.8-ka-long palaeoenvironmental record revealed by subfossil ostracod data from lacustrine freshwater tufa in Lake Sinijärv, northern Estonia. J. Paleolimnol. 40, 809–821. doi: 10.1007/s10933-008-9200-z
Sonesson, M. (1974). Late Quaternary forest development of the Torneträsk area, North Sweden. 2. Pollen analytical evidence. Oikos 1974, 288–306. doi: 10.2307/3543947
Stančikaitë, M., Gedminienë, L., Edvardsson, J., Stoffel, M., Corona, C., Gryguc, G., et al. (2019). Holocene vegetation and hydroclimatic dynamics in SE Lithuania–Implications from a multi-proxy study of the čepkeliai bog. Quatern. Int. 501, 219–239. doi: 10.1016/j.quaint.2017.08.039
Steenstrup, J. J. S. (1842). Geognostisk-geologisk Undersøgelse af Skovmoserne Vidnesdam og Lillemose i det nordlige Sjælland, ledsaget af sammenlignende Bemærkninger hentede fra Danmarks Skov-, Kjær og Lyngmoser i Almindelighed. Kongelige Danske Videnskabernes Selskabs Afhandlinger 9, 17–120.
Stivrins, N., Aakala, T., Ilvonen, L., Pasanen, L., Kuuluvainen, T., Vasander, H., et al. (2019). Integrating fire-scar, charcoal and fungal spore data to study fire events in the boreal forest of northern Europe. Holocene 29, 1480–1490. doi: 10.1177/0959683619854524
Stoffel, M., and Bollschweiler, M. (2008). Tree-ring analysis in natural hazards research–an overview. Nat. Hazards Earth Syst. Sci. 8, 187–202. doi: 10.5194/nhess-8-187-2008
Stoffel, M., Khodri, M., Corona, C., Guillet, S., Poulain, V., Bekki, S., et al. (2015). Estimates of volcanic-induced cooling in the Northern Hemisphere over the past 1,500 years. Nat. Geosci. 8, 784–788. doi: 10.1038/ngeo2526
Sugita, S. (1994). Pollen representation of vegetation in Quaternary sediments: Theory and method in patchy vegetation. J. Ecol. 82, 881–897. doi: 10.2307/2261452
Sugita, S. (2007a). Theory of quantitative reconstruction of vegetation I: pollen from large sites REVEALS regional vegetation composition. Holocene 17, 229–241. doi: 10.1177/0959683607075837
Sugita, S. (2007b). Theory of Quantitative Reconstruction of Vegetation. II. All You Need Is LOVE. Holocene 17, 243–257. doi: 10.1177/0959683607075838
Swindles, G. T., Lawson, I. T., Matthews, I. P., Blaauw, M., Daley, T. J., Charman, D. J., et al. (2013). Centennial-scale climate change in Ireland during the Holocene. Earth Sci. Rev. 126, 300–320.
Tauber, H. (1965). Differential pollen dispersion and the interpretation of pollen diagrams. DGU. Copenhagen: Reitzels Forlag.
Telford, R. J., Heegaard, E., and Birks, H. J. B. (2004). All age-depth models are wrong: but how badly? Quat. Sci. Rev. 23, 1–5. doi: 10.1016/j.quascirev.2003.11.003
Theuerkauf, M., Dräger, N., Hupfer, M., Mrotzek, A., Prager, A., et al. (2019). Using Annual Resolution Pollen Analysis to Synchronize Varve and Tree-Ring Records. Quaternary 2:23. doi: 10.3390/quat2030023
von Arx, G., Crivellaro, A., Prendin, A. L., čufar, K., and Carrer, M. (2016). Quantitative wood anatomy—practical guidelines. Front. Plant. Sci. 7:781. doi: 10.3389/fpls.2016.00781
von Post, L. (1918). Skogsträdpollen i sydsvenska torvmosselagerföljder. Forhandlinger ved de 16, 433–465.
von Post, L. (1924). Ur de sydsrenska skogarnas regionala historia under post-arktisk tid. Geologiska Föreningen i Stockholm Förhandlingar 46, 83–128. doi: 10.1080/11035892409444880
von Post, L. (1930). Ñorrländska torvmossestudier. II: Några huvudpunkter i skogens och myrarnas postarktiska historia inom södra Norrland. Geologiska Föreningen i Stockholm Förhandlingar 52, 63–90. doi: 10.1080/11035893009454835
Wanner, H., Beer, J., Bütikofer, J., Crowley, T. J., Cubasch, U., Flückiger, J., et al. (2008). Mid-to Late Holocene climate change: an overview. Quat. Sci. Rev. 27, 1791–1828.
Whitlock, C., and Larsen, C. (2002). Charcoal as a fire proxy. In Tracking environmental change using lake sediments. Dordrecht: Springer, 75–97.
Keywords: the Holocene, dendrochronology, pollen analysis, stratigraphic data, landscape development, long-term climate change
Citation: Edvardsson J, Helama S, Rundgren M and Nielsen AB (2022) The Integrated Use of Dendrochronological Data and Paleoecological Records From Northwest European Peatlands and Lakes for Understanding Long-Term Ecological and Climatic Changes—A Review. Front. Ecol. Evol. 10:781882. doi: 10.3389/fevo.2022.781882
Received: 23 September 2021; Accepted: 31 January 2022;
Published: 21 February 2022.
Edited by:
Niina Kuosmanen, University of Helsinki, FinlandReviewed by:
Jennifer Clear, Liverpool Hope University, United KingdomZicheng Yu, Lehigh University, United States
Copyright © 2022 Edvardsson, Helama, Rundgren and Nielsen. This is an open-access article distributed under the terms of the Creative Commons Attribution License (CC BY). The use, distribution or reproduction in other forums is permitted, provided the original author(s) and the copyright owner(s) are credited and that the original publication in this journal is cited, in accordance with accepted academic practice. No use, distribution or reproduction is permitted which does not comply with these terms.
*Correspondence: Johannes Edvardsson, am9oYW5uZXMuZWR2YXJkc3NvbkBnZW9sLmx1LnNl