- 1Quaternary Division, Birbal Sahni Institute of Palaeosciences, Lucknow, India
- 2Academy of Scientific and Innovative Research (AcSIR), Ghaziabad, India
- 3Geodynamics Unit, Jawaharlal Nehru Centre for Advanced Scientific Research, Bengaluru, India
We reviewed the available climate records for the past 2 millennia based on the analyzed sediment and speleothem archives from different regions of South Asia. Speleothem records from the core-monsoon regions of the Indian sub-continent have revealed the Little Ice Age (LIA) as a climatically dry phase, whereas the same from the western and central Himalaya recorded LIA as wet. Moreover, the sediment-derived vegetation proxy records [pollen-spores and stable organic carbon isotope (δ13Corg)] from the western Himalaya also reported LIA as a dry phase. Heterogeneous results by different proxies during LIA enhanced our interest to understand the response of the proxies toward the primary precipitation sources, Indian summer monsoon (ISM) and winter westerly disturbances (WDs), over the Himalaya. We emphasize that in the Himalayan region, the vegetation predominantly responds to the ISM dynamics, whereas speleothem also captures the WD effect.
Introduction
The late Holocene is essential in understanding the hydroclimatic conditions with emphasis on the climate anomalies of the last 2 millennia. Medieval Warm Period (MWP) and Little Ice Age (LIA) were observed in the Northern Hemisphere primarily impacting the European landmass and parts of the North Atlantic (Crowley and Lowery, 2000). Globally, the MWP is documented between 900 and 1300 C.E. (Graham et al., 2011) and the LIA is between 1500 and 1850 C.E. (Grove, 2001). However, the time intervals of their respective peak warm and cold phases and the regional hydroclimatic variability remain globally debated (Bradley et al., 2003; Wanner et al., 2008; Mann et al., 2009). Chen et al. (2019) highlighted the anti-phased hydrological variations between the arid central Asia and mid-latitude monsoonal Asia on different timescales of the Holocene. Dixit and Tandon (2016) provided a synoptic view of the late Holocene hydroclimate intricacies based on the 57 records from different regions of the Indian subcontinent. They found poorly documented MWP and LIA signals, either due to age constraints or poor temporal resolution of the studied archives.
The Himalayan region, under the influence of Indian summer monsoon (ISM) and winter westerly disturbances (WD; Polanski et al., 2014; Dimri et al., 2016), remains highly dynamic hydroclimatically and ecologically. The region is unique for inferring the climate and monsoonal variability in time and space. Fluvio-lacustrine sediment deposits, tree-rings of climatically sensitive tree taxa, and cave deposits (speleothem) are the most extensively used archives for past climate reconstructions. A good number of climate reconstructions from the Himalaya have used sediment archives with the pollen-spores and organic carbon isotope (δ13Corg) as major proxies (Roy et al., 2022 and references therein). Only a few sedimentary records with climate reconstructions at centennial to decadal time resolution could capture the MWP and LIA anomalies (Phadtare, 2000; Kar et al., 2002; Chauhan, 2006; Dixit and Bera, 2013; Bali et al., 2015; Rawat et al., 2015; Srivastava et al., 2017; Ali et al., 2018; Shah et al., 2020; Sharma et al., 2020; Roy et al., 2022). The speleothem and tree-rings provided the high-resolution decadal to annual-scale climate records of the past few centuries, with speleothem records extending beyond the late Holocene. But such high-resolution long-term climate records are less from the Himalayan and other regions of the Indian subcontinent. This is due to the limited existence of old forest patches and less number of explored speleothem sites. However, the biotic and abiotic proxy-based hydroclimatic records from different regions of the Indian subcontinent lack the comparative proxy response analysis toward precipitation dynamics.
The regions of Himalaya and peninsular India experienced wet conditions due to strong ISM during MWP (Dixit and Tandon, 2016 and references in Supplementary Table 1). The sediment archives from different precipitation zones of Himalaya and south India inferred moist conditions between 1.8 and 0.5 ka (Supplementary Table 1 references). The oxygen isotope (δ18O) records of speleothem from caves of the western and central Himalaya (Sanwal et al., 2013; Kotlia et al., 2015, 2017; Liang et al., 2015; Sinha et al., 2015) and in central India (Sinha et al., 2011a) also exhibited decisive phase of summer monsoon between ∼1.15 and 0.65 ka. The increasing strength of the ISM during MWP was attributed to the northward shifting of the inter-tropical convergent zone (ITCZ; Haug et al., 2001) caused by the higher solar insolation (Fleitmann et al., 2003; Gadgil, 2003) coupled with the oceanic circulations (Berkelhammer et al., 2010; Liang et al., 2015).
Subsequent to the moist MWP phase, the sediment derived pollen-spores and δ13Corg records from the Himalaya reported a dry climate between ca. 0.8 and 0.2 ka bracketing the LIA phase (Supplementary Table 1 references). On the other hand, the speleothem studies from ISM dominant regions of the central and western Himalaya such as Dharamjali cave (Sanwal et al., 2013), Sainji cave (Kotlia et al., 2015), Panigarh cave (Liang et al., 2015), and Chulerasim cave (Kotlia et al., 2017) revealed wet conditions during the LIA. Presently these sites receive around 70% of precipitation during the summer monsoon and have an influence of the winter precipitation through westerly disturbances. Hence, there is an observed contrast between the climatic signals produced by the plant archives (pollen-spores and δ13Corg) and the cave deposits (speleothem). Most of the tree-ring records do not extend back to the MWP time interval but a few older tree-ring chronologies from the Asian region recorded frequent drought conditions during the last millennium (Cook et al., 2010). These records highlight the proxy response heterogeneity toward the Asian summer and winter monsoon precipitation systems. The earlier records suggested a considerable variability in the latitudinal monsoon precipitation during the LIA due to the rapid southward migration of the ITCZ (Newton et al., 2006; Kotlia et al., 2012; Sanwal et al., 2013). We carried out a comparative study of the past 2 millennia of climate records to understand the response behavior of proxies toward the monsoon systems so as to assess the role of WD and ISM in the Himalayan region. For this, we reviewed the speleothem and sediment (pollen and δ13Corg) studies from different regions of Himalaya (Figures 1A,B and Supplementary Table 1) and discussed the possible mechanisms behind the observed proxy response heterogeneity.
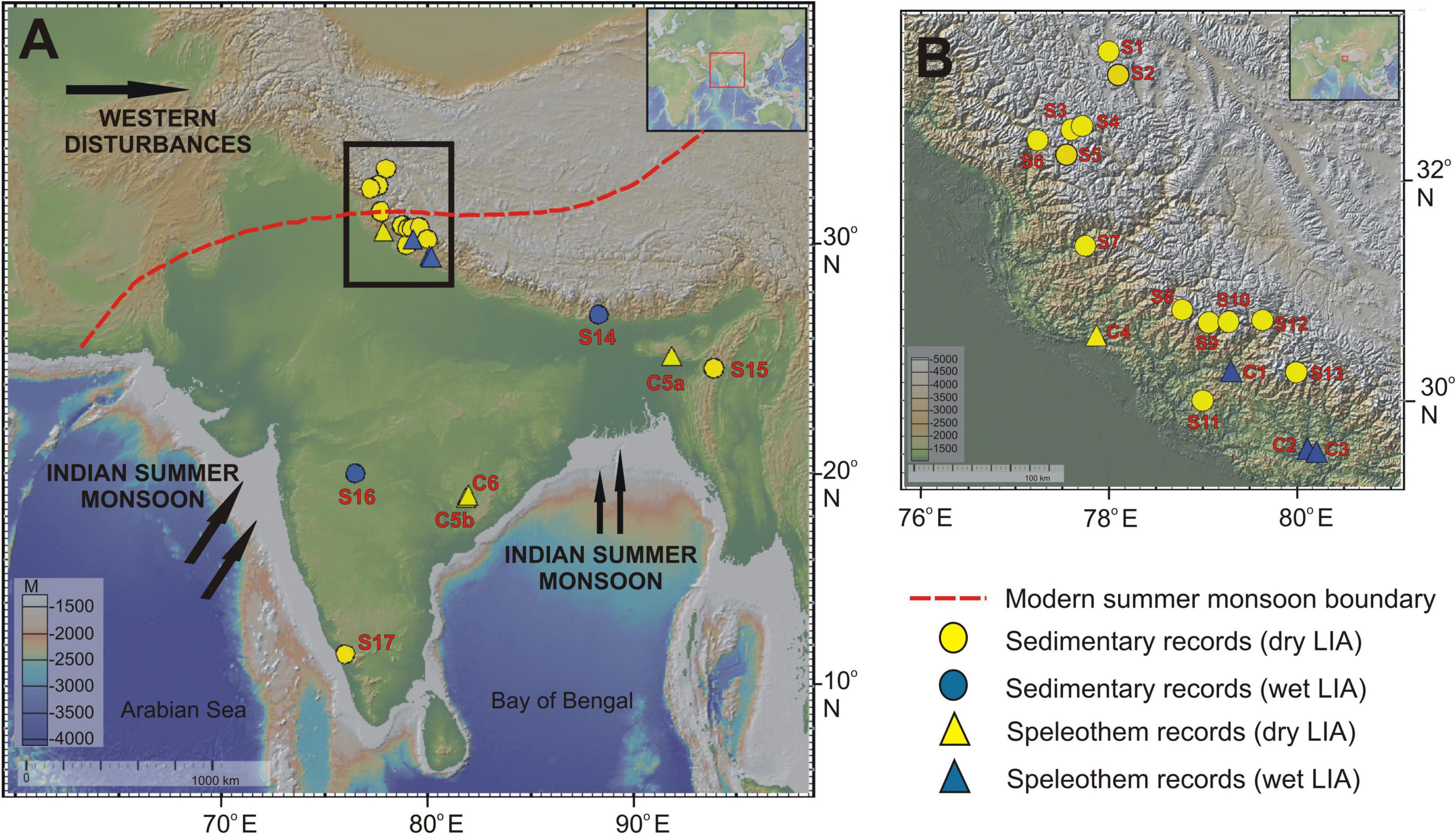
Figure 1. (A,B) Locations of archives as mentioned in Figure 2. Detailed information about the sedimentary and cave archives can be found in the Supplementary Table 1. Maps are modified after Chen et al. (2019); they have been plotted by using GeoMapApp v.3.6.10.
Proxy Response to Hydroclimatic Variabilities During the Last 2 Millennia
We compared the late Holocene climate records of sediment derived pollen and δ13Corg proxies from different monsoonal zones of Himalaya (Figure 2). The limited number of studies available from the WD dominated Trans-Himalaya, such as Tso Kar Lake (Demske et al., 2009) and Tso Moriri Lake (Leipe et al., 2014) showed the commencement of moist conditions, respectively, since ca. 1.3 and 1.1 ka due to the strengthening of the southwest monsoon. Moist conditions existed till ca. 0.5 ka followed by dry conditions since ca. 0.4 ka leading to decline in agro-pastoral activities (Leipe et al., 2014). The pollen and δ13Corg of peat deposits from WD dominant Lahaul-Spiti region also revealed moist conditions ca. 1.16–0.65 ka and cool-dry condition ca. 0.65–0.35 ka (Rawat et al., 2015). However, from the Lahaul-Spiti region, Mazari et al. (1996) and Chauhan et al. (2000) recorded warm-moist conditions ca. 1.5–0.9 ka. The regions of western and central Himalaya under the high ISM precipitation domain with the additional influence of winter precipitation through WD such as Rohtang (Bhattacharyya, 1988), Kinnaur (Chakraborty et al., 2006), Dokriani valley (Phadtare, 2000), Gangotri valley (Kar et al., 2002; Roy et al., 2022), Nachiketa (Roy et al., 2022), and Pindar valley (Bali et al., 2015), recorded the increase in moisture since ca. 1.8 ka. Some studies within the ISM-WD region reported moisture increase even later, such as since ca. 1.2 ka from Kedarnath, Uttarakhand (Srivastava et al., 2017), ca. 1.3 ka from Parvati Valley, (Chauhan, 2006), and ca. 1.4 ka from Dewar Taal, Uttarakhand (Chauhan and Sharma, 2000). Hence in the western-central Himalaya, we could observe a variability in the commencement of the moist phase prior to the last millennium. The WD dominant regions were distinctly moist since ca. 1.3 ka, whereas the ISM-WD influenced regions remained variable where most studies showed moist trends since ca. 1.8 ka and few reported the same since ca. 1.4 ka or after (Figure 2 and Supplementary Table 1). Moreover, available studies from the ISM dominated eastern Himalaya also showed a maximum strengthening of ISM rainfall ca. 1.3 ka (Nautiyal and Chauhan, 2009; Agrawal et al., 2015; Ali et al., 2018; Ghosh et al., 2018).
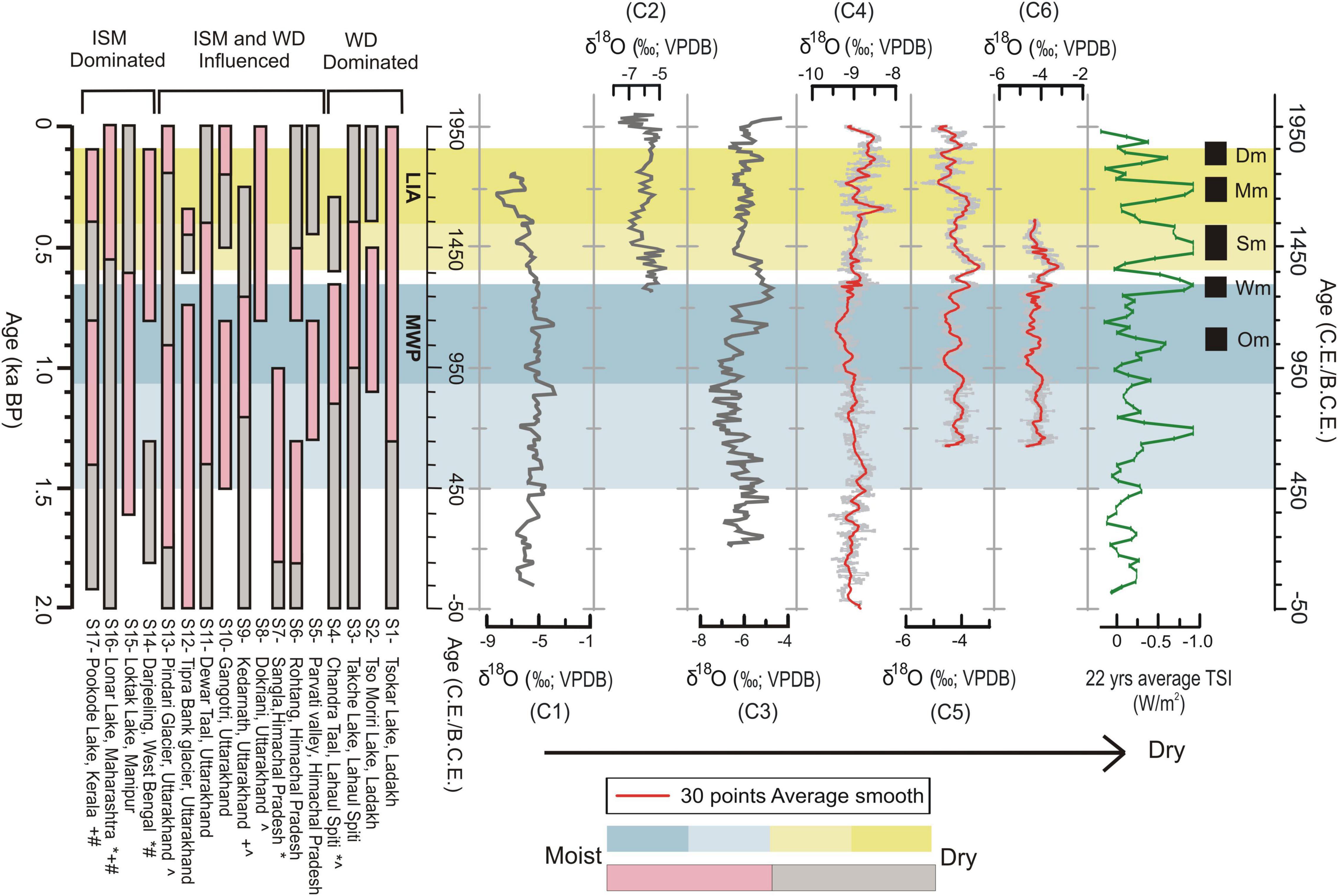
Figure 2. Climatic episodes in sedimentary records of (S1) Demske et al. (2009); (S2) Leipe et al. (2014); (S3) Mazari et al. (1996); (S4) Rawat et al. (2015); (S5) Chauhan (2006); (S6) Bhattacharyya (1988); (S7) Chakraborty et al. (2006); (S8) Phadtare (2000); (S9) Srivastava et al. (2017); (S10) Kar et al. (2002); (S11) Chauhan and Sharma (2000); (S12) Bhattacharyya and Chauhan (1997); (S13) Bali et al. (2015); (S14) Ghosh et al. (2018); (S15) Nautiyal and Chauhan (2009); (S16) Prasad et al. (2014); (S17) Veena et al. (2014). All inferences are derived based on pollen assemblages additionally with δ13Corg (*), Environment magnetism (∧), Other geochemical proxies (+), and other biotic proxies (#) as indicated in the figure. Palaeoclimate data of cave deposits; (C1) δ18O Sainji Cave (Kotlia et al., 2015); (C2) δ18O Panigarh Cave (Liang et al., 2015); (C3) δ18O Dharamjali Cave (Sanwal et al., 2013); (C4) δ18O Sahiya Cave (Sinha et al., 2015); (C5) δ18O Wah Shikar Cave (Sinha et al., 2011b); (C6) δ18O Dandak Cave (Berkelhammer et al., 2010). The 22 years average Total Solar Irradiance (TSI) dataset from Steinhilber et al. (2012) and sunspot minima activities: Oort (Om), Wolf (Wm), Spörer (Sm), Maunder (Mm), and Dalton (Dm) compared with the datasets. C1–C4 are influenced both by ISM and WD whereas C5–C6 are from ISM dominant regions.
Contemporary to this phase the speleothem records from the Himalayan caves (Sinha et al., 2007, 2015; Sanwal et al., 2013; Kotlia et al., 2015) also retrieved strong ISM precipitation from ca. 950 to 1,250 C.E. (ca. 1.0–0.7 ka). The moist phase corresponding to MWP existed till ca. 0.8 ka as recorded by the majority of the sediment and cave records from Himalaya. This phase is also comparable to the terrestrial and marine records from core monsoon regions of peninsular India. Pollen and phytolith assemblage dataset from the Pookode Lake, Kerala (Veena et al., 2014) inferred dry climate ca. 2–0.4 ka interrupted by moist conditions between ca. 1.4 and 0.8 ka. Marine records from the Arabian Sea and Bay of Bengal (Gupta et al., 2003; Tiwari et al., 2006; Chauhan et al., 2010; Ponton et al., 2012) also recorded strong ISM precipitation ca. 950–1,250 C.E. However, a study from Lonar Lake located in the Indian core monsoon region (Prasad et al., 2014; Mishra et al., 2018) discussed the weak influence of ISM between ca. 810 and 1,300 C.E.
Subsequent post-MWP weakening of ISM as evident in the Himalaya and peninsular India is responsible for the high-intensity monsoon mega-drought (MMD) events since ca. 1300 C.E. (ca. 0.65 ka). The sediment records of the Himalayan region showed that the region experienced the weakest ISM between ca. 0.6 and 0.3 ka (Figure 2 and Supplementary Table 1), corresponding to the LIA. A network of tree-ring width reconstructions (Cook et al., 2010) also pointed to a general weakening of the Asian monsoon during the last millennium, responsible for mega-droughts in the Asian region. The annual precipitation reconstruction since ca. 1330 C.E. based on the tree-ring data of Himalayan cedar (Cedrus deodara) from the Lahaul-Spiti region, western Himalaya, showed drought conditions during the 14th and 15th century C.E. (Yadav et al., 2011). High-intensity MMD events ca. 1300 C.E. (ca. 0.65 ka) were observed as well in the records from the Arabian Sea (Gupta et al., 2003; Tiwari et al., 2006) and Andaman (Laskar et al., 2013). The speleothem records of Jhumar and Dandak caves located in the Indian peninsular region (C5b, C6 in Figure 1) and Wah Shikar Cave in north-eastern India (C5 in Figure 2) also observed the MMD events between ca. 1250 and 1450 C.E. Subsequently, 1400–1700 C.E. was persistently drier with monsoon breaks followed by the moist conditions with an active summer monsoon in peninsular India (Sinha et al., 2011b). Contrary to this, the speleothem records of Sainji cave (Kotlia et al., 2015), Panigarh cave (Liang et al., 2015), and Dharamjali cave (Sanwal et al., 2013) from the central Himalaya (C1, C2, and C3 in Figure 2) experienced wetter conditions around 1450–1750 C.E. (Figure 2). This anti-correlation between the speleothem records of peninsular India and the Himalayan region during the LIA has been attributed to the additional role of WD precipitation in the Himalayan region (Sanwal et al., 2013; Liang et al., 2015; Kumar et al., 2019). Variability in the hydroclimate records by the sediment proxies and cave deposits is thus evident from the Himalayan region for the LIA time period (ca. 1300–1800 C.E.). Here the speleothem records of caves located in the ISM and WD influenced region showed wet conditions and the sediment-based pollen and δ13Corg proxy data recorded the dry climatic conditions (Figure 2).
Possible Mechanisms Behind Hydroclimatic Variabilities and Proxy Responses
Precipitation over the Indian sub-continent received from different moisture sources (Polanski et al., 2014) shows great diversity due to the seasonal shifting of the ITCZ. The climate of peninsular India is predominantly influenced by the ISM. The northward shifting of the ITCZ controls the ISM due to low pressure over the Indian landmass after drawing moisture from the Bay of Bengal (BoB) and the Arabian Sea (AS). Earlier studies have found the role of the North Atlantic sea surface temperatures (Berkelhammer et al., 2010) and the solar irradiance in governing the Indian monsoon system by controlling the north-south migration of ITCZ (Agnihotri et al., 2002; Fleitmann et al., 2003; Kathayat et al., 2016). The strength of the ISM is determined by the variations in solar irradiance that controls the frequency of the El Niño and La Niña events over time (Terray and Dominiak, 2005). The eastern Himalaya is strongly influenced by the BoB branch (Mooley and Parthasarathy, 1982). The western and central Himalaya receives summer precipitation from the AS and BoB branches from June to September and from extra-tropical WD from December to February (Sinha et al., 2015; Dimri et al., 2016). Moreover, total annual precipitation over the Himalaya shows an inverse correlation with precipitation over the core monsoon areas of the Indian subcontinent (Kripalani et al., 2003). This inverse relationship between the winter/spring and the summer monsoon precipitations is clearly visible over the peninsular India and the Himalayan region by the speleothem δ18O records for the LIA phase (Dimri et al., 2016; Dixit and Tandon, 2016; Kumar et al., 2019). Drought conditions in the core monsoon area of the south Asian region were the result of more frequent El Niño events during the LIA (Sinha et al., 2011a; Shi et al., 2017). But the same triggered more “monsoon breaks” in the Himalayan foothills thus bringing in the higher winter precipitation as recorded by the cave deposits in the Himalaya (Kotlia et al., 2012, 2015, 2017; Sanwal et al., 2013). The high El Niño conditions during the LIA (Henke et al., 2015) also reduced the flow of warm ocean water to higher Northern latitudes causing cooling of the North Atlantic Ocean and the Eurasian landmass. This resulted in high snow-cover over Eurasia and the Himalaya due to the strengthened WD. Enhanced winter-time precipitation over northwest India is observed within a phase of the warm equatorial sea-surface temperature and vice-versa (Dimri, 2013; Yadav et al., 2013). Managave et al. (2020) also reconstructed cool-wet conditions ca. 1300–1560 C.E. and ca. 1650–1800 C.E. based on tree-ring δ18O from Lahaul-Spiti region of Himalaya, comparable to a demonstrated expansion of Himalayan glaciers between ca. 1300 and 1600 C.E. by Rowan (2017).
The pollen and δ13Corg data recorded dry LIA in the Himalayan region when the ISM (WD) was weak (strong). Vegetation primarily gets affected by hydroclimatic changes, which is evident in the present vegetation distribution across the Himalayan arc (Champion and Seth, 1968; Rawat, 2017). Moisture availability during the growth season of vegetation (spring to pre-winter months) is vital for the annual phenological activities of plants (Pangtey et al., 1990; Rawal et al., 1991). The interannual climate variations could affect the phenological activities of the plants as they depend on climatic factors such as air and soil temperature, precipitation, solar radiation, snow cover, etc. (Walker et al., 1995; Bijalwan et al., 2013). The strong and weak phases of WD and ISM over the mountain regions could alter the growth period of vegetation by affecting the phenological cycle of seasonal ground vegetation. The enhanced WD during the LIA brought in more winter precipitation to the Himalayan region in the form of snow resulting in relatively cool conditions with an increased number of snow stand days. This might have created prolonged freezing soil conditions, thus shortening the growth cycle of warm and moist ground vegetation. The weak ISM precipitation resulted in low soil-moisture during summers that remained suitable to support the growth of dry steppe taxa. On the other hand, the wetter conditions corresponding to LIA phase indicated by speleothem records of caves (C1, C2, C3 in Figure 2) located in the ISM-WD influenced zone could be the result of “amount effect” as the δ18O of rainfall is also influenced by the rainfall amount (Kotlia et al., 2017). The LIA time-period ca. 0.5–0.25 ka with higher precipitation due to strong WD maintained comparatively lower δ18O values (Sanwal et al., 2013; Kotlia et al., 2015) due to higher humidity with minimum evaporation under reduced kinetic fractionation (Kotlia et al., 2015). Sinha et al. (2015) also highlighted the role of humidity, evaporation and soil moisture saturation conditions influencing the 18O fractionation as a classic amount-effect. Also, wet signals in the speleothem records (C4 in Figure 2) during MWP were the effect of strong monsoon circulation with an enhanced flux of isotopically depleted moisture from the BoB branch and a reduced flux of isotopically enriched moisture from AS branch (Sinha et al., 2015). In the Himalayan region, vegetation thus responds to the weak (strong) ISM by the expansion of dry (moist) taxa. Vegetation, therefore, highlights the ISM dynamics, whereas speleothem could provide the signatures of winter precipitation dynamics as well.
Pollen assemblage could also refer to land-use activities. Land use could further be influenced by climate change as has been the case for the agricultural pattern in some regions (Li et al., 2008; Demske et al., 2009; Yang et al., 2012). The Himalaya remains inhabited since the Neolithic time period with 80% of agriculture being practiced in terraced fields (Mittal et al., 2008; Demske et al., 2016). Primary crops cultivated include Cerealia and species of Amaranthus, Chenopodium, Moraceae, Rumex, Solanum, Viburnum, Fagopyrum, Polygonatum, Rhododendron, etc. (Tiwari et al., 2010; Joshi et al., 2018). Amaranthaceae, a ruderal community (Behre, 1981) is intermediate between cultivated and grazed areas; both indicate human activities (Court-Picon et al., 2005). Some studies (Li et al., 2014; Mishra et al., 2018) showed that at times the pollen inferred climate dataset could be an artifact of the possible human interferences and not completely reflect the climate-induced vegetation dynamics. It is difficult to dissociate the respective parts of climate and land-use on a vegetation dataset based on pollen, as vegetation, land-use and climate are greatly interconnected in the region over the last centuries to millennia. The present review discusses the climatic aspects while further works should be done to explore the land-use as a proxy for change. Comparison between the regional pollen assemblages, other environmental proxies and regional land-use/archeological data could help to differentiate the climate and human signals on vegetation.
Conclusion
A comparison between the responses of sediment based biotic proxies (Pollen and δ13Corg) and speleothem (δ18O) records toward the Indian monsoon system showed heterogeneity among proxies even within the Himalayan region. The pollen and δ13Corg records derived from sedimentary archives inferred dry climate during the LIA attributed to weak ISM precipitation. Whereas speleothem records showed wet climatic conditions due to the enhanced winter precipitation resulting from the strong WD. Thus, vegetation could be taken as an indicator of ISM variations while speleothem records the WD variability as well. Moreover, the comparison of the sediment records also represented temporal incongruence for the MWP among the sites within the Himalayan region. This could be the response time to capture the signals of changes in climate variability at different precipitation regimes. However, errors in the interpolated ages due to less number of absolute dates or a small sample size in most of the available sediment-based proxy studies could also be the factors for diluting the finer scale climate signals and hence decadal to centennial-scale incongruence amongst the proxy records.
Assessment of the heterogeneous behavior of various proxies toward the different monsoonal systems on the spatial and temporal scales is important to significantly facilitate understanding of the monsoonal complexities over the South Asian region. This requires more high-resolution decadal-scale climate datasets generated from biotic and abiotic proxies of sediments and other archives from different monsoonal regimes of the South Asian region. The influence of land use on vegetation patterns should also be explored and quantified.
Author Contributions
IR and PSR conceptualized the theme and objectives of the manuscript. NT aided in technical editing of the manuscript. JS provided inputs in the discussion part on speleothem studies. All authors contributed to the article and approved the submitted version.
Funding
We are thankful to BSIP, Lucknow for the in-house project 5.8 funding. IR is thankful to DST-INSPIRE fellowship (IF150998) from Department of Science and Technology, New Delhi, India.
Conflict of Interest
The authors declare that the research was conducted in the absence of any commercial or financial relationships that could be construed as a potential conflict of interest.
Publisher’s Note
All claims expressed in this article are solely those of the authors and do not necessarily represent those of their affiliated organizations, or those of the publisher, the editors and the reviewers. Any product that may be evaluated in this article, or claim that may be made by its manufacturer, is not guaranteed or endorsed by the publisher.
Acknowledgments
We thank the Director, Birbal Sahni Institute of Palaeosciences (BSIP), Lucknow, India for permission to carry out this work and for publication (BSIP/RDCC/Publication No. 69/2020-21).
Supplementary Material
The Supplementary Material for this article can be found online at: https://www.frontiersin.org/articles/10.3389/fevo.2022.778825/full#supplementary-material
References
Agnihotri, R., Dutta, K., Bhushan, R., and Somayajulu, B. L. K. (2002). Evidence for solar forcing on the Indian monsoon during the last millennium. Earth Planet. Sci. Lett. 198, 521–527. doi: 10.1016/S0012-821X(02)00530-7
Agrawal, S., Srivastava, P., Meena, N. K., Rai, S. K., Bhushan, R., Misra, D. K., et al. (2015). Stable (δ13C and δ15N) isotopes and magnetic susceptibility record of late Holocene climate change from a lake profile of the northeast Himalaya. J. Geol. Soc. India 86, 696–705.
Ali, S. N., Dubey, J., Ghosh, R., Quamar, M. F., Sharma, A., Morthekai, P., et al. (2018). High frequency abrupt shifts in the Indian summer monsoon since Younger Dryas in the Himalaya. Sci. Rep. 8:9287. doi: 10.1038/s41598-018-27597-6
Bali, R., Ali, S. N., Bera, S. K., Patil, S. K., Agarwal, K. K., and Nautiyal, C. M. (2015). “Impact of Anthropocene vis-a-vis Holocene climatic changes on central Indian Himalayan glaciers,” in Engineering Geology for Society and Territory-Volume 1, eds G. Lollino, A. Manconi, J. Clague, W. Shan, and M. Chiarle (Cham: Springer), 467–471. doi: 10.1034/j.1600-0889.2000.d01-7.x
Behre, K. E. (1981). The interpretation of anthropogenic indicators in pollen diagrams. Pollen et Spores 23, 225–245.
Berkelhammer, M., Sinha, A., Mudelsee, M., Cheng, H., Edwards, R. L., and Cannariato, K. (2010). Persistent multidecadal power of the Indian Summer Monsoon. Earth Planet. Sci. Lett. 290, 166–172. doi: 10.1016/j.epsl.2009.12.017
Bhattacharyya, A. (1988). Vegetation and climate during post glacial period in the vicinity of Rohtang Pass, Great Himalayan Range. Pollen et Spores 30, 417–427.
Bhattacharyya, A., and Chauhan, M. S. (1997). Vegetational and climatic changes during recent past around Tipra bank glacier, Garhwal Himalaya. Curr. Sci. 72, 408–412.
Bijalwan, R., Vats, M., and Joshi, S. P. (2013). Plant phenological response to microclimatic variations in an alpine zone of Garhwal Himalaya. J. Appl. Nat. Sci. 5, 47–52. doi: 10.31018/jans.v5i1.280
Bradley, R. S., Hughes, M. K., and Diaz, H. F. (2003). Climate in medieval time. Science 302, 404–405. doi: 10.1126/science.1090372
Chakraborty, S., Bhattacharya, S. K., Ranhotra, P. S., Bhattacharyya, A., and Bhushan, R. (2006). Palaeoclimatic scenario during Holocene around Sangla valley, Kinnaur northwest Himalaya based on multi proxy records. Curr. Sci. 91, 777–782.
Champion, H. G., and Seth, S. K. (1968). A Revised Survey Of The Forest Types Of India. Delhi: Manager of publications.
Chauhan, M. S. (2006). Late Holocene vegetation and climate change in the alpine belt of Himachal Pradesh. Curr. Sci. 91, 1562–1567.
Chauhan, M. S., Mazari, R. K., and Rajagopalan, G. (2000). Vegetation and climate in upper Spiti region, Himachal Pradesh during late Holocene. Curr. Sci. 79, 373–377.
Chauhan, M. S., and Sharma, C. (2000). Late holocene vegetation and climate in Dewar Tal area, inner lesser Garhwal Himalaya. Palaeobotanist 49, 509–514.
Chauhan, O. S., Vogelsang, E., Basavaiah, N., and Kader, U. S. A. (2010). Reconstruction of the variability of the southwest monsoon during the past 3 ka, from the continental margin of the southeastern Arabian Sea. J. Quat. Sci. 25, 798–807. doi: 10.1002/jqs.1359
Chen, F., Chen, J., Huang, W., Chen, S., Huang, X., Jin, L., et al. (2019). Westerlies Asia and monsoonal Asia: spatiotemporal differences in climate change and possible mechanisms on decadal to sub-orbital timescales. Earth-Sci. Rev. 192, 337–354. doi: 10.1016/j.earscirev.2019.03.005
Cook, E. R., Anchukaitis, K. J., Buckley, B. M., D’Arrigo, R. D., Jacoby, G. C., and Wright, W. E. (2010). Asian monsoon failure and megadrought during the last millennium. Science 328, 486–489. doi: 10.1126/science.1185188
Court-Picon, M., Buttler, A., and de Beaulieu, J. L. (2005). Modern pollen–vegetation relationships in the Champsaur valley (French Alps) and their potential in the interpretation of fossil pollen records of past cultural landscapes. Rev. Palaeobot. Palynol. 135, 13–39. doi: 10.1016/j.revpalbo.2005.02.003
Crowley, T. J., and Lowery, T. S. (2000). How warm was the medieval warm period? AMBIO J. Hum. Environ. 29, 51–54. doi: 10.1579/0044-7447-29.1.51
Demske, D., Tarasov, P. E., Leipe, C., Kotlia, B. S., Joshi, L. M., and Long, T. (2016). Record of vegetation, climate change, human impact and retting of hemp in Garhwal Himalaya (India) during the past 4600 years. Holocene 26, 1661–1675.
Demske, D., Tarasov, P. E., Wünnemann, B., and Riedel, F. (2009). Late glacial and Holocene vegetation, Indian monsoon and westerly circulation in the Trans-Himalaya recorded in the lacustrine pollen sequence from Tso Kar, Ladakh, NW India. Palaeogeogr. Palaeoclimatol. Palaeoecol. 279, 172–185. doi: 10.1016/j.palaeo.2009.05.008
Dimri, A. P. (2013). Relationship between ENSO phases with Northwest India winter precipitation. Int. J. Climatol. 33, 1917–1923.
Dimri, A. P., Yasunari, T., Kotlia, B. S., Mohanty, U. C., and Sikka, D. R. (2016). Indian winter monsoon: present and past. Earth-Sci. Rev. 163, 297–322. doi: 10.1016/j.earscirev.2016.10.008
Dixit, S., and Bera, S. K. (2013). Pollen-inferred vegetation vis-à-vis climate dynamics since Late Quaternary from Western Assam, Northeast India: signal of global climatic events. Quat. Int. 286, 56–68. doi: 10.1016/j.quaint.2012.06.010
Dixit, Y., and Tandon, S. K. (2016). Hydroclimatic variability on the Indian subcontinent in the past millennium: review and assessment. Earth-Sci. Rev. 161, 1–15. doi: 10.1016/j.earscirev.2016.08.001
Fleitmann, D., Burns, S. J., Mudelsee, M., Neff, U., Kramers, J., Mangini, A., et al. (2003). Holocene forcing of the Indian monsoon recorded in a stalagmite from southern Oman. Science 300, 1737–1739. doi: 10.1126/science.1083130
Gadgil, S. (2003). The Indian monsoon and its variability. Annu. Rev. Earth Planet. Sci. 31, 429–467.
Ghosh, R., Biswas, O., Paruya, D. K., Agrawal, S., Sharma, A., Nautiyal, C. M., et al. (2018). Hydroclimatic variability and corresponding vegetation response in the Darjeeling Himalaya, India over the past ~ 2400 years. Catena 170, 84–99. doi: 10.1016/j.catena.2018.05.043
Graham, N. E., Ammann, C. M., Fleitmann, D., Cobb, K. M., and Luterbacher, J. (2011). Support for global climate reorganization during the “Medieval Climate Anomaly”. Clim. Dyn. 37, 1217–1245. doi: 10.1007/s00382-010-0914-z
Grove, J. M. (2001). The initiation of the” Little Ice Age” in regions round the North Atlantic. Clim. Chang. 48, 53–82. doi: 10.1023/A:1005662822136
Gupta, A. K., Anderson, D. M., and Overpeck, J. T. (2003). Abrupt changes in the Asian southwest monsoon during the Holocene and their links to the North Atlantic Ocean. Nature 421, 354–357. doi: 10.1038/nature01340
Haug, G. H., Hughen, K. A., Sigman, D. M., Peterson, L. C., and Rohl, U. (2001). Southward migration of the intertropical convergence zone through the Holocene. Science 293, 1304–1308. doi: 10.1126/science.1059725
Henke, L., Lambert, F., and Charman, D. (2015). Was the Little Ice Age more or less El Nino-like than the Mediaeval Climate Anomaly? Evidence from hydrological and temperature proxy data. Clim. Past. Discuss. 11, 5549–5604.
Joshi, S. K., Ballabh, B., Negi, P. S., and Dwivedi, S. K. (2018). Diversity, distribution, use pattern and evaluation of wild edible plants of Uttarakhand, India. Def. Life Sci. J. 3, 126–135. doi: 10.14429/dlsj.3.12579
Kar, R., Ranhotra, P. S., Bhattacharyya, A., and Sekar, B. (2002). Vegetation vis-à-vis climate and glacial fluctuations of the Gangotri Glacier since the last 2000 years. Curr. Sci. 82, 347–351.
Kathayat, G., Cheng, H., Sinha, A., Spötl, C., Edwards, R. L., Zhang, H., et al. (2016). Indian monsoon variability on millennial-orbital timescales. Sci. Rep. 6, 1–7. doi: 10.1038/srep24374
Kotlia, B. S., Ahmad, S. M., Zhao, J. X., Raza, W., Collerson, K. D., Joshi, L. M., et al. (2012). Climatic fluctuations during the LIA and post-LIA in the Kumaun Lesser Himalaya, India: evidence from a 400 y old stalagmite record. Quat. Int. 263, 129–138. doi: 10.1016/j.quaint.2012.01.025
Kotlia, B. S., Singh, A. K., Joshi, L. M., and Dhaila, B. S. (2015). Precipitation variability in the Indian Central Himalaya during last ca. 4,000 years inferred from a speleothem record: impact of Indian Summer Monsoon (ISM) and Westerlies. Quat. Int. 371, 244–253. doi: 10.1016/j.quaint.2014.10.066
Kotlia, B. S., Singh, A. K., Zhao, J. X., Duan, W., Tan, M., Sharma, A. K., et al. (2017). Stalagmite based high resolution precipitation variability for past four centuries in the Indian Central Himalaya: chulerasim cave re-visited and data re-interpretation. Quat. Int. 444, 35–43. doi: 10.1016/j.quaint.2016.04.007
Kripalani, R. H., Kulkarni, A., and Sabade, S. S. (2003). Western Himalayan snow cover and Indian monsoon rainfall: a re-examination with INSAT and NCEP/NCAR data. Theor. Appl. Climatol. 74, 1–18. doi: 10.1007/s00704-002-0699-z
Kumar, P., Sanwal, J., Dimri, A. P., and Ramesh, R. (2019). Contribution of diverse monsoon precipitation over Central and Northern India during mid to Late Holocene. Quat. Int. 507, 217–223. doi: 10.1016/j.quaint.2018.10.003
Laskar, A. H., Yadava, M. G., Ramesh, R., Polyak, V. J., and Asmerom, Y. (2013). A 4 kyr stalagmite oxygen isotopic record of the past Indian Summer Monsoon in the Andaman Islands. Geochem. Geophys. Geosyst. 14, 3555–3566. doi: 10.1002/ggge.20203
Leipe, C., Demske, D., Tarasov, P. E., and Members, H. P. (2014). A Holocene pollen record from the northwestern Himalayan lake Tso Moriri: implications for palaeoclimatic and archaeological research. Quat. Int. 348, 93–112.
Li, J., Zhao, Y., Xu, Q., Zheng, Z., Lu, H., Luo, Y., et al. (2014). Human influence as a potential source of bias in pollen-based quantitative climate reconstructions. Quat. Sci. Rev. 99, 112–121.
Li, Y., Zhou, L., and Cui, H. (2008). Pollen indicators of human activity. Chin. Sci. Bull. 53, 1281–1293.
Liang, F., Brook, G. A., Kotlia, B. S., Railsback, L. B., Hardt, B., Cheng, H., et al. (2015). Panigarh cave stalagmite evidence of climate change in the Indian Central Himalaya since AD 1256: monsoon breaks and winter southern jet depressions. Quat. Sci. Rev. 124, 145–161. doi: 10.1016/j.quascirev.2015.07.017
Managave, S., Shimla, P., Yadav, R. R., Ramesh, R., and Balakrishnan, S. (2020). Contrasting centennial-scale climate variability in High Mountain Asia revealed by a tree-ring oxygen isotope record from Lahaul-Spiti. Geophys. Res. Lett. 47:e2019GL086170. doi: 10.1029/2019GL086170
Mann, M. E., Zhang, Z., Rutherford, S., Bradley, R. S., Hughes, M. K., Shindell, D., et al. (2009). Global signatures and dynamical origins of the Little Ice Age and Medieval Climate Anomaly. Science 326, 1256–1260. doi: 10.1126/science.1177303
Mazari, R. K., Bagati, T. N., Chauhan, M. S., and Rajagopalan, G. (1996). “Palaeoclimatic record of last 2000 years in trans-Himalayan Lahaul-Spiti region,” in Proceedings of the IGBP-PAGES/PEP-II Symposium on Palaeoclimate and Environmental Variability an Austral – Asian Transect During the Past 2000 Years, (Nagoya, Japan), 262–269.
Mishra, P. K., Prasad, S., Marwan, N., Anoop, A., Krishnan, R., Gaye, B., et al. (2018). Contrasting pattern of hydrological changes during the past two millennia from central and northern India: regional climate difference or anthropogenic impact? Glob. Planet. Chang. 161, 97–107.
Mittal, S., Tripathi, G., and Sethi, D. (2008). Development strategy for the hill districts of Uttarakhand. Working Paper No. 217. New Delhi: Indian Council for Research on International Economic Relations.
Mooley, D. A., and Parthasarathy, B. (1982). Fluctuations in the deficiency of the summer monsoon over India, and their effect on economy. Arch. Meteorol. Geophys. Bioclimatol. Ser. B 30, 383–398. doi: 10.1007/BF02324678
Nautiyal, C. M., and Chauhan, M. S. (2009). Late Holocene vegetation and climate change in Loktak Lake region, Manipur, based on pollen and chemical evidence. Palaeobotanist 58, 21–28.
Newton, A., Thunell, R., and Stott, L. (2006). Climate and hydrographic variability in the Indo-Pacific Warm Pool during the last millennium. Geophys. Res. Lett. 33:L19710. doi: 10.1029/2006GL027234
Pangtey, Y. P. S., Rawal, R. S., Bankoti, N., and Samant, S. S. (1990). Phenology of high-altitude plants of Kumaun in Central Himalaya, India. Int. J. Biometeorol. 34, 122–127. doi: 10.1007/BF01093457
Phadtare, N. R. (2000). Sharp decrease in summer monsoon strength 4000–3500 cal yr BP in the Central Higher Himalaya of India based on pollen evidence from alpine peat. Quat. Res. 53, 122–129. doi: 10.1006/qres.1999.2108
Polanski, S., Fallah, B., Befort, D. J., Prasad, S., and Cubasch, U. (2014). Regional moisture change over India during the past Millennium: a comparison of multi-proxy reconstructions and climate model simulations. Glob. Planet. Chang. 122, 176–185.
Ponton, C., Giosan, L., Eglinton, T. I., Fuller, D. Q., Johnson, J. E., Kumar, P., et al. (2012). Holocene aridification of India. Geophys. Res. Lett. 39:3. doi: 10.1029/2011GL050722
Prasad, S., Anoop, A., Riedel, N., Sarkar, S., Menzel, P., Basavaiah, N., et al. (2014). Prolonged monsoon droughts and links to Indo-Pacific warm pool: a Holocene record from Lonar Lake, central India. Earth Planet. Sci. Lett. 391, 171–182.
Rawal, R. S., Bankoti, N. S., Samant, S. S., and Pangtey, Y. P. S. (1991). Phenology of tree layer species from the timber line around Kumaun in Central Himalaya, India. Vegetatio 93, 108–118. doi: 10.1007/BF00033205
Rawat, G. S. (2017). “The Himalayan Vegetation along Horizontal and Vertical Gradients,” in Bird Migration across the Himalayas: Wetland Functioning amidst Mountains and Glaciers, eds H. H. T. Prins and T. Namgail (Cambridge: Cambridge University Press), 189–204.
Rawat, S., Gupta, A. K., Sangode, S. J., Srivastava, P., and Nainwal, H. C. (2015). Late Pleistocene–Holocene vegetation and Indian summer monsoon record from the Lahaul, northwest Himalaya, India. Quat. Sci. Rev. 114, 167–181. doi: 10.1016/j.quascirev.2015.01.032
Rowan, A. V. (2017). The ‘Little Ice Age’ in the Himalaya: a review of glacier advance driven by Northern Hemisphere temperature change. Holocene 27, 292–308.
Roy, I., Ranhotra, P. S., Tomar, N., Shekhar, M., Agrawal, S., Bhattacharyya, A., et al. (2022). Reconstruction of the late Holocene climate variability from the summer monsoon dominated Bhagirathi valley, western Himalaya. J. Asian Earth Sci. 227:105080. doi: 10.1016/j.jseaes.2022.105080
Sanwal, J., Kotlia, B. S., Rajendran, C., Ahmad, S. M., Rajendran, K., and Sandiford, M. (2013). Climatic variability in Central Indian Himalaya during the last∼ 1800 years: evidence from a high resolution speleothem record. Quat. Int. 304, 183–192. doi: 10.1016/j.quaint.2013.03.029
Shah, R. A., Achyuthan, H., Lone, A. M., Kumar, S., Kumar, P., Sharma, R., et al. (2020). Holocene palaeoenvironmental records from the high-altitude Wular Lake, Western Himalayas. Holocene 30, 733–743.
Sharma, C. P., Rawat, S. L., Srivastava, P., Meena, N. K., Agnihotri, R., Kumar, A., et al. (2020). High-resolution climatic (monsoonal) variability reconstructed from a continuous~ 2700-year sediment record from Northwest Himalaya (Ladakh). Holocene 30, 441–457.
Shi, F., Fang, K., Xu, C., Guo, Z., and Borgaonkar, H. P. (2017). Interannual to centennial variability of the South Asian summer monsoon over the past millennium. Clim. Dyn. 49, 2803–2814. doi: 10.1007/s00382-016-3493-9
Sinha, A., Cannariato, K. G., Stott, L. D., Cheng, H., Edwards, R. L., Yadava, M. G., et al. (2007). A 900-year (600 to 1500 AD) record of the Indian summer monsoon precipitation from the core monsoon zone of India. Geophys. Res. Lett. 34:L16707. doi: 10.1029/2007GL030431
Sinha, A., Kathayat, G., Cheng, H., Breitenbach, S. F., Berkelhammer, M., Mudelsee, M., et al. (2015). Trends and oscillations in the Indian summer monsoon rainfall over the last two millennia. Nat. Commun. 6, 1–8. doi: 10.1038/ncomms7309
Sinha, A., Stott, L., Berkelhammer, M., Cheng, H., Edwards, R. L., Buckley, B., et al. (2011a). A global context for megadroughts in monsoon Asia during the past millennium. Quat. Sci. Rev. 30, 47–62. doi: 10.1016/j.quascirev.2010.10.005
Sinha, A., Berkelhammer, M., Stott, L., Mudelsee, M., Cheng, H., and Biswas, J. (2011b). The leading mode of Indian Summer Monsoon precipitation variability during the last millennium. Geophys. Res. Lett. 38:L15703. doi: 10.1029/2011GL047713
Srivastava, P., Agnihotri, R., Sharma, D., Meena, N., Sundriyal, Y. P., Saxena, A., et al. (2017). 8000-year monsoonal record from Himalaya revealing reinforcement of tropical and global climate systems since mid-Holocene. Sci. Rep. 7, 1–10. doi: 10.1038/s41598-017-15143-9
Steinhilber, F., Abreu, J. A., Beer, J., Brunner, I., Christl, M., Fischer, H., et al. (2012). 9,400 years of cosmic radiation and solar activity from ice cores and tree rings. Proc. Natl. Acad. Sci. U. S. A. 109, 5967–5971. doi: 10.1073/pnas.1118965109
Terray, P., and Dominiak, S. (2005). Indian Ocean sea surface temperature and El Niño–Southern Oscillation: a new perspective. J. Clim. 18, 1351–1368. doi: 10.1175/JCLI3338.1
Tiwari, J. K., Ballabha, R., and Tiwari, P. (2010). Some promising wild edible plants of Srinagar and its adjacent area in Alaknanda valley of Garhwal Himalaya, India. J. Am. Sci. 6, 167–174.
Tiwari, M., Ramesh, R., Somayajulu, B. L. K., Jull, A. J. T., and Burr, G. S. (2006). Paleomonsoon precipitation deduced from a sediment core from the equatorial Indian Ocean. Geo-Mar. Lett. 26, 23–30. doi: 10.1007/s00367-005-0012-0
Veena, M. P., Achyuthan, H., Eastoe, C., and Farooqui, A. (2014). A multi-proxy reconstruction of monsoon variability in the late Holocene, South India. Quat. Int. 325, 63–73.
Walker, M. D., Ingersoll, R. C., and Webber, P. J. (1995). Effects of interannual climate variation on phenology and growth of two alpine forbs. Ecology 76, 1067–1083. doi: 10.2307/1940916
Wanner, H., Beer, J., Bütikofer, J., Crowley, T. J., Cubasch, U., Flückiger, J., et al. (2008). Mid-to Late Holocene climate change: an overview. Quat. Sci. Rev. 27, 1791–1828. doi: 10.1016/j.quascirev.2008.06.013
Yadav, R. K., Ramu, D. A., and Dimri, A. P. (2013). On the relationship between ENSO patterns and winter precipitation over North and Central India. Glob. Planet. Chang. 107, 50–58.
Yadav, R. R., Braeuning, A., and Singh, J. (2011). Tree ring inferred summer temperature variations over the last millennium in western Himalaya, India. Clim. Dyn. 36, 1545–1554. doi: 10.1007/s00382-009-0719-0
Keywords: palynology, stable carbon isotope, speleothem, oxygen isotope, late Holocene
Citation: Roy I, Tomar N, Ranhotra PS and Sanwal J (2022) Proxy Response Heterogeneity to the Indian Monsoon During Last Millennium in the Himalayan Region. Front. Ecol. Evol. 10:778825. doi: 10.3389/fevo.2022.778825
Received: 17 September 2021; Accepted: 11 May 2022;
Published: 13 June 2022.
Edited by:
Laurent Marquer, Max Planck Institute for Chemistry, GermanyReviewed by:
Anupama K, French Institute of Pondicherry, IndiaXianyong Cao, Institute of Tibetan Plateau Research (CAS), China
Copyright © 2022 Roy, Tomar, Ranhotra and Sanwal. This is an open-access article distributed under the terms of the Creative Commons Attribution License (CC BY). The use, distribution or reproduction in other forums is permitted, provided the original author(s) and the copyright owner(s) are credited and that the original publication in this journal is cited, in accordance with accepted academic practice. No use, distribution or reproduction is permitted which does not comply with these terms.
*Correspondence: Parminder Singh Ranhotra, cmFuaG90cmEucEBnbWFpbC5jb20=