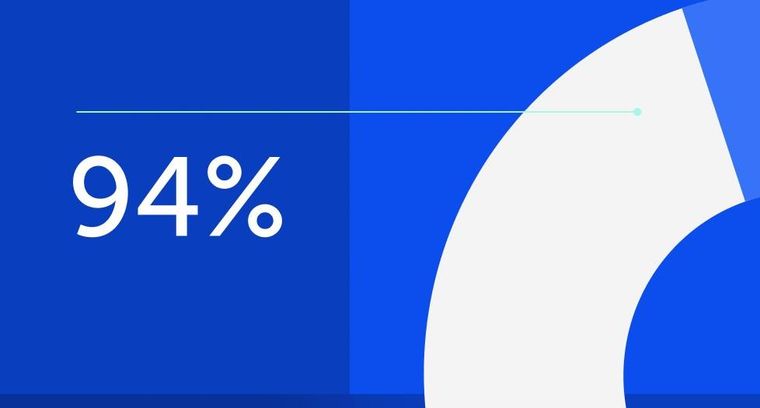
94% of researchers rate our articles as excellent or good
Learn more about the work of our research integrity team to safeguard the quality of each article we publish.
Find out more
ORIGINAL RESEARCH article
Front. Ecol. Evol., 26 April 2022
Sec. Behavioral and Evolutionary Ecology
Volume 10 - 2022 | https://doi.org/10.3389/fevo.2022.773568
This article is part of the Research TopicDrivers of Small-Mammal Community Structure in Tropical SavannasView all 14 articles
Vast stretches of East and Southern Africa are characterized by a mosaic of deciduous woodlands and evergreen riparian forests, commonly referred to as “miombo,” hosting a high diversity of plant and animal life. However, very little is known about the communities of small-sized mammals inhabiting this heterogeneous biome. We here document the diversity and abundance of 0.5–15 kg sized mammals (“meso-mammals”) in a relatively undisturbed miombo mosaic in western Tanzania, using 42 camera traps deployed over a 3 year-period. Despite a relatively low diversity of meso-mammal species (n = 19), these comprised a mixture of savanna and forest species, with the latter by far the most abundant. Our results show that densely forested sites are more intensely utilized than deciduous woodlands, suggesting riparian forest within the miombo matrix might be of key importance to meso-mammal populations. Some species were captured significantly more often in proximity to (and sometimes feeding on) termite mounds (genus Macrotermes), as they are a crucial food resource. There was some evidence of temporal partitioning in activity patterns, suggesting hetero-specific avoidance to reduce foraging competition. We compare our findings to those of other miombo sites in south-central Africa.
Miombo woodlands cover a vast area of eastern and southern Africa, and typically consist of a mosaic of open to dense deciduous woodlands dominated by Brachystegia and Julbernardia trees, interspersed with patches of evergreen riparian forests and wetlands (Frost, 1996; Banda et al., 2008; Munishi et al., 2011; Gumbo et al., 2018). Despite sustaining a diverse plant life, mainly due to the high annual rainfall (up to 700 mm/year; Frost, 1996; Godlee et al., 2020; Muvengwi et al., 2020), these habitats are characterized by shallow, nutrient-poor soils, and by unpalatable grasses (Frost, 1996; Loveridge and Moe, 2004; Montfort et al., 2021). Coupled with the scarcity of surface water during the dry season, and with annual fires depleting standing vegetation biomass, these factors contribute to differences in diversity and abundance of large mammal species compared to other savanna-woodland ecosystems in Sub-Saharan Africa, especially in the dry season when abundance drops (Caro, 1999; Waltert et al., 2009; Kavanna et al., 2014; Amaya et al., 2021; Davis et al., 2021). However, little is known about the effects of this environment on communities of small-sized mammals.
We know that some of miombo system characteristics could prove highly favorable for small (0.01–0.5 Kg) and “meso-mammals” (0.5–20 Kg; Frost, 1996; Timberlake et al., 2010). The availability of fruit, flowers, and seeds from a diverse woody plant flora, for example, might offer ample foraging opportunities for herbivorous rodents (and the small carnivores that prey on them), and allow for dietary niche differentiation and co-existence among multiple species (Frost, 1996). Furthermore, the low densities of ungulates and large carnivores (e.g., felids, canids) could attenuate the intensity of interspecific competition over food resources, and thus open up novel ecological niches for smaller species (Caro, 2001, 2002; Easter et al., 2019), especially if canopy cover prevents abundant grasses from sustaining large herbivore communities. Nonetheless, although such combined conditions (abundant food, reduced competition, and lower predation risk) are predicted to promote species-rich small and meso-mammal communities and large population sizes, available data point elsewhere.
Most studies reveal a general pattern of low rodent diversity and abundance in miombo systems characterized by vast expanses of uniform deciduous woodlands (Linzey and Kesner, 1997; Caro, 2001, 2002; Gaynor et al., 2021). By contrast, relatively larger numbers of small and meso-mammals (both at the species and population levels) are found in those miombo systems encompassing patches of well-watered, evergreen riparian forest or wetlands (e.g., Pettorelli et al., 2010; Rovero et al., 2017). This suggests that woodland resources might be of overall little value to small-sized mammals [possibly due to lower plant diversity, plant unpalatability, or water shortages, as proposed for larger mammals (Frost, 1996; Waltert et al., 2009)] with higher numbers found in more productive and botanically heterogeneous areas within the woodland matrix.
Rodent distribution in the miombo also appears to mirror that of widely dispersed biotic features, such as open grassland patches, human settlements, or termite mounds (Linzey and Kesner, 1997; Caro, 2001, 2002; Fleming and Loveridge, 2003). For example, in a test of the importance of termite mounds for small vertebrates in a Zimbabwe miombo system, results from live-trapping individuals revealed how several species were substantially more common on mounds, to the point that some would burrow only in proximity to termite colonies while avoiding the surrounding woodlands (Fleming and Loveridge, 2003). It is thus possible that the observed patterns of small-sized mammal diversity and abundance across a miombo system could also be heavily influenced by the availability of such “resource islands,” likely representing an important food source in an otherwise low-diversity biome.
The association of small and meso-mammals with highly localized botanical communities and biotic features (e.g., riparian forests, termite mounds) could also promote high levels of interspecific competition. Rare or widely scattered food and water have been widely documented to increase the rate of encounter among competing mammalian species sharing the same resources, with substantial fitness costs in terms of direct interference competition (e.g., injuries, unsustainable energetic expenses; Dickman, 1991; Valeix et al., 2007). This is in turn is reflected by fine-scale strategies to avoid interference, among which temporal partitioning in activity patterns is the most common (Kronfeld-Schor and Dayan, 2003; Frey et al., 2017). One study of small carnivores reported activity patterns shifts among co-existing miombo-dwelling species with a similar diet (e.g., insects and rodents), therefore pointing to temporal partitioning as an important behavioral strategy to cope with limited resource availability (Easter et al., 2019).
So which habitat types and environmental features favor the presence of small-sized mammalian taxa within a miombo ecosystem? In the current study, we investigated the environmental factors underlying smaller mammal diversity and abundance in a relatively undisturbed miombo mosaic habitat, the Issa Valley of western Tanzania (“Issa” from now onward) using a network of motion-triggered camera traps (CTs) over a 3-year period (2016–2018). Since CTs are usually not triggered by animals < 0.5 kg in body mass (Rowcliffe and Carbone, 2008), we restricted our investigation to meso-mammals (rodents, elephant shrews, lagomorphs, pangolins, and small carnivores weighing more than 0.5 kg). This excluded a large proportion of small mammals which could not be detected by CTs, such as the entire guild of small rodents. We report also results for species smaller than 0.5 kg, with the caveat that the frequency of detection might be negatively biased by smaller body sizes. While there are published species lists of large mammals from the study area (Piel et al., 2019), little is known about the meso-mammal community. We first used the CT network to compute an inventory of the species found in the study area. Issa is characterized by a highly varied ecological landscape, with deciduous woodlands on high-lying plateaus, and an extensive system of river valleys hosting closed-canopy, evergreen riparian forests. We expected to find higher species diversity in river valleys characterized by riparian forests than in deciduous woodlands. Relative abundance was considered as an indicator of the intensity of habitat use rather than an estimate of local densities, given that individual identification was not possible and the same individuals could have been captured multiple times on CTs.
We then applied CT data to identify the ecological drivers of the intensity of site use by meso-mammals, using capture rates as indicators of relative abundance (Rovero and Marshall, 2009; Rovero et al., 2014). Specifically, we tested the hypothesis that the deciduous woodlands at Issa would represent a less suitable habitat for meso-mammals than the riparian forests, as proposed previously for larger species (Frost, 1996; Waltert et al., 2009). We further predicted that meso-mammals would be relatively more abundant at CT sites located in the riparian forests of river valleys (characterized by steep slopes and low elevations) rather than on plateau woodlands, given the supposedly greater availability of forage, cover, and water in the forests. We also predicted that all insectivorous species would be more frequently captured at CT sites located in proximity to termite mounds (of the genus Macrotermes), as the latter might provide important but highly localized food and shelter sources. Relative abundance was considered as an indicator of the intensity of habitat use rather than an estimate of local densities, given that individual identification was not possible and the same individuals could have been captured multiple times on CTs.
Finally, we explored whether the presumed scarcity and/or wide dispersion of miombo resources would translate into fine-scale temporal partitioning in activity patterns throughout the day and night among meso-mammal species. We thus predicted that species would exhibit little temporal overlap in primary time of activity between each species pair that shared similar diets. In particular, insectivorous, nocturnal species were expected to show a high degree of differentiation among them in night-time activity patterns, to avoid direct interference over shared food resources.
The Issa valley lies in the Tongwe West Forest Reserve, western Tanzania (Figure 1). Issa comprises steep river valleys (900 m asl) and high-lying plateaus (1,800 m asl; Piel et al., 2015, 2019; Mayengo et al., 2020). The entire region is dominated by deciduous miombo woodlands and closed-canopy, evergreen riparian forests, typically located in the valleys between steep slopes (Piel et al., 2019). Woodlands are dominated by Brachystegia and Julbernardia trees, while a variety of woody plant species typical of the central African lowland rainforests is found in the riparian forests (Piel, 2018; Piel et al., 2019). Mean annual temperatures range between 11 and 38°C, while mean annual rainfall ranges between 900 and 1,400 mm. Rainfall patterns denote distinctive wet (November–April) and dry seasons (May–October) (Piel et al., 2019; Mayengo et al., 2020). Common medium/large mammals include chimpanzee (Pan troglodytes), yellow baboon (Papio cynocephalus), roan antelope (Hippotragus equinus), Lichtenstein’s hartebeest (Alcelaphus lichtensteinii), and bushpig (Potamochoerus larvatus), with larger predators such as lion (Panthera leo), leopard (P. pardus), wild dog (Lycaon pictus), and spotted hyena (Crocuta crocuta) also present but at low densities (Piel et al., 2019). Issa is surrounded by small (formerly refugee) settlements established in the 1970s. Humans sporadically visit the area for wildlife snaring, small-scale logging, honey collection, or livestock herding, but anthropogenic impacts on the landscape remain modest (Piel et al., 2015, 2019).
Figure 1. Location of the camera traps (n = 42) deployed between January 2016 and December 2018 in the Issa Valley, in relation to the different vegetation types. The Issa station represented the main research base for the study. The camera trap site in “grassland/swamp” was instead located in a patch of deciduous woodland, and thus corrected upon field examination of the site.
We deployed a total of 42 motion-triggered, infrared CTs (Bushnell Trophy Cam HD Aggressor; and Reconyx Hyperfire 2 HF2X) at Issa over a 3-year period (December 2015–December 2018). Due to mechanical camera failures, this was for a total of 21,517 camera days (Supplementary Material). Camera days were calculated as the number of calendar days in which a CT was deployed and active in the field. Distances between single CT sites were at least 150 m, covering an area of 14.45 km2. We selected CT sites to sample different vegetation types, with half in deciduous woodlands (n = 21; camera days mean ± S.E. = 427.57 ± 68.82; range = 47–986), and the other half in riparian forests (n = 21; camera days mean ± S.E. = 597.05 ± 67.51; range = 49–940; Supplementary Material). We attached CTs to large trees, 30–90 cm above the ground, following recommendations for camera trapping of small- and medium-sized mammals (Rowcliffe and Carbone, 2008; Ortmann and Johnson, 2021). To maximize capture probability, we deployed CTs primarily along forest and woodland trails—commonly utilized by terrestrial vertebrates (Rovero et al., 2010; Cusack et al., 2015). Moreover, tall grass and woody plants growing in the CTs line of sight were artificially removed at regular intervals. Of the 42 CTs deployed, 15 were positioned in proximity of large termite mounds of the genus Macrotermes (the most common in the study area; Mayengo et al., 2020; Table 1). CTs faced the mounds, which were confirmed to be actively occupied by termite colonies in the course of a parallel study on primate feeding behavior. All CTs were set on video mode (video duration: day = 60 s; night = 15 s) with a 1 s delay between exposures, and visited once a month to retrieve SD cards and change batteries. For statistical analyses, CTs active for less than 30 calendar days (“camera days” from now onward) were removed from the dataset.
Table 1. Relative Abundance Indices (RAIs) for the meso-mammal species (n = 19) of Issa Valley, Tanzania.
We identified all meso-mammals (rodents, hares, elephant-shrews, small carnivores, and pangolins) captured on CTs to species level (except when lighting conditions or poor video quality prevented an unequivocal identification). The giant and southern ground pangolins (Smutsia gigantea and S. temmincki, respectively) were considered together as “pangolins,” since they could not be easily distinguished from each other based on the resolution of the available video material.
In QGIS v. 3.14.1, we extracted the elevation (m) of each CT site from a Digital Elevation Model (DEM) at 90 m spatial resolution. We then then calculated slope as a percentage value (%), using the Terrain Analysis toolbox in QGIS. The vegetation type of all CT sites was derived from a vegetation map based on Landsat satellite imagery, at a spatial resolution of 30 m. Vegetation type was classified as either “deciduous woodland” or “riparian forest” and later confirmed on the ground. In a small number of cases (n = 4), there was disagreement between the available vegetation-class map and ground observations, probably reflecting a failure of remote-sensing imagery to capture small-scale variation in habitat structure. This was therefore rectified in our final dataset. Finally, we classified all CT sites as either located at termite mounds or not.
We conducted all statistical analyses in R v. 3.5.2 (R Core Team, 2020). We calculated the Relative Abundance Index (RAIs) for all identified meso-mammals as the number of videos obtained for each species, divided by the overall number of camera days (Rovero et al., 2010; Palmer et al., 2018). We excluded multiple capture events of the same species on the same camera day from analyses. We did this to minimize the risk of pseudo-replication generated by double-counting the same individual on the same day, which was more conservative than the threshold adopted by other studies (Bowkett et al., 2008). Although not excluding the possibility of capturing the same individuals on different days and at different CT sites, this conservative approach was deemed necessary to exclude repeated sequences of videos from the same individuals of certain species remaining in proximity of CT sites with termite mounds for very long periods while feeding on termites or waiting for swarming events. RAIs also were calculated separately for woodland vs. forest vegetation types, to compare differences in meso-mammal relative abundance between the two main vegetation types found at Issa. We performed all computations in the package unmarked (Fiske et al., 2015).
To determine the drivers of meso-mammal relative abundance, we used the number of camera days on which different species were captured at each CT site as a proxy for the intensity of site use (Bowkett et al., 2008; Rovero et al., 2010). We limited our analyses to five species that were captured by CTs on > 50 camera days (Mori et al., 2020), namely: Bushy-tailed Mongoose (Bdeogale crassicauda), Chequered Elephant-shrew (Rhynchocyon cirnei), Crested Porcupine (Hystrix cristata), Giant Pouched Rat (Cricetomys gambianus), and Large-spotted Genet (Genetta maculata). General characteristics of these species are presented in Table 2. We used Generalized Linear Models (GLMs) for count data to describe the relationship between relative abundance and the environmental covariates recorded at each CT site (elevation, slope, vegetation type, and presence of termite mounds; Bowkett et al., 2008). Models were fitted separately for each of the study species. Elevation was transformed from continuous to categorical (>1,450 m and < 1,450 m) following multiple convergence issues (Allison et al., 2004). The number of camera days also was entered in the models as an offset variable to control for variation in “effort” between CTs. Due to the small sample size for most species (≤150 captures over 3 years for three out of five species), and our GLM-based approach, it was not possible to account for seasonal variation in relative abundance, and this should be considered when interpreting our results. We selected a zero-inflated negative binomial distribution for all GLMs, given significant over-dispersion in the count data and the large number of non-captures (i.e., species absent at certain CT sites for the entire study period). All models were built in the glmmTMB package (Magnusson et al., 2017).
Table 2. Averaged coefficient estimates and significance levels for predictors of relative abundance of five most common meso-mammal species in the Issa Valley, Western Tanzania.
We tested model fit to a zero-inflated negative binomial distribution by using the dispersion test and the Kolmogorov-Smirnov test for fitted vs. simulated residual distribution in the DHARMa package (Hartig, 2017). All tests returned a P-value larger than 0.2, therefore confirming the goodness-of-fit of our models. Exact binomial tests did not detect any outliers. The potential for multicollinearity among environmental covariates was checked by computing Variance Inflation Factors (Kutner et al., 2005). Since all VIFs were < 2, and therefore well below the accepted threshold of concern (5–10; Kutner et al., 2005), we excluded that multicollinearity had a significant effect on model coefficient estimates.
We selected models with the lowest Akaike’s Information Criterion (AIC, corrected for small sample size) and the largest Akaike’s weights (wAIC) as those best explaining the relative abundance of each meso-mammal species (Burnham and Anderson, 2002; Wagenmakers and Farrell, 2004; Burnham et al., 2011). AIC values were compared among competing models containing all possible combinations of environmental covariates as main effects. For models differing by ≤ 4 in AIC, conditional averaged coefficients were calculated in the package MuMiN (Barton, 2015). To estimate the overall degree of temporal overlap in activity patterns among the five most common study species (see above), we reported the local time at which each species was captured on CT in our dataset. Capture events were again restricted to one per day (randomly selected) to minimize the risk of pseudo-replication; as the same individuals visited CT sites for prolonged periods while feeding on termite mounds, the choice of one event per day ensured that each observation corresponded to an independent activity bout, given the body size of the study species (a 1-day interval was taken as longer than the time required by meso-mammals to traverse a home range; Swihart et al., 1988). In the overlap package (Meredith and Ridout, 2014), we calculated the overlap coefficient of temporal activity pattern for each species pair (delta; Monterroso et al., 2014). We adopted the delta1 estimator when at least one of the study species was captured in ≤75 events, and the delta4 when both species were captured in ≥ 75 events (Monterroso et al., 2014). The 95% confidence interval for the delta obtained from each species pair was then computed using percentile intervals of 999 bootstrap samples (Monterroso et al., 2014). Overlap in activity patterns was considered low when < 0.05, moderate when between 0.05 and 0.75, and high when > 0.75 (Mori et al., 2021). We then tested for finer-scale differences in activity patterns between species pairs by using bootstrap tests (number of bootstrap repetitions = 999) with the function compareCKern in the package activity (Rowcliffe et al., 2014; Mori et al., 2021). Bootstrap tests compare two sets of circular observations (i.e., daily activity patterns of two study species over a 24-h period) and determine the statistical significance that the two observations belong to the same temporal distribution. Rejection of the null hypothesis (P < 0.05) was considered as indicative of significantly different activity distributions between species pairs. A limited sample size prohibited our estimating temporal activity overlap between woodlands and riparian forests. However, our study covered a small area, and thus spatial segregation between species is unlikely.
We detected 19 species of meso-mammals on CTs in Issa (Table 1). RAIs indicated that two species, namel the Bushy-tailed Mongoose and the Giant Pouched Rat, were substantially more common than the others and captured on a total of 897 and 1,078 camera days, respectively. Only three other species were captured on videos on more than 50 camera days. According to our expectations, most species (n = 10) exhibited a higher relative abundance at CT sites located in riparian forests rather than woodlands. Conversely, seven species were more often observed in the woodlands, while two (the Honey Badger Mellivora capensis and Rock Hyrax Procavia capensis) presented qualitatively similar RAIs between the two vegetation types. We also observed that some species completely avoided one vegetation type or the other (Table 1). For example, meso-mammals typical of open habitats, like the Serval (Leptailurus serval) and the Savanna Hare (Lepus victoriae) were never captured at riparian forest CT sites. On the other hand, species that are typical of dense habitats or are highly dependent on available surface water, such as the African Clawless Otter (Aonyx capensis), Marsh Mongoose (Atilax paludinosus), and White-tailed Mongoose (Ichneumia albicauda), were found only in the water-rich and well-vegetated riparian forests. These patterns suggest that species typical of different ecosystems coexist in Issa thanks to habitat heterogeneity. However, RAIs should be interpreted with caution, as most of them were computed for very small sample sizes and without taking into account the potential for spatial auto-correlation among CT sites. The use of RAIs also does not allow for estimating variation in the probability of detection among CT sites due to local micro-site conditions, and this should be taken into account for the interpretation of our results.
Through our modeling approach, we found that three of the five most common meso-mammal species at Issa, namely the Bushy-tailed Mongoose, Giant Pouched Rat, and Chequered Elephant-shrew, were significantly more abundant at CT sites located in forests rather than woodlands (Table 2 and Figure 2). Moreover, the relative abundance of the Giant Pouched Rat and of the Chequered Elephant-shrew was also higher at lower elevations (<1,450 m) and positively correlated with slope (Table 2), indicating that these two species utilized the Issa valley system more intensely than the plateaus. Similar trends, although non-significant, were also observed for the Bushy-tailed Mongoose (positive correlation with slope) and for the Large-spotted Genet (more abundant at lower elevations; Table 2). According to our expectations, three of the four insectivorous-omnivorous species, the Bushy-tailed Mongoose, Giant Pouched Rat, and Large-spotted Genet, were also more frequently captured at CT sites located near termite mounds, rather than along game trails (Table 2 and Figure 3). Only the Chequered Elephant-shrew, despite its insect-based diet, was not more common at CT sites with termite mounds than at sites without mounds (Table 2 and Figure 3). No environmental covariates explained the variation in relative abundance among CT sites for the Crested Porcupine.
Figure 2. Average number of camera days (Mean ± S.E.) in which each meso-mammal species in Issa Valley was detected, compared between CT sites in deciduous woodland (n = 20) and riparian forest (n = 22).
Figure 3. Average number of camera days (Mean ± S.E.) in which each meso-mammal species was detected, compared between CT sites located at termite mounds (n = 15) and sites with no termite mounds in close proximity (n = 27).
We observed that four out of the five most common meso-mammals at Issa, excluding the Chequered Elephant Shrew, were largely nocturnal. Circadian overlap in overall activity patterns was thus high (>0.75) between all species (excluding the diurnal elephant shrew), irrespective of dietary similarities (Table 3 and Figure 3). For example, the Large-spotted Genet, which is carnivorous but also consumes large quantities of insects at certain times of the year, exhibited substantial activity overlap (Δ > 0.75; Table 3) with the Bushy-tailed Mongoose, and with the Giant Pouched Rat, which also often feed on insects. Nonetheless, some partitioning in activity patterns could be detected using bootstrap testing, as all species exhibited significant finer-scale differences in their temporal activity distributions between each other (Table 3 and Figure 4). The Giant Pouched Rat was more active during earlier night hours compared to the Bushy-tailed Mongoose, in spite of substantial overlap in overall activity times (Δ > 0.75; both species largely nocturnal; Figure 4). Similarly, the Large-spotted Genet was also active at night, but with more detections during early morning than the mongoose and the rat (Table 3 and Figure 4). The Chequered Elephant-shrew, which is largely diurnal, appeared to almost completely segregate temporally from the larger Bushy-tailed Mongoose, Giant Pouched Rat, and Large-spotted Genet (Table 3 and Figure 4) by concentrating its activity peaks in the late morning and early evening (09:00–12:00 a.m. and 08:00–09:00 p.m., respectively). Surprisingly, all primarily insectivorous species showed significantly different temporal distributions of activity from the herbivorous Crested Porcupine, which feeds mostly on roots and therefore does not represent a potential competitor over shared forage resources (Table 3 and Figure 4).
Table 3. Estimates of temporal overlap in activity patterns (Δ ± 95% C.I.) for pairs of the five most common meso-mammal species in the Issa Valley.
Figure 4. Overlap in activity patterns between the five most common meso-mammal species in the Issa Valley, Tanzania. The plots show time over a 24-h period on the x-axis (note that at the latitude of Issa, sunrise is at about 06:00 h, and sunset at 18:00 h, throughout the year); and density of detection events, on the y-axis.
In this study, we used motion-triggered camera traps to investigate the ecology of a meso-mammal community in the miombo mosaic ecosystem of the Issa Valley, western Tanzania. We hypothesized that the vast deciduous woodlands characterizing the miombo would support a relatively low diversity of meso-mammal species due to the scarcity of resources for terrestrial vertebrates reported in other studies. We found that the meso-mammal community in the study area comprised a relatively small number of species (n = 19), most of them occurring at very low relative abundance. We also found that meso-mammals were more abundant in the riparian forests associated with river valleys than in the deciduous woodlands, likely due to the greater availability of forage, protective cover, and water in the wetter vegetation. Moreover, we observed that some insectivorous and omnivorous species were more abundant in immediate proximity to termite mounds, which could thus represent important resource islands for meso-mammals in the miombo. Finally, we investigated whether the presumed resource scarcity in the miombo could translate into temporal partitioning in activity patterns, so as to avoid interference competition among those species sharing similar diets, and we found evidence in support of this hypothesis.
Landscape heterogeneity may increase meso-mammal diversity through various mechanisms, from increasing food diversity (e.g., seeds, plants, invertebrates), to providing cover from predators, to increasing dispersal success through habitat connectivity (Price et al., 2010). At Issa, the species composition and relative abundance of meso-mammals mirrors that of other study sites characterized by high vegetative heterogeneity, such as Udzungwa and Mahale (Pettorelli et al., 2010; Rovero et al., 2017). Although forested areas are better represented in these sites, results from Udzungwa and Mahale also revealed that the most abundant meso-mammals were the Bushy-tailed Mongoose and the Giant Pouched Rat (Pettorelli et al., 2010; Rovero et al., 2017), similar to our findings. These were the only two species with RAIs > 1; only five other species occurred at RAIs between 0.1 and 0.5 (Table 1). All other species occurred at very low relative abundance, with RAI scores < 0.1. These patterns suggest that the Issa system might not represent a highly suitable environment for meso-mammals, given that only a handful of species thrive in it.
The greater relative abundance of several species in riparian forests suggests that these are important resources to meso-mammals in this mosaic system. Riparian forests usually have a very high diversity of plant species, offer nutrient-rich plant forage due to eutrophic soils, do not burn seasonally, and are located in proximity to permanent water sources (Naiman et al., 2010; Timberlake and Chidumayo, 2011). Moreover, the closed canopy cover of riparian forests provides a dark undergrowth environment, which might offer protection against larger diurnal predators (Prugh and Golden, 2014). Deciduous woodlands, on the other hand, grow on nutrient-depleted soils (resulting in low-quality plant forage), are seasonally depleted of plant forage, burn during the dry season, are well-lit due to sparser canopy cover, and generally have little to no surface water (Frost, 1996; Timberlake and Chidumayo, 2011). Riparian forests thus likely hold important year-round resources for meso-mammals, especially in terms of forage, moisture, and protective cover. The same patterns were found for rodents in the Ruvuma Landscape in southern Tanzania (Nkwabi et al., 2018). On the other hand, a study in the Katavi National Park (∼200 km South of Issa) reported a smaller number of meso-mammal species in the same size range examined here (14 species vs. 19 species at Issa; Table 1; Caro, 2003). Since Katavi is largely dominated by deciduous woodlands and grasslands, with little evergreen forest cover (Banda et al., 2008), our findings highlight the importance of riparian forests as key habitat for meso-mammals across different miombo systems.
Another possibility is that the greater relative abundance of meso-mammals observed in riparian forests at Issa could derive from a greater detectability at riparian forest CT sites, caused by differences in micro-habitat conditions between the two vegetation types. This seems unlikely, however, given that riparian forests present low-lighting conditions and more complex vegetation backdrops, which should make CT detections less likely compared to woodlands (i.e., the opposite of what we observed). Additionally, some species that are arboreal or climbers (e.g., the genet and, to a lesser extent, the Giant Pouched Rat), given similar local densities, should have been more easily detected at woodland sites, where they are forced to move for longer distances on the ground. Taken together, these considerations indicate that the greater number of CT detections in riparian forests reflect a genuinely higher intensity of forest site use compared to woodland sites, and not an artifact of our sampling design.
Whereas some species exhibited clear preference for either open or closed vegetation, some generalist species (e.g., Crested Porcupine, Large-spotted Genet) were equally abundant in woodlands and forests. This could reflect their greater plasticity in habitat tolerance. Both the savanna/grassland species and the generalist species occurred at much lower relative abundances than the forest species; this may indicate an advantage for forest-dwelling species that lie in the miombo mosaic in the form of greater diversity of resources and greater availability of water and protective cover in riparian forests.
Termite mounds are known for their abundant supply of highly nutritious prey for mammals (Redford and Dorea, 1984) and role in increasing biodiversity (Fleming and Loveridge, 2003). Given increased RAI scores at termite mounds for 3/5 of the species we surveyed, these resources are clearly important predictors of meso-mammal habitat use in the miombo. The Bushy-tailed Mongoose is an ant and termite specialist (Kingdon, 2015), so we expected this relationship. On the contrary, the Large-spotted Genet usually feeds on beetles, grasshoppers, and small vertebrates where it has been studied previously (Roberts et al., 2007), but at Issa it may rely on termites in miombo areas between October-December when several swarming events are commonly observed (D’Ammando, pers. obs.). Similarly, the Giant Pouched Rat is reportedly granivorous, but we observed several instances of rats consuming termites from CT recordings. Termite mounds may offer protective cover to several mammal species, as nesting in mound burrows has been suggested to explain the greater abundance (both in terms of species and of individuals) of rodents in the vicinity of mounds in miombo ecosystems (Fleming and Loveridge, 2003). Future studies could resolve this issue by investigating whether the abundance of insectivorous mammals in miombo habitat increases in concomitance with alate swarming events (Dial and Vaughn, 1987), thereby indicating that the mounds may also act primarily as food sources.
The relatively low abundance of the Chequered Elephant-shrew on Macrotermes mounds is surprising, given that elephant shrews broadly (Woodall and Currie, 1989) and this species specifically (Redford, 1987) is known to feed on termites. It could be that the larger size of M. subhyalinus and M. bellicosus are chemically or anatomically prohibitive to consume (Longhurst et al., 1978; Phillips et al., 2021). Our interpretation is that this could reflect a behavioral strategy to avoid the other meso-mammals attracted to termite mounds. Chequered Elephant-shrews are much smaller than sympatric species at Issa and may thus incur substantial costs from competition with mongooses and genets. Moreover, the small carnivores commonly observed at termite mounds could also represent potential predators for the elephant shrew, thereby acting as a deterrent. These considerations were supported by the temporal segregation of the elephant shrew from other species.
High (temporal) overlap in activity patterns among three of four omnivorous and insectivorous species could be interpreted as evidence of little interference competition among meso-mammals in miombo ecosystems, implying that resources may not be limited. Most meso-mammal encounters were indeed in riparian forests and/or on termite mounds, which presumably offer plentiful resources (i.e., year-round green foliage, fruits, and termite swarms) when compared to deciduous woodlands. This interpretation is however, invalidated by the fact that significant differences in fine-scale temporal distributions of activity patterns were detected between all pairs of study species. These observations suggest that meso-mammals at Issa tend to actively avoid each other, thereby indicating a high potential for interference competition over limited resources. Indeed, although foraging opportunities might be abundant in riparian forests and on termite mounds, the small areal extent and patchy distribution of these habitat features likely constrains the amount of available food. Our findings thus corroborate the hypothesis that the miombo offers scarce and widely scattered resources to meso-mammals, reflected by the behavioral mechanisms of temporal avoidance observed within the omnivorous/insectivorous guild.
The unexpected differences in temporal activity distribution between the four insectivorous/omnivorous species and the root-eating Crested porcupine warrant further investigation. It is possible that the porcupine is avoided by other species due to its large size and quills, which could pose a serious danger to smaller mammals during direct encounters. Another possibility is that overlap in diet could be higher in miombo ecosystems, with species that are typically targeting insects or fruit also feeding on roots and other underground vegetal material favored by the porcupine. Detailed analyses of behavior and dietary preferences will be needed to clarify the ecological implications of this finding.
One of the distinguishing characteristics of miombo woodlands, especially in western Tanzania, is the extreme seasonality, with a 6-month wet season when most of the annual rain falls. Moreover, with anthropogenic fires burning the landscape a few months after the last rains, the physical environment is transformed in ways that are known to influence animal presence and behavior (Frost, 1996; Desanker et al., 1997; Mayengo et al., 2020). For example, rains stimulate termite activity, which can result in alate feasts for predators (Dial and Vaughn, 1987), and fires promote growth of new forage for herbivores (Green et al., 2015). Unfortunately, the effect of seasonality on the relative abundance of meso-mammals in the region remains unknown. For small mammals (<0.5 kg), which might share forage resources with meso-mammals (e.g., seeds, arthropods), reported seasonal effects on abundance are inconsistent. Using Sherman traps in Katavi National Park (∼250 km SE of Issa), Caro (2001, 2002) reported a slight increase in overall captures of rodents in the dry season compared to the wet but later, Fitzherbert et al. (2007) reported no seasonal difference in captures using the same method and from the same area. In the Ruvuma area, in southern Tanzania, although data were collected across a single season, Nkwabi et al. (2018) found an interaction between vegetation and burned stage with more rodent diversity in riparian forests during sprouting, compared to freshly burned periods, whereas for miombo woodlands, they reported more diversity during recently burned periods. In the most comprehensive study, Taylor and Green (1976) used “breakback traps” to study reproduction in small mammals in Kenya. They reported a drop in abundance from early to late wet season and from mid to late dry season, similar to results from Malawi a decade later (Happold and Happold, 1991). Nonetheless, all these studies focused on smaller species than those considered for this study (therefore making comparisons difficult), and adopted different sampling methodologies.
We were not able to study the effect of seasonality and especially fire in the current study due to a limited sample size, constraints to our study design (i.e., sampling effort was biased toward the wet season, while most species had too few captures per season to estimate meaningful effect sizes), and lack of field data (on the effects of burning events at the scale of CT sites). Analyses of seasonal effects were also incompatible with our modeling approach, as duplicating camera trap sites for wet vs. dry season, while keeping all other variables constant (i.e., vegetation type, presence of termite mounds, slope, elevation) would have severely violated GLM assumptions of independence between data points and thus undermined our interpretation of the results, However, we hope that future studies incorporate these likely important influences on meso-mammal presence.
Moreover, while the number of cameras deployed for this study and the temporal coverage (36 months) are sufficient to address questions of relative abundance, there remain limitations. First, we made no attempt to quantify resource availability or evaluate habitat suitability for any of these species. Additional investigation into micro-habitat features that may promote some species over others would be very useful to discussion on inter-specific competitive dynamics. Second, comparative work from other miombo systems would allow us to better contextualize our findings. All studies to date have adopted live-trapping as a method to assess miombo small-sized mammal abundance (e.g., Caro, 2001, 2002) thus focusing on smaller species than those we considered for our CT approach. There is a dearth of published literature on meso-mammal assemblages across dry tropical woodland biomes, which means that we are unable to be confident in whether our results are typical or not for miombo mosaic systems. Finally, by identifying individual animals, we could further extrapolate population densities of each species and clarify the effects of differences in the probability of detection between CT sites on estimates of relative abundance and intensity of habitat use. Given the rise of machine learning applications to camera trap footage, future studies that integrate AI would allow even more robust analyses on resulting data (Ahumada et al., 2020).
While there are numerous, global studies on mammal declines in the Anthropocene, many focus on larger, especially charismatic species (Harris et al., 2009; Junker et al., 2012). Far fewer have focused on meso-mammals (but see Kennerley et al., 2021). This study, along with others in this Special Issue, represent an attempt not just to census meso-mammal assemblages, but also to begin to understand what drives their (relative) abundance, especially in a system that characterizes a large portion of eastern and southern Africa.
Small-sized mammal communities are reliable reflectors of biodiversity (Keller and Schradin, 2008) and anthropogenic activity (Griffiths et al., 2015) and thus can serve as important proxies through low-cost, low-maintenance remote monitoring like camera trapping. Compared to sympatric larger species, their smaller ranges and enhanced adaptability may make them more resilient to natural and anthropogenic pressures. As such, data that inform on their natural habitat preferences, strategies to minimize inter-specific competition, and overall ecology are critical when habitat change is imposed on their system. We hope to have shed light on a small (geographic) system here, but one that can serve as a model for other parts of eastern-southern Africa where miombo mosaic landscapes dominate.
The data have been deposited in a Dryad Repository (https://datadryad.org/stash/share/Ep6xSDSXQITHh-nfb6cviz1x SXF0VqeIG5nZ6c-VbYo).
Ethical review and approval was not required for the animal study because data collection was based on camera traps, a non-invasive method which does not interfere with animal behavior, and does not involve contact with or manipulation of the study subjects- research was approved by Tanzania Commission for Science and Technology and by the Tanzania Wildlife Research Institute.
AP, FS, and GD’A conceived the study. GD’A, SP, and PS collected and cleaned the data. GD’A performed the statistical analyses. GD’A and AP wrote the manuscript to which TC, VO, FS, SP, and PS contributed. All authors contributed to the article and approved the submitted version.
We are grateful to the Salk Institute Centre for Academic Research in Anthropogeny/University of California San Diego for continuous support to the Issa field station.
The authors declare that the research was conducted in the absence of any commercial or financial relationships that could be construed as a potential conflict of interest.
All claims expressed in this article are solely those of the authors and do not necessarily represent those of their affiliated organizations, or those of the publisher, the editors and the reviewers. Any product that may be evaluated in this article, or claim that may be made by its manufacturer, is not guaranteed or endorsed by the publisher.
We express our gratitude to the Tanzania Commission for Science and Technology (COSTECH) and to the Tanzania Wildlife Research Institute (TAWIRI) for permission to conduct field work in the Issa Valley. We thank the past members of the UCSC Chimpanzee Video Coding Team (Gabriel Granado, Kaya Zepeda, Kelsea Ranks, Kimberly Zhu, Lindsay Gillespie, Naima Tucker, Sofia Jo, Stacy Goodwyll, Victoria Collins, Angelique Wong, Audrey Ahearn, Cielo De La Rosa, Jenna Di Napoli, Josephine Serpan, Julie Elliott, Margot Kilroy, Maria Borges, Ricky Sierra, Spencer Hardy, Spencer Witte, Allegra Stahl, Laura Tellez, Lauryn Hobbs, Ree Hawksworth-Lutzo, Sarah Copper, and Vanessa Morgan) for their considerable contribution to the screening and coding of the video material used in this study. We would also like to acknowledge the Greater Mahale Ecosystem Research and Conservation field team for their crucial contribution to various phases of the study. Long term support for GMERC was generously provided by the UCSD/Salk Center for Academic Research and Training in Anthropogeny (CARTA).
The Supplementary Material for this article can be found online at: https://www.frontiersin.org/articles/10.3389/fevo.2022.773568/full#supplementary-material
Ahumada, J. A., Fegraus, E., Birch, T., Flores, N., Kays, R., O’Brien, T. G., et al. (2020). Wildlife insights: a platform to maximize the potential of camera trap and other passive sensor wildlife data for the planet. Environ. Conserv. 47, 1–6. doi: 10.1017/S0376892919000298
Allison, P., Altman, M., Gill, J., and McDonald, M. P. (2004). “Convergence problems in logistic regression,” in Numerical Issues in Statistical Computing for the Social Scientist, eds W. A. Shewhart and S. S. Wilks (New York, NY: Wiley), 238–252. doi: 10.1002/0471475769.ch10
Amaya, P. C., Nourtier, M., Montfort, F., Fusari, A., Randrianary, T., Richard, E., et al. (2021). Are elephants attracted by deforested areas in miombo woodlands? Afr. J. Ecol. 59, 742–748. doi: 10.1111/aje.12882
Banda, T., Mwangulango, N., Meyer, B., Schwartz, M. W., Mbago, F., Sungula, M., et al. (2008). The woodland vegetation of the Katavi-Rukwa ecosystem in western Tanzania. For. Ecol. Manage. 255, 3382–3395. doi: 10.1016/j.foreco.2008.01.079
Bowkett, A. E., Rovero, F., and Marshall, A. R. (2008). The use of camera-trap data to model habitat use by antelope species in the Udzungwa Mountain forests, Tanzania. Afr. J. Ecol. 46, 479–487. doi: 10.1111/j.1365-2028.2007.00881.x
Burnham, K. P., and Anderson, D. (2002). Model Selection and Multi-model Inference. New York: Springer.
Burnham, K. P., Anderson, D. R., and Huyvaert, K. P. (2011). AIC model selection and multimodel inference in behavioral ecology: some background, observations, and comparisons. Behav. Ecol. Sociobiol. 65, 23–35. doi: 10.1007/s00265-010-1029-6
Caro, T. M. (2002). Factors affecting the small mammal community inside and outside Katavi National Park, Tanzania. Biotropica 34, 310–318. doi: 10.1646/0006-3606(2002)034[0310:fatsmc]2.0.co;2
Caro, T. M. (1999). Densities of mammals in partially protected areas: the Katavi ecosystem of western 490, Tanzania. J. Appl. Ecol. 36, 205–217. doi: 10.1046/j.13652664.1999.00392.x
Caro, T. M. (2001). Species richness and abundance of small mammals inside and outside an African national park. Biol. Conserv. 98, 251–257. doi: 10.1016/s0006-3207(00)00105-1
Caro, T. M. (2003). Umbrella species: critique and lessons from East Africa. Anim. Conserv. 6, 171–181. doi: 10.1017/S1367943003003214
Cusack, J. J., Swanson, A., Coulson, T., Packer, C., Carbone, C., Dickman, A. J., et al. (2015). Applying a random encounter model to estimate lion density from camera traps in Serengeti National Park, Tanzania. J. Wildl. Manage. 79, 1014–1021. doi: 10.1002/jwmg.902
Davis, R. S., Stone, E. L., Gentle, L. K., Mgoola, W. O., Uzal, A., and Yarnell, R. W. (2021). Spatial partial identity model reveals low densities of leopard and spotted hyaena in a miombo woodland. J. Zool. 313, 43–53. doi: 10.1111/jzo.12838
Desanker, P. V., Frost, P. G. H., Justice, C. O., and Scholes, R. J. (1997). The Miombo Network: Framework for a Terrestrial Transect Study of Land-Use and Land-Cover Change in the Miomno Ecosystems of Central Africa IGBP Report 41. Stockholm, Sweden: International Geosphere-Biosphere Programme.
Dial, K. P., and Vaughn, T. A. (1987). Opportunistic predation on Alate termites in Kenya. Biotropica 19, 185–187. doi: 10.2307/2388744
Dickman, C. R. (1991). Mechanisms of competition among insectivorous mammals. Oecologia 85, 464–471. doi: 10.1007/BF00323757
Easter, T., Bouley, P., and Carter, N. (2019). Opportunities for biodiversity conservation outside of Gorongosa National Park, Mozambique: a multispecies approach. Biol. Conserv. 232, 217–227. doi: 10.1016/j.biocon.2019.02.007
Fiske, I., Chandler, R., Miller, D., Royle, A., Kery, M., Hostetler, J., et al. (2015). Package ‘unmarked’. Vienna, Austria: R Project for Statistical Computing.
Fitzherbert, E., Gardner, T., Caro, T., and Jenkins, P. (2007). Habitat preferences of small mammals in the Katavi ecosystem of western Tanzania. Afr. J. Ecol. 45, 249–257. doi: 10.1111/j.1365-2028.2006.00699.x
Fleming, P. A., and Loveridge, J. P. (2003). Miombo woodland termite mounds: resource islands for small vertebrates? J. Zool. 259, 161–168. doi: 10.1017/S0952836902003084
Frey, S., Fisher, J. T., Burton, A. C., and Volpe, J. P. (2017). Investigating animal activity patterns and temporal niche partitioning using camera-trap data: challenges and opportunities. Remote Sens. Ecol. Conserv. 3, 123–132. doi: 10.1002/rse2.60
Frost, P. (1996). “The Ecology of Miombo Woodlands,” in The Miombo in Transition: Woodlands and Welfare in Africa, ed. B. Campbell (Bogor, Indonesia: Centre for International Forestry Research), 11–57.
Gaynor, K. M., Daskin, J. H., Rich, L. N., and Brashares, J. S. (2021). Postwar wildlife recovery in an African savanna: evaluating patterns and drivers of species occupancy and richness. Anim. Conserv. 24, 510–522. doi: 10.1111/acv.12661
Godlee, J. L., Gonçalves, F. M., Tchamba, J. J., Chisingui, A. V., Muledi, J. I., Shutcha, M. N., et al. (2020). Diversity and structure of an arid woodland in Southwest Angola, with comparison to the wider miombo ecoregion. Diversity 12:140. doi: 10.3390/D12040140
Green, D. S., Roloff, G. J., Heath, B. R., and Holekamp, K. E. (2015). Temporal dynamics of the reponses by African mammals to prescribed fire. J. Wildl. Manage. 79, 235–242. doi: 10.1002/jwmg.827
Griffiths, A. D., Garnett, S. T., and Brook, B. W. (2015). Fire frequency matters more than fire size: testing the pyrodiversity-biodiversity paradigm for at-risk small mammals in an Australian tropical savanna. Biol. Conserv. 186, 337–346. doi: 10.1016/j.biocon.2015.03.021
Gumbo, D. J., Dumas-Johansen, M., Muir, G., Boesrstler, F., and Zuzhang, X. (2018). Sustainable management of Miombo woodlands: food security, nutrition and wood energy. Rome: FAO.
Happold, D. C. D., and Happold, M. (1991). An ecological study of small rodents in the thicket-clump savanna of Lengwe National Park, Malawi. J. Zool. 223, 527–547. doi: 10.1111/j.1469-7998.1991.tb04386.x
Harris, G. T., Thirgood, S., Hopcraft, J. G. C., Cromsight, J. P. M., and Berger, J. (2009). Global decline in aggregated migrations of large terrestrial mammals. Endanger. Species Res. 7, 55–76. doi: 10.3354/esr00173
Junker, J., Blake, S., Boesch, C., Campbell, G., du Toit, L., Duvall, C., et al. (2012). Recent decline in suitable environmental conditions for African great apes. Divers. Distrib. 18, 1077–1091. doi: 10.1111/ddi.12005
Kavanna, P. Y., Alexander, V., and Kakengi, M. (2014). Feed resources abundance for wild and domestic herbivores in Miombo woodlands of Western Tanzania. Res. Opin. Anim. Vet. Sci. 4, 150–156.
Keller, C., and Schradin, C. (2008). Plant and small mammal richness correlate positively in a biodiversity hotspot. Biodivers. Conserv. 17, 911–923. doi: 10.1007/s10531-008-9341-4
Kennerley, R. J., Lacher, T. E., Hudson, M. A., Long, B., McCay, S. D., Roach, N. S., et al. (2021). Global patterns of extinction risk and conservation needs for Rodentia and Eulipotyphla. Divers. Distrib. 27, 1792–1806. doi: 10.1111/ddi.13368
Kingdon, J. (2015). The Kingdon Field Guide to African Mammals, Second Edition, ed. London: Bloomsbury Publishing.
Kronfeld-Schor, N., and Dayan, T. (2003). Partitioning of time as an ecological resource. Annu. Rev. Ecol. Evol. Syst. 34, 153–181. doi: 10.1146/annurev.ecolsys.34.011802.132435
Kutner, M. H., Nachtsheim, C. J., and Neter, J. (2005). Applied Linear Regression Models, 5th Edn. New York: Irwin/McGraw-Hill.
Linzey, A. V., and Kesner, M. H. (1997). Small mammals of a woodland-savannah ecosystem in Zimbabwe. II. Community structure. J. Zool. 243, 153–162. doi: 10.1111/j.1469-7998.1997.tb05761.x
Longhurst, C., Johnson, R. A., and Wood, T. G. (1978). Predation by Megaponera foetens (Fabr.) (Hymenoptera: Formicidae) on termites in the Nigerian southern Guinea Savanna. Oecologia 32, 101–107. doi: 10.1007/BF00344694
Loveridge, J. P., and Moe, S. R. (2004). Termitaria as browsing hotspots for African megaherbivores in miombo woodland. J. Trop. Ecol. 20, 337–343. doi: 10.1017/S0266467403001202
Magnusson, A., Skaug, H., Nielsen, A., Berg, C., Kristensen, K., Maechler, M., et al. (2017). Package ‘glmmTMB’. Vienna, Austria: R Development Core Team.
Mayengo, G., Piel, A. K., and Treydte, A. C. (2020). The importance of nutrient hotspots for grazing ungulates in a Miombo ecosystem, Tanzania. PLoS One 15:e0230192. doi: 10.1371/journal.pone.0230192
Meredith, M., and Ridout, M. (2014). Overview of the overlap package. Vienna, Austria: R Development Core Team.
Monterroso, P., Alves, P. C., and Ferreras, P. (2014). Plasticity in circadian activity patterns of mesocarnivores in Southwestern Europe: implications for species coexistence. Behav. Ecol. Sociobiol. 68, 1403–1417. doi: 10.1007/s00265-014-1748-1
Montfort, F., Nourtier, M., Grinand, C., Maneau, S., Mercier, C., Roelens, J. B., et al. (2021). Regeneration capacities of woody species biodiversity and soil properties in Miombo woodland after slash-and-burn agriculture in Mozambique. For. Ecol. Manage. 488:119039. doi: 10.1016/j.foreco.2021.119039
Mori, E., Bagnato, S., Serroni, P., Sangiuliano, A., Rotondaro, F., Marchianò, V., et al. (2020). Spatiotemporal mechanisms of coexistence in an European mammal community in a protected area of southern Italy. J. Zool. 310, 232–245. doi: 10.1111/jzo.12743
Mori, E., Paniccia, C., Munkhtsog, B., Cicero, M., and Augugliaro, C. (2021). Temporal overlap among small-and medium-sized mammals in a grassland and a forest–alpine meadow of Central Asia. Mamm. Biol. 101, 153–162. doi: 10.1007/s42991-020-00085-z
Munishi, P. K. T., Temu, R. P. C., and Soka, G. (2011). Plant communities and tree species associations in a Miombo ecosystem in the Lake Rukwa basin, Southern Tanzania : implications for conservation. J. Ecol. Nat. Environ. 3, 63–71.
Muvengwi, J., Chisango, T., Mpakairi, K., Mbiba, M., and Witkowski, E. T. F. (2020). Structure, composition and regeneration of miombo woodlands within harvested and unharvested areas. For. Ecol. Manage. 458:117792. doi: 10.1016/j.foreco.2019.117792
Naiman, R. J., Fetherston, K. L., McKay, S. J., and Chen, J. (2010). “Riparian forests,” in Ecology and Management of Streams and Rivers in the Pacific Northwest Coastal Ecoregion, eds R. J. Naiman and R. E. Bilby (Berlin: Springer-Verlag), 265–299. doi: 10.1017/cbo9780511613029.010
Nkwabi, A. K., John, B., Kija, H., Otsyina, R. M., Monjare, J. F., and Kajuni, A. R. (2018). Abundance and distribution of small mammals relative to human activities in the wildlife management areas of Ruvuma landscape, southern Tanzania. Int. J. Fauna Biol. Stud. 5, 156–162.
Ortmann, C. R., and Johnson, S. D. (2021). How reliable are motion-triggered camera traps for detecting small mammals and birds in ecological studies? J. Zool. 313, 202–207. doi: 10.1111/jzo.12849
Palmer, M. S., Swanson, A., Kosmala, M., Arnold, T., and Packer, C. (2018). Evaluating relative abundance indices for terrestrial herbivores from large-scale camera trap surveys. Afr. J. Ecol. 56, 791–803. doi: 10.1111/aje.12566
Pettorelli, N., Lobora, A. L., Msuha, M. J., Foley, C., and Durant, S. M. (2010). Carnivore biodiversity in Tanzania: revealing the distribution patterns of secretive mammals using camera traps. Anim. Conserv. 13, 131–139. doi: 10.1111/j.1469-1795.2009.00309.x
Phillips, S., Scheffrahn, R. H., Piel, A., Id, F. S., Agbor, A., Brazzola, G., et al. (2021). Limited evidence of C 4 plant consumption in mound building Macrotermes termites from savanna woodland chimpanzee sites. PLoS One 16:e0244685. doi: 10.1371/journal.pone.0244685
Piel, A. K. (2018). Temporal patterns of chimpanzee loud calls in the Issa Valley, Tanzania: evidence of nocturnal acoustic behavior in wild chimpanzees. Am. J. Phys. Anthropol. 166, 530–540. doi: 10.1002/ajpa.23609
Piel, A. K., Bonnin, N., RamirezAmaya, S., Wondra, E., and Stewart, F. A. (2019). Chimpanzees and their mammalian sympatriates in the Issa Valley, Tanzania. Afr. J. Ecol. 57, 31–40. doi: 10.1111/aje.12570
Piel, A. K., Cohen, N., Kamenya, S., Ndimuligo, S. A., Pintea, L., and Stewart, F. A. (2015). Population status of chimpanzees in the Masito-Ugalla Ecosystem, Tanzania. Am. J. Primatol. 77, 1027–1035. doi: 10.1002/ajp.22438
Price, B., Kutt, A. S., and McAlpine, C. A. (2010). The importance of fine-scale savanna heterogeneity for reptiles and small mammals. Biol. Conserv. 143, 2504–2513. doi: 10.1016/j.biocon.2010.06.017
Prugh, L. R., and Golden, C. D. (2014). Does moonlight increase predation risk? Meta-analysis reveals divergent responses of nocturnal mammals to lunar cycles. J. Anim. Ecol. 83, 504–514. doi: 10.1111/1365-2656.12148
R Core Team (2020). R: A Language And Environment For Statistical Computing. Vienna, Austria: R Foundation for Statistical Computing.
Redford, H., and Dorea, J. G. (1984). The nutritional value of invertebrates with emphasis on ants and termites as food for mammals. J. Zool. 204, 385–395. doi: 10.1111/j.1469-7998.1984.tb02339.x
Redford, K. H. (1987). “Ants and Termites As Food,” in Current Mammalogy, ed. H. H. Genoways (Boston, MA: Springer US), 349–399. doi: 10.1007/978-1-4757-9909-5_9
Roberts, P. D., Somers, M. J., White, R. M., and Nel, J. A. (2007). Diet of the South African large-spotted genet Genetta tigrina (Carnivora, Viverridae) in a coastal dune forest. Acta Theriol. 52, 45–53. doi: 10.1007/BF03194198
Rovero, F., and Marshall, A. R. (2009). Camera trapping photographic rate as an index of density in forest ungulates. J. Appl. Ecol. 46, 1011–1017. doi: 10.1111/j.1365-2664.2009.01705.x
Rovero, F., Martin, E., Rosa, M., Ahumada, J. A., and Spitale, D. (2014). Estimating species richness and modelling habitat preferences of tropical forest mammals from camera trap data. PLoS One 9:e103300. doi: 10.1371/journal.pone.0103300
Rovero, F., Owen, N., Jones, T., Canteri, E., Iemma, A., and Tattoni, C. (2017). Camera trapping surveys of forest mammal communities in the Eastern Arc Mountains reveal generalized habitat and human disturbance responses. Biodivers. Conserv. 26, 1103–1119. doi: 10.1007/s10531-016-1288-2
Rovero, F., Tobler, M., and Sanderson, J. (2010). “Camera trapping for inventorying terrestrial vertebrates,” in Manual on field recording techniques and protocols for all taxa biodiversity inventories and monitoring., eds J. Eymann, J. Degreef, C. Häuser, J. C. Monje, Y. Samyn, and D. VandenSpiegel (Belgium: Belgian Development Cooperation).
Rowcliffe, J. M., and Carbone, C. (2008). Surveys using camera traps: are we looking to a brighter future? Anim. Conserv. 11, 185–186. doi: 10.1111/j.1469-1795.2008.00180.x
Rowcliffe, J. M., Kays, R., Kranstauber, B., Carbone, C., and Jansen, P. A. (2014). Quantifying levels of animal activity using camera trap data. Methods Ecol. Evol. 5, 1170–1179. doi: 10.1111/2041-210x.12278
Swihart, R. K., Slade, N. A., and Bergstrom, B. J. (1988). Relating body size to the rate of home range use in mammals. Ecology 69, 393–399. doi: 10.2307/1940437
Taylor, K. D., and Green, M. G. (1976). The influence of rainfall on diet and reproduction in four African rodent species. J. Zool. 180, 367–389. doi: 10.1016/S0140-6736(01)63523-2
Timberlake, J., and Chidumayo, E. (2011). Miombo Ecoregion Vision Report 2001. Biodiversity Foundation for Africa: Occasional Publications Number 20. Zimbabwe: Biodiversity Foundation for Africa.
Timberlake, J., Chidumayo, E., and Sawadogo, L. (2010). “Distribution and characteristics of African dry forests and woodlands,” in The dry forest and woodlands of Africa: Managing for products and services, eds E. Chidumayo and D. J. Gumbo (London: Earthscan), 11–42.
Valeix, M., Chamaillé-Jammes, S., and Fritz, H. (2007). Interference competition and temporal niche shifts: elephants and herbivore communities at waterholes. Oecologia 153, 739–748. doi: 10.1007/s00442-007-0764-5
Wagenmakers, E. J., and Farrell, S. (2004). AIC model selection using Akaike weights. Psychon. Bull. Rev. 11, 192–196. doi: 10.3758/BF03206482
Waltert, M., Meyer, B., and Kiffner, C. (2009). Habitat availability, hunting or poaching: what affects distribution and density of large mammals in western Tanzanian woodlands? Afr. J. Ecol. 47, 737–746. doi: 10.1111/j.1365-2028.2009.01080.x
Keywords: small mammals, miombo, habitat use, termite mounds, riparian forests, woodlands, meso-mammals
Citation: D’Ammando G, Caro T, Oelze VM, Phillips S, Sime P, Stewart FA and Piel AK (2022) Ecological Drivers of Habitat Use by Meso Mammals in a Miombo Ecosystem in the Issa Valley, Tanzania. Front. Ecol. Evol. 10:773568. doi: 10.3389/fevo.2022.773568
Received: 10 September 2021; Accepted: 17 February 2022;
Published: 26 April 2022.
Edited by:
Bradley J. Bergstrom, Valdosta State University, United StatesReviewed by:
Adam Ferguson, Field Museum of Natural History, United StatesCopyright © 2022 D’Ammando, Caro, Oelze, Phillips, Sime, Stewart and Piel. This is an open-access article distributed under the terms of the Creative Commons Attribution License (CC BY). The use, distribution or reproduction in other forums is permitted, provided the original author(s) and the copyright owner(s) are credited and that the original publication in this journal is cited, in accordance with accepted academic practice. No use, distribution or reproduction is permitted which does not comply with these terms.
*Correspondence: Alexander K. Piel, YS5waWVsQHVjbC5hYy51aw==
Disclaimer: All claims expressed in this article are solely those of the authors and do not necessarily represent those of their affiliated organizations, or those of the publisher, the editors and the reviewers. Any product that may be evaluated in this article or claim that may be made by its manufacturer is not guaranteed or endorsed by the publisher.
Research integrity at Frontiers
Learn more about the work of our research integrity team to safeguard the quality of each article we publish.