- 1Faculty of Science, University of South Bohemia, České Budějovice, Czechia
- 2Department of Botany and Plant Pathology, Purdue University, West Lafayette, IN, United States
- 3Biology Centre of the Czech Academy of Sciences, Institute of Entomology, České Budějovice, Czechia
- 4Department of Ecology of Tropical Agricultural Systems, University of Hohenheim, Stuttgart, Germany
- 5Institute of Biological Sciences, Cardinal Stefan Wyszyński University, Warsaw, Poland
- 6CABI, Delémont, Switzerland
- 7Institute of Evolutionary Biology, Faculty of Biology, University of Warsaw, Warsaw, Poland
- 8Botanic Garden, Faculty of Biology, University of Warsaw, Warsaw, Poland
- 9Department of Ecology, Faculty of Environmental Sciences, Czech University of Life Sciences Prague, Prague, Czechia
- 10Public Health Institute of the Istrian Region, Pula, Croatia
- 11Department of Molecular Biotechnology and Microbiology, University of Debrecen, Debrecen, Hungary
- 12Institute of Forest Ecology, Slovak Academy of Sciences, Zvolen, Slovakia
Hesperomyces virescens (Ascomycota, Laboulbeniales), a fungal ectoparasite, is thus far reported on Harmonia axyridis from five continents: North and South America, Europe, Africa, and Asia. While it is known that He. virescens can cause mortality of Ha. axyridis under laboratory conditions, the role of biotic and abiotic factors in influencing the distribution of He. virescens in the field is unknown. We collected and screened 3,568 adult Ha. axyridis from 23 locations in seven countries in Central Europe between October and November 2018 to test the effect of selected host characters and climate and landscape variables on the infection probability with He. virescens. Mean parasite prevalence of He. virescens on Ha. axyridis was 17.9%, ranging among samples from 0 to 46.4%. Host sex, climate, and landscape composition did not have any significant effect on the infection probability of He. virescens on Ha. axyridis. Two color forms, f. conspicua and f. spectabilis, had a significantly lower parasite prevalence compared to the common Ha. axyridis f. novemdecimsignata.
Introduction
Parasites may be the least studied life form on the planet (Price, 1980; Windsor, 1990, 1995). In their call for a “global parasite conservation plan,” Carlson et al. (2020) proposed 12 major goals within four themes. These themes are data collection and synthesis (aimed at describing parasites and incorporating them into biodiversity surveys, among others), risk assessment and prioritization (documenting drivers of parasite declines and develop regional and global Red Lists), conservation practice (e.g., building parasite conservation capacity), and outreach and education. One of the most common parasites of the globally invasive harlequin ladybird Harmonia axyridis (Coleoptera, Coccinellidae) is Hesperomyces virescens, which was for the first time observed on this host in 2002 (Garcés and Williams, 2004).
Hesperomyces virescens (Ascomycota, Laboulbeniales) is a biotrophic fungus that has a very wide distribution with confirmed reports in North and South America, Europe, Africa, and Asia (Haelewaters et al., 2017). Based on the results of an integrative taxonomic approach, we know that He. virescens is a complex of multiple species segregated by hosts (Haelewaters et al., 2018a). Thus far, two species have been formally described in the complex, He. halyziae (Haelewaters and De Kesel, 2020) and He. parexochomi (Crous et al., 2021). The other species, including the one associated with Ha. axyridis, are awaiting formal description. The parasite prevalence of He. virescens on Ha. axyridis differs among geographic regions and, exceptionally, may be as high as 96.5% in a given ladybird population, as reported in Meise, Belgium (February 2012, n = 107) and in Westmoreland, New Hampshire, United States (December 2012; n = 83) (Haelewaters et al., 2017). As a result, He. virescens has recently gained traction among entomologists as a potential biological control agent against Ha. axyridis.
Little information is available about the ecology of He. virescens. A recent experimental study demonstrated that infection with He. virescens affects the survival of Ha. axyridis on its own and when ladybirds are co-infected with either of two entomopathogenic fungi, Beauveria bassiana and Metarhizium brunneum (Haelewaters et al., 2020). Thus far, however, potential effects of biotic and abiotic factors on the distribution of He. virescens and its parasite prevalence on Ha. axyridis are unknown. This information is fundamental for studies in conservation, applied ecology, and biocontrol strategies (Ferrier, 2002; Rushton et al., 2004; Magan, 2021). In this study, we collected adult specimens of Ha. axyridis across Central Europe and evaluated how selected host traits and climate and landscape variables affect infection patterns with He. virescens. Variables tested included host sex, host color form, color of elytra, proportion of agricultural and forested areas (European Environment Agency, 2020), temperature, and precipitation (Fick and Hijmans, 2017).
Materials and Methods
Ladybirds were collected either by hand or using a mouth-operated aspirator from October to November 2018 in different Central European countries (Figure 1): Croatia (Istria County), the Czech Republic (Central Bohemian Region, South Bohemian Region, Plzeň Region), Germany (State of Bavaria), Hungary (Hajdú-Bihar County), Poland (Mazovian Voivodeship), Slovakia (Nitra Region), and Switzerland (Canton of Jura). Contributors were asked to collect at least 100 specimens from each ladybird population. Geographic coordinates were recorded and can be found in Supplementary File 1. Specimens were preserved in 70% ethanol until examination in the laboratory.
Ladybirds were screened under 40–50× magnification for the presence of non-hyphal thalli of He. virescens (sensu De Kesel, 2011; Haelewaters et al., 2018a). For each ladybird, the following traits were recorded: sex (see McCornack et al., 2007); color form [non-melanic f. novemdecimsignata (also referred to as succinea), and melanic f. conspicua, f. axyridis, and f. spectabilis]; color of elytra (for non-melanics) or spots (for melanics) (yellow, orange, red; Fiedler and Nedvěd, 2019). For novemdecimsignata specimens, we described whether spots were well-circumscribed (0), missing or fewer in number than typically present (−), or large and touching each other (+) (Fiedler and Nedvěd, 2019). For each population, no matter the number of sampled ladybirds, we screened 100 randomly selected specimens. When available, we screened and processed additional specimens of the melanic forms to avoid statistical restrictions due to these forming in low percentages. Screening results for all processed ladybirds are available in Supplementary File 1. After processing, voucher specimens were deposited in the Purdue Entomology Research Collection (West Lafayette, IN, United States) under the following accession numbers: PERC 0147670–0147680.
All statistical analyses were performed using the R software, version 3.6.3 (R Core Team, 2020). To identify the variables influencing the infection probability of He. virescens on Ha. axyridis, we used generalized mixed effect models (GMEM) with a binomial data distribution (infected yes/no) [function glmer(), R package lme4; Bates et al., 2015]. We included host sex, host color form, color of elytra, the proportion of agricultural, and forested areas in a buffer surrounding each sampling location (100 m, 300 m, 600 m, 1 km, and 2.5 km), and climate variables. Urban area was excluded from the analysis because it was highly collinear with the other predictor variables. For each buffer radius, a separate model was calculated, resulting in five distinct models. The landscape variables were obtained by extracting the landscape composition of Copernicus Corine Land Cover images taken in 2018 (European Environment Agency, 2020) with the help of R package raster, using the function extract (Hijmans et al., 2020). We pooled values in the categories “broad-leaved forest,” “coniferous forest,” and “mixed forest” into the forested area variable, whereas the agricultural area variable consisted of values for the categories “non-irrigated arable land,” “fruit trees and berry plantations,” “pastures,” “complex cultivation patterns,” and “land principally occupied by agriculture, with significant areas of natural vegetation” (Supplementary File 2). We further calculated three models using a “ring buffer” or annulus (sensu Rey et al., 2020), one for each of the following radius combinations: 100 m inner and 300 m outer radius, 300 m inner and 600 m outer radius, and 600 m inner and 1 km outer radius.
Climate variables were extracted from WorldClim with a resolution of 30 arc seconds (ca. 1 × 1 km) (Fick and Hijmans, 2017) (Supplementary File 2). As variables for temperature and humidity are generally collinear, we decided to summarize the following variables using a principal component analysis (PCA) [prcomp(), R package stats; R Core Team, 2020]: annual mean temperature, mean maximum temperature in the hottest month, mean minimum temperature in the coldest month, annual precipitation, precipitation during the wettest month, and precipitation during the driest month. We extracted the values for the first two dimensions for all sampling sites (Supplementary File 2). Statistical processing revealed two principal dimensions that explained 64 and 30.1% of the climatic variation, respectively. Finally, we included the random intercept collection nested within sampling region to address repeated sampling at the same location and also spatial autocorrelation as suggested by Zuur et al. (2010).
Hypothesis testing was done using likelihood ratio tests, with p values calculated based on χ2 distributions, declaring an effect significant when p ≤ 0.05. Nine models were compared, namely, the Null Model and the model with variables of interest within the different buffer radii (100 m, 300 m, 600 m, 1 km, and 2.5 km) and annulus radii (100–300 m, 300–600 m, and 600 m–1 km). Model selection happened using the Akaike Information Criterion (Akaike, 1974). For all models, we calculated pseudo-R2 values to estimate model fit by accounting for the variation explained by both fixed and random effects [function r2(), R package performance; Lüdecke et al., 2020].
Results
We screened a total of 3,568 ladybirds, resulting in a mean infection prevalence of 17.9%, ranging from 0 to 46.4% among sampled populations (Figure 1). The population with the highest prevalence of He. virescens was from Levice in southwestern Slovakia. Two populations showed no visible signs of He. virescens infection, both of which were from Poland. Forty-seven individuals were excluded from statistical analyses, including a single f. intermedia specimen from Warsaw, Poland and 46 specimens with missing information on elytral color. Likelihood ratio tests confirmed that each of the eight models explained the observed variance better than chance (Table 1), while the conditional pseudo R2-values estimated model fit at around 0.33, indicating good fit (Table 1). We only found variables on host individual characters to have significant effects on the parasite prevalence of He. virescens on Ha. axyridis (Tables 2, 3). The significant effect of color form was consistent in all five models, with the color forms f. conspicua and f. spectabilis being less often infected compared to the common form f. novemdecimsignata(0). There was a trend for f. novemdecimsignata(-) to be less likely infected by He. virescens compared to the common form f. novemdecimsignata(0). This trend was consistent over all candidate models but not significant. Finally, the color of elytra had a significant effect on the infection probability, with individuals with red elytra being more likely and individuals with yellow elytra less likely infected compared to individuals with orange elytra. Host sex, climate, and habitat composition resulted in not having any significant effect on the infection probability of He. virescens on Ha. axyridis.
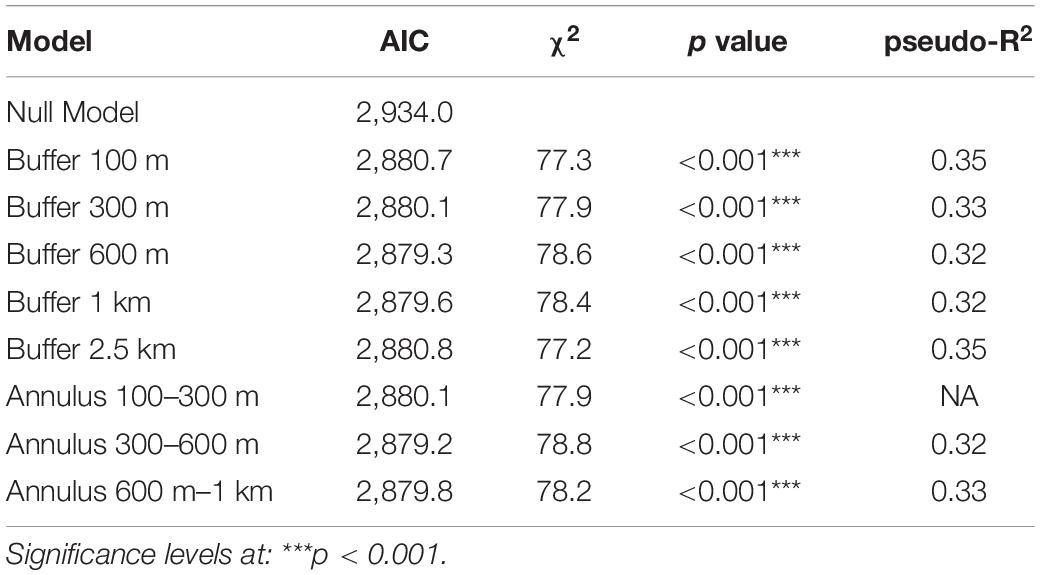
Table 1. Results of model (dependency of Hesperomyces virescens prevalence on Harmonia axyridis on coloration and climate and habitat properties) evaluation showing candidate models being significantly better than the null model and estimated pseudo-R2 values indicating model fit.
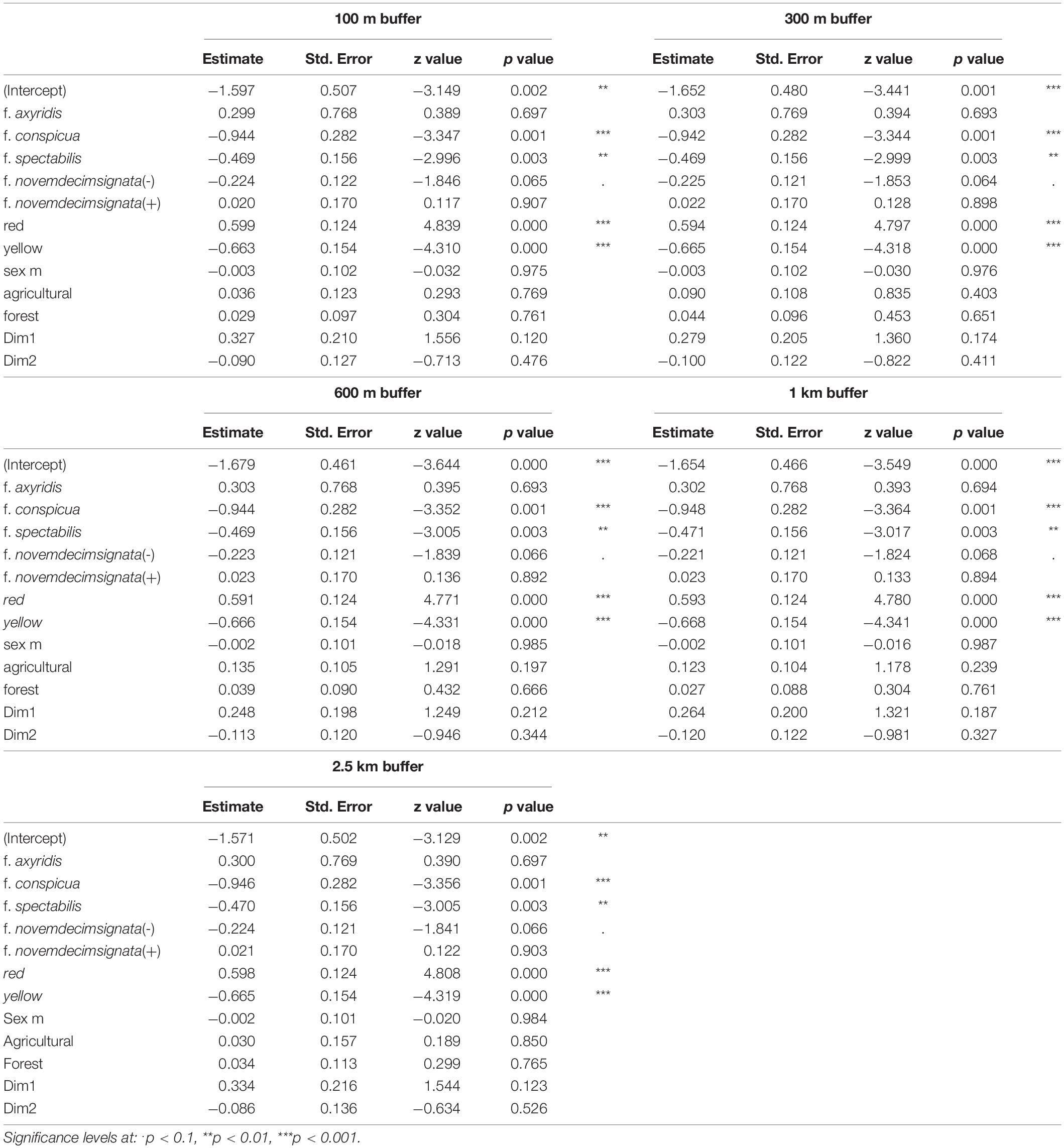
Table 2. Obtained parameters of each candidate model addressing the prevalence of infection by Hesperomyces virescens for different buffer radii (100 m, 300 m, 600 m, 1 km, 2.5 km).
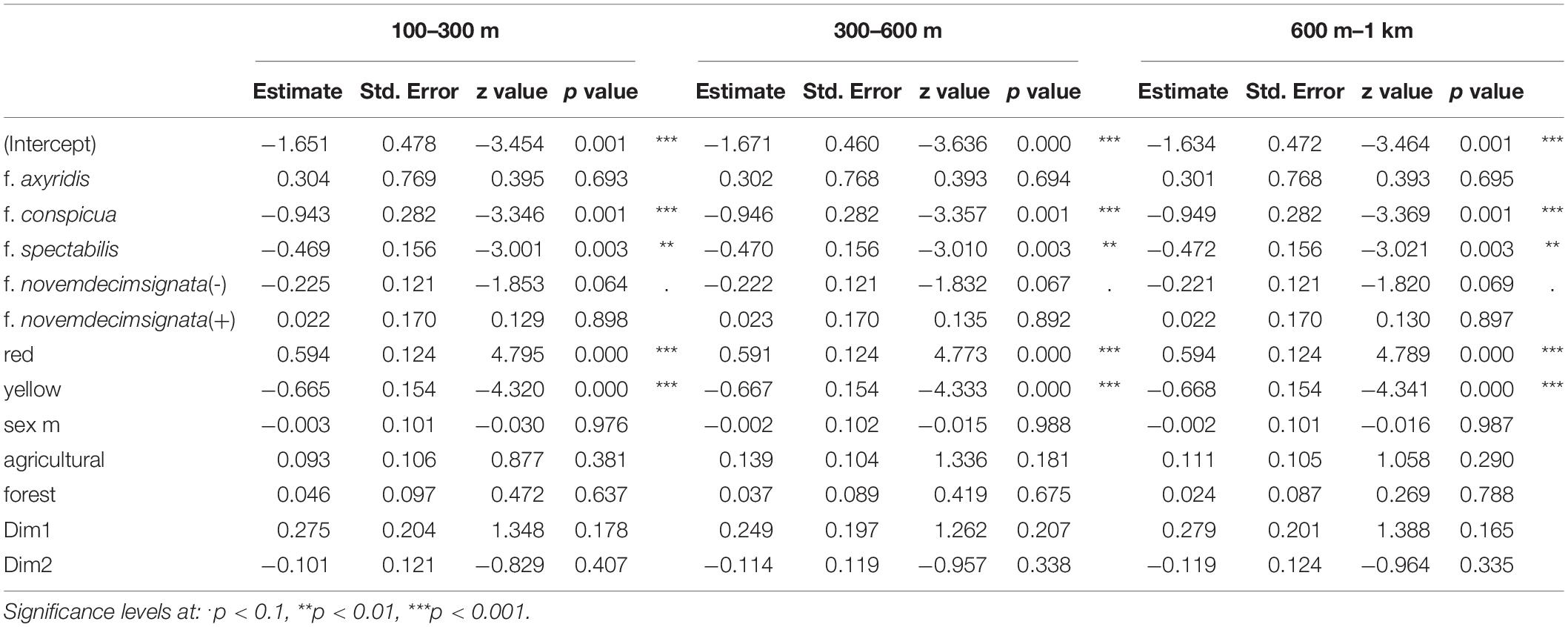
Table 3. Obtained parameters of each candidate model addressing the prevalence of infection by Hesperomyces virescens for different annulus radii (100–300 m, 300–600 m, 600 m–1 km).
Discussion
Ha. axyridis–He. virescens
The first published record of He. virescens on Ha. axyridis in Europe was made in the winter of 2006–2007, from Meise in Belgium (De Kesel, 2011). Other country records followed quickly, from the Netherlands (2008), Germany (2008–2009), Croatia and the Czech Republic (2013), Hungary and Poland (2014), Slovakia (2015), Bulgaria and Greece (2017), and most recently European Russia and Switzerland (2018) (Herz and Kleespies, 2012; Ceryngier and Twardowska, 2013; Ceryngier et al., 2013; Pfliegler, 2014; Gorczak et al., 2016; Ceryngier and Romanowski, 2017; Haelewaters et al., 2017; van Wielink, 2017; Orlova-Bienkowskaja et al., 2018; this paper). Many of these papers show that the parasite prevalence of He. virescens differs significantly over time and in space (Raak-van den Berg et al., 2014; Haelewaters et al., 2017). Differences in Laboulbeniales prevalence among locations have been attributed to host population density and habitat type (Scheloske, 1969; De Kesel, 1996), but to date, no data were thus far available with regard to the He. virescens–Ha. axyridis association.
Effect of Biotic Factors
A remarkable finding from our study is that the melanic color forms f. conspicua and f. spectabilis were less often infected with He. virescens compared to the common nineteen-spotted f. novemdecimsignata(0). Two other studies investigated the relationship between the degree of melanization and infection patterns. Haelewaters et al. (2018b) observed a slight trend to higher intensity of parasitism in more melanic males of Ha. axyridis f. novemdecimsignata. Fiedler and Nedvěd (2019) found (i) a positive association between putative age groups of Ha. axyridis estimated as carotenoid content and infection with He. virescens and (ii) a negative association between elytral melanization of Ha. axyridis f. novemdecimsignata specimens and infection. The latter was explained by the fact that younger ladybirds emerged later in the year, with lower temperatures inducing extensive melanization; since they were younger, they had less opportunities to be parasitized by He. virescens. Our results are in line with the findings of Fiedler and Nedvěd (2019), but a definitive answer as to how elytral melanization affects the susceptibility to infection with He. virescens remains unclear. The black coloration of melanic forms of Ha. axyridis is negatively correlated with the total content of alkaloids (Bezzerides et al., 2007), which serve as defense against predators and pathogens (Röhrich et al., 2011). Then, heavier melanization should result in more infection with He. virescens, but our results are inconsistent with this hypothesis. Future work—performing bioassays in controlled settings and analyzing expression levels of immune genes—is needed to shed light on the susceptibility of different color morphs to He. virescens.
The results of our modeling approach show a significant correlation between elytral color and the infection probability of Ha. axyridis with He. virescens. Carotenoid accumulation, and thus red color intensity, is a function of ladybird age (Bezzerides et al., 2007; Nedvěd et al., 2019). In our study, older individuals of Ha. axyridis accumulated more He. virescens inoculum. Similar observations were made by Fiedler and Nedvěd (2019), particularly that individuals with red elytra are more likely to be infected. Hesperomyces virescens transmits among ladybirds through physical contacts (during mating and in overwintering aggregations), but auto-transmission by grooming or cleaning also occurs. These factors contribute to parasite prevalence (at the level of population) and thallus density (at the individual level) being positively correlated with host age (Riddick and Schaefer, 2005; Nalepa and Weir, 2007; Haelewaters et al., 2017) and thus, incidentally, with elytral color.
Effect of Abiotic Factors
Mean parasite prevalence did not significantly change according to any of the temperature variables tested [Kruskal–Wallis test, function kruskal.test(), R package stats; R Core Team, 2020], but our sampling scheme was somewhat limited, with only localities in Central European countries. For a broader understanding of the associations between He. virescens and Ha. axyridis within Europe, we recommend incorporation of data from northern and southern European countries in order to compile a dataset with a larger range in temperature. Collections also need to be expanded to city centers (e.g., in parks, community gardens, edges of playgrounds). A negative correlation was found between parasite prevalence of He. virescens sensu lato on Adalia bipunctata and distance from the city center of London (Welch et al., 2001). Prevalence in central London was as high as 40% (n = 105), whereas it was 0% outside of the urban area at a distance of 25 km. This could be linked to increased temperatures in urban environments (urban heat island effect), but this has not yet been tested. Adriaens et al. (2008) highlighted the idea that Ha. axyridis is less frequently found in natural landscapes compared to more urbanized and anthropogenic landscapes. Habitat preference may also be an important factor in the parasitism with He. virescens. Factors promoting Ha. axyridis may indirectly promote He. virescens. Particularly, a higher dominance of Ha. axyridis in a given ladybird community will be beneficial for ascospore transmission among individuals. The number of generations of Ha. axyridis in Central Europe varies from two to three and is probably dependent on habitat summer temperatures and prey availability. Population densities can increase due to the use of various prey patches enabled by high mobility in combination with the weak tendency for diapause extending the breeding period (Honek et al., 2018). In urbanized areas, the breeding season of Ha. axyridis is advanced by 2–3 weeks (Honek et al., 2021).
In addition to our buffer radius models, which are often used for forest management on a landscape scale (Brouwers et al., 2010), we also employed annulus radii to better model the behavior of Ha. axyridis in autumn. Our sampling localities are not where ladybirds became infected with He. virescens. As a result, the habitat variables might not be accurate for the habitats that the ladybirds occupied when they became infected with the fungus. Generally, we estimate that Ha. axyridis ladybirds fly 500 m during autumn migration. Detailed observations of ladybirds in České Budějovice, Czech Republic allowed the exact measurement of migration distance from before flight and after flight, which was 200–500 m toward the north (O. Nedvěd, unpublished). The longest flights measured were around 1,800 m in laboratory flight mills (Růžička, 1984). When Nalepa et al. (2005) conducted their experiments to test the role of visual contrast in autumn behavior of Ha. axyridis in 4 ha of open pasture, the required flight was about 200 m (Nalepa et al., 2005). In open fields in Japan, the median flight distance was around 400 m (Seko et al., 2008). We tested three different annulus radii (100–300 m, 300–600 m, and 600 m–1 km), but the results of our candidate models were highly similar to the buffer radius models. Aggregations of ladybirds are often formed on walls of building oriented toward the south or west (Kidd et al., 1995; Raak-van den Berg et al., 2012; Haelewaters et al., 2018b). As a result, we suggest that the annulus modeling approach could be made even more specific by obtaining landscape variables for an annulus section (or ring pie chart) facing the direction where ladybirds likely migrated from. This is methodologically complex and out of the scope of this paper, but is a consideration for future studies.
Community ecology research of Laboulbeniales is still in its infancy, with thus far only two published studies. Szentiványi et al. (2019) investigated whether climatic variables (temperature, humidity) influenced the distribution of ant- and bat fly-associated Laboulbeniales. They found that both the presence and prevalence of Laboulbeniales on their hosts were positively associated with low annual mean temperature and humidity. In addition, based on the study of more than 9,374 workers of the invasive ant Lasius neglectus in 66 colonies, Gippet et al. (2021) found that the presence of Laboulbenia formicarum on the ants was positively linked to warmer and dryer conditions at lower elevations. These are seemingly contrary results, and our data render drawing general conclusions for these microfungi even more complex. However, the direction of the effects of some of these variables may be species-specific, as suggested by Dumolein (2021). One could make the case that the combined analysis of presence/absence data in Szentiványi et al. (2019) may obscure true interactions, and thus that separate analyses would give a more accurate picture of how bioclimatic variables affect the distribution of the two assessed study systems—the bat fly-associated Arthrorhynchus spp. vs. the ant-associated Rickia wasmannii. We note that the studies of Szentiványi et al. (2019) and Gippet et al. (2021) used outside climatic data as obtained from MERRAclim, but many of these ant–Laboulbeniales and bat fly–Laboulbeniales interactions and the host dynamics resulting in fungal transmission take place in ant nests and bat roosts, respectively. Ant nest and bat roosting environments are characterized by their own microclimatic conditions, which likely play a role in shaping the distribution of these species of Laboulbeniales. Efforts should be redirected at collecting temperature and relative humidity data (e.g., through automated readers) within these environments to test for the effect of these microclimate-specific abiotic traits on parasitism with Laboulbeniales.
Our results are the first for the Ha. axyridis–He. virescens study system based on specimens collected during autumn migration. Understanding the factors influencing the infection of Laboulbeniales on invasive ladybirds—including climatic and landscape variables as well as seasonality and host behavior (e.g., Raak-van den Berg et al., 2014; Haelewaters et al., 2015, 2017; Markó et al., 2016)—will help understand their global spread as they cross many different ecosystems and environmental conditions. Resolving this question will also inform potential biocontrol strategies because it will inform us under which conditions He. virescens may (or may not) thrive. The collection of standardized multi-year, multi-site field data will help in this regard in addition to controlled laboratory experiments.
Data Availability Statement
The original data from this study are included in the article/Supplementary Material, further inquiries can be directed to the corresponding author.
Author Contributions
DH and ON designed the study. DH, TH, PC, RE, MG, KK, MK, NL, WPP, PZ, and ON collected the data. DH, TH, PC, and MH performed data analysis. DH, MCA, and ON acquired funding. DH and TH drafted the manuscript. DH revised the manuscript. All authors edited and approved the final version of the manuscript.
Funding
This work was supported by a Junior Postdoctoral Fellowship from the Research Foundation–Flanders (1206620N to DH); the Polish Ministry of Science and Higher Education (grant no. DI2014012344 to MG); funds from the project Improvement in Quality of the Internal Grant Scheme at CZU, CZ.02.2.69/0.0/0.0/19_073/0016944 (students grant 71/2021); the Scientific Grant Agency of the Ministry of Education, Science, Research and Sport of the Slovak Republic and the Slovak Academy of Sciences (VEGA, grant no. 2/0032/19 to PZ); a USDA National Institute of Food and Agriculture Hatch project (1010662 to MCA), and the Czech Science Foundation (grant no. 20-10003S to ON). RE was supported by CABI with core financial support from its member countries (and lead agencies) including the United Kingdom (Foreign, Commonwealth and Development Office), China (Chinese Ministry of Agriculture and Rural Affairs), Australia (Australian Centre for International Agricultural Research), Canada (Agricultural and Agri-Food Canada), Netherlands (Directorate-General for International Cooperation), and Switzerland (Swizz Agency for Development and Cooperation).
Conflict of Interest
The authors declare that the research was conducted in the absence of any commercial or financial relationships that could be construed as a potential conflict of interest.
Publisher’s Note
All claims expressed in this article are solely those of the authors and do not necessarily represent those of their affiliated organizations, or those of the publisher, the editors and the reviewers. Any product that may be evaluated in this article, or claim that may be made by its manufacturer, is not guaranteed or endorsed by the publisher.
Acknowledgments
We thank Aaron D. Smith (Purdue Entomological Research Collection) for curatorial support, Thomas E. Martin (Operation Wallacea) for textual edits, Michal Řeřicha (Czech University of Life Sciences Prague) for help with the processing of Czech specimens, and two reviewers for critical feedback that considerably improved the manuscript.
Supplementary Material
The Supplementary Material for this article can be found online at: https://www.frontiersin.org/articles/10.3389/fevo.2022.773423/full#supplementary-material
References
Adriaens, T., San Martin y Gomez, G., and Maes, D. (2008). Invasion history, habitat preferences and phenology of the invasive ladybird Harmonia axyridis in Belgium. Biocontrol 53, 69–88. doi: 10.1007/s10526-007-9137-6
Akaike, H. (1974). New look at statistical-model identification. IEEE Trans. Autom. Control 19, 716–723. doi: 10.1109/TAC.1974.1100705
Bates, D., Maechler, M., Bolker, B., and Walker, S. (2015). Fitting linear mixed-effects models using lme4. J. Stat. Softw. 67, 1–48. doi: 10.18637/jss.v067.i01
Bezzerides, A. L., McGraw, K. J., Parker, R. S., and Husseini, J. (2007). Elytra color as a signal of chemical defense in the Asian ladybird beetle Harmonia axyridis. Behav. Ecol. Sociobiol. 61, 1401–1408. doi: 10.1007/s00265-007-0371-9
Brouwers, N. C., Newton, A. C., Watts, K., and Bailey, S. (2010). Evaluation of buffer-radius modelling approaches used in forest conservation and planning. For. Int. J. For. Res. 83, 409–421. doi: 10.1093/forestry/cpq023
Carlson, C. J., Hopkins, S., Bell, K. C., Doña, J., Godfrey, S. S., Kwak, M. L., et al. (2020). A global parasite conservation plan. Biol. Conserv. 250:108596. doi: 10.1016/j.biocon.2020.108596
Ceryngier, P., and Romanowski, J. (2017). Harmonia axyridis (Pallas, 1773) (Coleoptera: Coccinellidae) and its parasite in south-western Bulgaria and northern Greece. Bioinvasions Rec. 6, 307–310. doi: 10.3391/bir.2017.6.4.02
Ceryngier, P., Romanowski, J., Szymańska, J., Galaniciak, M., Bardzińska, M., and Romanowski, M. (2013). Population of the invasive harlequin ladybird Harmonia axyridis (Coleoptera: Coccinellidae) from Pelješac Peninsula, southern Croatia. Stud. Ecol. Bioeth. 11, 79–91. doi: 10.21697/seb.2013.11.4.06
Ceryngier, P., and Twardowska, K. (2013). Harmonia axyridis (Coleoptera: Coccinellidae) as a host of the parasitic fungus Hesperomyces virescens (Ascomycota: Laboulbeniales, Laboulbeniaceae): a case report and short review. Eur. J. Entomol. 110, 549–557. doi: 10.14411/eje.2013.075
Crous, P. W., Osieck, E. R., Jurjević, Ž, Boers, J., van Iperen, A. L., Starink-Willemse, M., et al. (2021). Fungal planet description sheets: 1184–1382. Persoonia 47, 178–374. doi: 10.3767/persoonia.2021.47.06
De Kesel, A. (1996). Host specificity and habitat preference of Laboulbenia slackensis. Mycologia 88, 565–573. doi: 10.1080/00275514.1996.12026687
Dumolein, I. (2021). The Bat as a House for Different Residents: A Tritrophic Survey of Bats, Flies, and Fungi in a Honduran Cloud Forest. Unpublished Master Thesis. Ghent: Ghent University.
European Environment Agency (2020). Corine Land Cover (CLC) 2018, Version 2020_20u1. Available online at: https://land.copernicus.eu/pan-european/corine-land-cover/clc2018 (accessed March 14, 2021).
Ferrier, S. (2002). Mapping spatial pattern in biodiversity for regional conservation planning: where to from here? Syst. Biol. 51, 331–363. doi: 10.1080/10635150252899806
Fick, S. E., and Hijmans, R. J. (2017). WorldClim 2: new 1km spatial resolution climate surfaces for global land areas. Int. J. Climatol. 37, 4302–4315. doi: 10.1002/joc.5086
Fiedler, L., and Nedvěd, O. (2019). Fifty shades of the harlequin ladybird and a sexually transmitted fungus. J. Insect Sci. 19, 10. doi: 10.1093/jisesa/iez107
Garcés, S., and Williams, R. (2004). First record of Hesperomyces virescens Thaxter (Laboulbeniales: Ascomycetes) on Harmonia axyridis (Pallas) (Coleoptera: Coccinellidae). J. Kansas Entomol. Soc. 77, 156–158. doi: 10.2317/0304.18.1
Gippet, J. M., Colin, T., Grangier, J., Winkler, F., Haond, M., Dumet, A., et al. (2021). Land-cover and climate factors contribute to the prevalence of the ectoparasitic fungus Laboulbenia formicarum in its invasive ant host Lasius neglectus. Fungal Ecol. 51:101045. doi: 10.1016/j.funeco.2021.101045
Gorczak, M., Tischer, M., Pawlowska, J., and Wrzosek, M. (2016). First record of Hesperomyces virescens (Laboulbeniales, Ascomycota) on Harmonia axyridis (Coccinellidae, Coleoptera) in Poland. Acta Mycol. 51:1071. doi: 10.5586/am.1071
Haelewaters, D., Boer, P., Gort, G., and Noordijk, J. (2015). Studies of Laboulbeniales (Fungi, Ascomycota) on Myrmica ants (II): variation of infection by Rickia wasmannii over habitats and time. Anim. Biol. 65, 219–231. doi: 10.1163/15707563-00002472
Haelewaters, D., and De Kesel, A. (2020). Checklist of thallus-forming Laboulbeniomycetes from Belgium and the Netherlands, including Hesperomyces halyziae and Laboulbenia quarantenae spp. nov. MycoKeys 71, 23–86. doi: 10.3897/mycokeys.71.53421
Haelewaters, D., De Kesel, A., and Pfister, D. H. (2018a). Integrative taxonomy reveals hidden species within a common fungal parasite of ladybirds. Sci. Rep. 8:15966. doi: 10.1038/s41598-018-34319-5
Haelewaters, D., Hiller, T., Gorczak, M., and Pfister, D. H. (2018b). Influence of elytral color pattern, size, and sex of Harmonia axyridis (Coleoptera, Coccinellidae) on parasite prevalence and intensity of Hesperomyces virescens (Ascomycota, Laboulbeniales). J. Insects 9:67. doi: 10.3390/insects9020067
Haelewaters, D., Hiller, T., Kemp, E. A., van Wielink, P. S., Shapiro-Ilan, D. I., Aime, M. C., et al. (2020). Mortality of native and invasive ladybirds co-infected by ectoparasitic and entomopathogenic fungi. PeerJ 8:e10110. doi: 10.7717/peerj.10110
Haelewaters, D., Zhao, S. Y., Clusella-Trullas, S., Cottrell, T. E., De Kesel, A., Fiedler, L., et al. (2017). Parasites of Harmonia axyridis: current research and perspectives. Biocontrol 62, 355–371. doi: 10.1007/s10526-016-9766-8
Herz, A., and Kleespies, R. G. (2012). Occurrence of natural enemies in different populations of the invasive ladybird Harmonia axyridis (Pallas, 1771) (Coleoptera, Coccinellidae) in Germany. Mitt. Dtsch. Ges. Allg. Angew. Entomol. 18, 201–206.
Hijmans, R. J., van Etten, J., Sumner, M., Cheng, J., Baston, D., Bevan, A., et al. (2020). raster: Geographic Data Analysis and Modeling. Version 3.4-5. Available online at: https://CRAN.R-project.org/package=raster (accessed March 14, 2021).
Honek, A., Martinková, Z., Dixon, A. F. G., Skuhrovec, J., Roy, H. E., Brabec, M., et al. (2018). Life cycle of Harmonia axyridis in central Europe. Biocontrol 63, 241–252. doi: 10.1007/s10526-017-9864-2
Honek, A., Skuhrovec, J., Martinkova, Z., Kulfan, J., Jauschova, T., and Zach, P. (2021). Warm mesoclimate advances the seasonal dynamics of Harmonia axyridis in urban habitats. Front. Ecol. Evol. 9:725397. doi: 10.3389/fevo.2021.725397
Kidd, K. A., Nalepa, C. A., Day, E. R., and Waldvogel, M. G. (1995). Distribution of Harmonia axyridis (Pallas) (Coleoptera: Coccinellidae) in North Carolina and Virginia. Proc. Entomol. Soc. Washington 97, 729–731.
Lüdecke, D., Makowski, D., Waggoner, P., and Patil, I. (2020). Assessment of Regression Models Performance. CRAN. Available online at: https://easystats.github.io/performance/ (accessed March 14, 2021).
Magan, N. (2021). “Importance of ecological windows for efficacy of biocontrol agents,” in How Research Can Stimulate the Development of Commercial Biological Control Against Plant Diseases, eds A. De Cap, P. Melgarejo, and N. Magan (Cham: Springer), 1–14. doi: 10.1007/978-3-030-53238-3_1
Markó, B., Csata, E., Erős, K., Német, E., Czekes, Z., and Rózsa, L. (2016). Distribution of the myrmecoparasitic fungus Rickia wasmannii (Ascomycota: Laboulbeniales) across colonies, individuals, and body parts of Myrmica scabrinodis. J. Invert. Pathol. 136, 74–80. doi: 10.1016/j.jip.2016.03.008
McCornack, B. P., Koch, R. L., and Ragsdale, D. W. (2007). A simple method for in-field sex determination of the multicolored Asian lady beetle Harmonia axyridis. J. Insect Sci. 7, 10. doi: 10.1673/031.007.1001
Nalepa, C. A., Kennedy, G. G., and Brownie, C. (2005). Role of visual contrast in the alighting behavior of Harmonia axyridis (Coleoptera: Coccinellidae) at overwintering sites. Environ. Entomol. 34, 425–431. doi: 10.1603/0046-225X-34.2.425
Nalepa, C. A., and Weir, A. (2007). Infection of Harmonia axyridis (Coleoptera: Coccinellidae) by Hesperomyces virescens (Ascomycetes: Laboulbeniales): role of mating status and aggregation behavior. J. Invert. Pathol. 94, 196–203. doi: 10.1016/j.jip.2006.11.002
Nedvěd, O., Muhammad, A., Abdolahi, R., Sakaki, S., and Soares, A. O. (2019). Age and temperature effects on accumulation of carotenoids in ladybirds. IOBC WPRS Bull. 145, 33–36.
Orlova-Bienkowskaja, M. J., Spiridonov, S. E., Butorina, N. N., and Bieńkowski, A. O. (2018). Coinvasion by the ladybird Harmonia axyridis (Coleoptera: Coccinellidae) and its parasites, Hesperomyces virescens (Ascomycota: Laboulbeniales) and Parasitylenchus bifurcatus (Nematoda: Tylenchida, Allantonematidae), in the Caucasus. PLoS One 13:e0202841. doi: 10.1371/journal.pone.0202841
Pfliegler, W. P. (2014). First Hungarian record of the fungus Hesperomyces virescens (Ascomycota: Laboulbeniales), parasitic on the harlequin ladybird (Coccinellidae: Harmonia axyridis). e-Acta Nat. Pannon. 7, 139–142.
R Core Team (2020). R: A Language and Environment for Statistical Computing. Vienna: R Foundation for Statistical Computing.
Raak-van den Berg, C. L., Stam, J. M., de Jong, P. W., Hemerik, L., and van Lenteren, J. C. (2012). Winter survival of Harmonia axyridis in The Netherlands. Biol. Control 60, 68–76. doi: 10.1016/j.biocontrol.2011.10.001
Raak-van den Berg, C. L., van Wielink, P. S., de Jong, P. W., Gort, G., Haelewaters, D., Helder, J., et al. (2014). Invasive alien species under attack: natural enemies of Harmonia axyridis in the Netherlands. Biocontrol 59, 229–240. doi: 10.1007/s10526-014-9561-3
Rey, S. J., Arribas-Bel, D., and Wolf, L. (2020). “Spatial feature engineering,” in Geographic Data Science with PySAL and the PyData Stack, eds S. J. Rey, D. Arribas-Bel, and L. Wolf (Jupyter Book). Available online at: https://geographicdata.science/book/ (accessed January 31, 2022).
Riddick, E. W., and Schaefer, P. W. (2005). Occurrence, density and distribution of the parasitic fungus Hesperomyces virescens Thaxter (Laboulbeniales: Laboulbeniaceae) on the multicolored Asian lady beetle (Coleoptera: Coccinellidae). Ann. Entomol. Soc. Am. 98, 615–624. doi: 10.1603/0013-8746(2005)098[0615:odadop]2.0.co;2
Röhrich, C. R., Ngwa, C. J., Wiesner, J., Schmidtberg, H., Degenkolb, T., Kollewe, C., et al. (2011). Harmonine, a defence compound from the harlequin ladybird, inhibits mycobacterial growth and demonstrates multi-stage antimalarial activity. Biol. Lett. 8, 308–311. doi: 10.1098/rsbl.2011.0760
Rushton, S. P., Ormerod, S. J., and Kerby, G. (2004). New paradigms for modelling species distributions? J. Appl. Ecol. 41, 193–200. doi: 10.1111/j.0021-8901.2004.00903.x
Růžička, Z. (1984). Two simple recording flight mills for the behavioural study of insects. Acta Entomol. Bohemoslov. 81, 429–433.
Scheloske, H.-W. (1969). Beiträge zur biologie, ökologie und systematik der laboulbeniales (Ascomycetes) unter besondere berücksichtigung des parasit-wirt-verhältnisses. Parasitol. Schriftenr. 19, 1–176.
Seko, T., Yamashita, K., and Miura, K. (2008). Residence period of a flightless strain of the ladybird beetle Harmonia axyridis Pallas (Coleoptera: Coccinellidae) in open fields. Biol. Control 47, 194–198. doi: 10.1016/j.biocontrol.2008.08.007
Szentiványi, T., Haelewaters, D., Rádai, Z., Mizsei, E., Pfliegler, W. P., Báthori, F., et al. (2019). Climatic effects on the distribution of ant- and bat fly-associated fungal ectoparasites (Ascomycota, Laboulbeniales). Fungal Biol. 39, 371–379. doi: 10.1016/j.funeco.2019.03.003
van Wielink, P. S. (2017). Harmonia axyridis (Coleoptera: Coccinellidae): 13 jaar gevolgd met lichtvangsten in De Kaaistoep, Noord-Brabant. Entomol. Ber. 77, 97–105.
Welch, V. L., Sloggett, J. J., Webberley, K. M., and Hurst, G. D. D. (2001). Short-range clinal variation in the prevalence of a sexually transmitted fungus associated with urbanisation. Ecol. Entomol. 26, 547–550. doi: 10.1046/j.1365-2311.2001.00352.x
Windsor, D. A. (1995). Equal rights for parasites. Conserv. Biol. 9, 1–2. doi: 10.1046/j.1523-1739.1995.09010001.x
Keywords: community ecology, Hesperomyces, Laboulbeniales, parasite prevalence, precipitation, temperature, spatial modeling, agricultural landscape
Citation: Haelewaters D, Hiller T, Ceryngier P, Eschen R, Gorczak M, Houston ML, Kisło K, Knapp M, Landeka N, Pfliegler WP, Zach P, Aime MC and Nedvěd O (2022) Do Biotic and Abiotic Factors Influence the Prevalence of a Common Parasite of the Invasive Alien Ladybird Harmonia axyridis? Front. Ecol. Evol. 10:773423. doi: 10.3389/fevo.2022.773423
Received: 09 September 2021; Accepted: 10 March 2022;
Published: 13 April 2022.
Edited by:
Mathew Samuel Crowther, The University of Sydney, AustraliaReviewed by:
Gabriele Rondoni, University of Perugia, ItalyTamara Szentivanyi, Centre for Ecological Research, Hungarian Academy of Science, Hungary
Copyright © 2022 Haelewaters, Hiller, Ceryngier, Eschen, Gorczak, Houston, Kisło, Knapp, Landeka, Pfliegler, Zach, Aime and Nedvěd. This is an open-access article distributed under the terms of the Creative Commons Attribution License (CC BY). The use, distribution or reproduction in other forums is permitted, provided the original author(s) and the copyright owner(s) are credited and that the original publication in this journal is cited, in accordance with accepted academic practice. No use, distribution or reproduction is permitted which does not comply with these terms.
*Correspondence: Danny Haelewaters, ZGFubnkuaGFlbGV3YXRlcnNAZ21haWwuY29t
†Present Address: Danny Haelewaters, Department of Biology, Ghent University, Ghent, Belgium