- 1Subtidal Ecology Laboratory, Estación Costera de Investigaciones Marinas, Facultad de Ciencias Biologicas, Pontificia Universidad Católica de Chile, Santiago, Chile
- 2Victoria University Coastal Ecology Lab, School of Biological Sciences, Victoria University of Wellington, Wellington, New Zealand
- 3Millennium Nucleus for Ecology and Conservation of Temperate Mesophotic Reef Ecosystem (NUTME), Valparaiso, Chile
- 4Dragonfly Data Science, Wellington, New Zealand
- 5Centro de Estudios Avanzados en Zonas Áridas (CEAZA), Universidad Católica del Norte, Coquimbo, Chile
- 6Departamento de Biología Marina, Facultad de Ciencias del Mar, Universidad Católica del Norte, Coquimbo, Chile
- 7Departamento de Biología, Universidad de La Serena, La Serena, Chile
Patterns of species richness and their structuring forces at multiple scales provide a critical context for research efforts focusing on ecology, evolution, and conservation. Diversity gradients have been demonstrated in tropical reef fish, but corresponding patterns and mechanisms remain poorly understood in temperate regions. We conducted hierarchical (spatially nested) sampling of temperate reef fish faunas across > 140 degrees of longitude in the eastern and western South Pacific Ocean. Our sampling efforts spanned five distinct provinces: the Southeast Australian Shelf (SAS), Northern and Southern New Zealand (N-SNZ), Juan Fernandez and Desventuradas Islands (JFD), and the Warm Temperate Southeastern Pacific (WTPA). We evaluated (i) spatial variation in patterns of species richness and abundance (using Chao 1 index), and distribution of functional diversity (using several functional attributes: max body size, trophic groups, feeding guilds, trophic level, habitat use, gregariousness, and activity patterns) and (ii) scale-dependencies in these patterns. Species richness declined from west to east across the temperate South Pacific, but this pattern was detectable only across larger spatial scales. A functional redundancy index was significantly higher in the western South Australian Shelf at multiple scales, revealing that species contribute in equivalent ways to an ecosystem function such that one species may substitute for another. We also detected that patterns of variation in functional diversity differed from patterns of variation in species richness, and were also dependent on the spatial scale of analysis. Lastly, we identified that species’ traits are not equally distributed among reef fish assemblages, where some provinces are characterized by a distinct functional component within their reef fish assemblages. Planktivorous and schooling species, for instance, dominated the assemblages in the eastern Pacific, which is characterized by higher primary productivity and steep bathymetric slopes favoring these traits. Demersal and pairing behavior traits dominated the reef fish assemblages in western Pacific provinces (SAS, SNZ). We conclude that combining the identifies and species’ traits allow us to disentangle historical, biogeographic and environmental factors that structure reef fish fauna.
Introduction
Patterns of species distribution reflect how past and present environmental conditions shape the way in which biodiversity is linked with ecosystem functioning (Hooper et al., 2005). Biogeographic patterns contribute to a broader understanding of the distribution of taxonomic identities (Gaston, 2000), and more specifically, how species’ traits (morphological, behavioral, physiological aspects of species) are established within communities (Villéger et al., 2008; McLean et al., 2019, 2021). Linking both species richness and species trait distributions will determine which biogeographical, environmental and/or evolutionary processes are important in describing patterns (Kraft et al., 2008). This level of knowledge will help to identify the ecosystem services that communities offer to humans, and to inform appropriate management and conservation strategies (Bender et al., 2013; Violle et al., 2014; Veach et al., 2017).
Several patterns have been described determining how species composition are distributed and longitudinal gradients in the tropics have been particularly well-studied for coral reef fishes (Briggs, 2007; Cornell et al., 2007; Smith et al., 2014). Early studies suggested that strong longitudinal gradients in species richness may arise from a combination of geometric constraints on large-scale distribution and habitat availability (i.e., reef area, Connolly et al., 2003; Bellwood et al., 2005). More recent studies have emphasized the role of historical and evolutionary drivers of longitudinal gradients (Renema et al., 2008; Cowman and Bellwood, 2012). Less research has focused on the generality of longitudinal gradients, and we know little about longitudinal gradients in diversity in temperate reef regions. However, there is evidence of longitudinal gradients in species composition, and these gradients suggest that reef fish assemblages contain essential functional richness components (Smith et al., 2014).
Patterns and explanations for longitudinal gradients in temperate areas remain uncertain at both level, species identifies and species traits composition. A global study conducted with fine spatial resolution (i.e., at the scale of individual transects) showed no apparent longitudinal gradients in temperate reef fishes (Stuart-Smith et al., 2013). However, this study incorporated relatively few sites in warm temperate areas from the South Pacific that were outside of Australia; hence, ability to make inferences for the wider temperate region of the South Pacific remains limited.
Patterns and processes shaping biodiversity also may be scale-dependent (Tuya et al., 2011; Francisco-Ramos and Arias-González, 2013). For example, diversity of reef fishes is largely explained by beta-diversity within both the Caribbean (Francisco-Ramos and Arias-González, 2013) and West Australia (Tuya et al., 2011). Certainly, Witman et al. (2004) stated that the slope of the species-area relationship is shallower in temperate regions than in the tropics. Despite our understanding of patterns of species richness across the oceans, the distribution of functional traits in species that make up communities has not been fully understood to date (McLean et al., 2021).
Functional traits are measurable features that increase fitness in a particular environment (Violle et al., 2007, 2014). Thus, certain processes of the habitat act as hierarchical filters that select only those species that share appropriate functional traits for that environment (Wiescher et al., 2012; Bejarano et al., 2017) which make up the composition of a community within a region. In aquatic environments, research efforts have focused on understanding how the different processes that influence local assemblages vary through the different spatial scales (Bellwood et al., 2002; Edgar et al., 2017; Yeager et al., 2017; Barneche et al., 2019). Several lines of evidence indicate that large scale variation in the functional composition of an assemblage is given by oceanographic environmental filters that limit the dispersal of species (e.g., sea surface temperature, productivity, climatic instability), and modulated by evolutionary history and biogeographic processes (i.e., immigration, speciation, extinction) characteristic of a region (Bender et al., 2013; McLean et al., 2019).
In addition to large-scale processes, two main limiting filters of local species assemblage structure occur at fine spatial scales (e.g., site, locality) within a given area or biogeographic province. These are (1) biological interactions (e.g., competition, trophic status, facilitation) and, (2) local environmental filters (e.g., upwelling zones, temperature, substrate complexity, bottom rugosity) (Yeager et al., 2017; Ford and Roberts, 2020). This filter results in a spatial mosaic in the composition species, and their abundance and distribution of functional traits within a community (Longo et al., 2014; Bejarano et al., 2017). As such, functional diversity (i.e., functional richness, evenness, divergence) is an important feature of biological assemblages (Mason et al., 2005) but may not be related to the number of species in a community. For instance, local environmental filters lead to a decrease in the variation of functional traits as expected by the random selection of a group of species. This results in a convergence of functional traits and a decrease in the functional diversity of the assemblage (Boyé et al., 2019). Stuart-Smith et al. (2013) noted that temperate reefs, on average, have greater functional diversity than tropical counterparts, and hypothesized that this may be related to the increased evenness among local assemblages (Stuart-Smith et al., 2013). This is consistent with the idea that more specious regions have greater functional redundancy (Halpern and Floeter, 2008; Martins et al., 2012). However, it is unclear if these conclusions might change if patterns were evaluated at different spatial scales. In tropical ecosystems, Yeager et al. (2017) found that at larger spatial scales, functional diversity was predicted by primary productivity and reef slope, whereas at small spatial scales, habitat quality accounted for the variability of in functional diversity (mirroring the effects of species richness). We predict that the complexity and variability of local habitat (i.e., transect level) obscures processes at larger spatial scales which determine the regional species pool due to the dissociation of the species richness and functional diversity.
Reef fishes of temperate marine areas of the South Pacific are highly diverse, and they have been increasingly studied in recent years (Pérez-Matus et al., 2007, 2017a; Wellenreuther et al., 2008; Pérez-Matus and Shima, 2010; Jones, 2013; Ramírez et al., 2013; Fernández-Cisternas et al., 2021), and offer an excellent study system to evaluate diversity patterns along a longitudinal distance of more than 10,000 km. Here we used a hierarchical (spatially nested) sampling design to evaluate scale-dependency of a putative longitudinal gradient in temperate reef fish richness and functional diversity indices. We test a hypothesis that longitudinal diversity gradients for temperate reef fish species richness mirror those of tropical counterparts. Secondly, we evaluate the degree to which richness is decoupled from functional diversity, and its implications of how functional traits vary across the South Pacific; and the spatial scale-dependencies in these patterns.
Materials and Methods
Study Area and Data Collection
We implemented a spatially nested (hierarchical) sampling design that incorporated different scales of data aggregation. We defined and sampled within two distinct “realms” of the temperate South Pacific: (1) western Pacific (Warm Temperate Australasia) and (2) eastern Pacific (Warm Temperate South America). Within these two realms, we selected and sampled 5 five biogeographic “provinces.” Three of these provinces were located within the western realm: Southwestern Australian Shelf (SAS), Northern New Zealand (NNZ), Southern New Zealand (SNZ). The remaining two provinces were in the eastern realm: Juan Fernandez and Desventuradas (JFD), and Temperate Eastern South Pacific (WTSP) (Figure 1). Within each of these five provinces, we selected two localities (except for JFD where only one locality was selected) and sampled at least three “sites” (each separated by > 1 km). Nested within each site, we surveyed a minimum of 3 × 40 m belt transects. Collectively, the sampled area encompassed a longitudinal spread of more than 10,000 km and provided representation of most temperate shallow-water rocky reef habitats of the South Pacific. All study sites met basic criteria that included having rocky reef habitat with significant macroalgal cover and safe diving/boating conditions. In general, all study sites shared similar thermal conditions (Figure 1), yet important changes in other oceanographic conditions are observed (Table 1). For instance, the Juan Fernandez and Desventuradas province is comparatively oligotrophic, with a primary productivity that is an order of magnitude lower than other areas. In contrast, the Warm Temperate Southeastern Pacific is characterized by high primary productivity, associated with nearby coastal upwelling cells (Tapia et al., 2014). Both provinces within the western realm have a very high bathymetric slope, owning to the comparatively small coastal shelves (Table 1).
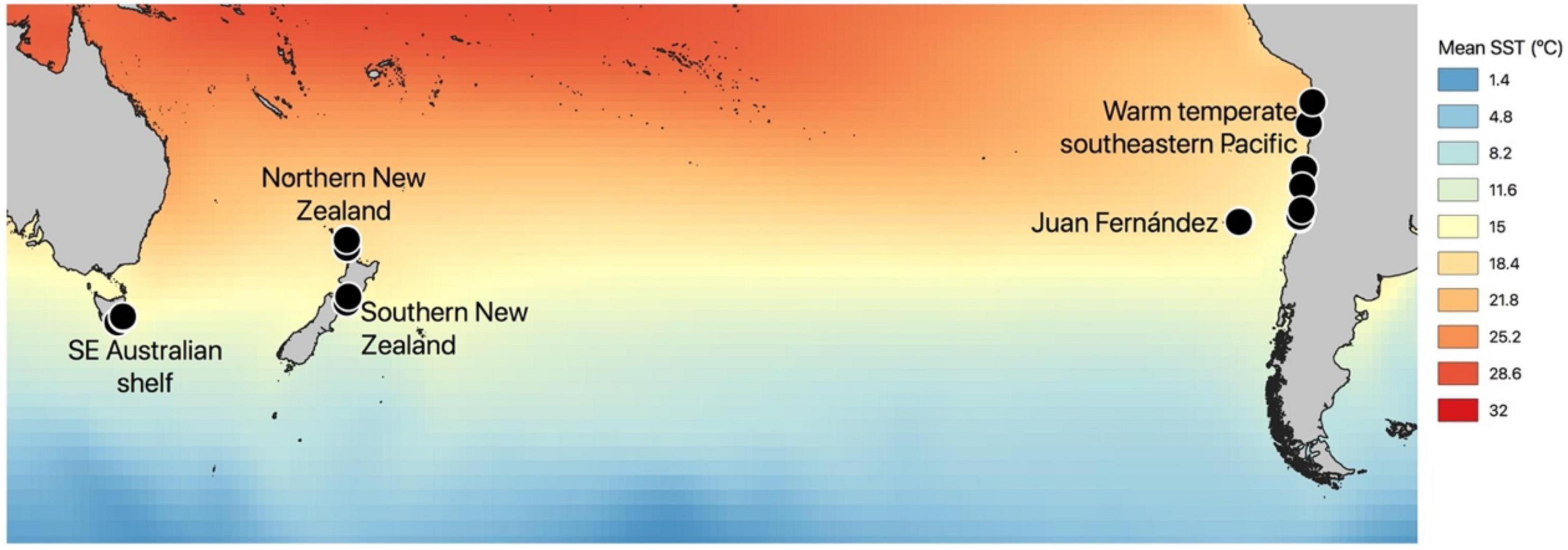
Figure 1. Map of the study region showing locations of the five major marine biogeographic provinces. The domain is distributed across the temperate South Pacific Ocean, and includes samples from all provinces with temperate rocky reef habitat.
To minimize potential confounding effects of fishing activities, we conducted our sampling in sites that were either marine reserves or managed areas that precluded substantial harvesting activity (Territorial Users Rights for Fisheries, TURFS in Chile), or at sites where fishing access (particularly spearfishing) was difficult (e.g., oceanic islands) (Pérez-Matus et al., 2017a). Within each site, shallow-water reef fishes were surveyed along “belt transects” (each 40 m long). At each site a minimum of 2 and a maximum of 5 transects were conducted (median of 5 transects) that were positioned perpendicular to coastline and separated by 50 m. We sampled reef fish along 138 transects in total. All surveys were conducted during austral spring (October through December).
We counted fish along each belt transect by swimming along a straight line at constant speed (with the aid of SCUBA) and recording all large [>10 cm total length (TL)] and small (<10 cm in TL) fish within the survey area. Large fish were identified to species level, and abundance and size structure was estimated for all individuals observed within 2 m on either side of the transect and up to 5 m above the transect. Larger species were counted while the diver was swimming at a constant speed in the “outbound” direction along the transect. Smaller cryptic species were recorded by the diver on his return to the starting point of the sampling. Small fish species were recorded within 1 m either side of the transect and to a height of 1 m in the water column. Each visual census (including both small and large fish) took ∼20–25 min per transect to complete, and was conducted by the same observer (APM) to minimize inter-observer sampling biases (see Pérez-Matus et al., 2007; Pérez-Matus and Shima, 2010; Ramírez et al., 2013 for more details about the methodology).
Sampled sites were all located along relatively wave-exposed coastlines that had substantial coverage of macroalgae. Kelp species (i.e., Macrocystis pyrifera, Ecklonia radiata, Lessonia variegata, or L. trabeculata) occurred in high frequency in all study sites. Mixed assemblages of large brown macroalgae (Ecklonia radiata) with Fucalean species (e.g., Carpophyllum spp.) dominated southern North Island, New Zealand, and northern Tasmania. Foliose algae (i.e., Dyctiota spp., Padina spp.) dominated Juan Fernández Islands only whereas single Laminariales (i.e., Lessonia trabeculata) dominated the subtidal habitats in central and northern Chile (Pérez-Matus et al., 2014; Figure 2).
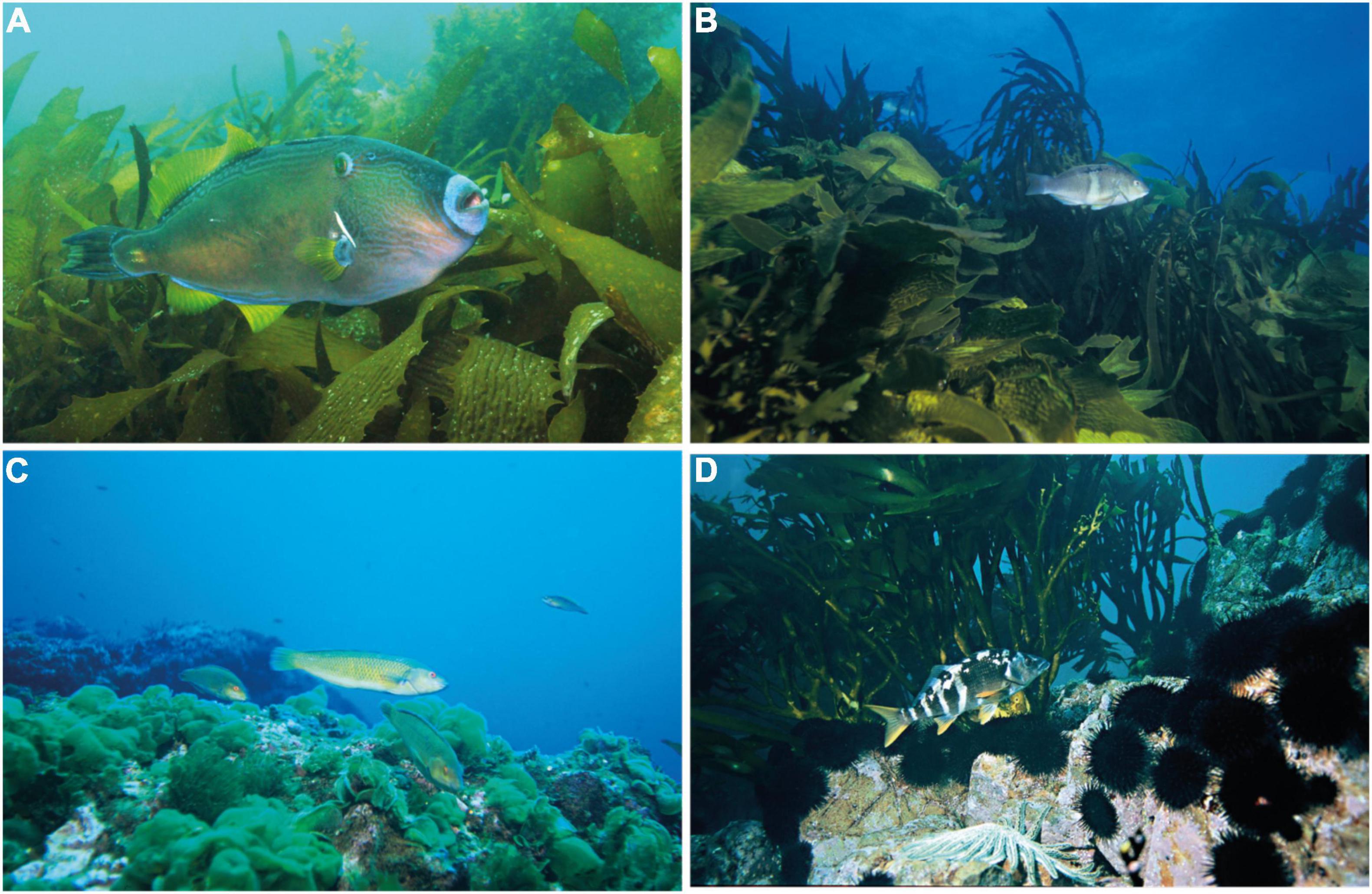
Figure 2. Characteristic fish and habitat-forming macroalgal species from biogeographic provinces surveyed across the temperate South Pacific Ocean: (A) Southeast Australian Shelf (SAS), (B) Northern and Southern New Zealand (NNZ and SNZ), (C) Juan Fernandez and Desventuradas (JFD), and (D) Warm Temperate Western Southeatern Pacific (WTSP).
To account for functional aspects of fish diversity, we extracted information on several traits of the species that we counted (see Supplementary Table 1). We included maximum body size (TL), trophic status (mean trophic level; Froese and Pauly, 2014), and each surveyed fish species was assigned to a “coarse” trophic group (i.e., carnivores, herbivores, ommivores, and planktivores), then we assigned each species to a fine trophic category (i.e., general carnivore, herbivores and detritivores, mobile and sessile invertivores, omnivore or planktivore) (Gajdzik et al., 2019; Siqueira et al., 2020). We also evaluated more finely resolved trophic guilds (hereafter “fine trophic groups”), assigning each species to one of the following: (1) herbivore (which included browsers and grazers macroalgal consumers, scrapers, and turf algal consumers; Bellwood et al., 2014), (2) planktivores, (3) macroinvertivores of soft-shelled prey (i.e., amphipods, isopods, polychaetes) and hard-shelled prey (i.e., mollusks), (4) pelagic piscivores, and (5) planktivore and zooplanktivores (Pérez-Matus et al., 2012, 2017b). We compiled other categorical traits such as habitat preference using water column position such as benthic (sedentary in the bottom), demersal (swimming near the bottom), pelagic species (including species with a high site fidelity or species that migrate seasonally to a reefs). Gregariousness based on species mainly solitary, pairing species (that may form schools occasionally) and schooling species. We also accounted for species that are active during the day (diurnal) or at night (nocturnal) or both (Supplementary Table 1).
Data Analysis
We used coverage-based estimations of the species richness to evaluate and account for possible sampling biases (Chao and Jost, 2012) at the three spatial scales studied (locality, site, and transect). First, we estimate rarefacted/extrapolated species richness for different levels of sampling. Secondly, we evaluate the observed species richness as a function of the sample coverage. Thirdly, we used the Chao 1 extrapolation index as a measure of asymptotic species richness. Analyses were carried out using the libraries vegan (Oksanen et al., 2007) and iNEXT (Hsieh et al., 2016).
We used mixed linear models to evaluate biogeographic and scale-dependent patterns in fish species richness and functional diversity indices. These are appropriate for data with multiple levels and suit unbalanced designs (see Table 1). Analyses were carried out at two spatial scales: site and transect. We use these two spatial scales resolution only due to the insufficient replication of hierarchical sampling at location (locality) scales (n = 2, Table 1). To evaluate patterns at the scale of sites across provinces and realms, we pooled data collected on replicate transects. We estimated the effect size of longitudinal differences at each spatial scale using a fixed effect for realm (western vs. eastern), and we included the higher spatial scales (i.e., provinces and sites) as random effects. We also carried out a variance component analysis to estimate the relative contribution of each nested spatial level (realms/provinces/transects) to variability in species richness and functional diversity. All analyses were conducted using lme4 (Bates et al., 2015) in R.
We estimated alpha-functional diversity using a set of five indices (estimated separately at site-level and transect-level), including functional richness (FRic), evenness (FEve), divergence (FDiv), dispersion (FDis), and redundancy (FRed). These five metrics emphasize different aspects of the functional diversity of assemblages (Laliberte and Legendre, 2010; Boyer and Jetz, 2014). FRic, FEve, and FDiv are based on principal coordinates analyses obtained from a dissimilarity matrix built on Gower’s index (Villéger et al., 2008). FRic is measured as the convex hull volume of the multivariate trait space, where higher values indicate a larger functional space. FEve is measured as the minimum spanning tree linking all the species within the trait space, where high values of FEve imply a more regular filling of the functional space (Villéger et al., 2008). FDiv measures the species deviance from the centroid of the multivariate trait space, and increases if species are far from the centroid, implying the existence of more extreme trait values in the functional space (Villéger et al., 2008). FDis is the average distance to the centroid of the multivariate trait space, where higher values indicate a higher dispersion of species over the trait space (Laliberte and Legendre, 2010). FRed a measure of the whole functional potential minus the observed functional diversity that was estimated using the expression proposed by Bello et al. (2007), as the difference between Gini index of dominance and Rao’s Q, where values close to 1 correspond to assemblages with a high functional redundancy.
To further explore spatial changes in trait variability (i.e., beta-functional diversity), we analyzed changes in the abundance proportion of each trait category (for categorical traits) or abundance-weighted trophic level and body size (for continuous traits) among provinces. The former analysis was summarized using a canonical analysis of principal coordinates (CAP; Anderson and Willis, 2003) carried out at site scale. A trait vs. site matrix was used, estimating the proportion of individuals in each trait category. For continuous traits, values were rescaled to 0–1, using the maximum and minimum values across sites as bounds. CAP was carried out based on the Bray-Curtis similarity index. Finally, a permutational analysis of variance (PERMANOVA, Anderson and Walsh, 2013) was carried out to test the existence of differences in trait relative abundance among provinces nested within realms, where p-value was based on 10,000 permutations. A post-hoc test was carried out to test the significance of pair-wise differences between provinces, with p-values corrected using the Benjamin-Hochberg method implemented in the library RVAideMemoire (Hervé, 2021) in R.
Results
We counted more than 19,000 individuals comprising 133 species from 85 genera, 47 families and 11 orders (Supplementary Table 1). In general, coverage was very high at locality (mean = 0.99, SD = 0.005), site (mean = 0.991, SD = 0.009), and transect scales (mean = 0.940, SD = 0.090). The asymptotic species richness estimator was positively correlated to observed species richness at all scales (0.784, 0.881, 0.808, at locality, site, and transect, respectively, p < 0.05 in all cases). Species richness declined from west to east across the sampled region of the South Pacific, but this pattern was scale-dependent (Figure 3 and Table 2). The pattern was strongest when data were pooled across localities within provinces (Figure 3). When data were pooled across transects within sites, species richness varied between realms (P = 0.019, Pseudo-R2 = 0.50, Table 2). At the scale of site, species richness was greatest in the SE Australian Shelf and N New Zealand provinces, where estimated richness was twice as high as in eastern areas of the Temperate Eastern South Pacific and Juan Fernandez and Desventuradas (Figure 3). At transect scale, species richness was largely invariant across provinces and realms (P = 0426, Pseudo-R2 = 0.019; Figure 3 and Table 2).
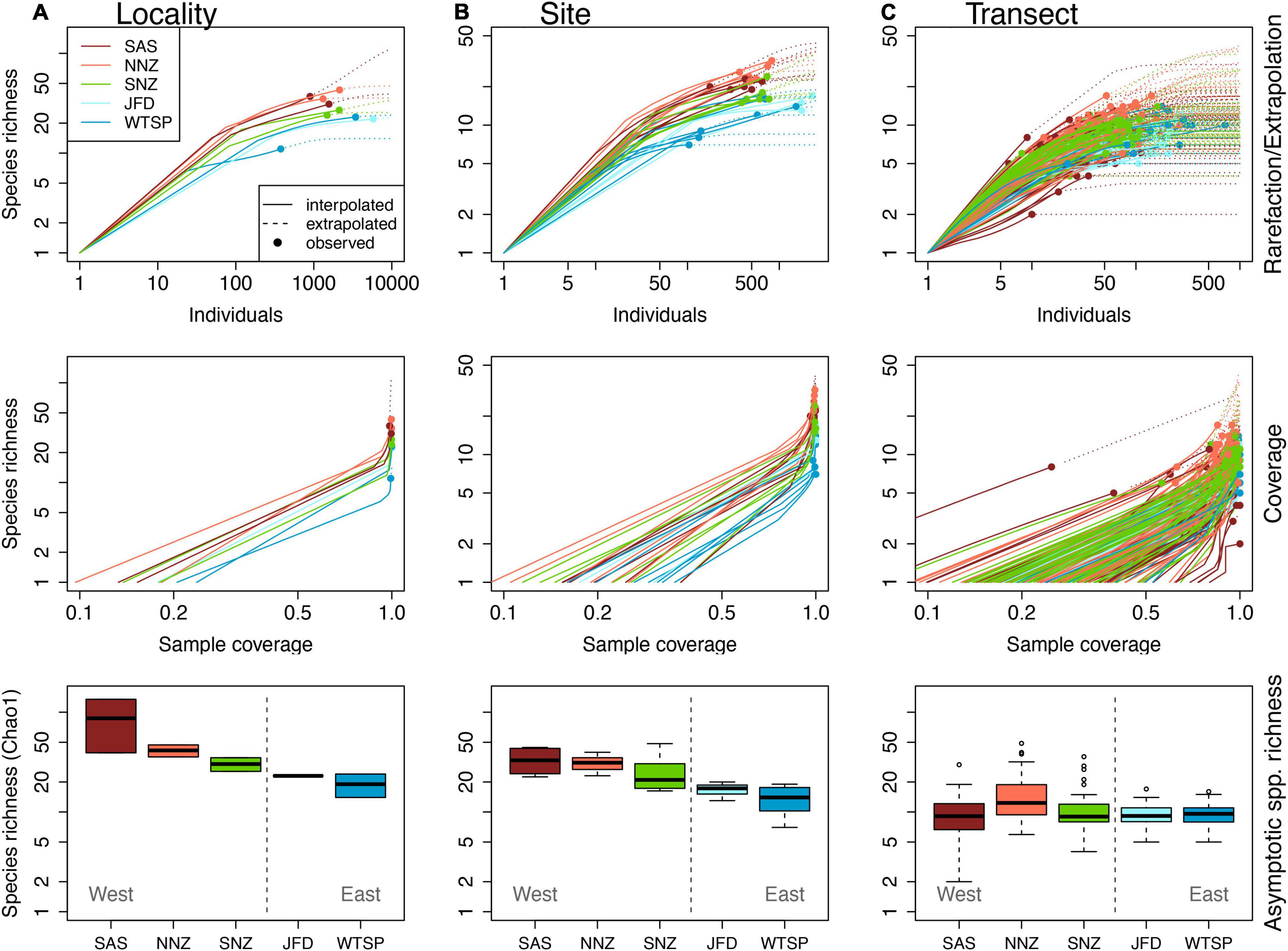
Figure 3. Local patterns of reef fish species richness across biogeographic provinces, using size-based rarefaction/extrapolation, coverage-based rarefaction/extrapolation, and asymptotic species richness (Chao 1) at locality (A), site (B), and transect (C) scales.
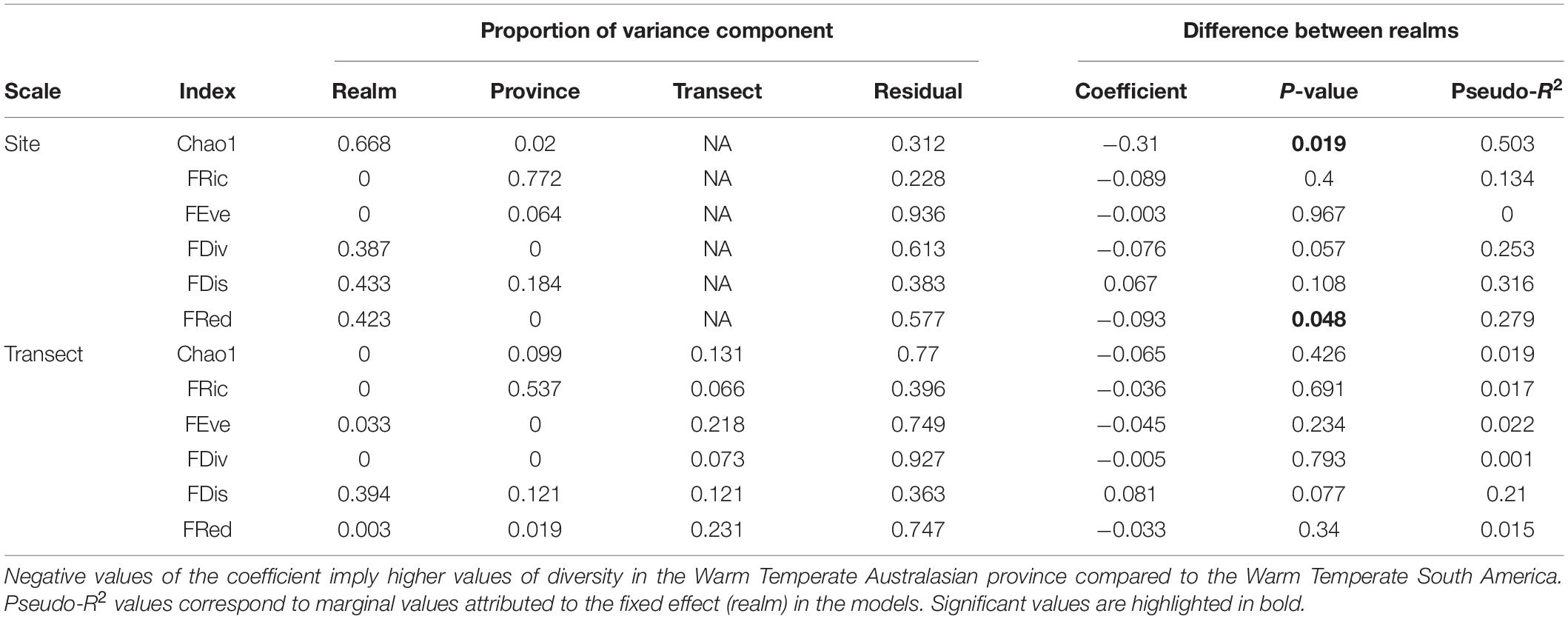
Table 2. Summary of linear mixed models for species richness (Chao 1 index) and functional diversity at the two spatial scales of analysis.
Alpha-functional diversity indices showed different biogeographic trends (Supplementary Figure 1 and Table 2). Differences between realms were significant only for FRed measured at site scale, showing a reduction from east to west Pacific (P = 0.048, Pseudo-R2 = 0.279, Supplementary Figure 1 and Table 2). The relative abundance of traits levels was highly variable among provinces (Figures 4, 5). The beta-functional diversity analysis showed that two first axes of CAP explain 74 and 12% of variance, respectively (Figure 5); whereas four provinces (SAS, NNZ, SNZ, and WTSP) are sorted along the first CAP axis, the JFD province is mainly differentiated along the second CAP axis (Figure 5). The PERMANOVA showed the existence of significant differences in trait compositions among provinces nested within realms (R2 = 0.661, p < 0.0001); all provinces where different each other (post-hoc test, p < 0.05 in all cases). SAS and SNZ provinces share more similarities compared to NNZ, and they characterized for a higher relative abundance of mobile invertivores, demersal, and pairing forms. In NNZ the frequency of zooplanktivore and schooling forms was higher than in other provinces. The WTSP is characterized for the prevalence of pelagic site attached forms, whereas the high dominance of general carnivores is distinctive of the JFD province.
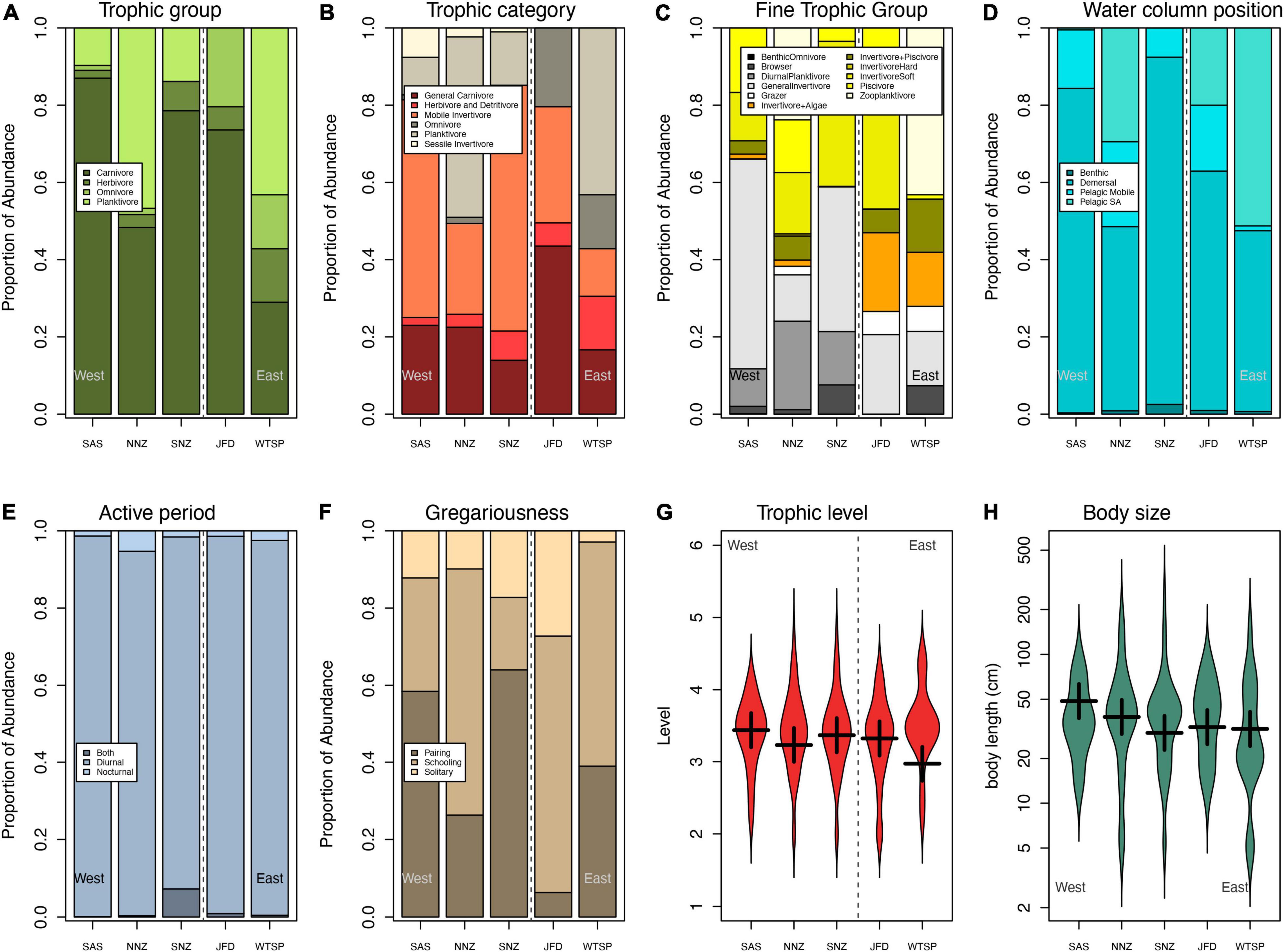
Figure 4. Functional traits of species (A) the proportion of the abundance within coarse trophic groups (i.e., planktivore, herbivores, omnivores, and carnivores), (B) proportion of the abundance within different trophic categories (general carnivores, mobile invertivores, omnivores, planktivores, and sessile invertivores), (C) fine-feeding guilds (planktivores, benthic omnivores, browser and grazer herbivores, invertivores of soft, hard, both types of prey, invertivores and piscivores, and piscivores); (D) proportion of the abundance of species within water column position (benthic species, demersal, pelagic mobile, and Pelagic site attached); (E) proportion of the abundance within Active period (diurnal, nocturnal, or both species); (F) proportion of the abundance Gregariousness (solitary, pairing, schooling species); violin plots depicting the median (black bar), the horizontal dotted line facilitating comparison of multiple provinces, the upper and lower adjacent values, the first and the third quantile (depicted by the vertical dotted lines) and outside points depicted by the narrow vertical line of (G) trophic level, and (H) max body size at the 5 sampled provinces such as Southeast Australian Shelf (SAS), Northern New Zealand (NNZ), Southern New Zealand (SNZ), Juan Fernandez and Desventuradas (JFD), Warm Temperate Southeastern Pacific (WTSP).
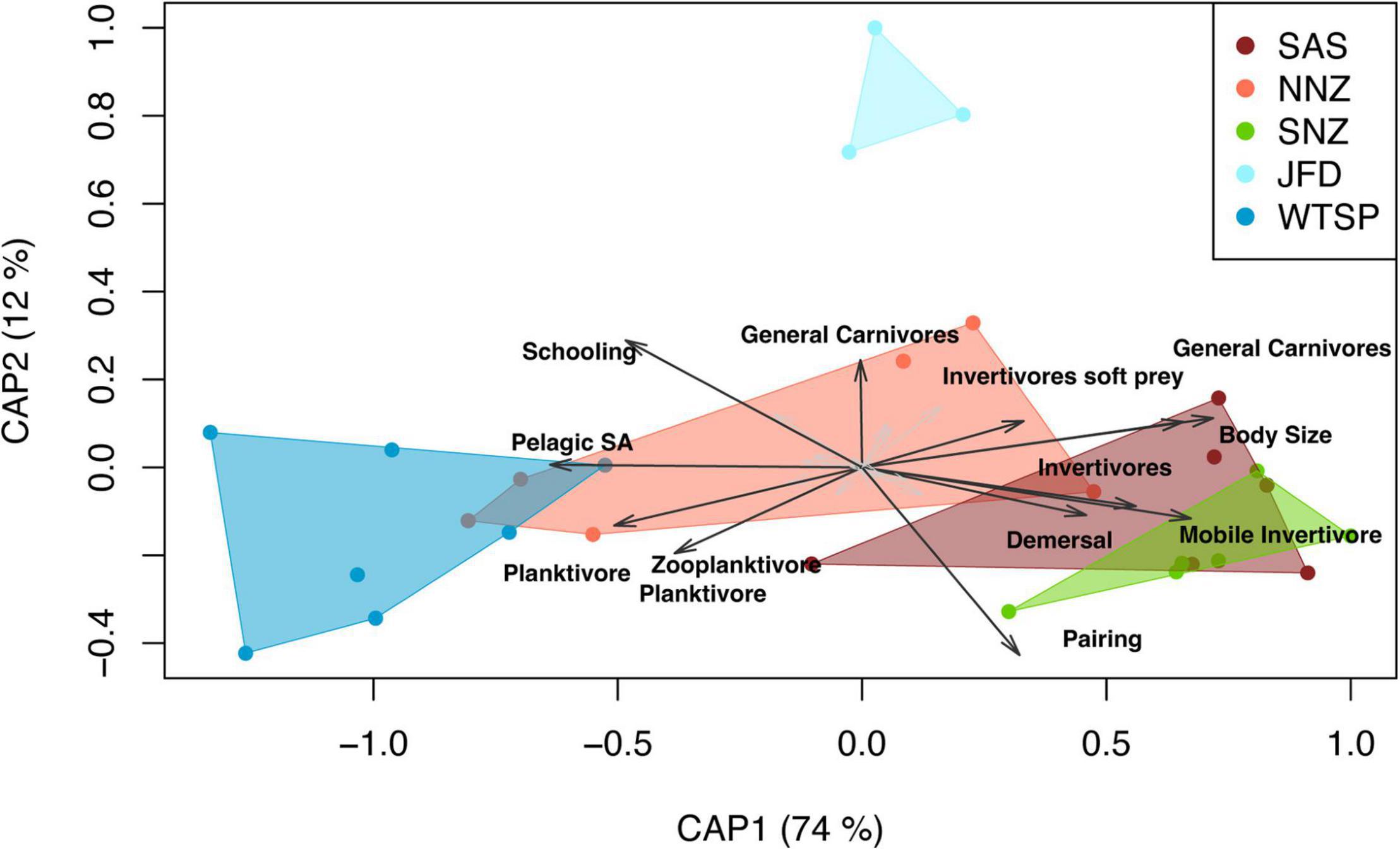
Figure 5. Biplot of constrained Analysis of Principal Coordinates (CAP) explaining the proportion of the abundance of each trait category (for categorical traits) or abundance-weighted trophic level and body size (for continuous traits) of sampled provinces.
Discussion
We identify gradients in species richness and functional diversity of temperate reef fishes across the South Pacific basin that vary with spatial scales of observation. At the coarsest spatial scale, we found a specious western Pacific but high redundancy in the diversity of species functional traits evaluated. Conversely, we found that provinces with the lowest richness were comprised of species with a diverse set of functions. However, each biogeographic province had a distinctive structure of functional traits.
We found the gradients in fish richness, expressed at the scale of realms, regions and localities, indicated that temperate reef fish assemblages are numerous in terms of identities in the temperate western Pacific (SAS and NS—NZ) than in the eastern Pacific. These gradient in species richness have been well documented and the mechanism is explained by the maximum diversity center in the Indo-Australian Archipelago (IAA). This diversity hotspot covers just 3% of the surface area of the tropical Indo-west central Pacific and contains 52% of all reef fishes found in the regional species pool (Allen, 2008; Renema et al., 2008; Parravicini et al., 2013). The IAA is approximately centered on a span of ca. 8,000 km (75°) in latitude and ca. 15,000 km (245°) in longitude, and for a wide range taxa, diversity declines with distance from the IAA, in all directions (Randall, 1998; Connolly et al., 2003; Bellwood et al., 2005; Allen, 2008; Tittensor et al., 2010). Cowman and Bellwood (2013) defined a global biodiversity hotspot in the Indo-Pacific region; the SAS and northern and southern New Zealand are close to this hot spot of reef fish biodiversity region which explains the largest number of species of fish. Our analysis confirms the existence of this difference in reef fish species richness from west to east provinces and seems to reflect patterns occurring for coral reef fishes. This mean that most of the species that make up the temperate fish assemblages of the South Pacific are related at least at the Family level with those of the adjacent tropical areas (Parravicini et al., 2013; Hemingson and Bellwood, 2018).
The comparatively low species richness in the eastern realm could be associated to historical biogeographical explanations, linked to their peculiar environmental conditions. Despite SST is comparatively similar between west and east provinces, the salinity of WTSP and JFD is up to 1 PSU lower than the western provinces. Also the seabed of WTSP and JFD have a very steep slopes (i.e., narrow shelves), and whereas the WTSP is hypertrophic the JFD is characterized by oligotrophic conditions (Table 1). The reef fish fauna off the WTSP is mainly composed of species of both subtropical origin and subantarctic species (Ojeda et al., 2000; Navarrete et al., 2014), but their evolutionary history has been scarcely studied (but see, Beldade et al., 2020; Delrieu-Trottin et al., 2022) WTSP represents the end point of the historical radiation of fish from the Indo-Pacific, and therefore, perciform fish of tropical origin have not had enough time to adapt to the oceanographic conditions of this system, explaining the low species richness (Navarrete et al., 2014). Possibly similar processes could have occurred independently in Juan Fernandez and Desventuradas (Burridge et al., 2006). This entire region area has faced continued isolation and extinction, reducing impacts on adjacent temperate regions such as those present in this study (Bellwood et al., 2002). Bender et al. (2013) concluded that differences in the taxonomic structure of reef fish assemblages in the Atlantic reef reflect the influences of biogeography and historical processes such as the closure of the Isthmus of Panama and Pleistocene Glaciations generating extinctions and reduction of shallow-reefs biota which may be comparable to the low WTSP reef fish fauna.
In general, alpha-functional diversity did not follow the spatial trends observed for species richness. The functional diversity indices showed different trends among provinces depending on the scale. Only functional redundancy showed a significant trend, being higher in the west realm. This implies that the observed increase in species richness at higher spatial scales occurs is not paralleled with an increase in functional traits novel to this realm. Functional redundancy is positively associated with ecological resilience and stability, as ecosystem functions of communities with more redundant species (those that have similar functions) should be buffered against the loss of individual species after a disturbance (Biggs et al., 2020). Our results indicated that the high functional redundancy detected in the Southeast Australian Shelf makes this province particularly robust to species extirpations. On the other hand, low functional redundancy in the Warm Temperate Southeastern Pacific makes ecosystems more vulnerable to disturbances due to the low number of species with similar roles and functions within the fish assemblages. The increase in functional redundancy toward the west may explain why species richness at transect scale was relatively invariant across sampled regions, suggesting that ecological interactions may limit the arrival of species richness at smaller scales (Ricklefs, 1987; He et al., 2005; Fernández-Cisternas et al., 2021). The westward increase in functional redundancy may be also linked to a change in environmental conditions (Yeager et al., 2017), but the limited number of provinces analyzed precluded a formal test of this idea. Beta-functional diversity, estimated from the CAP, showed that sampled provinces were highly differentiable each other based on the relative abundance of the different functional traits. This suggest that environmental filtering, associated to the marked differences in environmental conditions among regions, may be acting to create such differences in trait dominance (Yeager et al., 2017; Ford and Roberts, 2020). For instance, mean body size increases westward, particularly at SAS and SNZ. At global biogeographic scale, Barneche et al. (2014, 2019) found that fish body size is one of the major predictors of reef fish species richness. Body size is responsible for the dispersal capacity of individuals in which large body size individual tends to live longer and have larger home ranges than smaller individuals (Barneche et al., 2014; Nash et al., 2015). Traits related to different aspects of trophic ecology were also important differentiating provinces. For instance, general carnivores prevail at the isolated oligotrophic JFD province. Interestingly, Siqueira et al. (2020) indicated that the trophic groups that have had a greater diversification rate, are those families of reef fish species that consume prey of low nutritional value, that is, detritivores, herbivorous, and omnivorous fishes (Bellwood et al., 2014; Floeter et al., 2018; Siqueira et al., 2020). The prevalence of the pelagic species forms in WTSP may be due to existence of very narrow coastal shelves that limits the effective area to find benthic food items. Again, a more detailed analysis of the role of environmental filtering, based on expanded datasets may be used to put this idea to a test.
The influence of local scale processes in determining patterns of functional diversity of reef fishes may be beneficial for conservation efforts of small areas (i.e., marine reserves, well enforced Territorial Users Right for Fisheries, TURF) as these areas may safeguard important reef fish functions (Pérez-Matus et al., 2017a). These regions are facing increasing direct threats primarily due fishing and habitat removal (Pérez-Matus et al., 2017b). Further the indirect effects of reef fish removal have generated a series of warning signals of potential regime shifts, a result that has been described at both large and small spatial scales. Processes that maintain current ecological states and spatial and temporal heterogeneity in temperate kelp-dominated habitats will be vital to maintaining biodiversity.
Data Availability Statement
The raw data supporting the conclusions of this article will be made available by the authors, without undue reservation.
Ethics Statement
The animal study was reviewed and approved by the Comité de Etica y Seguridad en la Investigacion, Pontificia Universidad Católica de Chile.
Author Contributions
All authors conceived the study. AP-M conducted the field work and provided the first draft. MR, PN, and AP-M conducted the stats and analyses. All authors approved its submission.
Funding
Funding for this research has been provided by the CONICYT (ANID)-VUW Ph.D. scholarship, Education-New Zealand Study Abroad Scholarship (ENZTA); FONDECYT # 1151094 and # 1210216 to AP-M, # 1140841 to MR, and FRST contract C01 × 0501 to JS. The research of MR was funded by ANID-CENTROS REGIONALES R20F0008 and ANID/FONDECYT 1200843.
Conflict of Interest
PN was employed by the company Dragonfly Data Science.
The remaining authors declare that the research was conducted in the absence of any commercial or financial relationships that could be construed as a potential conflict of interest.
Publisher’s Note
All claims expressed in this article are solely those of the authors and do not necessarily represent those of their affiliated organizations, or those of the publisher, the editors and the reviewers. Any product that may be evaluated in this article, or claim that may be made by its manufacturer, is not guaranteed or endorsed by the publisher.
Acknowledgments
We would like to thank people who assisted us in the field and provided logistic support to finish the fish and habitat surveys at all provinces. We thank Carolina Zagal, Simon Gartenstein, and Paul Di Benedetto from Tasmania, Australia; Antonio do Santos, Dr. Tyler Eddy, John van der Sman, Daniel McNaugtan and Dr. Shane Geange from South and Northern New Zealand; Eduardo Sorensen, Marcelo Rossi (Refugio Nautico, JF), Dr. Sergio Carrasco and Dr. Erasmo Macaya, Teresa Navarrete, Catalina Ruz from Juan Fernandez as well as Warm Temperate South Pacific regions. We thank to Dr. Malcolm Francis (NIWA) for valuable conversations about the observed patterns and comments on earlier drafts of the manuscript. Clinton Duffy from the Department of Conservation New Zealand for providing logistic support at the Poor Knights Islands, NZ. We thank the editor and both reviewers that have provided comments and help us to improve the manuscript. Lastly, we dedicate this study to Russell Cole (RIP).
Supplementary Material
The Supplementary Material for this article can be found online at: https://www.frontiersin.org/articles/10.3389/fevo.2022.768707/full#supplementary-material
References
Allen, G. (2008). Conservation hotspots of biodiversity and endemism for Indo-Pacific coral reef fishes. Mar. Freshw. Ecosyst. 18, 541–556.
Anderson, M. J., and Willis, T. J. (2003). Canonical analysis of principal coordinates: a useful method of contrained ordination for ecology. Ecology 84, 511–525. doi: 10.1890/0012-9658(2003)084[0511:caopca]2.0.co;2
Anderson, M. J., and Walsh, D. C. (2013). Permanova, Anosim, and the Mantel test in the face of heterogeneous dispersions: what null hypothesis are you testing? Ecol. Monogr. 83, 557–574.
Assis, J., Tyberghein, L., Bosch, S., Verbruggen, H., Serrão, E. A., and De Clerck, O. (2018). Bio-ORACLE v2.0: extending marine data layers for bioclimatic modelling. Glob. Ecol. Biogeogr. 27, 277–284. doi: 10.1111/geb.12693
Barneche, D. R., Kulbicki, M., Floeter, S. R., Friedlander, A. M., Maina, J., and Allen, A. P. (2014). Scaling metabolism from individuals to reef-fish communities at broad spatial scales. Ecol. Lett. 17, 1067–1076. doi: 10.1111/ele.12309
Barneche, D. R., Rezende, E. L., Parravicini, V., Maire, E., Edgar, G. J., Stuart-Smith, R. D., et al. (2019). Body size, reef area and temperature predict global reef-fish species richness across spatial scales. Glob. Ecol. Biogeogr. 28, 315–327.
Basher, Z., Bowden, D. A., and Costello, M. J. (2018). GMED: global marine environment datasets for environment visualisation and species distribution modelling. Earth Syst. Sci. Data Discuss. 2018, 1–62.
Bates, D., Mächler, M., Bolker, B., and Walker, S. (2015). Fitting linear mixed-effects models Usinglme4. J. Stat. Softw. 67, 1–48.
Bejarano, S., Jouffray, J. B., Chollett, I., Allen, R., Roff, G., Marshell, A., et al. (2017). The shape of success in a turbulent world: wave exposure filtering of coral reef herbivory. Funct. Ecol. 31, 1312–1324.
Beldade, R., Longo, G. C., Clements, K. D., Robertson, D. R., Perez-Matus, A., Itoi, S., et al. (2020). Evolutionary origin of the Atlantic Cabo Verde nibbler (Girella stuebeli), a member of a primarily Pacific Ocean family of antitropical herbivorous reef fishes. Mol. Phylogenet. Evol. 156:107021. doi: 10.1016/j.ympev.2020.107021
Bello, F. D., Lepš, J., Lavorel, S., and Moretti, M. (2007). Importance of species abundance for assessment of trait composition: an example based on pollinator communities. Commun. Ecol. 8, 163–170. doi: 10.1556/comec.8.2007.2.3
Bellwood, D. R., Goatley, C. H., Brandl, S. J., and Bellwood, O. (2014). Fifty million years of herbivory on coral reefs: fossils, fish and functional innovations. Proc. R. Soc. B 281:20133046. doi: 10.1098/rspb.2013.3046
Bellwood, D. R., Hughes, T. P., Connolly, S. R., and Tanner, J. (2005). Environmental and geometric constraints on Indo-Pacific coral reef biodiversity. Ecol. Lett. 8, 643–651. doi: 10.1111/j.1461-0248.2005.00763.x
Bellwood, D. R., Wainwright, P. C., Fulton, C. J., and Hoey, A. (2002). Assembly rules and functional groups at global biogeographical scales. Funct. Ecol. 16, 557–562. doi: 10.1046/j.1365-2435.2002.00655.x
Bender, M. G., Pie, M. R., Rezende, E. L., Mouillot, D., and Floeter, S. R. (2013). Biogeographic, historical and environmental influences on the taxonomic and functional structure of Atlantic reef fish assemblages. Glob. Ecol. Biogeogr. 22, 1173–1182. doi: 10.1111/geb.12099
Biggs, C. R., Yeager, L. A., Bolser, D. G., Bonsell, C., Dichiera, A. M., Hou, Z., et al. (2020). Does functional redundancy affect ecological stability and resilience? A review and meta-analysis. Ecosphere 11:e03184.
Boyé, A., Thiébaut, E., Grall, J., Legendre, P., Broudin, C., Houbin, C., et al. (2019). Trait-based approach to monitoring marine benthic data along 500 km of coastline. Diversity Distribut. 25, 1879–1896. doi: 10.1111/ddi.12987
Boyer, A. G., and Jetz, W. (2014). Extinctions and the loss of ecological function in island bird communities. Glob. Ecol. Biogeogr. 23, 679–688. doi: 10.1111/geb.12147
Briggs, J. C. (2007). Marine longitudinal biodiversity: causes and conservation. Diversity Distribut. 13, 544–555. doi: 10.1111/j.1472-4642.2007.00362.x
Burridge, C., Meléndez, C. R., and Dyer, B. (2006). Multiple origins of the juan fernández kelpfish fauna and evidence for frequent and unidirectional dispersal of cirrhitoid fishes across the South Pacific. Syst. Biol. 55, 566–578. doi: 10.1080/10635150600812585
Chao, A., and Jost, L. (2012). Coverage-based rarefaction and extrapolation: standardizing samples by completeness rather than size. Ecology 93, 2533–2547. doi: 10.1890/11-1952.1
Connolly, S. R., Bellwood, D. R., and Hughes, T. P. (2003). Indo-Pacific biodiversity of coral reefs: deviations from a mid-domain model. Ecology 84, 2178–2190. doi: 10.1890/02-0254
Cornell, H. V., Karlson, R. H., and Hughes, T. P. (2007). Scale-dependent variation in coral community similarity across sites, islands, and island groups. Ecology 88, 1707–1715. doi: 10.1890/06-0742.1
Cowman, P. F., and Bellwood, D. R. (2012). The historical biogeography of coral reef fishes: global patterns of origination and dispersal. J. Biogeogr. 40, 209–224. doi: 10.1111/jbi.12003
Cowman, P. F., and Bellwood, D. R. (2013). The historical biogeography of coral reef fishes: global patterns of origination and dispersal. J. Biogeogr. 40, 209–224.
Delrieu-Trottin, E., Hartmann-Salvo, H., Saenz-Agudelo, P., Landaeta, M. F., and Pérez-Matus, A. (2022). DNA reconciles morphology and colouration in the drunk blenny genus Scartichthys (Teleostei: Blenniidae) and provides insights into their evolutionary history. J. Fish Biol. 100, 507–518. doi: 10.1111/jfb.14960
Edgar, G. J., Alexander, T. J., Lefcheck, J. S., Bates, A. E., Kininmonth, S. J., Thomson, R. J., et al. (2017). Abundance and local-scale processes contribute to multi-phyla gradients in global marine diversity. Sci. Adv. 3:e1700419. doi: 10.1126/sciadv.1700419
Fernández-Cisternas, I., Majlis, J., Ávila-Thieme, M. I., Lamb, R. W., and Pérez-Matus, A. (2021). Endemic species dominate reef fish interaction networks on two isolated oceanic islands. Coral Reefs 40, 1081–1095. doi: 10.1007/s00338-021-02106-w
Floeter, S. R., Bender, M. G., Siqueira, A. C., and Cowman, P. F. (2018). Phylogenetic perspectives on reef fish functional traits. Biol. Rev. 93, 131–151. doi: 10.1111/brv.12336
Ford, B. M., and Roberts, J. D. (2020). Functional traits reveal the presence and nature of multiple processes in the assembly of marine fish communities. Oecologia 192, 143–154. doi: 10.1007/s00442-019-04555-1
Francisco-Ramos, V., and Arias-González, J. E. (2013). Additive partitioning of coral reef fish diversity across hierarchical spatial scales throughout the caribbean. PLoS One 8:e78761. doi: 10.1371/journal.pone.0078761
Froese, R., and Pauly, D. (2014). Fishbase [Online]. Available online at: www.fishbase.org (accessed June 15 2018).
Gajdzik, L., Aguilar-Medrano, R., and Frederich, B. (2019). Diversification and functional evolution of reef fish feeding guilds. Ecol. Lett. 22, 572–582. doi: 10.1111/ele.13219
Halpern, B. S., and Floeter, S. R. (2008). Functional diversity responses to changing species richness in reef fish communities. Mar. Ecol. Progr. Ser. 364, 147–156. doi: 10.3354/meps07553
He, F., Gaston, K. J., Connor, E. F., and Srivastava, D. S. (2005). The local-regional relationship: immigration, extinction, and scale. Ecology 86, 360–365.
Hemingson, C. R., and Bellwood, D. R. (2018). Biogeographic patterns in major marine realms: function not taxonomy unites fish assemblages in reef, seagrass and mangrove systems. Ecography 41, 174–182.
Hervé, M. (2021). RVAideMemoire: Testing and Plotting Procedures for Biostatistics, in: R package version 0.9-81.
Hooper, D. U., Chapin Iii, F. S., Ewel, J. J., Hector, A., Inchausti, P., Lavorel, S., et al. (2005). Effects of biodiversity on ecosystem functioning: a consensus of current knowledge. Ecol. Monogr. 75, 3–35.
Hsieh, T. C., Ma, K. H., and Chao, A. (2016). iNEXT: an R package for rarefaction and extrapolation of species diversity (Hill numbers). Methods Ecol. Evol. 7, 1451–1456.
Jones, G. P. (2013). Ecology of rocky reef fish of northeastern New Zealand: 50 years on. N. Z. J. Mar. Freshw. Res. 47, 334–359. doi: 10.11646/zootaxa.4878.3.2
Kesner-Reyes, K., Segschneider, J., Garilao, C., Schneider, B., Rius-Barile, J., Kaschner, K., et al. (2019). AquaMaps Environmental Dataset: Half-Degree Cells Authority File (HCAF ver.7, 10/2019). Available online at: https://www.aquamaps.org/main/envt_data.php (accessed December 21, 2021).
Kraft, N. J. B., Valencia, R., and Ackerly, D. D. (2008). Functional traits and niche-based tree community assembly in an amazonian forest. Science 322, 580–582.
Laliberte, E., and Legendre, P. (2010). A distance-based framework for measuring functional diversity from multiple traits. Ecology 91, 299–305. doi: 10.1890/08-2244.1
Longo, G. O., Ferreira, C. E., and Floeter, S. R. (2014). Herbivory drives large-scale spatial variation in reef fish trophic interactions. Ecol. Evol. 4, 4553–4566. doi: 10.1002/ece3.1310
Martins, G. M., Arenas, F., Neto, A. I., and Jenkins, S. R. (2012). Effects of fishing and regional species pool on the functional diversity of fish communities. PLoS One 7:e44297. doi: 10.1371/journal.pone.0044297
Mason, N. W. H., Mouillot, D., Lee, W. G., and Wilson, J. B. (2005). Functional richness, functional evenness and functional divergence: the primary components of functional diversity. Oikos 111, 112–118.
McLean, M., Mouillot, D., Lindegren, M., Villéger, S., Engelhard, G., Murgier, J., et al. (2019). Fish communities diverge in species but converge in traits over three decades of warming. Glob. Change Biol. 25, 3972–3984. doi: 10.1111/gcb.14785
McLean, M., Stuart-Smith, R. D., Villeger, S., Auber, A., Edgar, G. J., Macneil, M. A., et al. (2021). Trait similarity in reef fish faunas across the world’s oceans. Proc. Natl. Acad. Sci. U.S.A. 118:e2012318118. doi: 10.1073/pnas.2012318118
Nash, K. L., Welsh, J. Q., Graham, N. A., and Bellwood, D. R. (2015). Home-range allometry in coral reef fishes: comparison to other vertebrates, methodological issues and management implications. Oecologia 177, 73–83. doi: 10.1007/s00442-014-3152-y
Navarrete, A. H., Lagos, N. A., and Ojeda, F. P. (2014). Latitudinal diversity patterns of Chilean coastal fishes: searching for causal processes. Revista Chilena de Historia Natural 87, 1–11.
Ojeda, F. P., Labra, F., and Muñoz, A. (2000). Biogeographic patterns of Chilean littoral fishes. Revista Chilena de Historia Natural 73, 625–641.
Oksanen, J., Kindt, R., Legendre, P., O’hara, B., Stevens, M., and Oksanen, M. (2007). The vegan package. Community Ecology package. Available online at: http://www.R-project.Org (accessed October, 2008).
Parravicini, V., Kulbicki, M., Bellwood, D., Friedlander, A., Arias-Gonzalez, J., Chabanet, P., et al. (2013). Global patterns and predictors of tropical reef fish species richness. Ecography 36, 1254–1262. doi: 10.1371/journal.pone.0056245
Pérez-Matus, A., Carrasco, S. A., Gelcich, S., Fernandez, F., and Wieters, E. A. (2017a). Exploring the effects of fishing pressure and upwelling intensity over subtidal kelp forest communities in Central Chile. Ecosphere 8:e01808.
Pérez-Matus, A., Ospina-Alvarez, A., Camus, P. A., Carrasco, S. A., Fernandez, M., Gelcich, S., et al. (2017b). Temperate rocky subtidal reef community reveals human impacts across the entire food web. Mar. Ecol. Progr. Ser. 567, 1–16.
Pérez-Matus, A., Ferry-Graham, L. A., Cea, A., and Vásquez, J. A. (2007). Community structure of temperate reef fishes in kelp dominated subtidal habitats of northern Chile. Mar. Freshw. Res. 58, 1069–1085.
Pérez-Matus, A., Pledger, S., Díaz, F. J., Ferry, L. A., and Vásquez, J. A. (2012). Plasticity in feeding selectivity and trophic structure of kelp forest associated fi shes from northern Chile. Revista Chilena de Historia Natural 85, 29–48. doi: 10.4067/s0716-078x2012000100003
Pérez-Matus, A., Ramirez, F., Eddy, T. D., and Cole, R. (2014). Subtidal reef fish and macrobenthic community structure at the temperate Juan Fernandez Archipelago, Chile. Latin Am. J. Aquatic Res. 42, 814–826. doi: 10.3856/vol42-issue4-fulltext-9
Pérez-Matus, A., and Shima, J. S. (2010). Disentangling the effects of macroalgae on the abundance of temperate reef fishes. J. Exp. Mar. Biol. Ecol. 388, 1–10.
Ramírez, F., Pérez-Matus, A., Eddy, T. D., and Landaeta, M. F. (2013). Trophic ecology of abundant reef fish in a remote oceanic island: coupling diet and feeding morphology at the Juan Fernandez Archipelago, Chile. J. Mar. Biol. Assoc. UK 93, 1457–1469.
Randall, J. E. (1998). Zoogeography of shore fishes of the Indo-Pacific region. Zool. Stud. 37, 227–268.
Renema, W., Bellwood, D., Braga, J., Bromfield, K., Hall, R., Johnson, K., et al. (2008). Hopping hotspots: global shifts in marine biodiversity. Science 321, 654–657. doi: 10.1126/science.1155674
Ricklefs, R. E. (1987). Community diversity: relative roles of local and regional processes. Science 235, 167–171. doi: 10.1126/science.235.4785.167
Siqueira, A. C., Morais, R. A., Bellwood, D. R., and Cowman, P. F. (2020). Trophic innovations fuel reef fish diversification. Nat. Commun. 11:2669.
Smith, H. L., Anderson, M. J., Gillanders, B. M., and Connell, S. D. (2014). Longitudinal variation and effects of habitat on biodiversity of Australasian temperate reef fishes. J. Biogeogr. 41, 2128–2139. doi: 10.1111/jbi.12359
Stuart-Smith, R. D., Bates, A. E., Lefcheck, J. S., Duffy, J. E., Baker, S. C., Thomson, R. J., et al. (2013). Integrating abundance and functional traits reveals new global hotspots of fish diversity. Nature 501, 539–542. doi: 10.1038/nature12529
Tapia, F. J., Largier, J. L., Castillo, M., Wieters, E. A., and Navarrete, S. A. (2014). Latitudinal discontinuity in thermal conditions along the nearshore of central-northern Chile. PLoS One 9:e110841. doi: 10.1371/journal.pone.0110841
Tittensor, D. P., Mora, C., Jetz, W., Lotze, H. K., Ricard, D., Berghe, E. V., et al. (2010). Global patterns and predictors of marine biodiversity across taxa. Nature 466, 1098–1101. doi: 10.1038/nature09329
Tuya, F., Wernberg, T., and Thomsen, M. S. (2011). The relative influence of local to regional drivers of variation in reef fishes. J. Fish Biol. 79, 217–234.
Veach, V., Di Minin, E., Pouzols, F. M., and Moilanen, A. (2017). Species richness as criterion for global conservation area placement leads to large losses in coverage of biodiversity. Diversity Distribut. 23, 715–726.
Villéger, S., Mason, N. W., and Mouillot, D. (2008). New multidimensional functional diversity indices for a multifaceted framework in functional ecology. Ecology 89, 2290–2301. doi: 10.1890/07-1206.1
Violle, C., Navas, M.-L., Vile, D., Kazakou, E., Fortunel, C., Hummel, I., et al. (2007). Let the concept of trait be functional! Oikos 116, 882–892.
Violle, C., Reich, P. B., Pacala, S. W., Enquist, B. J., and Kattge, J. (2014). The emergence and promise of functional biogeography. Proc. Natl. Acad. Sci. U.S.A. 111, 13690–13696. doi: 10.1073/pnas.1415442111
Wellenreuther, M., Syms, C., and Clements, K. D. (2008). Consistent spatial patterns across biogeographic gradients in temperate reef fishes. Ecography 31, 84–94.
Wiescher, P. T., Pearce-Duvet, J. M. C., and Feener, D. H. (2012). Assembling an ant community: species functional traits reflect environmental filtering. Oecologia 169, 1063–1074. doi: 10.1007/s00442-012-2262-7
Witman, J. D., Etter, R. J., and Smith, F. (2004). The relationship between regional and local species diversity in marine benthic communities: a global perspective. Proc. Natl. Acad. Sci. U.S.A. 101, 15664–15669. doi: 10.1073/pnas.0404300101
Keywords: functional diversity, fish species traits, diversity index, longitudinal gradient of diversity, environmental filters
Citation: Pérez-Matus A, Neubauer P, Shima JS and Rivadeneira MM (2022) Reef Fish Diversity Across the Temperate South Pacific Ocean. Front. Ecol. Evol. 10:768707. doi: 10.3389/fevo.2022.768707
Received: 01 September 2021; Accepted: 24 February 2022;
Published: 24 March 2022.
Edited by:
Simone Baldanzi, Universidad de Valparaiso, ChileReviewed by:
Jonathan Belmaker, Tel Aviv University, IsraelAnthony Bernard, South African Institute for Aquatic Biodiversity, South Africa
Copyright © 2022 Pérez-Matus, Neubauer, Shima and Rivadeneira. This is an open-access article distributed under the terms of the Creative Commons Attribution License (CC BY). The use, distribution or reproduction in other forums is permitted, provided the original author(s) and the copyright owner(s) are credited and that the original publication in this journal is cited, in accordance with accepted academic practice. No use, distribution or reproduction is permitted which does not comply with these terms.
*Correspondence: Alejandro Pérez-Matus, aperez@bio.puc.cl
†ORCID: Alejandro Pérez-Matus, orcid.org/0000-0001-9591-6721; Jeffrey S. Shima, orcid.org/0000-0001-5770-4859