- 1Grupo de Investigación en Genética Aplicada (GIGA), Instituto de Biología Subtropical (IBS), Universidad Nacional de Misiones y Consejo Nacional de Investigaciones Científicas y Técnicas (UNAM-CONICET), Posadas, Argentina
- 2CONICET—Consejo Nacional de Investigaciones Científicas y Tecnológicas, Buenos Aires, Argentina
- 3Departamento de Microbiología, Inmunología, Biotecnología y Genética, Cátedra de Genética Forense y Servicio de Huellas Digitales Genéticas, Facultad de Farmacia y Bioquímica, Universidad de Buenos Aires, Buenos Aires, Argentina
- 4Centro de Bioinvestigaciones (CeBio), Universidad Nacional del Noroeste de la Provincia de Buenos Aires (UNNOBA-CICBA)/Centro de Investigaciones y Transferencia del Noroeste de la Provincia de Buenos Aires CITNOBA (UNNOBA-CONICET), Pergamino, Argentina
- 5Centro Nacional de Pesquisa e Conservação de Primatas Brazileiros, Instituto Chico Mendes de Conservação da Biodiversidade (ICMBio/CPB), Cabedelo, Brazil
- 6Departamento de Genética e Morfologia, Universidade de Brasília, Brasília, Brazil
- 7Escola de Ciências da Saúde e da Vida, Pontifícia Universidade Católica do Rio Grande do Sul, Porto Alegre, Brazil
Black and gold howler monkeys (Alouatta caraya) inhabit several eco-regions in South America with the highest population densities in riverine forests. Dam construction for electricity production represents a severe human alteration of ecosystems with consequences for primate conservation. To evaluate the possible loss of genetic diversity in A. caraya, we analysed and compared the genetic structure of the species across 22 study sites in Argentina (14), Paraguay (1), and Brazil (7). Four of these study sites (referred to as flooded) were sampled before dam-linked flooding which most likely caused a drastic decline or functional extinction of these populations. The genetic variability of 256 individuals was evaluated using 10 autosomal microsatellites (STRs) and 112 individuals by sequencing a fragment of 507 bp of mtDNA. DNA was extracted from tissue, blood, and faecal samples. Significantly higher values of genetic variability were observed for the flooded populations both in mtDNA and STRs. Population genetic structure showed a K = 1, 2, or 5 depending on the method, separating Argentinian and Paraguayan sites from Brazilian sites and, in the case of K = 5, two clusters were mostly represented by flooded populations. Isolation-by-distance analyses showed that geographic distances influence gene flow. Analytical methods, such as Pairwise Fst’s and Nei’s and regression model of Harpending and Ward, were concordant in detecting significant genetic structuring between flooded and remaining sites examined. Although some sites have very low sample sizes, these samples are of great importance since these sampling sites are currently flooded. Our results show that the study sites where dams were built had the greatest genetic diversity. As A. caraya is currently severely threatened by yellow fever outbreaks, the remaining populations may be more vulnerable to disease outbreaks due to impoverished genetic variability. Accordingly, it is essential to implement management actions to conserve the remaining populations. Our results underline the importance for Environmental Impact Assessments (EIA) to include data on the genetic structure of species in the affected sites prior to their alteration or destruction. These genetic data are also remarkably important for determining where to relocate specific individuals to help avoid biodiversity loss.
Introduction
Hydroelectric dams have been built in primate habitat countries since the 1950s. More than 45,000 large dams (≥ 15 m tall) have been built worldwide, affecting > 50% of the largest rivers of the world, including the most biogeographically diverse ones (World Commission on Dams [WCD], 2000; Nilsson et al., 2005).
Dams store river water which, when released, powers turbines to generate electricity. Although the media commonly refer to dams as the largest source of renewable electricity in the world and are popularly considered as clean or green energy (Gibson et al., 2017), the alterations by dams of the natural flow of rivers may cause, among other disturbances, extensive modification to aquatic communities (Poff et al., 1997; Lemly et al., 2000; Prowse et al., 2002; Tockner and Stanford, 2002) and promote extensive deforestation and fragmentation of pristine forests (Finer and Jenkins, 2012). Moreover, the associated flooding of often extensive tracts of land can also destroy adjacent terrestrial ecosystems (Bauni et al., 2015) and cause anoxia which releases greenhouse gases and generates sedimentation (Rosa et al., 2004; Fearnside and Pueyo, 2012).
Additionally, many conflicts have risen from the resettlement programs associated with the inundation of indigenous and local communities. Such programs can result in adverse human health effects and substantial changes in land use patterns (Indrabudi et al., 1998; Gillet and Tobias, 2002; Richter et al., 2010; Verdum, 2012).
In recent decades, Environmental Impact Assessments (EIA) have been used to predict the potential adverse effects of dams prior to their construction (Robinson, 1992; Tullos, 2008). However, compliance with the EIA recommendations is rarely mandatory, and as a result, is not always incorporated into the final design. Hence, biodiversity impact mitigation is unlikely to occur (McAllister et al., 2001; Rodrigues, 2006). For example, populations of anuran species became extinct due to the construction of hydroelectric reservoirs in the Casca and Manso rivers in Brazil (Brandao and Araujo, 2008; Lima et al., 2015; Silva et al., 2018) despite EIA warnings.
The long-term effects of dams on biodiversity have rarely been evaluated. The World Commission on Dams (WCD) produced the most comprehensive review of dam impacts, but it was dissolved in 2001. Benchimol and Peres (2015) assessed the long-term impacts (26 years later) of a mega-hydroelectric dam on medium and large-bodied vertebrates in Central Brazilian Amazon. They found that most islands formed by the dam cannot ensure the local persistence of even a modest fraction of the original middle and large-sized vertebrate fauna.
Primates are clear candidates to be affected by dam construction, not only because of the number of islands and the areas of riverside forest lost as a result of flooding, but also with indirect impacts even before the construction itself, such as deforestation caused by clearing of the area, constructions of roads, and hunting of primates for food or capture for illegal trade (Rodrigues, 2006; Harrison-Levine et al., 2019). Primates are strongly affected by anthropogenic activities that cause loss, fragmentation, reduction, or isolation of native forest habitats (Michalski and Peres, 2005; Estrada et al., 2017), triggering secondary processes such as dispersal restrictions, resource depletion, and pathogen exposure (Oklander et al., 2010, 2017; Lecompte et al., 2017). In combination, these can reduce genetic diversity, decrease the adaptive potential of populations, increase local extinction risks, and affect long-term species survival (Frankham, 2005).
Although there are several studies of the effects of dams on primates, most have been carried out in the short term (Kinzey et al., 1988; Terborgh et al., 1997, 2001; Cosson et al., 1999; Ferrari et al., 2004). However, Benchimol and Peres (2014) found evidence of the detrimental impacts of dams on a primate community 21 years after dam construction was completed. They found that primate species richness significantly decreased according to the area and forest cover of the remaining islands in the flooded landscape, with howlers (Alouatta macconnelli) and brown capuchin monkeys (Sapajus apella) showing greater tolerance to habitat changes than other species. The rescue of fauna and their transfer to adjacent areas is a procedure used widely during the filling of the reservoirs associated with hydroelectric dams and is commonly promoted as a conservation measure. However, this procedure further destabilises adjacent areas that are already occupied by conspecifics, thereby increasing population densities (Woodford and Rossiter, 1993; Schneider, 2001; Jones et al., 2016). Many thousands of primate rescues have been conducted during and/or after flooding, but most of them have been poorly documented and very few have had subsequent follow-ups (Fournier-Chambrillon et al., 2000; Schneider, 2001). Rodrigues (2006) provides empirical evidence that the fate of the majority of individuals rescued and transported to unknown habitats is death, affirming that wildlife rescue projects are a fallacy or an unscientific measure to ameliorate the ecological impact of filling a dam in terms of public opinion.
Black and gold howler monkeys (Alouatta caraya, Humboldt, 1812) inhabit several ecoregions in Brazil, Argentina, Paraguay, and Bolivia (Brown and Zunino, 1994; Lynch Alfaro et al., 2015) and show a remarkable variation in density across their distribution (Zunino et al., 2001; Bicca-Marques et al., 2008; Agostini et al., 2015; Jardim et al., 2020; Gorostiaga et al., 2021). These differences could be attributed to resource availability, interspecific competition, or the effect of epidemic diseases like yellow fever (Holzmann et al., 2010; Kowalewski et al., 2011; Lynch Alfaro et al., 2015; Oklander et al., 2019). However, the density and group size of A. caraya in the island system along the Paraná River under low-level human impact was found to be the highest (3.6 individuals per ha) anywhere, indicating the importance of this environment for the species (Aguiar et al., 2009; González et al., 2014). These populations also show special demographic features, with multimale groups being more common (Rumiz, 1990; Zunino et al., 2001). Demographic records show that, in continuous areas, both females and males leave their natal groups (Rumiz, 1990), but habitat fragmentation modifies the A. caraya dispersal pattern from bisexual to male biased (Oklander et al., 2010).
Although the International Union for Conservation of Nature (IUCN) classifies A. caraya as “Near Threatened” Bicca-Marques et al. (2021), Oklander et al. (2019) recommended recategorisation to “Vulnerable” based on the limited genetic variability reported in the southernmost populations, the dramatic reduction in density, and the loss of entire populations in the Atlantic forest of Brazil and Misiones Province in Argentina after two sylvatic yellow fever outbreaks (2008–2009 and 2016–2019; Holzmann et al., 2010; Almeida et al., 2012; Bicca Marques et al., 2017; Ministério da Saúde do Brasil [MS-BR], 2018, 2019, 2021). Howlers are described as the most susceptible genus to yellow fever virus, developing clinical illness and fatal disease few days after infection (Laemmert and Kumm, 1950). As such, as sentinels for virus detection (Klitting et al., 2018), they have great importance for epidemiological surveillance, allowing the early establishment of control and prevention measures, such as targeted human vaccination campaigns in adjacent areas contributing to public health (Bicca-Marques and de Freitas, 2010; Siconelli et al., 2019).
The genetic structure of primate populations is affected by several factors, including effective population size, dispersal distances, genetic drift, and demographic events such as bottlenecks (Di Fiore, 2009). The decrease in the population size generated by local extinctions or epizootic events implies a loss of genetic variability and fixation of allelic and/or haplotype variants due to the effect of genetic drift within small and/or isolated groups (Ayala-Burbano et al., 2017; Baden et al., 2019; Zhao et al., 2019). In this sense, it is interesting to study the genetic consequences derived from the high mortality of individuals in A. caraya after the flooding of several sites within its distribution.
Given the projection that there will be a rise in dam construction in primate range countries for the next 10–20 years (Tundisi et al., 2014), this study aimed to use molecular tools that use genetic markers with different mutation rates and inheritance characteristics to elucidate genetic consequences of high mortality of A. caraya after dam construction, analyzing 22 sites along its distribution in Argentina, Paraguay, and Brazil. Specifically, alterations in the genetic structure of the population at different time scales, with the mtDNA Control Region for ancient genetic processes and microsatellite markers (STRs) for recent population genetic processes (Oklander et al., 2010, 2017; Kolleck et al., 2013; Wang et al., 2019). The precise evaluation of the current genetic structure and gene flow among the populations in these sites is needed to contribute to the development of management strategies aimed at ensuring the long-term survival of this species, which is a unique ecological sentinel.
We are interested in the comparison between the locally extinct and remaining populations to analyse historical genetic variability and to identify variability in remaining populations. If populations were already isolated prior to flooding, we expect to find: (1) genetically differentiated populations, (2) that the genetic variability of some populations was irreversibly lost due to dam constructions, and (3) that remaining populations have low genetic variation perhaps making them more vulnerable to future disease outbreaks. Our findings may help in the detection of remaining A. caraya populations with important genetic variation to conserve and suggest management actions to preserve them.
Materials and Methods
Study Sites Description and Sampling
Black-and-gold howler monkeys were sampled from three countries: Argentina, Paraguay, and Brazil, with a total of 256 individuals in 22 populations (Table 1 and Figure 1). Tissue or blood samples were obtained by the capture of adult individuals in the context of EIA or during flooding for dam construction in four sites (GO.BR, MT.BR, MS1.BR, and MS2.BR; Table 1 and Figures 1, 2). Consequently, we are analysing individuals that were relocated or dead.
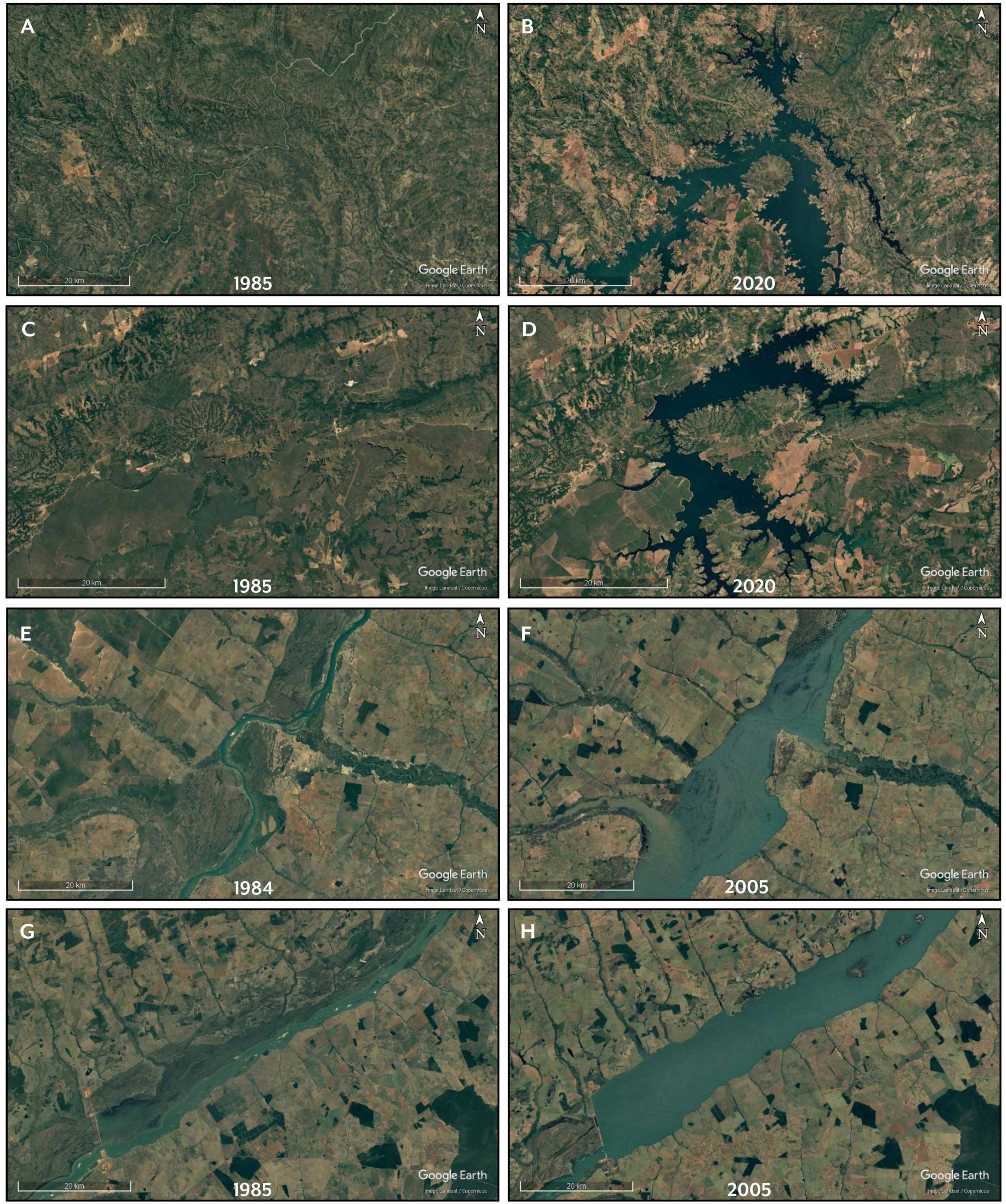
Figure 2. Flooded study sites before dam construction (1985) and currently. (A) Tocantins River and (B) of Serra da Mesa Hydroelectric Power Plant in Goiás state, Brazil (site GO.BR). (C) Manso River and (D) Represa de Manso Hydroelectric Power Plant, in Mato Grosso state, Brazil (site MT.BR). (E,G) Parana River, (F,H) Engenheiro Sérgio Motta Hydroelectric Plant (Porto Primavera), in Mato Groso do Sul state, Brazil (sites MS1.BR and MS2.BR).
Samples from GO.BR were collected in the EIA of Serra da Mesa Hydroelectric Power Plant, located in the Alto Tocantins Basin, Goiás state, Brazil (GO). This dam is located on the main course of the Tocantins River, and its reservoir is the largest in Brazil in terms of water volume, with 54.4 billion m3 and an area of 1,784 km2. Samples from MT.BR were collected in the EIA of the Represa de Manso hydroelectric dam located on the Manso River, a tributary of the Cuiabá River, Mato Grosso state, Brazil. The reservoir has a volume of 7.3 billion m3 and an area of 427 km2. During construction, many human families were displaced from the area now covered by the reservoir, and the submerged, decaying vegetation has had a negative impact on water quality and has affected edible fish stocks (Hylander et al., 2006). Samples MS1.BR and MS2.BR were collected during the flooding of the Engenheiro Sérgio Motta Hydroelectric Power Plant (also called Porto Primavera Hydroelectric Power Plant) on the Paraná River, 28 km upstream from the confluence with the Paranapanema River. This dam lies between the Municipality of Porto Primavera in the eastern part of the Mato Grosso do Sul (MS) state and southwestern-most São Paulo state. This dam has the greatest surface area in Brazil, being 10,186.20 m long with a reservoir formed by water from the Paraná River that has a flooded area of 2,250 km2. The other three samples’ sites in Brazil belong to the states of Paraná (PR), São Pablo (SP), and Minas Gerais (MG), in areas where no dams have been built.
Tissue or faecal material was obtained from monkeys in sites where the habitats remain intact and accessible for sampling (Table 1; Oklander et al., 2017, 2020). For some analyses, the following study sites in Brazil GO.BR, MT.BR, MS1.BR, and MS2.BR were grouped as “Flooded.” The Brazilian sites PR.BR, SP.BR, MG.BR, and all sites in Paraguay and Argentina were grouped as “Remaining” (either Rem.BR or Rem.PY.AR; Table 1).
Sampling complied with Argentine, Paraguayan, and Brazilian laws and was conducted with permission from the National Resources Board (Brazil: SISBIO n. 19927-12 by ICMBio, Paraguay: Ministerio de Salud Pública, Mercosur/GMC/Res. n. 50/08, Argentina: CONICET n. 11420110100322CO and Ministry of Ecology, Misiones province, Argentina n. 9910-00086/17). We adhered to the American Society of Primatologists’ Principles for the Ethical Treatment of Primates.1
DNA Extraction
DNA was extracted from faecal samples using the QIAamp DNA Stool Mini Kit (QIAGEN, Valencia, United States), according to the manufacturer’s protocols, with slight modifications (Oklander et al., 2017). DNA was extracted from tissue and blood samples using standard SDS/Proteinase K digestion followed by phenol:chloroform organic extraction and Microcon P-100 counter-dialysis filters (Green and Sambrook, 2012).
Microsatellite or Short Tandem Repeats Amplification
Ten microsatellites or Short Tandem Repeats (STRs) characterised for A. caraya and previously used in population genetics studies, were amplified from each sample: AC14, AC17, AC45, TGMS1, TGMS2, D8S165, D17S804, LL1118, LL157, and AB07 (Tomer et al., 2002; Di Fiore and Fleischer, 2004; Gonçalves et al., 2004; Oklander et al., 2007; Supplementary File 1). Genotyping PCRs were performed using recommendations from previous studies, with a final volume of 25 μl using 5–10 ng of DNA template for tissue samples or 5 μl of the extraction of faecal samples, 20 mM Tris–HCl, 50 mM KCl, 1.5 mM MgCl2, 0.2 mM each dNTP, 1 U of GoTaq DNA polymerase (Promega, Madison, United States), 1 pmol of each forward primer bearing an M13 tail, 4 pmol of each reverse primer, and 4 pmol of M13 labelled with fluorescent dyes (6-FAM, HEX or NED) on its 5′ end (Schuelke, 2000; Oklander et al., 2007). Singleplex PCRs were carried out for faecal samples and multiplex of 3 or 4 STRs for tissue and blood samples (Supplementary File 1).
All amplifications were performed in a Gen Amp ABI 9,700 machine (Thermo Fisher, Palo Alto, United States). PCR products labelled with different fluorochromes were combined and co-injected with HD400-ROX as an internal size standard to be separated by electrophoresis on an ABI PRISM 310 Genetic Analyzer (Thermo Fisher Scientific, Palo Alto, United States) or in MegaBACE 1000 (Pharmacia, Upsala, Seden) automated sequencer (Amersham Biosciences) according to the protocols of the manufacturer. Alleles were manually scored by performing a visual inspection of electropherograms after developing the bin panel for each locus in GeneMapper ID-X v. 1.2 (Thermo Fisher, Palo Alto, United States) or software Genetic Profiler 2.2 (GE Healthcare).
For DNA extracted from faecal samples, PCR and sizing were repeated two times (in the case of a heterozygous genotype call) or four times (in case of a homozygous genotype call) to minimise possible genotyping errors due to allelic dropout (Pritchard et al., 2000; Peakall et al., 2003). We only recorded an allele if it was observed at least two times in different amplifications from the same DNA extract. All amplification assays included negative controls. Every step of the lab work was carried out in specific laboratory spaces (DNA extraction room and PCR room), inside laminar flow hoods with negative pressure, and using aerosol-resistant filter tips to avoid between sample cross-contamination.
Short Tandem Repeat Statistical Analysis
Genetic Diversity
Genotypes were screened for null-alleles and to discriminate between errors in allele frequency estimates caused by null-alleles, allele dropout, or stutter bands using Micro-Checker v2.2.332 (Van Oosterhout et al., 2004).
Numbers of different alleles (Na), effective and private alleles (Ne), and observed heterozygosity (Ho) were computed using GenAlEx v6.5 software (Peakall and Smouse, 2012) for each locus and study site. Deviations from Hardy-Weinberg equilibrium (HWE) were assessed by employing an exact test using Arlequin v 3.5 software (Excoffier and Lischer, 2010).
Allelic richness (AR) and gene diversity (Hs) were calculated for each locus in a population using Fstat software v2.9.4 (Goudet, 2003):
where Ni represents the number of alleles of type i between the 2N genes and n is sample size based on a minimum sample size of 1 diploid individual. To account for differing sample sizes, we computed a rarefied measure of allelic diversity (Allelic Richness) in Fstat based on a standard sample size of n = 1.
where n represents the number of alleles, p the allele frequency, and Ho the observed heterozygosity.
Based on the results of the Structure analysis (see below), and to increase the n between comparisons, we gather the study sites into three groups: 1-Flooded, 2-remaining in Brazil (Rem.BR), and 3-remaining in Paraguay and Argentina (Rem. PY.AR, Table 1).
For statistical comparison, AR, Hs, and Ho between these groups were compared using Fstat (Goudet, 2003), and Na and Ne were compared with the Welch t-test (Excel).
Pairwise Fst’s and Nei’s average number of pairwise differences between study sites (Nei, 1972) and the statistical comparison were determined with Arlequin v3.5 software and plotted with R-lequin (Excoffier and Lischer, 2010).
Structure Analysis
The genetic structure was evaluated using the non-spatial Bayesian clustering with the Structure v.2.3.4 program (Pritchard et al., 2000). A series of 20 independent runs per K (ranging from 1 to 7) was conducted using the admixture model with correlated allele frequencies, without prior information about sampling locations, independent allele frequencies, and 1,000,000 Monte Carlo-Markov iterations after a burn-in of 50,000 replicates. Several K-estimation methods were tested, including the Pr[X|K] method (Pritchard et al., 2000), the ΔK method (Evanno et al., 2005), and the parsimony method (Wang, 2019) using the KFinder software (Wang, 2019). A bar plot was constructed with the software pophelper (Francis, 2016).
Mitochondrial Amplification
We amplified a 507-bp fragment of the left domain of the mitochondrial DNA Control Region (mtDNA, CR) using primers How RA-1 (5′-CTACCATCAACACCCAAAGC-3′, Ascunce et al., 2007) and RC-BugioR (5′-CCAGGTTAAGAGGGTGATAGC-3′, Oklander et al., 2017). The amplified zone corresponds to the position 15,412–15,903 bp from the mitogenome published by Finstermeier et al. (2013) and deposited at Genbank: NC_021938. Amplification reactions were performed in a final volume of 25 μl containing 25 ng of DNA extractions, 1X of GoTaq buffer (Promega, United States), 1.75 mM of MgCl2, 0.2 mM of each dNTP, 1U GoTaq DNA polymerase (Promega, United States), 4 pmol of each primer, and 4 mg/ml of bovine serum albumin (BSA). Cycling parameters were an initial denaturation (5 min at 94°C) followed by 35–40 cycles of denaturation (1 min at 94°C), annealing (30 s at 50°C), and extension (1 min at 72°C), then a final extension step (3 min at 72°C). Sequencing was carried out using the same reverse and forward primers used for initial amplification. All samples were sequenced bidirectionally using the BigDye® Terminator v3.1 Cycle Sequencing Kit (Applied Biosystems, Foster City, CA) and visualised in an Applied Biosystems 3,500 Genetic Analyzer or the DYEnamic™ ETDye Terminator Cycle Sequencing Kit (GE Healthcare) on a MegaBACE 1,000 automated sequencer. Sequences quality was assessed with Sequencher 5.3 (LifeCodes, United States).
Mitochondrial Diversity and Structure Analysis
Sequences were edited and aligned using the CLUSTAL W algorithm (Thompson et al., 1994) in MEGA10.2.6 (Kumar et al., 2018).
Haplotype frequencies, the number of polymorphic sites (S), haplotypic (Hd), and nucleotidic (Pi) diversity were calculated in DNASP 5.0 (Librado and Rozas, 2009). Standard tests of selective neutrality and Tajima’s D (Tajima, 1989) and Fu’s FS (Fu, 1997) with 95% confidence intervals were computed in DNASP with 1,000 simulations and a neutral infinite-sites model assuming a large constant population size (Hudson, 1990). All these parameters were performed for each study site and for the three groups (Flooded, Rem.BR, and Rem.PY.AR, Table 2).
A haplotype network was built using Median-Joining (Bandelt et al., 1999), and implemented in POPART (Leigh and Bryant, 2015).
Pairwise Fst’s and Nei’s average number of pairwise differences between populations (Nei, 1972) were determined with Arlequin v3.5 software and plotted with R-lequin (Excoffier and Lischer, 2010). This analysis involved 112 nucleotide sequences, with all ambiguous positions removed for each sequence pair (pairwise deletion option). There were a total of 491 positions in the final dataset from the original 507 pb.
As there is evidence of hybridisation between A. caraya and A. guariba in some of the sampled sites (Aguiar et al., 2007; Agostini et al., 2008), we also carried out amplifications of the same mtDNA fragment in 4 samples of A. guariba (2 from Argentina and 2 from Brazil) in order to obtain the evolutionary relationships for these two taxa. The optimal Neighbor-Joining phylogenetic tree obtained shows reciprocal monophyly between taxons (Supplementary File 2), ruling out that the differences found in our study are due to hybridisation with this species.
Isolation-by-Distance Analyses
We conducted Mantel tests between genetic Fst (mtDNA and STRs) and geographic distances (in Km, computed from geographical coordinates) using the mantel function (method = “spearman,” permutations = 9,999) implemented in the “vegan” v2.4 ± 3 package (Dixon, 2003) in R. The proportion of shared alleles by pairs of populations was calculated with PopGenReport v2.2.2 package (Adamack and Gruber, 2014) in R. If geographic distances influence gene flow, we would expect the Mantel statistic to be significant (Chiappero et al., 2016).
Regression Model Analysis
R-matrix analysis and the regression model of Harpending and Ward (1982) D2 distances derived from R-matrix analysis (Harpending et al., 1973) were employed for gaining insights into the patterns of external gene flow and genetic drift due to isolation under the island model. The broken line is the expected relationship predicted by the model according to the formula hi = H (1-ri), where ri is the distance from the centroid. hi is the heterozygosity of population i, and H is heterozygosity of the total population according to Genocline v1.4 software (Peña et al., 2020). If gene flow from outside the region varies substantially from population to population, this linear relationship no longer holds. Isolated groups will be less heterozygous than the linear prediction and will therefore lie below the theoretical regression line, whereas populations receiving more gene flow from outside will be more heterozygous and lie above the line (Lakshmi et al., 2002). Consequently, the outliers above and below the theoretical regression line may give some insights into the population structure.
Results
Genetic Diversity and Demographic Parameters
For the majority of the samples (n = 243 or 94.9%), all 10 STRs loci were amplified. For the remaining 13 samples, a minimum of nine loci were amplified. We found no evidence of significant deviations from the Hardy-Weinberg equilibrium, with the 256 A. caraya individuals from 22 populations exhibiting moderate levels of microsatellite diversity (Table 1).
The highest population of genetic variability statistics were found in the four flooded study sites. The highest mean number of different alleles (NA), number of effective alleles (Ne), private alleles (PA), and observed heterozygosity (Ho) were found in the two study sites, now submerged by the Porto Primavera Hydroelectric Power Plant in Brazil (MS1.BR and MS2.BR; Table 1), and the highest mean gene diversity (Hs) and allelic richness (AR) in the other two (GO.BR, and MT.BR; Table 1 and Figures 1, 2). On the other hand, the lowest values were detected in the remaining study sites in Paraguay and Argentina (Table 1). A detailed analysis of Argentine and Paraguayan study sites is given in Oklander et al. (2017, 2020).
Mean NA, Ne, Ho, Hs, and AR showed significant differences between groups (AR p = 0.004, Ho p = 0.001, Hs p = 0.001, Na p = 0.0006, Ne p = 0.00009; Table 1).
The BLAST search of the 112 A. caraya 491-bp mtDNA fragment sequences as queries retrieved only mitochondrial sequences. Comparison showed an average identity of 94.20% (Alouatta caraya), 82.44% (Aluoatta guariba, Aluoatta palliate, and Aluoatta seniculus), 77.26% (Ateles sp.), 77.66% (Brachyteles arachnoides), 77.30% (Cebus olivaceus castaneus), 77.24% (Lagothrix sp.), 76.82% (Saimiri), and 77.21% (Sapajus sp.). Therefore, the presence of nuclear mitochondrial DNA sequences (NUMTs) in our fragment sequences can be considered negligible.
The 112 mtDNA sequences were grouped in 42 haplotypes, of which 21 were new to this study (Genbank accession numbers: OK033252-OK033272) and the rest of haplotypes were taken from Oklander et al. (2017; MF095740- MF095754). For the complete dataset, 80 polymorphic (segregating) sites, an overall haplotypic diversity of 0.963 ± 0.007, and an overall nucleotide diversity of Pi = 0.0338 ± 0.001 were observed. The median-joining network showed a complex pattern of relationship among observed haplotypes (Figure 3 and Supplementary File 2). The Flooded mtDNA variation, represented by the haplotypes Hap1-Hap11 (MS1.BR and MS2.BR), Hap20 (GO.BR), and Hap21-Hap22 (MT.BR) are well distributed along the network, showing a higher number of mutation steps among these 14 Control Region variants. For the rest of the remaining populations sampled in Brazil (Rem.BR) and Paraguay-Argentina (Rem.PY.AR), 8 and 21 haplotypes were obtained, respectively, with lower values of variation indices (S, Hd, and Pi; Table 2). Although the overall pattern of the network showed strong haplotype relationships, only one haplotype (Hap8) was shared between MS1 (Flooded) and PIM (Rem.PY.AR). Similarly, the general pattern of the haplotype network supports a relative demographic stability and absence of a recent population expansion, as suggested by the values obtained for the Fs of Fu and the D of Tajima (all p-values > 0.1; Table 2).
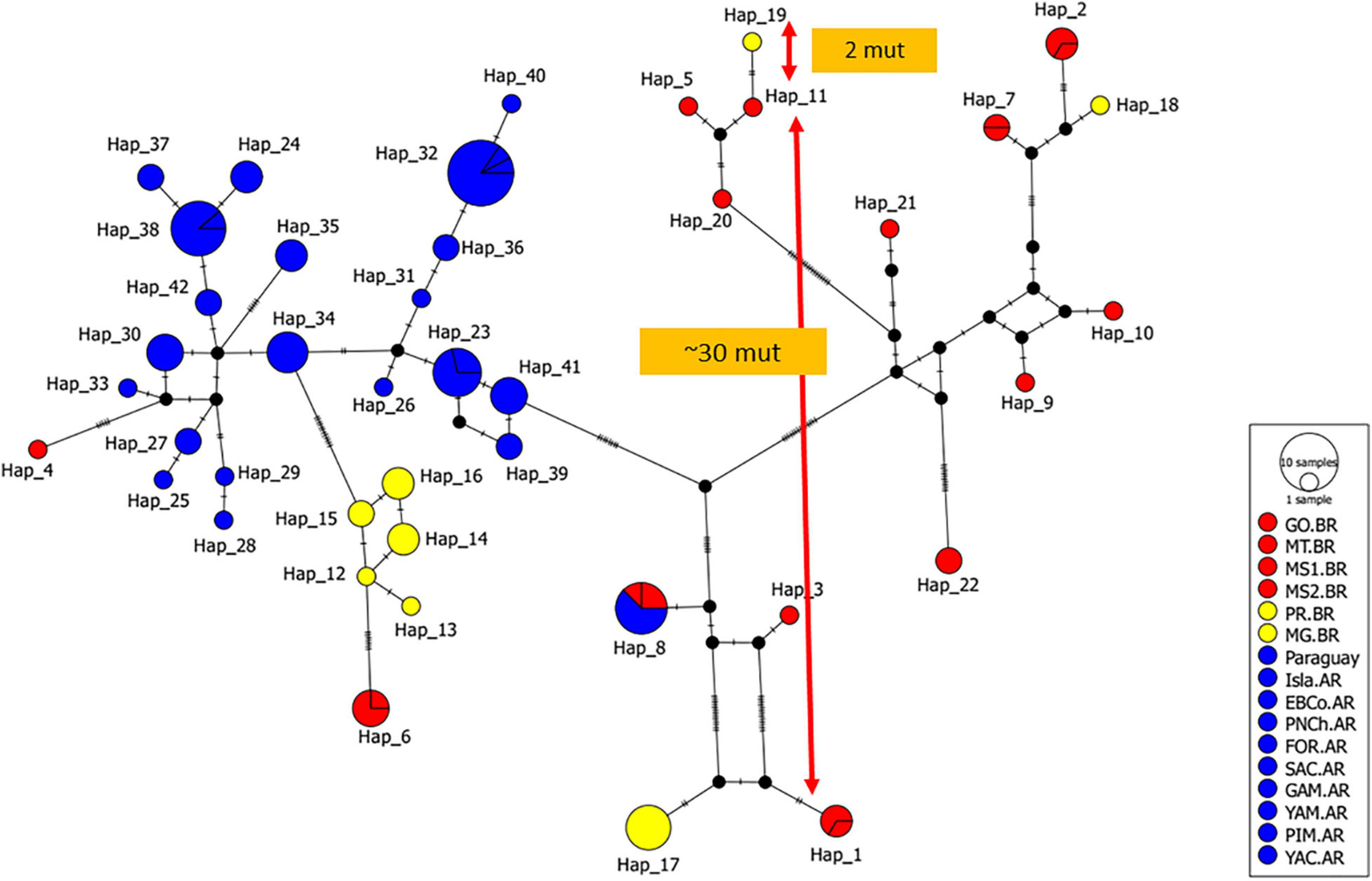
Figure 3. Median-Joining network for the 42 Control Region haplotypes (491 bp) observed in 16 study sites of black-and-gold howler monkeys (Table 1). Circle colours represent groups of study sites analysed, flooded (red), remaining in Brazil (Rem.BR, yellow), and remaining in Paraguay and Argentina (Rem.PY.AR, blue). Circle sizes are proportional to haplotypic frequencies; small black circles indicate median vectors; black lines indicate mutational steps.
Mantel statistic based on Spearman’s rank correlation rho showed significant results for mtDNA (r = 0.3847, p = 0.0023) and STRs (r = 0.4603, p = 0.0002) which implies an inverse proportion of effective dispersal to geographical distance.
However, for “short” distances (< 300 km) a wide range of Fsts is observed (from almost zero to 1; Figure 4), perhaps related to what is observed in the haplotype network. Particularly, close study sites with high genetic differentiation, up to ∼30 difference mutations (Hap 1 and 11 from MS1.BR and MS2.BR), and low differentiation (2 mutations) between haplotypes in distant sites (Hap 11 from MS2 and Hap 19 from MG.BR; Figure 3).
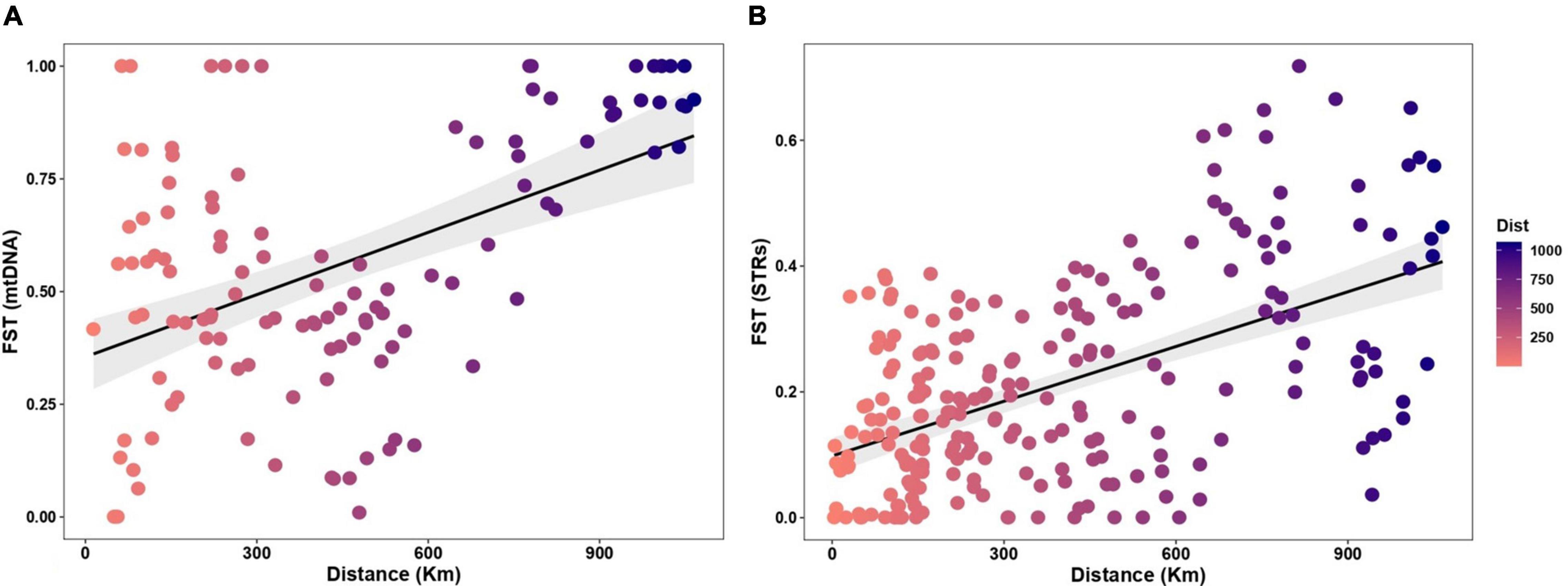
Figure 4. Linear regression lines showing the correlations among genetic (Fst) and geographic distances (km) as supported by the Mantel test, based on (A) mtDNA Control Region sequences of A. caraya and (B) 10 autosomal STRs.
Genetic Structure
The STRUCTURE results identified a genetically different number of clusters (K) depending on the method. The parsimony method only detected one cluster grouping in the 22 study sites (Figure 5; K = 1). The Evanno method results showed K = 2 with a clear separation between the remaining study sites of Argentina and Paraguay (Rem.PY.AR, represented as green in Figure 5; K = 2) from all other studied sites—all of which were in Brazil (represented as blue in Figure 5; K = 2). Finally, the Pr[X| K] method results detected five genetically differentiated clusters (K = 5) as best explaining the nuclear genetic variation observed in A. caraya. Two of these clusters are mostly represented by the four-flooded sites for dam construction coloured as yellow and blue in Figure 5, K = 5 (Flooded: GO.BR, MT.BR, MS1.BR, and MS2.BR), although the yellow cluster is still represented in the remaining MG:BR study site. The third cluster is represented in orange in Figure 5, K = 5, and is mostly represented in the remaining study sites in Brazil (PR.BR and SP.BR). Finally, the remaining Argentine and Paraguayan study sites were grouped in two clusters (green and grey) with this method (Figure 5; K = 5). These study sites, grouped as Rem.PY.AR, were previously studied in Oklander et al. (2017, 2020) and remain currently relatively undisturbed since no major infrastructure projects or deforestation have been carried out in these sites since sampling occurred.
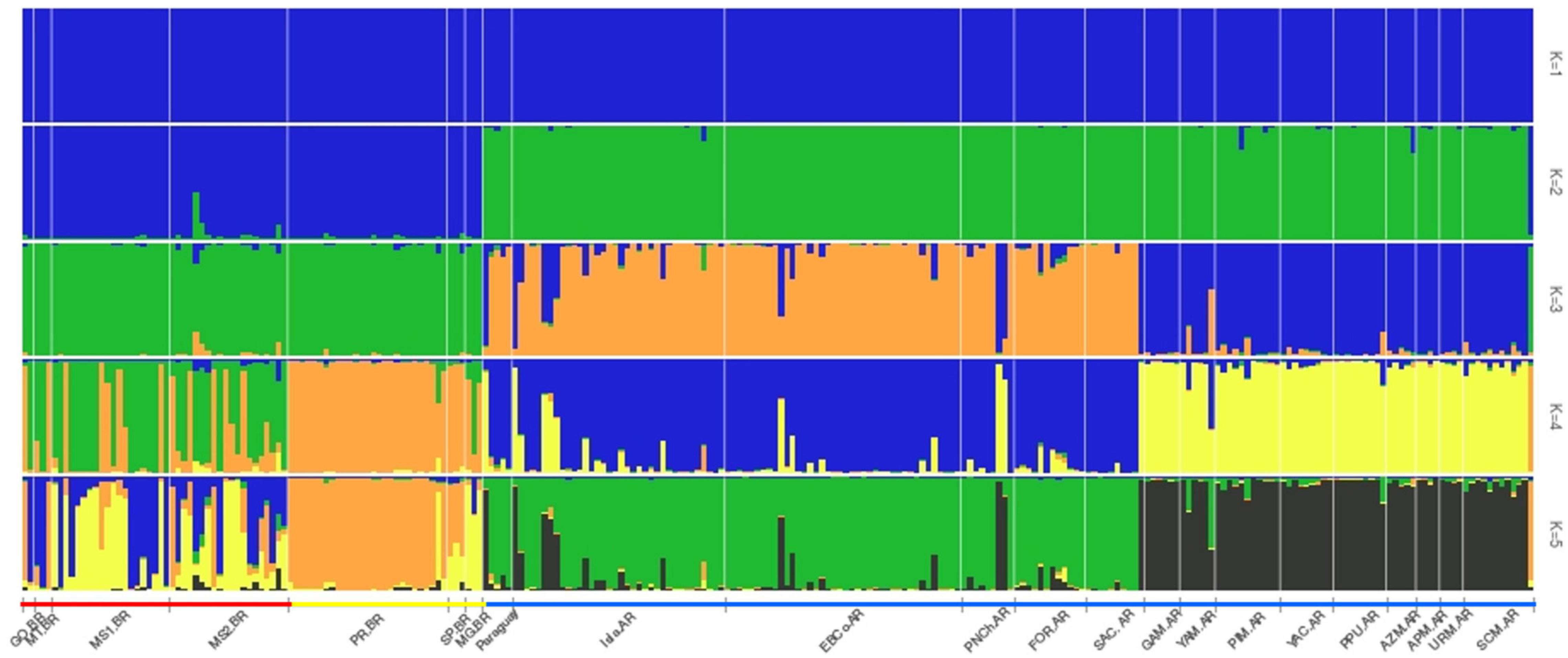
Figure 5. Structure analyses using the KFinder software. Represented with parsimony method detected one cluster grouping the 22 study sites (K = 1). Evanno method results showed K = 2 differentiating Flooded + Rem.BR from Rem.PY.AR. Pr[X| K] method detected K = 5 where most of the yellow and blue clusters are represented by flooded sites.
Genetic Differences Between Flooded and Remaining Sites
Pairwise Fst’s and Nei’s were used to further investigate the genetic differences between flooded (Flooded) and remaining sites (Rem.BR and Rem.PY.AR), with both markers revealing different gene flow situations in time (Figure 6). The mtDNA analysis showed that the flooded study sites showed the highest genetic diversity estimates and shared one haplotype with one Argentine site (Table 2 and Figure 3). In addition, the highest average pairwise between-population differences occur between the Flooded group and the two remaining groups (Flooded vs. Rem.BR p = 0.009; Flooded vs. Rem.AR p = 0.0000; Rem.PY.AR vs. Rem BR p = 0.0000; Figure 6A), suggesting that they historically exchanged migrants with other populations. The remaining populations of Brazil showed an intermediate variability (Table 1) and differentiated mtDNA haplotypes from both the flooded populations and the remnants of Argentina, suggesting isolation processes between all the sites (Table 2 and Figure 3).
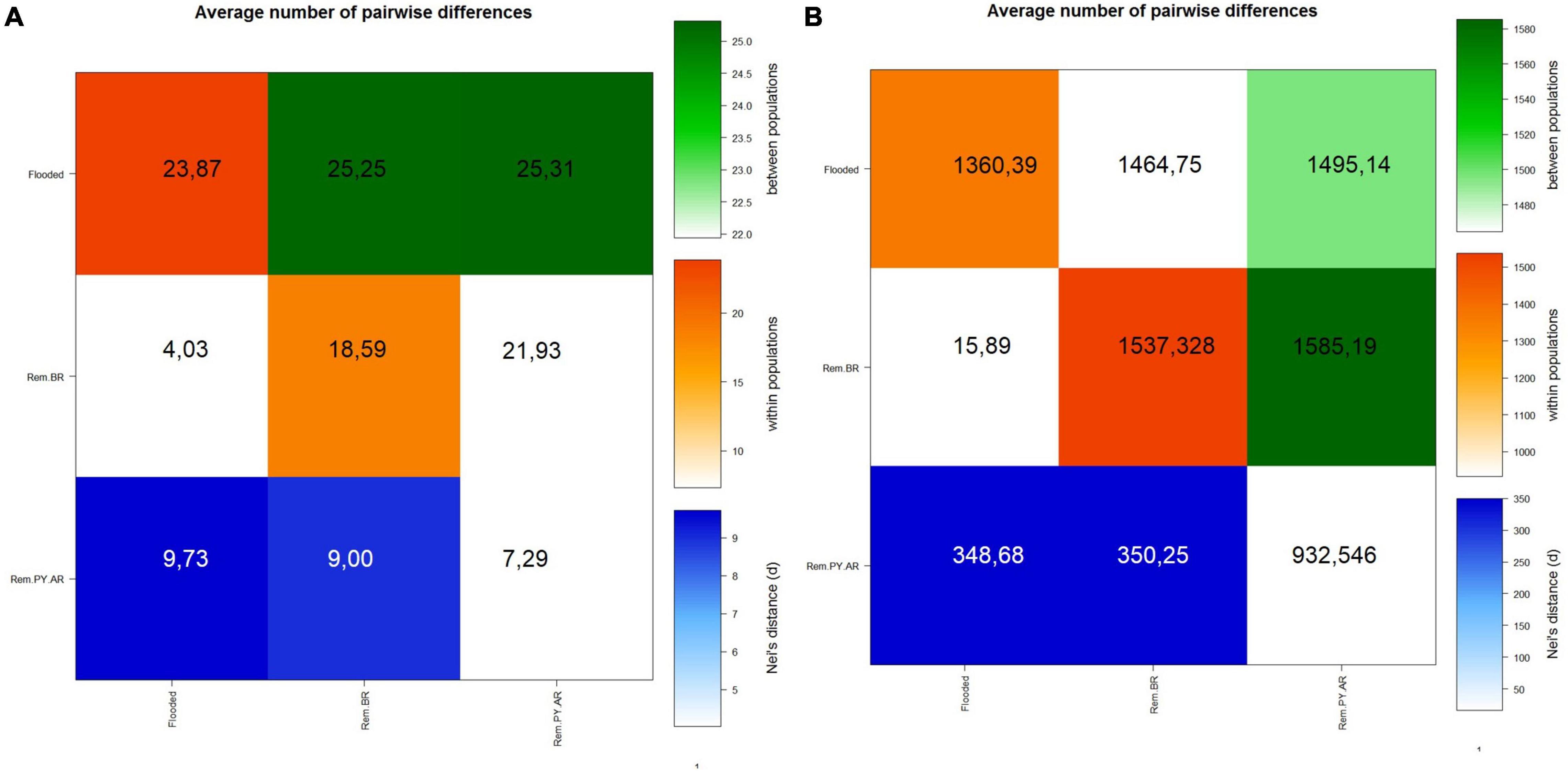
Figure 6. (A) Genetic distance based on a 491 bp fragment of mtDNA Region Control, and (B) based on 10 autosomal Shot Tandem Repeats (STRs). Above diagonal (green) average number of pairwise differences between populations (PiXY; Diagonal elements (orange): within a population (PiX); Below diagonal (blue): Corrected Nei’s distance [PiXY-(PiX + PiY)/2]. An mtDNA: Flooded vs. Rem.BR p = 0.009; Flooded vs. Rem.AR p = 0.0000; Rem.PY.AR vs. Rem BR p = 0.0000. B STRs Flooded vs. Rem.BR p = 0.1273; Flooded vs. Rem.AR p = 0.0000; Rem.PY.AR vs. Rem BR p = 0.0000.
On the other hand, STR analysis showed that the major mean within-population pairwise difference occurs in the Rem.BR group, and the major average pairwise differences between populations are from both Flooded and Rem.BR groups compared to the Rem.PY.AR group. Meanwhile, Nei’s distance shows greater values for both molecular markers between the two Brazilian groups (Flooded and Rem.BR) compared to the Argentine and Paraguayan sites (Flooded vs. Rem.BR p = 0.1273; Flooded vs. Rem.AR p = 0.0000; Rem.PY.AR vs. Rem BR p = 0.0000, Figure 6B).
Tajima’s Neutrality Test comparison of the mtDNA from three groups (Table 2) again showed significantly higher parameter values for the Flooded group compared to those in the remaining groups. According to R-matrix analysis and the regression model of Harpending and Ward (1982), the Brazilian study sites received more gene flow as they have greater heterozygosity and lie above the theoretical regression line. In contrast, Argentine and Paraguayan sites are more isolated and less heterozygous, hence they lie below the theoretical regression line (Figure 7A). When we perform the same analysis grouping in the three groups already mentioned, we see that only the Flooded group lies above the theoretical regression line (Figure 7B).
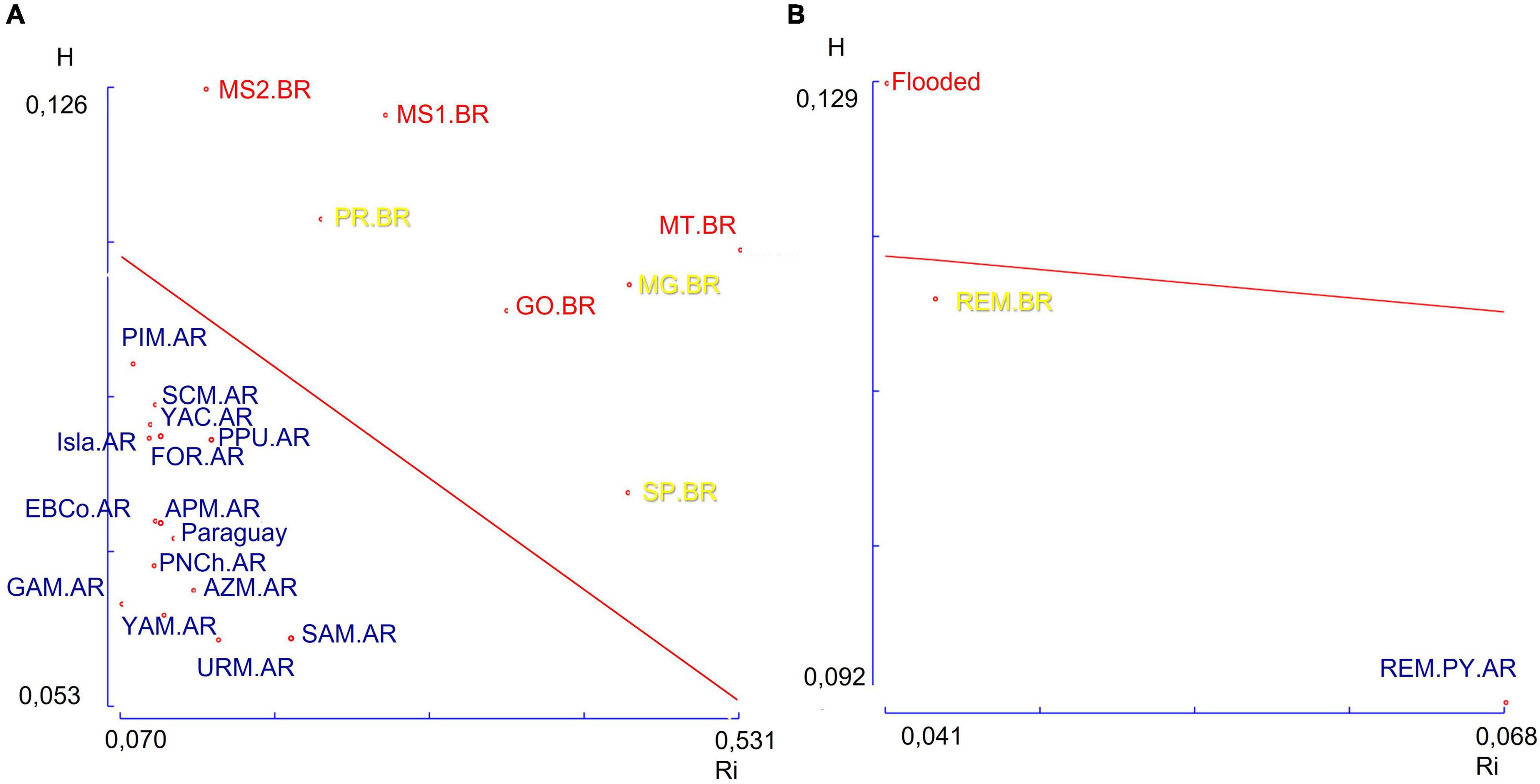
Figure 7. (A) Regression of average heterozygosity on the allele frequency centroid of the 22 study sites analysed based on 10 polymorphic autosomal STRs loci. (B) Same analysis grouping in the three groups flooded, remaining in Brazil (Rem.BR), and remaining in Paraguay and Argentina (Rem.PY.AR).
Discussion
The populations of primates are declining with an unprecedented proportion of species at elevated risk for extinction (66%) due to numerous anthropogenic activities (Estrada et al., 2017). While many primates inhabit flooded environments to which they have adapted in evolutionary times, modifications in the habitat resulting from dam construction forces primates to make significant changes to their ecology virtually overnight (Harrison-Levine et al., 2019).
Alouatta caraya is considered a resilient species, presenting phenotypic plasticity and ability to survive in fragmented environments (Zunino et al., 2007; Bicca-Marques, 2013), even incorporating unusual resources in its diet such as eggs (Bicca-Marques et al., 2009). However, the immense loss of native forest and yellow fever outbreaks during the last decades has exceeded its resistance capacity, as is evident with its status of Vulnerable in Argentina, Endangered in Misiones, and several Brazilian states (Margarido and Braga, 2004; Oklander et al., 2019; Rio Grande do Sul, Decreto Estadual n° 51.797 de 8/9/2014).
Yellow fever outbreaks between 2016 and 2020 in Brazil have been considered the most significant ones of the last 70 years, with more than 15,000 primate epizootics (Ministério da Saúde do Brasil [MS-BR], 2018, 2019, 2021). In 2016, a yellow fever outbreak started in Minas Gerais state and spread to São Paulo, Espírito Santo, and Rio de Janeiro states in 2017 and 2018 before finally reaching Santa Catarina and Paraná states in 2019 (Silva et al., 2020). Notably, these were all states where A. caraya is endemic. However, our analysis was carried out with samples taken before this outbreak. Therefore, our results are independent of them.
Genetic Diversity Gone With the Water
Genetic diversity is important in ensuring species survival as populations that have high levels of genetic diversity are better able to face environmental change than those that have low levels (Hoban et al., 2020). The sites of A. caraya included in the current study cover a large part of the distribution of the species (Figure 1). Our results showed: (1) genetically differentiated populations between the Brazilian and the southernmost populations of the species, (2) that a large part of the genetic variability and unique genetic lineages of the Brazilian populations were probably lost due to dam constructions, and (3) that remaining studied populations have low genetic variation, perhaps making them more vulnerable to future threats as fragmentation and disease outbreaks.
As our sampling does not cover all the occurrence sites or the entire species range of Alouatta caraya. we cannot assert that the genetic variability lost in the flooded sites is not represented in any other remaining population. However, the densities and group sizes recorded for the species on the upper and middle Parana River (Aguiar et al., 2009) were larger than those reported elsewhere in Brazil (Moura, 2007; Silva and Codenotti, 2007). Therefore, the processes of dam construction described in these areas undoubtedly affected at least the frequency of these variants as a whole for this species. On the other hand, as we have detected that genetic variability is related to geographic distance, the unsampled populations at the extreme west of the distribution are also highly likely to be differentiated from those sampled in this study.
At the mtDNA level, of the 42 haplotypes described in the 22 study sites, 14 belong to the flooded sites, of which only one is shared with one of the remaining sampled sites. In other words, these 13 haplotypes found only in the now submerged areas are absent from any other studied population and have possibly disappeared from the genetic variability of the species.
At the autosomal level, the study sites of A. caraya included in the current study had a mean microsatellite diversity of 0.531 ± 0.095. This is slightly lower than most congeneric species studied with some of the same markers employed here [Alouatta pigra: 0.588 (Van Belle et al., 2012), Alouatta belzebul: 0.640 (Gonçalves et al., 2004), Alouatta palliata: 0.584 (Milton et al., 2009)]. Flooded study sites were the most diverse, as shown by the genetic indexes and the presence of exclusive alleles and haplotypes (Tables 1, 2). If we now consider only the variability of the remaining sites (0.504 ± 0.074), it is likely that, on average, they are genetically impoverished compared to other howler species.
The results from the population structure can be hierarchical as evidenced in the different k-value estimation methods that detect one, two, or five clusters depending on the model used. The Pr[X| K] method results detect five genetically differentiated population clusters (k = 5), of which two are practically gone with the water, with only one poorly represented in the remaining MG:BR study site.
To preserve what is left of the gene pool of A. caraya, we recommend that the remaining four genetic clusters must be given high conservation priority. Our evidence supports significant genetic differentiation between populations, indicating contemporary restricted gene flow between A. caraya geographic distribution. These results have direct implications for the conservation of howlers and should be considered by policymakers in future management plans, as well as during dam construction in the habitat of these vulnerable Neotropical primates.
Implications for the Conservation of Alouatta caraya
During the flooding to create the lake for the Engenheiro Sérgio Motta Hydroelectric Plant (sites MS1.BR and MS2.BR in the current study), 4,000 A. caraya individuals were rescued along a 180 km stretch of the Paraná River, with their subsequent release in nearby forest areas (Inglêz, 2006). However, as can be seen in Figure 2, there are almost no remnants of forest on the sides of the flooded area.
The impact of dams on primate populations is not exclusively due to the loss of the flooded forest area. Before and during construction, numerous anthropogenic modifications are also generated in the surrounding areas, such as clearings, arrival of workers, resettlement of local people, and construction of roads and infrastructure, housing, and agricultural needs for these people, all of which diminishes the quality of adjacent forests (Tan and Yao, 2006; Moore et al., 2010; Harrison-Levine et al., 2019). After construction is complete, animals that have remained in the area of the reservoir either drown, remain in treetops that rot and die shortly after, or may try to disperse by swimming. During the flooding of a dam in the Brazilian Amazonia, caiman and jaguars were observed capturing howler monkeys while swimming toward the banks, while eagles captured the animals that remained on emergent trees (Harrison-Levine et al., 2019).
Both “rescued” animals and those arriving in non-flooded areas by their own means are exposed to an unfamiliar environment, difficulties finding resources, such as food, water, and a social group, and a higher risk of stress, disease, predation, and/or human hunting (World Commission on Dams [WCD], 2000; Martin, 2004). There are reports of primates found malnourished, injured, infected with parasites, and stressed during post-flood rescue operations at dams in Thailand (Nakhasathien, 1989), French Guiana (de Thoisy et al., 2001), and Brazil (Harrison-Levine et al., 2019). De Sá (2004) observed an increase in the population density of Plecturocebus, Pithecia, and Samiri in the adjacent habitat just after the release, but an abrupt decrease in the same in subsequent years. Another example, also in Brazil, reports a telemetry follow-up that found that only 7% translocated Cebuella pygmaea survived 3 months after release (Dias et al., 2015).
Historically, our work has aimed to discern the genetic diversity of this species to provide guidelines for the future management of populations (Oklander et al., 2017, 2020, 2021). For all the reasons mentioned above, we assume that the populations analysed in the flooded areas are functionally extinct (Säterberg et al., 2013). Therefore, in the current study, we unfortunately present results of “fossil” genetics. Since the largest number of dams in the future is scheduled in South America (Fearnside, 2006; Zarfl et al., 2015), an awareness of both their long-term detrimental impacts on biodiversity and developing strategies to mitigate such effects should be considered to be of utmost importance, especially in the case of A. caraya, a species demonstrated to be of public health importance. We believe this work provides strong evidence for the need for attention when planning and implementing future hydroelectric dams.
It has already been reported that pre-construction EIA studies fail to: (1) estimate the indirect impact on surrounding areas (Tan and Yao, 2006; Moore et al., 2010), (2) include intra-specific measures of biodiversity, such as genetic variation of species (Simões et al., 2014), and (3) implement management plans to minimise biodiversity losses (Schneider, 2001; Alho, 2011). The occurrence of a severe population declines of the already “Critically Endangered” (CR) Tonkin snub-nosed monkey (Rhinopithecus avunculus) (from 130 to 40 individuals in 13 years, Hoang, 2010) was predicted by an EIA conducted prior to dam construction in Vietnam (Scott Wilson Asia Pacific Ltd [SWAPL], 2000). Despite this, the dam was still built, and few of the recommended mitigation measures were implemented. In the current study, we show an important consequence of the construction of dams for the species A. caraya as the extensive loss of genetic variation, leaving surviving populations greatly genetically impoverished.
To prevent this type of irreversible biodiversity loss from continuing, it should be mandatory for hydropower infrastructure to include all items mentioned in EIA in the final construction. Construction of future hydroelectric plants should explicitly consider appropriate release sites for animal rescues with sufficient resources, minimal anthropogenic threats, and relatively low population densities of conspecifics. In addition, affected primate populations should be part of long-term monitoring programs, including impacts on behavior, health status, and genetics (McAllister et al., 2001).
Data Availability Statement
The datasets presented in this study can be found in online repositories. The names of the repository/repositories and accession number(s) can be found in the article/Supplementary Material.
Ethics Statement
Ethical review and approval was not required for the animal study because some of the samples used in this study belong to previous research such as Environmental Impact Assessments and were in the collection of the Escola de Ciências da Saúde e da Vida, Pontifícia Universidade Católica do Rio Grande do Sul, Porto Alegre, Rio Grande do Sul, Brazil. Other samples are feces, the collection of which does not represent any danger or prejudice in the primate populations studied.
Author Contributions
LO, DC, and SB formulated the research idea. LO, LJ, and SO conducted fieldwork. LO, MC, and GF performed laboratory analyses. MC, GF, and DC performed statistical analyses. LO, MC, GF, LJ, and DC prepared the figures and tables. LO, MC, GF, and DC wrote the manuscript. All authors reviewed and approved the final submission.
Funding
This work was supported by the CONICET grants to LO and the DNA Fingerprinting Service (SHDG), School of Pharmacy and Biochemistry, University of Buenos Aires, Argentina, and by CNPq, CAPES, and FAPERGS grants from Brazil to SB. LO, MC, and DC were members of the Carrera de Investigator (CONICET-Argentina). GF was funded by the Universidad Nacional del Noroeste de la Provincia de Buenos Aires (UNNOBA-Argentina). LJ was funded by the Instituto Chico Mendes de Conservação da Biodiversidade (ICMBio), Ministry of Environment, Brazil.
Conflict of Interest
The authors declare that the research was conducted in the absence of any commercial or financial relationships that could be construed as a potential conflict of interest.
Publisher’s Note
All claims expressed in this article are solely those of the authors and do not necessarily represent those of their affiliated organizations, or those of the publisher, the editors and the reviewers. Any product that may be evaluated in this article, or claim that may be made by its manufacturer, is not guaranteed or endorsed by the publisher.
Acknowledgments
We greatly appreciate the collaboration with the Ministerio de Ecología y Recursos Sustentables de la Provincia de Misiones, Instituto Misionero de Biodiversidad – IMiBio, Programa Macacos Urbanos (Universidade Federal do Rio Grande do Sul, Brazil), and Programa de Revitalização da Bacia Hidrográfica do Rio São Francisco (Ministério do Meio Ambiente, Brazil). We thank Maria de Nazaré Klautau-Guimarães for collaborating with samples. We are grateful to Luciana G. Pacca (ICMBio/CPB) and Vinicius A. Roberto (ICMBio/CPB), for the map in Figure 1, and Keoma C. Rodrigues (ICMBio/CPB) for producing Figure 2. LO wants to especially thank Atenea, Ivy, and Julian Baigorria for their time and patience. We are grateful to Adrian Barnett, Sam Shanee, and Karen DeMatteo for providing comments on the manuscript.
Supplementary Material
The Supplementary Material for this article can be found online at: https://www.frontiersin.org/articles/10.3389/fevo.2022.768652/full#supplementary-material
Footnotes
References
Adamack, A. T., and Gruber, B. (2014). PopGenReport: simplifying basic population genetic analyses in R. Methods Ecol. Evol. 5, 384–387.
Agostini, I., Holzmann, I., and Di Bitetti, M. S. (2008). Infant hybrids in a newly formed mixed-species group of howler monkeys (Alouatta guariba clamitans and Alouatta caraya) in northeastern Argentina. Primates 49, 304–307. doi: 10.1007/s10329-008-0106-1
Agostini, I., Pizzio, E., De Angelo, C., and Di Bitetti, M. S. (2015). Population Status of Primates in the Atlantic Forest of Argentina. Int. J. Primatol. 36, 244–258.
Aguiar, L. M., Ludwig, G., and Passos, F. C. (2009). Group size and composition of black-and-gold howler monkeys (Alouatta caraya) on the Upper Paraná River, Southern Brazil. Primates 50, 74–77. doi: 10.1007/s10329-008-0115-0
Aguiar, L. M., Mellek, D. M., Abreu, K. C., Boscarato, T. G., Bernardi, I. P., Miranda, J. M., et al. (2007). Sympatry between Alouatta caraya and Alouatta clamitans and the rediscovery of free-ranging potential hybrids in Southern Brazil. Primates 48, 245–248. doi: 10.1007/s10329-007-0039-0
Alho, C. (2011). Environmental effects of hydropower reservoirs on wild mammals and freshwater turtles in Amazonia: a review. Oecol. Austral. 15, 593–604. doi: 10.4257/oeco.2011.1503.11
Almeida, M. A. B. D., Santos, E. D., Cardoso, J. D. C., Fonseca, D. F. D., Noll, C. A., Silveira, V. R., et al. (2012). Yellow fever outbreak affecting Alouatta populations in southern Brazil (Rio Grande do Sul State), 2008-2009. Am. J. Primatol. 74, 68–76. doi: 10.1002/ajp.21010
Ascunce, M. S., Hasson, E., Mulligan, C. J., and Mudry, M. D. (2007). Mitochondrial sequence diversity of the southernmost extant New World monkey, Alouatta caraya. Mol. Phylogenet. Evol. 43, 202–215. doi: 10.1016/j.ympev.2006.10.004
Ayala-Burbano, P. A., Caldano, L., Junior, P. M. G., Pissinatti, A., Marques, M. C., Wormell, D., et al. (2017). Genetic assessment for the endangered black lion tamarin Leontopithecus chrysopygus (Mikan, 1823), Callitrichidae, Primates. Am. J. Primatol 79:e22719. doi: 10.1002/ajp.22719
Baden, A. L., Mancini, A. N., Federman, S., Holmes, S. M., Johnson, S. E., Kamilar, J., et al. (2019). Anthropogenic pressures drive population genetic structuring across a Critically Endangered lemur species range. Sci. Rep. 9:16276. doi: 10.1038/s41598-019-52689-2
Bandelt, H., Forster, P., and Röhl, A. (1999). Median-joining networks for inferring intraspecific phylogenies. Mol. Biol. Evol 16, 37–48. doi: 10.1093/oxfordjournals.molbev.a026036
Bauni, V., Schivo, F., Capmourteres, V., and Homberg, M. (2015). Ecosystem loss assessment following hydroelectric dam flooding: the case of Yacyretá, Argentina. Remote Sens. App. 1, 50–60.
Benchimol, M., and Peres, C. A. (2014). Predicting primate local extinctions within “real-world” forest fragments: a pan-neotropical analysis. Am. J. Primatol. 76, 289–302. doi: 10.1002/ajp.22233
Benchimol, M., and Peres, C. A. (2015). Widespread Forest Vertebrate Extinctions Induced by a Mega Hydroelectric Dam in Lowland Amazonia. PLoS One 10:e0129818. doi: 10.1371/journal.pone.0129818
Bicca Marques, J. C., Calegaro Marques, C., Rylands, A., Strier, K. B., Mittermeier, R., De Almeida, M. A., et al. (2017). Yellow fever threatens Atlantic Forest primates. Sci. Adv. 3:e1600946.
Bicca-Marques, J. C. (2013). “How do howler monkeys cope with habitat fragmentation?,” in Primates in Fragments: Complexity and Resilience, eds L. K. Marsh and C. A. Chapman (New York, USA: Springer Press), 283–303.
Bicca-Marques, J. C., and de Freitas, D. S. (2010). The role of monkeys, mosquitoes, and humans in the occurrence of a yellow fever outbreak in a fragmented landscape in south Brazil: protecting howler monkeys is a matter of public health. Trop. Conserv. Sci. 3, 78–89.
Bicca-Marques, J. C., Muhle, C. B., Prates, H. M., Oliveira, S. G., and Calegaro-Marques, C. (2009). Habitat impoverishment and egg predation by Alouatta caraya. Int. J. Primatol. 30, 743–748.
Bicca-Marques, J. C., Prates, H. M., de Aguiar, F. R. C., and Jones, C. B. (2008). Survey of Alouatta caraya, the black-and-gold howler monkey, and Alouatta guariba clamitans, the brown howler monkey, in a contact zone, State of Rio Grande do Sul, Brazil: evidence for hybridization. Primates 49, 246–252. doi: 10.1007/s10329-008-0091-4
Bicca-Marques, J. C., Rumiz, D. I., Ludwig, G., Rímoli, J., Martins, V., da Cunha, R. G. T., et al. (2021). Alouatta caraya (amended version of 2020 assessment). IUCN Red List Threat. Species 2021:e.T41545A190414715. doi: 10.2305/IUCN.UK.2021-1.RLTS.T41545A190414715.en
Brandao, R. A., and Araujo, A. F. B. (2008). Changes in Anuran Species Richness and Abundance Resulting from Hydroelectric Dam Flooding in Central Brazil. Biotropica 40, 263–266.
Brown, A. D., and Zunino, G. E. (1994). Hábitat, densidad y problemas de conservación de los primates de Argentina. Vida Silv. Neotrop. 3, 30–40.
Chiappero, M. B., Sommaro, L. V., Priotto, J. W., Wiernes, M. P., Steinmann, A. R., and Gardenal, C. N. (2016). Spatio-temporal genetic structure of the rodent Calomys venustus in linear, fragmented habitats. J. Mammal. 97, 424–435. doi: 10.1093/jmammal/gyv186
Cosson, J. F., Ringuet, S., Claessens, O., de Massary, J. C., Dalecky, A., Villiers, J. F., et al. (1999). Ecological changes in recent land-bridge islands in French Guiana with emphasis on vertebrate communities. Biol. Conserv. 91, 213–222.
De Sá, R. M. (2004). “Impacts of damming on primate community structure in the Amazon,” in People in Nature: Wildlife Conservation in South and Central America, eds K. M. Silvius, R. E. Bodmer, and J. M. Fragoso (New York, USA: Columbia University Press), 240–256.
de Thoisy, B., Vogel, I., Reynes, J.-M., Pouliquen, J.-F., Carme, B., Kazanji, M., et al. (2001). Health Evaluation of Translocated Free-Ranging Primates in French Guiana. Am. J. Primatol. 54, 1–16. doi: 10.1002/ajp.1008
Di Fiore, A. (2009). “Genetic approaches to the study of dispersal and kinship in New World primates,” in South American Primates. Comparative Perspectives in the Study of Behavior, Ecology, and Conservation, eds P. A. Garber, A. Estrada, J. C. Bicca-Marques, E. W. Heymann, and K. B. Strier (New York, USA: Springer Press), 211–250.
Di Fiore, A., and Fleischer, R. C. (2004). Microsatellite markers for woolly monkeys (Lagothrix lagotricha) and their amplification in other New World primates (Primates: Platyrrhini). Mol. Ecol. Notes 4, 246–249.
Dias, C. A. R., Queirogas, V. L., and Pedersoli, M. A. (2015). Translocation and radio- telemetry monitoring of pygmy marmoset, Cebuella pygmaea (Spix, 1823), in the Brazilian Amazon. Braz. J. Biol. 75, 91–97. doi: 10.1590/1519-6984.07813
Estrada, A., Garber, P. A., Rylands, A. B., Roos, C., Fernandez-Duque, E., Di Fiore, A., et al. (2017). Impending extinction crisis of the world’s primates: why primates matter. Sci. Adv. 3:e1600946. doi: 10.1126/sciadv.1600946
Evanno, G., Regnaut, S., and Goudet, J. (2005). Detecting the number of clusters of individuals using the software STRUCTURE: a simulation study. Mol. Ecol. 14, 2611–2620. doi: 10.1111/j.1365-294X.2005.02553.x
Excoffier, L., and Lischer, H. E. L. (2010). Arlequin suite ver 3.5: a new series of programs to perform population genetics analyses under Linux and Windows. Mol. Ecol. Resour. 10, 564–567. doi: 10.1111/j.1755-0998.2010.02847.x
Fearnside, P. M. (2006). Dams in the Amazon: belo Monte and Brazil’s hydroelectric development of the Xingu River Basin. Environ. Manage. 38, 16–27. doi: 10.1007/s00267-005-0113-6
Fearnside, P. M., and Pueyo, S. (2012). Greenhouse-gas emissions from tropical dams. Nat. Clim. Chang. 2, 382–384.
Ferrari, S. F., Ghilardi, R., Lima, E. M., Pina, A. L., and Martins, S. S. (2004). Tucuruí fifteen years on: long term effects of reservoir flooding on primate populations in Southeastern Amazonia. Folia Primatol. 75, 262–272.
Finer, M., and Jenkins, C. N. (2012). Proliferation of hydroelectric dams in the Andean Amazon and implications for Andes-Amazon connectivity. PLoS One 7:e35126. doi: 10.1371/journal.pone.0035126
Finstermeier, K., Zinner, D., Brameier, M., Meyer, M., Kreuz, E., Hofreiter, M., et al. (2013). Mitogenomic phylogeny of living primates. PLoS One 16:e69504. doi: 10.1371/journal.pone.0069504
Fournier- Chambrillon, C., Fournier, P., Gaillard, J.-M., Genty, C., Hansen, E., and Vié, J.-C. (2000). Mammal trap efficiency during the fragmentation by flooding of a Neotropical rain forest in French Guiana. J. Trop. Ecol. 16, 841–851. doi: 10.1017/S0266467400001759
Francis, R. M. (2016). POPHELPER: an R package and web app to analyse and visualise population structure. Mol. Ecol. Res. 17, 27–32. doi: 10.1111/1755-0998.12509
Frankham, R. (2005). Genetics and extinction. Biol. Conserv. 126, 131–140. doi: 10.1016/j.biocon.2005.05.002
Fu, Y. X. (1997). Statistical tests of neutrality of mutations against population growth, hitchhiking and background selection. Genetics 147, 915–925. doi: 10.1093/genetics/147.2.915
Gibson, L., Wilman, E. N., and Laurance, W. F. (2017). How green is ‘green’ energy? Trends Ecol. Evol. 32, 922–935. doi: 10.1016/j.tree.2017.09.007
Gillet, R. M., and Tobias, P. V. (2002). Human growth in southern Zambia: a first study of Tonga children predating the Kariba Dam (1957-1958). Am. J. Hum. Biol. 14, 50–60. doi: 10.1002/ajhb.10019
Gonçalves, E. C., Silva, A., Barbosa, M. S. R., and Schneider, M. P. C. (2004). Isolation and characterization of microsatellite loci in Amazonian red-handed howlers Alouatta belzebul (Primates, Plathyrrini). Mol. Ecol. Notes. 4, 406–408. doi: 10.1111/j.1471-8286.2004.00667.x
González, V., Zunino, G., Kowalewski, M., and Bravo, S. (2014). Densidad de monos aulladores (Alouatta caraya) y composición y estructura de la selva de inundación en una isla del Río Paraná medio. Revista del Museo Argentino de Ciencias Naturales nueva serie 4, 7–11.
Gorostiaga, N., Zamboni, L. P., Cerezo, A., and Pavé, R. (2021). Influencia de los atributos del paisaje en la ocupación de parches de bosque y en la demografía de Alouatta caraya en un ambiente fragmentado del noreste de Argentina. Rev. Mex. Biodiv. 92:e923475.
Goudet, J. (2003). Fstat (ver. 2.9.4). A Program to Estimate and Test Population Genetics Parameters. Available from http://www.unil.ch/izea/softwares/fstat.html. Updated from Goudet 1995
Green, M. R., and Sambrook, J. (2012). Molecular Cloning: A Laboratory Manual, 4th Edn. New York, USA: Cold Spring Harbor Laboratory Press, 47–54.
Harpending, H. C., Jenkins, T., Crawford, M. H., and Workman, P. L. (1973). Methods and Theories of Anthropological Genetics. Albuquerque, NM: University of New Mexico Press.
Harpending, H. C., and Ward, R. (1982). “Chemical systematics and human evolution,” in Biochemical Aspects of Evolutionary Biology, ed. M. Nitecki (Chicago, IL: University of Chicago Press), 213–256.
Harrison-Levine, A., Covert, H., Norconk, M., Santos, R., Barnett, A., and Fearnside, P. (2019). “Chapter 36 - Dams: Implications of Widespread Anthropic Flooding for Primate Populations,” in Primates in Flooded Habitats: Ecology and Conservation, eds K. Nowak, A. Barnett, and I. Matsuda (Cambridge, UK), 285–292.
Hoang, T. M. (2010). Ensuring the Survival of Tonkin Snub-Nosed Monkey (Rhinopithecus 598 Avunculus) in Na Hang Nature Reserve, Tuyen Quang Province, Northeastern Vietnam. 599. Rufford Small Grants Foundation, 16. Available online at: http://ruffordorg.s3.amazonaws.com/media/project_reports/8228-1%20Interim%20Report.pdf
Hoban, S., Bruford, M., Jackson, J. D. U., Lopes-Fernandes, M., Heuertz, M., Hohenlohe, P. A., et al. (2020). Genetic diversity targets and indicators in the CBD post-2020 Global Biodiversity Framework must be improved. Biol. Conserv. 248:108654. doi: 10.1016/j.biocon.2020.108654
Holzmann, I., Agostini, I., Areta, J. I., Ferreyra, H., Beldomenico, P., and Di Bitetti, M. S. (2010). Impact of yellow fever outbreaks on two howler monkey species (Alouatta guariba clamitans and A. caraya) in Misiones. Argentina. Am. J. Primatol. 72, 475–480. doi: 10.1002/ajp.20796
Humboldt, A. (1812). “Tableu synoptique des singes de l’Amérique,” in Recueil D’observations de Zoologie et D’anatomie Comparée: Faites dans L’océan Atlantique, dans L’intrieur du Nouveau Continent et dans la mer du sud Pendant les Années 1799–1803. Voyage de Humboldt et Bonpland, Deuxième Partie. Observations de Zoologie et D’anatomie Comparée, Premier Volume, eds A. Von Humboldt and A. Bonpland (Paris: Schoell and Dufour & Co.), 353–363.
Hylander, L. D., Gröhn, J., Tropp, M., Vikström, A., Wolpher, H., Silva, E. D. C., et al. (2006). Fish mercury increase in Lago Manso, a new hydroelectric reservoir in tropical Brazil. J. Environ. Manage. 81, 155–166. doi: 10.1016/j.jenvman.2005.09.025
Indrabudi, H., De Gier, A., and Fresco, L. O. (1998). Deforestation and its driving forces: a case study of Riam Kanan watershed, Indonesia. Land. Degrad. Dev. 9, 311–322.
Inglêz, A. P. D. (2006). Caracterização Genética De Alouatta Caraya (Primates, Atelidae) Utilizando Marcadores Heterólogos do Tipo Microssatélites. [Ph.D.thesis]. Brasilia, DF: Universidade Federal de Brasília.
Jardim, M. M., Queirolo, D., Peters, F. B., Mazim, F. D., Favarini, M. O., Tirelli, F. P., et al. (2020). Southern extension of the geographic range of black-and-gold howler monkeys (Alouatta caraya). Mammalia 84, 102–106.
Jones, I. L., Bunnefeld, N., Jump, A. S., Peres, C. A., and Dent, D. H. (2016). Extinction debt on reservoir land-bridge islands. Biol. Conserv. 199, 75–83.
Kinzey, W. G., Norconk, M. A., and Alvarez-Cordero, E. (1988). Primate survey of Eastern Bolívar, Venezuela. Primate Conserv. 9, 66–70.
Klitting, R., Gould, E., Paupy, C., and de Lamballerie, X. (2018). What Does the Future Hold for Yellow Fever Virus? (I). Genes 9:291.
Kolleck, J., Yang, M., Zinner, D., and Roos, C. (2013). Genetic diversity in endangered Guizhou snub-nosed monkeys (Rhinopithecus brelichi): contrasting results from microsatellite and mitochondrial DNA data. PLoS One 8:e73647. doi: 10.1371/journal.pone.0073647
Kowalewski, M. M., Salzer, J. S., Deutsch, J. C., Raño, M., Kuhlenschmidt, M. S., and Gillespie, T. R. (2011). Black and gold howler monkeys (Alouatta caraya) as sentinels of ecosystem health: patterns of zoonotic protozoa infection relative to degree of human–primate contact. Am. J. Primatol. 73, 75–83. doi: 10.1002/ajp.20803
Kumar, S., Stecher, G., Li, M., Knyaz, C., and Tamura, K. (2018). MEGA X: molecular Evolutionary Genetics Analysis across computing platforms. Mol. Biol. Evol. 35, 1547–1549. doi: 10.1093/molbev/msy096
Laemmert, H. W. Jr., and Kumm, H. W. (1950). The susceptibility of Howler monkeys to yellow fever virus. Am. J. Trop. Med. 30, 723–731.
Lakshmi, N., Demarchi, D. A., Veerraju, P., and Rao, T. V. (2002). Population structure and genetic differentiation among the substructured Vysya caste population in comparison to the other populations of Andhra Pradesh, India. Ann. Hum. Biol. 29, 538–549. doi: 10.1080/03014460110114707
Lecompte, E., Bouanani, M.-A., de Thoisy, B., and Crouau-Roy, B. (2017). How do rivers, geographic distance, and dispersal behavior influence genetic structure in two sympatric new world monkeys? Am. J. Primatol. 79:e22660. doi: 10.1002/ajp.22660
Leigh, J. W., and Bryant, D. (2015). PopART: full-feature software for haplotype network construction. Methods Ecol. Evol. 6, 1110–1116.
Lemly, A. D., Kingsford, R. T., and Thompson, J. R. (2000). Irrigated Agriculture and Wildlife Conservation: conflict on a Global Scale. Environ. Manage. 25, 485–512. doi: 10.1007/s002679910039
Librado, P., and Rozas, J. (2009). DnaSP v5: a software for comprehensive analysis of DNA polymorphism data. Bioinformatics 25, 1451–1452.
Lima, J. R., Galatti, U., Lima, C. J., Fáveri, S. B., Vasconcelos, H. L., and Neckel-Oliveira, S. (2015). Amphibians on Amazonian Land-Bridge Islands are Affected More by Area Than Isolation. Biotropica 47, 369–376.
Lynch Alfaro, J. W., Cortés-Ortiz, L., Di Fiore, A., and Boubli, J. P. (2015). Comparative biogeography of Neotropical primates. Mol. Phylogenet. Evol. 82, 518–529. doi: 10.1016/j.ympev.2014.09.027
Margarido, T. C. C., and Braga, F. G. (2004). “Mamíferos,” in Livro Vermelho Da Fauna Ameaçada no Estado do Paraná, eds S. B. Mikich and R. S. Bérnils (Curitiba, PR: Governo do Paraná/SEMA/IAP), 27–142.
Martin, B. (2004). “Conservation of the Tonkin snub- nosed monkey (Rhinopithecus avunculus) and its habitat at Na Hang Nature Reserve during dam construction,” in Conservation of Primates in Vietnam, eds T. Nadler, U. Streicher, and H. T. Long (Frankfurt: Frankfurt Zoological Society), 103–105.
McAllister, D. E., Craig, J. F., Davidson, N., Delany, S., and Seddon, M. (2001). Biodiversity Impacts of Large Dams. Background Paper Nr. 1. Switzerland: IUCN.
Michalski, F., and Peres, C. A. (2005). Anthropogenic determinants of primate and carnivore local extinctions in a fragmented forest landscape of southern Amazonia. Biol. Conserv. 124, 383–396.
Milton, K., Lozier, J. D., and Lacey, E. A. (2009). Genetic structure of an isolated population of mantled howler monkeys (Alouatta palliata) on Barro Colorado Island, Panama. Conserv. Genet. 10:347.
Moore, D., Dore, J., and Gyawali, D. (2010). The World Commission on Dams + 10: revisiting the large dam controversy. Water Altern. 3, 3–13.
Moura, A. C. (2007). Primate group size and abundance in the Caatinga dry forest, Northeastern Brazil. Int. J. Primatol. 28, 1279–1297. doi: 10.1002/ajp.23126
Ministério da Saúde do Brasil [MS-BR] (2018). Monitoramento do Período Sazonal da Febre Amarela Brasil – 2017/2018. Secretaria de Vigilância em Saúde (SVS), Informe n° 15, 28 Fev. 2018. Available online at: https://saude.campinas.sp.gov.br/doencas/febre_amarela/2018/Informe_MS_15_F_Amarela_monitoramento.pdf (accessed February 12, 2022).
Ministério da Saúde do Brasil [MS-BR] (2019). Monitoramento de Febre Amarela Brasil 2019. Secretaria de Vigilância em Saúde (SVS), Informe n° 17, 3 Jun. 2019. Available online at: https://saude.es.gov.br/Media/sesa/Imuniza%C3%A7%C3%A3o/Informe-de-Monitoramento-de-Febre-Amarela-Brasil-n17-1.pdf (accessed February 12, 2022).
Ministério da Saúde do Brasil [MS-BR] (2021). Boletim Epidemiológico, Secretaria de Vigilância em Saúde, Ministério da Saúde 20 Volume 52, n° 31 Ago. 2021. Available online at: https://www.gov.br/saude/pt-br/centrais-de-conteudo/publicacoes/boletins/boletins-epidemiologicos/edicoes/2021/boletim_epidemiologico_svs_31.pdf (accessed February 12, 2022).
Nilsson, C., Reidy, C. A., Dynesius, M., and Revenga, C. (2005). Fragmentation and flow regulation of the world’s large river systems. Science 308, 405–408. doi: 10.1126/science.1107887
Oklander, L., Kowalewski, M., Peker, S., Pavé, R., Agostini, I., and Holzmann, I. eds (2019). “Alouatta caraya,” in Categorización 2019 de los mamíferos de Argentina según su riesgo de extinción, (Mendoza: Lista Roja de los mamíferos de Argentina).
Oklander, L. I., Caputo, M., Solari, A., and Corach, D. (2020). Genetic assignment of illegally trafficked neotropical primates and implications for reintroduction programs. Sci. Rep. 10:3676. doi: 10.1038/s41598-020-60569-3
Oklander, L. I., Kowalewski, M. M., and Corach, D. (2010). Genetic consequences of habitat fragmentation in black-and-gold howler (Alouatta caraya) populations from northern Argentina. Int. J. Primatol. 31, 813–832.
Oklander, L. I., Miño, C. I., Fernández, G., Caputo, M., and Corach, D. (2017). Genetic structure in the southernmost populations of black-and-gold howler monkeys (Alouatta caraya) and its conservation implications. PLoS One 12:e0185867. doi: 10.1371/journal.pone.0185867
Oklander, L. I., Willoughby, J. R., Corach, D., and Cortés-Ortiz, L. (2021). Using Genetic Diversity Estimates in the Assessment of the Conservation Status of Neotropical Primates. Mol Ecol. Conserv. Genet. Neotrop. Mamm. 11, 261–275. doi: 10.1007/978-3-030-65606-5
Oklander, L. I., Zunino, G. E., Di Fiore, A., and Corach, D. (2007). Isolation, characterization and evaluation of 11 autosomal STRs suitable for population studies in black and gold howler monkeys Alouatta caraya. Mol. Ecol. Notes. 7, 117–120.
Peakall, R., Ruibal, M., and Lindenmayer, D. B. (2003). Spatial autocorrelation analysis offers new insights into gene flow in the Australian bush rat. Rattus fuscipes. Evolution 57, 1182–1195. doi: 10.1111/j.0014-3820.2003.tb00327.x
Peakall, R., and Smouse, P. E. (2012). GenAlEx 6.5: genetic analysis in Excel. Population genetic software for teaching and research-an update. Bioinformatics 28, 2537–2539. doi: 10.1093/bioinformatics/bts460
Peña, J., Gómez-Pérez, L., and Alfonso-Sánchez, M. (2020). GenoCline: On the trail of spatial patterns of genetic variation. Authorea
Poff, N. L., Allan, J. D., Bain, M. B., Karr, J. R., Prestegaard, K. L., Richter, B. D., et al. (1997). The natural flow regime: a paradigm for river conservation and restoration. Bioscience 47, 769–784. doi: 10.2307/1313099
Pritchard, J. K., Stephens, M., and Donnelly, P. (2000). Inference of population structure using multilocus genotype data. Genetics 155, 945–959.
Prowse, T. D., Peters, D., Beltaos, S., Pietroniro, A., Romolo, L., Töyrä, J., et al. (2002). Restoring Ice-jam Floodwater to a Drying Delta Ecosystem. Water Int. 27, 58–69. doi: 10.1080/02508060208686978
Richter, B. D., Postel, S., Revenga, C., Lehner, B., and Churchill, A. (2010). Lost in development’ s shadow: the downstream human consequences of dams. Water Altern. 3, 14–42.
Robinson, N. A. (1992). International trends in environmental impact assessment. Pace Law Faculty Publications, Paper 382. New York, NY: Pace University.
Rodrigues, M. (2006). Hidroelétrica, ecologia comportamental e resgate de fauna: uma falácia. Nat. Conserv. 4, 29–38.
Rosa, L. P., dos Santos, M. A., Matvienko, B., dos Santos, E. O., and Sikar, E. (2004). Greenhouse gases emissions by hydroelectric reservoirs in tropical regions. Clim. Change 66, 9–21.
Rumiz, D. I. (1990). Alouatta caraya: population density and demography in northern Argentina. Am. J. Primat. 21, 279–294. doi: 10.1002/ajp.1350210404
Säterberg, T., Sellman, S., and Ebenman, B. (2013). High frequency of functional extinctions in ecological networks. Nature 499, 468–470. doi: 10.1038/nature12277
Schneider, M. (2001). Mastofauna da bacia hidrográfica do rio Manso, MT - Uma abordagem de Ecologia de Paisagem para avaliação da perda de hábitats. [PhD thesis]. São Carlos, BR: Universidade Federal de São Carlos.
Schuelke, M. (2000). An economic method for the fluorescent labeling of PCR fragments. Nat. Biotechnol. 18, 233–234. doi: 10.1038/72708
Scott Wilson Asia Pacific Ltd [SWAPL] (2000). Gam River Dam: Preliminary Environmental 587 Impact Assessment. PARC Project VIE/95/G31. Hanoi: Government of 588 Vietnam/UNOPS/UNDP/Scott Wilson Asia-Pacific Ltd, 26.
Siconelli, M. J. L., Espósito, D. L. A., Moraes, N. C., Ribeiro, J. M., Perles, L., Dias, M. A., et al. (2019). The importance of coordinated actions in preventing the spread of yellow fever to human populations: the experience from the 2016-2017 yellow fever outbreak in the northeastern region of São Paulo State. Can. J. Infect. Dis. Med. Microbiol. 2019, 1–11. doi: 10.1155/2019/9464768
Silva, N. I. O., Sacchetto, L., de Rezende, I. M., de Souza Trindade, G., LaBeaud, A. D., de Thoisy, B., et al. (2020). Recent sylvatic yellow fever virus transmission in Brazil: the news from an old disease. Virol. J. 17, 1–12. doi: 10.1186/s12985-019-1277-7
Silva, V. M., and Codenotti, T. L. (2007). “Mapeamento das áreas de ocorrência de Alouatta caraya em fragmentos florestais de Tupanciretã, Rio Grande do Sul,” in A Primatologia no Brasil 10, ed. J. C. Bicca-Marques (Porto Alegre: Sociedade Brasileira de Primatologia), 181–191.
Silva, Y. B. D. S. E., Ribeiro, B. R., Thiesen Brum, F., Soares-Filho, B., Loyola, R., and Michalski, F. (2018). Combined exposure to hydroelectric expansion, climate change and forest loss jeopardies amphibians in the Brazilian Amazon. Divers. Distrib 24, 1072–1082.
Simões, P. I., Stow, A., Hödl, W., Amézquita, A., Farias, I. P., and Lima, A. P. (2014). The value of including intraspecific measures of biodiversity in environmental impact surveys is highlighted by the Amazonian brilliant-thighed frog (Allobates femoralis). Trop. Conserv. Sci. 7, 811–828.
Tajima, F. (1989). Statistical methods to test for nucleotide mutation hypothesis by DNA polymorphism. Genetics 123, 585–595.
Tan, Y., and Yao, F. (2006). Three Gorges Project: effects of resettlement on the environment in the reservoir area. Pop. Envir. 27, 351–371.
Terborgh, J., Lopez, L., Nuñez, P., Rao, M., Shahabuddin, G., Orihuela, G., et al. (2001). Ecological meltdown in predator-free forest fragments. Science 2904, 1923–1926.
Terborgh, J., Lopez, L., Tello, J., Yu, D., and Bruni, A. R. (1997). “Transitory states in relaxing ecosystems of land bridge islands,” in Tropical Forest Remnants: Ecology, Management, and Conservation of Fragmented Communities, eds W. L. Laurance and R. O. Bierregarrd (Chicago, IL: University of Chicago Press), 256–274.
Thompson, J. D., Higgins, D. G., and Gibson, T. J. (1994). CLUSTAL W: improving the sensitivity of progressive multiple sequence alignment through sequence weighting, position-specific gap penalties and weight matrix choice. Nucleic Acids Res. 22, 4673–4680.
Tockner, K., and Stanford, J. A. (2002). Riverine flood plains: present state and future trends. Environ. Conserv 29, 308–330.
Tomer, Y., Greenberg, D. A., Concepcion, E., Ban, Y., and Davies, T. F. (2002). Thyroglobulin is a thyroid specific gene for the familial autoimmune Thyroid diseases. J. Clin. Endocrinol. Metab. 87, 404–407. doi: 10.1210/jcem.87.1.8291
Tullos, D. (2008). Assessing the influence of environmental impact assessments on science and policy: an analysis of the Three Gorges project. J. Environ. Manage. 90, 1–16. doi: 10.1016/j.jenvman.2008.07.031
Tundisi, J. G., Goldemberg, J., Matsumura-Tundisi, T., and Saraiva, A. C. F. (2014). How many more dams in the Amazon? Energy Policy 74, 703–708. doi: 10.1016/j.enpol.2014.07.013
Van Belle, S., Estrada, A., Strier, K. B., and Di Fiore, A. (2012). Genetic structure and kinship patterns in a population of black howler monkeys, Alouatta pigra, at Palenque National Park, Mexico. Am. J. Primatol. 74, 948–957. doi: 10.1002/ajp.22047
Van Oosterhout, C., Hutchinson, W. F., Wills, D. P. M., and Shipley, P. (2004). MICRO-CHECKER: Software for identifying and correcting genotyping errors in microsatellite data. Mol. Ecol. Notes. 4, 535–538.
Verdum, R. (2012). As obras de infraestrutura do PAC e os povos indígenas na Amazônia brasileira. (Brasília: INESC).
Wang, J. (2019). A parsimony estimator of the number of populations from a STRUCTURE-like analysis. Mol. Ecol. Resour. 19, 970–981. doi: 10.1111/1755-0998.13000
Wang, W., Zheng, Y., Zhao, J., and Yao, M. (2019). Low genetic diversity in a critically endangered primate: shallow evolutionary history or recent population bottleneck? BMC Evol. Biol. 19:134. doi: 10.1186/s12862-019-1451-y.
Woodford, M. H., and Rossiter, P. B. (1993). Disease risks associated with wildlife translocation projects. Rev. Sci. Tech. Office Int. des. Epizoot. 12, 115–135. doi: 10.20506/rst.12.1.667
World Commission on Dams (WCD) (2000). Dams and Development: A New Framework for Decision Making. London: Earthscan Publications. www.internationalrivers.org/files/attachedfiles/world_commission_on_dams_final_report.pdf
Zarfl, C., Lumsdon, A. E., Berlekamp, J., Tydecks, L., and Tockner, K. (2015). A global boom in hydropower dam construction. Aquat. Sci. 77, 161–170. doi: 10.1038/s41598-020-73939-8
Zhao, X., Ren, B., Li, D., Garber, P. A., Zhu, P., Xiang, Z., et al. (2019). Climate change, grazing, and collecting accelerate habitat contraction in an endangered primate. Biol. Conserv. 231, 88–97.
Zunino, G. E., González, V., Kowalewski, M., and Bravo, S. (2001). Alouatta carayaRelationships among habitat, density and social organization. Primate Rep. 61, 37–46.
Keywords: Alouatta caraya, howler monkeys, dam construction, genetic structure, conservation
Citation: Oklander LI, Caputo M, Fernández GP, Jerusalinsky L, de Oliveira SF, Bonatto SL and Corach D (2022) Gone With the Water: The Loss of Genetic Variability in Black and Gold Howler Monkeys (Alouatta caraya) Due to Dam Construction. Front. Ecol. Evol. 10:768652. doi: 10.3389/fevo.2022.768652
Received: 01 September 2021; Accepted: 21 January 2022;
Published: 10 March 2022.
Edited by:
Joelisoa Ratsirarson, University of Antananarivo, MadagascarReviewed by:
Jessica Lynch, University of California, Los Angeles, United StatesPavel Munclinger, Charles University, Czechia
Copyright © 2022 Oklander, Caputo, Fernández, Jerusalinsky, de Oliveira, Bonatto and Corach. This is an open-access article distributed under the terms of the Creative Commons Attribution License (CC BY). The use, distribution or reproduction in other forums is permitted, provided the original author(s) and the copyright owner(s) are credited and that the original publication in this journal is cited, in accordance with accepted academic practice. No use, distribution or reproduction is permitted which does not comply with these terms.
*Correspondence: Luciana Inés Oklander, bGlva2xhbmRlckBjb25pY2V0Lmdvdi5hcg==
†These authors have contributed equally to this work