- 1School of Life Sciences, Arizona State University, Tempe, AZ, United States
- 2Division of Natural Sciences, College of Mount Saint Vincent, Riverdale, NY, United States
Despite the prominence of kin selection as a framework for understanding the evolution of sociality, many animal groups are comprised of unrelated individuals. These non-kin systems provide valuable models that can illuminate drivers of social evolution beyond indirect fitness benefits. Within the Hymenoptera, whose highly related eusocial groups have long been cornerstones of kin selection theory, groups may form even when indirect fitness benefits for helpers are low or absent. These non-kin groups are widespread and abundant, yet have received relatively little attention. We review the diversity and organization of non-kin sociality across the Hymenoptera, particularly among the communal bees and polygynous ants and wasps. Further, we discuss common drivers of sociality across these groups, with a particular focus on ecological factors. Ecological contexts that favor non-kin sociality include those dominated by resource scarcity or competition, climatic stressors, predation and parasitism, and/or physiological constraints associated with reproduction and resource exploitation. Finally, we situate Hymenopteran non-kin sociality within a broader biological context by extending insights from these systems across diverse taxa, especially the social vertebrates. Non-kin social groups thus provide unique demonstrations of the importance of ecological factors in mediating the evolutionary transition from solitary to group living.
Introduction
Social animals represent some of the most ubiquitous and ecologically dominant organisms globally (Hölldobler and Wilson, 1990; Krause and Ruxton, 2002; Ward and Webster, 2016). To date, our understanding of how social groups emerge has been rooted overwhelmingly in the study of family groups. From these groups have emerged useful theoretical frameworks for explaining cooperation in nature, especially kin selection theory, which posits that indirect fitness benefits of helping kin can compensate for direct fitness costs (Hamilton, 1964; West-Eberhard, 1975; Trivers and Hare, 1976; Abbot et al., 2011; Bourke, 2014). Nevertheless, many animals form groups with non-relatives, and in these societies direct fitness gains are generally the major component of inclusive fitness (Clements and Stephens, 1995; Dugatkin, 2002; Goodnight, 2005; Clutton-Brock, 2009; Queller, 2011). These social groups, which exist across diverse animal taxa (Bernasconi and Strassmann, 1999; Clutton-Brock, 2009; Riehl, 2013; Wilkinson et al., 2016; Brask et al., 2019; Suarez and Goodisman, 2021), demonstrate the value of examining the diversity of selection contexts for understanding the evolution of sociality, and provide useful models for examining ecological drivers of social evolution.
Kin selection has proven critically valuable for understanding the evolution of eusociality, especially within the highly related colonies of the social insects (West-Eberhard, 1975; Queller and Strassmann, 1998; Hughes et al., 2008; Abbot et al., 2011; Bourke, 2011; Linksvayer and Wade, 2011). However, eusociality is rare; even among the Hymenoptera; other forms of group living are considerably more common (Heinze et al., 2017; Hunt and Toth, 2017; Wcislo and Fewell, 2017; Fewell and Abbot, 2018). Perhaps due to the prominence of kin selection as a framework for understanding insect sociality, non-kin groups in insects have received relatively little attention, despite advances in our understanding of non-kin vertebrate groups (Clutton-Brock, 2009; Riehl, 2013; Wilkinson et al., 2016; Brask et al., 2019). Departures from a kin-centric framework for understanding insect social evolution may enable valuable connections to other animal groups, contributing to a broader body of evolutionary theory. Further, these systems may be neglected because interactions among non-kin rarely (if ever) constitute altruism—that is, behavior that reduces the fitness of the actor and increases the fitness of the group—which has been a major focus of social evolutionary research in the eusocial Hymenoptera (Hamilton, 1972; Simon, 1990; Foster et al., 2006; Kennedy et al., 2018). Rather, non-kin associations provide examples of cooperation based on mutual benefits of grouping, with or without reproductive division of labor.
We review advances in our understanding of non-kin social groups in the Hymenoptera, with a focus on patterns of diversity in social structure and ecological context. We characterize variation in the organization of these groups and describe the distribution of non-kin sociality across the bees, ants, and wasps. Across these groups, we then highlight common ecological drivers of non-kin sociality, particularly environmental challenges and intra- and inter-specific interactions. Finally, we synthesize insights from the current body of research on non-kin sociality and highlight promising directions for future research. In doing so, we emphasize the role of ecological context in shaping sociality at its evolutionary origins.
Non-kin Cooperation in the Hymenoptera
Non-kin sociality is found broadly among the social ants, wasps, and bees, and ranges in complexity from simple, facultative nest sharing in primarily solitary populations to cooperative founding of eusocial colonies (Figure 1). For the purposes of this review, we define sociality as any long-term association between conspecifics characterized by mutual tolerance and/or cooperation within shared nesting space (Costa, 2006; Fewell and Abbot, 2018). By “long-term,” we refer to an extended or significant portion of an individual’s lifespan, as opposed to more transient interactions like mating. Further, we emphasize mutual tolerance as a minimum requirement in our definition of sociality for the sake of including even groups characterized by limited cooperative behavior. Mutual tolerance serves as a preadaptation for the evolution of cooperation, by enabling individuals to share nest space and providing opportunities for more complex social interactions (Michener, 1974, 1990a).
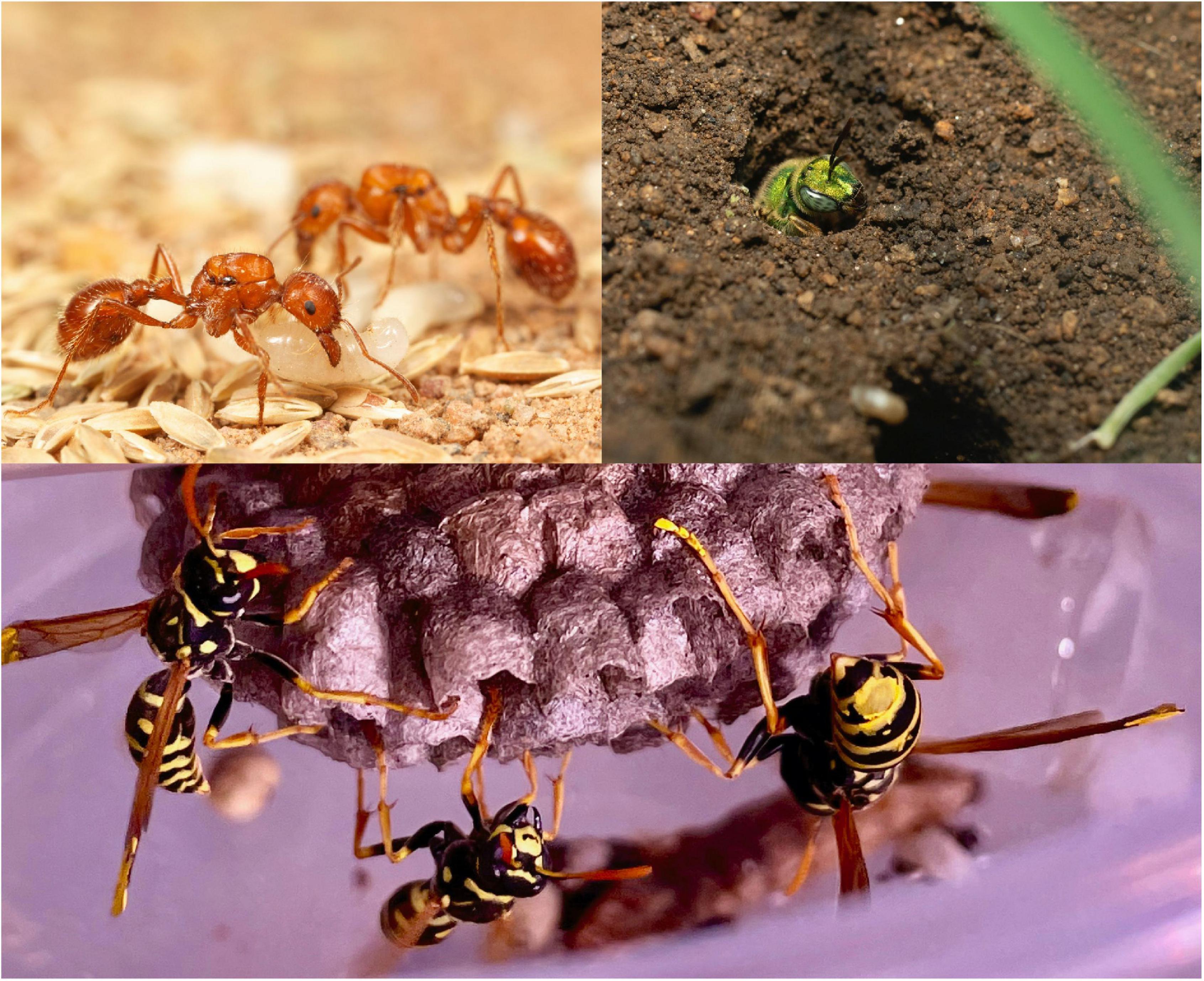
Figure 1. Examples of non-kin sociality are widespread across hymenopteran taxa. In the ants, unrelated foundresses may cooperate to rear eusocial colonies, as in the harvester ant Pogonomyrmex californicus (top left; photo by Elizabeth Cash). Similarly, foundresses of some wasp species, like the paper wasp Polistes dominula (bottom; photo by Meagan Simons), may cooperatively found eusocial nests with non-relatives. Non-kin associations are also found among the communal bees, such as the sweat bee Agapostemon virescens (top right; photo by Nicholas Dorian), which shares nest-entrance guarding duties with unrelated nestmates.
Specifically, we examine social interactions in the context of breeding and offspring care, because behavioral decisions in these contexts have important fitness impacts. We emphasize nest sharing to exclude from our definition of sociality those animals living within aggregations of spatially clustered nests, but otherwise living solitarily. Though some Hymenoptera (such as army ants) are non-nesting, nests are used predominantly by this taxon as an essential physical site for the prolonged interactions intrinsic to social living. Additionally, we define sociality as distinct from intraspecific social parasitism, and therefore exclude from our discussion those systems in which non-kin relationships arise through parasitic behavior (Beekman and Oldroyd, 2008), including adoption of unrelated offspring (Klahn, 1988; Nonacs and Reeve, 1993) and cleptoparasitism (Michener, 1974; Rozen, 1991).
Non-kin associations vary considerably in the degree of cooperation, and thus serve as an important counterpoint to vertebrate sociality. However, discussions of cooperation for social insects and social vertebrates have historically been treated separately. For example, cooperation in the social insects is often studied in the context of task allocation and division of labor (Hölldobler and Wilson, 1990; Seeley, 1996; Beshers and Fewell, 2001), while social vertebrate sociality is more often discussed in terms of the costs and benefits of cooperative interactions (Hamilton, 1964; Dugatkin, 2002; Clutton-Brock, 2009). Defining cooperation itself has also presented challenges, with debate surrounding the questions of whether cooperative interactions may incur differential costs for actor and recipient, and whether cooperative sociality can be maintained under such conditions without indirect fitness gains (Lehmann and Keller, 2006; West et al., 2006, 2007; Bergmüller et al., 2009). Within such discussions, however, has emerged a central theme that cooperation broadly entails behaviors that benefit the social group (Clutton-Brock, 2009).
Social Evolution in the Hymenoptera
The evolution of cooperative behaviors is shaped by ecological context and by the phylogenetic pathway that group has taken to sociality. The task of categorizing the various forms of sociality and their evolutionary histories has been the subject of considerable debate (Wilson, 1971; Michener, 1974; Crespi and Yanega, 1995; Toth et al., 2016; Boomsma and Gawne, 2018; Richards, 2019). A well-established hypothesis has proposed a stepwise evolutionary progression from simple forms of sociality to complex eusociality (Evans, 1956; Wilson, 1971; Evans and West-Eberhard, 1973; Rehan and Toth, 2015). Recent, renewed discussion of this topic has challenged the theoretical presumption of a “social ladder” in which less complex social forms represent intermediate “levels” along an evolutionary trajectory toward eusociality (Linksvayer and Johnson, 2019; Holland and Bloch, 2020). Accordingly, we consider the diversity of cooperative systems in the social insects not as transitional forms in the evolution of sociality, but instead in terms of their shared cooperative behavioral repertoires that are adaptive in a given ecological context.
One of the simplest forms of sociality, known as communal living, refers to societies in which multiple same-generation females (often unrelated) share nesting space but independently forage and provision their own offspring (Michener, 1974). Communal groups are characteristically casteless: group members are not distinguished behaviorally or morphologically by their capacity for reproduction. Only a subset of tasks—typically nest construction and nest defense—are shared cooperatively. Communal groups often exist among otherwise solitary populations of bees and wasps, and are characterized by behavioral repertoires similar to those of solitary females: they mass-provision brood at the egg stage, and do not engage in further direct parental care (Wcislo and Tierney, 2009; Wcislo and Fewell, 2017). In contrast, other social insects, including ants, wasps, and some bee taxa, perform direct parental care in which larvae are fed progressively (Field, 2005). The cooperative repertoire of these groups is similarly expanded. These associations occur when related or unrelated females found nests cooperatively (pleometrosis) by sharing or dividing such tasks as provisioning, nest construction, and defense (Ross and Matthews, 1991; Heinze et al., 2017).
Social Diversity in the Hymenoptera
Here we describe the diversity of non-kin sociality defined as long-term adult nest sharing, with groups often characterized by cooperative behaviors and task sharing. Because relatedness is a relative attribute (Pamilo, 1989), we do not strictly define kin vs. non-kin, but rather focus on groups in which individuals may be no more related to their nestmates than they are to non-nestmates. For some of the systems we discuss, non-kinship in social groups has been evaluated with high confidence by inferring relatedness from molecular markers. In many other cases, the presence of non-relatives in social groups has been inferred from observations of nest-joining behavior, often by individuals from distant nests (in bees and wasps), or of cooperative nest founding by presumed unrelated foundresses (in wasps and ants). Though these observations cannot confirm the degree of relatedness between joiners and their nestmates, they provide suggestions of potential flexibility in tolerance toward unrelated conspecifics. Because the data on kinship in these groups is so incomplete, we highlight these uncertain cases as promising avenues for future genetic investigation.
Within the Hymenoptera, we explore non-kin groups among wasps, bees, and ants, finding limited evidence for true sociality among the sawflies (Hymenoptera: Symphyta), which have short adult lifespans and are non-nesting (Kudo et al., 1998). For each group, we describe patterns and diversity of non-kin social systems. We do not present an exhaustive review of all known non-kin groups in the Hymenoptera, but instead highlight common patterns of social organization across the major suborders.
Wasps: Communal Societies and Foundress Associations
The wasps (Hymenoptera: Apocrita) comprise more than 37 families, among which only three (Aculeata: Pompilidae, Sphecidae, and Vespidae) contain social species (Hunt and Toth, 2017). Non-kin groups are found within all three of these families (Table 1). Communal nesting has been described for several species, and among these, nest-joining by non-relatives is possible, though unconfirmed, for the spider wasp Auplopus semialatus (Pompilidae: Pepsinae); (Wcislo et al., 1988), the digger wasp Crabro cribrellifer (Crabronidae: Crabroninae); (Wcislo et al., 1985), and the pollen wasp Trimeria howardii (Vespidae: Masarinae); (Zucchi et al., 1976). Facultative nest sharing is likewise known among the hover wasps (Vespidae: Stenogastrinae), where unrelated females can join established foundresses (Strassmann et al., 1994; Turillazzi, 2012). Similarly, among the primitively eusocial paper wasps (Vespidae: Polistinae), foundress associations often form among sisters or other close relatives (West-Eberhard, 1969; Ross and Matthews, 1991), but in many cases may be comprised of non-kin (Queller et al., 2000; Hunt, 2007; Mora-Kepfer, 2014). For the paper wasp Polistes dominula, 15–35% of foundress associations consist of unrelated females (Queller et al., 2000; Zanette and Field, 2008; Leadbeater et al., 2011). Co-founding by non-relatives is also known, but uncommon, in Polistes fuscatus (Klahn, 1979) and Polistes exclamans (MacCormack, 1982). Unlike communal groups, these societies are characterized by high reproductive skew, so unrelated joiners often become subordinate helpers with limited reproductive opportunities (Queller et al., 2000; Leadbeater et al., 2010; Mora-Kepfer, 2014).
Bees: Communal and Parasocial Societies
Communal nesting occurs across all six major bee families (Wcislo, 1993; Kukuk et al., 2005), and many of these communal groups are known or expected to consist of non-kin. This social strategy is perhaps best known among the sweat bees (Halictidae), which are known for their incredible diversity of social behaviors (Michener, 1974, 1990b,2007; Brady et al., 2006). Halictid communal nesting has been described within the subfamilies Halictinae and Nomiinae; for most of these species, relatedness among communal nestmates is unknown (Michener, 1969; Wcislo, 1993; Vogel and Kukuk, 1994; Wcislo and Engel, 1996), but may be inferred to be low through observations of nest-joining behavior (Michener and Lange, 1958; Abrams and Eickwort, 1981; Richards et al., 2003). Kukuk and Sage analyzed two polymorphic genetic loci among colonies of the sweat bee Lasioglossum hemichalceum (Halictidae: Halictinae) and found relatedness within reproductively active nests to be indistinguishable from zero (1994). Communal nesting is present but less common among the Colletid bees (Sakagami and Zucchi, 1978), with low relatedness (r = 0.26) confirmed among nestmates of Amphylaeus morosus (Colletidae: Hylaeinae) (Spessa et al., 2000). Similarly, non-kin nesting is possible among the communal Andrenidae (Danforth, 1991; Paxton et al., 1999), and has been confirmed for two species: Andrena scotica (formerly jacobi); (Andrenidae: Andreninae) (Paxton et al., 1996) and Macrotera (formerly Perdita) texana (Andrenidae: Panurginae) (Danforth et al., 1996).
In other cases, the social organization of some non-kin bee groups is more aptly described by the umbrella term “parasocial,” which includes all associations of same-generation adults, which may be cooperative or non-cooperative, and which may exhibit high or low reproductive skew (Michener, 1974). This is the case for many bees of the family Apidae, which includes both solitary and highly social species. For example, bees in the genus Exomalopsis (Apidae: Apinae) form multi-female nests, which may be characterized by cooperative provisioning (Michener, 1966) and even reproductive skew (Raw, 1977). Relatedness in this genus has not been formally investigated, but is likely to be low for many species, considering the high number of females per nest (884 in one nest of E. aureopilosa; Rozen, 1984). Non-kin associations could also be found among pleometrotic foundresses of eusocial colonies, though this is rare within the bees. Low relatedness has been described for co-foundresses of the primitively eusocial sweat bee Halictus ligatus, likely arising from chance encounters among females emerging from their winter hibernacula (Richards and Packer, 1998).
An interesting case of non-kin sociality exists among the large carpenter bees in the genus Xylocopa (Apidae: Xylocopinae). Nest-joining behavior has been observed in several species, in many cases by unrelated bees (Gerling, 1982; Gerling et al., 1983; Velthuis, 1987; Michener, 1990a; Hogendoorn and Leys, 1993; Peso and Richards, 2011). However, low relatedness in social groups has only been demonstrated with molecular evidence for two species, X. sonorina and X. virginica (Ostwald et al., 2021a, this issue; Vickruck and Richards, 2021, this issue). Sociality in these groups is not easily classified, given variation and ambiguity in helping behavior, reproductive skew, and generational overlap (Gerling et al., 1989; Michener, 1990a; Hogendoorn and Velthuis, 1993). In most cases, a single dominant female per social nest will monopolize egg laying and provisioning behavior, with nestmates potentially contributing to nest guarding (Gerling et al., 1983, 1989; Hogendoorn and Velthuis, 1999; Buchmann and Minckley, 2019).
Ants: Foundress Associations and Primary Polygyny
In the ants, non-kin sociality through pleometrosis is relatively commonplace in incipient colonies, but usually ends with a queen culling event triggered by worker emergence (Bernasconi and Strassmann, 1999). However, permanent non-kin social groups can form when a pleometrotic queen association extends past worker emergence and into colony maturity. This results in primary polygyny, a group of unrelated worker lineages that share a nest, colony resources, and colony tasks. Importantly, workers in polygynous colonies may be close kin if they were produced by the same queen. Nevertheless, overall worker nestmate relatedness is often low in polygynous colonies (DeHeer and Herbers, 2004; Kellner et al., 2007). More importantly, the queens themselves represent prominent examples of non-kin cooperative behavior, analogous to cooperative breeders in other taxa, regardless of offspring group relatedness. Primary polygyny is generally found interspersed between monogynous colonies or as the majority structure in discrete populations, but has never been documented as the only social structure of an ant species.
Primary polygyny is represented in several ant subfamilies but is especially well documented in the Myrmicinae. Moser and Lewis (1981) first observed multiple unrelated queens in mature colonies of the Texas leaf-cutter ant Atta texana. Mintzer and Vinson subsequently found that these cooperative associations are stable and beneficial to A. texana queen survival in the lab (Mintzer and Vinson, 1985; Mintzer, 1987). Shortly afterward, Rissing et al. (1989) utilized allozyme markers to directly show that cohabiting Acromyrmex versicolor queens were not related and also reared stable multi-queen colonies in the lab. There is also genetic evidence, using isoenzymes, that two South American Acromyrmex species practice primary polygyny, A. striatus and A. heyeri (Diehl et al., 2001). Multiple, unrelated queens were also found in colonies of Myrmica gallienii using enzyme electrophoresis (Seppä, 1996), however colony age was not reported in this study. Primary polygyny may also occur in the fungus growing ant species, Cyphomyrmex transversus. Multiple queens were found in 37.7% of colonies examined by Ramos-Lacau et al. (2012) but it is unknown if these queens were related. Within the Myrmicinae, there are also several harvester ant species that practice primary polygyny. Pogonomyrmex californicus displays primary polygyny in southern California, as confirmed with field observation (Johnson, 2004), laboratory colonies (Clark and Fewell, 2014; Overson et al., 2014), and microsatellite analysis (Overson et al., 2016). Primary polygyny also occurs in a California population of the seed harvester Veromessor pergandei, also confirmed using microsatellites (Helms and Helms Cahan, 2012). Queens of another species in the same genus, Messor barbarous, can be induced into stable cooperative associations in the lab, but no polygynous colonies have been found in the field (Provost and Cerdan, 1990).
Within the subfamily Formicinae, the honeypot ant Myrmecosystus mimicus also practices primary polygyny in an Arizona population as confirmed by microsatellite analysis by Hölldobler et al. (2011). The mound building ant Formica podzolica exhibits primary polygyny in Colorado, as suggested by field excavation (Deslippe and Savolainen, 1995) and confirmed through microsatellite analysis (DeHeer and Herbers, 2004). Finally, multiple unrelated queens have been found in mature colonies of the pleometrotic weaver ant Oecophylla smaragdina, strongly suggesting primary polygyny (Schlüns et al., 2009).
Some of the most detailed genetic and behavioral research has been performed on species in the Ponerinae subfamily. Primary polygyny has been confirmed in Neoponera inversa through behavioral observation in the field and lab (D’Ettorre et al., 2005) as well as with multiple microsatellite analyses (Heinze et al., 2001; Kolmer et al., 2002). In a closely related species, Neoponera villosa, queen cooperation has been demonstrated in the lab (Trunzer et al., 1998) and unrelated queens have been documented in field colonies (Kellner et al., 2007), strongly suggesting primary polygyny. Mature Neoponera striata Smith colonies have also been found with multiple queens, but more work is needed on queen relatedness to confirm primary polygyny (Rodrigues et al., 2011). The arboreal trap jaw ant Odontomachus hastatus has been found in colonies containing several queens and workers, but it is unknown if these queens are related (Oliveira et al., 2011).
Primary polygyny has also been confirmed via microsatellite analysis in two species of the Myrmeciinae: the Australian jumper ant Myrmicia pilosula (Qian et al., 2012) and the red ant Myrmicia rubra (Pearson, 1982, 1983; Seppä and Walin, 1996).
Finally, in the Dolichoderinae subfamily, Hölldobler and Carlin (1985) found that the Australian meat ant Iridomyrmex purpureus is oligogynous, i.e., multiple queens share a nest but do not tolerate each other and relegate themselves to different areas of the nest. Further genetic analysis confirmed that oligogynous I. purpureus queens are unrelated and share a workforce (Carew et al., 1997). Oligogyny has also been documented in the subfamily Formicidae (Camponotus ligniperdus, Gadau et al., 1998; Camponotus herculeanus, Seppä and Gertsch, 1996).
Ecological Drivers of Non-kin Sociality
Group living may have its evolutionary origins across a particular set of ecological conditions that favor nest sharing and/or cooperation (Arnold and Owens, 1997; Krause and Ruxton, 2002; Rubenstein and Abbot, 2017). For non-kin groups especially, local ecology may be a prominent driver of group formation in the absence of strong indirect fitness benefits. Below, we discuss evidence for the evolution of non-kin sociality in the Hymenoptera as driven by five major ecological conditions/constraints: (1) predator and parasite pressures, (2) intraspecific competition, (3) physiological constraints, (4) productivity constraints, and (5) climatic stressors. Importantly, the distinctions we make between these five factors do not represent mutually exclusive conditions; rather, they are highly interactive and may even represent flip sides of the same environmental selective pressures (e.g., productivity constraints that arise from intense intraspecific competition). Together, these conditions may give rise to fitness differentials between solitary and social individuals when benefits of group living outweigh intrinsic costs of resource sharing.
Predator and Parasite Pressures
The need for communal defense represents one prominent benefit of nesting with non-kin. In particular, social defensive strategies often arise in contexts where brood is vulnerable to predation or parasitism (Alexander, 1974; Krause and Ruxton, 2002; Ward and Webster, 2016). Importantly, social nest defense can be a passive, emergent property of shared nesting rather than actively cooperative behavior. The presence of multiple females (or even males; Kukuk and Schwarz, 1988) in the nest can deter invaders by decreasing the daily time window in which the nest is unattended (Lin and Michener, 1972; Wcislo and Tierney, 2009). In other cases, labor may be divided such that guarding is a functional role of certain group members, often subordinates (Hogendoorn and Velthuis, 1995; Dunn and Richards, 2003). Indeed, task specialization on guarding can even emerge spontaneously among forced, unrelated associations of normally solitary individuals, suggesting that improved nest defense can arise in in communal nests from existing behavioral repertoires (Jeanson et al., 2005; Holbrook et al., 2009, 2013).
Although predator/parasite pressures have been broadly implicated in social evolutionary transitions (Michener and Lange, 1958; Lin and Michener, 1972; Krause and Ruxton, 2002; Wilson and Hölldobler, 2005), empirical demonstrations of the effectiveness of group defense in non-kin systems are sparse. For the sweat bee Agapostemon virescens, Abrams and Eickwort (1981) found that communal nests were more effectively defended against the cleptoparasite Nomada articulata than were solitary nests. Indeed, Lin and Michener (1972) consider parasite/predator pressures to be the major driver of sociality in the Halictidae (see also Michener and Lange, 1958). Similarly, co-founding wasps may experience reduced predation from birds and mammals relative to solitary foundresses, likely due to more continuous nest guarding (Strassman et al., 1988; Tindo et al., 2008). For other non-kin groups, guarding may function to repel conspecific intruders, but may not be an effective defense against predation and parasitism. For the facultatively social bees Xylocopa virginica and Halictus ligatus, rates of brood parasitism by Bombyliid flies were found to be no different between solitary and social nests, despite increased guard presence in social nests (Richards and Packer, 1998; Prager, 2014). Similarly, though multiple Polistes wasp foundresses may provide effective protection against intraspecific usurpation (Gamboa, 1978; Gamboa et al., 1978; Klahn, 1988), they may be no more effective in guarding against predators (Gamboa, 1978; Gamboa et al., 1978; Gibo, 1978) and parasites (Gamboa et al., 1978) than solitary foundresses, despite more continuous guard presence (Gamboa et al., 1978). However, co-founding may provide important benefits during recovery from predation attempts (Gibo, 1978; Strassman et al., 1988).
Intraspecific Competition and Resource Limitation
Grouping may arise as a response to limiting resources, especially nesting sites and food (Emlen, 1982; Hatchwell and Komdeur, 2000). Environments characterized by strong intraspecific competition may favor cooperative strategies that allow groups to exploit resources. In many cases grouping occurs in densely populated or saturated environments. Indeed, pleometrosis and primary polygyny in ants have been associated in several species with high population density (Tschinkel and Howard, 1983; Rissing and Pollock, 1986, 1991; Bennet, 1987; Trunzer et al., 1998). Likewise, for the facultatively polygynous harvester ant, Pogonomyrmex californicus, sites dominated by polygyny have higher colony density than primarily monogynous sites (Haney and Fewell, 2018). Further, colonies in the polygynous population have lower reproductive output than colonies from the monogynous population. Experimental food supplementation increased reproductive output of polygynous colonies to that of colonies from the monogynous population, suggesting that competitive, food-scarce conditions drive cooperation in this species (Haney and Fewell, 2018). Similarly, bees may adopt non-kin social strategies under food-scarce conditions, even in the absence of productivity benefits of group living. For the facultatively social carpenter bee X. pubescens, solitary nests typically outperform social nests in terms of reproductive output, due to brood mortality that results from dominance competitions in social nests (Hogendoorn, 1991, 1996). However, under conditions of food scarcity, social nesting can provide an important safeguard against pollen robbery, outweighing costs of nest sharing (Hogendoorn, 1991; Hogendoorn and Velthuis, 1993).
Nest sites can also be major limiting resources, favoring social strategies that enable nest sharing and/or increase the likelihood of nest inheritance. Carpenter bees are strongly limited by access to nest sites, creating intense competition for constructed nests that results in frequent supersedure and usurpation (Gerling et al., 1989; Buchmann and Minckley, 2019). Social nesting could feasibly provide an important defense against the threat of nest invasion, but empirical studies have demonstrated that guards of X. pubescens, though potentially valuable in preventing pollen robbery, do not effectively defend the nest from usurpers (Hogendoorn and Velthuis, 1993, 1995). Instead, subordinate joiners are likely hopeful reproductives that queue for reproductive opportunities upon the death of the dominant bee and subsequent nest inheritance (Hogendoorn and Velthuis, 1995; Richards and Course, 2015; Vickruck and Richards, 2018). Nest inheritance is likewise important for co-founding wasps (Reeve, 1991; Leadbeater et al., 2011), especially for species that reuse old nests (Queller and Strassmann, 1988). Similarly, for many communal bees, group living enables shared exploitation of valuable nest sites (Michener, 1974). In all these cases, intraspecific competition for nests promotes group living and interacts with other ecological constraints, especially energetic and labor constraints on nest construction.
Energetic and Physiological Constraints
Non-kin groups may also form in contexts that impose steep physiological costs on independent breeders. For example, animals that invest in energetically costly nest building behaviors may experience selection for strategies that reduce founding costs, such as cooperative building and/or nest inheritance (Hansell, 1987). Cooperative nest building has been documented broadly across Hymenopteran non-kin groups (West-Eberhard, 1969; Bartz and Hölldobler, 1982; Tschinkel and Howard, 1983; Rissing and Pollock, 1986; Peeters and Andersen, 1989; Danforth, 1991; Bernasconi and Strassmann, 1999; Hunt and Toth, 2017). In some cases, these benefits have been linked to ecological conditions and energetic constraints. The ground-nesting communal bee Perdita portalis excavates nests through a dense, clay layer of soil, prompting Danforth (1991) to propose energetic costs of nest construction as a major driver of sociality in this environment. Challenging excavation through hard soil may likewise favor cooperative nest construction strategies in the communal bee Macrotera texana (Danforth et al., 1996). Carpenter bees may also face particularly high energetic costs of nest building, due to the tendency of many Xylocopa species to nest in dense wood substrate. For the carpenter bee X. sonorina, the energetic cost of new nest construction is higher on average than the cost of nest inheritance, even accounting for the potential cost of renovating overused tunnels (Ostwald et al., 2021b). In this group, and more broadly, high costs of nest building can underlie intraspecific competition for existing nests. These costs may incentivize social strategies such as reproductive queuing or communal nesting, even at the expense of uncertain reproductive opportunities.
Beyond energetic costs, nest building behavior can impose physiological wear and damage. In arid habitats, nest construction behaviors could be constrained more by desiccation risk than by energetic costs. For many desert ants, nest excavation causes cuticular abrasion that increases water loss rates (Johnson, 2000), exacerbating desiccation risk, which is a major cause of foundress mortality (Johnson, 1998). Cooperative nest excavation during founding poses an important possible solution to this challenge. However, the physiological costs of excavation may not be shared equally among co-foundresses (Fewell and Page, 1999). Cahan and Fewell (2004) measured excavation task specialization in experimental pairs of the facultatively polygynous Pogonomyrmex californicus, with foundresses collected either from a typically group-founding or typically solitary-founding population. For both populations, more than half of foundress pairs divided excavation labor asymmetrically, with one foundress emerging as an excavation specialist. However, pairs from the group-founding population showed smaller asymmetries in excavation performance (Cahan and Fewell, 2004). These findings suggest that while some foundresses may experience disproportionate costs of excavation, cooperative strategies overall can reduce physiological costs of excavation for a significant portion of the population. Cooperative nest excavation and maintenance may likewise be important for some ground-nesting social bees (Danforth, 1991), but the extent to which nest excavation behavior is physiologically constrained in these groups is still unclear.
Productivity Constraints
Cooperation among non-kin can also improve productivity under harsh or competitive conditions. In particular, cooperative founding may provide competitive advantages in conditions that favor rapid nest establishment via worker production. Group founding in ants has been associated both with faster initial worker production and accelerated colony growth (Tschinkel and Howard, 1983; Rissing and Pollock, 1987; Deslippe and Savolainen, 1995; Eriksson et al., 2019; Ostwald et al., 2021c). Rapid production of a large workforce may beneficially accelerate incipient groups through the vulnerable founding period, providing a critical survival advantage for cooperatively founded colonies (Clark and Fewell, 2013; Ostwald et al., 2021c). These advantages may be especially important for colonies vulnerable to intraspecific brood raiding. Cooperative founding has been shown to improve colony survival and success during brood raiding, likely due to the protective effect of larger colony sizes (Bartz and Hölldobler, 1982; Rissing and Pollock, 1987, 1991; Eriksson et al., 2019). Increased colony size in multi-foundress nests is also associated with reduced colony failure rates for the paper wasp Polistes dominula (Tibbetts and Reeve, 2003). Importantly, cooperative foundresses may experience enhanced colony growth without increasing costly individual investment in sterile worker production. Multi-queen colonies of the harvester ant P. californicus experience faster colony growth than single queen colonies, but lower per-queen worker production (Ostwald et al., 2021c). The ability to assemble a large workforce while minimizing individual investment in non-reproductive offspring may represent an important physiological benefit of cooperation with non-relatives.
Specifically, individuals may face productivity constraints associated with resource exploitation. For example, the communal bee Macrotera texana faces severe reproductive time constraints due to its foraging dependence on Opuntia flowers that bloom for only 2–3 weeks per year (Danforth et al., 1996). Cooperative nest excavation likely enables females to exploit this time-limited resource by accelerating nest founding (Danforth et al., 1996). Similarly, increased colony activity levels in polygynous P. californicus colonies suggests both increased worker production and corresponding enhanced efforts to capitalize upon limiting food resources (Haney and Fewell, 2018). In this way, productivity constraints interact strongly with resource limitation and intraspecific competition.
Importantly, worker production benefits may not translate to enhanced production of reproductives. For P. californicus as well as for the sweat bee, Halictus ligatus, group-founding nests produce more workers but fewer reproductive offspring than solitary-foundress nests (Richards and Packer, 1998; Haney and Fewell, 2018). Polistes foundress associations are likewise associated with reduced per-capita reproductive output (Queller and Strassmann, 1988; Reeve, 1991), despite increased worker production in some species (Tibbetts and Reeve, 2003). These cases suggest that cooperation often functions not as a means to enhance reproductive output under ideal conditions, but rather as a strategy to minimize losses under constraining or challenging environmental conditions.
Climatic Stressors
Climatic factors represent fundamental ecological drivers of group living across animal taxa. In particular, cooperation may be favored in harsh or stochastic climates (Arnold and Owens, 1997; Jetz and Rubenstein, 2011; Rubenstein, 2011; Griesser et al., 2017; Lukas and Clutton-Brock, 2017; Kennedy et al., 2018). In insects, climate likewise mediates the expression of social behavior, especially through impacts on development time and seasonal activity windows, which affect the available time for rearing workers and therefore the potential for colony life to emerge (Eickwort et al., 1996; Hunt and Amdam, 2005; Hirata and Higashi, 2008; Fucini et al., 2009). These factors may be important in the evolution of eusociality by promoting generation overlap in the nest. For non-kin groups, however, that arise from stable cooperative relationships between unrelated individuals, the effects of climate on group formation are relatively unexplored.
Nevertheless, several studies point to prominent roles for climatic conditions, especially environmental temperatures, in facilitating non-kin cooperation. Among Polistes paper wasps, which can found nests with non-relatives, cooperative nest founding is associated with high temperature variability, perhaps due to buffering cooperation of sociality in unpredictable environments (Sheehan et al., 2015). Polygyny in ants has also been associated with harsh thermal environments (Heinze, 1993; Heinze and Hölldobler, 1994; Heinze and Rüppel, 2014) and with success of invasive species in their introduced environments (Holway et al., 2002; Tsutsui and Suarez, 2003). Future work should clarify mechanisms underlying this link between cooperation and success in harsh, variable, or novel thermal environments.
Precipitation can also influence the relative costs and benefits of grouping. Arid environments and drought conditions can increase soil hardness, potentially increasing excavation costs and exacerbating nest limitation for ground nesting bees, ants, and wasps (Wcislo, 1997; Michener, 2007; Purcell, 2011). Under drought conditions, Bohart and Youssef (1976) found that 30% of nests of the normally solitary sweat bee Lasioglossum galpinsiae were provisioned by multiple females. In desert ants, group founding may be a by-product of the tendency to seek refuge from desiccating conditions in shared belowground spaces (Pfennig, 1995). Under desiccating conditions, group-founding by the desert seed-harvester ant Veromessor pergandei enhanced queen survival and water content relative to solitary queens, though the mechanism for this advantage is unclear (Johnson, 2021). Shared foraging duties could feasibly reduce risk of desiccation in desert habitats. Cahan and Fewell (2004) suggest that a group-founding population of the harvester ant P. californicus occupies a habitat with lower and less predictable summer precipitation than sites occupied by solitary founding populations, suggesting possible desiccation constraints. In less arid habitats, extended periods of rain can cause nest failure for ground-nesting species. For the sweat bee Halictus ligatus, foundress cooperation may provide protection against rain-induced nest failure through enhanced nest maintenance (Richards and Packer, 1998). As such, like environmental temperature, precipitation can alternately promote or constrain cooperative behavior among non-relatives.
Discussion
Sociality can be understood as an adaptive response to ecological conditions. Non-kin groups present valuable test cases for hypotheses about the ecological drivers of group formation, in particular, because communal and co-founding strategies are nearly always facultative at the individual or population level (Ross and Matthews, 1991; Michener, 2007; Heinze et al., 2017). Studying non-kin groups usefully controls for indirect fitness benefits, thus enhancing our understanding of other, relatively neglected drivers of group formation. These systems have yielded important intraspecific demonstrations of the role of ecology in determining the adaptive value of grouping behavior. Here, we have explored five central ecological factors expected to interact with the expression of social behavior: interspecific pressures from predators and parasites, intraspecific pressures over limited resources, environmental constraints on individual physiology and productivity, and stressors associated with climate. Evidence from across Hymenopteran systems indicates that these conditions play a pivotal role in shaping non-kin social strategies.
Importantly, these ecological drivers of sociality are highly interactive. Efforts to understand sociality across a single environmental axis are limiting and often yield contradictory results (e.g., sociality alternately increasing and decreasing with latitude; Purcell, 2011). Instead, integrative approaches that accommodate these interactions can provide important insights into the complex conditions underlying grouping responses. Studies in Hymenopteran systems have emphasized interactions among intraspecific, interspecific, and abiotic selective pressures. For example, sociality can be a response to intraspecific competition for access to nests (Gerling et al., 1989; Leadbeater et al., 2011). This competition is often a direct product of physiological constraints associated with nest construction behavior (Johnson, 2000; Ostwald et al., 2021b), which can be exacerbated by climatic stressors such as low precipitation (Wcislo, 1997; Purcell, 2011). This particular nexus of challenges is an important driver of group formation among the communal and parasocial bees and polygynous ants (Danforth, 1991; Danforth et al., 1996; Cahan and Fewell, 2004). Highly competitive environments can also give rise to cooperative strategies that mitigate worker production constraints experienced by solitary foundresses. Accelerated worker production is a major benefit of cooperation among ant foundresses vulnerable to brood raiding in contexts dominated by intraspecific competition (Bartz and Hölldobler, 1982; Rissing and Pollock, 1987, 1991; Eriksson et al., 2019). Productivity constraints may also be important drivers of grouping in environments dominated by predation pressures; for group-founding wasps, increased colony sizes can provide essential resilience following predation attempts (Strassman et al., 1988). Together, these examples suggest shared sets of ecological conditions that favor cooperative behavior even when relatedness is low or absent among group members. Importantly, these conditions are not restricted geographically but instead occur at intersections of particular selective pressures that can occur across a wide variety of habitat types.
These findings in non-kin groups of ants, bees, and wasps parallel known drivers of social evolution in non-insect social systems, both kin and non-kin. Ecological constraints are prominent, known drivers of cooperative breeding in birds and mammals (Emlen, 1982, 1984; Arnold and Owens, 1997; Hatchwell and Komdeur, 2000; Shen et al., 2017). Inheritance tactics in nest-limiting environments may favor delayed dispersal and nest joining (Woolfenden and Fitzpatrick, 1978; Emlen, 1984). As with the ground-nesting ants and bees, nesting constraints may be physiological, and can be exacerbated by climatic conditions: nest excavation costs in arid conditions have been proposed as a major driver of sociality in the African mole-rats (Jarvis et al., 1994; Faulkes et al., 1997; Hansell, 2005). More broadly, low and unpredictable rainfall has been associated with the global biogeography of cooperatively breeding mammals (Lukas and Clutton-Brock, 2017). Environmental stochasticity has also been implicated in the global distribution of cooperative breeding in birds (Jetz and Rubenstein, 2011), suggesting important links between cooperation and environmental uncertainty that parallel trends described in Polistes foundress associations (Sheehan et al., 2015).
Strengthening the conceptual links among Hymenopteran and vertebrate sociality has great potential for the development of broader evolutionary frameworks explaining non-kin cooperation. Vertebrate research has benefited from a more comprehensive understanding of the taxonomic distribution of kin and non-kin sociality, especially among the cooperatively breeding birds. This knowledge base has enabled valuable phylogenetic studies highlighting the roles of environmental and life history factors in shaping social organization (Riehl, 2013; Downing et al., 2015, 2020; Cornwallis et al., 2017). The social Hymenoptera likewise present special opportunities to study non-kin sociality because it occurs frequently across closely related lineages. To our knowledge, this comparative approach has not yet been applied to the Hymenoptera in the context of kin vs. non-kin social evolution, but may be feasible for those taxa in which non-kin sociality is better documented, especially the polygynous ants.
Beyond this comparative framework, the literature on vertebrate social systems can provide social insect researchers with valuable approaches for studying direct benefits of cooperation. The social vertebrate literature is rich in explorations of the costs and benefits of well-defined cooperative behaviors, from hunting and defending food (Packer and Ruttan, 1988; Lucas and Brodeur, 2001) to detecting and repelling predators (Hamilton, 1971; Foster and Treherne, 1981) or successfully rearing offspring (Ebensperger et al., 2007; Hodge et al., 2009). Likewise, studies should investigate direct benefits of cooperative behaviors in Hymenopteran societies, for example, the effectiveness of nest defense in social vs. solitary bee nests (as in Hogendoorn and Velthuis, 1993; Prager, 2014), or the consequences of shared foraging duties in ant and wasp foundress associations (Cahan and Fewell, 2004). Importantly, the exchange of theories and ideas between vertebrate and invertebrate sociality research should be bi-directional. Insights from Hymenopteran systems have the potential to overcome many of the limitations of work with vertebrate systems. Especially given their short generation times and experimental tractability in lab settings, insect systems have the potential to fill gaps in our broader understanding of the long-term direct fitness outcomes of cooperation over multiple generations.
Current understanding of social evolution among unrelated individuals is constrained by limited knowledge of the full diversity of Hymenopteran taxa that form non-kin groups. The incidence of non-kin cooperation is likely to be greatly underestimated due to the tendency of non-kin groups to occur within otherwise solitary populations (Ross and Matthews, 1991; Michener, 2007; Heinze et al., 2017), and due to limitations associated with quantifying relatedness in some species. This knowledge gap can be addressed with simple behavioral techniques (e.g., mark-recapture or observations of nest joining; Abrams and Eickwort, 1981; Peso and Richards, 2011) and inexpensive genotyping methods (e.g., microsatellites; Moore and Kukuk, 2002). Other techniques, like radio-frequency tracking (Sumner et al., 2007; Kissling et al., 2014), have the potential to reveal nest switching patterns that maintain low relatedness in some insect groups. A first priority in future research on non-kin sociality should be to expand our understanding of the diversity of non-kin systems via integrated behavioral and molecular research. Many of the species highlighted in Table 1 currently possess incomplete evidence for non-kin sociality, especially among the wasps and bees. It is likely that non-kin groups form among many other, related species for which kinship has not yet been quantified. The same may be true for similarly structured social groups outside the Hymenoptera, especially among the termites, which can form polygynous colonies through colony fusion (DeHeer and Vargo, 2004, Deheer and Vargo, 2008; Korb and Roux, 2012).
Beyond characterizing the organization and formation of these groups, studies that relate social founding strategies to ecological conditions or compare social and solitary strategies in sympatry represent promising directions for future research. Particularly illuminating would be controlled experimental studies relating social condition to ecological conditions and, especially, to fitness outcomes. The abundance of facultatively social non-kin groups provides diverse, experimentally tractable systems in which social condition can be observed and even manipulated within a single species, thus avoiding the pitfalls of comparisons across species with very different evolutionary histories. Manipulative studies such as these could rigorously test hypotheses about proposed drivers of sociality, providing insights into the ecological conditions at the origins of group living.
Conclusion
The ecological drivers of non-kin cooperation represent a highly overlapping suite of conditions that interact to constrain solitary reproductive opportunities. Integrative research that accommodates these interactions has the potential to reveal common principles underlying social evolution broadly across animal taxa and across kin and non-kin groups. Our current understanding of the full diversity of non-kin sociality in the Hymenoptera is highly limited, but existing analyses suggest that groups containing non-relatives are more widespread than previously acknowledged. Future work should quantify relatedness across a diversity of species, and leverage these systems as models for evaluating the ecological conditions that favor group formation. Studies of known non-kin groups in the Hymenoptera have emphasized the role of harsh, competitive environments in selecting for cooperative strategies even in the absence of indirect fitness benefits. These findings parallel patterns more broadly across animal groups that indicate a major role for ecological constraints in shaping diverse forms of sociality.
Author Contributions
MO wrote the main manuscript. BH wrote the section on ant sociality. All authors contributed to manuscript editing and developing the concept for the review.
Funding
This research was supported by an NSF Graduate Research Fellowship to MO and a Momental Foundation Mistletoe Research Fellowship to MO.
Conflict of Interest
The authors declare that the research was conducted in the absence of any commercial or financial relationships that could be construed as a potential conflict of interest.
Publisher’s Note
All claims expressed in this article are solely those of the authors and do not necessarily represent those of their affiliated organizations, or those of the publisher, the editors and the reviewers. Any product that may be evaluated in this article, or claim that may be made by its manufacturer, is not guaranteed or endorsed by the publisher.
Acknowledgments
We thank Andrew Suarez and Michael Goodisman for their helpful feedback on a draft of the manuscript. We also thank Elizabeth Cash, Nicholas Dorian, and Meagan Simons for their photographs. Finally, we thank two reviewers whose comments improved the manuscript, as well as the editors of this special issue for useful feedback.
References
Abbot, P., Abe, J., Alcock, J., Alizon, S., Alpedrinha, J. A. C., Andersson, M., et al. (2011). Inclusive fitness theory and eusociality. Nature 471, E1–E4. doi: 10.1038/nature09831
Abrams, J., and Eickwort, G. (1981). Nest switching and guarding by the communal sweat bee Agapostemon virescens (Hymenoptera, Halictidae). Insectes Soc. 28, 105–116.
Arnold, K. E., and Owens, I. P. F. (1997). Cooperative breeding in birds: the role of ecology. Behav. Ecol. 10, 465–471.
Bartz, S. H., and Hölldobler, B. (1982). Colony founding in Myrmecocystus mimicus Wheeler (Hymenoptera?: Formicidae) and the evolution of foundress associations. Behav. Ecol. Sociobiol. 10, 137–147.
Beekman, M., and Oldroyd, B. P. (2008). When Workers Disunite: intraspecific Parasitism by Eusocial Bees. Annu. Rev. Entomol. 53, 19–37. doi: 10.1146/annurev.ento.53.103106.093515
Bennet, B. (1987). Ecological Differences between Monogynous and Polygynous Sibling Ant Species (Hymenoptera: Formicidae). Sociobiology 13, 249–270.
Bergmüller, R., Johnstone, R. A., Russell, A. F., and Bshary, R. (2009). Integrating cooperative breeding into theoretical concepts of cooperation. Behav. Process. 76, 61–72. doi: 10.1016/j.beproc.2007.07.001
Bernasconi, G., and Strassmann, J. E. (1999). Cooperation among unrelated individuals: the ant foundress case. Trends Ecol. Evol. 14, 477–482. doi: 10.1016/S0169-5347(99)01722-X
Beshers, S. N., and Fewell, J. H. (2001). Models of Divison of Labor in Social Insects. Ann. Rev. Entomol. 46, 413–440.
Bohart, G., and Youssef, N. (1976). The Biology and Behavior of Evylaeus galpinsiae Cockerell (Hymenoptera: Halictidae). Wasmann J. Biol. 34, 185–234.
Boomsma, J. J., and Gawne, R. (2018). Superorganismality and caste differentiation as points of no return: how the major evolutionary transitions were lost in translation. Biol. Rev. 31, 28–54. doi: 10.1111/brv.12330
Bourke, A. F. G. (2011). The validity and value of inclusive fitness theory. Proc. Biol. Sci. 278, 3313–3320. doi: 10.1098/rspb.2011.1465
Bourke, A. F. G. (2014). Hamilton’s rule and the causes of social evolution. Philos. Trans. R. Soc. Lond. B Biol. Sci. 369:20130362. doi: 10.1098/rstb.2013.0362
Brady, S. G., Sipes, S., Pearson, A., and Danforth, B. N. (2006). Recent and simultaneous origins of eusociality in halictid bees. Proc. Biol. Sci. 273, 1643–1649. doi: 10.1098/rspb.2006.3496
Brask, J. B., Croft, D. P., Edenbrow, M., James, R., Bleakley, B. H., Ramnarine, I. W., et al. (2019). Evolution of non-kin cooperation?: social assortment by cooperative phenotype in guppies. R. Soc. Open Sci. 6:181493. doi: 10.1098/rsos.181493
Buchmann, S. L., and Minckley, R. L. (2019). “Large Carpenter Bees (Xylocopa),” in Encyclopedia of Social Insects, ed. C. Starr (Cham: Springer), 1–4. doi: 10.1016/j.ympev.2008.07.024
Cahan, S. H., and Fewell, J. H. (2004). Division of labor and the evolution of task sharing in queen associations of the harvester ant Pogonomyrmex californicus. Behav. Ecol. Sociobiol. 56, 9–17. doi: 10.1007/s00265-003-0746-5
Carew, M. E., Tay, W. T., and Crozier, R. H. (1997). Polygyny via unrelated queens indicated by mitochondrial DNA variation in the Australian meat ant Iridomyrmex purpureus. Insectes Soc. 44, 7–14. doi: 10.1007/s000400050018
Clark, R. M., and Fewell, J. H. (2013). Transitioning from unstable to stable colony growth in the desert leafcutter ant Acromyrmex versicolor. Behav. Ecol. Sociobiol. 68, 163–171. doi: 10.1007/s00265-013-1632-4
Clark, R. M., and Fewell, J. H. (2014). Social dynamics drive selection in cooperative associations of ant queens. Behav. Ecol. 25, 117–123. doi: 10.1093/beheco/art093
Clements, K. C., and Stephens, D. W. (1995). Testing models of non-kin cooperation: mutualism and the Prisoner’s Dilemma. Anim. Behav. 50, 527–535.
Clutton-Brock, T. (2009). Cooperation between non-kin in animal societies. Nature 461, 51–57. doi: 10.1038/nature08366
Cornwallis, C. K., Botero, C. A., Rubenstein, D. R., Downing, P. A., West, S. A., and Griffin, A. S. (2017). Cooperation facilitates the colonization of harsh environments. Nat. Ecol. Evol. 1:57. doi: 10.1038/s41559-016-0057
Crespi, B. J., and Yanega, D. (1995). The definition of eusociality. Behav. Ecol. 6, 109–115. doi: 10.1146/annurev.ento.43.1.323
Danforth, B. N. (1991). Female Foraging and Intranest Behavior of a Communal Bee, Perdita portalis (Hymenoptera: Andrenidae). Ann. Entomol. Soc. Am. 84, 537–548.
Danforth, B. N., Neff, J. L., and Barretto-k, P. (1996). Nestmate relatedness in a communal bee, perdita texana (hymenoptera: andrenidae), based on DNA fingerprinting. Evolution 50, 276–284. doi: 10.1111/j.1558-5646.1996.tb04491.x
DeHeer, C., and Vargo, E. (2004). Colony genetic organization and colony fusion in the termite Reticulitermes flavipes as revealed by foraging patterns over time and space. Mol. Ecol. 13, 431–441. doi: 10.1046/j.1365-294x.2003.2065.x
DeHeer, C. J., and Herbers, J. M. (2004). Population genetics of the socially polymorphic ant Formica podzolica. Insectes Soc. 51, 309–316. doi: 10.1007/s00040-004-0745-1
Deheer, C. J., and Vargo, E. L. (2008). Strong mitochondrial DNA similarity but low relatedness at microsatellite loci among families within fused colonies of the termite Reticulitermes flavipes. Insectes Soc. 55, 190–199. doi: 10.1007/s00040-008-0999-0
Deslippe, R. J., and Savolainen, R. (1995). Colony Foundation and Polygyny in the Ant Formica podzolic. Behav. Ecol. Sociobiol. 37, 1–6. doi: 10.1007/s002650050166
D’Ettorre, P., Kellner, K., Delabie, J. H. C., and Heinze, J. (2005). Number of queens in founding associations of the ponerine ant Pachycondyla villosa. Insectes Soc. 52, 327–332. doi: 10.1007/s00040-005-0815-z
Diehl, E., De Araújjo, A. M., and Cavalli-Molina, S. (2001). Genetic variability and social structure of colonies in Acromyrmex heyeri and A. striatus (Hymenoptera: Formicidae). Braz. J. Biol. 61, 667–678. doi: 10.1590/s1519-69842001000400017
Downing, P. A., Cornwallis, C. K., Griffin, A. S., and Griffin, A. S. (2015). Sex, long life and the evolutionary transition to cooperative breeding in birds. Proc. Biol. Sci. 282:20151663. doi: 10.1098/rspb.2015.1663
Downing, P. A., Griffin, A. S., and Cornwallis, C. K. (2020). Group formation and the evolutionary pathway to complex sociality in birds. Nat. Ecol. Evol. 4, 479–486. doi: 10.1038/s41559-020-1113-x
Dugatkin, L. A. (2002). Animal cooperation among unrelated individuals. Naturwissenschaften 89, 533–541. doi: 10.1007/s00114-002-0379-y
Dunn, T., and Richards, M. H. (2003). When to bee social: interactions among environmental constraints, incentives, guarding, and relatedness in a facultatively social carpenter bee. Behav. Ecol. 14, 417–424. doi: 10.1093/beheco/14.3.417
Ebensperger, L. A., Hurtado, M. J., and León, C. (2007). An experimental examination of the consequences of communal versus solitary breeding on maternal condition and the early postnatal growth and survival of degu, Octodon degus, pups. Anim. Behav. 185, 185–194. doi: 10.1016/j.anbehav.2006.06.004
Eickwort, G. C., Eickwort, J., Gordon, J., and Eickwort, M. (1996). Solitary Behavior in a High-Altitude Population of the Social Sweat Bee Halictus rubicundus (Hymenoptera: Halictidae). Behav. Ecol. Sociobiol. 38, 227–233. doi: 10.1007/s002650050236
Emlen, S. T. (1982). The Evolution of Helping. I. An Ecological Constraints Model. Am. Nat. 119, 29–39. doi: 10.1086/283888
Emlen, S. T. (1984). “Cooperative breeding in birds and mammals,” in Behavioral Ecology: An Evolutionary Approach, eds J. R. Krebs and N. B. Davies (Sunderland, MA: Sinauer). doi: 10.1098/rspb.2011.2639
Eriksson, T., Hölldobler, B., Taylor, J., and Gadau, J. (2019). Intraspecific variation in colony founding behavior and social organization in the honey ant Myrmecocystus mendax. Insect. Soc. 66, 283–297. doi: 10.1007/s00040-019-00687-y
Evans, H. (1956). “The evolution of social life in wasps,” in Proceedings of the 1956 10th International Congress of Entomology, Montreal, 449–457.
Faulkes, C. G., Bennet, N. C., Bruford, M. W., O’Brien, H. P., Aguilar, G. H., and Jarvis, J. U. M. (1997). Ecological constraints drive social evolution in the African mole-rats. Proc. Biol. Sci. 264, 1619–1627. doi: 10.1098/rspb.1997.0226
Fewell, J., and Abbot, P. (2018). “Sociality,” in Insect Behavior: From Mechanisms to Ecological Consequences, eds A. Córdoba-Aguilar, D. González-Tokman, and I. González-Santoyo (Oxford: Oxford University Press), 219–235. doi: 10.1093/oso/9780198797500.003.0015
Fewell, J. H., and Page, R. (1999). The emergence of division of labour in forced associations of normally solitary ant queens. Evol. Ecol. Res. 1, 537–548.
Field, J. (2005). The evolution of progressive provisioning. Behav. Ecol. 16, 770–778. doi: 10.1093/beheco/ari054
Foster, K. R., Wenseleers, T., and Ratnieks, F. L. W. (2006). Kin selection is the key to altruism. Trends Ecol. Evol. 21, 57–60. doi: 10.1016/j.tree.2005.11.014
Foster, W. A., and Treherne, J. E. (1981). Evidence for the dilution effect in the selfish herd from fish predation on a marine insect. Nature 293, 466–467. doi: 10.1038/293466a0
Fucini, S., Di Bona, V., Mola, F., Piccaluga, C., and Lorenzi, M. (2009). Social wasps without workers: geographic variation of caste expression in the paper wasp Polistes biglumis. Insectes Soc. 56, 347–358. doi: 10.1007/s00040-009-0030-4
Gadau, J., Gertsch, P., Heinze, J., Pamilo, P., and Hölldobler, B. (1998). Oligogyny by unrelated queens in the carpenter ant, Camponotus ligniperdus. Behav. Ecol. Sociobiol. 44, 23–33. doi: 10.1007/s002650050511
Gamboa, G. J. (1978). Intraspecific defense: advantage of social cooperation among paper wasp foundresses. Science 199, 1463–1466. doi: 10.1126/science.199.4336.1463
Gamboa, G. J., Heacock, B., and Wiltjer, S. (1978). Division of labor and subordinate longevity in foundress associations of the paper wasp, Polistes Metricus (Hymenoptera: Vespidae). J. Kansas Entomol. Soc. 51, 343–352.
Gerling, D. (1982). Nesting biology and flower relationships of Xylocopa sonorina Smith in Hawaii (Hymenoptera: Anthophoridae). Pan Pac. Entomol. 58, 336–351.
Gerling, D., Hurd, P. D., and Hefetz, A. (1983). “Comparative behavioral biology of two Middle East species of carpenter bees (Xylocopa Latreille) (Hymenoptera:Apoidea). Smithson. Contr. Zool. 369, 1–33. doi: 10.5479/si.00810282.369
Gerling, D., Velthuis, H. H. W., and Hefetz, A. (1989). Bionomics of the large carpenter bees of the genus Xylocopa. Annu. Rev. Entomol. 34, 163–190. doi: 10.1146/annurev.en.34.010189.001115
Gibo, D. (1978). The selective advantage of foundress associations in Polistes fuscatus (Hymenoptera: Vespidae): a field study of the effects of predation on productivity. Can. Entomol. 110, 519–540.
Goodnight, C. J. (2005). Multilevel selection: the evolution of cooperation in non-kin groups. Popul. Ecol. 47, 3–12. doi: 10.1007/s10144-005-0207-2
Griesser, M., Drobniak, S. M., Nakagawa, S., and Botero, C. A. (2017). Family living sets the stage for cooperative breeding and ecological resilience in birds. PLoS Biol. 15:e2000483. doi: 10.1371/journal.pbio.2000483
Hamilton, W. D. (1964). The Genetical Evolution of Social Behaviour. I. J. Theor. Biol. 7, 1–16. doi: 10.1016/0022-5193(64)90038-4
Hamilton, W. D. (1971). Geometry for the Selfish Herd. J. Theor. Biol. 31, 295–311. doi: 10.1016/0022-5193(71)90189-5
Hamilton, W. D. (1972). Altruism and Related Phenomena, Mainly in Social Insects. Annu. Rev. Ecol. Syst. 3, 193–232. doi: 10.1146/annurev.es.03.110172.001205
Haney, B. R., and Fewell, J. H. (2018). Ecological drivers and reproductive consequences of non-kin cooperation by ant queens. Oecologia 187, 643–655. doi: 10.1007/s00442-018-4148-9
Hansell, M. H. (1987). “Nest building as a facilitating and limiting factor in the evolution of eusociality in the Hymenoptera,” in Oxford Surveys of Evolutionary BIology, eds P. H. Harvey and L. Partridge (Oxford: Oxford University Press), 155–181.
Hatchwell, B. J., and Komdeur, J. (2000). Ecological constraints, life history traits and the evolution of cooperative breeding. Anim. Behav. 59, 1079–1086. doi: 10.1006/anbe.2000.1394
Heinze, J., and Hölldobler, B. (1994). Ants in the cold. Mem. Zool. 48, 99–108. doi: 10.1093/ee/nvx061
Heinze, J., Kellner, K., and Seal, J. (2017). “Sociality in ants,” in Comparative Social Evolution, eds D. R. Rubenstein and P. Abbot (Cambridge: Cambridge University Press), 21–49. doi: 10.1017/9781107338319.003
Heinze, J., and Rüppel, O. (2014). The frequency of multi-queen colonies increases with altitude in a Nearctic ant. Ecol. Entomol. 39, 527–529.
Heinze, J., Trunzer, B., Hölldobler, B., and Delabie, J. H. C. (2001). Reproductive skew and queen relatedness in an ant with primary polygyny. Insectes Soc. 48, 149–153. doi: 10.1007/pl00001758
Helms, K., and Helms Cahan, S. (2012). Large-scale regional variation in cooperation and con fl ict among queens of the desert ant Messor pergandei. Anim. Behav. 84, 499–507. doi: 10.1016/j.anbehav.2012.05.019
Hirata, M., and Higashi, S. (2008). Degree-day accumulation controlling allopatric and sympatric variations in the sociality of sweat bees, Lasioglossum (Evylaeus) baleicum (Hymenoptera: Halictidae). Behav. Ecol. Sociobiol. 62, 1239–1247. doi: 10.1007/s00265-008-0552-1
Hodge, S. J., Bell, M. B. V., Mwanguhya, F., Kyabulima, S., Waldick, R. C., and Russell, A. F. (2009). Maternal weight, offspring competitive ability, and the evolution of communal breeding. Behav. Ecol. 20, 729–735. doi: 10.1093/beheco/arp053
Hogendoorn, K. (1991). Intraspecific competition in the carpenter bee Xylocopa pubescens and its implications for the evolution of sociality. Proc. Exp. Appl. Entomol. 2, 123–128.
Hogendoorn, K. (1996). Socio-economies of brood destruction during supersedure in the carpenter bee Xylocopa pubescens. J. Evol. Biol. 9, 931–952. doi: 10.1046/j.1420-9101.1996.9060931.x
Hogendoorn, K., and Leys, R. (1993). The superseded female’s dilemma: ultimate and proximate factors that influence guarding behaviour of the carpenter bee Xylocopa pubescens. Behav. Ecol. Sociobiol. 33, 371–381. doi: 10.1007/BF00170252
Hogendoorn, K., and Velthuis, H. (1995). The role of young guards in Xylocopa pubescens. Insectes Soc. 42, 427–448.
Hogendoorn, K., and Velthuis, H. H. W. (1993). The sociality of Xylocopa pubescens: Does a helper really help? Behav. Ecol. Sociobiol. 32, 247–257. doi: 10.1007/BF00166514
Hogendoorn, K., and Velthuis, H. H. W. (1999). Task allocation and reproductive skew in social mass provisioning carpenter bees in relation to age and size. Insectes Soc. 46, 198–207. doi: 10.1007/s000400050135
Holbrook, C. T., Clark, R. M., Jeanson, R., Bertram, S. M., Kukuk, P. F., and Fewell, J. H. (2009). Emergence and consequences of division of labor in associations of normally solitary sweat bees. Ethology 115, 301–310. doi: 10.1111/j.1439-0310.2009.01617.x
Holbrook, C. T. T., Kukuk, P. F., Feweu, J. H., Fewell, J. H., Holbrook, C. T. T., and Kukuk, P. F. (2013). Increased group size promotes task specialization in a normally solitary halictine bee. Behaviour 150, 1–18. doi: 10.1163/1568539X-00003104
Holland, J. G., and Bloch, G. (2020). The Complexity of Social Complexity: a quantitative multidimensional approach for studies of social organization. Am. Nat. 196, 525–540. doi: 10.1086/710957
Hölldobler, B., and Carlin, N. F. (1985). Colony Founding, Queen Dominance and Oligogyny in the Australian Meat Ant Iridomyrmex purpureus. Behav. Ecol. Sociobiol. 18, 45–58.
Hölldobler, B., Gillenberger, B., and Gadau, J. (2011). Queen number and raiding behavior in the ant genus Myrmecocystus (Hymenoptera?: Formicidae). Myremcol. News 15, 53–61.
Holway, D. A., Lach, L., Suarez, A. V., Tsutsui, N. D., and Case, T. J. (2002). The causes and consequences of ant invasions. Annu. Rev. Entomol. 33, 181–233. doi: 10.1146/annurev.ecolsys.33.010802.150444
Hughes, W. O. H., Oldroyd, B. P., Beekman, M., and Ratnieks, F. L. W. (2008). Ancestral monogamy shows kin selection is key to the evolution of eusociality. Science 320, 1213–1217. doi: 10.1126/science.1156108
Hunt, J. H., and Amdam, G. V. (2005). Bivoltinism as an antecedent to eusociality in the paper wasp genus Polistes. Science 308, 264–267. doi: 10.1126/science.1109724
Hunt, J. H., and Toth, A. L. (2017). “Sociality in Wasps,” in Comparative Social Evolution, eds D. R. Rubenstein and P. Abbot (Cambridge: Cambridge University Press), 84–123.
Jarvis, J. U. M., O’Riain, M. J., Bennett, N. C., and Sherman, P. W. (1994). Mammalian eusociality: a family affair. Trends Ecol. Evol. 9, 47–51. doi: 10.1016/0169-5347(94)90267-4
Jeanson, R., Kukuk, P. F., and Fewell, J. H. (2005). Emergence of division of labour in halictine bees: contributions of social interactions and behavioural variance. Anim. Behav. 70, 1183–1193. doi: 10.1016/j.anbehav.2005.03.004
Jetz, W., and Rubenstein, D. R. (2011). Environmental Uncertainty and the Global Biogeography of Cooperative Breeding in Birds. Curr. Biol. 21, 72–78. doi: 10.1016/j.cub.2010.11.075
Johnson, R. A. (1998). Foundress survival and brood production in the desert seed-harvester ants Pogonomyrmex rugosus and P. barbatus (Hymenoptera, Formicidae). Insectes Soc. 45, 255–266. doi: 10.1007/s000400050086
Johnson, R. A. (2000). Water loss in desert ants: caste variation and the effect of cuticle abrasion. Physiol. Entomol. 25, 48–53. doi: 10.1046/j.1365-3032.2000.00170.x
Johnson, R. A. (2004). Colony founding by pleometrosis in the semiclaustral seed-harvester ant Pogonomyrmex californicus (Hymenoptera?: Formicidae). Anim. Behav. 68, 1189–1200. doi: 10.1016/j.anbehav.2003.11.021
Johnson, R. A. (2021). Desiccation limits recruitment in the pleometrotic desert seed-harvester ant Veromessor pergandei. Ecol. Evol. 11, 294–308. doi: 10.1002/ece3.7039
Kellner, K., Trindl, A., Heinze, J., and D’Ettorre, P. (2007). Polygyny and polyandry in small ant societies. Mol. Ecol. 16, 2363–2369. doi: 10.1111/j.1365-294X.2007.03297x
Kennedy, P., Higginson, A. D., Radford, A. N., and Sumner, S. (2018). Altruism in a volatile world. Nature 555, 359–362. doi: 10.1038/nature25965
Kissling, W. D., Pattemore, D. E., and Hagen, M. (2014). Challenges and prospects in the telemetry of insects. Biol. Rev. 89, 511–530. doi: 10.1111/brv.12065
Klahn, J. (1979). Philopatric and nonphilopatric foundress associations in the social Wasp Polistes fuscatus. Behav. Ecol. Sociobiol. 5, 417–424. doi: 10.1007/bf00292528
Klahn, J. (1988). Intraspecific Comb Usurpation in the Social Wasp Polistes fuscatus. Behav. Ecol. Sociobiol. 23, 1–8. doi: 10.1007/bf00303051
Kolmer, K., Hölldobler, B., and Heinze, J. (2002). Colony and population structure in Pachycondyla cf. inversa, a ponerine ant with primary polygyny. Ethol. Ecol. Evol. 14, 37–41. doi: 10.1080/08927014.2002.9522754
Korb, J., and Roux, E. A. (2012). Why join a neighbour: fitness consequences of colony fusions in termites. J. Evol. Biol. 25, 2161–2170. doi: 10.1111/j.1420-9101.2012.02617.x
Kudo, S., Ohara, M., and Shinohara, A. (1998). Notes on Brooding Behavior in the Sawfly Nematus tiliae (Hymenoptera: Tenthredinidae: Nematinae). J. N. Y. Entomol. Soc. 106, 209–211.
Kukuk, P., and Sage, G. (1994). Reproductivity and relatedness in a communal halictine bee Lasioglossum (Chilalictus) hemichalceum. Insectes Soc. 41, 443–455. doi: 10.1007/bf01240647
Kukuk, P. F., Bitney, C. L. A., and Forbes, S. T. E. H. (2005). Maintaining low intragroup relatedness: evolutionary stability of nonkin social groups. Anim. Behav. 70, 1305–1311. doi: 10.1016/j.anbehav.2005.03.015
Kukuk, P. F., and Schwarz, M. (1988). Macrocephalic male bees as functional reproductives and probable guards. Pan Pac. Entomol. 64, 131–137.
Leadbeater, E., Carruthers, J. M., Green, J. P., Rosser, N. S., and Field, J. (2011). Nest inheritance is the missing source of direct fitness in a primitively eusocial insect. Science 333, 874–876. doi: 10.1126/science.1205140
Leadbeater, E., Carruthers, J. M., Green, J. P., and Van Heusden, J. (2010). Unrelated helpers in a primitively Eusocial wasp: Is helping tailored towards direct fitness? PLoS One 5:e11997. doi: 10.1371/journal.pone.0011997
Lehmann, L., and Keller, L. (2006). The evolution of cooperation and altruism – a general framework and a classification of models. J. Evol. Biol. 19, 1365–1376. doi: 10.1111/j.1420-9101.2006.01119.x
Linksvayer, T. A., and Johnson, B. R. (2019). Re-thinking the social ladder approach for elucidating the evolution and molecular basis of insect societies. Curr. Opin. Insect Sci. 34, 123–129. doi: 10.1016/j.cois.2019.07.003
Linksvayer, T. A., and Wade, M. J. (2011). The Evolutionary Origin and Elaboration of Sociality in the Aculeate Hymenoptera?: maternal Effects, Sib - Social Effects, and Heterochrony. Q. Rev. Biol. 80, 317–336. doi: 10.1086/432266
Lucas, E., and Brodeur, J. (2001). A fox in sheep’s clothing: furtive predators benefit from the communal defense of their prey. Ecology 82, 3246–3250. doi: 10.1890/0012-9658(2001)082[3246:afissc]2.0.co;2
Lukas, D., and Clutton-Brock, T. (2017). Climate and the distribution of cooperative breeding in mammals. R. Soc. Open Sci 4, 160897. doi: 10.1098/rsos.160897
MacCormack, A. (1982). Foundress Associations and Early Colony Failure in Polistes exclamans. Chapel Hill, NC: University of North Carolina at Chapel Hill.
Michener, C. D. (1966). Evidence of Cooperative Provisioning of Cells in Exomalopsis (Hymenoptera: Anthophoridae). J. Kansas Entomol. Soc. 39, 315–317.
Michener, C. D. (1969). Comparative social behavior of bees. Annu. Rev. Entomol. 14, 299–342. doi: 10.1146/annurev.en.14.010169.001503
Michener, C. D. (1974). The Social Behavior of the Bees: A Comparative Study. Cambridge, MA: Harvard University Press.
Michener, C. D. (1990a). “Castes in Xylocopine Bees,” in Social Insects: An Evolutionary Approach to Castes and Reproduction, ed. W. Engel (Berlin: Springer Verlag), 123–146. doi: 10.1007/978-3-642-74490-7_7
Michener, C. D. (1990b). “Reproduction and Castes in Social Halictine Bees,” in Social Insects: An Evolutionary Approach to Castes and Reproduction, ed. W. Engel (Berlin: Springer Verlag), 77–121. doi: 10.1007/978-3-642-74490-7_6
Michener, C. D. (2007). The Bees of the World, 2nd Edn. Baltimore, MD: Johns Hopkins University Press.
Michener, C. D., and Lange, R. B. (1958). Observations on the behavior of Brazilian Halictid bees (Hymenoptera, Apoidea) I. Pseudagapostemon. Ann. Entomol. Soc. Am. 51, 155–164. doi: 10.1093/aesa/51.2.155
Mintzer, A. (1987). Primary polygyny in the ant Atta texana: number and weight of females nad colony foundation success in the laboratory. Insectes Soc. 34, 108–117.
Mintzer, A., and Vinson, S. (1985). Cooperative Colony Foundation by Females of the Leafcutting Ant Atta texana in the Laboratory. J. N. Y. Entomol. Soc. 93, 1047–1051.
Moore, A. J., and Kukuk, P. F. (2002). Quantitative genetic analysis of natural populations. Nat. Rev. Genet. 3, 971–978. doi: 10.1038/nrg951
Mora-Kepfer, F. (2014). Context-dependent acceptance of non-nestmates in a primitively eusocial insect. Behav. Ecol. Sociobiol. 68, 363–371. doi: 10.1007/s00265-013-1650-2
Moser, J., and Lewis, J. (1981). Multiple nests of Atta texana (Buckley 1860): (Hymenoptera: formicidae). Turrialba 31, 256–257.
Nonacs, P., and Reeve, H. K. (1993). Opportunistic adoption of orphaned nests in paper wasps as an alternative reproductive strategy. Behav. Process. 30, 47–59. doi: 10.1016/0376-6357(93)90011-F
Oliveira, P. S., Camargo, R. X., and Fourcassié, V. (2011). Nesting patterns, ecological correlates of polygyny and social organization in the neotropical arboreal ant Odontomachus hastatus (Formicidae, Ponerinae). Insectes Soc. 58, 207–217. doi: 10.1007/s00040-010-0138-6
Ostwald, M., Dahan, R., Shaffer, Z., and Fewell, J. (2021a). Fluid nest membership drives variable relatedness in groups of a facultatively social bee. Front. Ecol. Evol. 9:767380. doi: 10.3389/fevo.2021.767380
Ostwald, M. M., Fox, T. P., Harrison, J. F., and Fewell, J. H. (2021b). Social consequences of energetically costly nest construction in a facultatively social bee. Proc. R. Soc. B 288:20210033. doi: 10.1098/rspb.2021.0033
Ostwald, M. M., Guo, X., Wong, T., Malaekeh, A., Harrison, J. F., and Fewell, J. H. (2021c). Cooperation among unrelated ant queens provides persistent growth and survival benefits during colony ontogeny. Sci. Rep. 11:8332. doi: 10.1038/s41598-021-87797-5
Overson, R., Fewell, J., and Gadau, J. (2016). Distribution and origin of intraspecific social variation in the California harvester ant Pogonomyrmex californicus. Insectes Soc. 63, 531–541. doi: 10.1007/s00040-016-0497-8
Overson, R., Gadau, J., Clark, R. M., Pratt, S. C., and Fewell, J. H. (2014). Behavioral transitions with the evolution of cooperative nest founding by harvester ant queens. Behav. Ecol. Sociobiol. 68, 21–30. doi: 10.1007/s00265-013-1618-2
Packer, C., and Ruttan, L. (1988). The evolution of cooperative hunting. Am. Nat. 132, 159–198. doi: 10.1007/s00427-019-00640-w
Pamilo, P. (1989). Estimating relatedness in social groups. Trends Ecol Evol. 4, 16–18. doi: 10.1016/0169-5347(89)90091-8
Paxton, R. J., Kukuk, P. F., and Tengö, J. (1999). Effects of familiarity and nestmate number on social interactions in two communal bees, Andrena scotica and Panurgus calcaratus (Hymenoptera, Andrenidae). Insectes Soc. 46, 109–118.
Paxton, R. J., Thoren, P., Tengö, J., Estoup, A., and Pamilo, P. (1996). Mating structure and nestmate relatedness in a communal bee, Andrena jacobi (Hymenoptera, Andrenidae), using microsatellites. Mol. Ecol. 5, 511–519. doi: 10.1111/j.1365-294x.1996.tb00343.x
Pearson, B. (1982). Relatedness of Normal Queens (Macrogynes) in Nests of the Polygynous Ant Myrmica rubra Latreille. Evolution 36, 107–112. doi: 10.1111/j.1558-5646.1982.tb05015.x
Pearson, B. (1983). Intra-Colonial Relatedness Amongst Workers in a Population of Nests of the Polygynous Ant, Myrmica rubra Latreille. Behav. Ecol. Sociobiol. 12, 1–4. doi: 10.1007/bf00296926
Peeters, C., and Andersen, A. (1989). Cooperation between dealate queens during colony foundation in the green tree ant, Oecophylla smaragdina. Psyche 96, 39–45.
Peso, M., and Richards, M. H. (2011). Not all who wander are lost: nest fidelity in Xylocopa virginica examined by mark recapture. Insectes Soc. 58, 127–133. doi: 10.1007/s00040-010-0125-y
Pfennig, W. (1995). Absence of joint nesting advantage in desert seed harvester ants: evidence from a field experiment. Anim. Behav. 49, 567–575. doi: 10.1016/0003-3472(95)90032-2
Prager, S. M. (2014). Comparison of social and solitary nesting carpenter bees in sympatry reveals no advantage to social nesting. Biol. J. Linn. Soc. 113, 998–1010. doi: 10.1111/bij.12395
Provost, E., and Cerdan, P. (1990). Experimental Polygyny and Colony Closure in the Ant Messor barbarus (L.) (Hym, Formicidae). Behaviour 115, 114–126. doi: 10.1163/156853990x00310
Purcell, J. (2011). Geographic patterns in the distribution of social systems in terrestrial arthropods. Biol. Rev. 86, 475–491. doi: 10.1111/j.1469-185X.2010.00156.x
Qian, Z., Schlick-Steiner, B., Steiner, F., Robson, S., Schlüns, H., Schlüns, E., et al. (2012). Colony genetic structure in the Australian jumper ant Myrmecia pilosula. Insectes Soc. 59, 109–117. doi: 10.1007/s00040-011-0196-4
Queller, D. C. (2011). Expanded social fitness and Hamilton’s rule for kin, kith, and kind. Proc. Natl. Acad. Sci. U.S.A. 108, 10792–10799. doi: 10.1073/pnas.1100298108
Queller, D. C., and Strassmann, J. E. (1988). “Reproductive success and group nesting in the paper wasp, Polistes annularis,” in Reproductive Success: Studies of Individual Variation in Contrasting Breeding Systems, ed. T. H. Clutton-Brock (Chicago, IL: University of Chicago Press), 76–96.
Queller, D. C., and Strassmann, J. E. (1998). Kin selection and social insects. Bioscience 48, 165–175.
Queller, D. C., Zacchi, F., Cervo, R., Turillazzi, S., Henshaw, M. T., Santorelli, L. A., et al. (2000). Unrelated helpers in a social insect. Nature 405, 784–787. doi: 10.1038/35015552
Ramos-Lacau, L. S., Silva, P. S. D., Lacau, S., Delabie, H. C., and Bueno, O. C. (2012). Nesting architecture and population structure of the fungus-growing ant Cyphomyrmex transversus (Formicidae: Myrmicinae: Attini) in the Brazilian coastal zone of Ilhéus, Bahia. Ann. Soc. Entomol. France 9271, 439–445. doi: 10.1080/00379271.2012.10697789
Raw, A. (1977). The biology of two Exomalopsis species (Hymenoptera: Anthophoridae) with remarks on sociality in bees. Rev. Biol. Trop. 25, 1–11.
Reeve, H. (1991). “Polistes,” in The Social Biology of Wasps, eds K. G. Ross and R. Matthews (Ithaca, NY: Comstock Publishing Associates), 99–143.
Rehan, S. M., and Toth, A. L. (2015). Climbing the social ladder: the molecular evolution of sociality. Trends Ecol. Evol. 30, 426–433. doi: 10.1016/j.tree.2015.05.004
Richards, M. H. (2019). ScienceDirect Social trait definitions influence evolutionary inferences: a phylogenetic approach to improving social terminology for bees. Curr. Opin. Insect Sci. 34, 97–104. doi: 10.1016/j.cois.2019.04.006
Richards, M. H., and Course, C. (2015). Ergonomic skew and reproductive queuing based on social and seasonal variation in foraging activity of eastern carpenter bees (Xylocopa virginica). Can. J. Zool. 93, 615–625. doi: 10.1139/cjz-2014-0330
Richards, M. H., and Packer, L. (1998). Demography and relatedness in multiple-foundress nests of the social sweat bee, Halictus ligatus. Insectes Soc. 45, 97–109. doi: 10.1007/s000400050072
Richards, M. H., Von Wettberg, E. J., and Rutgers, A. C. (2003). A novel social polymorphism in a primitively eusocial bee. Proc. Natl. Acad. Sci. U.S.A. 100, 7175–7180. doi: 10.1073/pnas.1030738100
Riehl, C. (2013). Evolutionary routes to non-kin cooperative breeding in birds. Proc. R. Soc. B 280:20132245. doi: 10.1098/rspb.2013.2245
Rissing, S., and Pollock, G. (1991). An experimental analysis of pleometrotic advantage in the desert seed-harvester ant Messor pergandei (Hymenoptera?; Formicidae). Insectes Soc. 38, 205–211. doi: 10.1007/bf01240970
Rissing, S. W., and Pollock, G. B. (1986). Social interaction among pleometrotic queens of Veromessor pergandei (Hymenoptera:Formicidae) during colony foundation. Anim. Behav. 34(PART 1), 226–233. doi: 10.1016/0003-3472(86)90027-8
Rissing, S. W., and Pollock, G. B. (1987). Queen aggression, pleometrotic advantage and brood raiding in the ant Veromessor pergandei (Hymenoptera: Formicidae). Anim. Behav. 35, 975–981. doi: 10.1016/S0003-3472(87)80154-9
Rissing, S. W., Pollock, G. B., Higgins, M. R., Hagen, R. H., and Smith, D. R. (1989). Foraging specialization without relatedness or dominance among co-founding ant queens. Nature 338, 420–422. doi: 10.1038/338420a0
Rodrigues, M. S., Vilela, E. F., Azevedo, D. O., and Hora, R. R. (2011). Multiple Queens in Founding Colonies of the Neotropical Ant Pachycondyla striata Smith (Formicidae: Ponerinae). Neotrop. Entomol. 40, 293–299. doi: 10.1590/s1519-566x2011000300001
Ross, K., and Matthews, R. (eds) (1991). The Social Biology of Wasps. Ithaca, NY: Comstock Publishing Associates.
Rozen, J. (1984). Comparative Nesting Biology of the Bee Tribe Exomalopsini (Apoidea, Anthophoridae). Am. Mus. Novit. 2798, 1–37.
Rozen, J. G. (1991). Evolution of cleptoparasitism in anthophorid bees as revealed by their mode of parasitism and first instars (Hymenoptera, Apoidea). Am. Mus. Novit. 3029, 1–36.
Rubenstein, D. R. (2011). Spatiotemporal environmental variation, risk aversion, and the evolution of cooperative breeding as a bet-hedging strategy. Proc. Natl. Acad. Sci. U.S.A. 108, 10816–10822. doi: 10.1073/pnas.1100303108
Rubenstein, D. R., and Abbot, P. (eds) (2017). Comparative Social Evolution. Cambridge: Cambridge University Press.
Sakagami, S., and Zucchi, R. (1978). Nests of Hylaeus (Hylaeopsis) tricolor: the First Record of Non-Solitary Life in Colletid Bees, with Notes on Communal and Quasisocial Colonies (Hymenoptera: Colletidae). J. Kansas Entomol. Soc. 51, 597–614.
Schlüns, E., Wegener, B., Schlüns, H., Azuma, N., Robson, S., and Crozier, R. (2009). Breeding system, colony and population structure in the weaver ant Oecophylla smaragdina. Mol. Ecol. 18, 156–167. doi: 10.1111/j.1365-294X.2008.04020.x
Seeley, T. D. (1996). The Wisdom of the Hive: The Social Physiology of Honey Bee Colonies. Cambridge, MA: Harvard University Press.
Seppä, P. (1996). Genetic relatedness and colony structure in polygynous Myrmica ants. Ethol. Ecol. Evol. 8, 279–290. doi: 10.1080/08927014.1996.9522918
Seppä, P., and Gertsch, P. (1996). Genetic relatedness in the ant Camponotus herculeanus. A comparison of estimates from allozyme and DNA microsatellite markers. Insectes Soc. 43, 235–246. doi: 10.1007/bf01242925
Seppä, P., and Walin, L. (1996). Sociogenetic Organization of the Red Ant Myrmica rubra. Behav. Ecol. Sociobiol. 38, 207–217. doi: 10.1007/s002650050234
Sheehan, M., Botero, C., Hendry, T., Sedio, B., Jandt, J., Weiner, S., et al. (2015). Different axes of environmental variation explain the presence vs. extent of cooperative nest founding associations in Polistes paper wasps. Ecol. Lett. 18, 1057–1067. doi: 10.1111/ele.12488
Shen, S., Emlen, S., Koenig, W., and Rubenstein, D. (2017). The ecology of cooperative breeding behaviour. Ecol. Lett. 20, 708–720. doi: 10.1111/ele.12774
Simon, H. A. (1990). A mechanism for social selection and successful altruism. Science 250, 1665–1668. doi: 10.1126/science.2270480
Spessa, A., Schwarz, M. P., and Adams, M. (2000). Sociality in Amphylaeus morosus (Hymenoptera: Colletidae: Hylaeinae). Ann. Entomol. Soc. Am. 93, 684–692. doi: 10.1603/0013-8746(2000)093[0684:siamhc]2.0.co;2
Strassman, J., Queller, D., and Hughes, C. (1988). Predation and the Evolution of Sociality in the Paper Wasp Polistes Bellicosus. Ecology 69, 1497–1505. doi: 10.2307/1941647
Strassmann, J. E., Hughes, C., Turillazzi, S., Solís, C., and Queller, D. C. (1994). Genetic relatedness and incipient eusociality in stenogastrine wasps. Anim. Behav. 48, 813–821. doi: 10.1006/anbe.1994.1305
Suarez, A. V., and Goodisman, M. A. D. (2021). Non-kin cooperation in ants. Front. Ecol. Evol. 9:736757. doi: 10.3389/fevo.2021.736757
Sumner, S., Lucas, E., Barker, J., and Isaac, N. (2007). Radio-tagging technology reveals extreme nest-drifting behavior in a eusocial insect. Curr. Biol. 17, 140–145. doi: 10.1016/j.cub.2006.11.064
Tibbetts, E. A., and Reeve, H. K. (2003). Benefits of foundress associations in the paper wasp Polistes dominulus: increased productivity and survival, but no assurance of fitness returns. Behav. Ecol. 14, 510–514. doi: 10.1093/beheco/arg037
Tindo, M., Kenne, M., and Dejean, A. (2008). Advantages of multiple foundress colonies in Belonogaster juncea juncea L.: greater survival and increased productivity. Ecol. Entomol. 33, 293–297. doi: 10.1111/j.1365-2311.2007.00966.x
Toth, A. L., Sumner, S., and Jeanne, R. L. (2016). Patterns of longevity across a sociality gradient in vespid wasps. Curr. Opin. Insect Sci. 16, 28–35. doi: 10.1016/j.cois.2016.05.006
Trivers, R. L., and Hare, H. (1976). Haplodiploidy and the Evoluti of the Social Insects. Science 191, 249–263. doi: 10.1098/rspb.2011.1257
Trunzer, B., Heinze, J., and Hölldobler, B. (1998). Cooperative colony founding and experimental primary polygyny in the ponerine ant Pachycondyla villosa. Insectes Soc. 45, 267–276.
Tschinkel, W. R., and Howard, D. F. (1983). Colony Founding by Pleometrosis in the Fire Ant, Solenopsis invicta. Behav. Ecol. Sociobiol. 12, 103–113. doi: 10.1007/bf00343200
Tsutsui, N. D., and Suarez, A. V. (2003). The colony structure and population biology of invasive ants. Conserv. Biol. 17, 48–58. doi: 10.1046/j.1523-1739.2003.02018.x
Velthuis, H. H. W. (1987). The Evolution of Sociality: ultimate and proximate factors leading to primitive social behavior in carpenter bees. Exp. Suppl. 54, 405–430.
Vickruck, J. L., and Richards, M. H. (2018). Linear dominance hierarchies and conditional reproductive strategies in a facultatively social carpenter bee. Insectes Soc. 64, 619–629. doi: 10.1007/s00040-018-0653-4
Vickruck, J., and Richards, M. (2021). Competition drives group formation and reduces within nest relatedness in a facultatively social carpenter bee. Front. Ecol. Evol. 9:738809. doi: 10.3389/fevo.2021.738809
Vogel, M. E., and Kukuk, P. F. (1994). Individual Foraging Effort in the Facultatively Social Halictid Bee, Nomia (Austronomia) australica (Smith). J. Kansas Entomol. Soc. 67, 225–235.
Ward, A., and Webster, M. (2016). Sociality: The Behaviour of Group-Living Animals. Berlin: Springer. doi: 10.1007/978-3-319-28585-6
Wcislo, W., Low, B., and Karr, C. (1985). Parasite pressure and repeated burrow use by different individuals of Crabro. Sociobiology 11, 115–125.
Wcislo, W. T. (1993). Communal Nesting in a North American Pearly-Banded Bee, Nomia tetrazonata, with Notes on Nesting Behavior of Dieunomia heteropoda (Hymenoptera: Halictidae: Nomiinae). Ann. Entomol. Soc. Am. 86, 813–821. doi: 10.1093/aesa/86.6.813
Wcislo, W. T. (1997). “Behavioral environments of sweat bees (Halictinae) in relation to variability in social organization,” in The Evolution of Social Behavior in Insects and Arachnids, eds J. Choe and B. Crespi (Cambridge: Cambridge University Press), 316–332. doi: 10.1017/cbo9780511721953.016
Wcislo, W. T., and Engel, M. S. (1996). Social Behavior and Nest Architecture of Nomiine Bees (Hymenoptera: Halictidae; Nomiinae). J. Kansas Entomol. Soc. 69, 158–167.
Wcislo, W. T., and Fewell, J. H. (2017). “Sociality in Bees,” in Comparative Social Evolution, eds D. R. Rubenstein and P. Abbot (Cambridge: Cambridge University Press), 50–83. doi: 10.1017/9781107338319.004
Wcislo, W. T., and Tierney, S. M. (2009). “The Evolution of Communal Behavior in Bees and Wasps: an Alternative to Eusociality,” in Organization of Insect Societies: From Genome to Sociocomplexity, eds J. Gadau and J. H. Fewell (Cambridge, MA: Harvard University Press), 148–169. doi: 10.2307/j.ctv228vr0t.11
Wcislo, W. T., West-Eberhard, M. J., and Eberhard, W. G. (1988). Natural History and Behavior of a Primitively Social Wasp, Auplopus semialatus, and Its Parasite, Irenangelus eberhardi (Hymenoptera: Pompilidae). J. Insect Behav. 1, 247–260. doi: 10.1007/bf01054524
West, S. A., Gardner, A., Shuker, D. M., Reynolds, T., Burton-chellow, M., Sykes, E. M., et al. (2006). Cooperation and the scale of competition in humans. Curr. Biol. 16, 1103–1106. doi: 10.1016/j.cub.2006.03.069
West, S. A., Griffin, A. S., and Gardner, A. (2007). Social semantics: altruism, cooperation, mutualism, strong reciprocity and group selection. J. Evol. Biol. 20, 415–432. doi: 10.1111/j.1420-9101.2006.01258.x
West-Eberhard, M. J. (1969). The social biology of polistine wasps. Univ. Mich. Mus. Zool. Misc. Publ. 140, 1–96.
West-Eberhard, M. J. (1975). The evolution of social behavior by Kin selection. Q. Rev. Biol. 50, 1–33.
Wilkinson, G. S., Carter, G. G., Bohn, K. M., Adams, D. M., and Wilkinson, G. S. (2016). Non-kin cooperation in bats. Philos. Trans. R. Soc. B 371:20150095. doi: 10.1098/rstb.2015.0095
Wilson, E. O., and Hölldobler, B. (2005). Eusociality: origin and consequences. Proc. Natl. Acad. Sci. U.S.A. 102, 13367–13371. doi: 10.1073/pnas.0505858102
Woolfenden, G. E., and Fitzpatrick, J. W. (1978). The inheritance of territory in group-breeding birds. Bioscience 28, 104–108. doi: 10.2307/1307423
Zanette, L., and Field, J. (2008). Genetic relatedness in early associations of Polistes dominulus: from related to unrelated helpers. Mol. Ecol. 17, 2590–2597. doi: 10.1111/j.1365-294X.2008.03785.x
Keywords: social evolution, relatedness, kinship, wasps, bees, ants
Citation: Ostwald MM, Haney BR and Fewell JH (2022) Ecological Drivers of Non-kin Cooperation in the Hymenoptera. Front. Ecol. Evol. 10:768392. doi: 10.3389/fevo.2022.768392
Received: 31 August 2021; Accepted: 05 January 2022;
Published: 09 February 2022.
Edited by:
Floria M. K. Uy, University of Rochester, United StatesReviewed by:
Heikki Helanterä, University of Oulu, FinlandAlessandro Cini, University College London, United Kingdom
Copyright © 2022 Ostwald, Haney and Fewell. This is an open-access article distributed under the terms of the Creative Commons Attribution License (CC BY). The use, distribution or reproduction in other forums is permitted, provided the original author(s) and the copyright owner(s) are credited and that the original publication in this journal is cited, in accordance with accepted academic practice. No use, distribution or reproduction is permitted which does not comply with these terms.
*Correspondence: Madeleine M. Ostwald, ostwald.madeleine@gmail.com