- 1GLOBE Institute, University of Copenhagen, Copenhagen, Denmark
- 2DOF-BirdLife Denmark, Copenhagen, Denmark
- 3Natural History Museum, Copenhagen, Denmark
- 4Center for Global Mountain Biodiversity, GLOBE Institute, University of Copenhagen, Copenhagen, Denmark
Seasonal variation in the composition of avian communities is poorly documented in African montane forests. Using field observations, mist-netting data (63,424 NMH), recording of mixed-species flocks (160 flocks noted), and of the feeding ecology of greenbuls (757 observations), we document marked seasonal changes in a bird community in an East African montane forest (1,340–2,130 m) in the Udzungwa Mountains in south-central Tanzania. This mountain range contains the largest remaining forests in the Eastern Arc Mountains in Tanzania, part of a key global biodiversity hotspot. Our analysis is based on data in the dry and wet season from about 12 months of fieldwork. Field observation data combined with mist-net data demonstrate noticeable seasonal changes in certain species’ abundances, indicating (i) seasonal movements out of the montane forest during the dry season and (ii) movements of part of the populations for other species. Our mist-net results show a significant difference in species abundances between the two seasons driven by 16 species. We also document significant changes in diet for two species of greenbuls, which shift feeding behavior from arthropods in the wet season to include a larger proportion of fruit in their dry season diet. Our results further show that birds are more active in mixed-species flocks in the dry season, with a significantly higher average number of species and of individuals in the dry season, i.e., 11.3 (±0.52 SE) species, 32.3 (±1.76 SE) individuals] compared to the wet season 9.7 (±0.78 SE) species, 20.8 (±1.85 SE) individuals]. One of two very distinctive types of mixed-species flocks – confined to the understory – exists only in the dry season. We discuss these changes to seasonal variability in climate, i.e., temperature and precipitation.
Introduction
Tropical mountains are centers of global biodiversity, endemism and threatened species (McCain and Colwell, 2011), with rich aggregations of small-ranged species that form centers of endemism (Rahbek et al., 2019a), and contribute disproportionately to the terrestrial biodiversity of Earth, especially in the tropics (Rahbek et al., 2019b). At the same time, the role of mountains as refugia for biodiversity is under serious human threat (IPBES, 2019; Rahbek et al., 2019b).
Climate change is accelerating (IPCC, 2021) and is considered one of the most important drivers of biodiversity loss after changes in land use (IPBES, 2019). Fluctuations in climate conditions are expected to intensify further due to increasing temperatures (IPCC, 2021). Coupled with the high rates of deforestation, forest fragmentation, and degradation in tropical regions (Laurance et al., 2011), biodiversity is severely threatened (Vieira et al., 2008; Eglington and Pearce-Higgins, 2012). This is particularly so in Africa, where deforestation rates are among the highest in the tropics (Lambina et al., 2001; Boko et al., 2007; IPBES, 2018).
On the mountains’ elevational gradients, projections of precipitation changes and rising temperature have been shown to have the potential of jeopardizing bird and mammal populations (McCain and Colwell, 2011). Especially, increased drying conditions seem to be developing in areas of Africa (Oguntunde et al., 2017). Additionally, elevational gradients present opportunities for species currently living near their upper thermal limits to track cooler temperatures upslope in warming climates, but only if changes in precipitation are sufficiently in step with temperature (McCain and Colwell, 2011). Temperate species generally have broad thermal tolerances because they are adapted to a more variable climate compared to tropical species (Khaliq et al., 2014), whereas in the tropics, montane species tend to have narrow tolerances, and therefore, montane species are expected to be particularly vulnerable to climate change (Araújo and Rahbek, 2006; Forero-Medina et al., 2011; Laurance et al., 2011; Santillan et al., 2018).
Species will differ in their sensitivity to climate and land-use change, depending on their niche breadth as it relates to habitat and diet (Carscadden et al., 2020). Tropical montane species often have narrow niches adapted to pockets of suitable habitats. Habitat specialists and insectivorous bird species will be more sensitive to disturbances than habitat generalists. The latter will have a greater chance of surviving and thriving in a wider variety of disturbed or seasonally variable habitats (Mulwa et al., 2012; Reif et al., 2013; Powell et al., 2015).
Seasonal changes in the structure of tropical and subtropical species communities have been known for decades (e.g., Karr, 1976), and temporal fluctuations in species abundance are widespread (e.g., Loiselle and Blake, 1991; Malizia, 2001), caused, e.g., by abiotic factors such as rainfall and temperature resulting in changes in food availability and thermal conditions. In particular, rainfall seasonality may result in a bottleneck of resources (insects, nectar, and fruit) during the dry season, which limits the population size of many species (Williams and Middleton, 2008). In Afrotropical climates with pronounced and severe dry/wet seasonal differences, anomalies may be expected to greatly affect the ecosystems (Parmesan and Yohe, 2003; Root et al., 2003), including the composition and dynamics of forest bird communities (see also Pounds et al., 1999). An increasing dryness and disruption of food resources may have a higher impact at higher elevations, where the temperature is lower and therefore influences, e.g., the likelihood of a low abundance of a given bird species. This could disrupt the connectedness among and between species and lead to a reformation of species communities and to extirpations (Root et al., 2003).
Here, we document the seasonal changes in an Afromontane forest bird community from the Ndundulu Mountains. In the Udzungwa Mountains, a global biodiversity hotspot in Tanzania (Wasser and Lovett, 1993; Myers et al., 2000; Dinesen et al., 2001). Based on three simultaneous studies from 1991 to 1992, we provide evidence of changes in community composition, abundance, and behavior between a cold and pronounced dry season and a warm, humid season. Each of the three simultaneous studies were designed to increase the likelihood of detecting seasonal changes by visiting the same forest sites in those two different seasons but focusing on three independent elements of the forest bird community. These are the understorey community as surveyed by mist-netting, as well as feeding behavior of the abundant greenbuls and composition of mixed-species flocks (MSFs) by direct observation. Additionally, systematic general bird observations were collected during extensive walks through the forest in-between MSF observations.
These different sets of forest bird data are investigated through the following null hypotheses: (1) There are no seasonal changes in bird species abundance, (2) there are no changes in diet between seasons, and (3) there are no differences in participation and abundance of forest bird species in mixed-species flocks between seasons. Moreover, we look into different explanations, such as a seasonal change in behavior or movements out of the montane forest.
Study Area
The study was conducted in the Ndundulu Mountains in the Udzungwa Mountains between June 30 and September 9, 1991 (dry season) and between November 5, 1991, and January 9, 1992 (wet season). The study area is situated within what is now Kilombero Nature Reserve at 36°27′ – 36°42′E, 07°39′ – 07°51′S (Dinesen et al., 1993). At the time of fieldwork, the area was classified as West Kilombero Scarp Catchment Forest Reserve (Moyer, 1992, see Figure 1). The study area is the western-most part of the largest forest patch on the Udzungwa Plateau with a continuous forest covering of about 161 km2 (Jensen et al., 2020), including the peak of Luhombero. The entire forest gradient extends from 1,200 to 2,460 m. This part of the Udzungwas is covered by submontane, montane, and upper montane forest, with typical high elevation Hagenia abyssinica dominating large areas above 2,100 m. The study area is described in more detail in Dinesen et al. (1993) and Frontier Tanzania (2001).
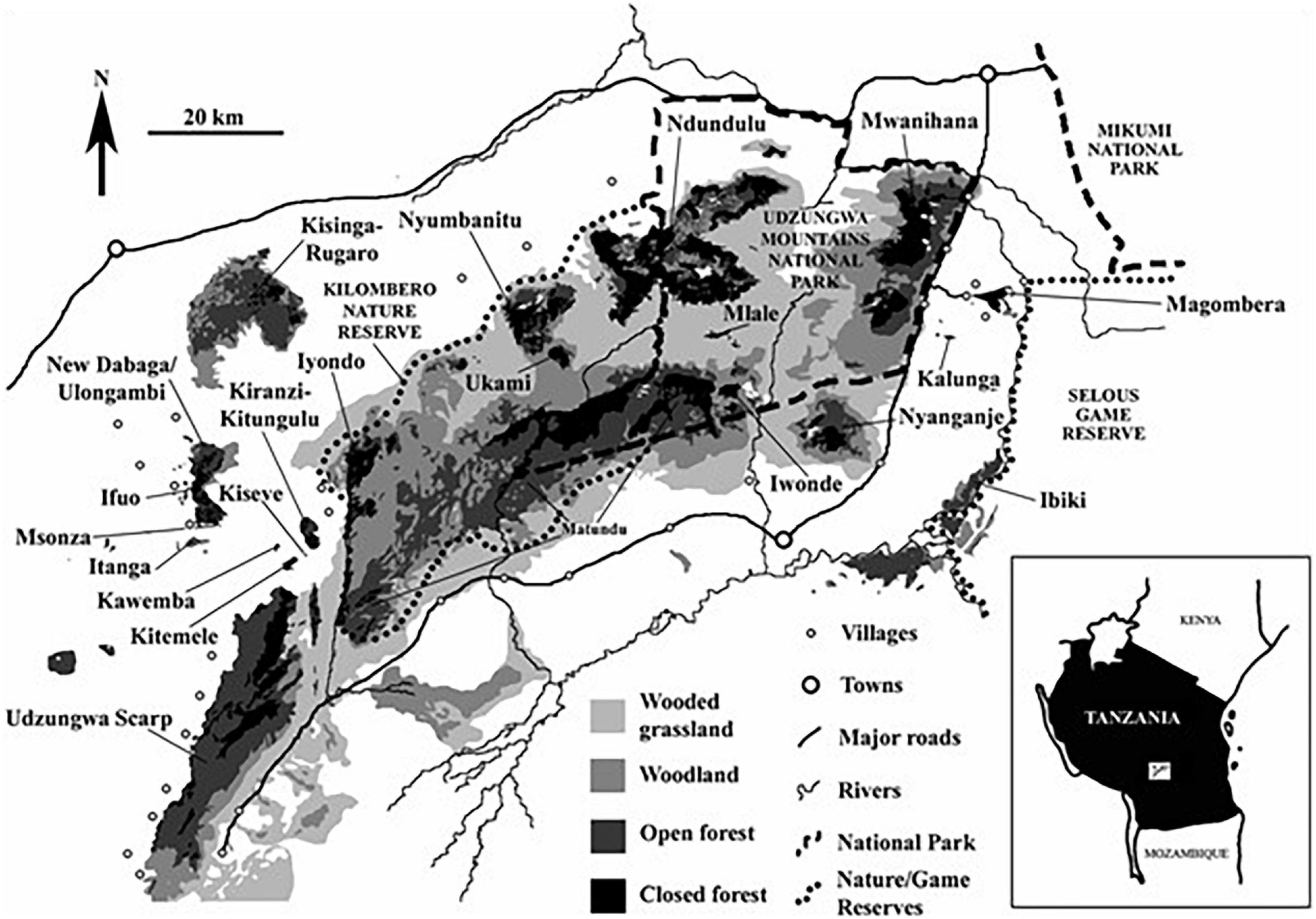
Figure 1. The Udzungwa Mountains in Tanzania. The study was conducted in the Ndundulu Mts. Adopted from Marshall et al. (2009).
Flora
“The submontane forest is pristine, with trees often reaching a height of 30 m, some over 40 m or 50 m. The dominant tree is Parinari excelsa. Other trees (and shrubs) present include Bersama abyssinica, Cassipourea gummiflua, Cola stelecantha, Craterospermum longipedunculatum, Dicranolepis usambarica, Ixora scheffleri, Myrianthus sp., Oxyanthus speciosus, Piper capense, Psychotria sp., Strombosia scheffleri, Strychnos sp., Tarenna pavettoides, Tarenna quadrangularis, Uvariopsis sp., Vitex amaniensis, and Zanthoxylum gilletii” (Ehardt and Butynski, 2006).
Geology and Topography
The chain of ancient gneissic mountains running from the Taita Hills in South-east Kenya to the Udzungwa Mountains in South-central Tanzania has been termed the Eastern Arc Mountains” (Lovett, 1993). They are defined as crystalline mountains under the direct influence of the Indian Ocean climate (Lovett, 1990), and regarded as part of the Tanganyika-Nyasa Mountain Forest Group (Moreau, 1966). As uplifted fault blocks, the mountains appear today as a chain of isolated forest-capped mountains across eastern and south-central Tanzania to the Taita Hills in south-eastern Kenya. Most mountains are habitat islands that rise steeply 1–2 km above the “ocean” of dry savanna plains and harbor a unique flora and fauna, of which many species are endemic to the mountain chain or even to single mountains (Wasser and Lovett, 1993; Burgess et al., 2006).
One of the largest mountain blocks of the Eastern Arc is the Udzungwas, located centrally in Tanzania at the Southwest terminus of the mountain chain. The distance to the Indian Ocean from the Udzungwas varies from about 270 km at Mwanihana (east), and 470 km at Mufindi (west). The length of the Udzungwa Mountains is roughly 240 km from south to north, and the topography varies from foothills and lowlands at c. 300 m to peaks well above 2,000 m.
Climate
Studies of marine sediments from the Indian Ocean reveal that the surface temperature here was only 1–2°C lower during the ice age and did not decline at all off the Tanzanian coast (Prell et al., 1980). This coastal zone may therefore have been permanently warm and humid, and the Eastern Arc Mountains, including the Udzungwas, may therefore have retained patches of humid forest permanently through very long periods (Raven and Axelrod, 1978; Prell et al., 1980). However, more recent studies in the Udzungwas indicate large variations in forest cover within the last 45,000 years and widespread burning (Finch et al., 2014). The high-altitude areas have a climate very different from the foothills as a result of precipitation and temperature changes. Mean annual rainfall in the Udzungwas along the south-eastern scarp is c. 2,000 mm, decreasing to 900 mm on the western plateau (Ehardt et al., 2005). At Mwanihana in the east, with a continuous elevational range of moist forest from 450 to 1,760 m, mean annual rainfall at the foot of the escarpment has been measured at 1,747 mm (at 366 m altitude with 14 years of records) (Lovett, 1996). Rainfall on the escarpment slopes is higher and probably 1,800–2,000 mm per year (Rodgers and Homewood, 1982). However, rainfall and local weather vary considerably between seasons (and years) and the specific localities, and the dry season is often with periods of prolonged drought. The warm and humid wet season generally starts in November, lasting to May, with on average greater than 100 mm of rain each month (Lovett, 1996); but with yearly changes from this general pattern. Temperatures drop rapidly, with altitude with a lapse rate of about 0.6°C per 100 m (Pocs, 1976). Frost has not been reported from the Udzungwas, but in the other Eastern Arc Mountains at high altitudes (e.g., Pocs, 1976).
Materials and Methods
Three of the authors (LH, TL, and LD) collected data used for this paper in both dry and wet seasons during a 4-month period of fieldwork in the Ndundulu Mts. in 1991–1992. Data on the bird community were gathered on a daily basis when the weather permitted it (on occasion rain and fog prevented data collection in the wet season). In general, studies often took advantage of the trails made by African savanna elephants (Loxodonta africana) and Cape buffaloes (Syncerus caffer), which facilitated walking during surveys. Here follows details on field observations, observations of mixed-species flocks, the diet of greenbuls, mist-netting, and weather data. We also assign each of the montane forest birds into specific feeding guilds comprising seven categories: carnivore, granivore-insectivore, frugivore-insectivore, insectivore, insectivore-frugivore, omnivore, and nectarivore-insectivore (see Annex 1). MSFs turned out to be a common and dominant feature in the forest just like in a wide range of other forested habitats worldwide, birds joining flocks benefit by increased foraging rates and ‘safety in numbers’ (see e.g., Sridhar et al., 2009). Moreover, greenbuls are among the most abundant species, and the six species involved in the study were highly abundant at certain altitudes, indicating successful feeding strategies. Furthermore, in order to detect changes in overall species composition, including elusive understory birds, mist-netting and general field observations were undertaken. The data collection methods are described in more detail below. We follow the nomenclature of IOC World Bird List (Gill et al., 2021).
Mixed-Species Flocks
We defined an MSF as consisting of two or more species, foraging less than 10 m apart, staying together for at least 5 min, and all members clearly moving together in the same direction, which is more rigid than, e.g., Goodale and Beauchamp (2010). The number of individuals of each participating species was noted. The passage of a flock often caused a burst of activity from other resident (territorial) birds. These could be singing, calling, and/or moving toward the flock. As aroused birds did not start feeding and moving with the flock, they have been excluded from the MSF material and analysis. In general, a flock was followed for as long as it was necessary to count and identify all individuals, resulting in a longer observation time for the large flocks. All MSFs were recorded within forest habitats. The composition of MSFs was recorded on a Dictaphone and transferred to notebooks on a daily basis. Unidentified birds were noted but not used in the analysis. Time and effort were not standardized for the different elevations. Data on MSF was collected by TL with an effort of 55 and 47 field days in the dry and wet seasons, respectively, between 1340 and 2000 m.
For the analysis of the MSF data, we use a generalized linear model (GLM) with a log link function to test for seasonal differences in (a) the number of flocks observed per day, (b) the number of species per flock, and (c) the number of individuals per flock. The GLM assumes that these three response variables follow a Poisson distribution. We then test for seasonal differences in species-level occupancy (presence–absence in flocks) and abundance (number of observed individuals per flock). The occupancy analyses are conducted using a GLM based on a Bernoulli probability distribution and a logit link function. For the abundance analyses, we use a GLM of the previous Poisson-type. To ensure sufficient data for each analysis, we exclude species observed in less than five flocks. Due to the numerous independent tests, we apply Bonferroni correction on the p-values (i.e., multiplying the p-values by the number of conducted tests; n = 28). Finally, the seasonal turnover in the mixed flocks’ species composition is illustrated using Non-metric Multi-dimensional Scaling (NMDS) from the ‘vegan’ R package (Oksanen et al., 2019).
Feeding Ecology of the Forest Greenbuls
Data on the following species were collected: Yellow-streaked Greenbul Phyllastrephus flavostriatus, Placid Greenbul Phyllastrephus placidus, Shelley’s Greenbul Arizelocichla masukuensis. Stripe-faced Greenbul Arizelocichla striifacies, Little Greenbul Eurillas virens, and Yellow-throated Greenbul Arizelocichla chlorigula. Observations were primarily done in the morning and in the afternoon. All feeding records were written in a notebook with notes on species, date, time, elevation, feeding behavior, diet, height above ground, the canopy height, the number of individuals, and whether the individuals took part in an MSF (see Dinesen, 1995 for details). When a greenbul was sighted, it was followed until the first food item was captured, or an unambiguous substrate search was observed and then used as a feeding record. No further records were obtained of that particular individual. Simultaneously feeding observations of individuals or groups are treated as being independent, even though the feeding behavior of one bird may influence that of another (see Hjejl et al., 1990; Recher and Gebski, 1990; Sherry, 1990). The observations were carried out between 1,340 and 2,000 m in both seasons with an effort of 55 and 40 days, respectively, undertaken by LD. Observation effort was not standardized along the elevational gradient. The classification scheme of foraging behavior proposed by Remsen and Robinson (1990) is followed. However, we only use diet in this analysis.
We use the Chi-squared test to analyze the changes in diet between seasons for the six species of greenbuls. The null hypothesis is that the distribution of food choices remains the same between the seasons. Because there are only two categories in each frequency class, we apply Yates’ correction of continuity (Yates, 1934), which involves subtracting 0.5 from the numerator component of the Chi-square formula:
Here, O and E represent the observed and expected frequency, respectively.
We also investigate shifts in food preferences for each species individually. Due to the low number of registered individuals for some classes generating expected frequencies < 5, we could not use Chi-squared tests. Instead, we applied Fisher’s exact test in R (see R Core Team, 2020), which does not have the same requirements for sample size. In the tests for individual species, we apply Bonferroni correction on the p-values to compensate for the increased type I error rate under multiple testing.
Mist-Netting
Data was collected by LAH on the elevational gradient of 1340 to 2130 m in both seasons. Mist-nets were of the brand North Ronaldsay, mesh-size was for passerine birds. The ringing stations were placed near the camp to reduce the handling time of the bagged birds. Mist-nets were erected for every 200 m along a more or less long “straight” line from the lowest camp to the upper camp, and all faced toward the southeast. Each net or group of nets were placed to cover as many different vegetation types as possible, but areas with no undergrowth were avoided as initial netting had shown that very few birds were caught here. Each net was opened shortly before the first song of the dawn chorus and remained open until sunset. We deviated from this standard only due to rain or thick wet fog. Each net was checked at least once per hour and opening and closing time were noted. If weather conditions permitted, each net was erected for at least two full successive days. A mist-net with, e.g., a length of 12 m operated for 12 h has a total NMH (net meter hours) of 144 NMH. Therefore, the rate at which birds are captured in mist-nets provides a “simple” index for comparison for a “mist-net abundance” of the undergrowth birds at different sites within the forest. As a minimum, we sampled each site in both seasons for 2,500 NMH.
We use a Chi-squared test to analyze the community turnover between the dry and wet seasons. This test is sensitive to rare species in the contingency Supplementary Table S1. As a rule of thumb, less than 20% of the expected frequencies should be <5, and none should be less than 1 (Fowler et al., 1998). To meet these criteria, we excluded 13 species from the analysis, all singletons (n = 10) or doubletons (n = 3): African Goshawk Accipiter tachiro, Black-headed Apalis Apalis melanocephala, Bar-tailed Trogon Apaloderma vittatum, Dapple-throat Arcanator orostruthus, Black-fronted Bushshrike Chlorophoneus nigrifrons, Klaas’s Cuckoo Chrysococcyx klaas, Olive Woodpecker Dendropicos griseocephalus, Collared Sunbird Hedydipna collaris, Scaly-throated Honeyguide Indicator variegatus, African Dusky Flycatcher Muscicapa adusta, Yellow-rumped Tinkerbird Pogoniulus bilineatus, Green Barbet Stactolaema olivacea, and Tambourine Dove Turtur tympanistria. We stepwise removed species and recalculated the Chi-squared test to identify the species contributing most to the seasonal community turnover. The few records of these 13 species can be explained by the fact that a few were low-density species (e.g., Dapple-throat) or occurred in mid-stratum and/or canopy or are species attracted to the forest edge and light gaps, making it less likely for these to be caught by a mist-net.
We apply the Chao 1 estimator (Chao, 1984; Colwell and Coddington, 1994) to estimate the ‘true’ species diversity in each season.
Here, Sobs is the sampled number of species. F1 is the number of species encountered once (i.e., singletons). F2 is the number of species encountered twice (i.e., doubletons).
Field Observation Data
We used 10x40 Zeiss binoculars. We use TL’s general field observation data while looking for mixed-species flocks. He noted all birds seen and heard during walks in the forest between 1,340 and 2,000 m on the entire elevation gradient. All individuals encountered were identified and noted with date, altitude, and time period for each field survey. Data were gathered during both the dry and wet season with an effort of 55 and 47 field days, respectively. We assess that TL’s field observations (including all bird observations in and between MSFs) provide a realistic picture of the composition of the bird community as the observations covered the different forest strata in the range from 1,340 to 2,000 m and were collected consistently.
Weather Data
A rain gauge (Wilh. Lembrech Göttingen with a capacity of 50 mm) was set up next to our campsite. Readings had an accuracy of 0.1–0.5 mm (the shape of the gauge was conical). All measurements of precipitation are of direct rainfall. The Temperature was measured with an electronic min–max thermometer with an accuracy of ±0.5°C, and manual readings were taken at 0600 h, 1200 h, 1500 h, and 1800 h. Only readings within ±10 min of the hour in question have been used. Most measurements were taken at 1,720 m. Temperature is sensitive to altitude, and measurements at other altitudes have been corrected for altitude and adjusted to this elevation, which has been used as standard. All measurements were taken from a fixed position in the shade at roughly 150 cm above the ground and under rainfall cover. Weather data were collected by LAH during both seasons.
Results
Seasonal Change in Local Weather
Figure 2 shows the marked seasonal change in local weather during our study. The temperature increased about 5°C from an average of 14.5°C to 19.2°C (Figure 2A). The minimum temperature recorded was 4°C (August 30 and September 8, 1991) in the dry season, and maximum was 25°C (18 December 1991) in the wet season. There was a marked change in rainfall as well (Figure 2B), as almost zero rainfall was recorded (7 mm) in our study area in the dry season, increasing to about 900 mm in the wet season (Figure 2B). The dry season was pronounced and long, as we have observed in other years of fieldwork in the same area (LD, TL, and LAH).
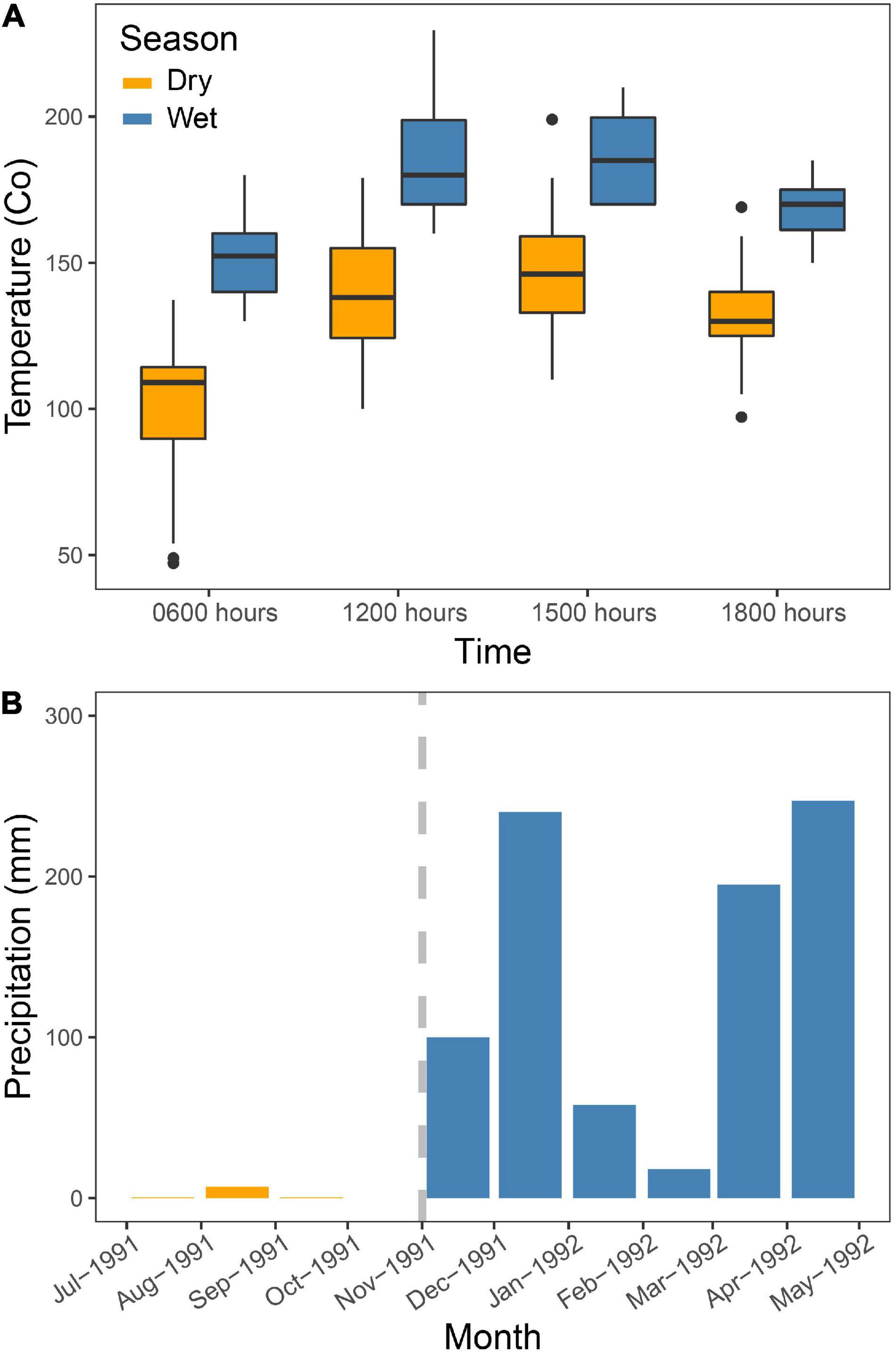
Figure 2. Rainfall and temperature measured at the study site and during the study period from July to August 1991 (dry season) and again from November to January 1992 (wet season) at different camp sites at different altitudes. (A) Temperature readings were made every hour between 0600 h and 1800 h. No measurements were taken during October. (B) Here, we present four values as averages measured at different campsites at different altitudes in the study area during the period from July to September1991 (dry season) and again from November 1991 to January 1992 (wet season).
We recorded a total of 94 montane forest bird species in the study area, excluding some temperate migrants such as, e.g., Blackcap Sylvia borin, and Thrush Nightingale Luscinia luscinia observed at the forest edge. Following Jensen et al. (2020) and including species in MSFs, the bird community comprises 40 (50%) insectivorous species, 17 (21%) mainly frugivore-insectivore (omnivorous) species, nine (11%) insectivore-nectarivore species, five (6.3%) granivorous species as well as six (7.5%) birds of prey (see Annex 1). Based on mist-net data, MSF, and field observation data, we developed species accumulation curves (Figure 3). The cumulative number of species increased drastically during the initial sampling period but eventually flattened (Figures 3A,B). This pattern indicates that our samples are good representations of the actual species communities, providing confidence in our results.
Almost 50 species were recorded in the mist-nets in the wet season (Figure 3A) where the species accumulation curve rise faster than in the dry season. Fewer bird species were caught in the dry season as well as fewer individuals, and the curve is still increasing. In total, 72 species in the dry and 74 in the wet season were recorded in the MSF and field observation data (Figure 3B) with a faster accumulation in the wet season as well. Thus, the graphs indicate that the speed of adding new species was slightly slower during the dry season; however, it should be noted that we were more familiar with the species identification in the wet season as it comprised our second field period. The two species accumulation curves (Figures 3A,B) should not be compared with each other because mist-netting was conducted over a longer gradient at the upper end than the MSF and the field observation data, and therefore mist-netting data includes species that was not encountered at lower elevation. The overall picture is that both species numbers and abundance are higher in the field observation data (including MSFs) because this method includes records of birds from all forest strata.
An additional number of species were recorded inside the forest during TL’s collection of field observation data, none of which were treated in Jensen et al. (2020) and also not further analyzed in this paper. None of these participated in the MSF’s, but all were recorded at altitudes from 1340 m and upward, and may satisfy the criteria set in Jensen et al. (2020) for qualifying as a montane forest species (>1200 m): Crowned Hornbill Lophoceros alboterminatus (common, both seasons, whole gradient), Tambourine Dove (>5 observations), Crested Guineafowl Guttera pucherani (once, dry season only, 1700 m) and Green-backed Honeybird Prodotiscus zambesiae. The following species were also recorded inside the forest, but are considered as forest edge species in our case and/or have other habitat preferences: African Black Duck Anas sparsa, African Green Pigeon Treron calves, Little Sparrowhawk Accipiter minullus, Black Sparrowhawk Accipiter melanoleucus, White-necked Raven Corvus albicollis, White-eyed Slaty Flycatcher Melaenornis fischeri, Ashy Flycatcher Muscicapa caerulescens, Yellow-bellied Waxbill Coccopygia quartinia, Lesser Seedcracker Pyrenestes minor.
Mixed Species Flocks
During the dry season, a total of 111 mixed-species flocks were recorded during 55 field days. Of these, 96 fulfilled the criteria of at least 95% of all individuals identified. This slightly reduced data set was used for analysis, with a total of 50 species identified as participating in the MSFs. During the wet season, 49 MSFs were recorded, with a total of 37 species in 47 field days. One distinct type of MSF (labeled canopy MSF) was generally confined to the higher mid-stratum and canopy and was recorded both during the dry (n = 42) and the wet (n = 40) season. The canopy MSF comprised the following species (n = the number of flocks attended): Yellow-streaked Greenbul (n = 81), Common Square-tailed Drongo Dicrurus ludwigii (n = 78), Dark-backed Weaver Ploceus bicolor (n = 77), Black-headed Apalis (n = 74), Yellow-throated Woodland Warbler Phylloscopus ruficapilla (n = 46), Brown-headed Apalis Apalis alticola (n = 44), Grey Cuckooshrike Ceblepyris caesius (n = 44), Black-fronted Bushshrike (n = 38), Olive Woodpecker (n = 37), Shelley’s Greenbul (n = 37), Southern Yellow White-eye Zosterops anderssoni (n = 29), Green Barbet (n = 28), Stripe-faced Greenbul (n = 24), Moustached Tinkerbird Pogoniulus leucomystax (n = 21), White-winged Apalis Apalis chariessa (n = 20), and Chapin’s Apalis Apalis chapini (n = 18). An additional 34 species were attending 15 or fewer canopy MSFs.
The only other distinct type of MSF was confined to the understorey (labeled understorey MSF), but was only encountered during the dry season (n = 16). The understorey MSF’s were mainly composed of the following species, where n = the number of flocks attended: Placid Greenbul Phyllastrephus placidus (n = 16), Dark Batis Batis crypta (n = 12), Shelley’s Greenbul Arizelocichla masukuensis (n = 9), Yellow-throated Woodland Warbler (n = 9), Bar-throated Apalis Apalis thoracica (n = 7), Red-capped Forest Warbler Artisornis metopias (n = 5), White-tailed Crested Flycatcher Elminia albonotata (n = 5), Fülleborn’s Boubou Laniarius fuelleborni (n = 4), and African Hill Babbler Sylvia abyssinica (n = 4). An additional 12 species were attending three or fewer understorey MSF’s. A total of 38 flocks in the dry season and nine flocks in the wet season could not be assigned to any of the above two distinct types/categories of MSFs and were disregarded in the analysis (see Annex 2, sheet ‘Table 1 and Figure 4,’ where the 38 and 9 flocks are marked with ‘3’).
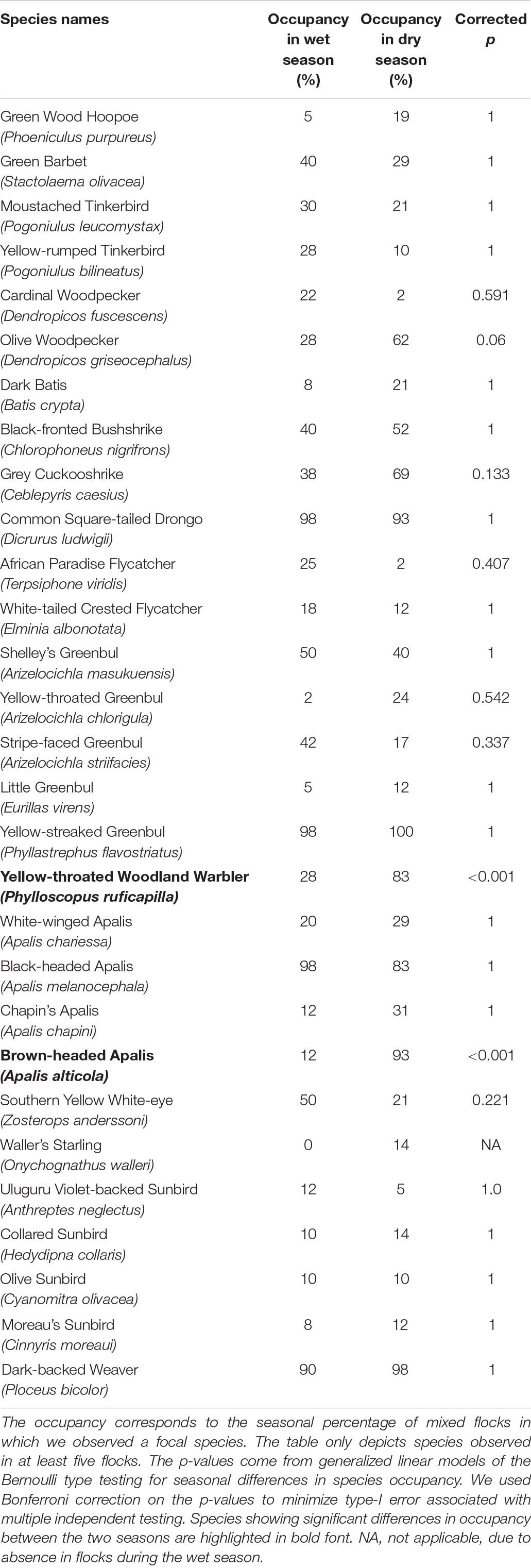
Table 1. Seasonal differences in occupancy for species participating in the 82 canopy mixed-species flock type.
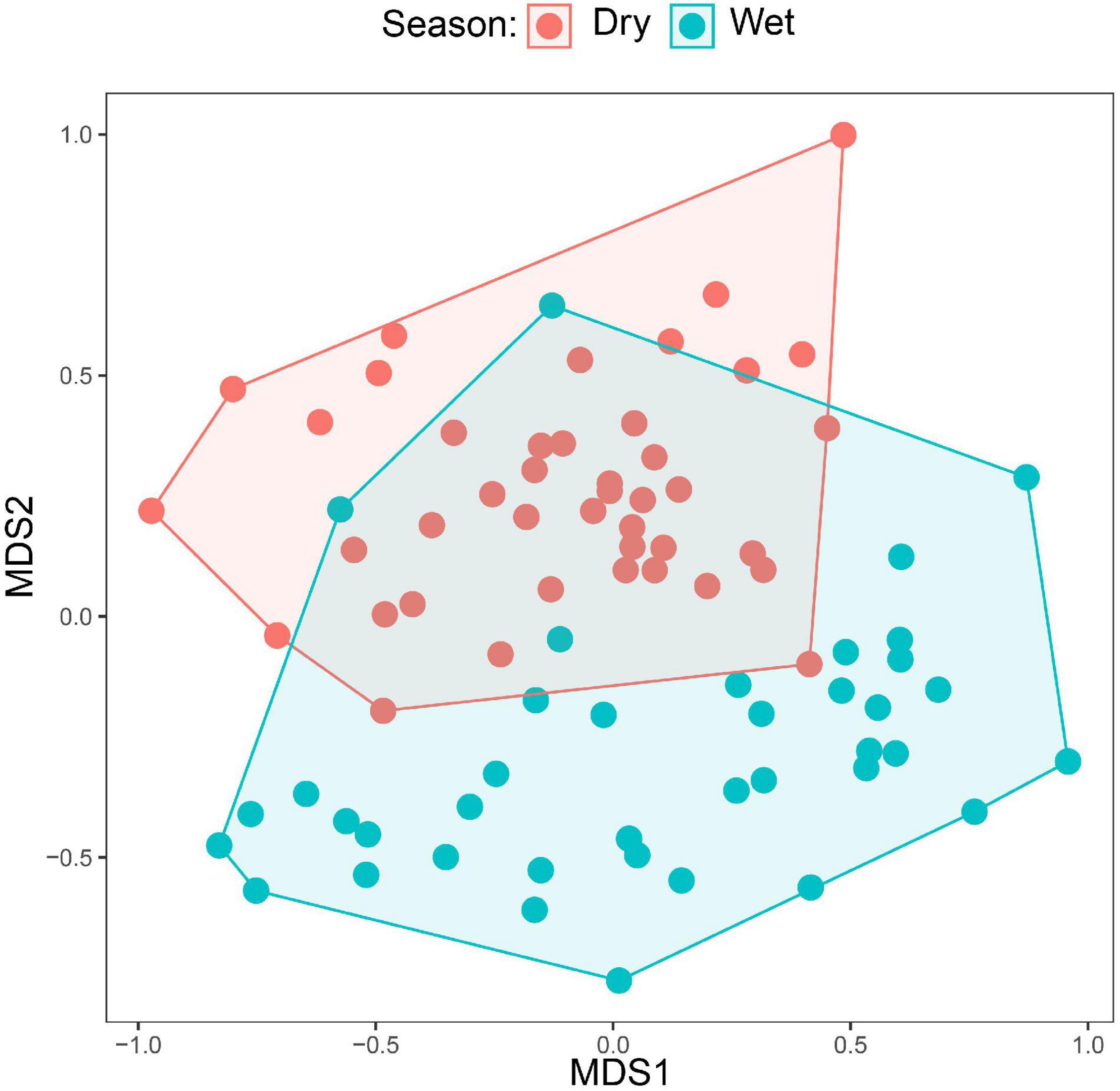
Figure 4. NMDS plots comparing the species compositions of mixed flocks between the wet and dry season. Each dot corresponds to a mixed-species flock sampled in either the wet or dry season. Dots closer to each other have more similar species composition (measured by Bray–Curtis dissimilarity). The plot includes only canopy flocks as understory flocks were absent in the wet season.
The average number of MSFs observed per day in the dry season was 2.02 (±0.13 SE) and 1.04 (±0.18 SE) during the wet season. There were two field days in the dry season with zero flocks and 15 days with zero flocks in the wet season. Using a Poisson GLM with a log link function, we found significantly more flocks per day during the dry season than in the wet season. β = 0.66, SE = 0.17, p-value < 0.001.
The average number of species in the canopy MSFs was 9.7 (±0.78 SE) in the wet season, and 11.3 (±0.52 SE) in the dry season (standardized coefficient (β) = 0.15, Standard error (SE) = 0.07, p-value = 0.025). Hence, there were significantly more species per flock in the dry season. There were also significantly more individuals per flock during the dry season, with an average number of individuals of 20.8 (1.85 SE) in the wet season, compared to 32.3 (±1.76 SE) in the dry season (β = 0.43, SE = 0.02, p-value < 0.001).
A total of 50 species were recorded in the canopy MSFs; however, only 36 species were observed in more than five flocks. We used five occurrences to fulfill the statistical analysis criteria. Among these 36 species, only two species showed significant differences in occupancy between the two seasons. Brown-headed Apalis and Yellow-throated Woodland Warbler occupied significantly more flocks during the dry season than during the wet season (see Table 1). In the analysis testing for seasonal differences in the species’ abundance, only Yellow-streaked Greenbul turned out as significantly more abundant in the dry season (β = 0.73, SE = 0.11, corrected p-value < 0.001). Lastly, we visually compared the canopy MSFs between seasons using an NMDS plot (Figure 4), which incorporates both the turnover in species composition and abundances. Here, it becomes apparent that only three flocks are compositionally similar between the wet and the dry season and hence a clear difference in composition between the dry and the wet season canopy MSFs.
Feeding Ecology of Greenbuls
Six species of forest greenbuls were present within the study area. All-together 757 feeding observations (320 from the wet and 430 from the dry seasons) were recorded: Shelley’s Greenbul (n = 192), Little Greenbul (n = 82), Yellow-streaked Greenbul (n = 178), Placid Greenbul (n = 138), Striped-faced Greenbul (n = 77) and Yellow-throated Greenbul (n = 90).
We found notable differences in food selection of two of the six species of greenbuls between the dry and wet seasons. Two species fed more on fruit during the dry season. Shelley’s Greenbul shifted from an omnivorous diet in the dry season to a predominant insectivorous diet in the wet season (Fishers’ exact p < 0.001, Table 2), and Striped-faced Greenbul shifted from a predominantly frugivorous diet in the wet season to an omnivorous diet in the dry season (Fishers’ exact p < 0.001, Table 2). Yellow-throated Greenbul was mainly frugivorous, but ate proportionally more fruits in the dry season. However, this was not statistically significant. In both seasons, Little Greenbul had a mixed insectivorous-frugivorous diet consisting of the same proportion of fruits and insects (Fishers’ exact p = 1). The two Phyllastrephus species, Yellow-streaked Greenbul and Placid Greenbul, were both highly insectivorous; only twice was the latter observed picking fruits (dry season), while Yellow-streaked was entirely insectivorous in both seasons, and an extremely active and numerous species in the study of canopy MSF’s (Table 2). In contrast, the Placid Greenbul was an active and numerous member of the understory MSFs in the dry season. Thus, Fishers’ exact test was not applicable for Yellow-streaked Greenbul and was highly non-significant for Placid Greenbul (Fishers’ exact p = 1).
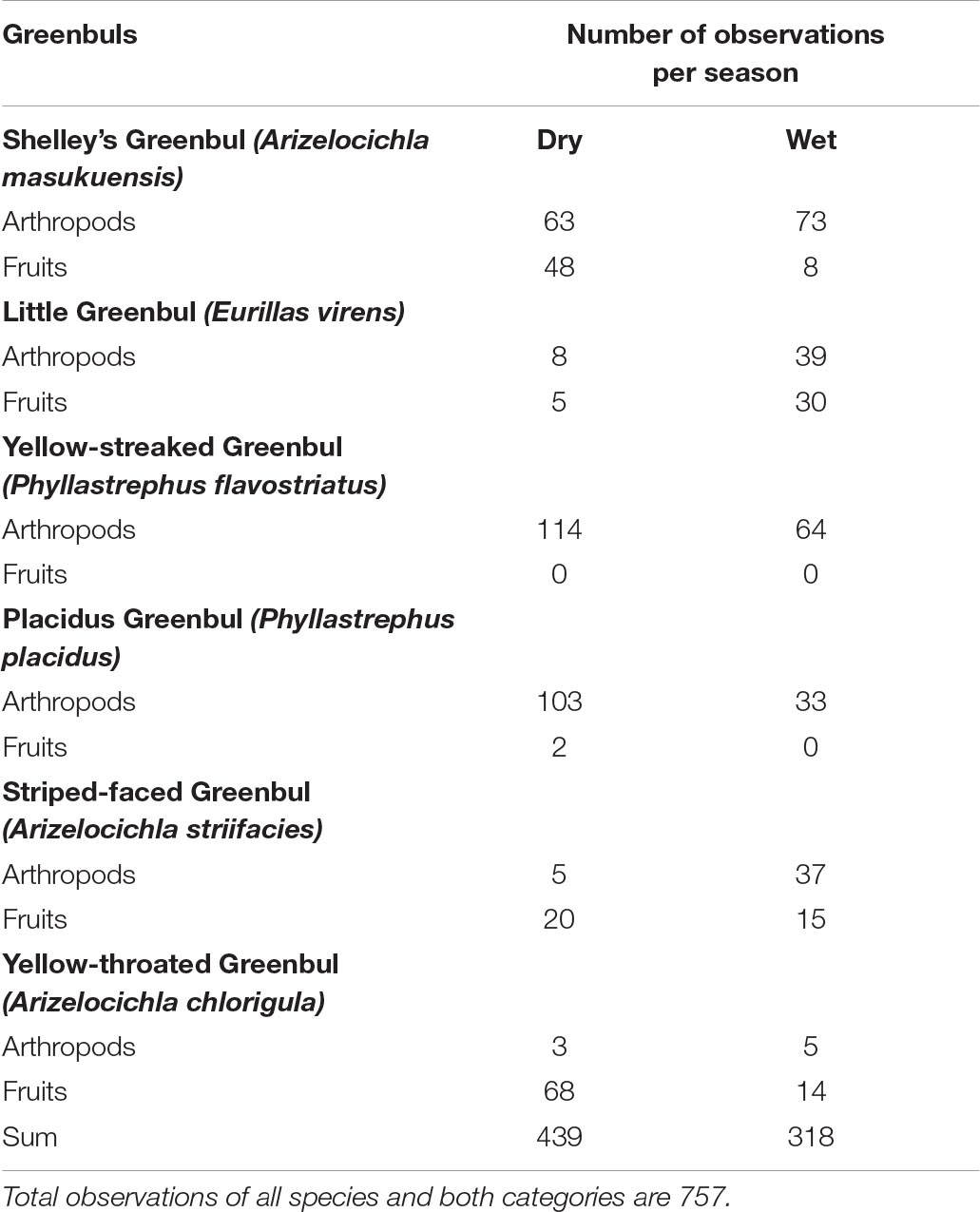
Table 2. Diet of six species of greenbuls in the dry and wet season in the Ndundulu study area including.
Mist-Netting
A total of 40,391 NMH was completed in the dry season versus 23,033 NHM in the wet season. Mist-netting was conducted at all mist-netting sites in both seasons (see the section “Materials and Methods”). The total number of individuals mist-netted was 1630, represented by 52 species: In the wet season 37 species of 675 individuals, and in the dry season: 48 species of 956 individuals (for accumulation curves, see Figure 3).
Four species were only seen during the dry season, while 15 other species were only observed during the wet season. The accumulation curve of species caught (Figure 3) indicates that mist-netting effectively catches the understorey species, apart from, e.g., gallinaceous birds. Certain species from higher strata were caught in small numbers, especially in the wet season, e.g., Striped-faced Greenbul, Yellow-throated Greenbul, Bar-throated Apalis, Oriole Finch, Yellow-streaked Greenbul, and White-tailed Crested Flycatcher.
We found a significant difference in species abundances between the two seasons: Chi-squared = 268.88, df = 38, p-value < 0.001. The statistical significance of the Chi-squared test was driven by 16 species having the largest change in relative abundance between the seasons. The overall result remains significant when any of these 16 species are included. If removing all 16 species, the overall Chi-squared test on the remaining 23 species is not significant (Table 3).
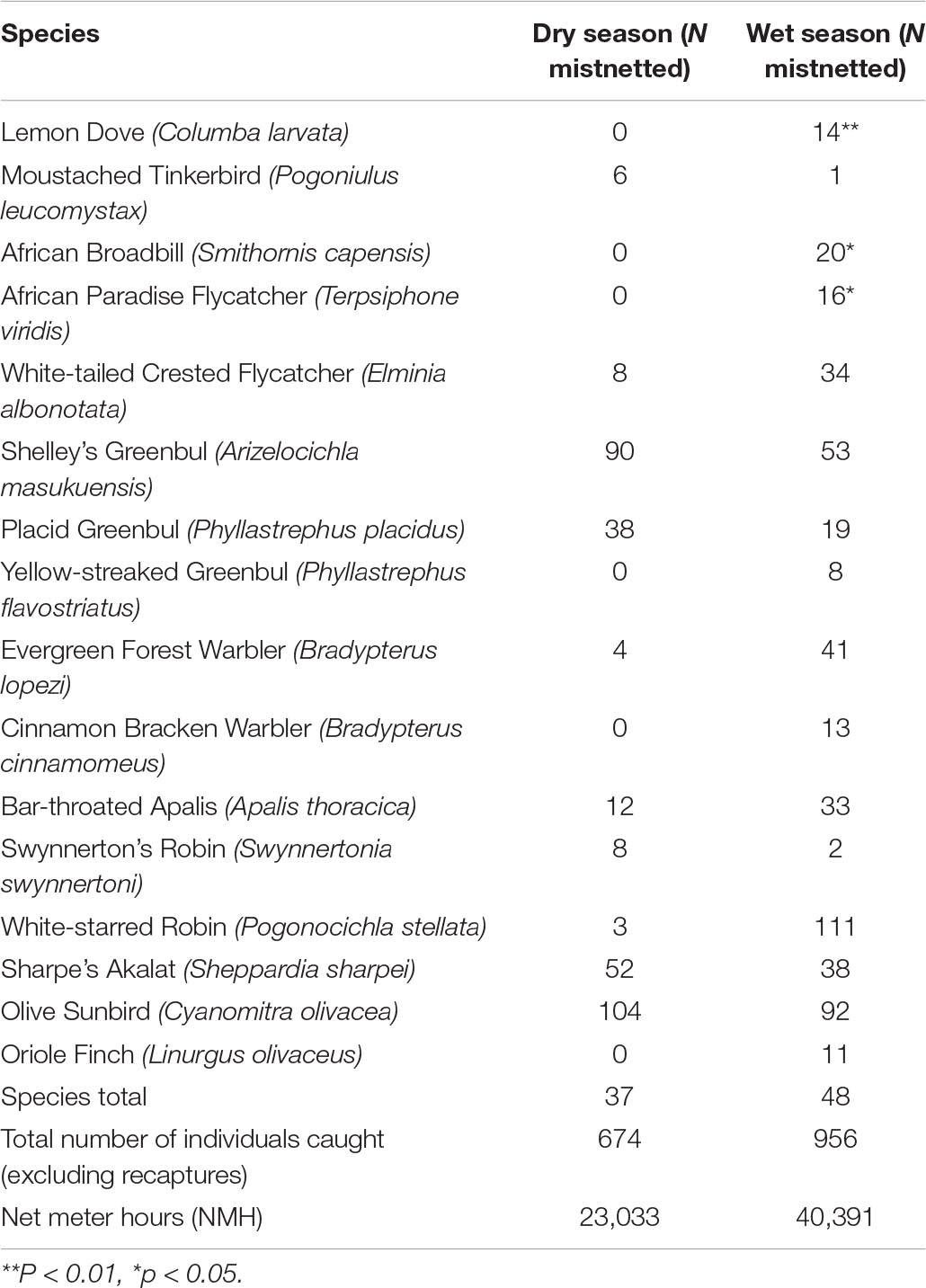
Table 3. List of the 16 species caught in the mist-nets, showing the community turnover between the dry and wet season.
The number of singletons in the mist-nets was ten and doubletons three. Rarely captured species include: African Goshawk (Accipiter tachiro), Black-headed Apalis (Apalis melanocephala), Bar-tailed Trogon, Dapple-throat, Black-fronted Bushshrike, Klaas’s Cuckoo, Olive Woodpecker, Collared Sunbird, Scaly-throated Honeyguide, African Dusky Flycatcher, Moustached Tinkerbird, Green Barbet, and Tambourine Dove.
Field Observation Data
The field observation data used for analysis (see Table 4) comprised a total of 70 species, with 65 species recorded in the wet season and 67 in the dry season. The results presented in Table 4 show that Eastern Bronze-naped Pigeon Columba delegorguei, Barred Long-tailed Cuckoo Cercococcyx montanus, Bar-tailed Trogon, Green Barbet, African Broadbill Smithornis capensis, African Paradise Flycatcher Terpsiphone viridis, Stripe-faced Greenbul, Black-headed Apalis, Red-capped Forest Warbler, Orange Ground Thrush Geokichla gurneyi, and White-starred Robin Pogonocichla stellata were all more common during the wet than during the dry season. Of these, four species were almost absent from the montane forest during the dry season, namely Barred Long-tailed Cuckoo, African Broadbill, African Paradise Flycatcher, and White-starred Robin, while African Dusky Flycatcher was completely absent during the dry season.
Discussion
Our data show that the montane forest bird community in the Ndundulu Mountains in the Udzungwas undergoes marked changes between the dry and wet seasons. This general result is clear in all the three independent studies: Some species move out of the montane forest in the dry season. Other species showed partial movements as they remained but with reduced numbers in the dry season and others again change diet or change the social behavior by participating or participating significantly more in mixed-species flocks. The seasonal turnover in community composition is particularly noticeable in the mixed-species flocks. As illustrated in the NMDS plot (Figure 4), only three canopy MSFs from the wet season were compositionally similar to flocks from the dry season. These were recorded between 15 and 30 November in the “short rains.” In any case, our results support previous findings that the majority of the populations, including the vast majority of the Eastern Arc endemics – of which many are insectivorous species – do not undertake seasonal movements to lower altitudes (see Table 4, Annex 1, Burgess and Mlingwa, 2000). It has previously been shown that Udzungwa Mountains are home to a distinct montane bird community, with another community found at lower elevations (Romdal and Rahbek, 2009).
We are quite confident that key environmental drivers are responsible for the observed changes in species composition and behavior between seasons. The long dry season in the mountains lasts several months with minimal precipitation compared to a warm and humid wet season on average 5°C warmer in our study period 100-fold more rain and with most forest bird species breeding (Brown and Britton, 1980). This results in food scarcity in the dry season, especially for insectivorous species (see e.g., Brown and Britton, 1980; Williams and Middleton, 2008; see also Malizia, 2001). For instance, a study by Mulwa et al. (2012) found species richness and the total abundance of frugivores and insectivores concurred with the seasonal availability of their respective resources. In comparison, our data indicate that frugivorous-insectivore species are perhaps less influenced by seasonal variation, presumably because fruiting is more variable in time and space in the forest (see e.g., Adamescu et al., 2018), e.g., in different microhabitats such as light gaps, forest edge and between tree species. Thus trees fruiting varies not only between species and season but also within the same species and even the same tree from year to year (Wheelwright, 1986).
Comparable to frugivore-insectivorous, nectarivorous sunbirds may be similarly impacted by the variability of flowering (and thus nectar) and occurrence of invertebrates. They feed on both nectar from flowering plants/epiphytes and on insects and the more common species appear abundant in both seasons. There were indications that several of the rare sunbird species move more around in the non-breeding season and were recorded in the study area in the dry season only. However, the most common Olive Sunbird is known from other localities to undertake altitudinal movements (Cordeiro, 1994a), however, we cannot find it in our data for the Ndundulu Mountains.
We did not collect data on food abundance, either flowering nor, fruits, nor arthropods, and we have anecdotal information from the study area only: During the wet season, a rain of larvae droppings from above to the tarpaulin at camp could be heard. During the daytime, there were bursts of cicada sounds, butterflies were numerous and a different insect life compared to the dry season. Fruits and flowering seemed abundant in the dry season. These seasonal findings on food abundance seem to be supported by a study in forests in northern Kenya (Borghesio and Laiolo, 2004) and a study on tropical savannah birds in West Africa showed a positive response in breeding and body mass to resource availability brought about by seasonal rainfall (Cox et al., 2011), which concur with our findings in the Udzungwas (unpublished data).
Change in Behavior Between Seasons
Our results show that many, mainly insectivorous species, attend MSFs in the dry season. The distinct understorey MSF with the participation of birds from the forest understorey comprising up to 10 species and up to 26 individuals were active in the dry season only. Therefore, there seems to be a clear advantage of participating in MSFs during the dry season, e.g., related to the scarcity of food in that season. Likewise, the distinct canopy MSF comprised mainly canopy and higher mid-stratum species, and had more species and more individuals of each species in the dry season (e.g., 50 species in dry season versus 37 in the wet season). Furthermore, the mobility of MSFs was considerably higher in the dry season, and flocks moved faster through the forest compared to the wet season where the MSFs were virtually sedentary (TL unpublished data).
Some of the most abundant species in the bird community were the six greenbuls (see also Keith et al., 1992; Britton, 1980), which responded to the presumed change in food availability by a flexible behavior (see also Dinesen, 1997). Two of the six species studied changed diet and feeding behavior, see also Dinesen (1995) toward significantly more fruits in the dry season and there are indications that Yellow-throated Greenbul do the same as it almost entirely ate fruits in the dry season and occasionally fed on nectar and possibly leaves in this season compared to more arthropods in the wet season. An extremely rapid feeding behavior of Little Greenbul of sallying out swiftly hovering and catching airborne insects in the wet season seemed not to be practiced to the same extent in the dry season; however, more data are needed to support this. This seasonal shift in diet is in contrast to a study in a much drier forest environment in Kenya (Borghesio and Laiolo, 2004), where most species did not change their behavior between seasons. However, Borghesio and Laiolo (2004) nonetheless concluded that omnivores shifted to frugivory in the dry season and fed more on invertebrates in the wet season, as in our study.
The two insectivorous greenbul species Yellow-streaked Greenbul and Placid Greenbul were very characteristic and dominant members of each of the two distinct types of MSF’s. Placid Greenbul ate fruits on a few occasions in the dry season, perhaps indicating the limitations of available arthropods in this season. The greenbul study, as well as the field and MSF data, support that these two insectivorous and gregarious greenbuls are more or less resident and abundant in both seasons (see also Dinesen, 1997).
We speculate that the scarcity of arthropods in the dry season challenge insect-eating species and force them to either change diet, change social behavior, or move away. The vast majority of the MSFs comprised insectivorous species. These species may have a clear advantage of joining MSFs where many individuals moving through the forest increase the chance of flushing insects (Sridhar et al., 2009). The function of avian mixed-species flocks in Madagascar has been reported to change function between seasons from a function of increased foraging efficiency in the non-breeding period to a function of anti-predator defense in the breeding period (Hino, 2009). Moreover, some species might not have an advantage of joining MSFs during the breeding season, as arthropods are more abundant. A much less pronounced seasonal difference (dry versus wet season) is seen in Brazil (Maldonado-Coelho and Marini, 2000), which is probably partly due to the lower altitude of the forests, hence also a shorter altitudinal gradient compared to the Ndundulu Mountains.
There are indications from the mist-net results that some insectivorous understory species regarded as residents changed behavior between the seasons, for example, Placid Greenbul, Swynnerton’s Robin Swynnertonia swynnertoni, and Sharpe’s Akalat Sheppardia sharpie. These ground dwellers are expected to move more around in the dry season with no indications of moving out of the study area and were caught most frequently in the mist-nets in the dry season. Other insectivorous mid-stratum species were caught more frequently in the wet season, such as White-tailed Crested Flycatcher and Bar-throated Apalis. Both species we regard as residents in the study area, and we believe the reason is that these two mid-stratum species are generally feeding lower in the wet season, where many species place their nests close to the ground.
Lemon Dove Columba larvata forages on the ground on fruits. It shows a pronounced change in mist-net captures, with two in the dry versus 40 in the wet season. Fruits of some tree species are more abundant in the wet season (especially toward the last part of the season). Studies in coastal forests in South Africa have shown that the species is much more habitat-specific in the breeding season but abundance was overall the same (David et al., 2017; Smith et al., 2017). This may apply to our study area as well; however, there are also indications of seasonal movements in the Udzungwas (Stuart et al., 1987). The insectivorous Yellow-throated Woodland Warbler also changes social behavior with a significantly higher occupancy in the MSFs during the dry season. The species is abundant from the mid-stratum to the canopy, and there are hence no significant trends in the mist-netting data and neither in its general abundance in field observation data.
Seasonal Movements
It is interesting that Moreau (1935) stated in his paper on the Usamabara that “whether Forest or non-forest birds are considered, there appears to be practically no seasonal movement up and down the mountains.” New evidence on movements have accumulated since (e.g., Burgess and Mlingwa, 2000 and many other references). Movement out of the montane forest is unambiguously shown by our mist-net and MSF data as well as field observations for five insectivorous species (6.3%) especially confined to the mid-stratum. These species appeared to leave the montane forest almost entirely, and they include: Barred long-tailed Cuckoo, African Broadbill, African Paradise Flycatcher, African Dusky Flycatcher, and White-starred Robin. White-starred Forest Robin is known to undertake downward movements on Kilimanjaro (Cordeiro, 1994b) and this species and African Dusky Flycatcher and are also known from South African forests and elsewhere to move to lower altitudes and African Paradise Flycatcher to be a breeding migrant (Craig and Hulley, 2019).
While our studies suggest that species leave the montane forest in the dry season, there are insufficient data/studies documenting where the birds move to. However, studies in the Uluguru Mountains have revealed an increase in the abundance of certain montane forest species in the foothills in the cold dry season (Werema et al., 2016) and in the foothills of the Udzungwa Mountains (Stuart et al., 1987). Observations in the lowlands during the dry season in Kimboza Forest Reserve and foothills of the Ukingu Mts (a.k.a. Nguru Mts) (LAH fieldwork, 2021) support the presence of many of the above-mentioned “montane” species. Burgess and Mlingwa (2000) were not able to tell whether occurrence in, e.g., coastal forests of possible seasonal migrants was based on a local resident population or the altitudinal migration from the montane areas of these species. Our perception of montane-lowland species is constantly challenged, e.g., with the observation of Swynnerton’s Robin in the lowlands of East Usambara (Anderson et al., 1997) and findings of two montane species on Zanzibar island at ca. 35 m (LAH Fieldwork, 2021).
Our results also indicate that part of the population of certain other species may undertake seasonal movements between montane forest habitats and lowland forests. These species are for example: Bar-tailed Trogon, Stripe-faced Greenbul, Little Greenbul, Evergreen Forest Warbler Bradypterus lopezi, Cinnamon Bracken Warbler Bradypterus cinnamomeus, Black-headed Apalis, Red-capped Forest Warbler, and Orange Ground Thrush (see also Stuart et al., 1987).
The ground dwellers and insect-eating Cinnamon Bracken Warbler and Evergreen Forest Warbler were caught most frequently in the wet season, indicating a movement out of the area during the dry season. We find support for this from Lemaire (1989) who reported a regular downward shift of Cinnamon Bracken Warbler and Evergreen Forest Warbler in Malawi and the latter also by Cordeiro (1994a) for Tanzania.
The Brown-headed Apalis, a canopy species in the MSFs in the study area, was attending the MSFs significantly more during the dry season (see Table 2). In combination, our data indicate that there are considerably fewer individuals present in the forest during the wet season. Therefore, it seems that the majority of Brown-headed Apalis leave the forest presumably to breed somewhere else. No source we have been able to find seems to mention this species as anything but a “resident species” (e.g., Urban et al., 1997; Dowsett-Lemaire and Dowsett, 2006) (the latter reference as A. cinerea).
Other species may undertake movements within the montane forest and move down in altitude in the dry season. The field data does not indicate movements out of the study area of Yellow-throated Greenbul in the dry season. However, unpublished data by LAH show a seasonal change in relative abundance at certain altitudes within the altitudinal span of the study area. Within the complex of the Mountain Greenbul Lemaire (1989) and Dowsett-Lemaire and Dowsett (2006) did report “Eastern Mountain Greenbul Andropadus nigriceps” (now known as Black-browed Greenbul Arizelocichla fusciceps) to undertake a regular downward shift in Malawi, and Stuart et al. (1993) reported it to undertake altitudinal movements in the Ulugurus Mountains and small vertical seasonal migrations in the Usambaras.
A Conservation and Evolutionary Perspective
The continuous fragmentation of forests in East Africa, including the Udzungwa Mountains may, in connection with accelerating climate change have severe consequences for these tropical bird communities (see also Root et al., 2003; Williams and Middleton, 2008; Willig and Presley, 2016; Santillan et al., 2018). The only hypothetical escape for some species may be to move to higher elevations. There are few data to suggest that they do. Yet, the greatest loss of species from climate disruption may be for tropical montane species (Araújo and Rahbek, 2006; Peh, 2007; Forero-Medina et al., 2011). The seasonal dynamics of these bird communities demonstrate the need to take the full habitat requirements of all species into account in future conservation planning.
This study further sheds light on some of the larger patterns in relation to seasonal changes in East African montane forests exemplified by the montane bird community. The harshness of the long cold dry season can be seen as an evolutionary bottleneck for many species (see Williams and Middleton, 2008 for an Australian case). A marked seasonal variation in climate seems likely to favor species that can adapt to the different food supplies over the year. Other studies show that insectivorous species in MSFs are the most vulnerable to habitat fragmentation and/or destruction (Cordeiro et al., 2015). In a study in Kenya, Mulwa et al. (2012) concluded that bird communities strongly respond to seasonal fluctuations in resource availability, but responses differ between feeding guilds. While frugivorous birds seem to respond flexibly to seasonal fluctuations, for instance, by having the ability to track down fruit resources across habitat boundaries, insectivorous birds appear to be more susceptible to the increase in seasonal fluctuations in resource availability.
Several of the more common species are evolutionary generalists, capable of responding to different seasonal climates and feeding opportunities by a flexible behavior and ability to change diet. This behavior is exemplified by the greenbuls in this study (all abundant at certain altitudes), as some of these do both change their diet and feeding behavior and undertake partial movements. Another strategy is to “stay” in the montane forest and participate in MSFs, especially in the cold dry season, where we document a higher number of species and individuals to optimize feeding opportunities (see also Brown, 2014). In contrast, high precipitation in the high Andes seems to impose important constraints on birds (Santillan et al., 2018). Diet specialists may be more vulnerable as they cannot shift to another food source and therefore suffer to a greater deal when major habitat and climate changes occur (see also Dulle et al., 2016). There is, however, little doubt that the dry season in East Africa is challenging for many montane forest organisms, including insect-eating birds and their prey. A number of different evolutionary strategies have come into force to adapt to and survive this season, as demonstrated in this paper, and it may be a bottleneck reinforced in future by the increasing effects of climate change.
Data Availability Statement
The raw data supporting the conclusions of this article is available as Supplementary Material.
Author Contributions
LD, TL, and LH contributed to the conception and design of the study. JS and TR performed the statistical analysis. LD and LH wrote the first draft of the manuscript. TL wrote sections of the manuscript. All authors contributed to manuscript revision, read, and approved the submitted version.
Funding
Aage V. Jensens Foundation funded the fieldwork of LD, LH, and TL in 1991–1995. JS was supported by the research Grant No. 25925 from VILLUM FONDEN.
Conflict of Interest
The authors declare that the research was conducted in the absence of any commercial or financial relationships that could be construed as a potential conflict of interest.
Publisher’s Note
All claims expressed in this article are solely those of the authors and do not necessarily represent those of their affiliated organizations, or those of the publisher, the editors and the reviewers. Any product that may be evaluated in this article, or claim that may be made by its manufacturer, is not guaranteed or endorsed by the publisher.
Acknowledgments
We are indebted to the Aage V. Jensen’s Foundation for covering our expenses and the Tanzanian Commission for Science and Technology (COSTECH) for permission to conduct research. We want to thank the late Jens Otto Svendsen, for sharing time with us in the field, Jon Fjeldså for his enthusiasm and for help in many ways, Norbert Cordeiro for numerous comments improving the manuscript, and Elin Løyche for the preliminary typing of the ringing data into a database. In Tanzania, the late Elizabeth (Liz) and Neil Baker introduced us to hands-on ringing and forest birding and hosted us on several occasions. The late Alan Rodgers who encouraged us to pick Ndundulu as our study area. In the field, we appreciated the company of Cuthbert L. Nahonyo and the late Robert Banda, University of Dar es Salaam, as well as our field assistants the late Jacob Kiure, and Elia Mulungu, and our skilled guides and friends: Janus Madanga, Malidadi, and the late Gwido Mhando. Finally, the whole of Udekwa Village for their support.
Supplementary Material
The Supplementary Material for this article can be found online at: https://www.frontiersin.org/articles/10.3389/fevo.2022.768020/full#supplementary-material
References
Adamescu, G. S., Plumptre, A. J., Abernethy, K. A., Polansky, L., Bush, E. R., Chapman, C. A., et al. (2018). Annual cycles are the most common reproductive strategy in African tropical tree communities. Biotropica 50, 418–430. doi: 10.1111/btp.12561
Anderson, G. Q. A., Evans, T. D., and Watson, L. G. (1997). The Tanzanian race of Swynnerton’s Robin Swynnertonia swynnertoni rodgersi. Bull. Afr. Bird Club 7, 83–89. doi: 10.5962/p.308933
Araújo, M. B., and Rahbek, C. (2006). How Does Climate Change Affect Biodiversity? Science 313, 1396–1397. doi: 10.1126/science.1131758
Boko, M. I., Niang, A., Nyong, C., Vogel, A., Githeko, Medany, M., et al. (2007). “Africa. Climate Change 2007: Impacts, Adaptation and Vulnerability,” in Contribution of Working Group II to the Fourth Assessment Report of the Intergovernmental Panel on Climate Change, eds M. L. Parry, O. F. Canziani, J. P. Palutikof, P. J. van der Linden, and C. E. Hanson (Cambridge, MA: Cambridge University Press).
Borghesio, N. L., and Laiolo, P. (2004). Seasonal foraging ecology on a forest avifauna of northern Kenya. J. Trop. Ecol. 20, 145–155. doi: 10.1017/s0266467403001159
P. L. Britton (ed.) (1980). Birds of East Africa - their habitat, status and distribution. Nairobi: East Africa Natural History Society.
Brown, J. H. (2014). Why are there so many species in the tropics? J. Biogeogr. 41, 8–22. doi: 10.1111/jbi.12228
Brown, L. H., and Britton, P. L. (1980). The breeding seasons of East African birds. Nairobi: The East African Natural History society.
Burgess, N. D., Butynski, T. M., Cordeiro, N. J., Doggart, N. H., Fjeldså, J., Howell, K. M., et al. (2006). The biological importance of the Eastern arc Mountains of Tanzania and Kenya. Biolog. Conserv. 134, 209–231. doi: 10.1016/j.biocon.2006.08.015
Burgess, N. D., and Mlingwa, C. O. F. (2000). Evidence for altitudinal migration of forest birds between montane Eastern Arc and lowland forests in East Africa. Ostrich 71, 184–190. doi: 10.1080/00306525.2000.9639908
Carscadden, K. A., Emery, N. C., Arnillas, C. A., Cadotte, M. W., Afkhami, M. E., Gravel, D., et al. (2020). Niche Breadth: Causes and Consequences for Ecology, Evolution, and Conservation. Q. Rev. Biol. 95, 179–214. doi: 10.1086/710388
Chao, A. (1984). Non-parametric estimation of the number of classes in a population. Scand. J. Stat. 11, 265–270.
Colwell, R. K., and Coddington, J. A. (1994). Estimating terrestrial biodiversity through extrapolation. Philosoph. Trans. R. Soc. B 345, 101–118. doi: 10.1098/rstb.1994.0091
Cordeiro, N. J. (1994a). Final report to the Royal Society for the Protection of Birds: an investigation of the forest avifauna in the North Pare mountains and Kahe II Forest Reserve, Tanzania. Unpublished.
Cordeiro, N. J., Borghesio, L., Joho, M. P., Monoski, T. J., Mkongewa, V. J., and Dampf, C. J. (2015). Forest fragmentation in an African biodiversity hotspot impacts mixed-species bird flocks. Biol. Conserv. 188, 61–71. doi: 10.1016/j.biocon.2014.09.050
Cox, D. T., Brandt, M. J., McGregor, R., Ottosson, U., Stevens, M. C., and Cresswell, W. (2011). Patterns of seasonal and yearly mass variation in West African tropical savannah birds. Ibis 153, 672–683. doi: 10.1111/j.1474-919x.2011.01150.x
Craig, A. J. F. K., and Hulley, P. E. (2019). Montane forest birds in winter: do they regularly move to lower altitudes? Observations from the Eastern Cape, South Africa. Ostrich 90, 89–94. doi: 10.2989/00306525.2019.1568316
David, A. E. S., Yvette, C. E. S., and Colleen, T. D. (2017). Seasonal habitat requirements of Lemon Dove (Aplopelia larvata) in coastal forest: camera-trap surveys of a reclusive species. Afr. Zool. 52, 199–207.
Dinesen, L. (1995). Seasonal variation in feeding ecology of Shelley’s greenbul in subtropical evergreen forests. Afr. J. Ecol. 33, 420–425. doi: 10.1111/j.1365-2028.1995.tb01051.x
Dinesen, L., Lehmberg, T., Rahner, M. C., and Fjeldså, J. (2001). Conservation priorities for the forests of the Udzungwa Mountains, Tanzania based on primates, duikers and birds. Biol. Conserv. 99, 223–236. doi: 10.1016/s0006-3207(00)00218-4
Dinesen, L., Lehmberg, T., Svendsen, J. O., and Hansen, L. (1993). Range extensions and other notes on some restricted-range forest birds from West Kilombero, in the Udzungwa Mountains, Tanzania. Scopus 47, 48–59.
Lemaire, F. (1989). Ecological and biogeographical aspects of forest communities in Malawi. Scopus 13, 1–80.
Dowsett-Lemaire, F., and Dowsett, R. J. (2006). The birds of Malawi. Belgium: Turaco Press and Aves a.s.b.l, Liége.
Dulle, H. I., Ferger, S. W., Cordeiro, N. J., Howell, K. M., Schleuning, M., Boehning-Gaese, K., et al. (2016). Changes in abundances of forest understorey birds on Africa’s highest mountain suggest subtle effects of climate change. Div. Distrib. 22, 288–299. doi: 10.1111/ddi.12405
Eglington, S. M., and Pearce-Higgins, J. W. (2012). Disentangling the Relative Importance of Changes in Climate and Land-Use Intensity in Driving Recent Bird Population Trends. PLoS One 7:e30407. doi: 10.1371/journal.pone.0030407
Ehardt, C. L., and Butynski, T. M. (2006). The Recently Described Highland Mangabey, Lophocebus kipunji (Cercopithecoidea, Cercopithecinae): current Knowledge and Conservation Assessment. Prim. Conserv. 21, 81–87. doi: 10.1896/0898-6207.21.1.81
Ehardt, C. L., Jones, T. P., and Butynski, T. M. (2005). Protective Status, Ecology and Strategies for Improving Conservation of Cercocebus sanjei in the Udzungwa Mountains, Tanzania. 3 Internat. J. Primat. 26:3.
Finch, J., Woller, M., and Marchant, R. (2014). Tracing long-term tropical montane ecosystem change in the Eastern Arc Mountains of Tanzania. J. Q. Sci. 29, 269–278. doi: 10.1002/jqs.2699
Forero-Medina, G., Terborgh, J., Socolar, S. J., and Pimm, S. L. (2011). Elevational ranges of birds on a tropical montane gradient lag behind warming temperatures. PLoS One 6:e28535. doi: 10.1371/journal.pone.0028535
Fowler, J., Cohen, L., and Jarvis, P. (1998). Practical statistics for field biology. Chichester: John Wiley & Sons.
Frontier Tanzania (2001). “West Kilombero Scarp Forest Reserve – botanical and forest use report,” in Report for the Udzungwa Mountains Forest Management and Biodiversity Conservation Project, eds K. Z. Doody, K. M. Howell, and E. Fanning (Iringa: MEMA), 1–45.
Gill, F., Donsker, D., and Rasmussen, P. (eds) (2021). IOC World Bird List (v 11.2). doi: 10.14344/IOC.ML.11.2
Goodale, E., and Beauchamp, G. (2010). The relationship between leadership and gregariousness in mixed-species bird flocks. J. Avian Biol. 41, 99–103. doi: 10.1111/j.1600-048x.2009.04828.x
Hino, T. (2009). Seasonality in the Function of Avian Mixed-Species Flocks in Madagascar. Ornithol. Sci. 8, 43–53. doi: 10.2326/048.008.0107
Hjejl, S. J., Verner, J., and Bell, G. W. (1990). Sequential versus initial observations in studies of avian foraging. Stud. Avian Biol. 13, 166–173.
IPBES (2018). “The IPBES regional assessment report on biodiversity and ecosystem services for Africa,” in Secretariat of the Intergovernmental Science-Policy Platform on Biodiversity and Ecosystem Services, eds E. Archer, L. Dziba, K. J. Mulongoy, M. A. Maoela, and M. Walters (Bonn: IPBES).
IPBES (2019). “Summary for policymakers of the global assessment report on biodiversity and ecosystem services of the Intergovernmental Science-Policy Platform on Biodiversity and Ecosystem Services,” in IPBES secretariat, eds S. Díaz, J. Settele, E. S. Brondízio, H. T. Ngo, M. Guèze, J. Agard, et al. (Bonn: IPBES).
IPCC (2021). “Summary for Policymakers,” in Climate Change 2021: The Physical Science Basis. Contribution of Working Group I to the Sixth Assessment Report of the Intergovernmental Panel on Climate Change, eds V. Masson-Delmotte, P. Zhai, A. Pirani, S. L. Connors, C. Péan, S. Berger, et al. (Cambridge, MA: Cambridge University Press).
Jensen, F. P., Dinesen, L., Hansen, L. A., Moyer, D. C., and Mulungu, E. (2020). Bird species richness in montane forest in the Udzungwa Mountains, Tanzania. Scopus 40, 39–49.
Karr, J. R. (1976). Seasonality, Resource Availability and Community Diversity in Tropical Bird Communities. Am. Natural. 110, 973–994. doi: 10.1086/283121
Keith, S., Urban, E. K., and Fry, C. H. (1992). Birds of Africa, Vol. IV. Cambridge, MA: Academic Press.
Khaliq, I., Hof, C., Prinzinger, R., Böhning-Gaese, K., and Pfenninger, M. (2014). Global variation in thermal tolerances and vulnerability of endotherms to climate change. Proc. R. Soc. B 281, 20141097. doi: 10.1098/rspb.2014.1097
Lambina, E. F., Turnerb, B. L., Geista, H. J., Agbolac, S. B., Angelsend, A., Brucee, J. W., et al. (2001). The causes of land-use and land-cover change: moving beyond the myths. Glob. Env. Change 11, 261–269.
Laurance, W. F., Camargo, J. L. C., Luizao, R. C. C., Laurance, S. G., Pimm, S. L., Bruna, E. M., et al. (2011). The fate of Amazonian forest fragments: a 32-year investigation. Biol. Conserv. 144, 56–67. doi: 10.1016/j.biocon.2010.09.021
Loiselle, B. A., and Blake, J. G. (1991). Temporal variation in birds and fruits along an elevational gradient in Costa Rica. Ecology 72, 180–193. doi: 10.2307/1938913
Lovett, J. (1993). “Eastern Arc Moist Forest Flora in Wasser,” in Biogeography and Ecology of the Rain Forests of Eastern Africa, ed. J. C. Lovett (Cambridge, MA: Cambridge University Press).
Lovett, J. C. (1990). Classification and status of the moist forests of Tanzania. Mitt. Inst. Allg. Bot. Hamb. 23, 287–300.
Lovett, J. C. (1996). Elevational and latitudinal changes in tree associations and diversity in the Eastern Arc mountains of Tanzania. J. Trop. Ecol. 12, 629–650. doi: 10.1017/s0266467400009846
Maldonado-Coelho, M., and Marini, M. A. (2000). Effects of forest fragment size and successional stage on mixed-species bird flocks in southeastern Brazil. Condor 102, 585–594. doi: 10.1093/condor/102.3.585
Malizia, L. R. (2001). Seasonal fluctuations of birds, fruits, and flowers in a subtropical forest of Argentina. Condor 103, 45–61. doi: 10.1093/condor/103.1.45
Marshall, A. R. Jørgensbye, H. L. O., Rovero, F., Platts, P. J., White, P. C. L., and Lovett, J. C. (2009). The species-area relationship and confounding variables in a threatened monkey community. Am. J. Primatol. 71:1.
McCain, C. M., and Colwell, R. K. (2011). Assessing the threat to montane biodiversity from discordant shifts in temperature and precipitation in a changing climate. Ecol. Lett. 14, 1236–1245. doi: 10.1111/j.1461-0248.2011.01695.x
Moreau, R. E. (1935). A critical analysis of the distribution of birds in a tropical African area. J. Anim. Ecol. 1935, 167–191. doi: 10.2307/1007
Moyer, D. C. (1992). “Report on the natural resources consultancy for the Udzungwa forest management project preparation mission,” in Annex 4 Udzungwa Forest Management Project. Ministry of Foreign Affairs, Vol. 3, ed. J. Lovett (Baton Rouge, LA: Louisiana State University).
Mulwa, R. K., Neuschulz, E. L., Böhning-Gaese, K., and Schleuning, M. (2012). Seasonal fluctuations of resources and avian feeding guilds across forest-farmland boundaries in tropical Africa. Oikos 122, 524–532. doi: 10.1111/j.1600-0706.2012.20640.x
Myers, N., Mittermeier, C. G., da Fonseca, G. A. B., and Kent, J. (2000). Biodiversity hotspots for conservation priorities. Nature 403, 853–858. doi: 10.1038/35002501
Oguntunde, P. G., Abiodun, B. J., and Lischeid, G. (2017). Impacts of climate change on hydro-meteorological drought over the Volta Basin, West Africa. Glob. Planet. Chang. 155, 121–132. doi: 10.1016/j.gloplacha.2017.07.003
Oksanen, J., Blanchet, F. G., Kindt, R., Legendre, P., Minchin, P. R., O’hara, R., et al. (2019). Vegan: Community ecology package version 2.5-6. R Package Version.
Parmesan, C., and Yohe, G. (2003). A globally coherent fingerprint of climate change impacts across natural systems. Nature 421, 37–42. doi: 10.1038/nature01286
Peh, K. S. H. (2007). Potential effects of climate change on elevational distributions of tropical birds in Southeast Asia. Condor 109, 437–441. doi: 10.1093/condor/109.2.437
Pocs, T. (1976). Bioclimatic studies in the Uluguru Mountains (Tanzania, East Africa) I. Acta. Bot. Acad. Sci. Hung. 20, 115–135.
Pounds, J. A., Fogden, M. P. L., and Campbell, J. H. (1999). Biological responses to climate change on a tropical mountain. Nature 398, 611–615. doi: 10.1038/19297
Powell, L. L., Wolfe, J. D., Johnson, E. I., Hines, J. E., Nichols, J. D., and Stouffer, P. C. (2015). Heterogeneous movement of insectivorous Amazonian birds through primary and secondary forest: A case study using multistate models with radiotelemetry data. Biol. Conserv. 188, 100–108. doi: 10.1016/j.biocon.2015.01.028
Prell, W., Hutson, W., Williams, D., Bé, A., Geitzenauer, K., and Molfino, B. (1980). Surface Circulation of the Indian Ocean during the Last Glacial Maximum, Approximately 18,000 yr B.P. Q. Res. 14, 309–336. doi: 10.1016/0033-5894(80)90014-9
R Core Team (2020). R: A language and environment for statistical computing. Vienna: R Foundation for Statistical Computing.
Rahbek, C., Borregaard, M. K., Colwell, R. K., Dalsgaard, B., Holt, B. G., and Morueta-Holme, N. (2019a). Humboldt’s enigma: What causes global patterns of mountain biodiversity? Science 365:6458. doi: 10.1126/science.aax0149
Rahbek, C., Borregaard, M. K., Antonelli, A., Colwell, R. K., Holt, B. G., Nogues-Bravo, D., et al. (2019b). Building mountain biodiversity: Geological and evolutionary processes. Science 365, 1114–1119. doi: 10.1126/science.aax0151
Raven, P. H., and Axelrod, D. I. (1978). Origin and relationships of the California flora. Univ. Calif. Publ. Botany 72, 1–134. doi: 10.2307/1929235
Recher, H. F., and Gebski, V. (1990). Analysis of the foraging ecology of Eucalyptus forest birds. Sequential versus single-point observations. Stud. Avian Biol. 13, 174–180. doi: 10.1016/j.foreco.2014.10.005
Reif, J., Marhoulc, P., and Koptík, P. (2013). Bird communities in habitats along a successional gradient: Divergentpatterns of species richness, specialisation and threat. Basic Appl. Ecol. 14, 423–431. doi: 10.1016/j.baae.2013.05.007
Remsen, J. V., and Robinson, S. K. (1990). A classification scheme for foraging behavior of birds in terrestrial habitats. Stud. Avian Biol. 13, 144–160.
Rodgers, W. A., and Homewood, K. M. (1982). Biological values and conservation prospects for the forests and primate populations of the Udzungwa Mountains, Tanzania. Biol. Conserv. 24, 285–304. doi: 10.1016/0006-3207(82)90016-7
Romdal, T. S., and Rahbek, C. (2009). Elevational zonation of afrotropical forest bird communities along a homogeneous forest gradient. Journal of Biogeography. 36, 327–336. doi: 10.1111/j.1365-2699.2008.01996.x
Root, T. L., Price, J. T., Hall, K. R., Schneider, S. H., Rosenzweig, C., and Pounds, J. A. (2003). Fingerprints of global warming on wild animals and plants. Nature 421, 57–60. doi: 10.1038/nature01333
Santillan, V. Q. M., Tinoco, B. A., Zarate, E., Schleuning, M., Böhning-Gaese, K., et al. (2018). Spatio-temporal variation in bird assemblages is associated with fluctuations in temperature and precipitation along a tropical elevational gradient. PLoS One 13:e0196179. doi: 10.1371/journal.pone.0196179
Sherry, T. W. (1990). When are birds dietarily specialised. Distinguish ecological from evolutionary approaches. Stud. Avian Biol. 13, 337–352.
Smith, D. A., Smith, Y. C. V., and Downs, C. T. (2017). Seasonal habitat requirements of Lemon Dove (Aplopelia larvata) in coastal forest: camera-trap surveys of a reclusive species. Afr. Zool. 52, 199–207.
Sridhar, H., Beauchamp, G., and Shanker, K. (2009). Why do birds participate in mixed-species foraging flocks? A large-scale synthesis. Anim. Behav. 78, 337–347. doi: 10.1016/j.anbehav.2009.05.008
Stuart, S. N., Jensen, F. P., and Brogger-Jensen, S. (1987). Altitudinal zonation of the avifauna in Mwanihana and Magombera forests, Eastern Tanzania. Le Gerfaut 77, 165–186.
Stuart, S. N., Jensen, F. P., Brøgger-Jensen, S., and Miller, R. I. (1993). “The zoogeography of the montane forest avifauna of eastern Tanzania,” in Biogeography and ecology of the rainforests of eastern Africa, eds J. C. Lovett and S. K. Wasser (Cambridge, MA: Cambridge Univ. Press).
Urban, E. K., Fry, H. C., and Stuart, K. (1997). The birds of Africa. Cambridge, MA: Academic Press.
Vieira, D. L. M., de Limac, V. V., Sevilha, A. C., and Scariot, A. (2008). Consequences of dry-season seed dispersal on seedling establishment of dry forest trees: Should we store seeds until the rains? For. Ecol. Manag. 256, 471–481. doi: 10.1016/j.foreco.2008.04.052
Wasser, S., and Lovett, J. C. (eds) (1993). Biogeography and Ecology of the Rain Forests of Eastern Africa. Cambridge, MA: Cambridge University Press.
Werema, C., Howell, K. M., and Ndangalasi, H. J. (2016). Seasonal use of remnant forest fragments by understorey forest birds in the Uluguru Mountains, Tanzania: a conservation priority. Ostrich 87, 255–262. doi: 10.2989/00306525.2016.1212120
Wheelwright, N. T. (1986). A seven-year study of individual variation in fruit production in tropical bird-dispersed tree species in the family Lauracea. TAVS 15, 19–35. doi: 10.1007/978-94-009-4812-9_3
Williams, S. E., and Middleton, J. (2008). Climatic seasonality, resource bottlenecks, and abundance of rainforest birds: implications for global climate change. Div. Distrib. 14, 69–77. doi: 10.1111/j.1472-4642.2007.00418.x
Willig, M. R., and Presley, S. J. (2016). Biodiversity and metacommunity structure of animals along altitudinal gradients in tropical montane forests. J. Trop. Ecol. 32, 421–436. doi: 10.1017/s0266467415000589
Keywords: altitudinal movement, Eastern Arc, mixed-species flocks, seasonality, Udzungwas
Citation: Dinesen L, Lehmberg T, Romdal TS, Sonne J and Hansen LA (2022) Seasonal Changes in an Afromontane Forest Bird Community in Tanzania. Front. Ecol. Evol. 10:768020. doi: 10.3389/fevo.2022.768020
Received: 31 August 2021; Accepted: 08 March 2022;
Published: 05 April 2022.
Edited by:
David Horák, Charles University, CzechiaReviewed by:
Norbert Cordeiro, Field Museum of Natural History, United StatesRobert Brian O’Hara, Norwegian University of Science and Technology, Norway
Copyright © 2022 Dinesen, Lehmberg, Romdal, Sonne and Hansen. This is an open-access article distributed under the terms of the Creative Commons Attribution License (CC BY). The use, distribution or reproduction in other forums is permitted, provided the original author(s) and the copyright owner(s) are credited and that the original publication in this journal is cited, in accordance with accepted academic practice. No use, distribution or reproduction is permitted which does not comply with these terms.
*Correspondence: Lars Dinesen, bGFycy5kaW5lc2VuQHN1bmQua3UuZGs=