- 1Afromontane Research Unit & Department of Geography, University of the Free State Qwaqwa Campus, Phuthaditjhaba, South Africa
- 2Buffelskloof Herbarium (BNRH), Lydenburg, South Africa
- 3C.E. Moss Herbarium, School of Animal, Plant and Environmental Sciences, University of the Witwatersrand, Johannesburg, South Africa
- 4Mpumalanga Tourism and Parks Agency, Lydenburg, South Africa
- 5Unit for Environmental Sciences and Management, North-West University, Potchefstroom, South Africa
Climatic, edaphic, and topographic differences between mountains and surrounding lowlands result in mountains acting as terrestrial islands with high levels of endemic biota. Conservation of mountains is thus key to successful biodiversity conservation. The Limpopo–Mpumalanga–Eswatini Escarpment (LMEE) in South Africa and the Kingdom of Eswatini is one of the largest components of southern Africa’s Great Escarpment. Despite botanical collecting effort over 150 years, there has never been a holistic and comprehensive synthesis of plant endemics data for the LMEE. For the first time, we define the LMEE as an orographic entity, covering 53,594 km2; it forms a contiguous highland area from the Pongola River in the south, north to the Woodbush area, and includes rugged western Eswatini. Using exhaustive literature mining, coupled with combined decades of fieldwork by the authors, and up-to-date taxonomic assessments of the 46 undescribed species, we provide the first robust list of plant endemics for the LMEE. The LMEE has 496 endemic plant taxa, comprising 10.7% of the provisional flora (4,657 taxa). This is more than double the endemic plant taxa in the Drakensberg Mountain Centre (DCM), and may be the richest concentration of montane endemics in southern Africa outside of the Core Greater Cape Floristic Region. Grassland hosts the largest number of endemics (74.2%), followed by Savanna (26.6%), then Forest (7.7%). Most endemics of conservation concern occur in Grassland (68.4%), in which one is Extinct and two are Extinct in the Wild. Evolutionary partitioning between Grassland, Savanna and Forest is suggested by low introgression of Biomes at family and genus level, and by a dominance of life-forms adapted to open habitats. High threat statuses for Grassland endemics can be attributed to the historical transformation of almost 20% of Grassland to forestry pre-1990, and ongoing degradation of primary Grassland. With conservation area coverage only 11.1% of the LMEE, the exceptional richness of the endemic flora—combined with major conservation threats—suggest that the LMEE should become a major focus of conservation effort between South Africa and Eswatini as a matter of urgency.
Introduction
Because of the climatic, edaphic, and topographic differences between mountains and surrounding lowlands, mountains are often terrestrial islands with high levels of endemic biota (Merckx et al., 2015; Noroozi et al., 2018; Perrigo et al., 2019). Conservation of mountains is thus key to successful biodiversity conservation (Noroozi et al., 2018; Callisto et al., 2019). However, effective conservation planning is dependent on accurate biodiversity data (Eken et al., 2004; Underwood et al., 2018; Hoveka et al., 2020)—notably for range-restricted species that may be more vulnerable to extinction than widespread ones (Shrestha et al., 2019; Loiseau et al., 2020). For both ecological and historical biogeographical research streams, biogeographical studies require robust biodiversity inventories that can be used for analyses (König et al., 2019). Despite this, the biological diversity of many mountain systems globally—and especially those in southern Africa (Clark et al., 2011a; Hoveka et al., 2020)—remains inadequately documented. Lack of detailed and accurate biodiversity data has resulted from: (i) A general decline in baseline taxonomic collecting effort, in favor of using databases “as are”—especially for difficult-to-access mountains; (ii) a predominant focus on phytosociological methods, that by nature do not record fine-scale diversity; and (iii) digital herbarium records only becoming available recently (Hoveka et al., 2020).
There is a growing body of work in southern Africa that attempts to combine physical geography (in this case “escarpment mountains”) and plant geography (in this case “endemism”) (e.g., Clark et al., 2009, 2011b,2014; Hahn, 2017, 2019; Carbutt, 2019), rather than matching endemism to “homogenous” vegetation units (e.g., the approach of Mucina and Rutherford, 2006). Determining the “mountain” (i.e., area) before the “endemic suite” is a well-established practice for determining mountain floras and patterns of endemism in mountains globally, despite it being seen with some skepticism by plant geographers. This approach provides the basis for mountain-specific statements relating to biodiversity (“mountains are rich in endemics”), and inclusion of mountains in key inter-governmental conventions (e.g., Agenda 21: Chapter 13, Intergovernmental Panel on Climate Change: High Mountain Areas, etc.; Hock et al., 2019). The challenge in southern Africa is that spatial delimitations informing endemism have been based on overall poor plant collecting coverage (Hoveka et al., 2020)—and a focus on macro-scale phytosociology—while fine-scale endemic-data remains under-appreciated. The exception is the celebrated Core of the Greater Cape Floristic region (hereafter referred to as the “Cape”; Born et al., 2007), which has seen an intense and sustained, c.350-year long focus on botanical exploration, discovery, taxonomic capacity, and an unrivaled appreciation for fine-scale endemism by a strong botanical community of practice (both professional and lay) (cf. Manning and Goldblatt, 2012; too many examples to mention here). Also, in the Cape region it is clear that physical geography (e.g., the Cape Fold Mountains) and plant geography (vegetation units associated to these mountains) overlay quite neatly (e.g., Mucina and Rutherford, 2006), thanks to the discrete linear sub-ranges surrounded (more or less) by pediplains on most sides (Linol and De Wit, 2016). Nevertheless, there would be value in teasing out the mountain contribution to endemism in the Cape Flora, to allow for equitable comparison, i.e., mountain endemism with mountain endemism—outside the Cape, regionally and globally.
“Simple” orographic delimitation is effectively used to define many of the world’s great, discrete massifs (e.g., Körner et al., 2011). In South Africa, this works for the Cape Fold Mountains, but it is more complicated for the extra-Cape mountains due to their orogenesis—they are mostly passive post-Gondwana Escarpments, cuestas, or inselbergs (Partridge and Maud, 1987). Inselbergs could be considered “easy,” but variation in size and elevation opens other discussions around inclusion, and inselberg swarms pose other problems. A more complex and nuanced method is thus required to define mountains or escarpments in southern Africa.
The mountainous north-eastern parts of South Africa and adjacent Kingdom of Eswatini (Eswatini)—formerly known as the “North-eastern Transvaal Drakensberg”—have been of long-standing fascination to botanists (Van Wyk and Smith, 2001). As a result, they have been relatively well-botanized over a period of some 150 years, and have a proven wealth of endemic species (e.g., Croizat, 1965; Deall and Backer, 1989; Matthews et al., 1993; Van Wyk and Smith, 2001; Dlamini and Dlamini, 2002). Various components of the area have been recognized for their endemic diversity and richness (e.g., Croizat, 1965)—largely along edaphic lines (e.g., Matthews et al., 1993)—leading to Van Wyk and Smith (2001) describing three Centers of Floristic Endemism: the Barberton, Sekhukhuneland, and Wolkberg Centers in this region. The Lydenburg Center was a later proposed addition by Emery et al. (2002), and these Grassland-focused centers have been incorporated into Mucina and Rutherford’s (2006) “Northern Sourveld Endemics” concept. Despite this effort, there has never been a holistic and comprehensive synthesis of plant endemic data for this section of the southern African Great Escarpment (in South Africa and Eswatini)—which we term the Limpopo–Mpumalanga–Eswatini Escarpment (LMEE). The aims of this paper are therefore to define the LMEE as a discrete orographic entity as part of the southern African Great Escarpment; provide the first robust list of plant endemics for the LMEE; determine in which Biome most LMEE endemics occur; determine which life-forms dominate amongst the endemic taxa; and determine the level of conservation concern for the endemic flora of the LMEE.
Materials and Methods
Defining the Limpopo–Mpumalanga–Eswatini Escarpment
The basic premise for the delimitation of the LMEE is based on geomorphology, i.e., an escarpment on which various biodiversity and ecological complexities occur. The starting point was the global dataset on mountainous areas, as defined and created by Körner et al. (2011). Because ruggedness (a change in elevation over a given distance) was the main determinant by Körner et al. (2011), we felt that it represented a rather crude indication of escarpments, as it focused on areas with a change in elevation rather than the identification of summits, hilltops or plateaus. Escarpments typically comprise relatively narrow areas where coastward planes meet interior plateaus, the rugged escarpment often merging seamlessly with the interior plateau (Ollier, 1982, 1991; Partridge and Maud, 1987; Moore and Blenkinsop, 2006). We therefore applied an approach where we combined “ruggedness” together with landscape position to more accurately delimit “escarpment mountains.” A Digital Elevation Model (DEM) raster was used, with a resolution of 30 arc-seconds (∼1 km) and covered most of Africa south of the equator (U.S. Geological Survey’s Center for Earth Resources Observation and Science., 1996). To determine ruggedness, we used ArcGIS Pro 1.3 and the geo-processing tool called Focal Statistics [using Range as statistics type with a Neighborhood search window of 8 × 8 cells, also as used in Körner et al. (2011)]. To calculate topographic position index (TPI) we used ArcMap 1.6 and the Land Facet Corridor Designer, v.1.2.884 add-in [from the Land Facet Corridor Designer toolbox of Weiss (2001) and Jenness et al. (2013)]. TPI reflects the difference in elevation between a focal cell and all cells in a defined search neighborhood; this approach is very useful for determining mountain summits, or higher-lying areas at a predetermined neighborhood search radius. The TPI was calculated using two search neighborhood distances to identify areas with the highest elevations (summit areas) within the surrounding landscape at distances of 200 km and 250 km. The TPI was then calculated at a 50 km neighborhood to determine regional/provincial scale summit and mountainous areas. The output GIS layers for each of the above GIS processes (2 × TPI and 1 × ruggedness) were rescaled to a common scale of 1–10 using the Rescale by Function tool in ArcGIS Pro. The values were summed using the Weighted Sum tool, effectively averaging the input from all three processes. This approach of rescaling and weighted sum is commonly used in habitat suitability analyses (Store and Kangas, 2001). Each layer was given the same weighting (33.3%). Output from the Weighted Sum tool was then classified using the upper standard deviation value limit to identify mountainous areas. The output was converted to a polygon, and cleaned up using a buffer distance of 750 m to eliminate small gaps/sinks. Fragments smaller than 35,000 ha were removed, and free-standing inselbergs were excluded (of which there are very few) resulting in a continuous study area boundary.
Listing the Endemics of the Limpopo–Mpumalanga–Eswatini Escarpment
It is important to be explicit about what one means by endemic before listing the endemic taxa of a geographical area. In this paper, “endemic species” means species confined to a particularly defined area based on current records and distributions (Anderson, 1994), while “near-endemic species” means species whose ranges are closely aligned with the same defined area as “endemic species” but not strictly confined to that area (Matthews et al., 1993; Carbutt and Edwards, 2006); near-endemics are much more subjective to define than endemics (Carbutt and Edwards, 2006). Endemism can be scaled geographically—“local (narrow) endemism,” confined to a relatively small area (e.g., Table Mountain); “regional endemism,” endemic to a larger area or region (e.g., southern Africa)—but also considered in terms of pre-defined vegetation units and biomes with areal delimitations, such as Grassland or Fynbos Biome endemics (e.g., Mucina and Rutherford, 2006).
A list of endemic species was compiled by extensive literature mining, building on the foundational works of inter alia (Burtt Davy, 1926; Croizat, 1965; Compton, 1976; Deall and Backer, 1989; Morrey et al., 1989; Matthews et al., 1993; Balkwill et al., 1997; Scott-Shaw, 1999; Van Wyk and Smith, 2001; Dlamini and Dlamini, 2002), merging data from these historical works, and updating the list with a combination of more recent taxonomic revisions, new species descriptions, and the expert knowledge of the authors (including not yet described endemic species). A total of 209 references (Supplementary Material 1) was used to build this list of endemic taxa. For many taxa, determining actual geographic distribution was difficult, and decisions as to endemicity were resolved using data from the South African National Biodiversity Institute [SANBI],1 the African Plant Database,2 herbarium records (BNRH, J), and unpublished data held by the authors (notably SS for Sekhukhuneland, KB for the Barberton area, and JB, BT, and ML for the Lydenburg area). This list was then refined by updating taxonomy through consultation with specialists, removing duplicates and synonyms, and citing the most relevant reference per species. Each endemic was assigned the most relevant publication citation, or—if not yet described—a herbarium specimen reference was provided. The final compilation was tabulated (Supplementary Material 2). For the sake of simplicity, we have not attempted to enumerate near-endemics in this study.
The ten families and genera with the largest number of taxa endemic to the LMEE were determined from Supplementary Material 2. The proportion of endemism in the LMEE flora was calculated using a provisional LMEE flora of 4,657 taxa (Timberlake and Clark, in preparation). We compared LMEE endemic floristic patterns with the Maloti-Drakensberg (Carbutt, 2019), being the only other large summer rainfall section of Escarpment with robust published data at this level. Endemism comparisons were made against other mesic southern African mountains for which endemism data are available, viz. the Cape Midlands Escarpment (Clark et al., in preparation), the Maloti-Drakensberg (Carbutt, 2019), the Manica Highlands (Timberlake and Clark, in preparation), Mount Mulanje (Strugnell, 2002), the Nyika Plateau (Burrows and Willis, 2005), and the Soutpansberg (Hahn, 2017). Comparative endemic richness (diversity by region) was plotted and graphed using log-transformed data.
Determining Life-Forms
Each endemic taxon was assigned a life-form based on a modified version of the Raunkiaer system (Raunkiaer, 1934). An additional category under Chamaephytes was added: geoxyles (geoxylic growth forms of woody subshrubs characterized by massive lignotubers, or underground woody axes, from which emerge aerial shoots which may be ephemeral), given the large number of species in this life-form category in the LMEE (and elsewhere in Africa) (Olivier et al., 2014) and their misfit in the existing Raunkiaer system. Proportions of life-forms in the endemic flora were calculated.
Determining Distribution of Endemics in Biomes
The Biomes present [Grassland, Savanna, and Forest; as defined by Mucina and Rutherford (2006)] and their extent in the LMEE were identified, calculated and mapped using the latest version of the South African Vegetation map. In the LMEE, “Savanna” includes lightly wooded areas on otherwise grassy mountain slopes as well as the conventional “vegetation with a grass-dominated herbaceous layer and low to tall trees” (Mucina and Rutherford, 2006). The endemic taxa were assigned to each of the three Biomes; to achieve this we used a combination of descriptions in the endemic literature (Supplementary Material 1), together with personal field experience of the authors, and from the labels of herbarium vouchers (at BNRH and J, and using JSTOR Global Plants). Proportional representation per Biome was determined accordingly, at family, generic, and (infra-)specific levels.
Determining Level of Conservation Concern
The Red Data category (International Union for Conservation of Nature [IUCN], 2021) of each endemic taxon was collated from the SANBI Threatened Species Programme for taxa occurring in South Africa, and from Dlamini and Dlamini (2002) for taxa only occurring in Eswatini. From this, the proportion of endemic taxa per Red Data category was determined. Biome and life-form (see below) partitioning among the categories in the threatened bundle (E, EW, CR, EN, VU, LRnt) were specifically determined.
As land transformation is typically the largest threat to the conservation of narrow endemics (Newbold et al., 2018), land-cover change (1990–2020) was determined for the LMEE (for South Africa only, as there is no data for Eswatini) to determine trends over these 30 years; the datasets were sourced from Thompson (2016, 2021) for the years 1990 and 2020 and analyzed in ArcGIS Pro. The 1990 and 2020 land-cover classes were first standardized into fewer common classes, before determining the size of each class and then calculating the rate of change. A current protected area (PA) map (formal PAs in addition to conservation areas (CA), i.e., those with conservation agreements but not formally gazetted as PAs) and proportion of the LMEE under conservation management was generated using the South Africa Protected Areas Database (SAPAD; Department of Forestry, Fisheries and the Environment [DFFE], 2021a) and the South Africa Conservation Areas Database (SACAD; Department of Forestry, Fisheries and the Environment [DFFE], 2021b), as an indicator of conservation success (or otherwise) in the LMEE. In addition, as climate change is a major risk to areas with high local endemism (Manes et al., 2021), anticipated long-term changes to rainfall patterns were determined using a linear trend in mean annual precipitation values over 39 years (1981–2020), and expressed as a rate of change for each pixel in the multidimensional raster [using CHIRPS data from Funk et al. (2015)]. The analysis was run using the Generate Trend Raster geoprocessing tool in ArcGIS Pro. Additionally, from literature and authors’ knowledge of the LMEE, general conservation threats to the endemic flora were identified.
Results
Definition of the Limpopo–Mpumalanga–Eswatini Escarpment
Covering 53,594 km2, the LMEE comprises that component of the eastern Great Escarpment in South Africa and Eswatini occurring between the Pongola River in the south, Woodbush in the north, the Highveld in the west, and the Lowveld in the east (23°31′′23″″S–27°49′′S and 29°50′′E–31°37′′E; Figure 1 and Supplementary Plate 1). The rugged, northern components were much easier to define than the southern components, due to the seamless merger in the south-west and south with the interior Highveld Plateau, and less of a distinct Escarpment edge in Eswatini in the south-east. More specifically, the study area is a complex region with a very irregular boundary that straddles the northern-most edge of KwaZulu-Natal (KZN) province in South Africa, western Eswatini, and almost all of the mountainous areas of Mpumalanga province in South Africa, and a good proportion of the mountainous areas in Limpopo province (those occurring outside the study area in this province being the Soutpansberg, Waterberg, and western Strydpoortberge). Just over half (53%) of the LMEE occurs in Mpumalanga province, followed by Limpopo province (22%), and KZN and Eswatini with roughly equal amounts (13 and 12%). Significantly, the LMEE comprises 37% of the surface area in both Mpumalanga province and Eswatini—indicating high significance for provincial and national policy for each (e.g., Emery et al., 2002)—but only 10% of the surface area of Limpopo province, and 7% of KZN.
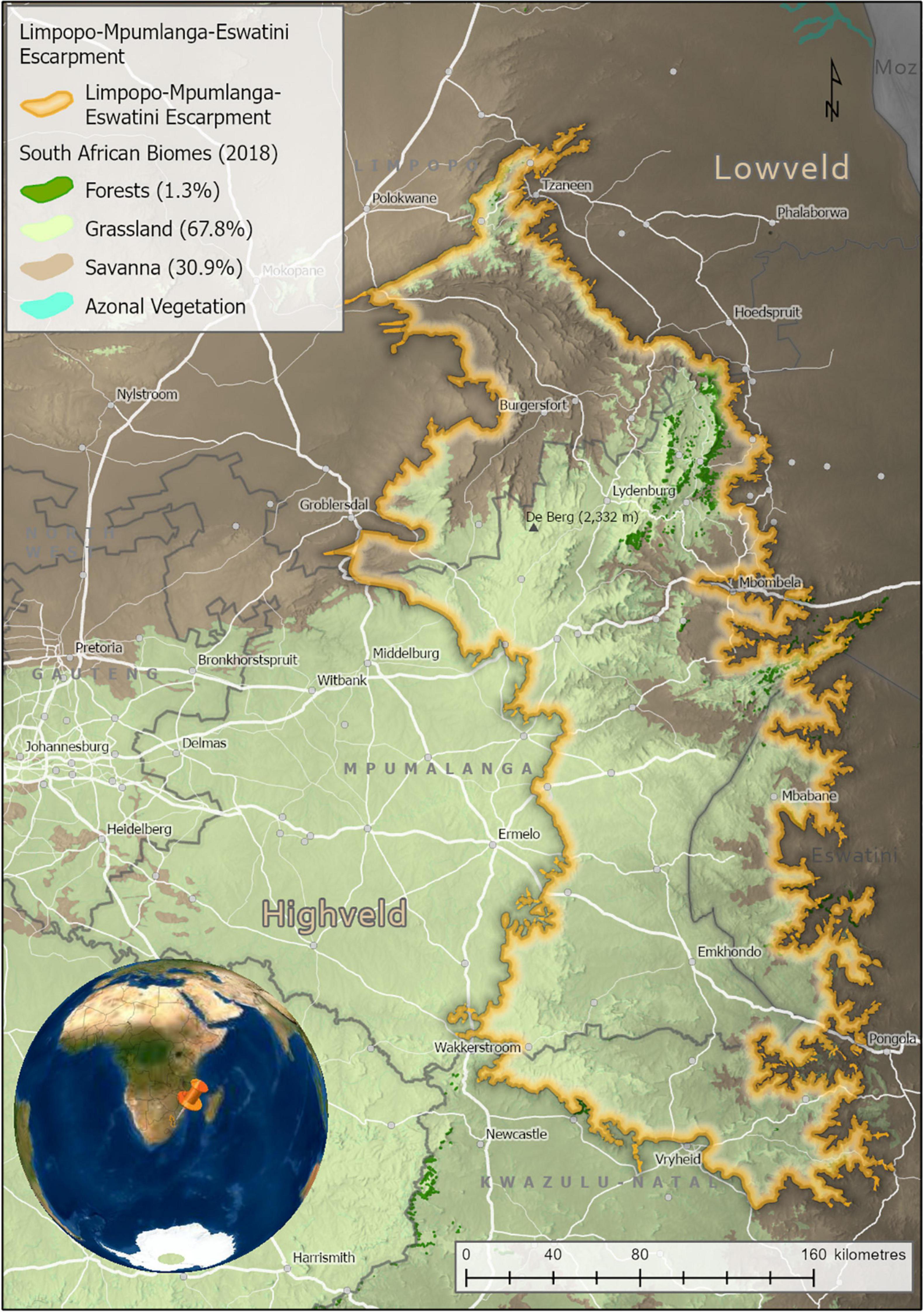
Figure 1. The Limpopo–Mpumalanga–Eswatini Escarpment (LMEE), South Africa, and Eswatini, showing key cadastral features and Biomes (percentages are of the total LMEE area). Biomes derived from the Vegetation of South Africa, Lesotho, and Swaziland (Mucina and Rutherford, 2006). Elevation values are displayed in dark (lower elevations) to light shading (higher elevations).
In the south, the LMEE is primarily of low topographic heterogeneity, comprising large, flat higher elevation areas stepping down relatively gently to the Eswatini Lowveld in the east and the KZN Middle-veld in the south. In the south-west it merges seamlessly with the uniform eastern Highveld plateau. The LMEE becomes progressively more topographically variable further north: the Barberton Mountainlands, lying perpendicular to the main Escarpment, run into northern Eswatini, while north of here the dramatic eastern ramparts of the “Transvaal Drakensberg” take shape from Kaapsehoop, and run north to the Wolkberg; this spectacular Escarpment is made more complex by numerous deep incisions from fluvial erosion, the most dramatic being the Olifants River valley and the Blyde River Canyon. North of the Wolkberg the Escarpment tapers into the lower-elevation, less rugged Haenertsburg–Woodbush–Modjadji area. In the far north-east, the LMEE ends at the “Nkumpi Interval” in the Strydpoortberge—separated from the further western mountains by this major fluvial incision. Compared to the south-west, the central-west and north-west are much more complex, adding major topographic interest to the study area in the form of the rugged Sekhukuneland area (part of the eastern limb of the Bushveld Igneous Basin). The highest point in the LMEE is “De Berg” (2,332 m) on the Steenkampsberg (Figure 1).
The biophysical environment of the LMEE has been well documented by many authors [too many to mention here, but see Deall and Backer (1989), Matthews et al. (1993), Van Wyk and Smith (2001)]. In summary, the LMEE is geologically extremely diverse, hosting some of the oldest geological formations currently recognized on earth (viz. the Barberton Greenstone Belt), and an array of formations that include basement granites, serpentinites, ultramafics, carboniferous rocks, various metamorphics, and sedimentary sequences (De Wit et al., 2011). Climatically, the LMEE is temperate to sub-tropical, with strong temperature and moisture gradients across the Escarpment from the Lowveld (hot and dry) in the east to the Highveld (cool and moist) in the south-west, and Bushveld (warm and semi-dry) in the north-west. The LMEE supports various bioregions and vegetation units associated with the Grassland, Savanna and Forest Biomes (Mucina and Rutherford, 2006).
Land-use in the LMEE is complex (Figure 2), comprising a mix of commercial forestry (12.6%), conservation land (e.g., Blyde River Canyon, Buffelskloof Private, and Lekgalameetse Nature Reserves; Wolkberg Wilderness Area; Blyde to Kruger Biosphere Reserve; and numerous Natural Heritage Sites—a total of 11.1% of the area), areas under customary law, small to medium-sized urban areas, rangeland, commercial and subsistence agriculture, mining, and tourism (centered on natural scenery—the study area is famous for its vistas, waterfalls, hiking trails, biodiversity, and historical and cultural sites). The LMEE is also the main provider of fresh water to the Lowveld, notably for large-scale irrigation of tropical fruit production and the rivers of the Kruger National Park. The area has a rich cultural, literary and historical heritage, being notable for several gold rushes in the later 1800s; the fabled “missing Kruger millions” of President Paul Kruger (last president of the Zuid-Afrikaanse Republiek, until 1900); the legendary Modjadji Rain Queen; and Anglo-Boer War battles. The rich history of the area has been immortalized in literary works such as Jock of the Bushveld (FitzPatrick, 1907), Lost Trails of the Transvaal (Bulpin, 1956), and Valley of the Mists (Klein, 1972), and the lore and cultures of the Ndebele, Pedi, Shangane and Swazi people groups.
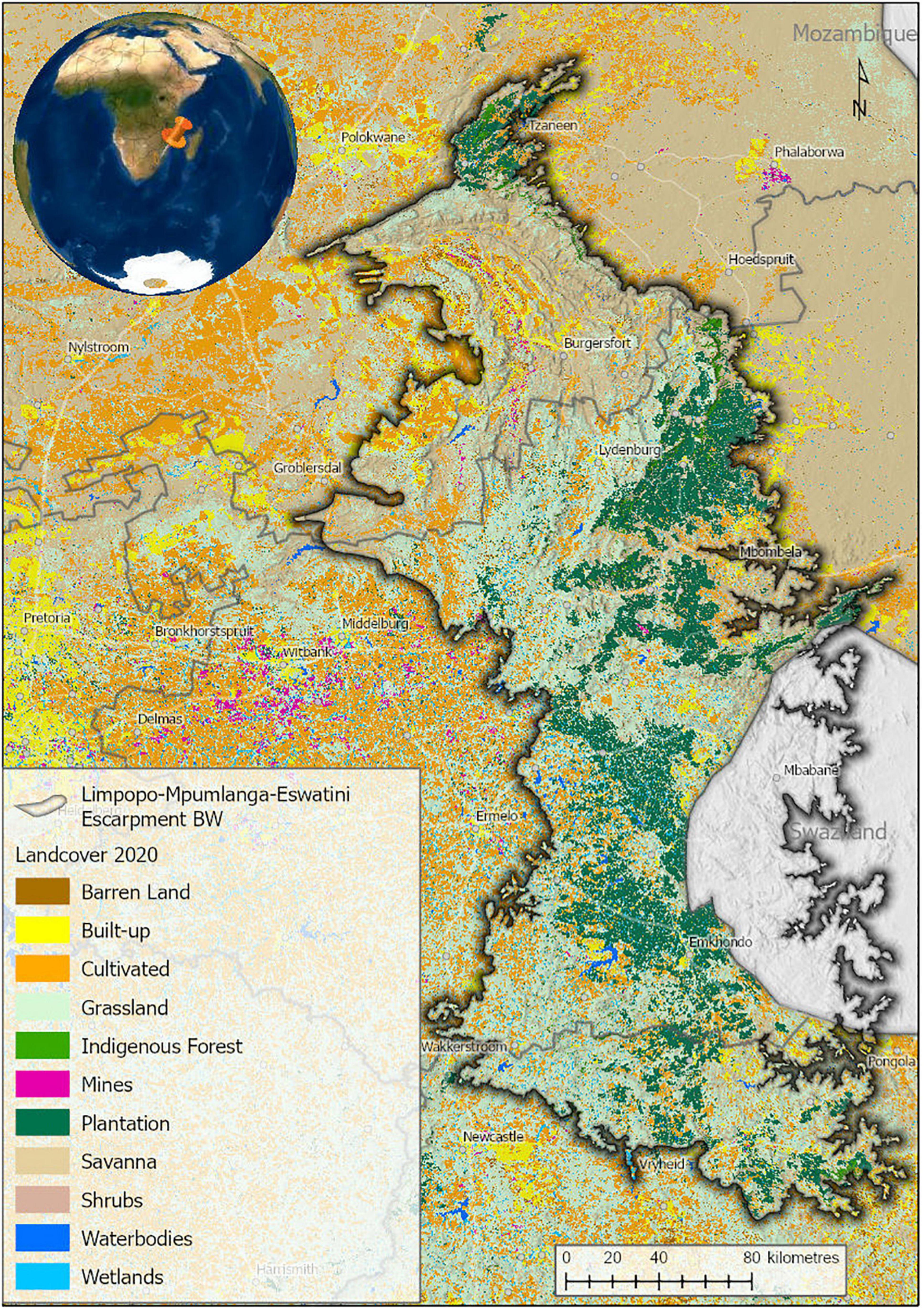
Figure 2. Land-cover in the Limpopo–Mpumalanga–Eswatini Escarpment (LMEE) in 2020. Land-cover generated from Sentinel 2 satellite imagery for South Africa (Geoterraimage, 2021).
List of Endemics of the Limpopo–Mpumalanga–Eswatini Escarpment
The LMEE has 496 endemic taxa, representing 52 families and 158 genera (Table 1 and Supplementary Material 2). This list includes two Pteridophytes, nine Gymnosperms (all Zamiaceae), and 485 Angiosperms; the latter are dominated by Dicotyledons (321, compared to 164 Monocotyledons). As a comparison, the DMC has six endemic Pteridophytes (Crouch et al., 2011), but no endemic Gymnosperms. There are no endemic families or genera in the LMEE—compared to no endemic families and two endemic genera in the DMC (Carbutt, 2019). However, the monotypic genus Prototulbaghia (currently sunk under Tulbaghia, Alliaceae; Stafford et al., 2016) may be a good genus (Vosa et al., 2011), and if resurrected would be an endemic genus in the LMEE. The LMEE is the richest montane area in southern Africa for Zamiaceae, with nine endemic taxa.
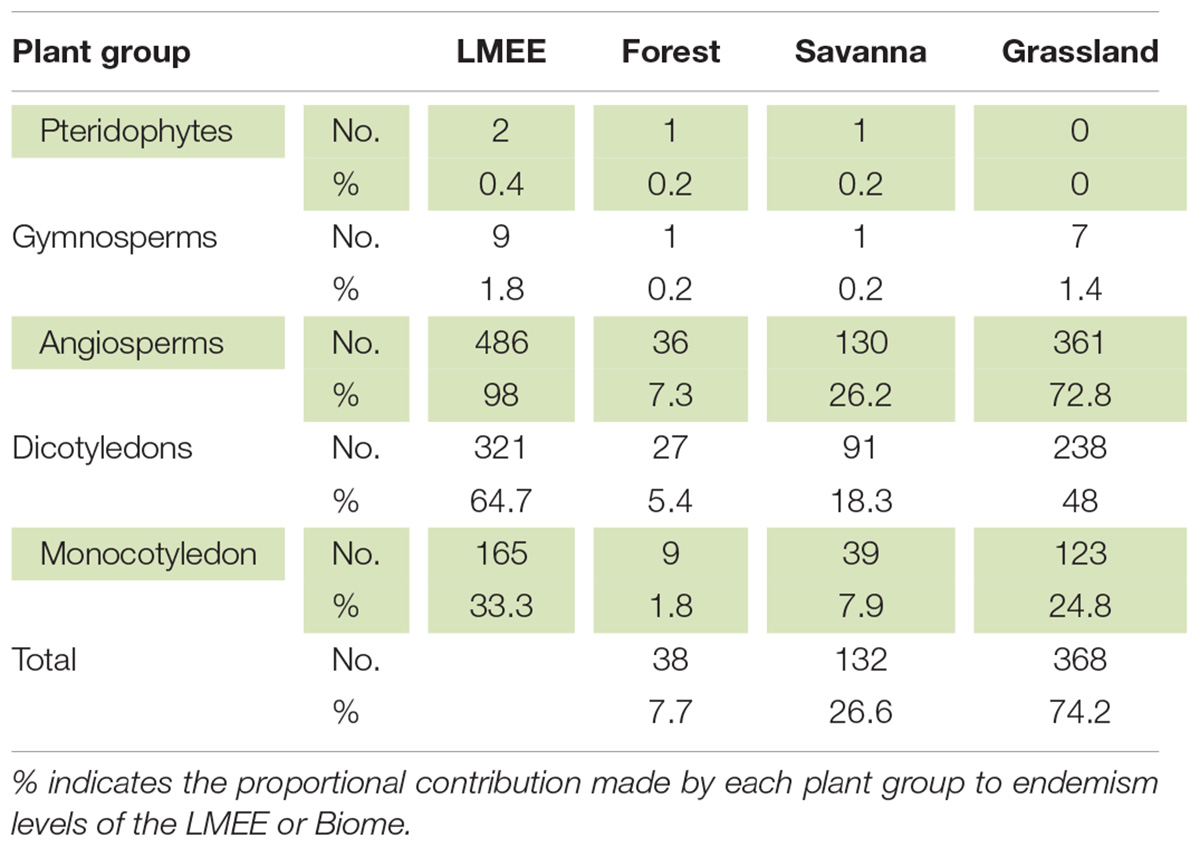
Table 1. Distribution of endemics per major plant group among Biomes in the Limpopo–Mpumalanga–Eswatini Escarpment (LMEE).
Asteraceae is the family with the most endemic taxa (57), followed by Lamiaceae (46) and Apocynaceae (42) (Figure 3A); this is contrary to the DMC, which has Scrophulariaceae and Iridaceae as second and third families (Carbutt, 2019). In the LMEE, 30 genera have ≥ 5 endemic taxa, and the three genera with the most endemic taxa are Aloe, Helichrysum, and Ledebouria; this compares to Helichrysum, Senecio, and Delosperma in the DMC (Carbutt, 2019). There are several tied contenders for the top ten endemic-rich genera, for example three share eighth place (Asparagus, Disa, Encepharlatos), four ninth place (Euphorbia, Ocimum, Searsia, Selago), while two tenth place (Protea, Senecio) (Figure 3B).
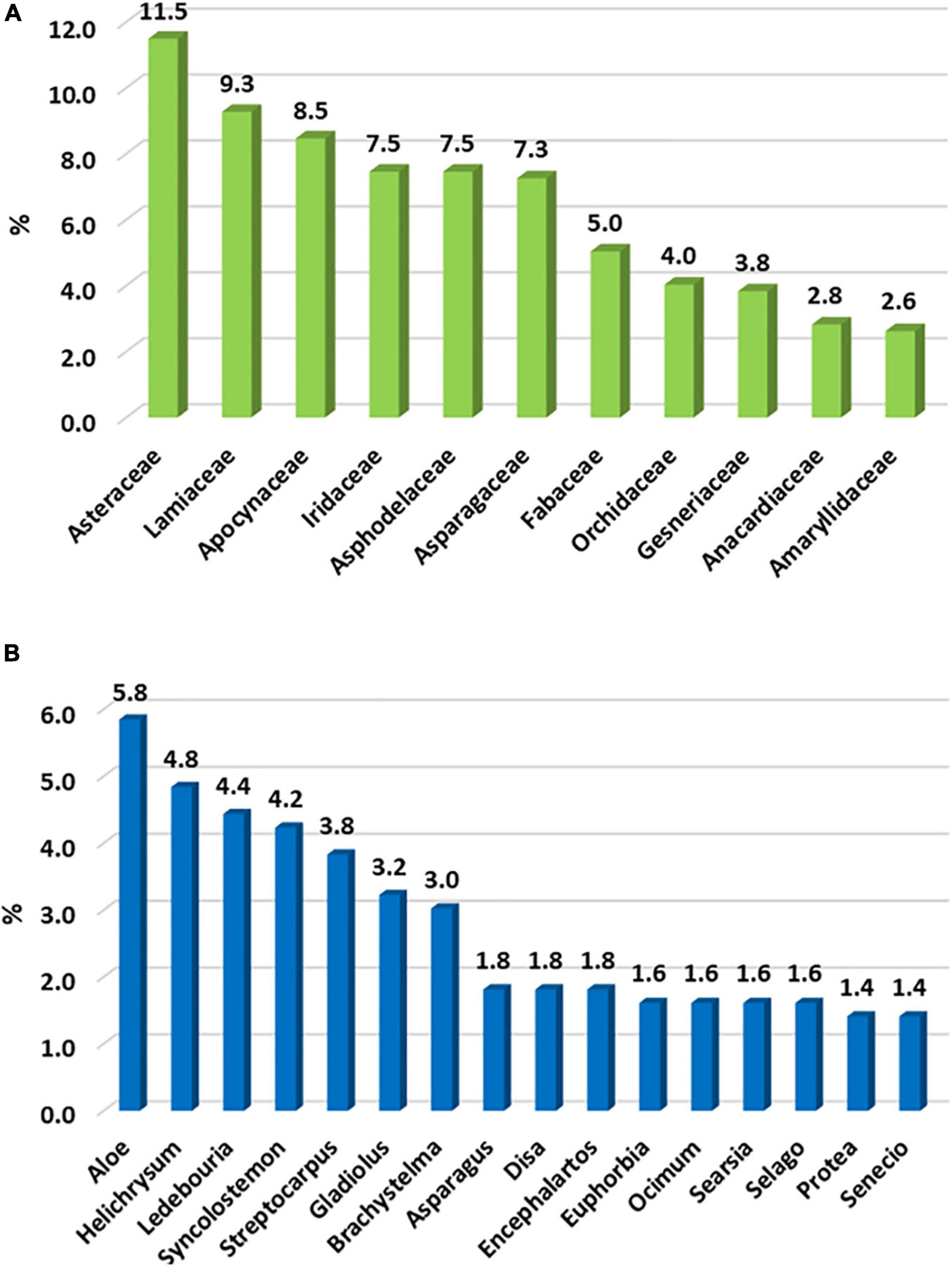
Figure 3. The top ten (A) families and (B) genera hosting the most endemic plant taxa in the Limpopo–Mpumalanga–Eswatini Escarpment (LMEE); we include shared results, resulting in 11 families and 16 genera holding the top ten positions.
Considering the estimated 56,451 vascular plant species known for the Afrotropical flora (Raven et al., 2020), the LMEE flora of 4,657 taxa contributes 8.25% to this continental diversity and comprises 23% of the South African flora (Klopper, 2021). Endemism comprises 10.7% of the provisional LMEE flora (Table 2). This may be the richest concentration of montane endemics in southern Africa outside of the Core of the Greater Cape Floristic Region (Manning and Goldblatt, 2012)—being more than double the number of endemics in the DMC, and having the highest endemic richness of any other summer rainfall mountain system in southern Africa for which we have data. While the slightly larger area size of the LMEE compared to the DMC and the Cape Midlands Escarpment (CME) may contribute to its richer endemism, area—as indicated by Clark et al. (2014)—appears overall to be a weak predictor of endemic richness in southern African mountains (Supplementary Material 3). Also, our refined LMEE endemics tally (compared with Clark et al.’s 2014 estimate) places the LMEE quite superior to the DMC.
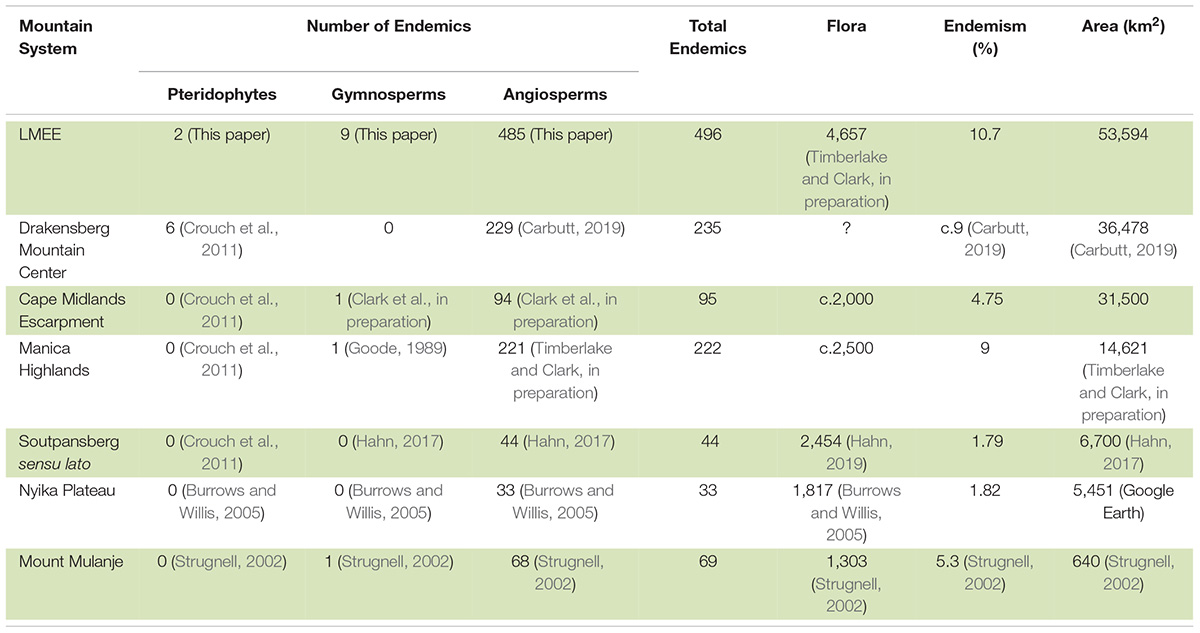
Table 2. Selected comparison of Limpopo–Mpumalanga–Eswatini Escarpment (LMEE) floral endemism with that of other mesic southern African mountains, arranged in descending order of areal extent.
Distribution of Endemics Among Biomes
Grassland is by far the dominant Biome in the LMEE (74.2%), covering large parts of the center and south, with a thin wedge extending along the Escarpment rim to the north (Figure 1). Although Savanna dominates in the north and central east, it is only a third (26.6%) of the total LMEE. Forest is by far the smallest Biome (7.7%), being confined to comparatively small patches as Mucina and Rutherford’s (2006) Northern Afrotemperate Forest (KZN–Mpumalanga border), Scarp Forest (mostly in Eswatini), and Northern Mistbelt Forest (remainder of the LMEE) along the prevailing/south-facing parts of the LMEE, and in riparian environments; it is worth noting that some of the largest patches of evergreen forest in South Africa outside of the southern Cape occur in the LMEE, notably in the Mariepskop and Wolkberg areas (cf. Van Wyk and Smith, 2001). Given that Grassland is the dominant Biome, it is perhaps not surprising then that this is the overwhelmingly dominant habitat for LMEE endemics, hosting almost three-quarters of the endemics, while Savanna hosts a quarter, and Forest less than ten percent (Figure 4). Different families dominate the lists of largest families in each Biome, with only Asteraceae and Lamiaceae being a top five family in all three Biomes (Supplementary Material 4A). Similarly, the top five genera with endemics per Biome are well partitioned, although Aloe occurs in the top five of each Biome and Helichrysum occurs in two (Supplementary Material 4B).
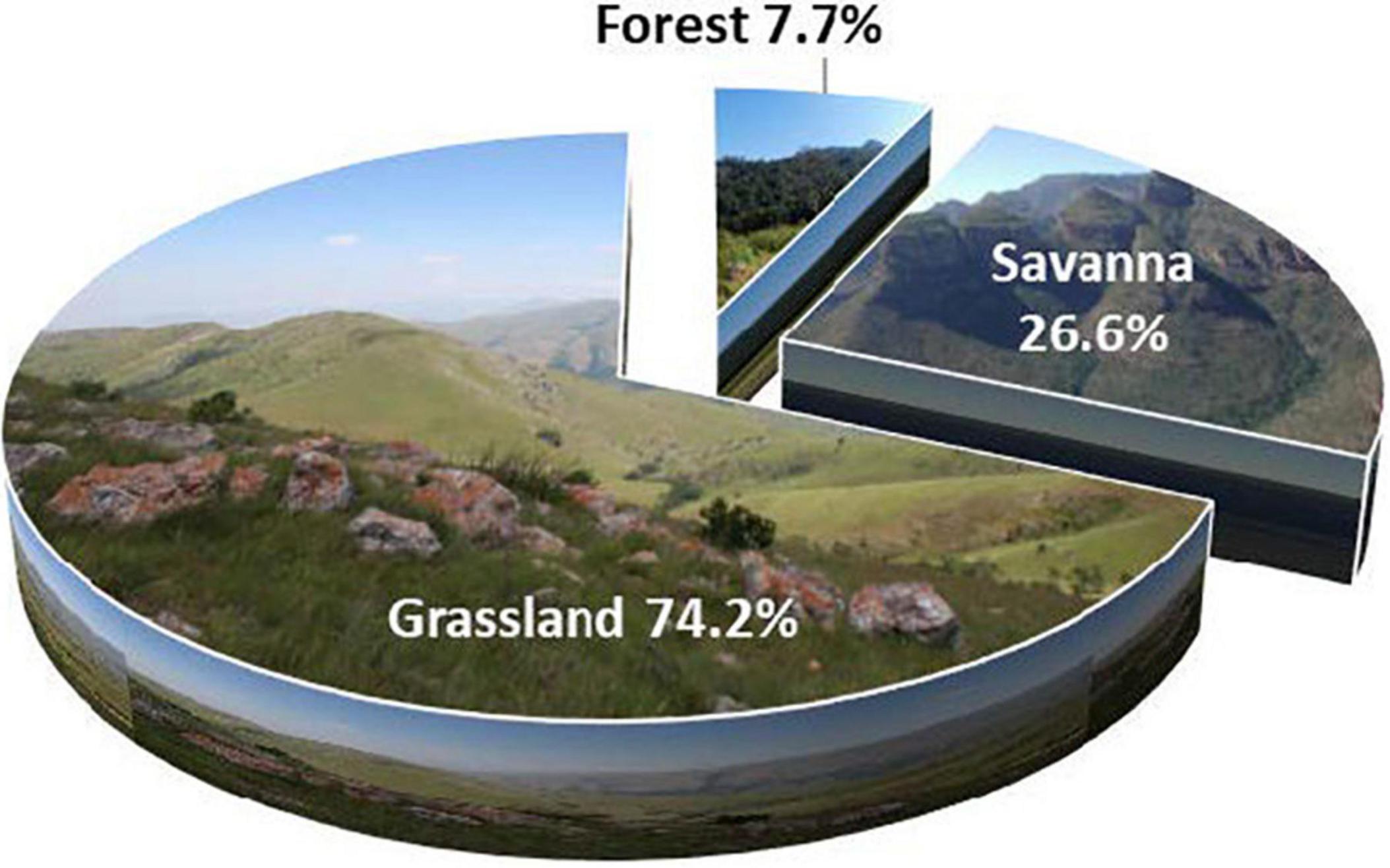
Figure 4. Partitioning of endemic plant taxa per Biome (Grassland, Savanna, and Forest) in the Limpopo–Mpumalanga–Eswatini Escarpment (LMEE).
Life-Forms
Of the 16 life-form categories used, four life-forms together represent 62.5% of all endemics, each of the four having more than 10% of the endemic taxa (Figure 5 and Supplementary Plates 2, 3). Caespitose Hemicryptophytes are the most numerous life-form, characterized by genera such as Helichrysum, which is a typical Grassland genus in the LMEE (Supplementary Material 4B). The second most numerous are bulbous Cryptophytes (geophytes), characterized by the rich petaloid monocotyledonous flora in the LMEE—represented in the top ten genera by Ledebouria, Gladiolus, and Disa; in the LMEE, these are typically Grassland and Savanna taxa (Supplementary Material 4B). Petaloid monocotyledons are also well represented in the LMEE by Iridaceae, Asparagaceae, and Orchidaceae—being in the overall top ten families (Figure 3A), also with a dominance of Grassland taxa, but with a strong Savanna component (Supplementary Material 4B). The third-largest group—woody Phanerophytes—are represented in the top 10 genera by Syncolostemon, Encephalartos, Searsia, and Protea, and again, many of these constitute Grassland rather than Savanna species (Supplementary Material 4B). Succulent Chamaephytes—the fourth-largest life-form—is best represented by Aloe, another primarily Grassland genus in the LMEE (Supplementary Material 4B).
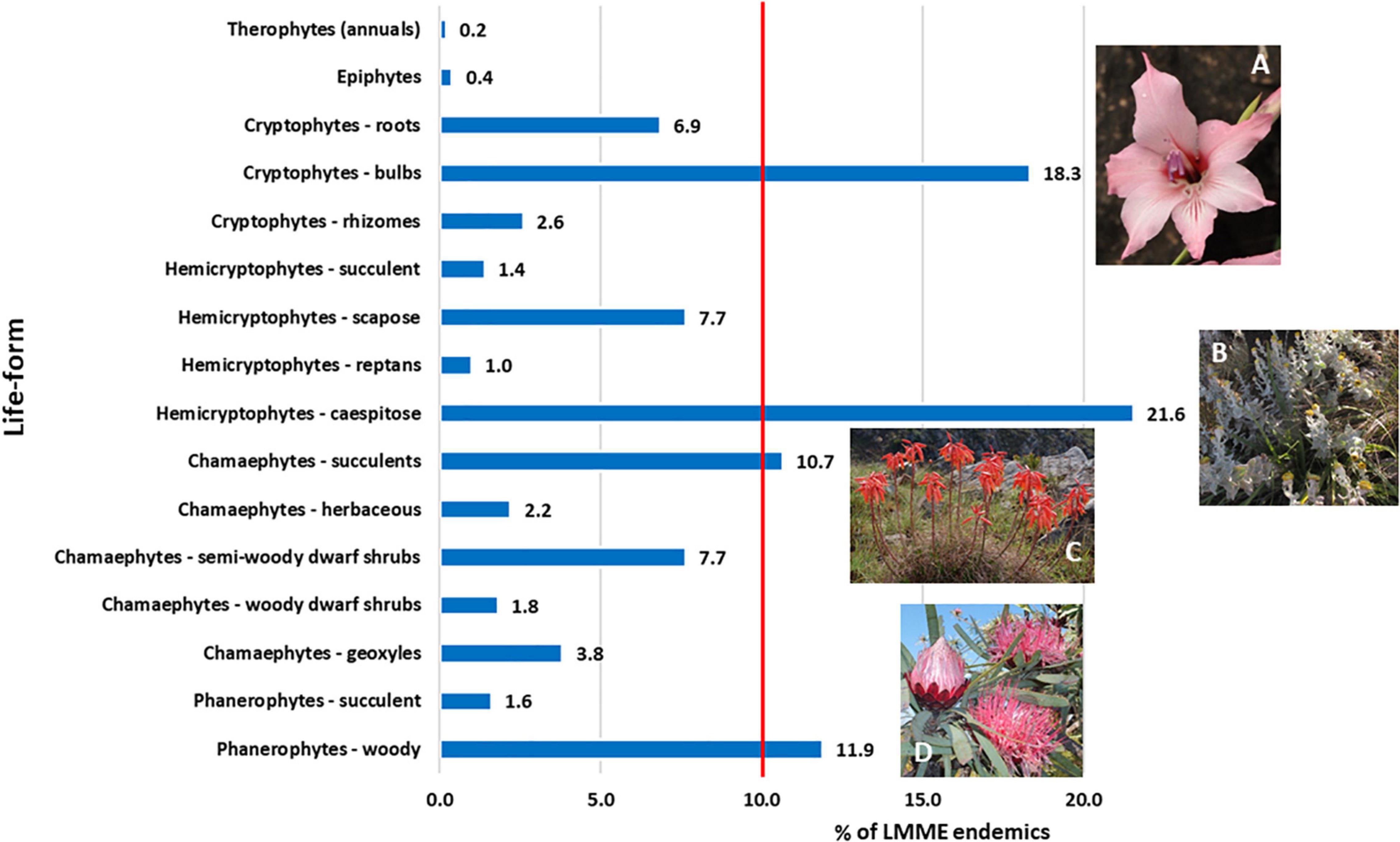
Figure 5. Life-form partitioning among Limpopo–Mpumalanga–Eswatini Escarpment (LMEE) endemic plant taxa. Life-form classes are based on a modified version of the Raunkiaer system (Raunkiaer, 1934); an additional category under chamaephytes was added, namely geoxyles: Geoxylic growth forms of woody subshrubs characterized by massive lignotubers, or underground woody axes, from which emerge aerial shoots which may be ephemeral given their misfit in the existing Raunkiaer system. (A) Gladiolus pavonia Goldblatt and J. C. Manning, an example of the abundant endemic bulbous cryptophytes in the LMEE; (B) Helichrysum lesliei Hilliard, a caespitose hemicryptophyte and an example of the dominant life-form endemic in the LMEE; (C) Aloe chortolirioides A. Berger is an example of a succulent chamaephyte in the LMEE; (D) woody phanerophytes such as Protea curvata N. E. Br. comprise a significant proportion of the LMEE endemic life-forms, many being endemic to the Grassland Biome. Credits: J. E. Burrows (A), B. C. Turpin (B), M. C. Lötter (C), D. Oosthuizen (D).
Level of Conservation Concern
We found that a total of 74.8% of the LMEE endemics have been assessed for conservation status, the remaining species being Data Deficient or Not Assessed (combined 25.2%). Currently, 191 endemic plant taxa are under some form of risk in the LMEE. The single largest group—almost a third—is Least Concern (Figure 6A). However, the combined total of those with a threat status from Near-Threatened to Extinct is more than a third of the endemics (38.5%), with almost a quarter in this threat bundle being Vulnerable (Figure 6A). It may also be that the number of taxa in the threat bundle may increase further, once the Data Deficient and Not Assessed taxa are assessed (particularly the novel taxa still to be described, many of which are narrow endemics). The Rare category—usually local endemics without immediate threats—while below 10% is still very significant, and these species could easily join categories of higher concern in time through loss of habitat. Pachycarpus stelliceps (Apocynaceae; endemic to Eswatini) is a Grassland taxon that has not been re-discovered in the type locality area of Mbabane; its identity is doubtful and it might remain a taxonomic mystery, but it is currently recognized as the only Extinct taxon in the LMEE. The genus (and family) with the most threatened taxa in the LMEE is Encephalartos (Zamiaceae): of the nine endemics, two (Encephalartos brevifoliolatus, E. nubimontanus) are Extinct in the Wild, five are Critically Endangered, and two are Vulnerable.
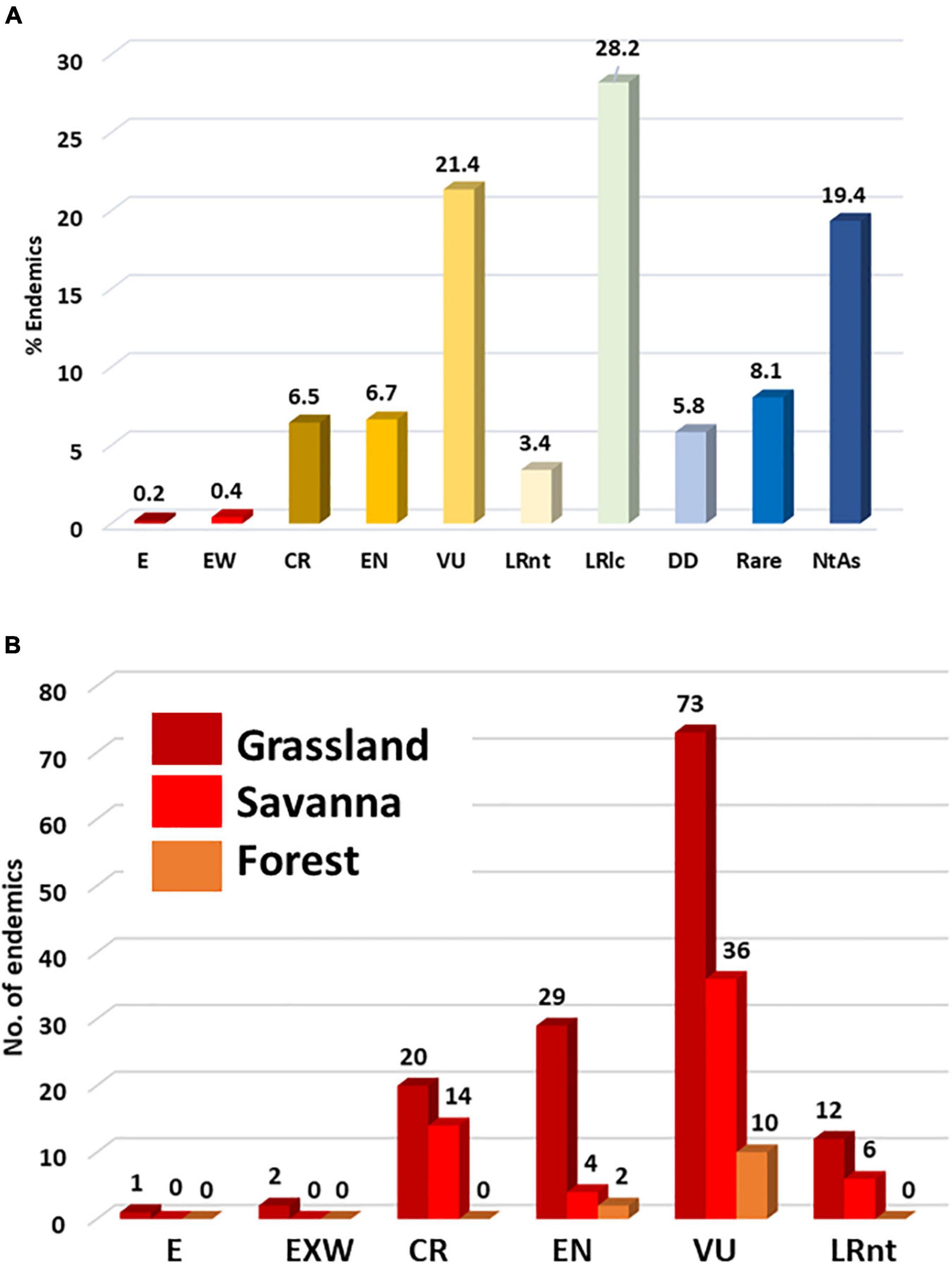
Figure 6. Distribution of endemic plant taxa in the Limpopo–Mpumalanga–Eswatini Escarpment (LMEE) according to (A) IUCN Red Data conservation status, and (B) according to those in the threat bundle (i.e., Lower Risk: Near Threatened to Extinct) per Biome. E, Extinct; EW, Exinct in the Wild; CR, Critically Endangered; VU, Vulnerable; LRnt, Lower Risk: near-threatened; LRlc, Lower Risk: least concern; DD, Data Deficient; NtAs, Not Assessed (Not Evaluated).
Proportion of conservation statuses per Biome again mirrors the dominance of Grassland by a very large margin (68.4%), followed by Savanna (24.5%), then Forest (7.1%). Taking just the threat bundle, Grassland has the most “at-risk” taxa in any of these categories (Figure 6B). While this may be simply a factor of area (a larger Grassland area will naturally host more endemics at risk than the other two Biomes), historically Grassland has seen the largest transformation in the LMEE. Much of this transformation can be attributed to afforestation for timber (Armstrong et al., 1998; Figure 2), which in 2020 accounted for 18.9% of the LMEE area (Supplementary Material 5). Although there was only an increase of 1.3% in total afforested area between 1990 and 2020 (Supplementary Material 5), the historical transformation of Grassland before 1990 represents a probably irreversible loss of a substantial amount of this Biome—with detrimental impacts on endemic flora and fauna (e.g., the Extinct Tetradactylus eastwoodae, Eastwood’s Long-tailed Seps lizard, in the heavily afforested Woodbush area; Bates et al., 2014). Coupled with other forms of transformation, an additional 3% of the LMEE that was previously Grassland was lost between 1990 and 2020 (Supplementary Material 5). Savanna experienced a loss of 3.4% of the area of the LMEE between 1990 and 2020 (likely due to increased mining and agricultural activities in that time—the latter being the biggest change in the LMEE over 30 years). It is worth noting that (indigenous) Forest expanded by 0.2% of the area of the LMEE in this time.
Only 10.4% of the LMEE is under formal conservation, with an additional 0.7% under stewardship conservation agreements (there is substantial overlap between the two categories in the Kruger-to-Canyons Biosphere Reserve). The distribution of PAs/conservation areas in the LMEE is scattered (Figure 7).
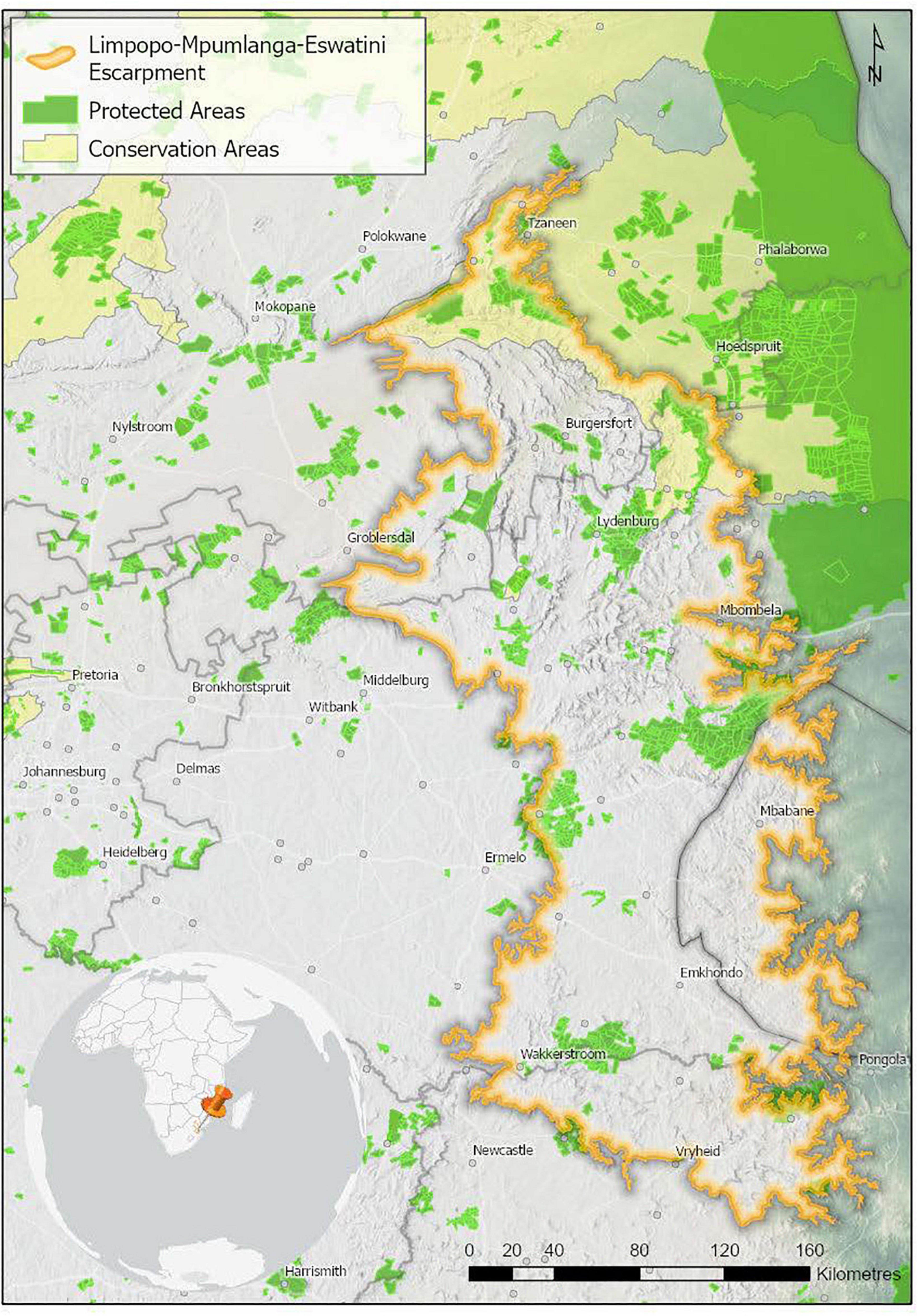
Figure 7. Protected and conservation areas in the Limpopo–Mpumalanga–Eswatini Escarpment (LMME) in 2020. Protected areas are recognized in terms of the National Environmental Management Protected Areas Act (Act No. 57 of 2003), and restrict certain kinds of developments that may have a negative impact. Conservation Areas are more loosely defined, and offer little protection against a change in land use. Data from Department of Forestry, Fisheries and the Environment [DFFE] (2021a, b).
The LMEE has areas of marginal increase in precipitation over 39 years, and areas of marginal decrease (Figure 8). These changes are not confined to any one Biome, with Grassland in the southern areas and on the Escarpment edge experiencing a decrease, while Grassland in the central-west an increase. Similarly, embedded Forest in these areas would experience the same effect as the surrounding Grasslands (positive or negative). Savanna, in contrast, seems to have experienced primarily a marginal increase in precipitation. As climate change may increase the risk of extinction for LMEE endemics, it is suggested that Grassland endemics localized to the south and extreme east of the LMEE are most at risk of climate change extinction, assuming that LMEE endemism is largely dependent on consistent summer moisture availability.
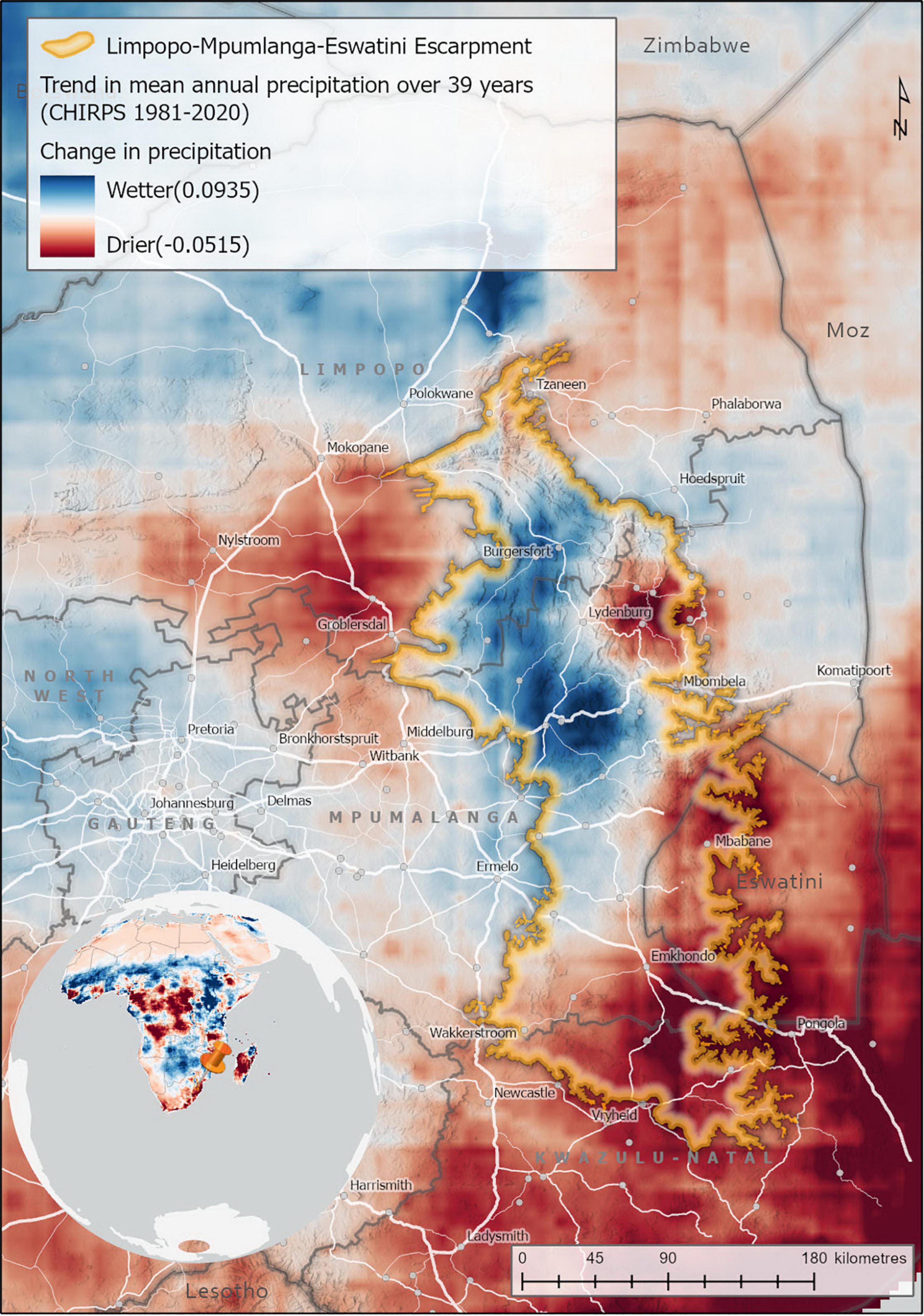
Figure 8. Trends in mean annual precipitation in the Limpopo–Mpumalanga–Eswatini Escarpment (LMME) (1981–2020). Trend analysis estimates the trend or rate of change in mean annual precipitation for each pixel over a 39-year period using a linear trend function. The precipitation data sets used was the Climate Hazards Group InfraRed Precipitation with Station (CHIRPS) data, measured annually between 1981 and 2020 (Funk et al., 2015).
Discussion
Definition of the Limpopo–Mpumalanga–Eswatini Escarpment
This work in the LMEE shows that it is possible to pragmatically delineate a section of Escarpment (mostly) objectively as a discrete orographic entity, and to define an endemic suite of species associated to it. The most similar example on the scale of our LMEE is Carbutt’s (2019) overlay of the Maloti-Drakensberg (i.e., discrete massif) as equal to his DMC—eliminating the original Drakensberg Alpine Center with its arbitrary 1,800 m lower contour (Van Wyk and Smith, 2001), but including a new alpine sub-center based on an isotherm and associated approximate elevation (2,800 m); there is thus a blend in the DMC between a physical (geomorphological) lower boundary (the base of the Maloti-Drakensberg), and an internal division based on an ecological indicator (an isotherm representing the lower bounds of the alpine zone). Other examples on smaller spatial scales are several of Van Wyk and Smith’s (2001) Centers: the Kamiesberg Center = Kamiesberg Escarpment (Namaqualand); the Hantam–Roggeveld Center = south-western Karoo Escarpment (later revised by Clark et al. (2011b) as both a physically and ecologically defined area); the Great Dyke Center (Zimbabwe) = a linear, igneous mega-intrusion, and the Chimanimani–Nyanga Center = (more or less) the Manica Highlands (Zimbabwe–Mozambique; currently being revised by Timberlake and Clark, in preparation). Similarly, Hahn; Hahn’s (2017; 2019) Soutpansberg Center = the Soutpansberg sensu lato (Limpopo Province); Van Staden et al.’s (2020) Griqualand West Center = three mountain ranges of differing geology; Clark et al.’s (2009) Sneeuberg Center = Sneeuberg mountains (Eastern Cape); and Clark et al.’s (2014) Great Winterberg–Amatholes (GWA; Eastern Cape), conservatively held back from being a potential Center, perhaps better as part of a revised larger Center including the Sneeuberg, Stormberg and GWA (the “Cape Midlands Center”—also an orographically defined concept; Clark et al., in preparation). What is significant in all of these is that the orographic definition precedes or is (nearly) equal to the endemic circumscription. From first principles of biogeography this is reasonable, as endemism is dependent on a pre-defined area (Anderson, 1994)—however, it should not be confused with the process of defining a Center of Endemism (Linder, 2001), which requires a different departure point based on an analysis of the spatial distribution of taxa (e.g., Van Wyk and Smith, 2001; Mucina and Rutherford, 2006). It is not always clear—however—in southern Africa’s Centers of Endemism as to which process has been followed, and the Center of Endemism concept is likely overdue for a complete reconsideration, redefinition, and re-analyses at a regional scale based on first principles. In this regard, we are cautious here and do not claim the LMEE as a Center of Endemism, or a replacement Center for the existing three Centeres associated to the LMEE. Nevertheless, it is generally agreed in biogeography that there is a high congruency between “mountains” and “endemism” (Noroozi et al., 2018), and mountains in southern Africa (both Cape and extra-Cape) are clearly important focal areas of endemism, and thus for biological conservation (Van Wyk and Smith, 2001; Mucina and Rutherford, 2006, etc.).
List of Endemics of the Limpopo–Mpumalanga–Eswatini Escarpment
The extra-Cape mountains in southern Africa have not benefited from the same enthusiasm for ongoing taxonomic discovery, inventory, and focus on narrow endemism at the same pace and capacity the Cape has. As a result, knowledge on absolute numbers of endemics—and enumeration thereof in the form of e.g., a Conspectus series—and a lack of the same level of sustained high research intensity, has limited an intimate knowledge of the drivers of speciation and patterns of endemism outside the Cape. The assumption has been that no-where else is as “special” floristically as the Cape, driving a positive feedback loop around ongoing intensive botanical work in the Cape. While the Cape region has the benefit of exceptional statistical power and analyses based on comprehensive plant distribution data, the extra-Cape region is somewhat behind on basic inventory and enumeration processes needed for more complex analyses. In the case of the LMEE, we provide the most comprehensive step toward rectifying this for one of the largest sections of Great Escarpment in the region, and certainly for what is probably the largest orographic entity in the summer rainfall region of southern Africa.
Various tallies of plant diversity and endemism in various parts of the LMEE have been published, mostly focusing on small sections of our LMEE. To date, Van Wyk and Smith’s (2001) three Centers (Barberton, Wolkberg, Sekhukhuneland) provided the most comprehensive estimate of endemic diversity, with an estimated combined endemic suite of c. 310 taxa. Our exhaustive literature and data mining, synthesis of all novel taxa, and intimate knowledge of the LMEE add another c. 186 taxa to this, and upend earlier estimates of local endemism. While the LMEE is still not in the same league as the Cape (nor can it be compared until the montane component of the Cape Flora is extracted), it ranks as one of the most significant summer rainfall sections of Escarpment for local endemism, and consequent conservation priorities.
Different families and genera dominate in different biomes in the LMEE, which suggests phylogenetic partitioning of endemics between Biomes, possibly with different evolutionary lineages and drivers of speciation—although one cannot rule out Biome transgression in local speciation processes (e.g., for Aloe and Helichrysum). Our results support the primacy of open habitats (here dominated by Grassland) rather than closed habitats (e.g., Forest) in southern African mountains as the most important habitat for endemic taxa, supporting the earlier findings of Matthews et al. (1993) and Van Wyk and Smith (2001).
Life-Forms Among the Endemics
The dominant life-forms among the endemics highlight the dominance of the Grassland Biome in the LMEE. The rich non-graminoid endemic flora mirrors that of other primary grassland areas in southern Africa (Carbutt and Edwards, 2006), and their irreplaceability for biodiversity conservation. Endemics were predominantly perennial herbaceous plants with seasonal shoot reduction due to frost, fire, or herbivory. Most of the endemics were expected to have this life-form, as the herbaceous flora of southern African grassy biomes is renowned for their resprouting ability in response to severe endogenous disturbances (Bombo et al., 2022). Noteworthy is that only four of these herbaceous endemics were graminoids and the rest were forbs.
Perennial herbaceous endemics were predominantly Cryptophytes and Hemicryptophytes. The largest group were Hemicryptophytes, which are characterized by a remnant shoot system with leaves that lie relatively flat on the ground. This group is globally the largest of the five major plant life-forms, and therefore this high endemism among Hemicryptophytes in the LMEE was predictable, as the group is well-adapted to open grassy ecosystems (Bond and Parr, 2010). Cryptophytes made up the second largest group of endemics—specifically geophytes that exhibit periodic reduction of the complete shoot system to storage organs that are imbedded in the soil and exhibit fire-stimulated flowering (Lamont and Downes, 2011). Bulbous geophytes are well represented throughout all the “open” Biomes of southern Africa, and are known for their endemism (Procheş et al., 2006); restricted range species would therefore be expected in the LMEE.
The well-represented endemic Phanerophytes comprise many species that are woody plants restricted to open grassy ecosystems rather than to forest (Matthews et al., 1993). These are fire-resistant/fire-tolerant small trees or shrubs (Hempson et al., 2014) that grow taller than 50 cm and have branches which do not die back periodically to that height limit, but which are often not more than 3 m tall in natural open habitat. These endemics are often scattered in the landscape and are typically associated with rocky outcrops and ridges (personal observations by the authors). The rich diversity of succulents in southern Africa also made a significant contribution to the LMEE endemic flora (Van Wyk and Smith, 2001): For example, endemic-richest genus Aloe are mostly succulent Chamaephytes that are prominent in both the Grassland and Savanna Biomes. Succulent Chamaephytes are tolerant of fire and frost and have mature shoot systems with succulent leaves that remain perennially 25–50 cm above the ground; even when growing taller, the shoots will die back periodically to that height limit due to endogenous disturbance (Pfab and Witkowski, 1999).
Distribution of Endemics Among Vegetation Types
Significantly, the exceptional endemism in the LMEE is driven by speciation in Grassland, most likely driven in response to herbivory, climate, and fire (Bond and Parr, 2010). This is significant—not because the value of Grassland for biodiversity is new knowledge in southern Africa—but because the fine-scale determination of endemism in an orographic context reveals (in the LMEE) to which extent this is true for southern African (montane) Grassland. In fact, ongoing fine-scale enumeration of endemism in the extra-Cape mountains may result in new areas of focal endemism that compete with the Cape for attention (such is the pattern emerging for the eastern Great Escarpment, of which the LMEE is the largest component). Similarly, the value of other open habitats (in this case Savanna) as a complementary endemic habitat to Grassland augment the value of the LMEE as an orographic entity. While Forest plays a less significant—but not unimportant (7.7% is a very significant proportion of endemics, considering the small area of Forest in the LMEE)—role in LMEE endemism, Forest in southern Africa typically hosts regional endemics rather than local endemics, and its value for endemism is at a regional rather than a local scale (Mucina et al., 2021).
Level of Conservation Concern
The most challenging conservation concerns in the LMEE are landscape transformation—especially conversion from mesic montane grassland to commercial forestry, i.e., plantations with alien timber (Kamffer, 2004); the rampant spread of alien invasive species; poaching of wild plant populations for horticulture and traditional uses (Emery et al., 2002); mining (particularly in Sekhukhuneland and in the south); and bush encroachment by indigenous woody species. Remaining Grassland in the LMEE should therefore be the primary focus of protection and conservation stewardship efforts (cf. Van Wyk and Smith, 2001).
Equally significant is that Grassland has not captured the public and policy imagination for conservation effort to the same extent as Forest (Van Wyk and Smith, 2001). As a result, open habitats in the LMEE are the least protected and the most transformed, resulting in a significant proportion of endemic taxa being at risk of extinction, with one species already Extinct and two Extinct in the Wild. Thus urgent focus on Grassland conservation is required in the LMEE. Coupled with this is the need for active trans-boundary co-operation with Eswatini. While there are numerous conservation areas “on paper” in the LMEE (i.e., the proportion of LMEE that is protected is encouraging), this is different to “effective conservation”—unfortunately many state-owned conservation areas are not receiving appropriate conservation management, as an example. Nor does it prevent continued harvesting of indigenous plants for various uses, including the extraction of cycads for the illegal market.
Future Research
While our treatment of LMEE endemics is considered the most comprehensive to date, the LMEE is rugged and difficult to access in many parts—notably the steep eastern scarp side; consequently—20 years after it was said by Van Wyk and Smith (2001)—there are still areas of the LMEE that remain unknown and warrant further careful exploration and biodiversity documentation (e.g., Von Staden, 2019 for the Thabakgolo Mountains). Such ongoing efforts are sure to yield additional novel taxa that will further increase the LMEE’s pole-position as the endemic-richest extra-Cape montane system in southern Africa. Determining the actual spatial patterns of the endemic taxa in the LMEE, and how these patterns affect the distinctiveness of each Center, would be a next logical step: For example, Van Wyk and Smith (2001) postulated that merging these into one Center might be warranted on further study, with 3–4 sub-centers.
Drivers of this phenomenal endemism in the LMEE have been explored in part by Van Wyk and Smith (2001) but need careful teasing out. However, they are likely a combination of the following: extensive surface area, lower latitude (sub-tropical), high edaphic diversity, great topographic heterogeneity (despite not having particularly high elevations compared to the DMC and CME), relatively high botanical sampling intensity from sustained intensive botanical exploration over c. 150 years, steep climatic gradients from east to west and north to south, and the LMEE likely acting as both a refugium through climatic cycles (preserving and accumulating palaeo-taxa) and a species pump (of neo-taxa—likely driven by sister speciation on different edaphic substrates).
As indicated by Van Wyk and Smith (2001) for the Wolkberg Center, it may be that there also is a misalignment between concentrations of endemics/taxa of higher conservation concern and PAs throughout the LMEE. A valuable exercise would be to determine the overlap between LMEE endemic taxa distributions and the PAs/conservation areas.
Conclusion
The Limpopo–Mpumalanga–Eswatini Escarpment (LMEE) is a definable and discrete orographic component of southern Africa’s Great Escarpment, and it is the largest component of the eastern Great Escarpment (i.e., between the Sneeuberg in the south and Nyanga in the north). With 496 endemic plant taxa, the LMEE has the highest plant endemism recorded for a mountain area in southern Africa to date—in terms of both absolute endemic numbers, and in terms of endemic richness (10.7%). The most similar montane system to the LMEE is the Maloti-Drakensberg (= Drakensberg Mountain Center), but the LMEE has almost double the number of endemic taxa, and a higher proportion endemism. In addition, botanical sampling intensity and intimate knowledge of the LMEE by the authors have played important roles in accurate and comprehensive listings of endemics, undoubtedly adding to the high tally. Grassland—and associated life-forms adapted to such open-habitats—is the most important habitat for endemics in the LMEE, from an evolutionary perspective and in terms of conservation concern.
Data Availability Statement
The original contributions presented in the study are included in the article/Supplementary Material, further inquiries can be directed to the corresponding author/s.
Author Contributions
VRC provided the first draft of endemics, wrote the text, and created the figures. JB, BT, ML, KB, and SS contributed conceptual ideas, developed the full list of endemics (Supplementary Material 3), undertook data analyses and interpreted the data, and contributed to revising the manuscript. ML generated the maps. BT generated the Supplementary Plates and Supplementary Table 1. SS generated the tables. The manuscript concept was developed by all authors.
Funding
Afromontane Research Unit (ARU), University of the Free State (South Africa), for a manuscript writing workshop at BNRH, and support for VRC; University of the Witwatersrand, Research Incentive Scheme for support of KB, and School of Biological Sciences, North-West University, for support of SS.
Conflict of Interest
The authors declare that the research was conducted in the absence of any commercial or financial relationships that could be construed as a potential conflict of interest.
Publisher’s Note
All claims expressed in this article are solely those of the authors and do not necessarily represent those of their affiliated organizations, or those of the publisher, the editors and the reviewers. Any product that may be evaluated in this article, or claim that may be made by its manufacturer, is not guaranteed or endorsed by the publisher.
Acknowledgments
We thank numerous experts for rapidly addressing our queries regarding the distribution ranges of LMEE endemics and for providing detailed information on undescribed endemic species: Andrew Hankey, Madeleen Struwig, Pieter Bester, Braam van Wyk, Delia Oosthuizen, Douglas McMurty, and Shane Burns. Linda Loffler and Kate Braun kindly provided input on some Eswatini endemics. We thank Buffelskloof Private Nature Reserve and Herbarium for use of facilities, including the reference library, and various staff at J, PRE, and PRU. We acknowledge the usage of the CHIRPS dataset from the Climate Hazards Group (http://chg.geog.ucsb.edu/data/chirps).
Supplementary Material
The Supplementary Material for this article can be found online at: https://www.frontiersin.org/articles/10.3389/fevo.2022.765854/full#supplementary-material
Supplementary Material 1 | References used for determining plant taxa endemic to the Limpopo Mpumalanga Eswatini Escarpment (LMEE).
Supplementary Material 2 | Plant taxa endemic to the Limpopo Mpumalanga Eswatini Escarpment (LMEE).
Supplementary Material 3 | Endemic plant taxa richness for selected southern African mountains (summer rainfall zone); LMEE, Limpopo Mpumalanga Eswatini Escarpment. Data sources are provided in Table 2 of the main text.
Supplementary Material 4 | The families (A) and genera (B) with the highest number of endemics in each Biome in the Limpopo Mpumalanga Eswatini Escarpment (LMEE), ranked in order (top five are bolded). The number of LMEE endemics is given in parentheses.
Supplementary Material 5 | Land-cover change in the Limpopo Mpumalanga Eswatini Escarpment (LMEE) in a 30-year period (1990–2020). Percentages are of the total LMEE area.
Supplementary Plate 1 | A selection of landscapes in the Limpopo–Mpumalanga–Eswatini Escarpment (LMME): (A) Blyde River Canyon; (B) summit of Mariepskop (1,945 m); (C) Leolo Mountains, Sekhukhuneland; (D) Malolotja Game Reserve, Eswatini; (E) Mount Anderson, near Lydenburg; (F) Afro-temperate Forest, Buffelskloof Nature Reserve, Lydenburg; (G) eastern Songimvelo Game Reserve, Barberton Mountains. Photographs: S. J. Siebert (A–D); M. C. Lötter (E); J. E. Burrows (F); K. Balkwill (G).
Supplementary Plate 2 | A selection of plant endemics found in the Limpopo–Mpumalanga–Eswatini Escarpment (LMME), Part 1: (A) Aloe chortolirioides var. woolliana, Angle Station, Barberton; (B) Brachystelma minor, Hermansburg, Barberton; (C) Brachystelma stellatum, Morgenzon, Pilgrims Rest; (D) Hesperantha brevicaulis, Buffelskloof Nature Reserve, Lydenburg; (E) Monopsis kowynensis, God’s Window; (F) Ochna barbertonensis, Bearded Man, Barberton. Photographs: M. C. Lötter (A–C); J. E. Burrows (D,F); B. C. Turpin (E).
Supplementary Plate 3 | A selection of plant endemics found in the Limpopo–Mpumalanga–Eswatini Escarpment (LMME), Part 2: (A) Pavetta glaucophylla, Abel Erasmus Pass; (B) Petopentia undulata, Penge, Olifants River Valley; (C) Tinnea barbata, Barberton Mountainlands; (D) Wurmbea burrowsii, Mount Anderson, Lydenburg; (E) Xerophyta purpurascens, Buffelskloof Nature Reserve, Lydenburg. Photographs: J. E. Burrows (A–C,E); K. Balkwill (D).
Footnotes
References
Armstrong, A. J., Benn, G., Bowland, A. E., Goodman, P. S., Johnson, D. N., Maddock, A. H., et al. (1998). Plantation forestry in South Africa and its impact on biodiversity. Sout. African For. J. 182, 59–65.
Balkwill, K., Williamson, S. D., Kidger, C. L., Robinson, E. R., Stalmans, M., and Balkwill, M.-J. (1997). “Diversity and conservation of serpentine sites in southern Mpumalanga (Eastern Transvaal), South Africa,” in The Ecology of Ultramafic and Metalliferous Areas. Documents Scientifiques et Techniques III, eds T. Jaffré, R. D. Reeves, T. Becquer, and N. Orstom (Nouméa: ORSTOM), 133–138.
Bates, M. F., Branch, W. R., Bauer, A. M., Burger, M., Marais, J., Alexander, G. J., et al. (2014). Atlas and Red List of the reptiles of South Africa, Lesotho, and Swaziland. Suricata 1. Pretoria: South African National Biodiversity Institute.
Bombo, A. B., Siebert, F., and Fidelis, A. (2022). Fire and herbivory shape belowground bud banks in a semi-arid African savanna. African J. Range Forage Sci. 39, 16–26. doi: 10.2989/10220119.2021.1982004
Bond, W. J., and Parr, C. L. (2010). Beyond the forest edge: ecology, diversity and conservation of the grassy biomes. Biol. Conserv. 143, 2395–2404.
Born, J., Linder, H. P., and Desmet, P. (2007). The Greater Cape Floristic Region. J. Biogeogr. 34, 147–162.
Burrows, J., and Willis, C. (2005). Plants of the Nyika Plateau. Southern African Botanical Diversity Report 31. Pretoria: SABONET.
Burtt Davy, J. (1926). A manual of flowering plants and ferns of the Transvaal with Swaziland, South Africa, Part I. London: Longmans, Green & Co.
Callisto, M., Solar, R., Silveira, F. A. O., Saito, V. S., Hughes, R. M., Fernandes, G. W., et al. (2019). A Humboldtian approach to mountain conservation and freshwater ecosystem services. Front. Environ. Sci. 7:195. doi: 10.3389/fenvs.2019.00195
Carbutt, C. (2019). The Drakensberg Mountain Centre: A necessary revision of southern Africa’s high-elevation centre of plant endemism. South African J. Bot. 124, 508–529.
Carbutt, C., and Edwards, T. J. (2006). The endemic and near-endemic angiosperms of the Drakensberg Alpine Centre. South African J. Bot. 72, 105–132. doi: 10.1016/j.sajb.2005.06.001
Clark, V. R., Barker, N. P., and Mucina, L. (2009). The Sneeuberg: a new centre of floristic endemism on the Great Escarpment. South Africa. South African J. Bot. 75, 196–238.
Clark, V. R., Barker, N. P., and Mucina, L. (2011a). The Great Escarpment of southern Africa: a new frontier for biodiversity exploration. Biodiver. Conserv. 20, 2543–2561.
Clark, V. R., Barker, N. P., and Mucina, L. (2011b). The Roggeveldberge – notes on a botanically hot area on a cold corner of the southern Great Escarpment. South Africa. S. Afr. J. Bot. 77, 112–126.
Clark, V. R., Dold, A. P., McMaster, C., McGregor, G., Bredenkamp, C., and Barker, N. P. (2014). Rich sister, poor cousin: Plant diversity and endemism in the Great Winterberg–Amatholes (Great Escarpment. Eastern Cape, South Africa). S. Afr. J. Bot. 92, 159–174.
Clark, V. R., Mucina, L., and Barker, N. P. (in preparation). The Cape Midlands Centre of Plant Endemism, Eastern Cape, South Africa.
Compton, R. H. (1976). The flora of Swaziland. J. S. Afr. Bot. Suppl. 11, 259–264. doi: 10.1016/s0378-8741(01)00381-6
Croizat, L. (1965). An introduction to the subgeneric classification of Euphorbia L., with stress on the South African and Malagasy species: I. Webbia 20, 573–706.
Crouch, N. R., Klopper, R. R., Burrows, J. E., and Burrows, S. (2011). Ferns of Southern Africa: a Comprehensive Guide. Cape Town: Struik.
De Wit, M. J., Furnes, H., and Robins, B. (2011). Geology and tectonostratigraphy of the Onverwacht Suite. Barberton Greenstone Belt, South Africa. Precambrian Res. 186, 1–27.
Deall, G. B., and Backer, A. P. (1989). The vegetation ecology of Eastern Transvaal Escarpment in the Sabie area. 3. Annotated checklist. Bothalia 19, 91–110.
Department of Forestry, Fisheries and the Environment [DFFE] (2021a). SAPAD – South Africa Protected Areas Database (SACAD_OR_2020_Q1). Available online at: https://egis.environment.gov.za/ (accessed August, 2021).
Department of Forestry, Fisheries and the Environment [DFFE] (2021b). SACAD – South Africa Conservation Areas Database (SAPAD_OR_2020_Q1). Available online at: http://www.egis.environment.gov.za/ (accessed August, 2021).
Dlamini, T. S., and Dlamini, G. M. (2002). “Swaziland,” in Southern African Plant Red Data Lists. Southern Africa Botanical Diversity Network Report, Vol. 14, ed. J. S. Golding (Pretoria: SABONET), 121–134.
Eken, G., Bennun, L., Brooks, T. M., Darwall, W., Fishpool, L. D. C., Foster, M., et al. (2004). Key Biodiversity Areas as Site Conservation Targets. BioScience 54, 1110–1118.
Emery, A. J., Lötter, M., and Williamson, S. D. (2002). Determining the conservation value of land in Mpumalanga. Nelspruit: Mpumalanga Parks Board.
Funk, C., Peterson, P., Landsfeld, M., Pedreros, D., Verdin, J., Shukla, S., et al. (2015). The climate hazards infrared precipitation with stations—a new environmental record for monitoring extremes. Scientific Data 2:150066. doi: 10.1038/sdata.2015.66
Geoterraimage (2021). 2020 South African National Land-Cover Dataset. Accuracy Assessment Report for the Department of Department of Forestry. South Africa: Fisheries and the Environment (DFFE).
Hahn, N. (2017). Endemic flora of the Soutpansberg, Blouberg and Makgabeng. S. Afr. J. Bot. 113, 324–336. doi: 10.1016/j.sajb.2017.09.006
Hahn, N. (2019). Indigenous vascular plants of the Soutpansberg, South Africa. Bothalia Afr. Biodiver. Conserv. 49:a2402. doi: 10.4102/abc.v49i1.2402
Hempson, G. P., Midgley, J. J., Lawes, M. J., Vickers, K. J., and Kruger, L. M. (2014). Comparing bark thickness: testing methods with bark–stem data from two South African fire-prone biomes. J. Veg. Sci. 25, 1247–1256.
Hock, R., Rasul, G., Adler, C., Cáceres, B., Gruber, S., Hirabayashi, Y., et al. (2019). “High mountain areas,” in IPCC Special Report on the Ocean and Cryosphere in a Changing Climate, eds H.-O. Pörtner, D. C. Roberts, V. Masson-Delmotte, P. Zhai, M. Tignor, E. Poloczanska, et al. (Cambridge: Cambridge University Press).
Hoveka, L. N., van der Bank, M., Bezeng, B. S., and Davies, T. J. (2020). Identifying biodiversity knowledge gaps for conserving South Africa’s endemic flora. Biodiver. Conserv. 29, 2803–2819. doi: 10.1007/s10531-020-01998-4
International Union for Conservation of Nature [IUCN] (2021). IUCN Red List of Threatened Species. https://www.iucn.org/resources/conservation-tools/iucn-red-list-threatened-species (accessed February, 2022).
Jenness, J., Brost, B., and Beier, P. (2013). Land Facet Corridor Designer: Extension for ArcGIS.Flagstaff: Jenness Enterprises
Kamffer, D. (2004). Community-level effects of fragmentation of the Afromontane grassland in the escarpment region of Mpumalanga, South Africa.[Ph.D thesis] Pretoria: University of Pretoria.
Klopper, R. R. (2021). South African National Plant Checklist statistics, version 1.2021. Pretoria: South African National Biodiversity Institute.
König, C., Weigelt, P., Schrader, J., Taylor, A., Kattge, J., and Kreft, H. (2019). Biodiversity data integration—the significance of data resolution and domain. PLoS Biol. 17:e3000183. doi: 10.1371/journal.pbio.3000183
Körner, C., Paulsen, J., and Spehn, E. M. (2011). A definition of mountains and their bioclimatic belts for global comparisons of biodiversity data. Alpine Bot. 121, 73–78. doi: 10.1007/s00035-011-0094-4
Lamont, B. B., and Downes, K. S. (2011). Fire-stimulated flowering among resprouters and geophytes in Australia and South Africa. Plant Ecol. 212, 2111–2125.
Linder, H. P. (2001). On areas of endemism, with an example from the African Restionaceae. System. Biol. 50, 892–912. doi: 10.1080/106351501753462867
Linol, B., and De Wit, M. J. (2016). Origin and Evolution of the Cape Mountains and Karoo Basin. Regional Geology Reviews. Berlin: Springer.
Loiseau, N., Mouquet, N., Casajus, N., Grenié, M., Guéguen, M., Maitner, B., et al. (2020). Global distribution and conservation status of ecologically rare mammal and bird species. Nat. Commun. 11:5071. doi: 10.1038/s41467-020-18779-w
Manes, S., Costello, M. J., Beckett, H., Debnath, A., Devenish-Nelson, E., Grey, K. A., et al. (2021). Endemism increases species’ climate change risk in areas of global biodiversity importance. Biol. Conserv. 257:109070. doi: 10.1016/j.biocon.2021.109070
Manning, J., and Goldblatt, P. (2012). Plants of the Greater Cape Floristic Region. 1: The Core Cape flora. Strelitzia 29. Pretoria: South African National Biodiversity Institute.
Matthews, W. S., Van Wyk, A. E., and Bredenkamp, G. J. (1993). Endemic flora of the north-eastern Transvaal Escarpment. Biol. Conser. 63, 83–94.
Merckx, V. S., Hendriks, K. P., Beentjes, K. K., Mennes, C. B., Becking, L. E., and Peijnenburg, K. T. (2015). Evolution of endemism on a young tropical mountain. Nature 524, 347–350. doi: 10.1038/nature14949
Moore, A., and Blenkinsop, T. (2006). Scarp retreat versus pinned drainage divide in the formation of the Drakensberg escarpment, southern Africa. S. Afr. J. Geol. 109, 599–610.
Morrey, D. R., Balkwill, K., and Balkwill, M.-J. (1989). Studies on serpentine flora: Preliminary analyses of soils and vegetation associated with serpentine rock formations in the south-eastern Transvaal. S. Afr. J. Bot. 55, 171–177.
Mucina, L., Lötter, M. C., Rutherford, M. C., van Niekerk, A., Macintyre, P. D., Tsakalos, J. L., et al. (2021). Forest biomes of Southern Africa. New Zealand J. Bot. 1–52. doi: 10.1080/0028825X.2021.1960383
Mucina, L., and Rutherford, M. C. (2006). The vegetation of South Africa, Lesotho and Swaziland. Strelitzia 19. Pretoria: South African National Biodiversity Institute.
Newbold, T., Hudson, L. N., Contu, S., Hill, S. L., Beck, J., Liu, Y., et al. (2018). Widespread winners and narrow-ranged losers: Land use homogenizes biodiversity in local assemblages worldwide. PLoS Biol. 16:e2006841. doi: 10.1371/journal.pbio.2006841
Noroozi, J., Talebi, A., Doostmohammadi, M., Rumpf, S. B., Linder, H. P., and Schneeweiss, G. M. (2018). Hotspots within a global biodiversity hotspot - areas of endemism are associated with high mountain ranges. Sci. Rep. 8:10345. doi: 10.1038/s41598-018-28504-9
Olivier, M., Davies, T. J., Burrows, J. E., Daru, B. H., Yessoufou, K., Muasya, A. M., et al. (2014). Savanna fire and the origins of the ‘underground forests’ of Africa. New Phytol. 204, 201–214. doi: 10.1111/nph.12936
Ollier, C. D. (1982). The Great Escarpment of eastern Australia: tectonic and geomorphic significance. J.Geol. Soc. Aus. 29, 13–23.
Partridge, T. C., and Maud, R. R. (1987). Geomorphic evolution of southern Africa since the Mesozoic. South African J. Geol. 90, 179–208.
Perrigo, A., Hoorn, C., and Antonelli, A. (2019). Why mountains matter for biodiversity. J. Biogeogr. 47, 315–325. doi: 10.1111/jbi.13731
Pfab, M. F., and Witkowski, E. T. F. (1999). Fire survival of the Critically Endangered succulent, Euphorbia clivicola RA Dyer – fire-avoider or fire-tolerant? Afr. J. Ecol. 37, 249–257.
Procheş, Ş, Cowling, R. M., Goldblatt, P., Manning, J. C., and Snijman, D. A. (2006). An overview of the Cape geophytes. Biol. J.Linnean Soc. 87, 27–43.
Raven, P. H., Gereau, R. E., Phillipson, P. B., Chatelain, C., Jenkins, C. N., and Ulloa Ulloa, C. (2020). The distribution of biodiversity richness in the tropics. Sci. Adv. 6:eabc6228. doi: 10.1126/sciadv.abc6228
Scott-Shaw, R. (1999). Rare and threatened plants of KwaZulu-Natal and neighbouring regions. Cascades: KwaZulu-Natal Nature Conservation Service.
Shrestha, N., Shen, X., and Wang, Z. (2019). Biodiversity hotspots are insufficient in capturing range-restricted species. Conserv. Sci. Pract. 1:e103. doi: 10.1111/csp2.103
Stafford, G. I., Wikkelsø, M. J., Nancke, L., and Jäger, A. K. (2016). The first phylogenetic hypothesis for the southern African endemic genus Tulbaghia (Amaryllidaceae: Allioideae) based on plastid and nuclear DNA sequences. Bot. J.Linnean Soc. 181, 156–170.
Store, R., and Kangas, J. (2001). Integrating spatial multi-criteria evaluation and expert knowledge for GIS-based habitat suitability modelling. Lands. Urban Plann. 55, 79–93.
Strugnell, A. M. (2002). Endemics of Mt. Mulanje. The Endemic Spermatophytes of Mt. Mulanje, Malawi. System. Geogr. Plants 72, 11–26.
Thompson, M. (2016). 1990 South African National Land-Cover Dataset. Data User Report and Metadata.Pretoria: Geoterraimage.
Thompson, M. (2021). South African National Land-Cover 2020 Accuracy Assessment Report. Pretoria: Geoterraimage
Timberlake, J., and Clark, V. R. (in preparation). First Quantification of Plant Endemics in the Manica Highlands (Zimbabwe–Mozambique), and Necessary Replacement of the Chimanimani–Nyanga Centre of Floristic Endemism with the Manica Highlands Centre.
U.S. Geological Survey’s Center for Earth Resources Observation and Science. (1996). 30 arc-second DEM of Africa. https://databasin.org/datasets/2965da954b114ff3b47621e99e3b29ba/ (accessed February, 2022).
Underwood, E., Taylor, K., and Tucker, G. (2018). The use of biodiversity data in spatial planning and impact assessment in Europe. Res. Ideas Outcomes 4:e28045. doi: 10.3897/rio.4.e28045
Van Staden, N., Siebert, S. J., Cilliers, D. P., Wilsenach, D., and Frisby, A. W. (2020). Floristic analysis of semi-arid mountain ecosystems of the Griqualand West Centre of plant endemism. Northern Cape, South Africa. Biodiversitas 21, 1989–2002. doi: 10.13057/biodiv/d210526
Van Wyk, A. E., and Smith, G. F. (2001). Regions of floristic endemism in Southern Africa. Hatfield: Umdaus Press.
Von Staden, L. (2019). ). Eumorphia davyi Bolus. National Assessment: Red List of South African Plants version 2020.1. available online at : http://redlist.sanbi.org/species.php?species=3000-2 (accessed August, 2021).
Vosa, C. G., Siebert, S. J., and van Wyk, A. E. (2011). Micromorphology and cytology of Prototulbaghia siebertii, with notes on its taxonomic significance. Bothalia 41, 311–314.
Weiss, A. (2001). “Topographic position and landforms analysis,” in ESRI User Conference. http://www.jennessent.com/arcview/TPI_Weiss_poster.htm (accessed February 2022).
Keywords: orographic, South Africa, Great Escarpment, conservation, grassland, savannah, forest, KwaZulu-Natal
Citation: Clark VR, Burrows JE, Turpin BC, Balkwill K, Lötter M and Siebert SJ (2022) The Limpopo–Mpumalanga–Eswatini Escarpment—Extra-Ordinary Endemic Plant Richness and Extinction Risk in a Summer Rainfall Montane Region of Southern Africa. Front. Ecol. Evol. 10:765854. doi: 10.3389/fevo.2022.765854
Received: 27 August 2021; Accepted: 02 May 2022;
Published: 03 June 2022.
Edited by:
Hang Sun, Kunming Institute of Botany (CAS), ChinaReviewed by:
Pedro Luiz Silva De Miranda, University of Liège, BelgiumRaymond Jansen, Tshwane University of Technology, South Africa
Copyright © 2022 Clark, Burrows, Turpin, Balkwill, Lötter and Siebert. This is an open-access article distributed under the terms of the Creative Commons Attribution License (CC BY). The use, distribution or reproduction in other forums is permitted, provided the original author(s) and the copyright owner(s) are credited and that the original publication in this journal is cited, in accordance with accepted academic practice. No use, distribution or reproduction is permitted which does not comply with these terms.
*Correspondence: Vincent Ralph Clark, Q2xhcmtWUkB1ZnMuYWMuemE=, dmluY2VudHJhbHBoLmNsYXJrQGdtYWlsLmNvbQ==