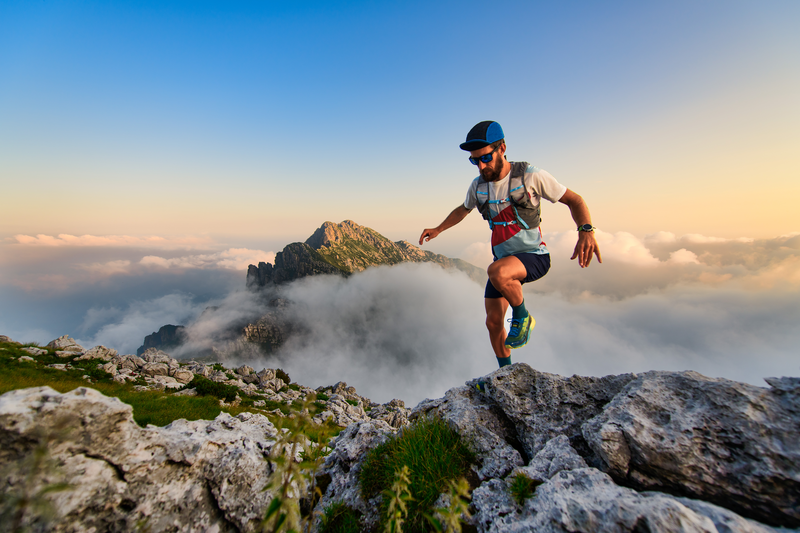
94% of researchers rate our articles as excellent or good
Learn more about the work of our research integrity team to safeguard the quality of each article we publish.
Find out more
ORIGINAL RESEARCH article
Front. Ecol. Evol. , 03 March 2022
Sec. Ecophysiology
Volume 10 - 2022 | https://doi.org/10.3389/fevo.2022.762146
This article is part of the Research Topic Ecophysiological Adaptations Associated with Animal Migration View all 15 articles
During migratory stopovers, birds must make decisions about when and where to travel and these decisions are likely contingent on their fuel stores, food availability, and antioxidant capacity as well as seasonal changes in key environmental factors. We conducted a field experiment on an offshore stopover site (Block Island, Rhode Island, United States: 41°130N, 71°330W) during autumn migration to test the hypothesis that birds with greater fuel stores and non-enzymatic antioxidant capacity have shorter stopovers than lean birds with low antioxidant capacity, and to determine the extent to which this depends on migration strategy. We used a 2 × 2 factorial field experiment (two levels each of available food and dietary polyphenols) with four species of songbirds kept in captivity for 3–5 days to produce experimental groups with different fuel stores and antioxidant capacity. We attached digital VHF transmitters to assess stopover duration and departure direction using automated telemetry. Non-enzymatic antioxidant capacity increased during refueling for Red-eyed Vireos (Vireo olivaceus) and Blackpoll Warblers (Setophaga striata) fed ad lib diets, and for ad lib fed Hermit Thrushes (Catharus guttatus) supplemented with polyphenols, but not for Yellow-rumped Warblers (Setophaga coronata coronata). Glutathione peroxidase (GPx) decreased during captivity and was influenced by dietary treatment only in Red-eyed Vireos. Oxidative damage decreased during captivity for all species except Yellow-rumped Warblers. Stopover duration was shorter for Vireos and Blackpolls fed ad lib as compared to those fed maintenance. Ad lib fed Hermit Thrushes supplemented with polyphenols had shorter stopovers than those fed ad lib, as did thrushes fed at maintenance and supplemented with polyphenols compared with those fed at maintenance alone. There was no influence of condition on stopover duration for Yellow-rumped Warblers. Departure direction was not strongly related to condition, and birds primarily reoriented north when departing Block Island. Thus, fat stores and oxidative status interacted to influence the time passerines spent on stopover, and condition-dependent departure decisions were related to a bird’s migration strategy. Therefore, seasonal variation in macro- and micro-nutrient resources available for refueling at stopover sites can affect body condition and antioxidant capacity and in turn influence the timing and success of migration.
Almost 19% of all extant bird species (∼1,855 species) undergo seasonal migrations, maximizing their fitness by taking advantage of spatially distinct habitats that vary in resources, environmental conditions, predation, parasites, or competition (Steadman, 2005; Somveille et al., 2013, 2015). Although some avian species are capable of traveling thousands of kilometers during a migratory flight (Gill et al., 2009; DeLuca et al., 2019), most migratory journeys, especially among passerines, are characterized by shorter flights punctuated by longer rest and refueling periods on stopover (Pomeroy et al., 2006; Seewagen and Guglielmo, 2010; Chernetsov, 2012; Covino et al., 2015). These stop-and-go migration strategies include alternately building and expending energy and nutrients at stopover and during flight, respectively (Cohen et al., 2012; Guglielmo, 2018; Schmaljohann and Klinner, 2020). The rate at which birds rebuild body condition likely influences the time they spend at a given stopover and may depend on available resources, and the migration ecology of a given species (Cohen et al., 2012; Toews et al., 2014; DeLuca et al., 2019; Packmor et al., 2020). Understanding how the behavioral decisions of migrants at stopover sites are influenced by their physiological condition and ecology requires field experiments that directly manipulate condition of individuals for multiple species at a given stopover site.
In general, birds stop during migration to rest and refuel prior to their next migratory flight, although doing so at a high rate may expose an individual to risk of predation (Sapir et al., 2004; Pomeroy et al., 2006; Woodworth et al., 2014) and may depend on the quality of the habitat (Smith and McWilliams, 2010; Oguchi et al., 2017; Clark and Seewagen, 2019). Prior research has highlighted how energetic condition can be a driving force behind departure decisions from stopover sites (Wikelski et al., 2003; Eikenaar et al., 2014; Smith and McWilliams, 2014; Deppe et al., 2015). Additionally, energetic condition and habitat quality can influence the direction a bird departs a stopover site (Deutschlander and Muheim, 2009; Covino and Holberton, 2011; Schmaljohann et al., 2011). For example, migrants with larger fat stores in the Gulf of Maine more regularly departed in a seasonally appropriate direction rather than departing on a temporary reverse migratory flight or a regional-scale movement (Covino and Holberton, 2011). However, the evidence for a relationship among fuel stores, time on a stopover site, and/or direction of subsequent flights is generally derived from an observation of the body condition of an individual migratory bird at capture and the amount of time it spends at that stopover after release (Seewagen and Guglielmo, 2010; Eikenaar et al., 2016b; Schmaljohann and Eikenaar, 2017). We know of only two studies to date that have directly manipulated fuel stores in migratory birds to examine how body condition affects stopover duration (Smith and McWilliams, 2014; Dossman et al., 2018). Hermit Thrush (Catharus guttatus) fed ad libitum (ad lib) and thus with large fat stores were twice as likely to depart a stopover site than lean birds fed a maintenance ration, and those fed ad lib were more likely to depart the stopover site in a seasonally appropriate direction (Smith and McWilliams, 2014). Experimentally food-restricted American Redstarts (Setophaga ruticilla) were four times less likely to depart a spring stopover site on a given night than paired controls (Dossman et al., 2018). Given the diversity of passerine species that pass-through a specific stopover site during migration (Baird et al., 1959; Parrish, 1997; Smith and Paton, 2011), additional experiments are needed that examine the impact of fuel stores on stopover behavior across multiple species that differ in migration and feeding strategies.
Stopover sites are likely also important for replenishing dietary antioxidant stores and recovering from oxidative damage caused during previous flights (Jenni-Eiermann et al., 2014; Skrip et al., 2015; Eikenaar et al., 2020). During migratory flights, birds have elevated metabolic rates and rely on stored fats as an efficient source of fuel (McWilliams et al., 2004), which may increase the production of reactive species (RS) and propagation of oxidative damage (Costantini, 2014; Skrip et al., 2015; McWilliams et al., 2020). Birds have evolved a multifaceted antioxidant system of endogenous molecules (non-enzymatic and enzymatic) and dietary-derived vitamins to combat or repair the damage created by RS (Cooper-Mullin and McWilliams, 2016). During fall migration, many passerines primarily find dietary antioxidants in the seasonally abundant fruit they rely on for refueling (Sapir et al., 2004; Catoni et al., 2008; Alan et al., 2013). For example, arrowwood (Viburnum spp.) fruit is more rapidly consumed than any other fruits on an island stopover site ca. 20 km off the coast of Rhode Island, United States (Block Island; 41°130N, 71°330W), and it has more fat, total lipophilic antioxidants, anthocyanins (a type of polyphenol) and other phenolics compared with other local fruits (Alan et al., 2013; Bolser et al., 2013). At that the same stopover site, circulating non-enzymatic antioxidant capacity was positively correlated with fat stores in Blackpoll Warblers (Setophaga striata) and Red-eyed Vireos (Vireo olivaceus, Skrip et al., 2015). In two different habitats in northern Columbia, Tennessee Warblers (Leiothlypis peregrina) refueling on insects and fruits during spring migration had higher fuel deposition rates than individuals refueling on insects alone (Bayly et al., 2019) and Garden Warblers (Sylvia borin) that were opportunistically resampled over several days at a Mediterranean coastal stopover site during spring migration increased circulating non-enzymatic antioxidant capacity while refueling (Costantini et al., 2007). However, the only study to examine an enzymatic antioxidant (glutathione peroxidase, GPx) during migration found that GPx concentration decreased throughout the day in European Robins (Erithacus rubecula) that were resting and refueling on stopover (Jenni-Eiermann et al., 2014), indicating that birds may downregulate endogenously produced antioxidants on stopover while increasing non-enzymatic antioxidant capacity from dietary (fruit-derived) antioxidants.
Birds also seem to recover from oxidative damage incurred during migratory flights while on stopover. Across individuals, oxidative damage was positively correlated with fat stores in Red-eyed Vireos and Blackpoll Warblers at a fall stopover site (Skrip et al., 2015), likely a result of consuming oxidatively vulnerable fats (Skrip and McWilliams, 2016). However, within individual Northern Wheatears (Oenanthe oenanthe) in the spring, oxidative damage to polyunsaturated fatty acids decreased over 3 days, indicating that birds can recover from the damage incurred during a previous flight (Eikenaar et al., 2020), although the contribution of dietary antioxidants from fruits to oxidative recovery is still unclear. Thus, the ability of a bird to forage effectively for dietary antioxidants, recover from oxidative damage, and to rebuild fat and antioxidant stores on a stopover site may influence behavioral decisions (e.g., length of stay on a stopover, direction of subsequent migratory flights) that affect overall timing and success of migration (McWilliams et al., 2021), but this has not been thoroughly studied.
Although many studies have focused on how amount of food affects fat stores and stopover behavior, no previous study has focused on how variation in dietary antioxidants could influence stopover behavior. We conducted a field experiment on an offshore stopover site in southern New England during autumn migration that involved manipulating the quantity of food as well as its quality (i.e., amount of antioxidants) offered to four sympatric species of migratory passerines (Table 1; Blackpoll Warbler, Setophaga striata; Red-eyed Vireo, Vireo olivaceus; Hermit Thrush, Catharus guttatus; Yellow-rumped Warbler, Myrtle subspecies, Setophaga coronata coronata). Individuals of each species were separated into two or four experimental groups and kept in captivity for 3–5 days to manipulate their underlying physiological condition. We then tracked these birds with miniature digital VHF transmitters using automated radio-telemetry stations (Taylor et al., 2017) to determine how condition (i.e., fat stores, oxidative status) affected the duration of stopover on the island and departure direction. We tested the following four hypotheses: (1) circulating antioxidant capacity changes with rest and refueling on stopover and the extent and direction depends on availability of dietary antioxidants and the component of antioxidant capacity measured (e.g., non-enzymatic vs. enzymatic), (2) oxidative damage decreases with rest and refueling on stopover but the extent likely depends on fat stores and availability of dietary antioxidants, (3) individuals that are able to build non-enzymatic antioxidant capacity and/or fuel stores (i.e., in better body condition) on stopover are able to depart more quickly and are less likely to exhibit reverse migration (sensu Smith and McWilliams, 2014), (4) species that migrate farther (Blackpoll Warblers and Vireos), or those that are more likely to undertake a long-distance flight (Blackpolls) exhibit a different degree of condition-dependent behavior while on stopover.
Table 1. Total migration distances and experimental treatment groups for Blackpoll Warblers, Red-eyed Vireos, Hermit Thrushes, and Yellow-rumped Warblers.
Block Island (41°130N, 71°330W) is an offshore stopover site located 15.5 km off the coast of Rhode Island, United States and 22.5 km northeast of Long Island, New York, United States (Figure 1). This 283 km2 island serves as a major stopover site for migratory birds in the fall (Reinert et al., 2002). Once on Block Island, migrating songbirds rest and refuel, typically consume large quantities of fruit while stopping over, and then depending on body condition continue migration to the south, or reorient back toward the mainland of Rhode Island (Able, 1977; Parrish, 1997; Bolser et al., 2013; Smith and McWilliams, 2014). Performing this experiment on an island also allowed us to be certain that we were accurately assessing an individual’s departure from the stopover site.
Figure 1. (A) Location of Block Island (41°130N, 71°330W), Rhode Island, United States with (B) the antennas of the two automated radio telemetry towers marked (NBI: northern Block Island and BISE: southeast Block Island). (C) Picture from the ground of a Hermit Thrush in the soft-release aviary that is entirely covered in canvas except for the bottom 1 m which was left open. Note that this was a re-enactment photo taken during the mid-day; in reality, birds were gently placed in this aviary 1.5–2.5 h after sunset and then were allowed to voluntarily depart, usually at dawn the next day (see section “Materials and Methods” for details). Photo by ©KarineAigner.
During a series of field experiments in fall 2015 and 2016, we manipulated the quality and quantity of food offered to short-term captive individuals of four passerine species and thus produced birds with different body condition prior to release to continue their fall migration (Table 1). We chose these four focal species because they are relatively common on Block Island during fall migration, yet they differ in migration strategy. Yellow-rumped Warblers are relatively short-distance migrants and they winter farther north than any other wood warbler species (Hunt and Flaspohler, 2020). Hermit Thrushes are medium-distance migrants that spend the winter in the southern United States and Central America (Smith and McWilliams, 2014; Dellinger et al., 2020). Red-eyed Vireos are long-distance neotropical migrants that typically follow eastern U.S. mountain ranges south until crossing or routing around the Gulf of Mexico to winter in northern South America (Callo et al., 2013; Deppe et al., 2015; Cimprich et al., 2018). All three of these species are presumably able to stopover many times after they depart Block Island (Callo et al., 2013; Skrip et al., 2015). In contrast, Blackpoll Warblers stage in New England during fall migration prior to a 3–5 day non-stop migratory flight over the western Atlantic ocean before reaching a wintering destination in the Caribbean and South America (DeLuca et al., 2015, 2019; Morris et al., 2016; Smetzer and King, 2020). Hatch year (HY) Red-eyed Vireos and Blackpoll Warblers exhibit prolonged stopovers (> 7 days) at coastal New England stopover sites (including Block Island) and so this fall stopover period could represent a crucial ecological bottleneck for these declining species (Smetzer et al., 2017; Smetzer and King, 2018). Although all four species are primarily insectivorous during the breeding season, they shift to a fruit-rich diet in late summer and into fall (Parrish, 1997; Mudrzynski and Norment, 2013; Woodworth et al., 2014; Marshall et al., 2016); however, only Yellow-rumped Warblers are able to consume and digest fruits from the waxy bayberry (Myrica spp.) (Place and Stiles, 1992; Afik et al., 1995; Parrish, 1997; McWilliams and Karasov, 2014) which is quite common on Block Island during fall and winter (Smith et al., 2007).
Mist nets were operated daily on Block Island from 60 min before dawn until sunset on all fair-weather days between September 1 and November 11, 2015 and 2016. When a target species was captured, we drew a up to 150 μL blood sample to measure antioxidant status and oxidative damage at capture (see below for details). Mist nets were monitored every 45–60 min and blood was drawn within 10 min of extraction for 75% of the birds and within 20 min of extraction for all birds (mean ± SE for all blood samples: 12.15 ± 1.49 min). All samples were centrifuged within 20 min of bleeding for 10 min at 5,000 rpm, and the separated plasma and red blood cells were flash frozen under liquid nitrogen and later stored at −80°C prior to analysis. After blood sampling, we measured subcutaneous fat score on a 0–8 scale (Kaiser, 1993), muscle score on a 0–3 scale (Bairlein et al., 1995), body mass (± 0.1 g), wing chord (± 0.5 mm) and tarsus (± 0.5 mm). However, we were unable to reliably determine sex for HY individuals of these four species captured during fall migration (Pyle, 1997).
Across the ca. 2-month fall season, we used cohort groups of 2 or 4 HY birds of the same species that were (a) captured within the same morning (1 h prior to sunrise to 6 h after sunrise), (b) of similar body size and weight for each species, and (c) all had fat score = 0 and muscle score = 0–1 at capture (see Table 2). For Yellow-rumped Warblers and Hermit Thrushes we had 4 birds per cohort (1 in each of the 4 treatment groups) and for Blackpolls and Vireos we had 2 birds per cohort (1 in each of 2 treatment groups). This careful selection of individuals that were captured on the same day in the same initial condition and of similar body size allowed us to maximize the chance these birds arrived on the same day on Block Island and allowed us to effectively control for any changes in response of individuals to the treatments across the fall season. This protocol produced up to eight cohorts of 2 or 4 individuals for each species over the fall migration season (Table 2).
Table 2. Target parameters used to select which individuals at capture could be included in each cohort per species and the measured values (mean ± SD) of these parameters, number of individuals included and the date of capture for each cohort.
We randomly assigned without replacement individuals within a cohort to one of the diet treatment groups (two diet groups for Blackpoll Warbler and Red-eyed Vireo, four diet groups for Hermit Thrush and Yellow-rumped Warbler). We manipulated food availability by providing individuals with ad lib food (ad lib treatment; Blackpoll Warblers: n = 8, Red-eyed Vireos: n = 8, Hermit Thrush: n = 16; Yellow-rumped warblers: n = 16) or restricting food availability to levels that allowed individuals to only maintain but not gain weight (maintenance treatment; Blackpoll Warbler: n = 8, Red-eyed Vireo: n = 8), Hermit Thrush: n = 16, Yellow-rumped warblers: n = 16). Ad lib treatment groups were provided with more live wax moth larvae (hereafter, waxworms, Galleria mellonela) than could be consumed per day. Maintenance groups were given enough waxworms to maintain an individual’s weight at capture as determined by preliminary studies (Hermit Thrush: 10 g per day; Blackpoll Warblers, Red-eyed Vireos, Yellow-rumped Warblers: 5 g per day). For Hermit Thrush and Yellow-rumped Warblers, we randomly selected half the ad lib and maintenance-fed birds to be supplemented each day with a dietary antioxidant. Specifically, these supplemented birds were gavaged once a day with 2.5 ccs elderberry powder (25 g/100 g anthocyanin and > 40 g/100 g other polyphenols: Artemis International, Inc., Fort Wayne, IN, United States) dissolved in water. This antioxidant concentration was chosen based on previous experiments with European blackcaps, Sylvia atricapilla (Catoni et al., 2008), and was equivalent to the amount of polyphenols wild birds would consume in berries in a day (Schaefer et al., 2008).
We housed birds for 3–6 days in standard stainless-steel cages (36 cm × 43 cm × 60 cm) in an outdoor aviary with protection from the elements (e.g., wind, sun, precipitation), but exposure to natural photoperiod and temperature fluctuations. Each day we monitored food intake, body weight, fat scores, and muscle scores. We aimed to release birds as soon as the ad lib treatment group reached a fat score of at least 6 (across all cohorts occurred in a max of 4 days), but had to delay release by 1 day for 3 of 32 cohorts due to inclement weather. On the day of release, we obtained a second blood sample of up to 150 μl to examine changes in antioxidant capacity and damage within individuals during captivity. We scaled the amount of blood drawn to the size of individuals so that the maximum amount of blood drawn (at capture plus before release) for each individual was less than 2% of their body weight which conforms to current guidelines (Fair et al., 2010; Owen, 2011).
On the day of release, we fit all birds with a digital VHF transmitter (Blackpoll Warblers, Red-eyed Vireos and Yellow-rumped Warblers: Avian NanoTag model NTQB-1; Hermit Thrush: Avian NanoTag model NTQB-2) attached with a leg-loop harness adjusted to body size (Naef-Daenzer, 2007). The combined weight of the tag and the harness was between 0.28 and 0.33 g (<3.0% body weight). Tags transmitted a coded burst at 166.380 MHz every 6.2 s with an expected battery life of about 40 days. We released individuals over time in up to eight cohorts with individuals in each cohort released on the same night. To ensure that the release location was standardized across cohorts and species, we constructed a “soft-release aviary” (following Smith and McWilliams, 2014) around a bayberry shrub (Myrica pensylvanica) that was 200 m from their experimental aviary and not visible from any dwelling. The soft-release aviary had a wooden frame (2.5 m tall × 1.5 m wide × 1.5 m long) with a heavy canvas covering with the bottom 1 m of the aviary left open to provide birds an exit when they voluntarily chose to do so (Figure 1). We placed birds in the soft-release aviary 1.5–2.5 h after sunset by removing them from their experimental aviary, placing them in a cloth bag for transport to the soft-release aviary (about a 10 min walk). We then, quietly and in the dark, removed each individual from the cloth bag and gently placed them on a branch in the aviary where they remained as we left the area. We visited the soft-release aviary within 30 min of sunrise and, therefore, we know that all birds left the aviary either during the night or within 30 min of sunrise next morning. Based on detections from the antenna facing the soft-release aviary compared to the other antennas on our local automated-telemetry tower (see below), we know that most birds exited the soft-release aviary during or within 30 min after sunrise. Only four of sixteen Blackpoll Warblers and two of sixteen Red-eyed Vireos departed the aviary during the night.
We used automated telemetry receiving stations in the Motus Wildlife Tracking System (Taylor et al., 2017) to determine how long individuals spent on stopover after release and their departure orientation. Importantly, two automated telemetry receivers were active on Block Island during Fall 2015 and Fall 2016 (Figure 1): we built a station near the north end of the island (NBI) and the U.S. Fish and Wildlife Service operated a station near the south end of the island (BISE). Both receiver stations consisted of six 9-element Yagi antennas mounted 12 m above the ground. All antennas monitored a frequency of 166.38 MHz continuously, allowing birds to be detected when within range (Crewe et al., 2019b). Departure was determined by visually inspecting signal strength in the minutes prior to the tag no longer being detected by either tower on Block Island, following Taylor et al. (2017). In this way a departure event could be distinguished from non-departure events such as an individual losing a tag or the tag becoming inoperable. Briefly, a bird’s departure from Block Island was estimated as the time at which a tag’s signal strength exhibited a peak (± 8 s), associated with a direct signal from a bird in flight, immediately followed by a rapid decline and loss of the signal as the bird moved out of antenna range (Mitchell et al., 2015; Taylor et al., 2017; Dossman et al., 2018). We were able to further verify that a bird departed the island by determining if it subsequently was detected by a Motus station not on the island. We only included in the analysis of stopover duration and departure direction those birds for which we could confirm departure in this way (n = 86).
We measured several indicators of antioxidant status and oxidative damage in blood of these birds including non-enzymatic antioxidant capacity (OXY), glutathione peroxidase (GPx, an important enzymatic antioxidant) (Costantini, 2011; Cooper-Mullin and McWilliams, 2016; Cooper-Mullin et al., 2019) and reactive oxygen metabolites (ROMs). Non-enzymatic antioxidant capacity was measured with the OXY-adsorbent test in plasma (5 μL per sample, concentration unit = mmol/L of HClO neutralized; Diacron International, Grosseto, Italy). OXY directly measures the ability of a plasma sample to quench the oxidant hypochlorous acid and provides an index of non-enzymatic antioxidant capacity, without being complicated by inclusion of uric acid (Costantini, 2011; Skrip and McWilliams, 2016). GPx activity in red blood cells was measured indirectly via a coupled reaction with glutathione reductase following the manufacturer’s protocol optimized for a small blood volume (1 μL per sample, concentration unit: nmol min–1 ml–1; Cayman Chemical glutathione peroxidase assay kit). Oxidized glutathione produced upon reduction of hydroperoxides by GPx is recycled to its reduced state by glutathione reductase and NADPH. The oxidation of NADPH to NADP + is accompanied by a decrease in absorbance at 340 nm. The rate of decrease in A340 is directly proportional to the GPx activity (Celi et al., 2013; Arazi et al., 2017). Oxidative damage was measured using the d-ROMs test (20 μL per sample, concentration unit = mmol/L H2O2 equivalents; Diacron International, Grosseto, Italy). This test works by first decreasing the pH of the plasma to release metal ions from proteins to cleave circulating ROMs through incubation with a solution of 0.01 M acetic acid/sodium acetate buffer. The subsequent products react with a chromogen (N,N-diethyl-p-phenylenediamine) which has a color intensity that is proportional to the concentration of ROMs in the plasma and was measured at 505 nm (Costantini, 2016, 2019). OXY and d-ROMs were measured in duplicate and GPx was measured in triplicate. We only included samples in the analysis if the coefficient of variation was less than 10% (85% of 288 plasma and red blood cell samples).
All analyses were done in R version 4.0.2 2021.1 We used an analysis of variance test (ANOVA) to determine the effect of diet treatments on change in weight, fat score and muscle score during captivity (individual measurement prior to release—measurement at capture) followed by one sample t-tests to determine whether this change in weight for maintenance birds of each species was significantly different from zero. For Blackpoll Warbler and Red-eyed Vireo cohorts, we used a one-way ANOVA with two diet treatments (ad lib, maintenance) whereas for Hermit Thrush and Yellow-rumped Warbler cohorts, we used a 2 × 2 factorial ANOVA with four diet treatments (ad lib or maintenance each with an antioxidant supplemented or not supplemented group).
For each species, we used linear mixed models (nlme package)2 with a fixed effect of treatment group and random effects for cohort and the number of days each bird spent in captivity to examine their effects on how each of the three oxidative parameters changed under each treatment condition: plasma non-enzymatic antioxidant capacity (OXY), GPx activity in red blood cells and plasma oxidative damage (d-ROMs). We analyzed the change in each oxidative measure during captivity (i.e., value prior to release minus that at capture) rather than a one-time measurement on the day of release. Between-individual variation in baseline (capture) measurements of oxidative status can be substantial because of a variety of uncontrolled factors including an individual’s diet at its previous stopover site or the type of fat an individual burned during their previous flight. Further, circulating antioxidants may be affected by the amount of circulating oxidative damage or the oxidative status of various tissues. Therefore, the change in oxidative status within an individual during captivity provided us a more robust assessment of the effect of each diet treatment. Cohort was included as a random effect for slope to control for change over the season or environmental conditions during captivity.
We used a linear mixed model with fixed effects for species and treatment group (two diet groups for Blackpoll Warbler and Red-eyed Vireo, four diet groups for Hermit Thrush and Yellow-rumped Warbler) and a random effect of cohort to examine their effects on stopover duration across all species. We also used separate linear or generalized mixed effects models for each species to investigate the effect of the treatment group and change in each oxidative parameter (measurement prior to release—measurement at capture) on stopover duration. Random factors that did not explain any variation in the respective dependent variable were removed from the final model. If no random effect explained any variation, we fit a normal or generalized linear model instead (Supplementary Table 1). For all tests, we checked whether data met the assumptions of homogeneity of variance, visually inspected residual plots, and did not find any noticeable deviations from homoscedasticity or normality. We evaluated the fixed effects in each model with likelihood ratio tests and Satterthwaite approximation for degrees of freedom.
We visually inspected plots of signal strength over time from each of the six antennas on each of the two towers to determine when and in which direction each individual departed Block Island. We calculated vanishing bearings for all individuals following the method outlined by Crewe et al. (2019a). We further categorized each vanishing bearing into two categories: “onward migration” to the south and “reverse migration” toward the mainland of Rhode Island. Across all species as well as within each species, we used a mixed effects model with a random effect of cohort to evaluate whether treatment (ad lib or maintenance) affected departure direction.
Individual birds spent a median of 3 days in captivity prior to release (Table 2, range = 2–6 days for the four species). Change in body mass was influenced by treatment group for Red-eyed Vireos and Blackpoll Warblers [Figure 2, F(1, 29) = 37.03, P < 0.001]. Change in body mass was also influenced by treatment group for Hermit Thrushes and Yellow-rumped Warblers [Figure 2, F(3, 56) = 19.55, P < 0.001], although post-hoc tests revealed that these changes were affected by food availability and not antioxidant treatment (Tukey HSD; Ad lib—Ad Lib + Dietary Antioxidants: p = 0.89, 95% CI = -0.53 to 1.43; Maintenance—Maintenance + Dietary Antioxidants: p = 0.99, 95% CI = 0.10–2.00). More specifically, birds fed at maintenance levels did not significantly change body mass during captivity as prescribed (Figure 2, Blackpoll Warbler: t = 5.4, P = 0.23, Red-eyed Vireo: t = 0.57, P = 0.59, Hermit Thrush: t = 1.26, P = 0.25, Yellow-rumped Warbler: t = 1.54, P = 0.17), whereas birds fed ad lib significantly increased body mass during captivity (Figure 2, Blackpoll Warbler: t = 5.44, P < 0.001; Red-eyed Vireo: t = 9.17, P < 0.001; Hermit Thrush: t = 7.49, P < 0.001; Yellow-rumped Warbler: t = 6.36, P < 0.001). Birds fed at maintenance levels did not significantly change fat or muscle score during captivity, whereas birds fed ad lib increased fat score but not muscle score during captivity (Supplementary Figure 1).
Figure 2. Change in body mass (g) during the ca. 3 days in captivity for Blackpoll Warblers and Red-eyed Vireos given ad lib food (gold with diagonal stripes) or maintenance amounts of food (light blue with diagonal stripes), and Hermit Thrushes and Yellow-rumped Warblers given ad lib food without and with supplemental antioxidants (gold with diagonal stripes and solid dark red, respectively) or maintenance amounts of food without and with supplemental antioxidants (light blue with diagonal stripes and solid dark blue, respectively). Boxes represent 95% confidence intervals; whiskers represent 1.5 times the interquartile range and outliers are represented by black dots. Labels indicate samples sizes for each species.
Changes in the three measured components of the antioxidant system (i.e., OXY, GPx, d-ROMs) in response to diet treatment are presented for each cohort for each of the four species (Figures 3, 4). Such a presentation emphasizes one of the strengths of the experimental design (i.e., the careful and consistent selection of individuals that were captured on the same day in the same initial condition and of similar body size), it is consistent with the statistical analyses that included cohort as a random effect, and it provides insights into how the different components of the antioxidant system (i.e., OXY, GPx, d-ROMs) changed within and across individuals. Reported in the text here are the treatment effects from each model (Supplementary Table 1). Non-enzymatic antioxidant capacity (OXY) increased during captivity for Blackpolls fed ad lib compared with Blackpolls fed at maintenance levels (Supplementary Table 1, t = −92.50, P = 0.007, n = 15) as well as for Red-eyed Vireos fed ad lib vs. maintenance levels (Supplementary Table 1, t = −71.67, P = 0.007, n = 14). GPx activity generally decreased during captivity for Blackpolls and we detected no difference between ad lib vs. maintenance-fed birds (Supplementary Table 1, t = 5.01, P = 0.84, n = 10). For Red-eyed Vireos, GPx activity decreased during captivity for four of the six ad lib-fed cohorts and for two of the three maintenance-fed cohorts (Figure 3D). On average, maintenance-fed birds decreased GPx activity more than ad lib-fed Vireos (Supplementary Table 1, t = −242.60, P = 0.02, n = 15). Oxidative damage generally decreased during captivity for both species, although there was no consistent difference in how oxidative damage changed among the treatment groups for Blackpolls (Figure 3E and Supplementary Table 1, t = 0.99, df = 9, P = 0.35, n = 14) or Red-eyed Vireos (Figure 3F and Supplementary Table 1, t = 1.56, P = 0.21, n = 15).
Figure 3. (A,B) Change in plasma non-enzymatic antioxidant capacity (OXY), (C,D) erythrocyte glutathione peroxidase activity (GPx), and (E,F) plasma oxidative damage (dROMs) for each individual fed ad lib (gold circles) or at maintenance levels (light blue diamonds) during captivity by cohort. Cohorts caught later in the season have higher numbers. Each dot represents an individual and the line indicates the magnitude of change during captivity (sample at release—sample at capture). Labels indicate samples sizes for each oxidative measurement per species.
Figure 4. (A,B) Change in plasma non-enzymatic antioxidant capacity (OXY), (C,D) erythrocyte glutathione peroxidase activity (GPx), and (E,F) plasma oxidative damage (dROMs) for each individual fed ad lib without supplemental polyphenols (light red) or with supplemental polyphenols (dark red), or at maintenance levels without supplemental polyphenols (light blue), or with supplemental polyphenols (dark blue) during captivity by cohort. Cohorts caught later in the season have higher numbers. Each dot represents an individual and the line indicates the magnitude of change during captivity (sample at release—sample at capture). Labels indicate samples sizes for each species.
OXY increased during captivity for almost all Hermit Thrushes and increased more for the thrushes given ad lib food with dietary AO (Figure 4A and Supplementary Table 1, ad lib + dietary AO: t = 4.18, P < 0.001, ad lib alone: t = 1.56, P = 0.13, maintenance + dietary AO: t = 1.69, P = 0.10; n = 27). In contrast, OXY did not significantly change in Yellow-rumped Warblers during captivity for any of the dietary treatments (Figure 4B and Supplementary Table 1, ad lib + dietary AO: t = 1.03, P = 0.31; ad lib alone: t = −0.11, P = 0.92; maintenance + AO: t = −0.38, P = 0.71). Most (25 out of 32) Hermit Thrush decreased GPx activity during captivity. However, change in GPx activity during captivity among Hermit Thrush was not consistently different between treatment groups (Figure 4C and Supplementary Table 1, ad lib + dietary AO: t = 1.40, P = 0.17, ad lib alone: t = 5.92, P = 0.91, maintenance + dietary AO: t = −0.33, P = 0.74; n = 27). GPx also generally decreased during captivity for Yellow-rumped Warblers and was not significantly different among the treatment groups (Figure 4D and Supplementary Table 1, F3 = 2.41, P = 0.09, n = 26). Oxidative damage significantly increased only for thrushes fed ad lib food with dietary AO (Figure 4E and Supplementary Table 1, ad lib + dietary AO: t = 2.43, P = 0.02; ad lib alone: t = 1.74, P = 0.083; maintenance + AO: t = 1.59; P = 0.11). Oxidative damage did not significantly change in any of the treatment groups for Yellow-rumped Warblers [Figure 4F and Supplementary Table 1, F(3, 20) = 0.16, P = 0.92, n = 26].
Blackpoll Warblers fed at maintenance levels and that were lean (Figure 2 and Supplementary Figure 1) departed Block Island later than their ad lib fed counterparts [Figure 5, stopover duration was 17.38 ± 8.16 vs. 2.32 ± 2.44 days, respectively; F(1, 3) = 86.43, P < 0.001]. Similarly, Red-eyed Vireos fed at maintenance levels and that were lean (Figure 2 and Supplementary Figure 1) departed Block Island later than their ad lib fed counterparts [Figure 5, stopover duration was 12.81 ± 7.16 vs. 3.24 ± 3.71 days, respectively; F(1, 4) = 4.82, P = 0.02]. Blackpoll Warblers fed at maintenance levels and Red-eyed Vireos fed at maintenance or ad libitum levels that were able to increase OXY more during captivity departed sooner than birds with lower OXY [Figure 6; F(1, 3) = 23.81, P = 0.02 and F(1, 6) = 6.21, P = 0.03, respectively]. When we re-ran the analysis without the maintenance-fed Blackpoll Warbler that remained on Block Island for 32 days, Blackpoll Warblers that were able to increase OXY during captivity across both treatment groups were able to depart Block Island sooner than those individuals that were not able to increase their non-enzymatic antioxidant capacity [F(1, 11) = 5.36, P = 0.04]. Change in OXY during captivity was not associated with stopover length in Hermit Thrush [F(1, 20) = 1.61, P = 0.22] or Yellow-rumped Warblers [χ2 = 0.07, P = 0.79]. Hermit Thrushes fed at maintenance levels had longer stopovers than those fed ad lib (Figure 5, t = −6.60, P < 0.001), and those fed at maintenance levels with supplemental AO had shorter stopover lengths than those fed at maintenance levels without supplemental AO (maintenance + AO: 9.72 ± 3.96; maintenance: 16.27 ± 3.85). Thrushes fed an ad lib diet supplemented with dietary AO had the shortest stopover lengths (ad lib + AO: 5.81 ± 0.82; ad lib: 6.78 ± 0.88). There was no effect of diet treatment on the number of days Yellow-rumped Warblers spent on stopover (maintenance: 17.97 ± 10.07, maintenance + dietary AO: 18.61 ± 6.83, ad lib: 13.45 ± 10.35, ad lib + dietary AO: 18.54 ± 6.00, χ2 = 0.53, P = 0.47). Across species, Red-eyed Vireos fed at maintenance levels had shorter stopover durations than Blackpoll Warblers fed at maintenance levels (P = 0.02), and Yellow-rumped Warblers had on average the longest stopover durations (P = 0.04).
Figure 5. Stopover duration (days on Block Island after release from captivity) for individuals fed ad lib without supplemental polyphenols (gold circles) or with supplemental polyphenols (dark red triangles), or at maintenance levels without supplemental polyphenols (light blue diamonds), or with supplemental polyphenols (dark blue stars). “***” indicates that P < 0.001.
Figure 6. Relationship between non-enzymatic antioxidant capacity during captivity (blood sample prior to release—blood sample at capture) and days spent on stopover after release for (A) Blackpoll Warblers fed ad lib (gold circles) or at maintenance levels (light blue diamonds). (B) Red-eyed Vireos fed ad lib (gold circles) or at maintenance levels (light blue diamonds). There were no significant relationships for Hermit Thrush or Yellow-rumped Warblers (not shown, see text for details).
We were able to obtain vanishing bearings for 14 Blackpoll Warblers, 14 Red-eyed Vireos, 31 Hermit Thrushes, and 27 Yellow-rumped Warblers (Figure 7). Departure direction was not influenced by feeding treatment group (χ2 = 0.33, P = 0.56), and the majority of birds departed in a reverse migratory direction (reverse migration: χ2 = 11.51, P < 0.001). Specifically, nine (75%) Blackpoll Warblers, 8 (67%) Red-eyed Vireos, 20 (69%) Hermit Thrushes, and 15 (68%) Yellow-rumped Warblers departed Block Island heading back to the mainland of Rhode Island.
Figure 7. Departure directions for fall-migrating Blackpoll Warblers (green), Hermit Thrushes (blue), Red-eyed Vireos (pink), and Yellow-rumped Warblers (yellow) fed ad lib or at maintenance levels. Departure decisions (shaded directions are onward, unshaded directions are reverse) were not influenced by feeding treatment, and the majority of birds departed in a reverse migratory direction to the north from their stopover on Block Island, RI, United States.
Our short-term field experiments with four species of free-living, migrating songbirds revealed that for 3 of the 4 species included, birds with enhanced fat stores and non-enzymatic antioxidant capacity departed sooner from a stopover site, and that the extent of this condition-dependent behavior was related to a species migratory strategy. Specifically, hatch-year Hermit Thrush, Red-eyed Vireos, and Blackpoll Warblers fed ad lib for several days in captivity had more substantial fuel stores and non-enzymatic antioxidant capacity and departed from an offshore stopover site at least two times faster than maintenance-fed, leaner birds. A similar response was not observed for Yellow-rumped Warblers, a shorter-distance migrant. We also found that songbirds decreased enzymatic antioxidant capacity during rest and recovery on stopover. These results indicate that an individual’s fat stores as well as antioxidant capacity can act as endogenous cues to continue migration.
Hypothesis 1: Circulating antioxidant capacity changes with rest and refueling on stopover and the extent and direction depends on availability of dietary antioxidants and the component of antioxidant capacity measured
During short-term captivity, ad lib-fed Blackpoll Warblers and Red-eyed Vireos increased their fat stores and non-enzymatic antioxidant capacity more than individuals of the same species fed at maintenance levels, indicating that food availability on stopover allows individuals to increase non-enzymatic antioxidant capacity. Although the exact mechanism is unknown, it is likely that increased food availability on stopover leads to higher levels of non-enzymatic antioxidant capacity either directly via dietary antioxidants (DeMoranville et al., 2021; McWilliams et al., 2021) or through upregulation of endogenous antioxidants to combat an increase in RS production from lipid peroxidation (Skrip and McWilliams, 2016). These results confirm previous work from Block Island that found fat stores were positively correlated with non-enzymatic antioxidant capacity in free-living Red-eyed Vireos and Blackpoll Warblers (Skrip et al., 2015). In addition, fasting-refeeding trials in captive Northern Wheatears indicated that fueling boosted total non-enzymatic antioxidant capacity (as measured by ferric reducing antioxidant power), although those results were primarily attributed to increased circulating uric acid (Eikenaar et al., 2016a). Since the OXY-adsorbent test excludes uric acid, our results indicate that Blackpoll Warblers and Red-eyed Vireos were able to increase other important circulating non-enzymatic antioxidants (e.g., other sacrificial molecules, precursors for enzymatic antioxidants) while building fat stores (Tsahar et al., 2006; Costantini, 2011; Carro et al., 2012; Skrip and McWilliams, 2016).
When we provided short-term captive Hermit Thrushes with supplementary hydrophilic antioxidants (i.e., daily doses of polyphenols), all birds increased OXY during captivity, and those fed ad lib and provided polyphenols were able to increase OXY more than any other treatment group. This indicates that hydrophilic antioxidants, such as those abundant in most fruits on Block Island and elsewhere (Schaefer, 2011; Alan et al., 2013; Bolser et al., 2013), are able to directly influence an individual’s non-enzymatic antioxidant capacity. Interestingly, Blackpolls and Vireos given an ad lib diet were also able to increase their non-enzymatic antioxidant capacity above those birds fed at maintenance levels and without supplementary dietary antioxidants. Since waxworms can have up to 69.2 mg/kg of vitamin E (Finke, 2015), it seems possible this lipophilic antioxidant was responsible for increased non-enzymatic antioxidant capacity for these ad lib fed birds. Hermit Thrushes fed at maintenance levels and supplemented with dietary antioxidants had comparable levels of non-enzymatic antioxidants to those fed ad lib and without additional antioxidants. This confirms that availability of hydrophilic antioxidants can increase non-enzymatic antioxidant capacity in migratory songbirds such as Hermit Thrushes. On stopover in the fall, birds primarily forage on locally abundant fruits, but not all fruits are high in both fat and antioxidant content (Benvenuti et al., 2004; Alan et al., 2013; Bolser et al., 2013; Schaefer et al., 2014). These results also suggest that consuming fruits with low fat content but sufficient antioxidants, such as Winterberry (Ilex verticillata; Alan et al., 2013), while on stopover may still allow birds to increase non-enzymatic antioxidant capacity, but in different ways. However, further research is needed on the absorption (Beaulieu and Schaefer, 2013; Skrip and McWilliams, 2016), possible microbial contribution (Laparra and Sanz, 2010; Saag et al., 2011), and the integration of dietary antioxidants into other physiological systems (Aboonabi and Singh, 2015; Hamilton et al., 2018; DeMoranville, 2020) in migratory birds for an adequate understanding of how dietary antioxidants improve antioxidant capacity.
In contrast, Yellow-rumped Warblers did not increase non-enzymatic antioxidant capacity even when supplemented with dietary antioxidants. There are three possible, non-mutually exclusive, explanations for why Yellow-rumped Warblers responded differently to our dietary treatments than Hermit Thrushes, Blackpoll Warblers, and Red-eyed Vireos. First, Yellow-rumped Warblers have specialized digestive enzymes that allow them to digest the waxy bayberry fruits (Place and Stiles, 1992; Afik et al., 1995). It is possible that this adaptation has changed how Yellow-rumped Warblers absorb and metabolize dietary antioxidants. Second, Yellow-rumped Warblers may rely more heavily on other aspects of their antioxidant system (e.g., enzymatic antioxidants) to combat damage during migration (Cooper-Mullin and McWilliams, 2016). Third, these warblers are shorter-distance migrants that winter relatively farther north than the other species in this study, and thus they may not need to increase non-enzymatic antioxidant capacity to the same extent as the other three species (Metzger and Bairlein, 2011). Therefore, the degree to which birds increase non-enzymatic antioxidant capacity while refueling may be linked to their migratory strategy, distance to migratory destination, and species-specific physiology.
For all four species, GPx activity decreased during short-term captivity indicating that birds downregulate enzymatic antioxidant capacity while resting on stopover. Further, change in GPx activity was unaffected by diet treatment, indicating that enzymatic antioxidants are physiologically regulated separately from non-enzymatic antioxidant capacity during stopover. These results are in line with the one other study that examined the effect of rest on circulating GPx activity during migration (Jenni-Eiermann et al., 2014). Free-living European Robins (Erithacus rubecula) caught while resting and refeeding at a stopover site exhibited the highest concentrations of GPx at dawn, presumably soon after setting down from a migratory flight, and GPx activity decreased throughout the day as robins rested and refueled regardless of fat score at capture (Jenni-Eiermann et al., 2014). Further, in a captive experiment, individual Zebra Finches rapidly upregulated GPx in response to acute exercise training yet returned to their baseline GPx activity by the next day (Cooper-Mullin et al., 2019). Therefore, it is likely that this enzymatic antioxidant acts as a first line of defense for responding to the oxidative challenges of flight, but that perhaps due to the ubiquitousness of GPx across body tissues (Halliwell and Gutteridge, 2007; Margis et al., 2008; Cooper-Mullin and McWilliams, 2016), maintaining high levels of circulating GPx is too costly during periods of rest when production of RS is expected to be low.
Hypothesis 2: Oxidative damage decreases with rest and refueling on stopover but the extent likely depends on fat stores and availability of dietary antioxidants
Although all birds fed ad lib in captivity increased fat stores, the relationship between fat and oxidative damage across species was varied. Blackpoll Warblers decreased oxidative damage during captivity, although those fed ad lib decreased oxidative damage less than those fed at maintenance levels. In contrast, oxidative damage decreased for Red-eyed Vireos during the few days in captivity regardless of treatment group. The only treatment group to notably increase oxidative damage during captivity was Hermit Thrushes given an ad lib diet supplemented with dietary antioxidants. It is possible that the incorporation of hydrophilic antioxidants from the diet interfered with the upregulation or circulation of lipophilic antioxidants to combat lipid damage (Skrip et al., 2016); clearly, more research on the integration of different aspects of the antioxidant system in migratory birds are needed. A decrease in circulating lipid hydroperoxides during captivity provides evidence that songbirds can recover from oxidative damage after flight, but it is important to note that our measure of oxidative damage may be the product of RS accumulated during flight, consumption of oxidatively-vulnerable fats during stopover, and the relative activity of repair mechanisms as well as the antioxidant system (Halliwell and Gutteridge, 2007; Cooper-Mullin and McWilliams, 2016). It is possible that increased levels of circulating fats prevented ad lib fed Blackpolls from reducing circulating oxidative damage as much as maintenance fed Blackpolls. Additionally, ad lib fed thrushes given dietary antioxidants increased ROMs, but also had the highest circulating levels of OXY. These results along with those reported by Skrip et al. (2015) and Eikenaar et al. (2016a) imply that circulating oxidative damage to lipids may decline as individuals build fat stores at stopover sites, but the extent depends on other ecologically relevant factors including diet quality as well as the intensity of the previous flight and the antioxidant system response.
Hypothesis 3: Individuals that are able to build non-enzymatic antioxidant capacity and/or fuel stores (i.e., in better body condition) on stopover are able to depart more quickly and are less likely to exhibit reverse migration
We experimentally demonstrated that Blackpoll Warblers, Red-eyed Vireos and Hermit Thrushes fed ad lib and thus with greater fuel stores departed an offshore stopover site more quickly than their maintenance-fed counterparts. These results generally agree with the other two single-species studies that have experimentally manipulated fuel stores of migrating songbirds on stopover sites (Smith and McWilliams, 2014; Dossman et al., 2018). Individuals on stopover with sufficient fuel stores are more likely to leave as soon as weather is favorable for migration (Schmaljohann et al., 2012; Smith and McWilliams, 2014). Past studies at other coastal stopover sites have shown that if birds lose energy stores or refuel at slower rates, as would occur at low-quality stopover sites, then the probability of departure from the stopover site was higher than that of birds refueling at higher rates (Schaub et al., 2008; Aamidor et al., 2011; Morganti et al., 2011; Schmaljohann et al., 2013; Paxton and Moore, 2017). Given that maintenance-fed Blackpoll Warblers, Red-eyed Vireos and Hermit Thrushes spent more time on Block Island than their ad lib-fed counterparts, presumably taking advantage of Block Island’s abundant fruiting plants for refueling, these results indicate that Block Island is apparently a higher quality stopover site.
Ours is the first study to demonstrate that non-enzymatic antioxidant capacity can directly influence stopover duration in long- and short-distance migratory songbirds. Hermit Thrushes that did not receive supplementary antioxidants had longer stopovers compared with those that consumed more dietary antioxidants. This effect of dietary antioxidants was most pronounced when birds were fed at maintenance levels indicating that availability of dietary antioxidants, independent of fat deposition, can influence stopover duration. Further, Red-eyed Vireos that were able to increase their non-enzymatic antioxidant capacity during captivity had shorter stopovers than those that did not, as did maintenance-fed Blackpoll Warblers. Therefore, increased non-enzymatic antioxidant capacity seems to provide migratory birds with an important cue that, in addition to fat stores, influences departure decisions from stopover sites, although more research on the mechanisms and molecular underpinnings of these signals is needed.
In contrast to the other three species, we saw no impact of diet quality and quantity on stopover duration of Yellow-rumped Warblers. Yellow-rumped Warblers are unusual compared to the other three focal species in that they have a widely available diet source (bayberry) on Block Island and other coastal stopover sites that only they can digest (Place and Stiles, 1992; Podlesak et al., 2005; Bolser et al., 2013). Thus, after release from our short-term captive experiments, regardless of diet treatment, Yellow-rumped Warblers may have been able to fuel relatively quickly, and this may essentially erase any short-term condition differences that we created with our diet quantity and quality manipulations. Yellow-rumped Warblers were also likely near the end of their migratory journey once they reach New England (Murray and Murray, 1979), although clear understanding of the migratory patterns among different populations of this species remains murky. Therefore, minimizing time on stopover may not be as important for Yellow-rumped Warblers as conserving energy (Hedenström and Alerstam, 1997) compared to the other three species.
If individuals that build high non-enzymatic antioxidant capacity can leave stopover faster, stopovers with fruits that are high in both fats and antioxidants represent extremely valuable resources for Neotropical and other frugivorous migrants (Alan et al., 2013; Bolser et al., 2013; Smith et al., 2013). There is evidence that birds may be able to distinguish the antioxidant content of fruits (Schaefer et al., 2008, 2014) and previous work on Block Island demonstrated that passerines preferentially consume fruits with higher fat and antioxidant content (e.g., arrowwood, Alan and McWilliams, 2013; Bolser et al., 2013). Such preferences for certain fruits have important ecological implications in that non-native or invasive plant species produce fruits that in general are less nutritious though abundant (Drummond, 2005; Mudrzynski and Norment, 2013; Smith et al., 2013; Smith and Hatch, 2017), although too little information is available on the antioxidants in fruits of non-native species.
For all four focal species in our study, the majority of individuals departed Block Island heading north and thus reoriented back to the mainland. Formative work by Able (1977) with Blackpoll Warblers on Block Island also demonstrated that birds departing stopover overwhelmingly reoriented back to the mainland. Further, for birds in our study, orientation of departure flight was not associated with condition. This contrasts with a previous experiment on Block Island that found Hermit Thrushes fed ad lib had a higher likelihood of continuing migration in a seasonally appropriate southerly direction (Smith and McWilliams, 2014). As fruit resources on Block Island can vary from year to year, as well as within a season, it is possible that the distribution of food on Block Island between years influenced Hermit Thrush departure orientation (Smith et al., 2007; Bolser et al., 2013; Smith and McWilliams, 2014).
In the Gulf of Maine, Blackpoll Warblers and Red-eyed Vireos often leave coastal stopovers by orienting inland (Brown and Taylor, 2017; Smetzer and King, 2018). However, those movements are often explained as birds fleeing poor refueling habitat at coastal sites (Mehlman et al., 2005; Woodworth et al., 2015). Since our experimental manipulations produced birds with more fat stores, and Block Island offers good habitat for refueling (Reinert et al., 2002; Smith and McWilliams, 2010; Skrip et al., 2015), there are likely other drivers of reorientation behavior for these species. Most birds that stopover on Block Island are hatch year individuals that presumably were displaced offshore on nights with strong northwest winds, so birds that reorient may be retracing their steps back to familiar habitat before continuing migration (Baird et al., 1959; Able, 1977; Åkesson et al., 1996; Mitchell et al., 2011). Although further research is necessary to determine whether individuals departing Block Island on a given night were exhibiting true migratory movement or were exhibiting extended stopover or landscape scale movement (Mills et al., 2011; Taylor et al., 2011; Brown and Taylor, 2017), initial departure direction was not influenced by condition.
Hypothesis 4: Species that migrate farther, or those that are more likely to undertake a long-distance flight exhibit a different degree of condition-dependent behavior while on stopover
Blackpoll Warblers, Red-eyed Vireos, and Hermit Thrushes fed at maintenance levels stayed on Block Island up to 6, 3, and 2 times longer, respectively, than those fed ad lib. However, Yellow-rumped Warblers had similar stopover durations regardless of treatment group. Therefore, departure decisions were condition-dependent for 3 out of the 4 species we studied, and the extent of condition-dependent effects on stopover duration may be related to differences in migratory strategies. For example, Red-eyed Vireos fed at maintenance levels departed from Block Island faster than maintenance-fed Blackpoll Warblers, possibly because Blackpoll Warblers face a greater geographical barrier (the ocean) upon departure from Block Island (DeLuca et al., 2015, 2019). Many Blackpoll Warblers initiate a trans-Atlantic crossing from New England and fly non-stop over the North Atlantic Ocean to the Caribbean or South America (Nisbet, 1970; DeLuca et al., 2015, 2019), a journey that requires at least 10–12 g of fat stores (Klaassen, 1996; Boal, 2014). Among Blackpolls measured at three coastal stopover sites and nine inland stopover sites during migration, individuals on Block Island were the heaviest and had the largest fat stores (Morris et al., 2016), emphasizing the importance of this stopover for Blackpolls. Additionally, the stopover duration measured in our experimental birds was within the range of other estimates of stopover for fall-migrating birds on Block Island that were simply captured, banded, and recaptured (Parrish, 2000). Thus, condition-dependent stopover behavior is common for migratory passerines (except Yellow-rumped Warblers) and there is variation in the extent to which condition may drive behavioral decisions that seems related to the migration strategy of the species.
Yellow-rumped Warblers had the longest stopovers of all species studied and may respond to fuel stores similarly to other medium-distance migrants. For example, there was no relationship between fuel deposition rate and departure fuel load in European Robins, a short-to-medium distance migrant, provided with ad lib mealworms at a stopover site in Sweden (Dänhardt and Lindström, 2001). Although Yellow-rumped Warblers and Hermit Thrush have similar migration travel distances, the wintering ecology of these species may shape time constraints on stopover (Kwit et al., 2004; Alvarado et al., 2014; Hunt and Flaspohler, 2020). Hermit Thrush often set up territories on their wintering grounds and individuals that arrive first choose the highest quality areas (Kwit et al., 2004). In contrast, Yellow-rumped Warblers usually forage together in flocks on the wintering grounds, a much less competitive lifestyle (Kwit et al., 2004). Therefore, Hermit Thrushes may be more time constrained on migration than Yellow-rumped Warblers.
Stopover sites (e.g., Block Island) are crucial for birds recovering from the physiological challenges of migratory flight (Ktitorov et al., 2010; Chernetsov, 2012; Smith et al., 2015) and here we demonstrated that the quality of resources available affects how long birds remain at a stopover site. This multi-species study provides direct experimental evidence for how the ability to rebuild fuel and antioxidant stores at a stopover can directly influence the pace of migration by influencing the time an individual spends at a stopover site, and how these responses vary across species with different migratory challenges. What remains to be determined is how variation in fruit species and availability across stopover sites may alter these decisions, how decisions at one stopover location may carryover to decisions at the next (Legagneux et al., 2012; McKinnon et al., 2015; Skrip et al., 2016). Also unknown is (1) exactly how absorption of dietary antioxidants and fats from fruits are affected by the physiological state of the organism (e.g., gut microbiome; Trevelline et al., 2019) or by other macro-and-micronutrients in the fruits and (2) how antioxidants consumed at a stopover site are integrated into other physiological systems (e.g., peroxisome proliferator-activated receptors, glucocorticoid production Hamilton et al., 2018; Casagrande et al., 2020). The results from our experiment can help inform future studies that examine whether the behavioral responses of these four species at one stopover site do in fact influence the overall pace and survival of birds during migration. Future studies should examine the carry-over effects of fueling and antioxidant status from one stopover site to the next, and how this relates to arrival times and behavior at wintering grounds.
The data underlying the results presented in the study are available from Dryad: https://doi.org/10.5061/dryad.9w0vt4bhh.
The animal study was reviewed and approved by University of Rhode Island Office of Research Integrity.
SM and CC-M: conceptualization, writing—review and editing, and visualization: CC-M: writing—original draft preparation. SM: funding acquisition, resources, and supervision. Both authors contributed to the article and approved the submitted version.
The authors were supported by the National Science Foundation (IOS-0748349) and the U.S. Department of Agriculture (RIAES-538748).
The authors declare that the research was conducted in the absence of any commercial or financial relationships that could be construed as a potential conflict of interest.
All claims expressed in this article are solely those of the authors and do not necessarily represent those of their affiliated organizations, or those of the publisher, the editors and the reviewers. Any product that may be evaluated in this article, or claim that may be made by its manufacturer, is not guaranteed or endorsed by the publisher.
We would like to thank Scott Comings, St. Clair Comings, Kim Gaffett and The Nature Conservancy for their support and contribution to our work on Block Island, as well as Steve Brenner and many other collaborators for endless fieldwork help. In particular, we would like to thank Olivia DaRugna for her constant and cheerful attitude while banding, taking wonderful care of our captive birds and her diligent waxworm counting skills. We are indebted to Pam Loring and Brett Still for advice and help while constructing our tower, and to Sue Smith and the rest of the Windhover family for allowing us to build and maintain the tower on their property. We are also very grateful for all the individuals who supported us through our experiment.com project. We would also like to thank Megan Skrip, Adam Smith, Jessica Bolser, and Rebecca Alan, who helped to make clear the importance of fruit as a source of fat and antioxidants for migrating songbirds.
The Supplementary Material for this article can be found online at: https://www.frontiersin.org/articles/10.3389/fevo.2022.762146/full#supplementary-material
Aamidor, S. E., Bauchinger, U., Mizrahy, O., McWilliams, S. R., and Pinshow, B. (2011). During stopover, migrating blackcaps adjust behavior and intake of food depending on the content of protein in their diets. Integr. Comp. Biol. 51, 385–393. doi: 10.1093/icb/icr054
Able, K. P. (1977). The orientation of passerine nocturnal migrants following offshore drift. Auk 94, 320–330. doi: 10.1093/auk/94.2.320
Aboonabi, A., and Singh, I. (2015). Chemopreventive role of anthocyanins in atherosclerosis via activation of Nrf2-ARE as an indicator and modulator of redox. Biomed. Pharmacother. 72, 30–36. doi: 10.1016/j.biopha.2015.03.008
Afik, D., Caviedes-Vidal, E., Martinez Del Rio, C., and Karasov, W. H. (1995). Dietary modulation of intestinal hydrolytic enzymes in yellow-rumped warblers. Am. Physiol. Soc. 269, R413–R420. doi: 10.1152/ajpregu.1995.269.2.r413
Åkesson, S., Karlsson, L., Walinder, G., and Alerstam, T. (1996). Bimodal orientation and the occurrence of temporary reverse bird migration during autumn in south Scandinavia. Behav. Ecol. Sociobiol. 38, 293–302. doi: 10.1007/s002650050245
Alan, R. R., and McWilliams, S. R. (2013). Oxidative stress, circulating antioxidants, and dietary preferences in songbirds. Comp. Biochem. Physiol. B, Biochem. Mol. Biol. 164, 185–193. doi: 10.1016/j.cbpb.2012.12.005
Alan, R. R., McWilliams, S. R., and McGraw, K. J. (2013). The importance of antioxidants for avian fruit selection during autumn migration. Wilson J. Ornithol. 125, 513–525. doi: 10.1676/13-014.1
Alvarado, A. H., Fuller, T. L., and Smith, T. B. (2014). Integrative tracking methods elucidate the evolutionary dynamics of a migratory divide. Ecol. Evol. 4, 3456–3469. doi: 10.1002/ece3.1205
Arazi, H., Mohammadjafari, H., and Asadi, A. (2017). Use of anabolic androgenic steroids produces greater oxidative stress responses to resistance exercise in strength-trained men. Toxicol. Rep. 4, 282–286. doi: 10.1016/j.toxrep.2017.05.005
Baird, J., Bagg, A. M., Nisbet, I. C. T., and Robbins, C. S. (1959). Report on mist-netting along the Atlantic Coast in 1958. Bird-Banding 30, 143–171.
Bairlein, F., Peach, W., and Spina, F. (1995). Manual of Field Methods. Wilhelmshaven: European Science Foundation.
Bayly, N. J., Rosenberg, K. V., Gómez, C., and Hobson, K. A. (2019). Habitat choice shapes the spring stopover behaviour of a Nearctic-Neotropical migratory songbird. J. Ornithol. 160, 377–388. doi: 10.1007/s10336-019-01624-3
Beaulieu, M., and Schaefer, H. M. (2013). Rethinking the role of dietary antioxidants through the lens of self-medication. Anim. Behav. 86, 17–24. doi: 10.1016/j.anbehav.2013.05.022
Benvenuti, S., Pellati, F., Melegari, M., and Bertelli, D. (2004). Polyphenols, anthocyanins, ascorbic acid, and radical scavenging activity of Rubus, Ribes, and Aronia. J. Food Sci. 69, 164–169.
Boal, C. W. (2014). Age-Ratios and Condition of En Route Migrant Blackpoll Warblers in the British Virgin Islands. Wilson J. Ornithol. 126, 568–574. doi: 10.1676/13-113.1
Bolser, J. A., Alan, R. R., Smith, A. D., Li, L., Seeram, N. P., and McWilliams, S. R. (2013). Birds select fruits with more anthocyanins during autumn migration. Wilson J. Ornithol. 125, 97–108.
Brown, J. M., and Taylor, P. D. (2017). Migratory blackpoll warblers (Setophaga striata) make regional-scale movements that are not oriented toward their migratory goal during fall. Mov. Ecol. 5, 1–13. doi: 10.1186/s40462-017-0106-0
Callo, P. A., Morton, E. S., and Stutchbury, B. J. M. (2013). Prolonged spring migration in the Red-eyed Vireo (Vireo olivaceus). Auk 130, 240–246. doi: 10.1525/auk.2013.12213
Carro, M. D., Settle, T., and Klandorf, H. (2012). “The role of uric acid in the avian species,” in Uric Acid, eds S. Castillo and E. Maldonado (Hauppauge: Nova Science Publishers, Inc), 2–30.
Casagrande, S., DeMoranville, K. J., Trost, L., Pierce, B., Bryła, A., Dzialo, M., et al. (2020). Dietary antioxidants attenuate the endocrine stress response during long-duration flight of a migratory bird. Proc. R. Soc. B Biol. Sci. 287:20200744. doi: 10.1098/rspb.2020.0744
Catoni, C., Schaefer, H. M., and Peters, A. (2008). Fruit for health: the effect of flavonoids on humoral immune response and food selection in a frugivorous bird. Funct. Ecol. 22, 649–654. doi: 10.1111/j.1365-2435.2008.01400.x
Celi, P., Selle, P. H., and Cowieson, A. J. (2013). “The effects of dietary supplementation with different organic selenium sources on oxidative stress in broilers,” in 24th Annual Australian Poultry Science Symposium, (Sydney, New South Wales: Poultry Research Foundation), 76–83.
Chernetsov, N. (2012). “Fuel deposition rate and energy efficiency of stopovers,” in Passerine Migration, ed. N. Chernetsov (Berlin: Heidelberg: Springer Berlin Heidelberg), 37–57. doi: 10.1007/978-3-642-29020-6_3
Cimprich, D. A., Moore, F. R., and Guilfoyle, M. P. (2018). “Red-eyed Vireo (Vireo olivaceus),” in The Birds of North America Online, ed. P. G. Rodewald (Ithaca, NY, USA: Cornell Lab of Ornithology), doi: 10.2173/bna.reevir1.01.1
Clark, R. E., and Seewagen, C. L. (2019). Invasive Japanese Barberry, Berberis thunbergii (Ranunculales: Berberidaceae) is associated with simplified brach-dwelling and leaf-littler arthropod communities in a New York forest. Environ. Entomol. 48, 1071–1078. doi: 10.1093/ee/nvz095
Cohen, E. B., Moore, F. R., and Fischer, R. A. (2012). Experimental evidence for the interplay of exogenous and endogenous factors on the movement ecology of a migrating songbird. PLoS One 7:e41818. doi: 10.1371/journal.pone.0041818
Cooper-Mullin, C., Carter, W. A., and McWilliams, S. R. (2019). Acute effects of intense exercise on the antioxidant system in birds: does exercise training help? J. Exp. Biol. 222:jeb210443. doi: 10.1242/jeb.210443
Cooper-Mullin, C., and McWilliams, S. R. (2016). The role of the antioxidant system during intense endurance exercise: lessons from migrating birds. J. Exp. Biol. 219, 3684–3695. doi: 10.1242/jeb.123992
Costantini, D. (2011). On the measurement of circulating antioxidant capacity and the nightmare of uric acid. Methods Ecol. Evol. 2, 321–325. doi: 10.1111/j.2041-210X.2010.00080.x
Costantini, D. (2014). Oxidative stress and hormesis in evolutionary ecology and physiology: A marriage between mechanistic and evolutionary approaches. Heidelberg, New York, Dordrecht, London: Springer-Verlag, doi: 10.1007/978-3-642-54663-1
Costantini, D. (2016). Oxidative stress ecology and the d-ROMs test: facts, misfacts and an appraisal of a decade’s work. Behav. Ecol. Sociobiol. 70, 809–820. doi: 10.1007/s00265-016-2091-5
Costantini, D. (2019). Understanding diversity in oxidative status and oxidative stress: the opportunities and challenges ahead. J. Exp. Biol. 222:jeb194688. doi: 10.1242/jeb.194688
Costantini, D., Cardinale, M., and Carere, C. (2007). Oxidative damage and anti-oxidant capacity in two migratory bird species at a stop-over site. Comp. Biochem. Physiol. C Toxicol. Pharmacol. 144, 363–371. doi: 10.1016/j.cbpc.2006.11.005
Covino, K. M., and Holberton, R. L. (2011). The influence of energetic condition on flight initiation and orientation of migratory songbirds in the Gulf of Maine region. Auk 128, 313–320. doi: 10.1525/auk.2011.09225
Covino, K. M., Holberton, R. L., and Morris, S. R. (2015). Factors influencing migratory decisions made by songbirds on spring stopover. J. Avian Biol. 46, 73–80. doi: 10.1111/jav.00463
Crewe, T. L., Deakin, J. E., Beauchamp, A. T., and Morbey, Y. E. (2019b). Detection range of songbirds using a stopover site by automated radio-telemetry. J. Field Ornithol. 90, 176–189. doi: 10.1111/jofo.12291
Crewe, T. L., Crysler, Z., and Taylor, P. D. (2019a). Motus R Book: a walk through the use of R for Motus automated radio-telemetry data. Port Rowan, ON: Bird Studies Canada.
Dänhardt, J., and Lindström, Å (2001). Optimal departure decisions of songbirds from an experimental stopover site and the significance of weather. Anim. Behav. 62, 235–243. doi: 10.1006/anbe.2001.1749
Dellinger, R., Wood, P. B., Jones, P. W., and Donovan, T. M. (2020). “Hermit Thrush (Catharus guttatus),” in Birds of the World, ed. A. F. Poole (Ithaca: Cornell Lab of Ornithology), doi: 10.2173/bow.herthr.01
DeLuca, W. V., Woodworth, B. K., Mackenzie, S. A., Newman, A. E. M., Cooke, H. A., Phillips, L. M., et al. (2019). A boreal songbird’s 20,000 km migration across North America and the Atlantic Ocean. Ecology 100:e02651. doi: 10.1002/ecy.2651
DeLuca, W. V., Woodworth, B. K., Rimmer, C. C., Marra, P. P., Taylor, P. D., McFarland, K. P., et al. (2015). Transoceanic migration by a 12 g songbird. Biol. Lett. 11, 1–4. doi: 10.1098/rsbl.2014.1045
DeMoranville, K. J. (2020). The Frequent Flyer’s Guide to Migration: How Songbird Metabolism and Oxidative Status Respond to Endurance Flight and Diet Quality. Ph.D. thesis. Rhode Island: University of Rhode Island, doi: 10.23860/DeMoranville-Kristen-2020
DeMoranville, K. J., Carter, W. A., Pierce, B. J., and McWilliams, S. R. (2021). Flight and dietary antioxidants influence antioxidant expression and activity in a migratory bird. Integr. Org. Biol. 4:obab035. doi: 10.1093/iob/obab035
Deppe, J. L., Ward, M. P., Bolus, R. T., Diehl, R. H., Celis-Murillo, A., Zenzal, T. J., et al. (2015). Fat, weather, and date affect migratory songbirds’ departure decisions, routes, and time it takes to cross the Gulf of Mexico. Proc. Natl. Acad. Sci. U. S. A. 112, E6331–E6338. doi: 10.1073/pnas.1503381112
Deutschlander, M. E., and Muheim, R. (2009). Fuel reserves affect migratory orientation of thrushes and sparrows both before and after crossing an ecological barrier near their breeding grounds. J. Avian Biol. 40, 85–89. doi: 10.1111/j.1600-048X.2008.04343.x
Dossman, B. C., Matthews, S. N., and Rodewald, P. G. (2018). An experimental examination of the influence of energetic condition on the stopover behavior of a Nearctic −- Neotropical migratory songbird, the American Redstart (Setophaga ruticilla). Auk Ornithol. Adv. 135, 91–100. doi: 10.1642/auk-17-67.1
Drummond, B. A. (2005). The selection of native and invasive plants by frugivorous birds in Maine. Northeast. Nat. 12, 33–44. doi: 10.1656/1092-6194(2005)012[0033:tsonai]2.0.co;2
Eikenaar, C., Bairlein, F., Stöwe, M., and Jenni-Eiermann, S. (2014). Corticosterone, food intake and refueling in a long-distance migrant. Horm. Behav. 65, 480–487. doi: 10.1016/j.yhbeh.2014.03.015
Eikenaar, C., Müller, F., Kämpfer, S., and Schmaljohann, H. (2016b). Fuel accumulation advances nocturnal departure: a migratory restlessness study on northern wheatears at stopover. Anim. Behav. 117, 9–14. doi: 10.1016/j.anbehav.2016.04.017
Eikenaar, C., Jönsson, J., Fritzsch, A., Wang, H. L., and Isaksson, C. (2016a). Migratory refueling affects non-enzymatic antioxidant capacity, but does not increase lipid peroxidation. Physiol. Behav. 158, 26–32. doi: 10.1016/j.physbeh.2016.02.033
Eikenaar, C., Winslott, E., Hessler, S., and Isaksson, C. (2020). Oxidative damage to lipids is rapidly reduced during migratory stopovers. Funct. Ecol. 34, 1215–1222. doi: 10.1111/1365-2435.13540
Fair, J. M., Paul, E., Jones, J., Clark, A. B., Davie, C., and Kaiser, G. (2010). The Use of Wild Birds in Research. Washington, D.C: Ornithological Council, doi: 10.2307/1369129
Finke, M. D. (2015). Complete nutrient content of four species of commercially available feeder insects fed enhanced diets during growth. Zoo Biol. 34, 554–564. doi: 10.1002/zoo.21246
Gill, R. E., Tibbitts, T. L., Douglas, D. C., Handel, C. M., Mulcahy, D. M., Gottschalck, J. C., et al. (2009). Extreme endurance flights by landbirds crossing the Pacific Ocean: ecological corridor rather than barrier? Proc. R. Soc. B Biol. Sci. 276, 447–457. doi: 10.1098/rspb.2008.1142
Guglielmo, C. G. (2018). Obese super athletes: fat-fueled migration in birds and bats. J. Exp. Biol. 221:jeb165753. doi: 10.1242/jeb.165753
Halliwell, B., and Gutteridge, J. (2007). Free Radicals in Biology and Medicine, 4th Edn. Oxford: Oxford University Press.
Hamilton, A., Ly, J., Robinson, J. R., Corder, K. R., DeMoranville, K. J., Schaeffer, P. J., et al. (2018). Conserved transcriptional activity and ligand responsiveness of avian PPARs: Potential role in regulating lipid metabolism in mirgratory birds. Gen. Comp. Endocrinol. 268, 110–120. doi: 10.1016/j.ygcen.2018.08.009
Hedenström, A., and Alerstam, T. (1997). Optimum fuel loads in migratory birds: distinguishing between time and energy minimization. J. Theor. Biol. 189, 227–234. doi: 10.1006/jtbi.1997.0505
Hunt, P. D., and Flaspohler, D. J. (2020). ““Yellow-rumped Warbler (Setophaga coronata),” in Birds of the World, ed. P. G. Rodewald (Ithaca: Cornell Lab of Ornithology), doi: 10.2173/bow.yerwar.01
Jenni-Eiermann, S., Jenni, L., Smith, S., and Costantini, D. (2014). Oxidative stress in endurance flight: An unconsidered factor in bird migration. PLoS One 9:e97650. doi: 10.1371/journal.pone.0097650
Kaiser, A. (1993). A new multi-category classification of subcutaneous fat deposits of songbirds. J. Field Ornithol. 64, 246–255.
Klaassen, M. (1996). Metabolic constraints on long-distance migration in birds. J. Exp. Biol. 199, 57–64. doi: 10.1242/jeb.199.1.57
Ktitorov, P., Tsvey, A., and Mukhin, A. (2010). The good and the bad stopover: Behaviours of migrant reed warblers at two contrasting sites. Behav. Ecol. Sociobiol. 64, 1135–1143. doi: 10.1007/s00265-010-0929-9
Kwit, C., Levey, D. J., Green Berg, C. H., Pear Son, S. F., McCarty, J. P., Sargent, S., et al. (2004). Fruit abundance and local distribution of wintering hermit thrushes (Cathaxus guttatus) and yellow-rumped warblers (Dendxoica coronata) in South Carolina. Auk 121, 46–57. doi: 10.2307/4090054
Laparra, J. M., and Sanz, Y. (2010). Interactions of gut microbiota with functional food components and nutraceuticals. Pharmacol. Res. 61, 219–225. doi: 10.1016/j.phrs.2009.11.001
Legagneux, P., Fast, P. L. F., Gauthier, G., and Bêty, J. (2012). Manipulating individual state during migration provides evidence for carry-over effects modulated by environmental conditions. Proc. R. Soc. B Biol. Sci. 279, 876–883. doi: 10.1098/rspb.2011.1351
Margis, R., Dunand, C., Teixeira, F. K., and Margis-Pinheiro, M. (2008). Glutathione peroxidase family - an evolutionary overview. FEBS J. 275, 3959–3970. doi: 10.1111/j.1742-4658.2008.06542.x
Marshall, T. J., Dick, M. F., and Guglielmo, C. G. (2016). Seasonal dietary shifting in yellow-rumped warblers is unrelated to macronutrient targets. Comp. Biochem. Physiol. A Mol. Integr. Physiol. 192, 57–63. doi: 10.1016/j.cbpa.2015.11.014
McKinnon, E. A., Stanley, C. Q., and Stutchbury, B. J. M. (2015). Carry-over effects of nonbreeding habitat on start-to-finish spring migration performance of a songbird. PLoS One 10:e0141580. doi: 10.1371/journal.pone.0141580
McWilliams, S., Carter, W., Cooper-Mullin, C., DeMoranville, K., Frawley, A., Pierce, B., et al. (2021). How Birds During Migration Maintain (Oxidative) Balance. Front. Ecol. Evol. 9:742642. doi: 10.3389/fevo.2021.742642
McWilliams, S., Pierce, B., Wittenzellner, A., Langlois, L., Engel, S., Speakman, J. R., et al. (2020). The energy savings-oxidative cost trade-off for migratory birds during endurance flight. eLife 9, 1–18. doi: 10.7554/eLife.60626
McWilliams, S. R., Guglielmo, C., Pierce, B., and Klaassen, M. (2004). Flying, fasting, and feeding in birds during migration: a nutritional and physiological ecology perspective. J. Avian Biol. 35, 377–393. doi: 10.1111/j.0908-8857.2004.03378.x
McWilliams, S. R., and Karasov, W. H. (2014). Spare capacity and phenotypic flexibility in the digestive system of a migratory bird: Defining the limits of animal design. Proc. R. Soc. B Biol. Sci. 281:20140308. doi: 10.1098/rspb.2014.0308
Mehlman, D. W., Mabey, S. E., Ewert, D. N., Duncan, C., Abel, B., Cimprich, D., et al. (2005). Conserving stopover sites for forest-dwelling migratory landbirds. Auk 122, 1281–1290.
Metzger, B. J., and Bairlein, F. (2011). Fat stores in a migratory bird: A reservoir of carotenoid pigments for times of need? J. Comp. Physiol. B Biochem. Syst. Environ. Physiol. 181, 269–275. doi: 10.1007/s00360-010-0511-9
Mills, A. M., Thurber, B. G., Mackenzie, S. A., and Taylor, P. D. (2011). Passerines Use Nocturnal Flights for Landscape-Scale Movements during Migration Stopover. Condor 113, 597–607. doi: 10.1525/cond.2011.100186
Mitchell, G. W., Taylor, P. D., and Warkentin, I. G. (2011). Movements of juvenile Blackpoll Warblers prior to autumn migration in Newfoundland reconsidered. Condor 113, 711–712. doi: 10.1525/cond.2011.110093
Mitchell, G. W., Woodworth, B. K., Taylor, P. D., and Norris, D. R. (2015). Automated telemetry reveals age specific differences in flight duration and speed are driven by wind conditions in a migratory songbird. Mov. Ecol. 3, 1–13. doi: 10.1186/s40462-015-0046-5
Morganti, M., Mellone, U., Bogliani, G., Saino, N., Ferri, A., Spina, F., et al. (2011). Flexible tuning of departure decisions in response to weather in black redstarts Phoenicurus ochruros migrating across the Mediterranean Sea. J. Avian Biol. 42, 323–334. doi: 10.1111/j.1600-048X.2011.05331.x
Morris, S. R., Covino, K. M., Jacobs, J. D., and Taylor, P. D. (2016). Fall migratory patterns of the blackpoll warbler at a continental scale. Auk 133, 41–51. doi: 10.1642/AUK-15-133.1
Mudrzynski, B. M., and Norment, C. J. (2013). Influence of habitat structure and fruit availability on use of a northeastern stopover site by fall songbirds. Wilson J. Ornithol. 125, 744–754. doi: 10.1676/13-060.1
Murray, B. G. Jr., and Murray, B. G. (1979). Fall migration of blackpoll and yellow-rumped warblers at Island Beach, New Jersey. Bird-Banding 50, 1–11.
Naef-Daenzer, B. (2007). An allometric function to fit leg-loop harnesses to terrestrial birds. J. Avian Biol. 38, 404–407. doi: 10.1111/j.2007.0908-8857.03863.x
Nisbet, I. C. T. T. (1970). Autumn Migration of the Blackpoll Warbler: Evidence for Long Flight Provided by Regional Survey. Bird-Banding 41:207. doi: 10.2307/4511673
Oguchi, Y., Smith, R. J., and Owen, J. C. (2017). Fruits and migrant health: Consequences of stopping over in exotic- vs. native-dominated shrublands on immune and antioxidant status of Swainson’s Thrushes and Gray Catbirds. Condor Ornithol. Appl. 119, 800–816. doi: 10.1650/CONDOR-17-28.1
Owen, J. C. (2011). Collecting, processing, and storing avian blood: a review. J. Field Ornithol. 82, 339–354. doi: 10.1111/j.1557-9263.2011.00338.x
Packmor, F., Klinner, T., Woodworth, B. K., Eikenaar, C., and Schmaljohann, H. (2020). Stopover departure decisions in songbirds: Do long-distance migrants depart earlier and more independently of weather conditions than medium-distance migrants? Mov. Ecol. 8, 1–14. doi: 10.1186/s40462-020-0193-1
Parrish, J. D. (1997). Patterns of frugivory and energetic condition in Nearctic landbirds during autumn migration. Condor 99, 681–697. doi: 10.2307/1370480
Paxton, K. L., and Moore, F. R. (2017). Connecting the dots: stopover strategies of an intercontinental migratory songbird in the context of the annual cycle. Ecol. Evol. 7, 6716–6728. doi: 10.1002/ece3.3227
Place, A. R., and Stiles, E. W. (1992). Living off the wax of the land: bayberries and yellow-rumped warblers. Auk 109, 334–345. doi: 10.2307/4088202
Podlesak, D. W., McWilliams, S. R., and Hatch, K. A. (2005). Stable isotopes in breath, blood, feces and feathers can indicate intra-individual changes in the diet of migratory songbirds. Oecologia 142, 501–510. doi: 10.1007/s00442-004-1737-6
Pomeroy, A. C., Butler, R. W., and Ydenberg, R. C. (2006). Experimental evidence that migrants adjust usage at a stopover site to trade off food and danger. Behav. Ecol. 17, 1041–1045. doi: 10.1093/beheco/arl043
Pyle, P. (1997). Identification Guide to North American Birds, Part I: Columbidae to Ploceidae. Bolinas, California: Slate Creek Press.
Reinert, S. E., Lapham, E., and Gaffett, K. (2002). “Landbird migration on Block Island: community composition and conservation implications for an island stopover habitat,” in The Ecology of Block Island, eds P. W. Paton, L. L. Gould, P. V. August, and A. O. Frost (Kingston, RI: The Rhode Island Natural History Survey), 151–168.
Saag, P., Tilgar, V., Mänd, R., Kilgas, P., and Mägi, M. (2011). Plumage bacterial assemblages in a breeding wild passerine: relationships with ecological factors and body condition. Microb. Ecol. 61, 740–749. doi: 10.1007/s00248-010-9789-0
Sapir, N., Abramsky, Z., Shochat, E., and Izhaki, I. (2004). Scale-dependent habitat selection in migratory frugivorous passerines. Naturwissenschaften 91, 544–547. doi: 10.1007/s00114-004-0564-2
Schaefer, H. M. (2011). Why fruits go to the dark side. Acta Oecol. 37, 604–610. doi: 10.1016/j.actao.2011.04.008
Schaefer, H. M., McGraw, K., and Catoni, C. (2008). Birds use fruit colour as honest signal of dietary antioxidant rewards. Funct. Ecol. 22, 303–310. doi: 10.1111/j.1365-2435.2007.01363.x
Schaefer, H. M., Valido, A., and Jordano, P. (2014). Birds see the true colours of fruits to live off the fat of the land. Proc. R. Soc. B Biol. Sci. 281:20132516. doi: 10.1098/rspb.2013.2516
Schaub, M., Jenni, L., and Bairlein, F. (2008). Fuel stores, fuel accumulation, and the decision to depart from a migration stopover site. Behav. Ecol. 19, 657–666. doi: 10.1093/beheco/arn023
Schmaljohann, H., Becker, P. J. J., Karaardic, H., Liechti, F., Naef-Daenzer, B., and Grande, C. (2011). Nocturnal exploratory flights, departure time, and direction in a migratory songbird. J. Ornithol. 152, 439–452. doi: 10.1007/s10336-010-0604-y
Schmaljohann, H., and Eikenaar, C. (2017). How do energy stores and changes in these affect departure decisions by migratory birds? A critical view on stopover ecology studies and some future perspectives. J. Comp. Physiol. A Neuroethol. Sens. Neural Behav. Physiol. 203, 411–429. doi: 10.1007/s00359-017-1166-8
Schmaljohann, H., Fox, J. W., and Bairlein, F. (2012). Phenotypic response to environmental cues, orientation and migration costs in songbirds flying halfway around the world. Anim. Behav. 84, 623–640. doi: 10.1016/j.anbehav.2012.06.018
Schmaljohann, H., and Klinner, T. (2020). A quasi-experimental approach using telemetry to assess migration-strategy-specific differences in the decision-making processes at stopover. BMC Ecol. 20:36. doi: 10.1186/s12898-020-00307-5
Schmaljohann, H., Korner-Nievergelt, F., Naef-Daenzer, B., Nagel, R., Maggini, I., Bulte, M., et al. (2013). Stopover optimization in a long-distance migrant: The role of fuel load and nocturnal take-off time in Alaskan northern wheatears (Oenanthe oenanthe). Front. Zool. 10:26. doi: 10.1186/1742-9994-10-26
Seewagen, C. L., and Guglielmo, C. G. (2010). Effects of fat and lean body mass on migratory landbird stopover duration. Wilson J. Ornithol. 122, 82–87. doi: 10.1676/09-088.1
Skrip, M. M., Bauchinger, U., Goymann, W., Fusani, L., Cardinale, M., Alan, R. R., et al. (2015). Migrating songbirds on stopover prepare for, and recover from, oxidative challenges posed by long-distance flight. Ecol. Evol. 5, 3198–3209. doi: 10.1002/ece3.1601
Skrip, M. M., and McWilliams, S. R. (2016). Oxidative balance in birds: An atoms-to-organisms-to-ecology primer for ornithologists. J. Field Ornithol. 87, 1–20. doi: 10.1111/jofo.12135
Skrip, M. M., Seeram, N. P., Yuan, T., Ma, H., and McWilliams, S. R. (2016). Dietary antioxidants and flight exercise in female birds affect allocation of nutrients to eggs: how carry-over effects work. J. Exp. Biol. 219, 2716–2725. doi: 10.1242/jeb.137802
Smetzer, J. R., and King, D. I. (2018). Prolonged stopover and consequences of migratory strategy on local-scale movements within a regional songbird staging area. Auk Ornithol. Adv. 135, 547–560. doi: 10.1642/auk-18-4.1
Smetzer, J. R., and King, D. I. (2020). Intrinsic markers reveal breeding origin and geographically-structured migration timing of two songbird species at a coastal stopover site. Anim. Migr. 7, 42–51. doi: 10.1515/ami-2020-0005
Smetzer, J. R., King, D. I., and Taylor, P. D. (2017). Fall migratory departure decisions and routes of blackpoll warblers Setophaga striata and red-eyed vireos Vireo olivaceus at a coastal barrier in the Gulf of Maine. J. Avian Biol. 48, 1451–1461. doi: 10.1111/jav.01450
Smith, A. D., and McWilliams, S. R. (2014). What to do when stopping over: behavioral decisions of a migrating songbird during stopover are dictated by initial change in their body condition and mediated by key environmental conditions. Behav. Ecol. 25, 1423–1435. doi: 10.1093/beheco/aru148
Smith, R. J., and Hatch, M. I. (2017). Loss of southern arrowwoods (Viburnum dentatum) is associated with changes in species composition and mass gain by spring migrants using early successional habitat. Wilson J. Ornithol. 129, 247–258. doi: 10.1676/16-025.1
Smith, S. B., DeSando, S. A., and Pagano, T. (2013). The value of native and invasive fruit-bearing shrubs for migrating songbirds. Northeast. Nat. 20, 171–184. doi: 10.1656/045.020.0114
Smith, S. B., McPherson, K. H., Backer, J. M., Pierce, B. J., Podlesak, D. W., and McWilliams, S. R. (2007). Fruit quality and consumption by songbirds during autumn migration. Wilson J. Ornithol. 119, 419–428. doi: 10.1676/06-073.1
Smith, S. B., and McWilliams, S. R. (2010). Patterns of fuel use and storage in migrating passerines in relation to fruit resources at autumn stopover site. Auk 127, 108–118. doi: 10.1525/auk.2009.09139
Smith, S. B., Miller, A. C., Merchant, C. R., and Sankoh, A. F. (2015). Local site variation in stopover physiology of migrating songbirds near the south shore of Lake Ontario is linked to fruit availability and quality. Conserv. Physiol. 3, 1–14. doi: 10.1093/conphys/cov036.Introduction
Smith, S. B., and Paton, P. W. C. (2011). Long-term shifts in autumn migration by songbirds at a coastal eastern North American stopover site. Wilson J. Ornithol. 123, 557–566. doi: 10.1676/10-139.1
Somveille, M., Manica, A., Butchart, S. H. M., and Rodrigues, A. S. L. (2013). Mapping Global Diversity Patterns for Migratory Birds. PLoS One 8:e70907. doi: 10.1371/journal.pone.0070907
Somveille, M., Rodrigues, A. S. L., and Manica, A. (2015). Why do birds migrate? A macroecological perspective. Glob. Ecol. Biogeogr. 24, 664–674. doi: 10.1111/geb.12298
Steadman, D. W. (2005). “Paleoecology and fossil history of migratory landbirds,” in Birds of Two Worlds: The Ecology and Evolution of Migration, eds R. Greenburg and P. P. Mara (Baltimore and London: John Hopkins University Press), 5–17.
Taylor, P. D., Crewe, T. L., Mackenzie, S. A., Lepage, D., Aubry, Y., Crysler, Z., et al. (2017). The Motus Wildlife Tracking System: a collaborative research network. Avian Conserv. Ecol. 12:8. doi: 10.5751/ACE-00953-120108
Taylor, P. D., Mackenzie, S. A., Thurber, B. G., Calvert, A. M., Mills, A. M., McGuire, L. P., et al. (2011). Landscape movements of migratory birds and bats reveal an expanded scale of stopover. PLoS One 6:e27054. doi: 10.1371/journal.pone.0027054
Toews, D. P. L., Mandic, M., Richards, J. G., and Irwin, D. E. (2014). Migration, mitochondria, and the yellow-rumped warbler. Evolution 68, 241–255. doi: 10.1111/evo.12260
Trevelline, B. K., Fontaine, S. S., Hartup, B. K., and Kohl, K. D. (2019). Conservation biology needs a microbial renaissance: A call for the consideration of host-associated microbiota in wildlife management practices. Proc. R. Soc. B Biol. Sci. 286:20182448. doi: 10.1098/rspb.2018.2448
Tsahar, E., Arad, Z., Izhaki, I., and Guglielmo, C. G. (2006). The relationship between uric acid and its oxidative product allantoin: A potential indicator for the evaluation of oxidative stress in birds. J. Comp. Physiol. B Biochem. Syst. Environ. Physiol. 176, 653–661. doi: 10.1007/s00360-006-0088-5
Wikelski, M., Tarlow, E. M., Raim, A., Diehl, R. H., Larkin, R. P., and Visser, G. H. (2003). Costs of migration in free-flying songbirds. Nature 423:704. doi: 10.1038/423704a
Woodworth, B. K., Francis, C. M., and Taylor, P. D. (2014). Inland flights of young red-eyed vireos Vireo olivaceus in relation to survival and habitat in a coastal stopover landscape. J. Avian Biol. 45, 387–395. doi: 10.1111/jav.00276
Keywords: oxidative stress, condition-dependence, fruit, glutathione peroxidase, micronutrients
Citation: Cooper-Mullin C and McWilliams SR (2022) Fat Stores and Antioxidant Capacity Affect Stopover Decisions in Three of Four Species of Migratory Passerines With Different Migration Strategies: An Experimental Approach. Front. Ecol. Evol. 10:762146. doi: 10.3389/fevo.2022.762146
Received: 21 August 2021; Accepted: 31 January 2022;
Published: 03 March 2022.
Edited by:
Arne Hegemann, Lund University, SwedenReviewed by:
Caroline Isaksson, Lund University, SwedenCopyright © 2022 Cooper-Mullin and McWilliams. This is an open-access article distributed under the terms of the Creative Commons Attribution License (CC BY). The use, distribution or reproduction in other forums is permitted, provided the original author(s) and the copyright owner(s) are credited and that the original publication in this journal is cited, in accordance with accepted academic practice. No use, distribution or reproduction is permitted which does not comply with these terms.
*Correspondence: Clara Cooper-Mullin, Y2Nvb3Blci1tdWxsaW5AdXJpLmVkdQ==
Disclaimer: All claims expressed in this article are solely those of the authors and do not necessarily represent those of their affiliated organizations, or those of the publisher, the editors and the reviewers. Any product that may be evaluated in this article or claim that may be made by its manufacturer is not guaranteed or endorsed by the publisher.
Research integrity at Frontiers
Learn more about the work of our research integrity team to safeguard the quality of each article we publish.