- Centre for Biodiversity Conservation and Management, G.B. Pant National Institute of Himalayan Environment, Almora, India
Plant biodiversity of some of the high-altitude national parks of Indian Himalaya is increasingly experiencing anthropogenic pressure. Consequently, plant species composition, vegetation structure, and diversity patterns of these protected areas are assumed to be substantially altered. However, limited efforts are made to quantify the impact of anthropogenic disturbances on the species richness of such high-altitude national parks of Indian Himalaya. To overcome this data gap, this study is aimed at quantifying the impact of anthropogenic disturbances on the vegetation community patterns of two Indian Himalayan National Parks along elevation transects, i.e., the Valley of Flowers National Park (VoFNP, altitude ranging from 3,200 to 6,700 m a.s.l.) and the Great Himalayan National Park (GHNP, altitude ranging from 1,500 to 6,000 m a.s.l.), India. The impact of anthropogenic disturbances along the elevation zone (3,200–4,500 m) on the vegetation community patterns was assessed by comparing plant species richness and diversity between selected disturbed and undisturbed sites along the altitudinal gradient. The disturbed sites, near to pathways within each national park, experienced the frequent human interventions than the undisturbed sites which were beyond 25–50 m from the pathway within each national park. Diversity profiles calculated as hill numbers (q = 0, 1, 2) showed significant variation between disturbed and undisturbed sites exhibiting high level of disturbance, especially in VoFNP. The non-metric multidimensional scaling of species composition data along the elevation of both national parks indicated that heterogeneously grouped plant species were much higher for disturbed sites than undisturbed ones. The quantum of anthropogenic pressure on disturbed sites of both VoFNP and GHNP was discernible through statistically significant lower values of soil moisture, bulk density, porosity, potassium, and phosphorous content than the undisturbed sites. As a consequence, disturbed sites were clearly noted to have lower numbers of threatened and endemic species (20 and 17, respectively) in comparison with undisturbed sites (32 and 31, respectively). The canonical correlation analyses of species compositions and soil properties of VoFNP and GHNP indicated that soil moisture and pH were the two major factors that control species composition for both disturbed and undisturbed sites, whereas soil porosity played a major role in species composition of undisturbed sites. The disturbed sites of lower elevation zones (3,200–3,500 m) of both parks were found with a higher density of invasive species (i.e., Polygonum polystachyum, Impatiens sulcata, Cuscuta europaea, etc.). Our study indicated that anthropogenic pressures on the high-altitude national parks of India not only altered the soil properties, but potentially threatened the species composition, thus accelerating urgent need to implement mitigation measures for restoration of degrading habitat of the native, endemic, and threatened plants in both the parks.
Introduction
During the last couple of decades, an assessment of plant species co-dominance and their interactions with biotic and abiotic factors under increasing natural and anthropogenic pressures has become a major area of research for ecologists and environmentalists (Cole et al., 2008; Kamlesh et al., 2012; Xu et al., 2016; Yao et al., 2016; Bhatt et al., 2021). Increased population pressure has led to progressive developmental activities that have greatly exploited the natural ecosystems leading to the continual loss of floral and faunal diversity, especially in tropical regions (Kueffer et al., 2014; Pandey et al., 2019; Yirga et al., 2019; Mehta et al., 2021; Kumar et al., 2021, 2022). Various anthropogenic activities such as agricultural expansion, livestock grazing, tourism, and so on have negatively affected the vegetation composition across the world and resulted in the introduction or expansion of invasive species (Pauchard and Villarroel, 2002; Tang et al., 2014). As a result, habitat fragmentation is becoming clearly evident (Spear et al., 2013). While natural habitats are more resistant to invasions by alien species, excessive anthropogenic pressures are noted to increase the habitat efficacy for immigration of non-native or weedy species (Hassan and Hassan, 2019).
To mitigate and adapt to the anthropogenic pressure-induced loss of natural habitat of plant species, establishment of protected areas (PAs) has been known to be a cornerstone for in situ biological conservation. While the worldwide system of PAs has grown exponentially during the recent decades, mission of PAs has also extended from biodiversity conservation to improving human welfare by allowing natives to selectively use bio-resources within PAs (Naughton-Treves et al., 2005). However, policies and management in and around the PAs are sometimes noted to be ineffective (Pressey et al., 2015). As a consequence, while conservationists celebrate this geographic and conceptual expansion of PAs, they often disagree about management strategies of PAs, particularly with respect to socio-economical effectiveness (Naughton-Treves et al., 2005; Watson et al., 2014). Furthermore, inefficient management is also noted to deteriorate ecosystems at alarming rates both inside and outside the PAs (Laurance et al., 2012; Kuniyal et al., 2021). As a result, substantial evidence across the world indicates the enhancement of invasive plants within poorly managed PAs, impacting native and endemic species and their ecosystems, having potential of complete devastation of the area (Rands et al., 2010). Therefore, it is imperative that health assessment of PAs is carried out at certain time intervals for assessing status of anthropogenic pressures and effectiveness of management practices, so that appropriate conservation measures could be planned.
The Valley of Flowers National Park (VoFNP), Uttarakhand and Great Himalayan National Park (GHNP), Himachal Pradesh, India are such PAs and known for their unique biodiversity, mesmerizing meadows, and stunning landscape. Although the floristic diversity and vegetal community of the area have been frequently surveyed by Smythe (1938), Ghildiyal (1957), Wadhwa et al. (1987), Kala (1998), Singh and Rawat (2000), Murthy (2011), Bisht et al. (2018), and Kumar et al. (2021), natural and anthropogenic disturbances persistently cause alterations in the floral and faunal composition of the alpine grasslands (Gao et al., 2010; Kamlesh et al., 2012; Xu et al., 2016; Yao et al., 2016). Despite being categorized as PAs, the scenic beauty and unique biodiversity of each park is a major attraction, making it prone to mass tourism pressure. As a result, waste accumulation, plastic disposal, etc., are becoming major problems leading to depletion of native plant diversity and environmental degradation. However, a detailed assessment of status of anthropogenic pressures on the floristic diversity patterns of both the national parks remained unaddressed. Since long-term protection of these parks is dependent on formulating efficient management plans by quantifying impact of anthropogenic pressure, the overarching aim of this study was to measure impact of disturbances on density of the native, endemic, and threatened plants.
As a result, this study aims to describe and discuss dynamics in species diversity patterns along altitude gradient ranging from 3,200 to 4,500 m in VoFNP and GHNP between sites categorized as anthropogenically disturbed and undisturbed and suggest conservation policies for the protection of the native population of the parks. This study has considered multiple representative altitude transects for providing answers to following questions: (i) How do species richness, diversity, and density patterns vary between disturbed and undisturbed transects along altitude gradient? (ii) Do vegetation compositional patterns as well as edaphic properties differ between disturbed and undisturbed sites as well along? and (iii) assess whether and how edaphic and anthropogenic pressures influence plant species abundance and composition in high altitudes?
Materials and Methods
Study Area
This study was carried out in VoFNP (latitude: 30°41′ to 30°48′ N and longitude: 79°33′ to 79°46′ E) in Chamoli, Uttarakhand, and GHNP (latitude: 31° 38′ 28″ N to 31° 51′ 58″ N and longitude: 77° 20′ 11″ E to 77° 45′ 52″ E) in Kullu, Himachal Pradesh, India. With an altitude ranging from 3,200 to 6,700 m a.s.l., VoFNP is one of the core zones of Nanda Devi Biosphere Reserve (NDBR) engraved as a World Heritage Site by United Nations Educational, Scientific, and Cultural Organization (UNESCO) in July 2005. It covers an area of 87.5 km2 and approximately 1.3% of the total geographical area of Chamoli district. Mean annual temperature of the valley is 2.64°C with minimum temperature of –8.2°C in winters and maximum of 11.8°C in summers1. Similarly, mean annual precipitation is 118 mm with minimum precipitation, 29 mm in dry season, and maximum 303 mm in wettest season (see footnote 1). The landscape is known for its spectacular beauty, rich floristic diversity, extreme topographic variations, alpine and sub-alpine ecosystems, and conflicting conservation issues. The national park is encircled by Gauri Parbat (6,590 m) and Rataban (6,126 m) in east, Kunth Khal (4,500 m) in west, Nilgiri Parvat (6,479 m) in north, and Saptsring (5,013 m) in south. Small snow-fed river Pushpawati, originating from the banks of Tipra Glacier, flows through the park and joins the Lakshaman Ganga at Ghangharia. GHNP, on the other hand, encompasses nearly 1,171 km2 area along altitude gradient ranging from 1,500 to 6,000 m a.s.l. Mean annual temperature of the valley is 6.15°C with minimum temperature of –5.9°C in winters and maximum of 14.8°C in summers (see footnote 1). Similarly, mean annual precipitation is 83 mm with minimum precipitation, 18 mm in dry season, and maximum 196 mm in wettest season (see footnote 1). It was formally declared as a national park in 1999 under Wildlife (Protection) Act 1972 by the Himachal Pradesh Government and inscribed as a World Heritage Site in 2014 by UNESCO. GHNP is one of the largest and important PAs for the conservation of the biological diversity in Western Himalayan region. GHNP is one of the two national parks in the world to support a population of endangered western tragopan (Tragopan melanocephalus) and a large number of rare and threatened plant species many of which are of medicinal value (Gaston and Garson, 1993). In this study conducted in Parvati Valley from Parvati Base camp (4,550 m) to after the Tunda Bhuj (3,200 m) on the way to Kheerganga of GHNP. We trekked from mud and crossed the Pin-Parvati Pass (5,334 m) to reach the study site and returned via Kheerganga (2,800 m)-Pulga-Manikaran.
Sampling Design
After proper survey and field visits (Bisht et al., 2018), representative disturbed and undisturbed sites within VoFNP and GHNP were established along an altitude range of 3,200–4,500 m a.s.l. The area of site along and nearby the pathway (i.e., within 25 m both sides of pathway) which experiences frequent human intervention was considered as disturbed, while area distant from general pathway with less or no human influence (i.e., area outside 25-m zone up to 50 m on both sides of pathway) was considered undisturbed. Beginning from 3,200 m, sample plots of 0.25 ha (50 m × 50 m) were established along elevation gradient (up to 4,500 m) in both sites (i.e., disturbed and undisturbed). In each plot, four (5m × 5 m) sub-plots were established to sample the shrub layer, and ten (1 m × 1 m) sub-plots were established to sample the herbaceous layer (only vascular plants such as angiosperms, gymnosperms, and pteridophytes were considered) (Figure 1). As the sampling elevation ranges were within the alpine landscapes, no tree habit was found.
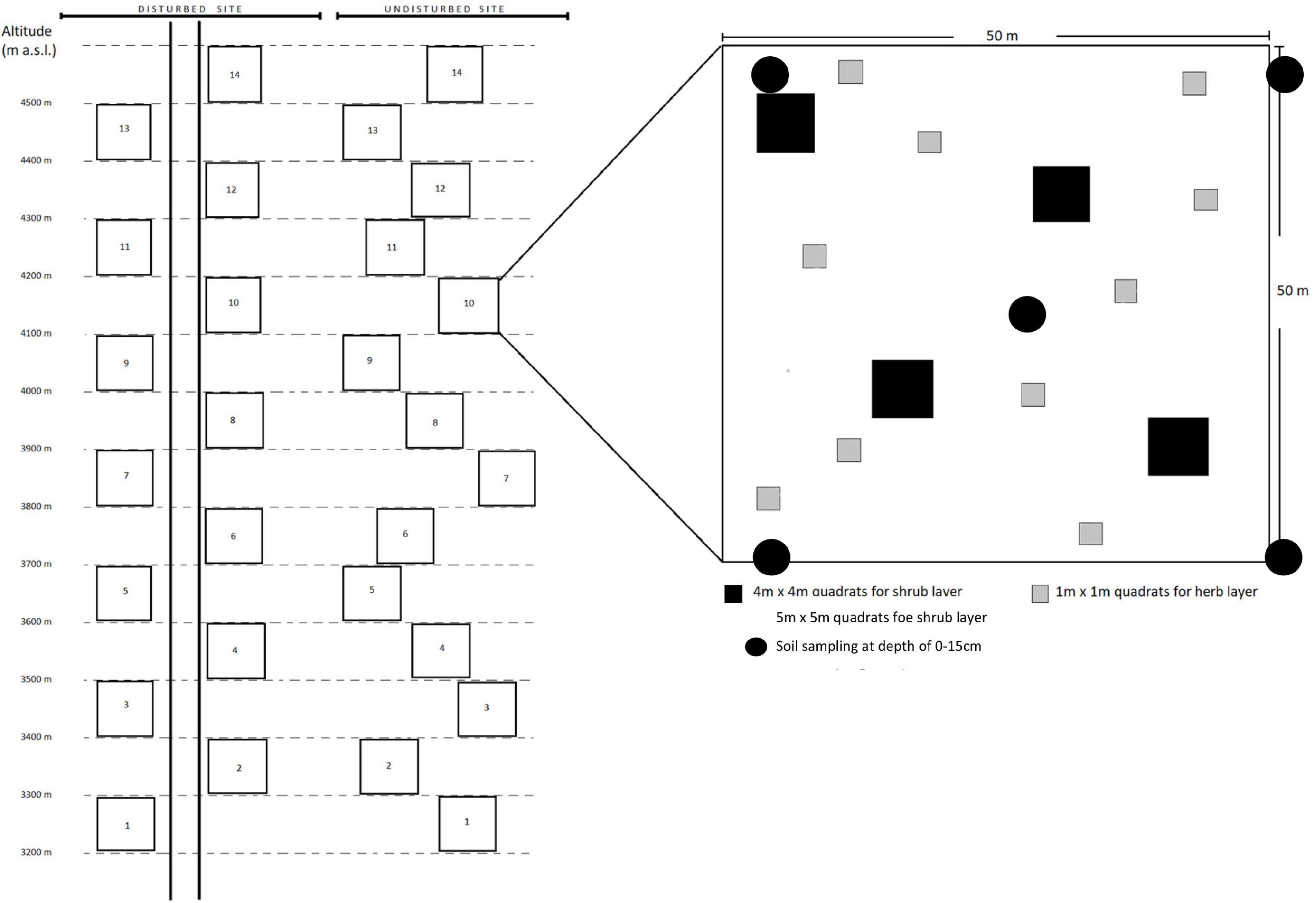
Figure 1. Sampling design. Schematic illustration of the two study sites, i.e., disturbed and undisturbed, along an altitude gradient from 3,200 to 4,500 m with a sample plot of 0.25 hac (50 m × 50 m) in each altitudinal zone. Plot design (right) is equivalent on each plot.
Collection and Identification of Plants
Intensive trips were conducted from June to August 2018 in the view of maximum blooming of alpine landscape. All plant specimens were collected as per standard procedures (Jain and Rao, 1977) and housed in herbarium of G.B. Pant National Institute of Himalayan Environment and Development, Kosi-Katarmal, Almora (GBP). The specimens were identified using regional floras and published literature (Naithani, 1984; Wadhwa et al., 1987; Gaur, 1999; Singh and Rawat, 2000; Kala and Mathur, 2002; Pusalkar and Singh, 2012; Chandra Sekar et al., 2014). Categorization of threatened plants was done using Red Data Book of India (Nayar and Shastry, 1987, 1988, 1990), Conservation Assessment and Management Prioritization (Ved et al., 2003), and International Union for Conservation of Nature (IUCN, 2020). For the identification of endemic and invasive plants, Chandra Sekar et al. (2012) and Singh et al. (2015) were followed, respectively.
Analyses of Phytosociological Parameters
The number of individuals of plant species per quadrat was taken, pooled, and analyzed as per the standard phytosociological approaches (Misra, 1968; Mueller-Dombois and Ellenberg, 1974; Zou et al., 2013; Gairola et al., 2014; Goswami and Das, 2018) and Important Value Index was calculated as per (Floeter et al., 2004). For each sampling site (disturbed and undisturbed) species diversity was quantified using Hill numbers (or effective number of species), i.e., the number of equally abundant species that are needed to give the same value of a diversity measures (Chao et al., 2014; Pardo et al., 2020). Hill numbers represent best choice to quantify abundance-based species diversity. They are the family of numbers which differ among themselves by the parameter q (where q controls the weights of species relative abundances) and encompasses three diversity measures:
(i) Species richness, S (diversity of order q = 0): 0D = ;
(ii) exponent of Shannon diversity, H′ (q = 1): 1D = ; and
(iii) inverse Simpson diversity, In(S) (q = 2): 2D (Chao et al., 2014).
The Hill numbers are very rich in information, since they combine information on species richness, species rarity (species relative abundances), and species dominance. Pielou’s (1996) was calculated as per formula
We used the data from sampling sites to illustrate rarefaction and extrapolation curves of sample coverage and separated for disturbed and undisturbed plots as given by Chao and Colwell (2017) using iNEXT statistical package2 at 95% confidence interval. Sample coverage has many advantages over putative completeness, especially since the estimates of “total” richness all have rather large levels of uncertainty, which need to be accounted for. Beta diversity (β) was calculated based on the presence or absence of data (Wilson and Schmida, 1984; Koleff et al., 2003) using the formula
where β = beta diversity, α = average number of species in the samples, g(H) = number of species gained along an environmental gradient, and l(H) = number of species lost along the same gradient.
To numerically compare patterns of diversity indices (Hill numbers) along elevation gradient, quadratic models were fitted and model performances were evaluated by r2 values computed at p-value < 0.01, except for evenness. Since richness is noted to have unimodal relationships with other diversity indices along elevation gradient (Sánchez-González and López-Mata, 2005), Pearson’s correlations were estimated between richness-Shannon index, richness-Simpson index, and richness-evenness. Statistically significant correlation coefficients among the above pairs of the undisturbed sites, having relatively homogeneous distribution of species, are expected to be higher than disturbed the sites.
Physicochemical Parameters of Soil
To further ascertain the level of anthropogenic disturbances, soil physicochemical parameters of the disturbed and undisturbed sites were analyzed. Soil samples from each 0.25 ha plot were collected from five sub-plots up to a depth of 15 cm using auger and pooled together as one sample (Figure 1). The collected samples were brought to laboratory, and soil parameters were analyzed using respective methodologies, such as moisture, pH (Black et al., 1965), bulk density (Miller and Donahue, 1990), water holding capacity (Cassel and Nielsen, 1986), organic carbon (OC) (Walkley and Black, 1934), total nitrogen (N) (Parkinson and Allen, 1975), available phosphorus (P) (Olsen et al., 1954), and available potassium (K). Subsequently, relationships between soil parameters and diversity indices of herbs and shrubs in undisturbed and disturbed sites were established through correlation matrices.
Non-metric Multidimensional Scaling
As non-metric multidimensional scaling (NMDS) is regarded as the most robust unconstrained ordination method in community ecology (Minchin, 1987), to assess the community composition of herbs and shrubs along the elevation gradient of the disturbed and undisturbed sites, ordination plots were produced using NMDS of the vegetation abundance and elevation. Elevations in the ordination plots were represented with “E,” where “E1” represents elevation at 3,200 m, “E2” represents 3,300 m, and so on. Similarly, abbreviated scientific names, density and frequency of herbs and shrubs were used (refer to Appendices S1–S4). The NMDS analyses were carried out using the R-Vegan package (version 2.5-3) function “metaMDS (Oksanen, 2019).” To select an optimum dimension for NMDS analyses for both herbs and shrubs, stress-dimension analyses were carried out, and dimension 2 was used for both NMDS analyses of shrubs and herbs using “Bray–Curtis” distance.
Canonical Correspondence Analysis
The canonical correspondence analysis (CCA) was carried out to quantify relationships between soil parameters and herbs or shrubs distribution of undisturbed and disturbed sites with elevation. The community data matrices of herbs and shrubs for both sites were used with the constrained matrices composing of soil parameters, (i) organic carbon (OC), (ii) nitrogen (N), (iii) phosphorus (P), (iv) potassium (K), (v) pH, (vi) temperature (temp), (vii) moisture, and (vii) porosity. The nature of relationships between soil parameters and vegetation distribution was depicted through ordination diagram. The CCA was carried out using the R-Vegan package (version 2.5-3) function “CCA,” and results were analyzed with respect to inertia, ranks, and eigen values of constrained matrices and parameters. Finally, a comparative assessment of the relationships between distribution and soil parameters of disturbed and undisturbed herbs and shrubs was made.
Results
Floristic Analysis
A total of 155 taxa (154 species and 1 variety) belonging to 41 families and 116 genera were recorded in VoFNP. Out of the total taxa, 139 taxa (138 species and 1 variety) belonging to 40 families and 105 genera were from undisturbed site, whereas 93 taxa (92 species and 1 variety) belonging to 36 families and 78 genera were from disturbed site. Moreover, 76 plant species were common to both the sites, whereas 63 species in undisturbed and 17 species in disturbed sites were unique. One fern species, i.e., Claytosmunda claytoniana was also recorded 280 from both the sites. Out of 155 taxa, 16 shrub species (9 families and 14 genera) were recorded from undisturbed site, whereas 15 shrub species (9 families and 13 genera) from disturbed site. Out of 16, 15 species were common to both the sites, whereas one species, i.e., Sorbus ursine was recorded only from undisturbed site. Summarily, anthropogenic disturbance reduced species richness by 6.3% in shrubs and 36.6% in herbs.
A total of 215 taxa (214 species and 1 variety) including 44 families and 145 genera were recorded in GHNP. Out of this, 185 taxa (184 species and 1 variety) belonging to 40 families and 126 genera were from undisturbed site, whereas 131 taxa (130 species and 1 variety) belonging to 35 families and 95 genera were from disturbed site. Moreover, 104 floras were common to both the sites, whereas 85 species in undisturbed and 29 species in disturbed sites were unique. One fern species, i.e., Claytosmunda claytoniana was also recorded 280 from both the sites. Out of 215 taxa, 26 shrub species (12 families and 19 genera) were recorded in GHNP and out of this, 25 shrub species (11 families and 18 genera) were recorded from undisturbed site, whereas 22 shrub species (12 families and 19 genera) from disturbed site. A total of 21 species were common to both the sites, whereas one species, i.e., Syringa emodi was recorded only from disturbed site. Summarily, anthropogenic disturbance was noted to reduce 12.0% of shrubs and 31.5% of herbs.
Threatened Species
Out of 26 threatened herbs (17 families and 23 genera) of VoFNP, a total of 24 (15 families and 21 genera) were recorded from undisturbed sites, whereas 12 (12 families and 12 genera) from disturbed sites. A total of 10 threatened species were common to both sites, 14 species were found only in undisturbed sites, and 2 species, namely, Urtica dioica and Verbascum thapsus were found only in disturbed sites (Supplementary Table 1). A total of two shrubs, namely, Juniperus communis and Rhododendron hypenanthum were recorded from both sites. The maximum number of threatened herbs (n = 8) was found at 3,500-m elevation in undisturbed sites, while in disturbed sites, the higher number of those plants (n = 4) occurred at 3,800 m. The number of threatened shrubs (n = 2) was higher in undisturbed sites, as compared to disturbed sites (n = 1). This can be attributed to the comparatively higher human interferences at lower elevations as compared to higher, risking the habitat of native species. Summarily, anthropogenic disturbance was noted to reduce 46.2% threatened herbs in VoFNP.
Out of 22 threatened herbs (17 families and 22 genera) of GHNP, a total of 19 (14 families and 19 genera) were recorded from undisturbed sites, whereas 9 (8 families and 9 genera) from disturbed sites. A total of 6 threatened species were common to both the sites, 13 species were found only in undisturbed sites, and 3 species, namely, Agrostis pilosula, Rheum webbianum, and Urtica dioica were found only in disturbed sites (Supplementary Table 1). A total of three shrubs, namely, Ephedra gerardiana, Juniperus communis, and Rhododendron hypenanthum were recorded from both the sites. The maximum number of threatened herbs (n = 05) was found in 3,600-m elevation in undisturbed site, while in disturbed site, the higher number of those plants (n = 04) occurred in 3,600 m. The maximum number of threatened shrubs (n = 2) was found in 3, 500-, 3, 600-, 3, 700-, 3, 800-, and 4,000-m elevations in both the sites. Summarily, anthropogenic disturbance was noted to reduce 52.6% threatened herbs in GHNP.
Endemic Species
A total of 14 endemic herbs (10 families and 14 genera) were recorded from undisturbed sites whereas 07 endemic species (07 families and 07 genera) were recorded from disturbed sites of VoFNP. Out of 14, seven species, namely, Anemone tetrasepala, Arisaema propinquum, Dactylorhiza hatagirea, Fritillaria roylei, Morina longifolia, Pleurospermum candollei, and Polygonum affine were common to both sites and seven species, namely, Aconitum violaceum, Allium humile, Angelica glauca, Cortia depressa, Meconopsis aculeata, Nardostachys jatamansi, and Strobilanthes atropurpureus were only found in undisturbed site. A total of five endemic shrubs, namely, Berberis jaeschkeana, Cassiope fastigiata, Cotoneaster microphyllus, Rhododendron campanulatum, and Rhododendron hypenanthum were common to both the sites, while one, namely, Sorbus ursine was recorded from undisturbed sites. The maximum number of endemic plants (n = 05) was found in 3, 500-, 3, 700-, 3, 900-, and 4,100-m elevations in the undisturbed sites. In disturbed sites, the highest endemic plants (n = 03) were found in 3, 400-, 3, 500-, 3, 800-, and 3,900-m elevations. Summarily, anthropogenic disturbance was noted to reduce 16.7% endemic shrubs and 50.0% endemic herbs in VoFNP.
A total of 25 endemic herbs (17 families and 24 genera) were recorded from undisturbed sites, whereas 10 endemic species (9 families and 9 genera) were recorded from disturbed sites of GHNP. Out of 28, seven species, namely, Arisaema propinquum, Bergenia stracheyi, Cortia depressa, Kobresia duthiei, Morina longifolia, Pleurospermum candollei, and Polygonum affine were common to both sites and 18 species, namely, Aconitum heterophyllum, Aconitum violaceum, Allium humile, Anemone tetrasepala, Angelica glauca, Codonopsis ovata, Dactylorhiza hatagirea, Eritrichium canum, Ferula kuhistanica, Fritillaria roylei, Gentianella tenella, Hippolytia dolicophylla, Jurinea macrocephala, Meconopsis aculeata, Nardostachys jatamansi, Nepeta eriostachys, Strobilanthes atropurpureus, and Swertia petiolata were only found in undisturbed sites. A total of three endemic shrubs, namely, Anemone tetrasepala, Artemisia roxburghiana, and Polygonum rumicifolium were only found in disturbed sites. Summarily, anthropogenic disturbance was noted to reduce 60.0% endemic herbs in GHNP.
Invasive Species
There were no invasive species in any undisturbed sites of VoFNP and GHNP, whereas in disturbed sites of VoFNP, one invasive species, namely, Cuscuta europaea was reported as an emerging threat to plant diversity. Similarly, two invasive species in disturbed sites, namely, Chenopodium album and Cuscuta europaea of GHNP were reported.
Phytosociological Assessment
Disturbed sites of VoFNP recorded higher densities of fast-growing, weedy, and invasive species such as Polygonum polystachyum, Impatiens sulcata, Claytosmunda claytoniana (Golden Fern), and Cuscuta europaea. Diversity (Hill numbers) profiles along elevation gradient formed a unimodal pattern, and a third-order polynomial regression showed a significant fit in undisturbed and disturbed sites (Figures 2, 3). In both disturbed and undisturbed sites of study area, species richness, density, and evenness decreased with increasing altitudes. In undisturbed site, diversity indices and species richness were higher in comparison with the disturbed site. This can be attributed to less human interference in the undisturbed site. Moreover, the variation between disturbed and undisturbed sites is more in VoFNP, thus exhibiting high level of disturbance (Figure 2). Whereas in GHNP, while the number of species (i.e., species richness) is less in undisturbed sites, Hill numbers for higher orders (i.e., 1 and 2) were not highly differing (Figure 3), thus showing relatively similar evenness between disturbed and undisturbed sites. Linear correlation between species richness and diversity indices was highly significant (p < 0.05) for undisturbed and disturbed sites (Figures 4, 5).
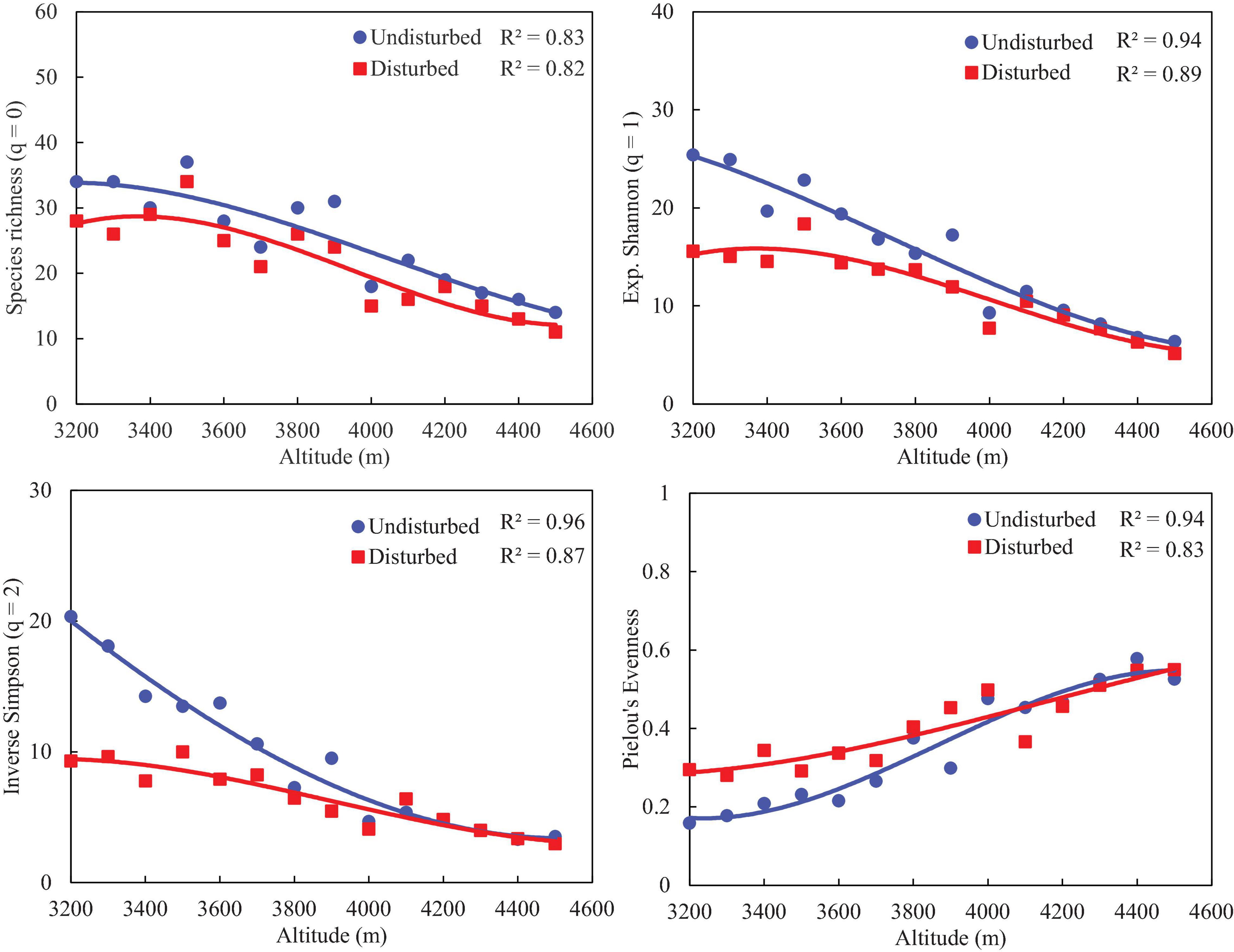
Figure 2. Comparison of diversity (Hill numbers) profiles between disturbed and undisturbed sites along altitude gradients in VoFNP.
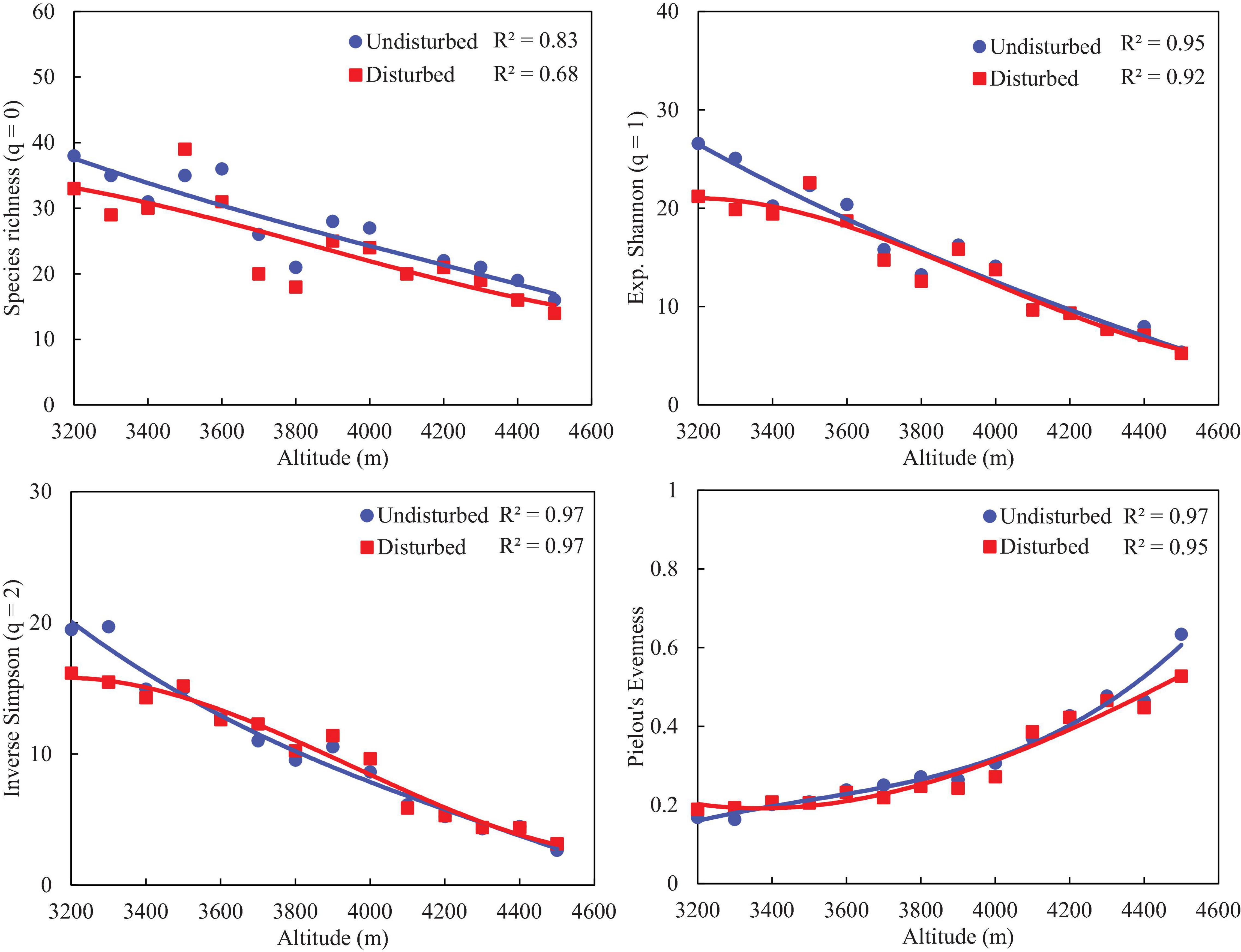
Figure 3. Comparison of diversity (Hill numbers) profiles between disturbed and undisturbed sites along altitude gradients in GHNP.
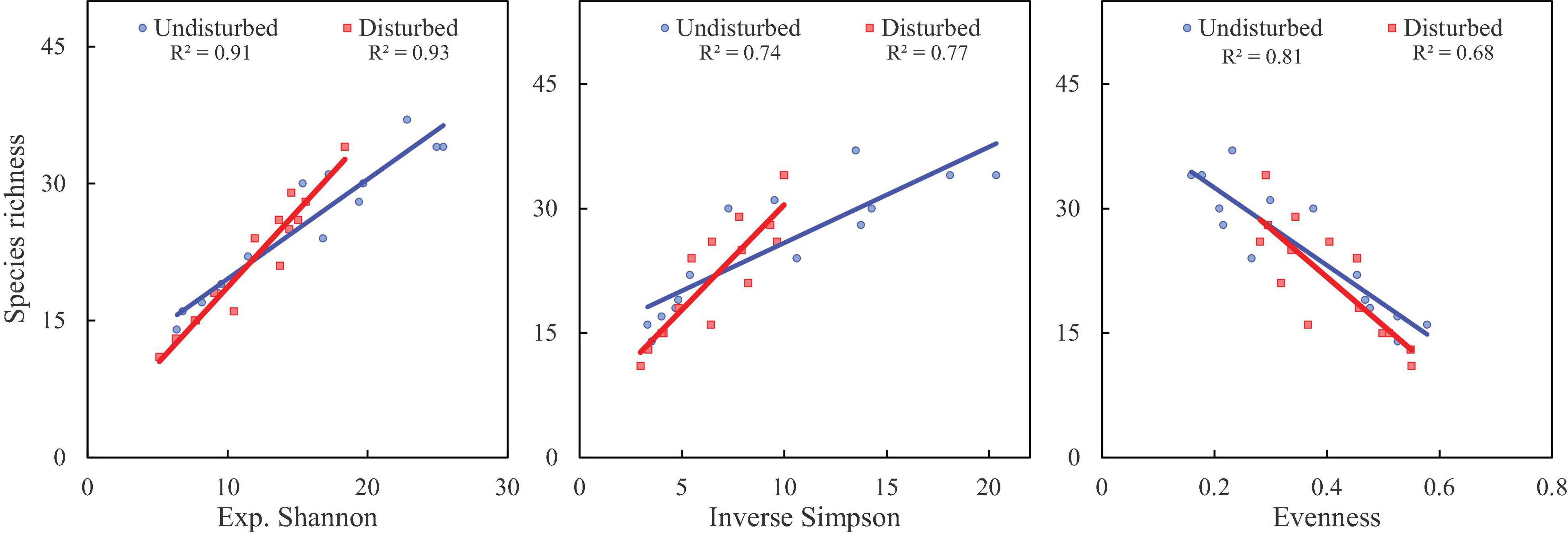
Figure 4. Correlation analysis of species richness with diversity indices (as per Hill numbers) in disturbed and undisturbed sites in VoFNP. The linear fits and r2 values (at a p-value < 0.05) are presented in boxes.
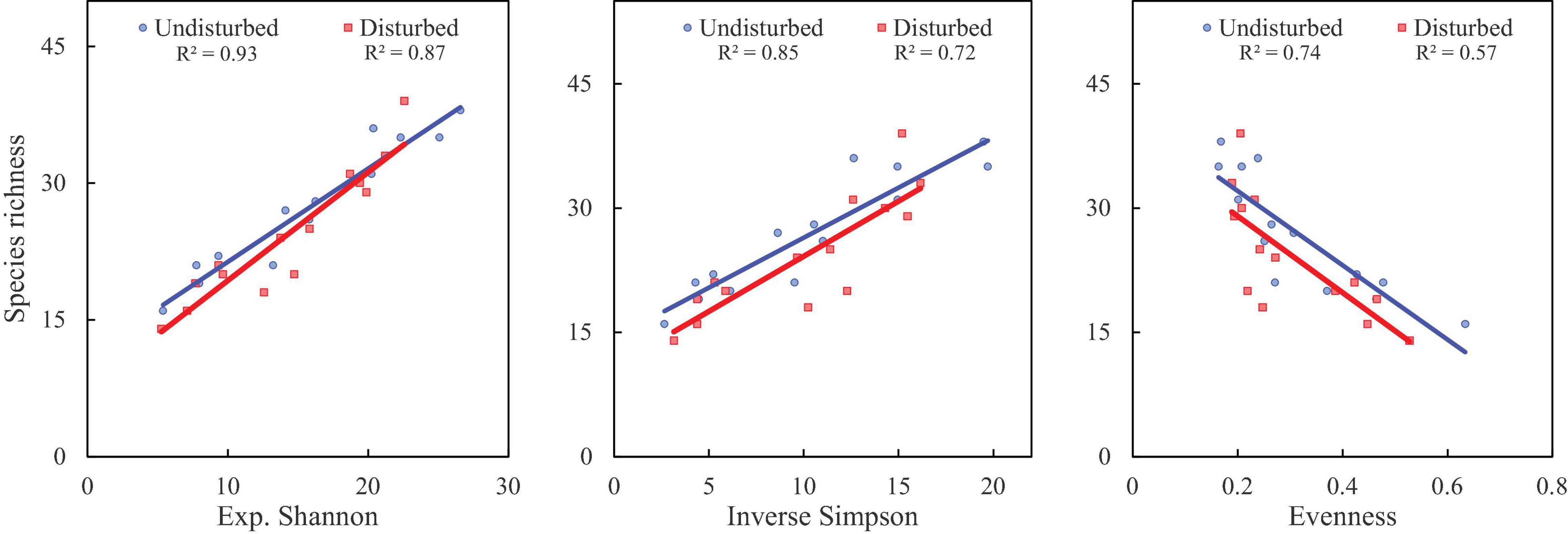
Figure 5. Correlation analysis of species richness with diversity indices (as per Hill numbers) in disturbed and undisturbed sites in GHNP. The linear fits and r2 values (at a p-value < 0.05) are presented in boxes.
Beta diversity values of herb and shrub in VoFNP ranged between 0.36–1 and 0.27–1, respectively, in undisturbed sites and 0.29–0.94 and 0.09–1, respectively, in disturbed. In both sites, the value of beta diversity was low in the middle elevation (3,800–3,900 m) which shared a large number of herb species, 17 herb species in undisturbed site, and 15 herb species in disturbed sites (refer to Supplementary Tables 2, 3). In undisturbed sites, beta diversity of shrub was low in the middle elevation (3,500–3,600 m) which shared a large number of species and in the disturbed sites, the beta diversity was low in 3,300- to 3,400-m-elevation gradient showing high number of shrub species (refer to Supplementary Tables 4, 5). Beta diversity increased gradually with the elevation gradient in both the sites of the national parks. The species replacement rate of herb and shrub was 100% in undisturbed and disturbed site.
In GHNP, the beta diversity values of herb and shrub ranged between 0.67–1 and 0.33–1, respectively, in undisturbed site and 0.27–1 and 0.11–1, respectively, in disturbed. In both sites, the value of beta diversity was low in the middle elevation (3,500 and 3,600 m) gradient which shared a large number of herb species, 9 herb species in undisturbed site, and 19 herb species in disturbed site (refer to Supplementary Tables 6, 7). In undisturbed site, beta diversity of shrub was low in the middle elevation (3,800 m) which shared a large number of species and in the disturbed sites, the beta diversity was low in 3,700- to 3,800-m elevation gradient showing high number of shrub species (refer to Supplementary Tables 8, 9).
The sample completeness or sample coverage curve (Figure 6) shows how sample coverage varies with the number of individuals, extrapolated up to sample size of 3,000. For any fixed number of individuals, the curve of the disturbed site lies consistently above that of undisturbed, but there is few differences between the two curves when the number of individuals exceeds 700 in VoFNP and 1,200 in GHNP. For the VoFNP sites, when the number of individuals is extended from 1,000 to 2,500, the sample coverage extends from 95 to almost 100%. For the GHNP sites, on the other hand, when the sample size is extended above 1,339 and 1,489, the coverage is extended from 94 to 99% (as shown in the unreported iNEXT output).
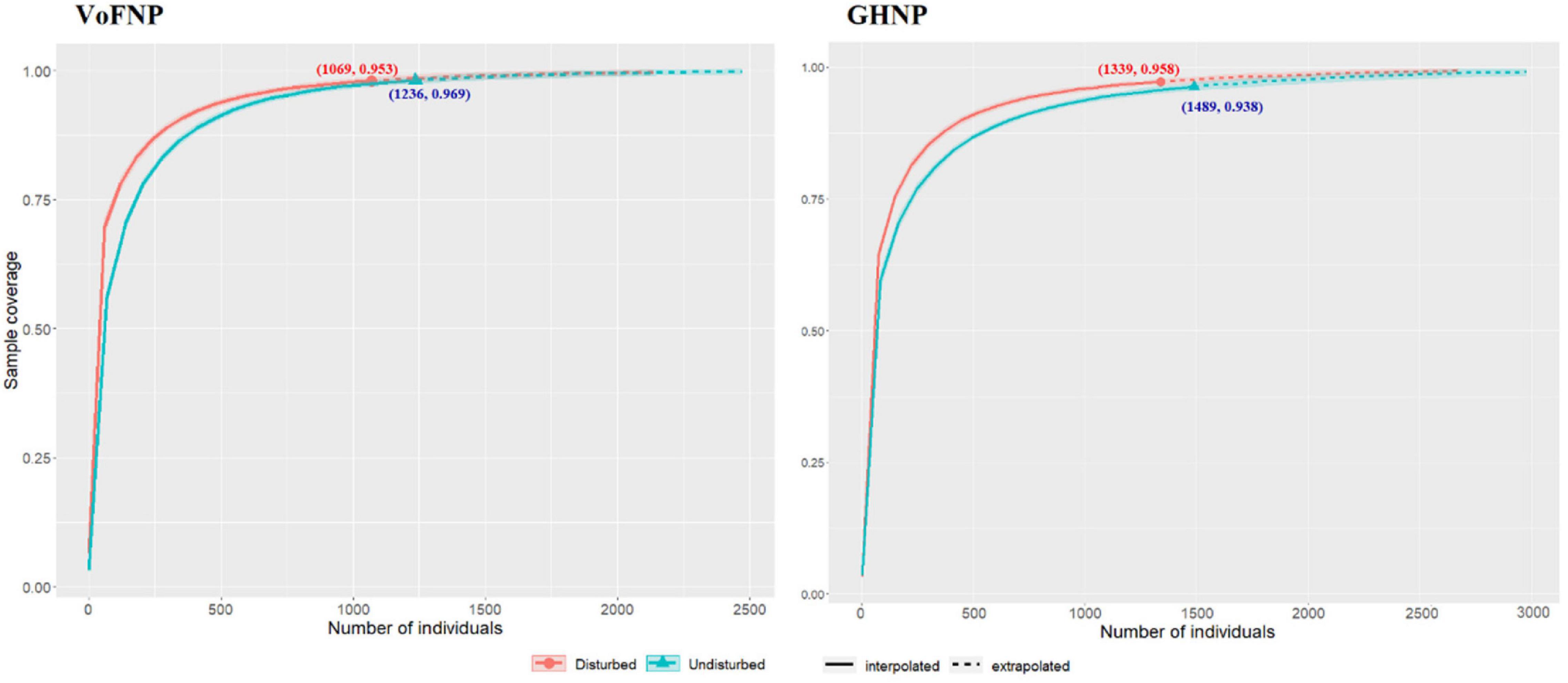
Figure 6. Sample coverage for rarefied samples (solid line) and extrapolated samples (dotted line) as a function of sample size for disturbed and undisturbed sites in VoFNP and GHNP. 95% confidence intervals (shaded areas) are obtained by a bootstrap method based on 200 replications.
Compositional Features of Representative Disturbed and Undisturbed Sites
Each of the representative transect (disturbed and undisturbed) was analyzed for vegetation composition along altitude gradient (3,200–4,500 m) and the dominant or codominant species are listed in Table 1, along with the Important Value Index (IVI). In VoFNP, in undisturbed study plots, there was a dominance of Potentilla atrosanguinea, Potentilla argyrophylla, Phleum alpinum, Geranium pratense, Geranium wallichianum, etc. toward lower elevation, while Corydalis meifolia, Potentilla cuneata, Bistorta vivipara, Rhodiola wallichiana, etc. were dominant toward higher elevations. This was totally different from the species composition in undisturbed sites, where the major dominance of Polygonum polystachyum, Impatiens sulcata, Polygonum affinis, and Rumex nepalensis was seen at all elevations. Similarly, in undisturbed study plots in GHNP, Potentilla atrosanguinea, Phleum alpinum, Polygonum affinis, Potentilla argyrophylla, Phleum alpinum, Geranium pratense, Geranium wallichianum, etc. were dominant, while in disturbed plots, Polygonum polystachyum, Impatiens scabrida, Impateins sulcata, etc. were dominant. However, toward higher elevations (>3,900 m), Potentilla argyrophylla and Polygonum affinis were more prominent. Furthermore, shrub species did not exhibit any such difference in composition between disturbed and undisturbed plots in both national parks. Major dominant shrubs included Cassiope fastigata, Rhododendron hypenanthum, Juniperus communis, Gaultheria trichophylla, Astragalus candolleanus, Lonicera myrtillus, etc. in the study area.
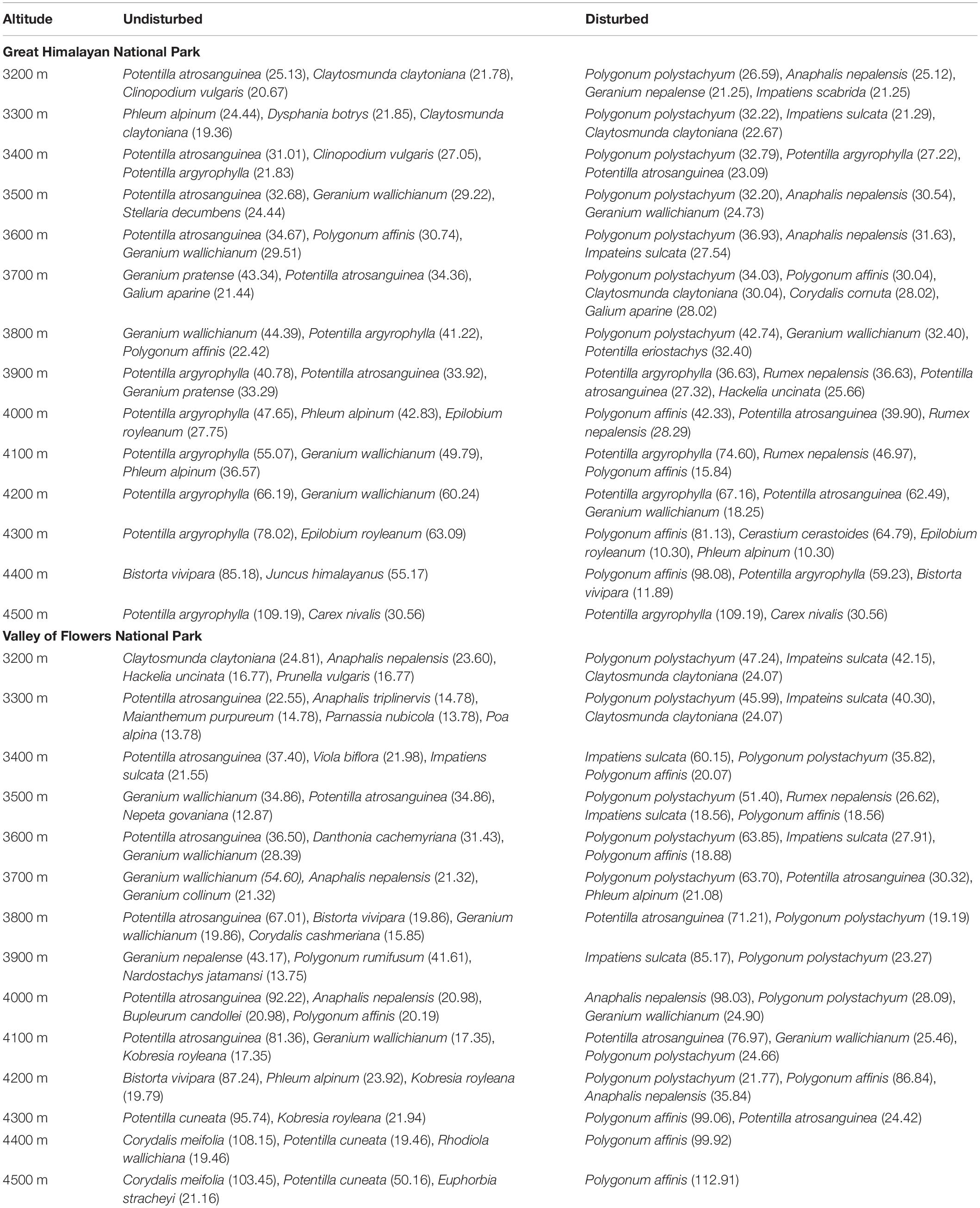
Table 1. Dominant and co-dominant plant taxa in the disturbed and undisturbed sites of GHNP and VoFNP.
Soil Characteristics of Undisturbed and Disturbed Sites
The general trends of soil parameters of undisturbed and disturbed sites of VoFNP, i.e., soil temperature, pH, and total nitrogen, showed decrease in concentration with increasing elevation, while phosphorous and potassium content increased with elevation (Figure 7). However, porosity increased with elevation in disturbed sites, while it decreased in undisturbed sites. A total of two of the important soil characteristics differentiating undisturbed and disturbed sites were porosity and organic carbon. Porosity was significantly higher (63.34–88.75%) in undisturbed sites compared to disturbed sites (range 0.29–4.63%) across the elevation transect of the VoFNP (p < 0.05). Organic carbon gradually increased with elevation in undisturbed sites and decreased with disturbed site. Porosity and organic carbon were inversely proportional for both the sites of the VoFNP. As for soil texture, sand was higher in disturbed site (3.20–83.78%) as compared to undisturbed sites (6.26–70.20%). Silt increased with elevation in undisturbed site while it showed no trend in the disturbed sites, and clay decreased with elevation in undisturbed sites and increased in disturbed sites of the VoFNP. However, soil moisture, bulk density, sand, phosphorus, and potassium did not show any trend with elevation in both sites. As compared to disturbed sites, the undisturbed sites exhibited higher values of soil moisture, bulk density, porosity, potassium content, and phosphorous content (Figure 7). Most of the soil physicochemical parameters were found significantly higher for undisturbed site compared to disturbed site. The correlation matrix between diversity indices and soil parameters of VoFNP is provided in Table 2. It could be noted that, irrespective of indices, undisturbed diversity had statistically significant (p-value < 0.05) highly positive correlation with (i) temperature and (ii) nitrogen, whereas a high negative correlation was observed with phosphorus. Similarly, disturbed diversity had statistically significant (p-value < 0.05) high-positive correlation with (i) temperature, (ii) bulk density, (iii) porosity, (iv) nitrogen, and (v) organic carbon. The average dependence of disturbed diversity on soil temperature was noted to be higher than that of undisturbed sites.
In GHNP, the soil parameters for undisturbed and disturbed sites, i.e., soil temperature and organic carbon decreased with increasing elevation while pH and clay content increased (Figure 8). In undisturbed sites, soil moisture, soil temperature, bulk density, porosity, silt, clay, organic carbon, and nitrogen were significantly higher compared to disturbed sites, whereas pH, phosphorus, and potassium were higher in disturbed sites. Organic carbon gradually increased with elevation in undisturbed sites and decreased in disturbed sites. As for soil texture, sand was high in disturbed sites (14.80–83.78%) as compared to undisturbed sites (10.00–38.00%). Silt increased with elevation in undisturbed sites while it showed no trend in disturbed sites, and clay decreased with elevation in undisturbed and increased in disturbed sites (Figure 8). In GHNP, only disturbed diversity had statistically significant (p-value < 0.05) high-positive correlation with (i) temperature and (ii) organic carbon (Table 3).
Non-metric Multidimensional Scaling
Non-metric multidimensional scaling of vegetation abundance and elevation for undisturbed and disturbed sites exhibited a clear difference in species composition along the elevation gradient for VoFNP (refer to Supplementary Figures 10, 11). It showed a strong gradient separating samples (quadrats) and species from low to high altitude along the axes. For herbs, most of the plots at the lower altitude formed a distinct cluster (E2, E3, E4, E5, and E6) at the negative end of first axis (NMDS1), and those lying at higher altitude formed a distinct cluster at the positive end of first axis (NMDS2) (E12, E13, and E14) for undisturbed site. For disturbed sites, plots at the lower altitude formed a distinct cluster (E1, E2, E3, E4, E5, E6, and E8) at the negative end of first axis (NMDS1), and those lying at higher altitude formed a distinct cluster at the positive end of first axis (NMDS2) (E11, E12, E13, and E14). Apart from this, E7, E9, and E10 occupied intermediate position along the axes. For undisturbed sites, the clusters were more scattered, while for disturbed sites, only three distinct clusters were recognized. Thus, it can be inferred that the undisturbed sites were more heterogeneous compared to the disturbed sites forming more scattered clusters. Distribution of many species was seen to be associated with a particular elevation type. For undisturbed sites, Prunella vulgaris, Parnassia nubicola, Gypsophila cerastioides, Iris kumaonensis, Hackelia uncinata, Claytosmunda claytoniana, Morina longifolia, Gentiana argentea, Valeriana jatamansi, etc. exhibited high association in lower altitudes. Similarly, species exhibiting greater association with higher altitude included Saussurea obvallata, Arenaria bryophylla, Aconitum violaceum, Corydalis meifolia, Gentiana tubiflora, Oxyria digyna, Euphorbia stracheyi, Potentilla cuneifolia, etc. However, for disturbed sites, more species were associated with the lower elevation zone, such as Bupleurum candollei, Erigeron multiradiatus, Silene vulgaris, Acomastylis elata, Lamium album, Nepeta govaniana, Galium acuminatum, Verbascum thapsus, Morina longifolia, Hackelia uncinata, Valeriana jatamansi, etc. For higher elevation, Danthonia cachemyriana, Polygonum affine, Rhodiola wallichiana, Pedicularis punctata, and Potentilla cuneifolia exhibited greater association. Similarly, for shrubs of VoFNP, most of the plots at the lower altitude formed a distinct cluster (E1, E2, E3, E4, and E5) at the negative end of first axis (NMDS1), and those lying at higher altitude formed a distinct cluster at the positive end of first axis (NMDS2) (E8, E9, E10, E11, E12, E13, and E14) for both undisturbed and disturbed sites. Distribution of many species was seen to be associated with a particular elevation type.
Non-metric multidimensional scaling of vegetation abundance and elevation for undisturbed and disturbed sites of GHNP exhibited a clear difference in species composition along the elevation gradient (refer to Supplementary Figures 12, 13). In GHNP, negative end of the first axis (NMDS1) and those lying at higher altitude formed a dense cluster at the positive end of first axis (NMDS2) (E7, E9, E10, E11, E12, and E14). For disturbed sites, plots at the lower altitude formed a distinct cluster (E1, E2, E3, E4, E5, E6, and E7) at the negative end of first axis (NMDS1), and those lying at higher altitude formed a distinct cluster at the positive end of first axis (NMDS2) (E8, E10, and E11). For undisturbed sites, the clusters were more scattered, while for disturbed sites, only three distinct clusters were recognized. Thus, it could be inferred that the undisturbed sites were more heterogeneous compared to the disturbed sites forming more scattered clusters. A similar observation was also made for the VoFNP. Furthermore, for undisturbed sites of GHNP, Claytosmunda claytoniana, Corydalis govaniana, Epipactis helleborine, Hackelia uncinata, Impatiens sulcata, Ligularia amplexicaulis, Parochetus communis, Phlomis bracteosa, Selinum wallichianum, Trigonella emodi, Viola biflora, etc. exhibited high association in lower altitudes. Similarly, species exhibiting greater association with higher altitude included Arenaria festucoides, Aconitum violaceum, Corydalis meifolia, Jurinea macrocephala, Leontopodium himalayanum, Nardostachys jatamansi, Primula reptans, Saussurea obvallata, Saxifraga sibirica, etc. However, for disturbed sites, more species were associated with the lower elevation zone, such as Bupleurum candollei, Erigeron multiradiatus, Silene vulgaris, Acomastylis elata, Lamium album, Nepeta govaniana, Galium acuminatum, Verbascum thapsus, Morina longifolia, Hackelia uncinata, Valeriana jatamansi, etc. For higher elevation, Danthonia cachemyriana, Polygonum affine, Rhodiola wallichiana, Pedicularis punctata, and Potentilla cuneifolia exhibited greater association.
Similarly, for shrubs of GHNP, most of the plots at the lower altitude formed a distinct cluster (E1, E2, E3, E4, and E8) at the negative end of first axis (NMDS1), and those lying at higher altitude formed a distinct cluster at the positive end of first axis (NMDS2) (E9, E10, E11, and E12) for both undisturbed and disturbed sites. Distribution of many species was seen to be associated with a particular elevation type.
Canonical Correspondence Analysis
The effects of physicochemical parameters of soil on herb and shrub species compositions were analyzed using the CCA (Figures 9, 10) for disturbed and undisturbed sites of VoFNP. The CCA for herbs and shrubs indicated that the total inertias of the undisturbed and disturbed herb sites were 5.36 and 3.06, respectively. The constrained soil parameters explained 66 and 65% of the total variances of undisturbed and disturbed herb sites, respectively. It was also observed that the undisturbed herb diversities within the elevation range 3,200–3,500 m were dependent on nitrogen, phosphorus, potassium, pH, temperature, and porosity. Similarly, undisturbed herb diversities within the elevation range of 3,600–4,000 m had strong dependence with soil moisture. Undisturbed herb diversities above 4,000-m elevation were not having significant association with soil parameters. However, the disturbed herb diversities within the elevation range of 3,200–3,600 m were dependent on nitrogen, pH, organic carbon, and temperature. The disturbed herb diversities along the 3,900-m elevation were strongly associated with moisture and potassium; the total inertias of the undisturbed and disturbed shrub sites were 2.79 and 2.49, respectively. The constrained soil parameters were explaining 68 and 76% of the total variances of undisturbed and disturbed shrub sites, respectively. The CCA of VoFNP also revealed that the undisturbed and disturbed shrub diversities within the elevation range of 3,200–3,400 m were dependent on nitrogen and pH. Similarly, undisturbed shrub diversities within the elevation range of 3,500 m were having strong dependence with potassium. Undisturbed and disturbed shrub diversities above 4,000-m elevation had significant association with phosphorus and soil moisture.
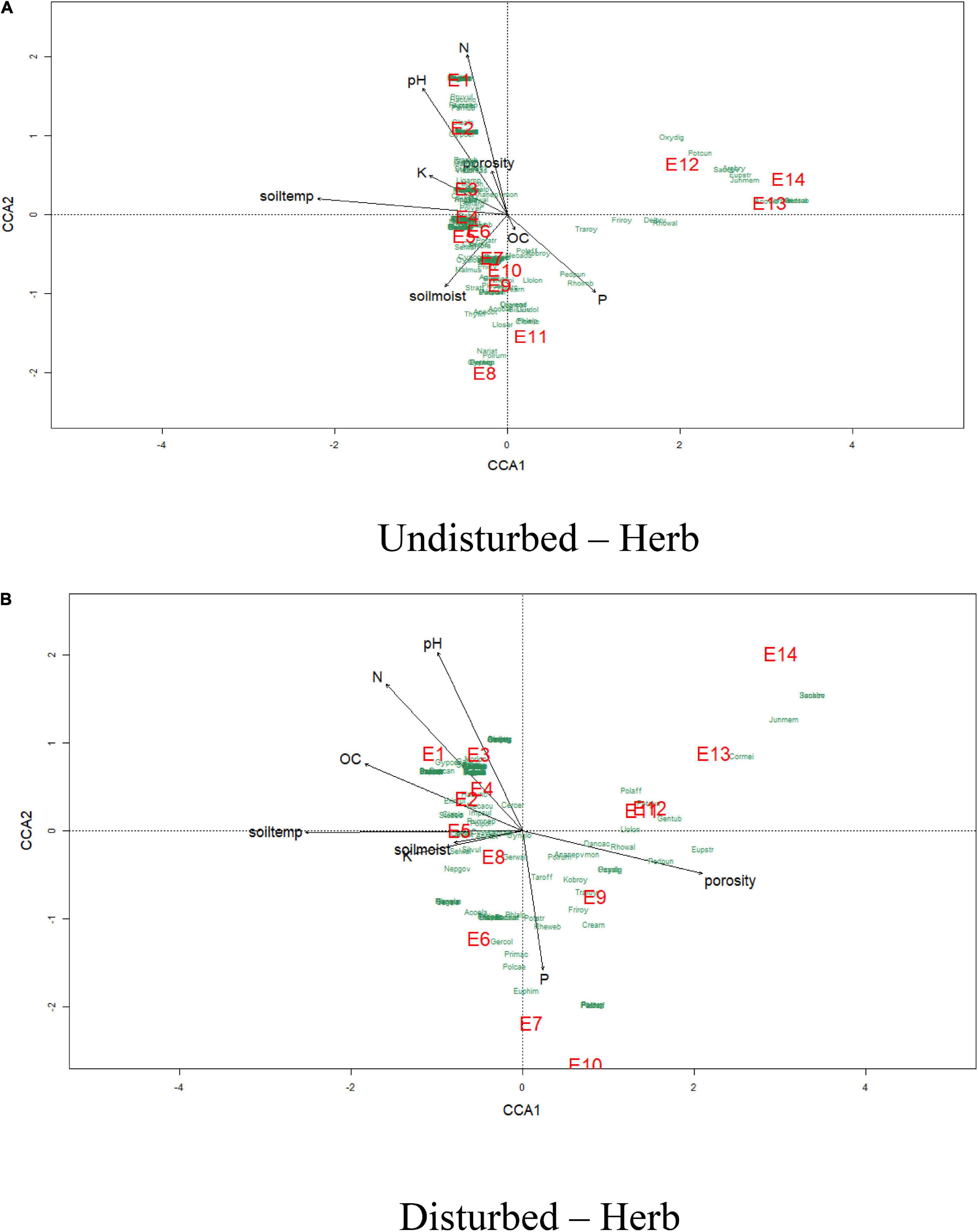
Figure 9. Canonical correspondence analysis of vegetation abundance and elevation (represented by E) overlaid to soil parameters at a p.max = 0.25 for undisturbed and disturbed quadrates of herbs in VoFNP.
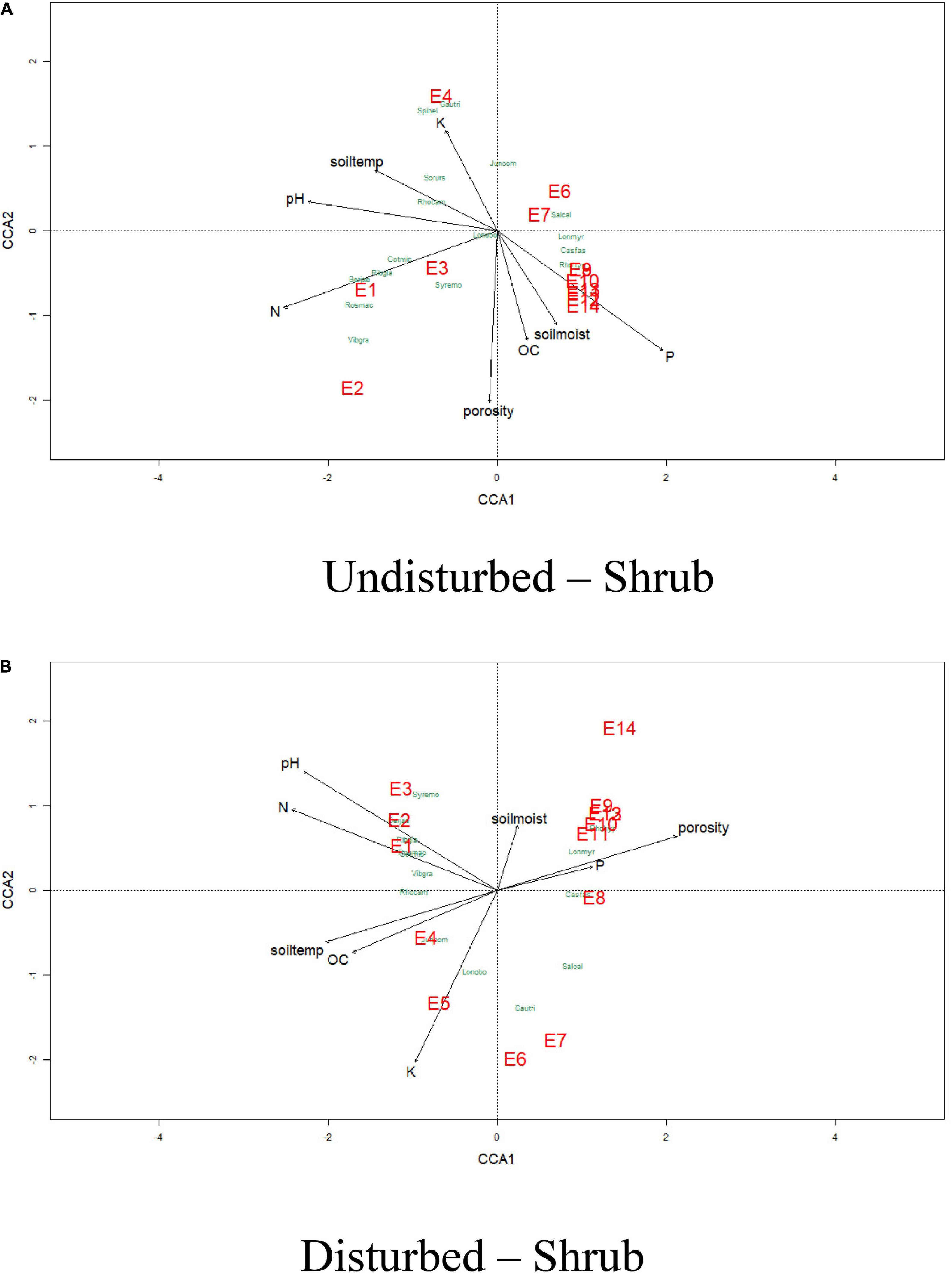
Figure 10. Canonical correspondence analysis of vegetation abundance and elevation (represented by E) overlaid to soil parameters at a p.max = 0.25 for undisturbed and disturbed quadrates of shrubs in VoFNP.
The CCA for herbs of GHNP indicated that the total inertias of the undisturbed and disturbed herb sites were 5.28 and 4.40, respectively (Figures 11, 12). The constrained soil parameters were explaining 54 and 50% of the total variances of undisturbed and disturbed herb sites, respectively. Further, the undisturbed herb diversities within the elevation range of 3,200–3,300 m were depending on sand and bulk density. Soil characteristics of sites at 3,500-m elevation were dominated by organic carbon, whereas the same at the elevation transects of 3,600 m dominated by phosphorus, and for elevations 3,400, 3,700, and 3,900 m dominated by pH. Similarly, herb density of 3,900-m elevation was having strong dependency on clay. Herb densities above 4,000-m elevation were having significant association with silt and nitrogen. However, the disturbed herb diversities within the elevation range of 3,300 and 3,500 m were noted to be dependent on pH. Soil properties of sampling sites at the 3,300, 3,700, 3,800, and 3,900-m elevations were strongly dependent to bulk density, phosphorus, potassium, and nitrogen, respectively, whereas the same at the 4,100- and 4,200-m elevations were noted to be dependent on sand (Figure 11). CCA further revealed that the total inertias of the undisturbed and disturbed shrub sites were 5.21. The constrained soil parameters were explaining 30 and 41% of the total variances of undisturbed and disturbed shrub sites, respectively, whereas the undisturbed shrub diversities within the elevation range of 3,200 and 3,500 m were dependent on bulk density and sand. Soil characteristics of the sampling sites at 3,500-m elevation were dependent on organic carbon. The same at 3,700 and 3,600-m elevations were noted to have strong dependency to the soil moisture and potassium, respectively. Furthermore, shrub density of 3,800-m elevation was noted to have strong dependence with the silt. Similarly, the shrub densities above 4,000-m elevation were having significant association with clay and nitrogen. However, the disturbed shrub diversities within the elevations of 3,600 and 3,800 m were noted to be dependent on clay and silt. The shrub densities above 4,200-m elevation were having significant association with nitrogen (Figure 12).
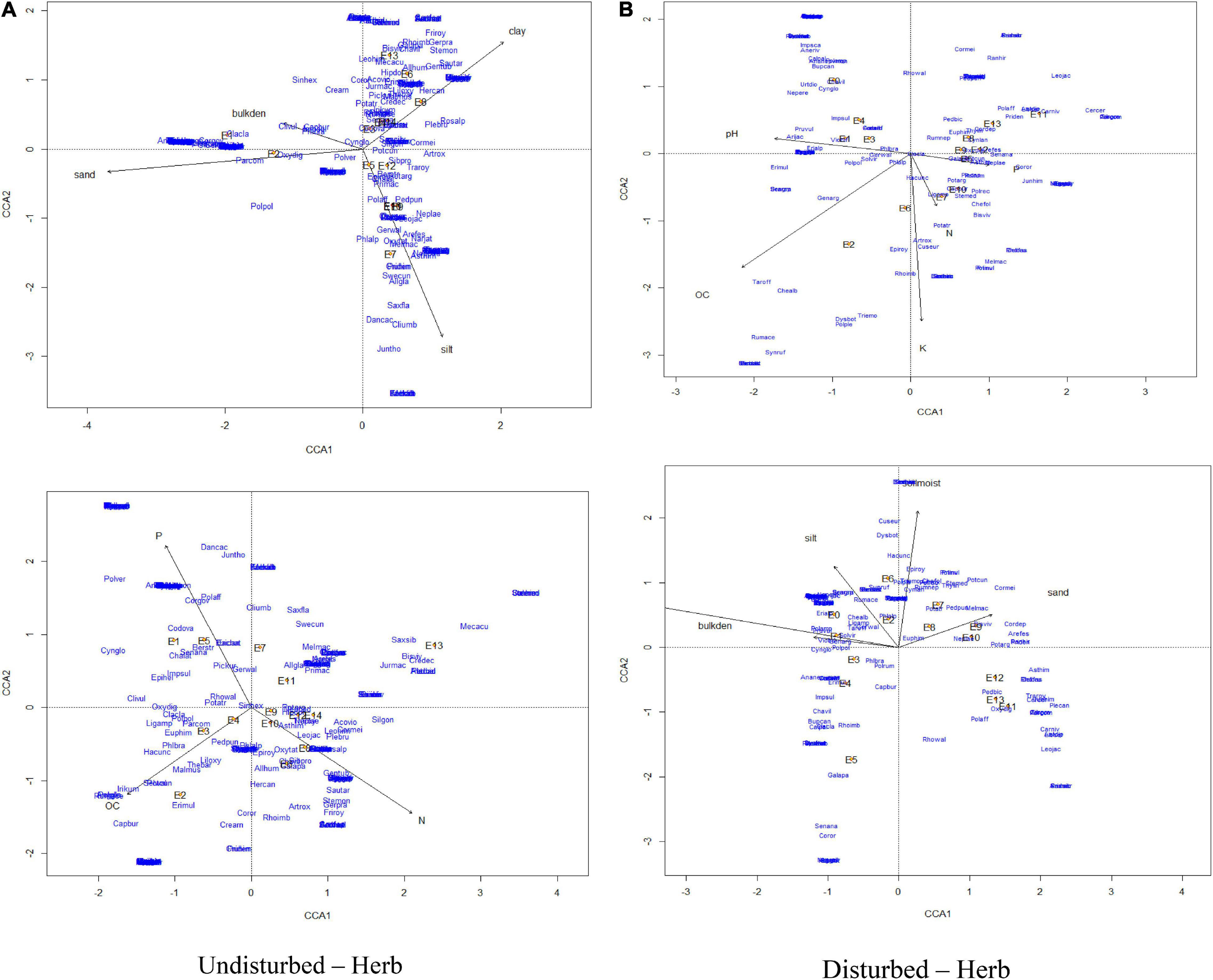
Figure 11. Canonical correspondence analysis of vegetation abundance and elevation (represented by E) overlaid to soil parameters at a p.max = 0.25 for undisturbed and disturbed quadrates of herbs in GHNP.
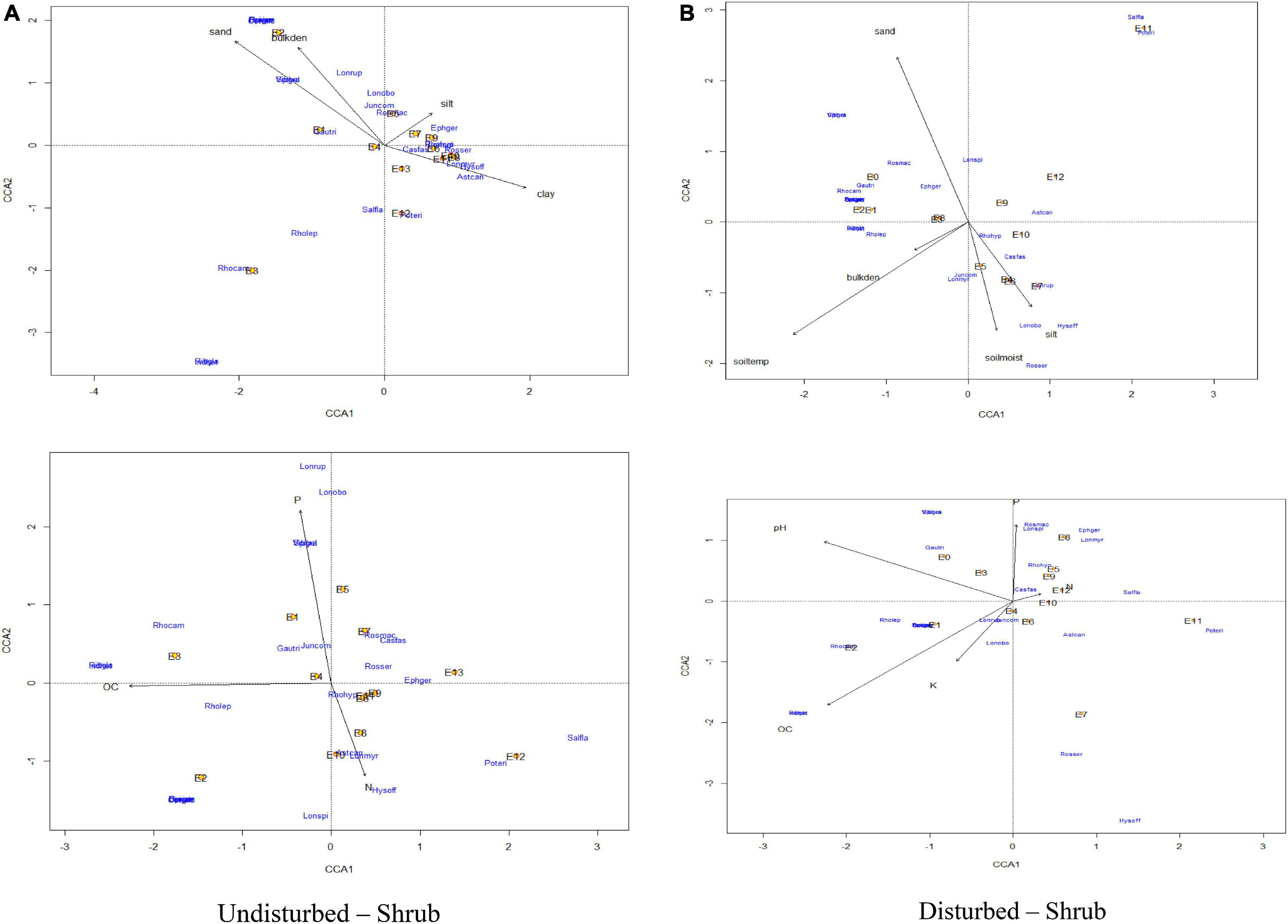
Figure 12. Canonical correspondence analysis of vegetation abundance and elevation (represented by E) overlaid to soil parameters at a p.max = 0.25 for undisturbed and disturbed quadrates of shrubs in GHNP.
Discussion
Floristic Diversity, Threatened and Invasive Species
A total of 614 taxa belonging to 277 genera, 70 families, and having 5 endemic and 31 threatened species have been documented in the VoFNP by Bisht et al. (2018). However, this study documented only 25% of the flora reported in Bisht et al. (2018) in the VoFNP, irrespective of disturbed and undisturbed sites. As per the recent datasets of threatened plants of IHR (Mehta et al., 2020), the study sites of VoFNP harbors 26 (5.7%) taxa. Moreover, the relationship between the degree of site disturbance and species richness is notable among the plant communities. Hence, higher species richness in undisturbed sites can be attributed to minimal or no human interferences in the area leading to an intact natural habitat. Anthropogenic activities viz. trampling, unsustainable harvesting for research-oriented work, etc. are the major causes of habitat depletion in protected sites of VoFNP. Dense colonies of weedy (i.e., Polygonum polystachyum, Impatiens sulcata, and Claytosmunda claytoniana) and invasive (Cuscuta europaea) species as well were prominent in the disturbed sites especially in 3,200–3,500-m elevation zone. Thus, species paucity recorded in the lower altitude zone in disturbed sites could be assigned to the high levels of anthropogenic disturbance and lesser plant diversity and richness of threatened, endemic, and native species (Naithani et al., 1992; Joshi and Gairola, 2003). Apart from this, 23 species recorded by Smythe (1938) in the valley could not be traced in recent explorations (Bisht et al., 2018). Along the altitude gradient, increasing values of beta diversity in both sites indicates heterogenous plant composition and occurrence of rare taxa at high altitudes as compared to lower elevations (Grime, 1998; Saha et al., 2016; Rawal et al., 2018; Bhat et al., 2020a,b; Thakur et al., 2021). While there is greater human interference in lower altitude zone, excessive habitat depletion could lead to homogenous composition. Similarly, disturbed sites with lower beta diversity, in comparison with that of undisturbed sites, exhibit high species packaging and homogenous composition with dominance of invasive and weedy species. Kueffer et al. (2014) also documented high level of invasion in lower elevations attributed to the increase in tourism, recreation, and residential habitation in mountains across the world.
Kala (1999) had reported the presence of aggressive Polygonum polystachyum (Himalayan knotweed) up to 3,800-m elevation, but we could found weedy species till 4,200-m elevation, implying that these species are moving toward higher elevations in the recent times and suppressing the native, threatened, and endemic population (Kala and Mathur, 2002). The manual removal of P. polystachyum has also led to enhancement in the density of Impatiens sulcata which is a fast-growing species in alpine region and a feed to migratory livestock. Therefore, a complete prohibition of migratory livestock grazing in the park may not be advised (Kala, 2005).
In disturbed sites, anthropogenic activities, such as trampling, reduce vegetation cover causing soil compaction (Bernstein and Svenning, 2018) and decrease in porosity. Porosity decrement is known to abate proliferation of seed-growing plants, while non-native or invasive species such as Polygonum polystachyum (Himalayan knotweed) spread uninterruptedly by thick rhizomes (Negi et al., 2017). For proper management of the national park, the continuous monitoring of plant diversity, especially the distribution of invasive, threatened, and endemic species, is essential. On average, anthropogenic disturbances are noted to reduce 49.4% threatened and 16.7 and 55% endemic shrubs and herbs; respectively.
Relation Between Floristic Diversity and Soil
The physicochemical properties of soil determine its nature and nutrient status (Jongmans et al., 2001), which are responsible for controlling erosion and increasing fertility of soil (Sharma and Bhatia, 2003). These properties are largely influenced by biotic and abiotic factors such as topography-induced microclimate differences, physiographic position, altitude, parent material, and vegetation community (Smeck, 1985; Johnson et al., 2000; Behari et al., 2004). In case of the microclimatic variations, altitude is the most important factor that influences weathering rates and leaching intensity which causes variations in soil parameters such as porosity, pH, organic matter content, and so on (Hutchins et al., 1976; Dahlgren et al., 1997). In this study, soil temperature, pH, and nitrogen content decreased with rising altitude while phosphorous content and potassium content increased. According to Sehgal et al. (1990) and Naiman et al. (1994), higher levels of organic matter have greater number of cation exchange, due to which pH decreases. The decrease in nitrogen content with altitude can be correlated with decreasing richness leading to lesser litter fall (Chen et al., 2000). Availability of potassium was also reported to increase with altitude in Garhwal Himalaya (Jha et al., 2004). Furthermore, the macronutrients, i.e., nitrogen, phosphorus, and potassium, which are required in large quantities to support plant growth and development, are directly taken from soil in the form of nitrate, nitrite, etc. (Reich et al., 1997; Schimel and Bennett, 2004) and are influenced by disturbances. In mountain ecosystem, nitrogen content affects the productivity, species diversity, community succession, and sustainability (Vitousek and Howarth, 1991). Similarly, potassium is vital in metabolic processes, influencing enzyme activity (Brady et al., 2008), water regulation, fruit development, and resistance to insects and diseases. Ecological studies on soil show that nitrogen and potassium availability and net mineralization rates vary not only with species composition but also with altitude and other geographical features of the forest (Zhong and Makeschin, 2004). In this study, we have found a positive correlation of nitrogen (p < 0.05) and potassium content with species richness in both disturbed and undisturbed sites. Apart from this, decomposition of plant and animal materials is responsible for the accumulation of organic matter and acts as a sink and source of carbon (Kirschbaum, 2000). Increase in soil carbon stock with elevation corresponds to the higher decomposition rate of organic content and has been previously reported by Lal (2005) for forest ecosystem. Our results also exhibit strong correlation between OM and soil carbon. In the undisturbed site, carbon content and nitrogen content greatly influence the species richness, while in disturbed sites, nitrogen and phosphorus were the factors that influence richness.
However, effect of physicochemical parameters of soil on plant species composition, performed by CCA, showed that soil moisture and pH were the most important variable exhibiting higher correlation in species composition in disturbed sites, while it was porosity, pH, and soil moisture showing higher correlation in undisturbed site. Further, the CCA also shows that, irrespective of soil physicochemical properties, the undisturbed herb diversity had higher affiliation to soil parameters than disturbed. However, unlike the herbs, shrub diversities had lesser affiliation to soil properties, and the undisturbed shrub diversity had marginally higher affiliation to soil parameters than disturbed.
Anthropogenic Pressure and Its Management
Natural calamities, human interferences, tourism pressure, and weedy invasions are the major instabilities in disturbed sites leading to decreased richness and diversity of threatened, endemic, and native species. As per the visitation data of VoFNP and GHNP acquired from Forest Department, Joshimath and DFO office, Shamshi, Kullu, there has been a continuous increase in the intensity of tourists visiting the protected national parks over a period of 2010 to 2017 (refer to Supplementary Figure 14), especially during the month of August–September (peak flowering season). With an average of 13,000 and 1,200 tourists in VoFNP and GHNP, respectively, the former can be said to be more prone to habitat and species loss. Although religious tourism is the major draw, the VoFNP is also important because the valley attracts a large number of foreign tourists and nature lovers. An increase in the number of tourists has greatly influenced the threatened medicinal plants of the area. Major segment of the villagers (95%) of the buffer zone depends on the tourists who visited this area in large numbers every year. This excessive pressure of tourism not only affects the angiosperm diversity but also the lichen (Rawat and Upreti, 2014) which are known to be the pioneer of succession. Therefore, there is an urgent need to conserve the endemic and threatened plants in VoFNP for the conservation of plant diversity, and the following conservation and management actions are proposed, (i) to monitor the population of invasive, threatened, and endemic species, (ii) proper control of opportunistic species frequently, (iii) to follow the strict rules and regulations for the maintenance of World Heritage Site and give permission to limited tourists during peak months to visit, (iv) tourists should not be allowed garbage accumulation, plastic disposals inside the park, and (v) encourage or inculcate awareness to local people, forest personals, and nature guides for the identification of plants and conservation importance of VoFNP.
Conclusion
This study documented significantly higher plant species richness and diversity in the undisturbed sites as compared to sites under human interferences by tourism activities, trampling, and over-exploitation causing habitat degradation. This has also given way to establishment of higher densities of weedy and invasive species such as Polygonum polystachyum, Impatiens sulcata, Claytosmunda claytoniana, Chenopodium album, Cuscuta europaea, Rumex nepalensis, and Urtica dioica. An assessment of tourism strength in both the PAs revealed a higher number of tourists in VoFNP as compared to GHNP, thus making the former more vulnerable to habitat degradation and fragmentation due to trampling. For better conservation of plant diversity in the target area, the following conservation strategy has been proposed: (i) promoting sustainable livestock grazing and utilization of alpine resources in the buffer zone area to minimize the population of fast-growing species and promoting unique or native species; (ii) monitoring the threatened, endemic, invasive, and fast-growing species in the park area in particular year interval with proper scientific approaches; (iii) promoting cultivation of endemic, threatened, and high-value medicinal plants in nearby village or barren area to reduce the collection form wild or natural population; (iv) educating the local or nearby inhabitants about the floristic wealth and importance of the national park for biodiversity conservation, and (v) promoting adventure- and nature-based tourism in the park area.
Data Availability Statement
The original contributions presented in the study are included in the article/Supplementary Material, further inquiries can be directed to the corresponding author/s.
Author Contributions
MB and KCS conceptualized the study. SM and NT involved in statistical analysis and interpretation. AB, DS, PB, PM, SU, and DD contributed in field data collection. All authors contributed to the article and approved the submitted version.
Funding
We wish to acknowledge the National Mission on Himalayan Studies (NMHS/MG-2016/006) for funding support.
Conflict of Interest
The authors declare that the research was conducted in the absence of any commercial or financial relationships that could be construed as a potential conflict of interest.
Publisher’s Note
All claims expressed in this article are solely those of the authors and do not necessarily represent those of their affiliated organizations, or those of the publisher, the editors and the reviewers. Any product that may be evaluated in this article, or claim that may be made by its manufacturer, is not guaranteed or endorsed by the publisher.
Acknowledgments
We thank Kireet Kumar, Director-in-Charge and R. S. Rawal, Former Director, G.B. Pant National Institute of Himalayan Environment for providing necessary facilities and support. We highly acknowledge the support received from Kumar Ambrish, Scientist-E, Botanical Survey of India for identification of plant specimens as well as necessary permission for herbarium consultation. Uttarakhand and Himachal Pradesh Forest Departments for necessary permissions and Deputy Conservator of Forest, Forest officials in Valley of Flowers National Park and Great Himalayan National Park for providing logistic help and field guidance are highly acknowledged.
Supplementary Material
The Supplementary Material for this article can be found online at: https://www.frontiersin.org/articles/10.3389/fevo.2022.751989/full#supplementary-material
Footnotes
References
Behari, B., Aggarwal, R., Singh, A. K., and Banerjee, S. K. (2004). Spatial variability of pH and organic carbon in soils under bamboo-based agroforestry models in a degraded area. Indian For. 130, 521–529.
Bernstein, M., and Svenning, J. C. (2018). Human path has positive impacts on plant richness and diversity, a meta-analysis. Ecol. Evol. 8, 11111–11121. doi: 10.1002/ece3.4578
Bhat, J. A., Kumar, M., Negi, A. K., Todaria, N. P., Malik, Z. A., Pala, N. A., et al. (2020a). Species diversity of woody vegetation along altitudinal gradient of the Western Himalayas. Glob. Ecol. Conserv. 24, 1–17.
Bhat, J. A., Kumar, M., Pala, N. A., Shah, S., Dayal, S., Gunathilake, C., et al. (2020b). Influence of altitude on the distribution pattern of flora in a protected area of Western Himalaya. Acta Ecol. Sin. 40, 30–43. doi: 10.1016/j.chnaes.2018.10.006
Bhatt, D., Chandra Sekar, K., and Rawal, R. S. (2021). Tree diversity, congruence and endemism: Himalaya ‘The land of diversity’. Biodivers. Conserv. 30, 2633–2654. doi: 10.1007/s10531-021-02227-2
Bisht, M., Chandra Sekar, K., Kant, R., Ambrish, K., Singh, P., and Arya, D. (2018). Floristic diversity in Valley of Flowers National Park, Indian Himalayas. Phytotaxa 379, 1–26. doi: 10.11646/phytotaxa.379.1.1
Black, C. A., Evans, D. D., White, J. L., Ensminger, L. E., Clarck, F. E., and Dinaver, R. C. (1965). Methods of Soil Analysis. Madison, WI: American Society of Agronomy.
Brady, N. C., Weil, R. R., and Weil, R. R. (2008). The Nature and Properties of Soils. Upper Saddle River, NJ: Prentice Hall.
Cassel, D. K., and Nielsen, D. R. (1986). Field capacity and available water capacity. Methods Soil Ana Part 1 Phys Mineral Methods, 5, 901–926.
Chandra Sekar, K., Manikandan, R., and Srivastava, S. K. (2012). Invasive alien plants of Indian Himalayan Region. Proc. Natl. Acad. Sci. India Section B Biol. Sci. 82, 375–383.
Chandra Sekar, K., Pandey, A., and Giri, L. (2014). Floristic diversity in Milam Valley: a cold desert region of Uttarakhand. Int. J. Eng. Res. 2, 143–147.
Chao, A., and Colwell, R. K. (2017). Thirty years of progeny from Chao’s inequality: estimating and comparing richness with incidence data and incomplete sampling. SORT, 3–54.
Chao, A., Chiu, C. H., and Jost, L. (2014). Unifying species diversity, phylogenetic diversity, functional diversity, and related similarity and di_erentiation measures through hill numbers. Annu. Rev. Ecol. Evol. Syst. 45, 297–324. doi: 10.1146/annurev-ecolsys-120213-091540
Chen, C. R., Condron, L. M., Davis, M. R., and Sherlock, R. R. (2000). Effects of afforestation on phosphorus dynamics and biological properties in a New Zealand grassland soil. Plant Soil 220, 151–163.
Cole, L., Buckland, S. M., and Bardgett, R. D. (2008). Influence of disturbance and nitrogen addition on plant and soil animal diversity in grassland. Soil Biol. Biochem. 40, 505–514. doi: 10.1016/j.soilbio.2007.09.018
Dahlgren, R. A., Boettinger, J. L., and Huntington, G. L. (1997). Soil development along an elevational transect in. Geoderma 78, 207–236. doi: 10.1016/s0016-7061(97)00034-7
Floeter, S. R., Ferreira, C. E. L. Dominici-Arosemena, A., and Zalmon, I. R. (2004). Latitudinal gradients in Atlantic reef fish communities: trophic structure and spatial use patterns. J. Fish. Biol. 64, 1680–1699.
Gairola, S., Rawal, R. S., Todaria, N. P., and Bhatt, A. (2014). Population structure and regeneration patterns of tree species in climate- sensitive subalpine forests of Indian western Himalaya. J. For. Res. 25, 343–349.
Gao, Q. Z., Wan, Y. F., Xu, H. M., Li, Y., Jiangcun, W. Z., and Borjigidai, A. (2010). Alpine grassland degradation index and its response to recent climate variability in Northern Tibet. China. Q. Int. 226, 143–150. doi: 10.1016/j.quaint.2009.10.035
Gaston, A. J., and Garson, P. J. (1993). A re-Appraisal on the Great Himalayan National Park. Report to HPDFFC. International Trust for Nature Conservation. Thiruvananthapuram: WWF-India.
Gaur, R. D. (1999). Flora of the District Garhwal North West Himalaya: With Ethnobotanical Notes. Srinagar: TransMedia.
Ghildiyal, B. N. (1957). A botanical trip to the valley of flowers. J. Bombay Natural Hist. Soc. 54, 365–386.
Goswami, N., and Das, D. (2018). Status study of medicinal plants at Purnapani forest of Jhargram in West Bengal. J. Pharm. Bio. Sci. 13, 1–12.
Grime, J. P. (1998). Benefits of plant diversity to ecosystems: immediate, filter and founder effects. J. Ecol. 86, 902–910. doi: 10.1046/j.1365-2745.1998.00306.x
Hassan, M. O., and Hassan, Y. M. (2019). Effect of human activities on floristic composition and diversity of desert and urban vegetation in a new urbanized desert ecosystem. Heliyon 5, 1–10. doi: 10.1016/j.heliyon.2019.e02283
Hutchins, R. B., Blevins, R. L., Hill, J. D., and White, E. H. (1976). The influence of soils and microclimate on vegetation of forested slopes in eastern Kentucky. Soil Sci. 121, 234–241. doi: 10.1097/00010694-197604000-00008
IUCN (2020). The IUCN Red List of Threatened Species, Version 2014.3. Available online at: http://www.iucnredlist.org (accessed November 10, 2019).
Jain, S. K., and Rao, R. R. (1977). A Handbook of Field and Herbarium Methods. New Delhi: Today and Tomorrow’s Printers and Publishers.
Jha, M. N., Prasad, A. N., and Misra, S. K. (2004). Influence of source of organics and soil organic matter content on cyanobacterial nitrogen fixation and distributional pattern under different water regimes. World J. Microbiol. Biotechnol. 20, 673–677. doi: 10.1007/s11274-004-2157-9
Johnson, D. W., Cheng, W., and Burje, I. C. (2000). Biotic and abiotic nitrogen retention in a variety of forest soils. Soil Sci. Soc. Am. J. 64, 1503–1514. doi: 10.2136/sssaj2000.6441503x
Jongmans, A. G., Pulleman, M. M., and Marinissen, J. C. Y. (2001). Soil structure and earthworm activity in a marine silt loam under pasture versus arable land. Biol. Fertil. Soil 33, 279–285. doi: 10.1007/s003740000318
Joshi, S. K., and Gairola, S. (2003). Cuscuta europaea Linn. (Dodder plant), an emerging threat to plant diversity of Valley of Flowers. Curr. Sci. 84, 1285–1286.
Kala, C. P. (1998). Ecology and Conservation of Alpine Meadows in the Valley of Flowers National Park, Garhwal Himalaya. Ph.D. thesis. Dehradun: Forest Research Institute, 1–180.
Kala, C. P. (2005). A multifaceted review on the biodiversity conservation of the Valley of Flowers National Park, India. Int. J. Biodivers. Sci. 1, 25–32. doi: 10.1080/17451590509618077
Kala, C. P., and Mathur, V. B. (2002). Patterns of plant species distribution in the Trans-Himalayan region of Ladakh, India. J. Veg. Sci. 13, 751–754. doi: 10.1111/j.1654-1103.2002.tb02104.x
Kamlesh, K., Avtar, S., Prabhbir, S., and Kuldeep, T. (2012). Geomorphic evaluation of valley of flower region Bhyunder Ganga catchment, Chamoli District, Uttarakhand Using: remote Sensing and GIS Technology. Earth Sci. Res. 1, 114.
Kirschbaum, M. U. (2000). Will changes in soil organic carbon act as a positive or negative feedback on global warming? Biogeochemistry 48, 21–51.
Koleff, P., Gaston, K. J., and Lennon, J. J. (2003). Measuring beta diversity for presence–absence data. J. Anim. Ecol. 72, 367–382. doi: 10.1046/j.1365-2656.2003.00710.x
Kueffer, C., Daehler, C., Dietz, H., McDougall, K., Parks, C., Pauchard, A., et al. (2014). The Mountain Invasion Research Network (MIREN). Linking local and global scales for addressing an ecological consequence of global change. GAIA Ecol. Perspect. Sci. Soc. 23, 263–265. doi: 10.14512/gaia.23.3.11
Kumar, A., Kant, R., Dogra, K. S., Chandra Sekar, K., Kharkwal, K., and Dash, S. S. (2022). Diversity of Threatened Plants and Conservation Efforts in Great Himalayan National Park, Himachal Pradesh, Western Himalaya. Indian For. 147, 1172–1175. doi: 10.36808/if/2021/v147i12/166614
Kumar, R., Arya, D., Chandra Sekar, K., and Bisht, M. (2021). Threat assessment and prioritization of high-value medicinal plants in Pindari valley, Nanda Devi Biosphere Reserve, India. Curr. World Environ. 16, 1–14.
Kuniyal, J. C., Maiti, P., Kumar, S., Kumar, A., Bisht, N., Chandra Sekar, K., et al. (2021). Dayara bugyal restoration model in the alpine and subalpine region of the Central Himalaya: a step toward minimizing the impacts. Sci. Rep. 11:16547. doi: 10.1038/s41598-021-95472-y
Lal, R. (2005). Forest soils and carbon sequestration. For. Ecol. Manag. 220, 242–258. doi: 10.1016/j.foreco.2005.08.015
Laurance, W. F., Useche, D. C., Rendeiro, J., Kalka, M., Bradshaw, C. J., Sloan, S. P., et al. (2012). Averting biodiversity collapse in tropical forest protected areas. Nature 489, 290–294. doi: 10.1038/nature11318
Mehta, P., Bisht, K., and Chandra Sekar, K. (2021). Diversity of threatened medicinal plants of Indian Himalayan Region. Plant Biosyst. Int. J. Dealing Aspects Plant Biol. 155, 1121–1132.
Mehta, P., Chandra Sekar, K., Bhatt, D., Tewari, A., Bisht, K., Upadhyay, S., et al. (2020). Conservation and prioritization of threatened plants in Indian Himalayan Region. Biodivers. Conserv. 29, 1723–1745. doi: 10.1007/s12298-021-01044-9
Miller, R. W., and Donahue, R. L. (1990). Soils, an Introduction to Soils and Plant Growth, 6th Edn. Englewood Cliffs, NJ: Prentice-Hall Inc.
Minchin, P. (1987). An evaluation of the relative robustness of techniques for ecological ordination. Plant Ecol. 69, 89–107. doi: 10.1007/978-94-009-4061-1_9
Mueller-Dombois, D., and Ellenberg, H. (1974). Aims and Methods of Vegetation Ecology. New York, NY: Wiley and Sons.
Murthy, K. R. K. (2011). Floral Gallery of Himalayan Valley of Flowers and Adjacent Areas. Banglore: Natraj Publishers.
Naiman, R. J., Pinay, G., Johnston, C. A., and Pastor, J. (1994). Beaver influences on the long-term biogeochemical characteristics of boreal forest drainage networks. Ecology 75, 905–921. doi: 10.2307/1939415
Naithani, H. B., Negi, J. D. S., Thapliyal, R. C., and Pokhriyal, T. C. (1992). Valley of flowers: need for conservation or preservation. Indian For. 118, 371–378.
Naughton-Treves, L., Holland, M. B., and Brandon, K. (2005). The role of protected areas in conserving biodiversity and sustaining local livelihoods. Annu. Rev. Environ. Resour. 30, 219–252. doi: 10.1146/annurev.energy.30.050504.164507
Nayar, M. P., and Shastry, A. R. K. (1987). Red Data Book of Indian Plants. Howrah: Botanical Survey of India.
Nayar, M. P., and Shastry, A. R. K. (1988). Red Data Book of Indian Plants. Howrah: Botanical Survey of India.
Nayar, M. P., and Shastry, A. R. K. (1990). Red data Book of Indian Plants. Howrah: Botanical Survey of India.
Negi, V., Maikhuri, R. K., and Maletha, A. (2017). Polygonum polystachyum, peril to biodiversity of the alpine ecosystem, western Himalaya, India. Curr. Sci. 113, 2249–2251.
Oksanen, J. (2019). R-vegan, Community Ecology package. Available online at: https://cran.r-project.org, https://github.com/vegandevs/vegan (accessed January 15, 2022).
Olsen, S. R., Cole, C. V., Watanabe, F. S., and Dean, L. A. (1954). Estimation of Available Phosphorus in Soils by Extraction with Sodium Bicarbonate. Washington, DC: United States Department of Agriculture.
Pandey, A., Chandra Sekar, K., Joshi, B., and Rawal, R. S. (2019). Threat assessment of high-value medicinal plants of cold desert areas in Johar valley, Kailash Sacred Landscape, India. Plant Biosyst. Int. J. Dealing Aspects Plant Biol. 153, 39–47. doi: 10.1080/11263504.2018.1448010
Pardo, A., Lopes, D. H., Fierro, N., and Borges, P. A. (2020). Limited effect of management on apple pollination: a case study from an oceanic island. Insects 11, 351–366. doi: 10.3390/insects11060351
Parkinson, J. A., and Allen, S. E. (1975). A wet oxidation procedure suitable for the determination of nitrogen and mineral nutrients in biological material. Commun. Soil Sci. Plant Anal. 6, 1–11. doi: 10.1080/00103627509366539
Pauchard, A., and Villarroel, P. (2002). Protected areas in Chile: history, current status, and challenges. Natural Areas J. 22, 318–330.
Pielou, E. (1966). The measurement of diversity in different types of biological collections. J. Theor. Biol. 13, 131–144.
Pressey, R. L., Visconti, P., and Ferraro, P. J. (2015). Making parks make a difference: poor alignment of policy, planning and management with protected-area impact, and ways forward. Philos. Trans. R. Soc. B Biol. Sci. 370:20140280. doi: 10.1098/rstb.2014.0280
Pusalkar, P. K., and Singh, D. K. (2012). Flora of Gangotri National Park, Western Himalaya, India. Kolkata: Botanical Survey of India.
Rands, M. R., Adams, W. M., Bennun, L., Butchart, S. H., Clements, A., Coomes, D., et al. (2010). Biodiversity conservation: challenges beyond 2010. Science 329, 1298–1303. doi: 10.1126/science.1189138
Rawal, R. S., Rawal, R., Rawat, B., Rawat, B., Negi, V. S., and Pathak, R. (2018). Plant species diversity and rarity patterns along altitude range covering treeline ecotone in Uttarakhand: conservation implications. Trop. Ecol. 59, 225–239.
Rawat, S., and Upreti, D. K. (2014). Macrolichen biomass in Nanda Devi Biosphere Reserve, Chamoli District, Uttarakhand, India. Geophytology 44, 155–167.
Reich, P. B., Grigal, D. F., Aber, J. D., and Gower, S. T. (1997). Nitrogen mineralization and productivity in 50 hardwood and conifer stands on diverse soils. Ecology 78, 335–347. doi: 10.1890/0012-9658(1997)078[0335:nmapih]2.0.co;2
Saha, S., Rajwar, G. S., and Kumar, M. (2016). Forest structure, diversity and regeneration potential along altitudinal gradient in Dhanaulti of Garhwal Himalaya. For. Syst. 25, 58–72.
Sánchez-González, A., and López-Mata, L. (2005). Plant species richness and diversity along an altitudinal gradient in the Sierra Nevada, Mexico. Divers. Distrib. 11, 567–575. doi: 10.1111/j.1366-9516.2005.00186.x
Schimel, J. P., and Bennett, J. (2004). Nitrogen mineralization: challenges of a changing paradigm. Ecology 85, 591–602. doi: 10.1126/science.aas9313
Sehgal, J. L., Mandal, D. K., Mandal, C., and Vadivelu, S. (1990). Agro-ecological regions of India. NBSS Publ. 24, 1–96.
Singh, S. K., and Rawat, G. S. (2000). Flora of Great Himalayan National Park, Himachal Pradesh. Dehradun: Bishen Singh Mahendra Pal Singh.
Sharma, B., and Bhatia, K. S. (2003). Correlation of soil physical properties with soil erodibility. Indian J. Soil Conserv. 31, 313–314.
Singh, P., Karthigeyan, K., Lakshminarasimhan, P., and Dash, S. S. (2015). Endemic Vascular Plants of India. Kolkata: Botanical Survey of India.
Smeck, N. E. (1985). Phosphorus dynamics in soils and landscapes. Geoderma 36, 185–199. doi: 10.1016/0016-7061(85)90001-1
Spear, D., Foxcroft, L. C., Bezuidenhout, H., and McGeoch, M. A. (2013). Human population density explains alien species richness in protected areas. Biol. Conserv. 159, 137–147. doi: 10.1016/j.scitotenv.2017.05.220
Tang, L., Dong, S., Sherman, R., Liu, S., Liu, Q., Wang, X., et al. (2014). Changes in vegetation composition and plant diversity with rangeland degradation in the alpine region of Qinghai-Tibet Plateau. Rangeland J. 37, 107–115. doi: 10.1071/rj14077
Thakur, U., Bisht, N. S., Kumar, M., and Kumar, A. (2021). Influence of Altitude on Diversity and Distribution Pattern of Trees in Himalayan Temperate Forests of Churdhar Wildlife Sanctuary, India. Water Air Soil Pollut. 232, 205–221.
Ved, D. K., Kinhal, G. A., Ravikumar, K., Prabhakaran, V., Ghate, U., Vijayashankar, R., et al. (2003). Conservation Assessment and Management Prioritisation. Bangalore: FRLHT, 9–11.
Vitousek, P. M., and Howarth, R. W. (1991). Nitrogen limitation on land and in the sea: how can it occur? Biogeochemistry 13, 87–115.
Wadhwa, B. M., Rao, R. R., and Hajra, P. K. (1987). Botany of the Valley of Flowers National Park and its environs. Nelumbo 29, 129–175.
Walkley, A., and Black, I. A. (1934). An examination of the Degtjareff method for determining soil organic matter, and a proposed modification of the chromic soil titration method. Soil Sci. 37, 1–38.
Watson, J. E. M., Dudley, N., Segan, D. B., and Hockings, M. (2014). The performance and potential of protected areas. Nature 515, 67–73. doi: 10.1038/nature13947
Wilson, M. V., and Schmida, A. (1984). Measuring beta diversity with presence–absence data. J. Ecol. 72, 1055–1064. doi: 10.2307/2259551
Xu, H. J., Wang, X. P., and Zhang, X. X. (2016). Alpine grasslands response to climatic factors and anthropogenic activities on the Tibetan Plateau from 2000 to 2012. Ecol. Eng. 92, 251–259. doi: 10.1016/j.ecoleng.2016.04.005
Yao, Z., Zhao, C., Yang, K., Liu, W., Li, Y., You, J., et al. (2016). Alpine grassland degradation in the Qilian Mountains, China—A case study in Damaying Grassland. Catena 137, 494–500. doi: 10.1016/j.catena.2015.09.021
Yirga, F., Marie, M., Kassa, S., and Haile, M. (2019). Impact of altitude and anthropogenic disturbance on plant species composition, diversity, and structure at the Wof-Washa highlands of Ethiopia. Heliyon 5:e02284. doi: 10.1016/j.heliyon.2019.e02284
Zhong, Z., and Makeschin, F. (2004). Comparison of soil nitrogen dynamics under beech, Norway spruce and Scots pine in central Germany. Eur. J. Res. 123, 29–37. doi: 10.1007/s10342-004-0021-y
Keywords: anthropogenic disturbance, elevation gradient, Great Himalayan National Park, Indian Himalayan Region, soil parameters, Valley of Flowers National Park
Citation: Bisht M, Chandra Sekar K, Mukherjee S, Thapliyal N, Bahukhandi A, Singh D, Bhojak P, Mehta P, Upadhyay S and Dey D (2022) Influence of Anthropogenic Pressure on the Plant Species Richness and Diversity Along the Elevation Gradients of Indian Himalayan High-Altitude Protected Areas. Front. Ecol. Evol. 10:751989. doi: 10.3389/fevo.2022.751989
Received: 02 August 2021; Accepted: 10 March 2022;
Published: 11 May 2022.
Edited by:
Damian Chmura, University of Bielsko-Biała, PolandReviewed by:
Cristian Atala, Pontificia Universidad Católica de Valparaíso, ChilePaulo A. V. Borges, University of the Azores, Portugal
Munesh Kumar, Hemvati Nandan Bahuguna Garhwal University, India
Copyright © 2022 Bisht, Chandra Sekar, Mukherjee, Thapliyal, Bahukhandi, Singh, Bhojak, Mehta, Upadhyay and Dey. This is an open-access article distributed under the terms of the Creative Commons Attribution License (CC BY). The use, distribution or reproduction in other forums is permitted, provided the original author(s) and the copyright owner(s) are credited and that the original publication in this journal is cited, in accordance with accepted academic practice. No use, distribution or reproduction is permitted which does not comply with these terms.
*Correspondence: K. Chandra Sekar, a2NzZWthcjEzMTJAcmVkaWZmbWFpbC5jb20=