- 1Animal Ecology, University of Potsdam, Potsdam, Germany
- 2Biologische Station Gülpe, Gülpe, Germany
Artificial light at night (ALAN) is altering the behaviour of nocturnal animals in a manifold of ways. Nocturnal invertebrates are particularly affected, due to their fatal attraction to ALAN. This selective pressure has the potential to reduce the strength of the flight-to-light response in insects, as shown recently in a moth species. Here we investigated light attraction of ground beetles (Coleoptera: Carabidae). We compared among animals (three genera) from a highly light polluted (HLP) grassland in the centre of Berlin and animals collected at a low-polluted area in a Dark Sky Reserve (DSR), captured using odour bait. In an arena setting tested at night time, HLP beetles (n = 75 across all genera) showed a reduced attraction towards ALAN. Tested during daytime, HLP beetles were less active in an open field test (measured as latency to start moving), compared to DSR (n = 143). However, we did not observe a reduced attraction towards ALAN within the species most common at both sides, Calathus fuscipes (HLP = 37, DSR = 118 individuals) indicating that not all species may be equally affected by ALAN. Reduced attraction to ALAN in urban beetles may either be a result of phenotypic selection in each generation removing HLP individuals that are attracted to light, or an indication for ongoing evolutionary differentiation among city and rural populations in their light response. Reduced attraction to light sources may directly enhance survival and reproductive success of urban individuals. However, decrease in mobility may negatively influence dispersal, reproduction and foraging success, highlighting the selective pressure that light pollution may have on fitness, by shaping and modifying the behaviour of insects.
Introduction
Light pollution is defined as the alteration of natural light levels at night by anthropogenic artificial light sources (Cinzano et al., 2001; Falchi et al., 2016). It is no longer confined to urban areas, with the effects also visible in remote and rural areas, sometimes many kilometres away from the light source, i.e., sky glow (Grubisic et al., 2018). Nocturnal invertebrates, including both flying and ground dwelling insects, are especially affected from light pollution due to their attraction towards outdoor illuminations, such as street lanterns, spotlights at sport events and festivals, tourist sightseeing highlights (Rich and Longcore, 2013; Owens and Lewis, 2018), and even ornamental LED garden tools (Eccard et al., 2018; Grubisic et al., 2018; Owens et al., 2020).
Various function of natural light insects may explain how artificial light at night (ALAN) can interfere in the life history of insects in a powerful manner (Sanders et al., 2021). Natural light sources play a crucial role in the orientation of many insect species (Grubisic et al., 2018; Owens et al., 2020). African Dung beetles (Scarabaeus satyrus) use the Milky Way (Dacke et al., 2013), moths (Noctua pronuba) are known to combine stars and moon light (Sotthibandhu and Baker, 1979), and sand hoppers (Talitrus saltator) maintain their nightly route by the moon (Ugolini et al., 1999). Further, visual communication can also be part of reproductive behaviour: for example, firefly beetles (Lampyridae) depend on light signals to find suitable mating partners (Ineichen and Rüttimann, 2012). Polarised light, reflected from incoming light by aquatic surfaces, is used by aquatic insects, such as mayflies, to detect suitable oviposition locations (Longcore and Rich, 2004; Szaz et al., 2015).
Previous studies have mainly investigated the attraction of invertebrates towards ALAN (flight-to-light response) which causes an increase in local mortality, either by higher risk of predation, exhaustion, and death by burning (Eisenbeis et al., 2006). One third of attracted insects will suffer death before the next sunrise due to one or more of the previously named causes (Frank et al., 2006; Owens et al., 2020). Epigaeal ground-dwelling organism such as ants, ground beetles, amphipods and harvestmen are also affected by ALAN and accumulate in higher abundances under artificial light sources (Davies et al., 2012; Eccard et al., 2018; Owens et al., 2020). This behaviour is the basis for the widespread use of light-traps as comprehensive method for assessing the diversity of insects in which the light-trap consist e.g., of a basic pit trap with an additional light source to increase the number of specimen captured. Also, light trapping is a very cost and time-efficient sampling method to produce qualitative and quantitative data on species occurrence and distribution in a short time period (Szentkirályi, 2002).
Artificial bright light sources can work as an ecological trap (Longcore and Rich, 2004; Frank et al., 2006), i.e., reduce the fitness of animals attracted to them. In some cases they work as evolutionary traps, (Hopkins et al., 2018) implying large-scale effects on insect populations by adding a light specific mortality. Given their crucial role in ecosystems as pollinators and as pest control, this can potentially lead to a cascade across species networks (Jankielsohn, 2018).
The massive decline of insect diversity and biomass across ecosystems in human dominated landscapes (Hallmann et al., 2017; Powney et al., 2019; Seibold et al., 2019) has often been attributed to habitat loss (e.g., land use change, agricultural intensification), chemical pollution (increased use of fertilizers), climate change, invasive species, or a combination of these factors. However, Owen et al. (2019) suggested that researchers possibly suffer a “diurnal bias,” in that most attention has been paid to daytime phenomena, and that we have not yet studied the potential effects of light on night time phenomena (Owens et al., 2020). Light pollution is itself potentially a severe threat to biodiversity (Hölker et al., 2010b; Grubisic et al., 2018). Still, ALAN it is often not recognised as potential driver: for example Hallmann et al. (2017) did not consider the potential role of ALAN on insect biomass decline, even though all study sites were located in the most light polluted areas of Germany [(Grubisic et al., 2018), see Krefeld lightpollutionmap.info/]. Since many taxa show alteration of physiological and behavioural traits by, and fatal attraction to ALAN [review see Sanders et al. (2021)], thus, light pollution as a driving factor for decline cannot be excluded (Hölker et al., 2010b; Grubisic et al., 2018). More recently, several years after Hölker et al.,’s proposal in 2010 to include light pollution in insects research, studies are beginning to investigate the role of light pollution when analysing cascading effects of insect decline (Kehoe et al., 2021).
When looking at potential long-term consequences of light pollution as a threat to insect populations, nocturnal, light-attracted micromoth populations suffer a stronger decline than corresponding diurnal species not attracted by light (van Langevelde et al., 2018; Wilson et al., 2018), demonstrating that, possibly combined with other factors, artificial night-time lighting affects moth populations (Wilson et al., 2018). Altermatt and Ebert (2016) also observed a reduced flight-to-light behaviour in moth population exposed to intense light pollution when compared to populations from Dark Sky Habitats (Altermatt and Ebert, 2016).
Given that alteration of activity patterns (e.g., increase, decrease, or shift of activity) are one of the main impacts of ALAN (Sanders et al., 2021), a possible, yet understudied outcome of constant light exposure in insects may be the general mobility decline, as suggested by Altermatt and Ebert (2016). In urban open spaces, highly mobile individuals encounter artificial light sources at a higher likelihood, hence, they are more easily captivated by light than less mobile individuals. Once attracted, insects are not able to escape from the illuminated zone (“captivity effect”) and face death (Eisenbeis et al., 2006), reducing fitness of more active individuals compared to less active ones in the same environment. It remains to be seen whether these disadvantages for highly mobile individuals have long-term consequences, including possible directional selection as suggested by Altermatt and Ebert (2016).
In this study we investigate the attraction to light sources, and the importance of long-time exposure of carabid beetle populations by comparing animals from two populations – either originating from a grassland habitat exposed to intense light pollution for many decades, or from location exposed to low light pollution levels. Carabid beetles are known to show a positive phototaxis response to ALAN [(Owens et al., 2020) and see Eccard et al. (2018) for some species turning immobile at illumination], and illumination can even result in a shift in species composition (Davies et al., 2012; Eccard et al., 2018). We hypothesised that (a) light polluted grassland harboured a different species community, than less polluted areas and (b) carabid beetles (from any genus) from highly light polluted (HLP) areas are less attracted to artificial light sources than their counterparts. Further, we investigated general activity pattern in an open field test and hypothesised that HLP beetles may be less mobile in their general activity when compared to beetles from low light pollution. We compared behaviour of several genera captured at both very light polluted urban or very dark rural sites, but also compared within populations of the same species captured at both sites.
Materials and Methods
Location and Animals
We collected carabid beetles from an urban area which has been exposed to intense light pollution over several decades in the Tiergarten (Central Berlin, Germany), and in rural areas with very low light pollution levels at the Biological Station Gülpe (Brandenburg, Germany), 80 km east of Berlin during August and September 2020 (Table 1 and Figure 1). The latter area was awarded as an “Sternenpark” [Dark Sky Reserve (DSR)] by the International Dark Sky Association (IDA) in 2014.

Table 1. Sampling sites of carabid beetles in Tiergarten (High Light pollution, HLP) and in Gülpe (Dark Sky Reserve, DSR) in 2020.
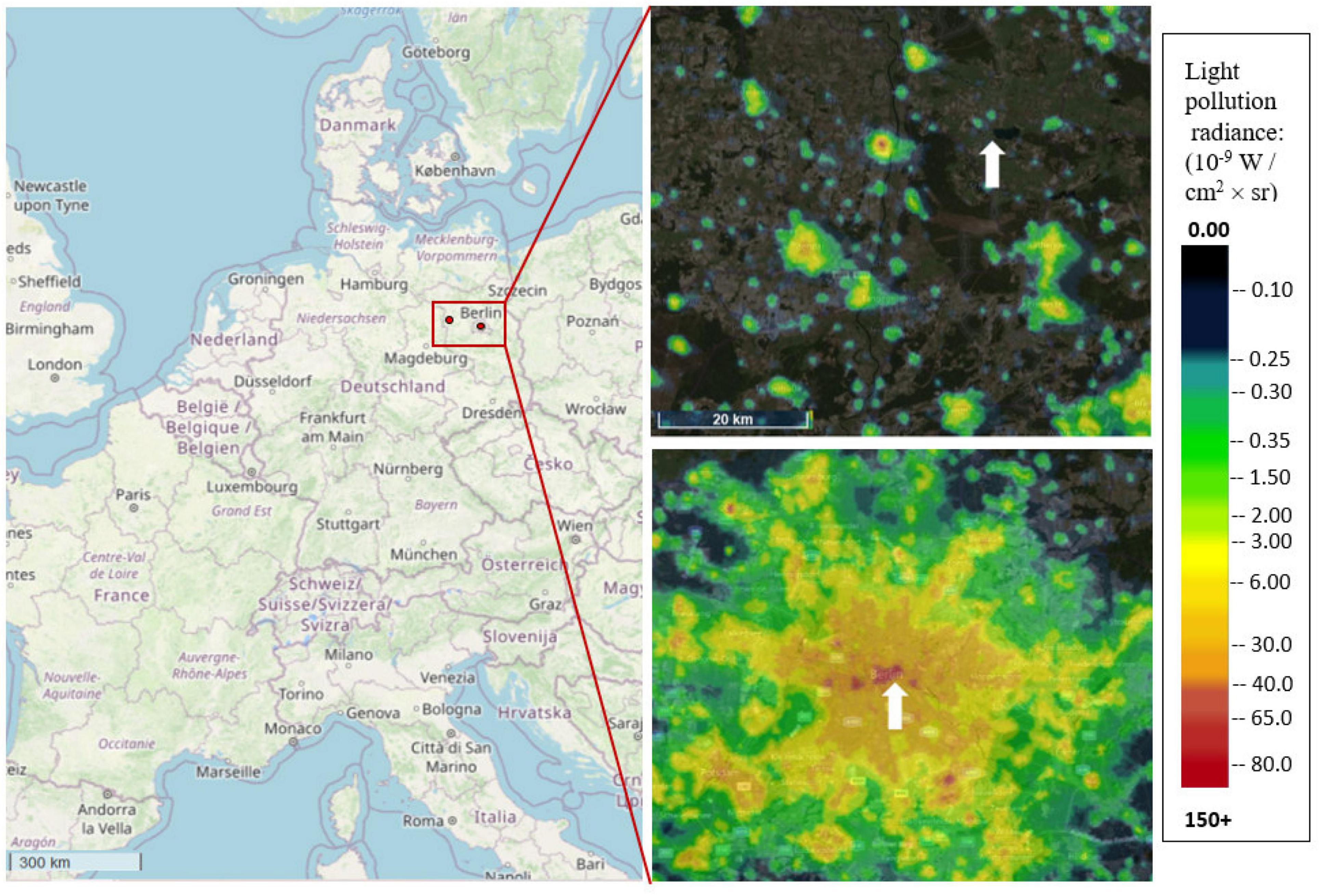
Figure 1. Location of the sites in Germany (map data OpenStreetMap contributors, CC-BY-SA), whereas red rectangle shows close-up from Berlin and its surrounding federal state Brandenburg where the red points mark the exact location of the sites. Light pollution radiance maps (VIIRS 2020) are showing the capital Berlin (bottom right) and part of the federal state Brandenburg (top right). White arrows are highlighting the urban site (Tiergarten, HLP) and the rural site (Biological Station Gülpe, DSR). Colours represent the radiance values in which bright colours (green, yellow, orange, and red) show higher light pollution values (see legend/lightpollutionmap.info).
We trapped adult carabid beetles with ten wine-baited pitfall traps at both sites for 4 days and nights in August 2020 (93 animals at DSR, 66 at HLP) and for 2 days and four nights in September 2020 (74 animals in DSR and 88 at HLP). Traps were emptied every 12 h, i.e., after sunrise and during sunset to distinguish dark and light capture intervals. Beetles were kept groupwise in plastic boxes (1.3 l, 12.5 cm × 19 cm × 7.5 cm; item number RK1285A) and were fed with pinkie maggots (Lucilia Caesar) or seeds every day. Boxes included shelters (pinecones, moss, leaves from originated site) and were moistened every day. Boxes were kept in an open garden shed outside the sun, in which beetles were exposed to the natural light-day cycle to ensure no effect of changing light conditions. During this time in captivity, beetles were not exposed to any artificial light sources unless they were tested in the arena.
Animals were kept from 3–44 days until the start of the experiment. The attraction experiment and the open field test were conducted on two separate days for each animal. Beetles were released at their origin afterwards.
Characterisation of Artificial Light Source Solar Powered LED
The artificial light source used in the experiment consisted of commercial solar powered LED (SPLED) garden lamps (Conrad Electronics, Hirschau, Germany, Model 5721129) which contained a 4 cm × 6 cm solar panel in its black plastic head (diameter 105 mm) and one diode (LED). Mainly cold white light was emitted from the integrated diode, but with a high percentage of blue light (correlated colour temperature = 7250 K). The spectral irradiance pattern of these lamps was published in Eccard et al. (2018).1 The position of the diode and the shape of the diffusor created a small highly illuminated zone close by and around the LED lamp (radius r = 25 cm, illuminance Ev = 0.8 lx) and a further, less illuminated outer zone (r = 2.5 m, Ev < 0.1 lx). Each day the solar panel was placed in the sun to recharge its battery (1.2 V, 600 mAh).
Behavioural Experiments
Attraction Towards Artificial Light at Night
We experimentally measured the attraction towards an artificial light source in elongated arenas (60 cm long × 8.5 cm wide tracks) where one SPLED was attached to one side of track (yellow field, Appendix). Approach to the lamp by a beetle was quantified by its presence in an area nearest to the lamp (8.5 × 8.5 cm, called illuminated zone). Six beetles were tested simultaneously (runs) in six adjacent tracks, and the position of the lamps was swapped between subsequent runs. The beetles were randomly selected from either HLP or DSR origins sites and species and placed gently into the middle of the track when the experiment started and observed for 15 min. Location within/outside the illuminated zone was recorded every 15 s (total: 60 observation intervals). Experiments were conducted at night.
Activity in the Open Field Test
Activity of the carabid beetles were observed for 3 min in an open field test (round plastic Petri dish, diameter 13.8 cm, rim height: 2.5 cm) under a desk light (110–240 V, E14, max. 40 W, mean lx 276. 62 ± 27.6). The open field was separated into eight 1/8 sectors. Activity was measured by counting the number of line crossings by an individual. The open field was further divided into an outer and inner zone with the same area size (A = 74.78 cm2). The inner concentric zone was within a radius of (r = 4.8 cm), the outer a ring between inner and outer radius (r = 6.9 cm). Each beetle was released at one of the sectors in the outer zone and the following behaviour was recorded: time to start moving [latency to move (sec)] and number of line crossings (number of crossings). Open field were conducted during day times to also test for possible differences of main activity pattern of species.
Behavioural Analyses
Analyses of the individual behaviour were conducted for 218 beetles all species that occurred in both sites divided into 3 genera. Attraction towards the artificial light source was analysed in two sequences (hurdle model): firstly, a binary variable of attraction towards the illuminated zone (binomial value yes/no for the entire experiment) and secondly, if yes, the number of 15 s intervals spent in the illuminated zone.
In both sequences, general linear distribution models (GLMs) were used to analyse the effect of the origin (HLP vs. DSR) including the genus of the beetle species, the interaction term of light pollution*genus, the side of the attached LED, the capture interval of emptying the trap (day/night), the days in captivity and species-specific activity pattern (diurnal/nocturnal) as fixed factors. The species-specific activity pattern was defined by literature records, to be either nocturnal or diurnal (Thiele, 1977; Van Dijk and Den Boer, 1992; Kielhorn, 2005).
Activity in the open field test was analysed in two separate models [linear model (lm) for continuous variable (latency to start moving (seconds), generalized linear model (glm) for count data (number of sectors crossed)]. We included either the latency (seconds) to start moving, or the number of crossings of sector borders as a response variable, and, as fixed factors, we used genus, capture interval of emptying the traps (day/night), the days in captivity and species-specific activity pattern (diurnal/nocturnal).
Full models including all fixed factors were reduced via stepwise backwards model selection by comparing the Akaike Information Criterion (AIC). Factors that improved the model were included, even if the factor did not explain the variation. Factors genus and origin were always kept in the model. The interaction term (pollution*genus) was removed if it did not improve the model fit.
Across some variables, the addition of fixed factors as species-specific activity pattern (nocturnal/diurnal) and the capture interval of emptying the pit traps (morning/evening) did not improve the model (AIC selection) and did not explain variation in the variable impro. Thus, these factors were removed during the model reduction process.
The species Calathus fuscipes was captured with > 20 animals per origin, thus, we conducted a within-species comparison of behaviour among origins.
All analyses were done with R Version [4.0.3 (2020-10-10) using the lme4 package (version 1.1-25, (Bates et al., 2015)] and figures always represent raw data.
Diversity Analysis
Species composition was compared among the plots, using the pit trapping rounds for animal collection as repeat. We analysed 11 sampling rounds (six rural and five urban with 11–60 individuals of 3–9 species captures) and removed three sampling rounds with < 3 species collected. Species composition was analysed using ordination to visualise the data, and multivariate, permutational analyses of variance (MPANOVA) to compare among the two plots (both with the R package vegan 2-5-5).
Results
Carabid Communities and Diversity
A total of 14 carabid species (n = 321, Genus Calathus, Harpalus, Amara and, only at DSR, Poecilus) were captured (Table 2), of which eight species (218 individuals, 3 genera) were recorded at both sites.
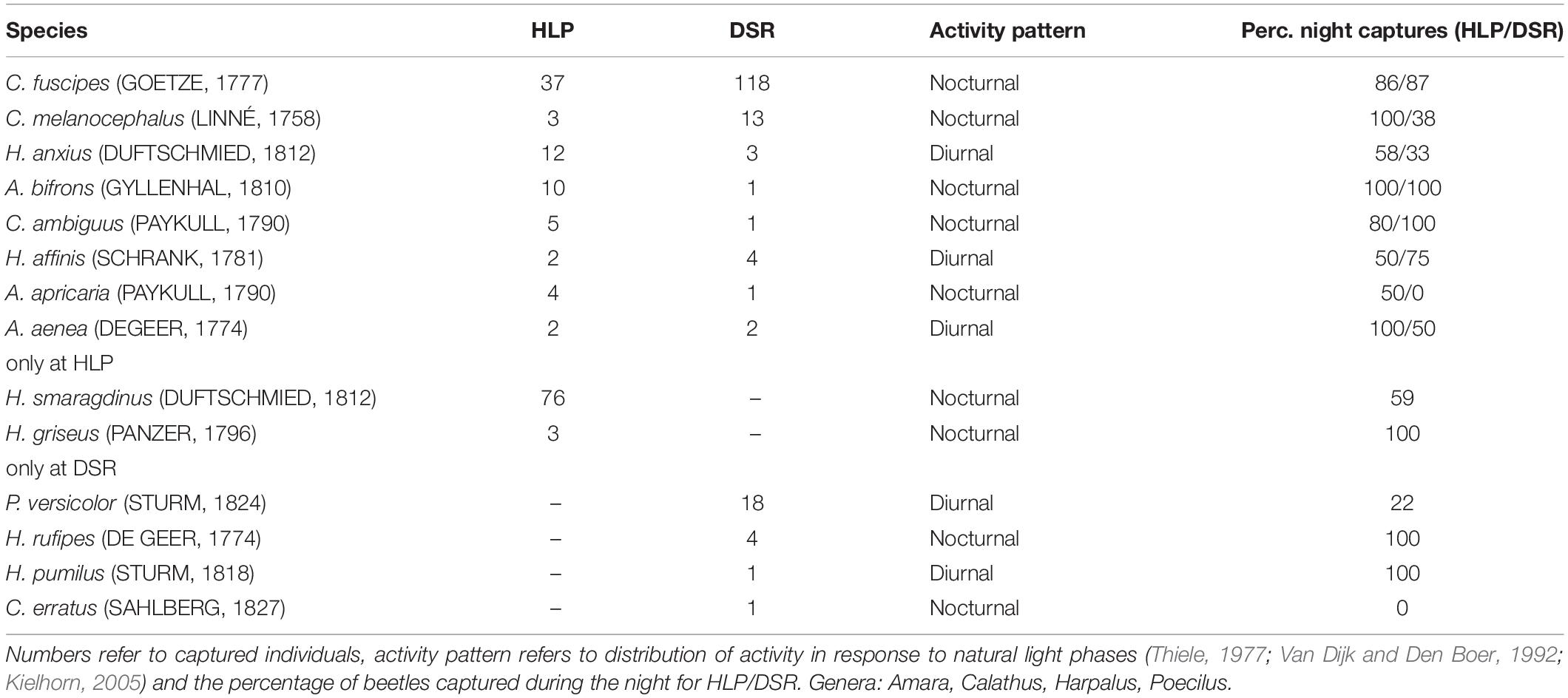
Table 2. Species captured by odour trapping in grassland habitats in the city (HLP) and in a Dark Sky Reserve (DSR).
The animal abundance [28.3 ± 14 (mean ± SD) animals per sampling round] and species richness (5 ± 1.9 species) did not differ among urban and rural sites (t-test abundance, t = 0.56, p = 0.59; Wilcoxon test species richness: W = 12.5, p = 0.711). However, their species composition was dissimilar between the rural and urban grassland plot [see Figure 2, MPANOVA (origin) = 9.9, p = 0.002, df = 1/10)], but without finding any difference in the Diversity Index (Shannon Wieder Index. urban: 1.2 ± 0.4, rural: 0.65 ± 0.2, student’s t = -1,5, df = 9, pr = 0.158).
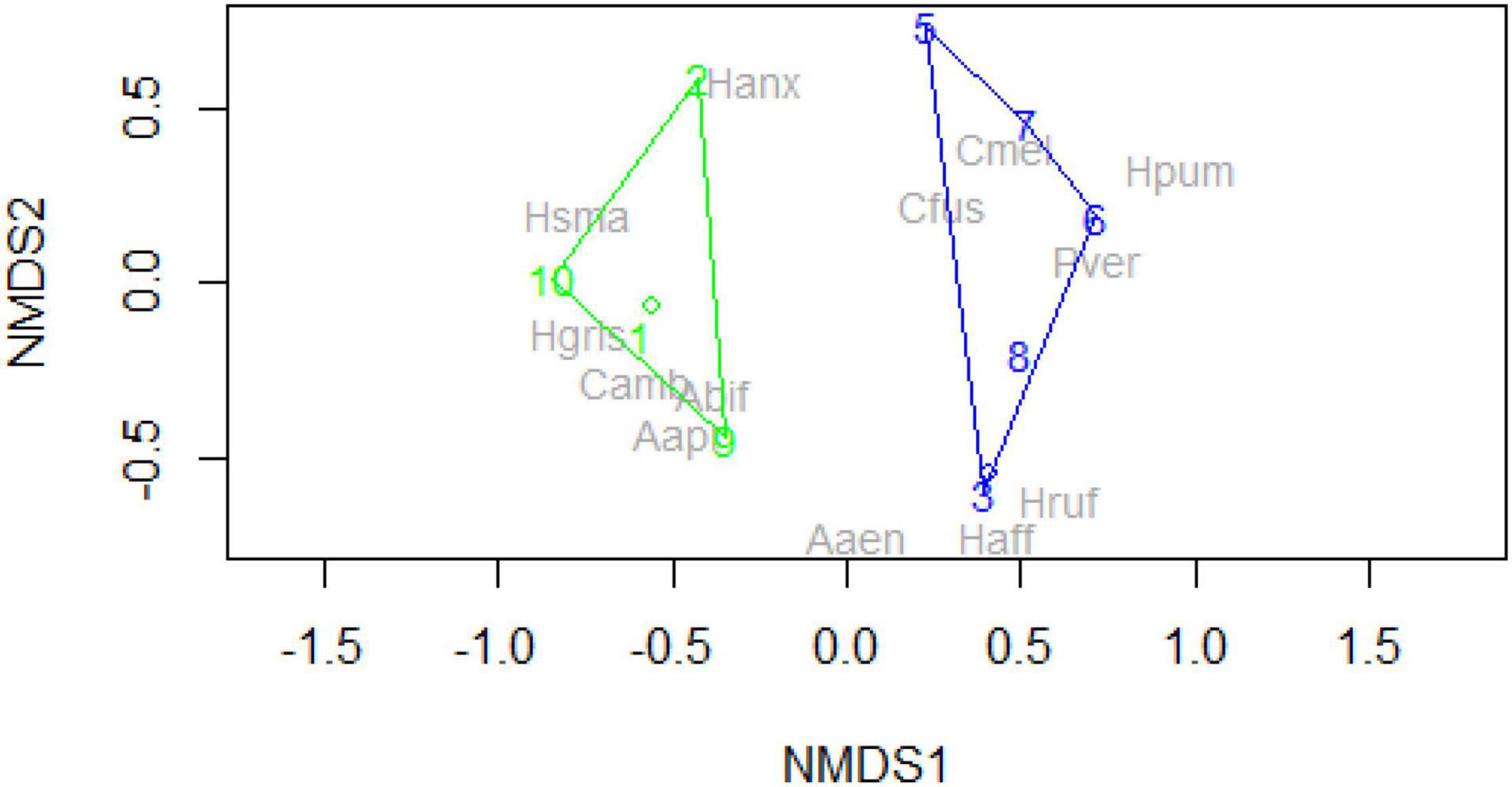
Figure 2. Ordination plot of ground beetle species data (grey names, Family species = Ospe, Families include Harpalus, Calathus, Poecilus, and Amara), captured in 11 sampling rounds (numbers) on an urban (green) and a rural (blue) dry grassland. Model with two clusters, stress = 0.009. NMDS1 maybe interpreted as a urban–rural gradient with species left occurring only on urban, centre on both, and the right only rural plots. NMDS2 may be interpreted as an abundance axis.
Behaviour
Behavioural experiments were performed with 218 beetles belonging to eight species present in both origins (Table 2) from three genera: Amara (20 individuals, 3 Species), Calathus (177 individuals, 3 Species), and Harpalus (21 individuals, 2 Species).
A lower proportion of beetles from HLP (57%) went into the illuminated sector compared to DSR beetles (72%, Chi2 -Test, X2 = 4.1, df = 1, p = 0.041, Figure 3A).
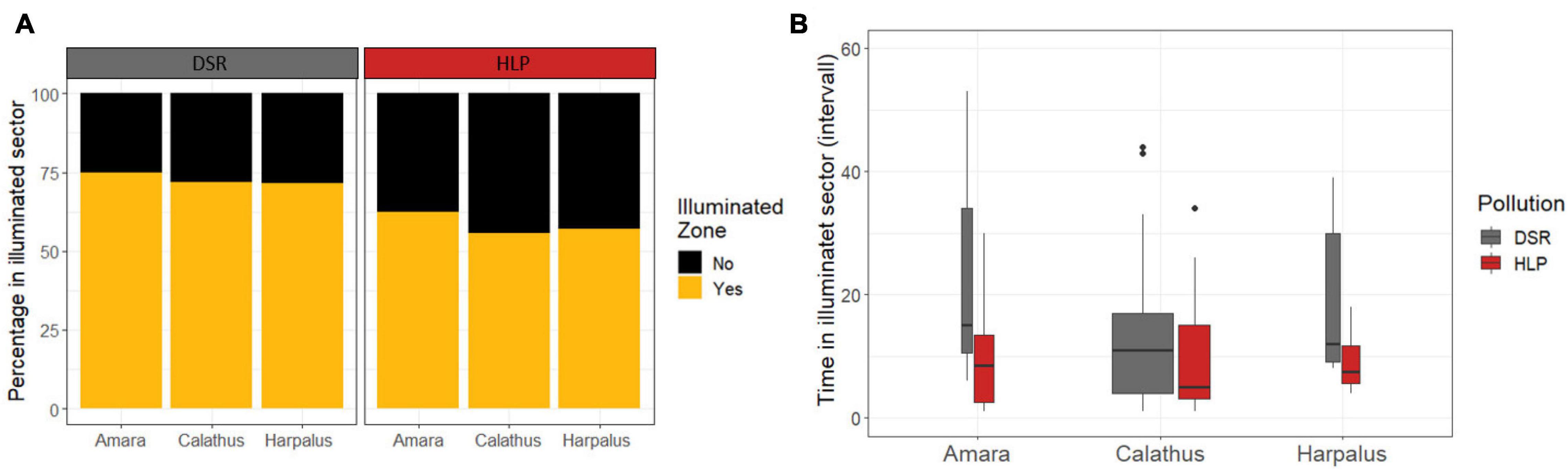
Figure 3. Use of the illuminated sector in an experiment on light attraction of carabid beetles captured in a Dark Sky Reserve (DSR) and in an urban area with high light pollution (HLP) divided into three genera Amara, Calathus, Harpalus. (A) Percentage of animals approaching the LED (total n = 218), (B) time spend near the LED based on those animals from HLP (red color) and DSR (grey color) that approached LED (n = 146) divided into genera. The boxplot presents the median and the quartiles. Width of box relates to sample size.
Among those beetles that entered the illuminated zone (n = 146), the number of time intervals in the illuminated zoner was lower for HLP [n = 43, 8.5 ± 1.29 time intervals (Mean ± SE)] than in DSR (n = 103, 10.4 ± 1.02 time intervals; Table 3), accordingly the DSR individuals stayed longer in the illuminated zone than their HLP counterparts (glm, pollution (HLP): Chi2, df = 1, p = 0.04*, Figure 3B).
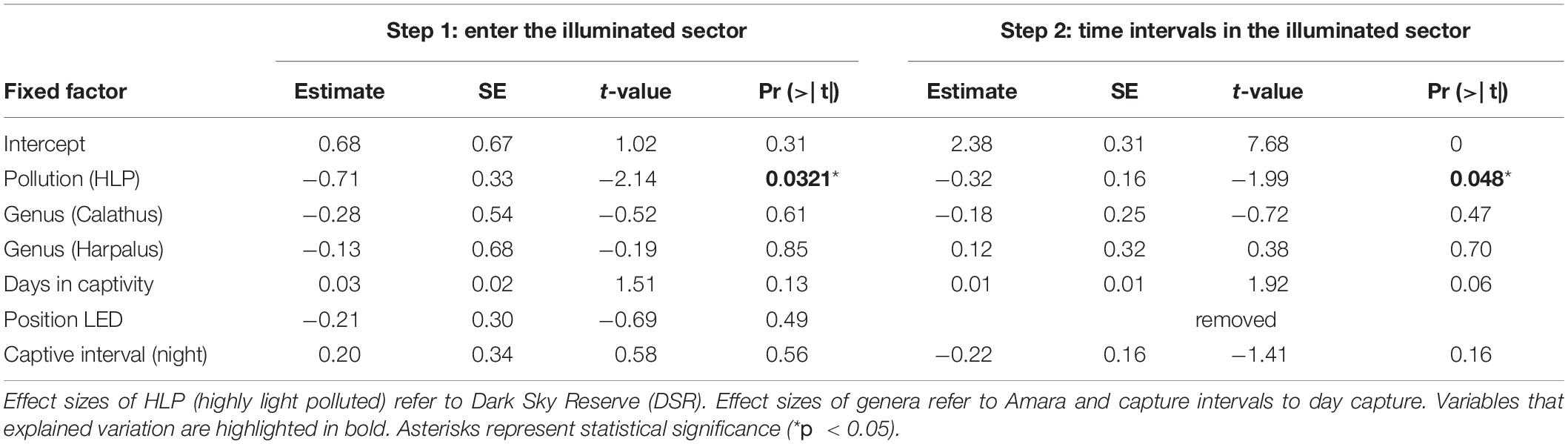
Table 3. General linear distribution model analysis of light attraction of ground beetles (step 1: n = 218, step 2: n = 146, three genera) investigated in an elongated arena comparing animals originating from two areas with different light pollution exposure.
Activity in Open Field
Beetles from HLP origin began moving slower [46 ± 6.7 (Mean ± SE) seconds] than beetles from DSR origin which initiated their movement after (26 ± 3.8) seconds [glm, pollution (HLP): Chi2, df = 1, p = 0.029].
Beetles originating from DSR crossed 42.7 ± 8.3 (mean ± SE) sectors and HLP beetles crossed 29.1 ± 5.4 sectors during the open-field test. Effect of pollution on the number of sectors crossed was only observed when looking at the effect size of the glm model (see Table 4), but were not supported in the final Anova output [negative glm, pollution (HLP): Chi2, df = 1, p = 0.106].
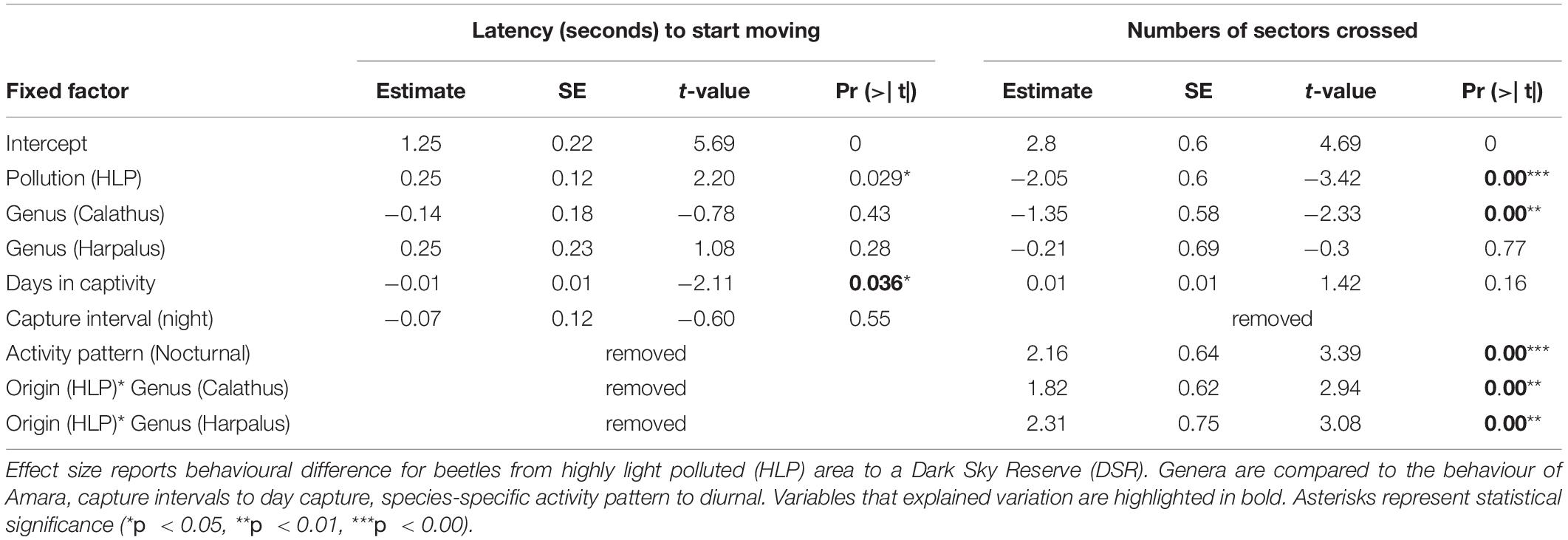
Table 4. General linear distribution model for behavioural variables measured in an open field test with 218 ground beetles captured at two origins differing in light pollution intensity.
Nocturnal beetles had a higher number of crossings 40.5 ± 3.9 than the diurnal species 18.8 ± 3.7, in which nocturnal ones are more active than diurnal species in the open field test [negative glm, species-specific activity pattern (nocturnal): Chi2 -Test, df = 1, p = 0.019, Figure 4A].
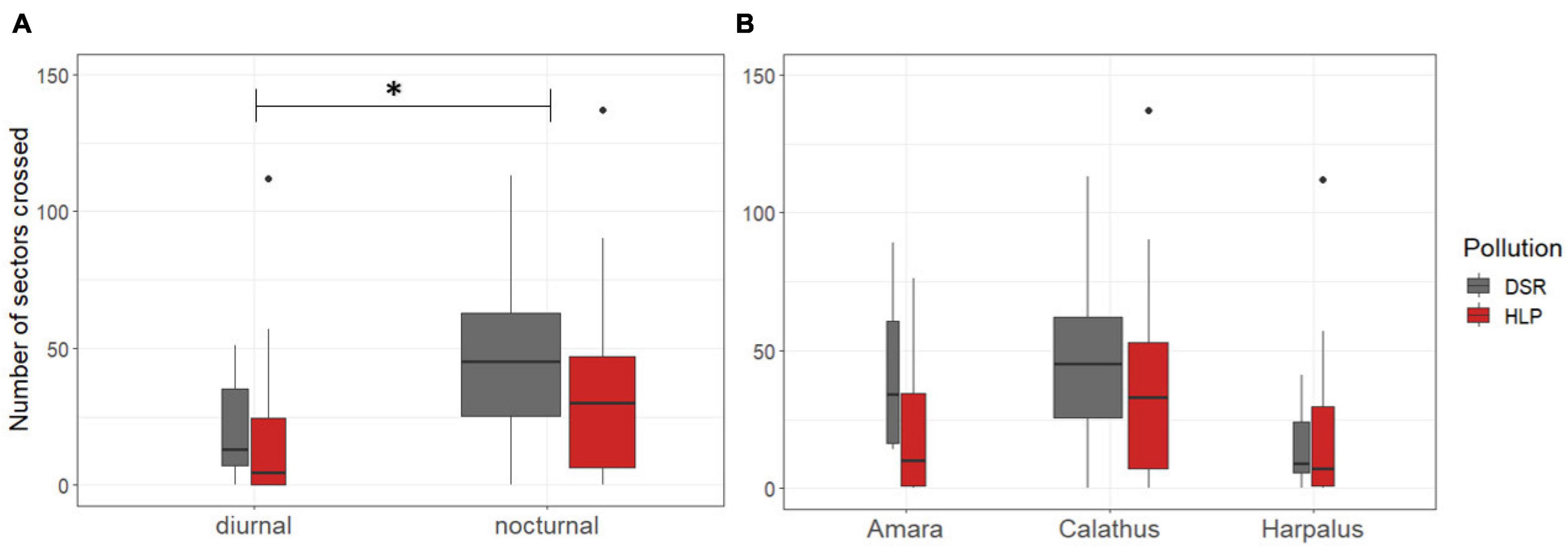
Figure 4. Number of crossings in in nocturnal beetles originating from DSR beetles (n = 134, dark grey) and HLP areas (n = 59, red). (A) Division between nocturnal (n = 193) and diurnal beetles (n = 25) and (B) division into three genera of all beetle species independent their main activity pattern. Boxplots show median and the quartiles. Width of box relates to sample size.
The effect of origin was different among genera (Interaction pollution*genus: chi2, df = 2, p = 0.047*, Figure 4B) but in post hoc analyses no effects of origin within each genus were detected (all Turkey, p > 0.1).
Analyses Within Calathus fuscipes
In the most abundant species C. fuscipes with 37 and 118 individuals (HLP and DSR), differences in behaviour obtained from the beetle community were not confirmed. Effects of light pollution did not explain the percentage of animals which went into the illuminated zone, the times spent in it, or the number of crossings in the open field test [glm, pollution (HLP): Chi2, p > 0.5, see Tables 5, 6].
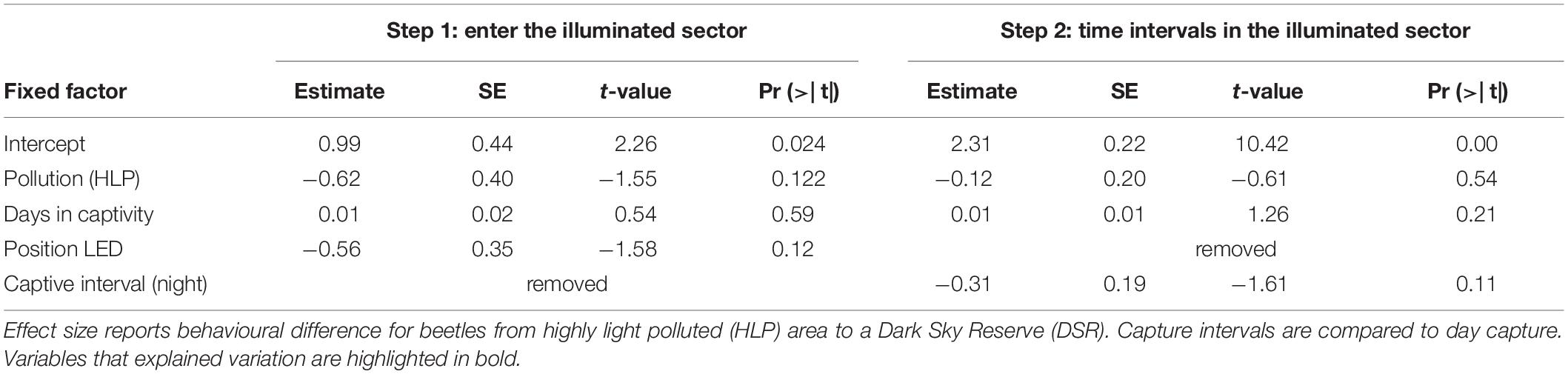
Table 5. General linear distribution model for behavioural variables measured in an open field test with 155 beetles C. fuscipes captured at two origins differing in light pollution intensity.
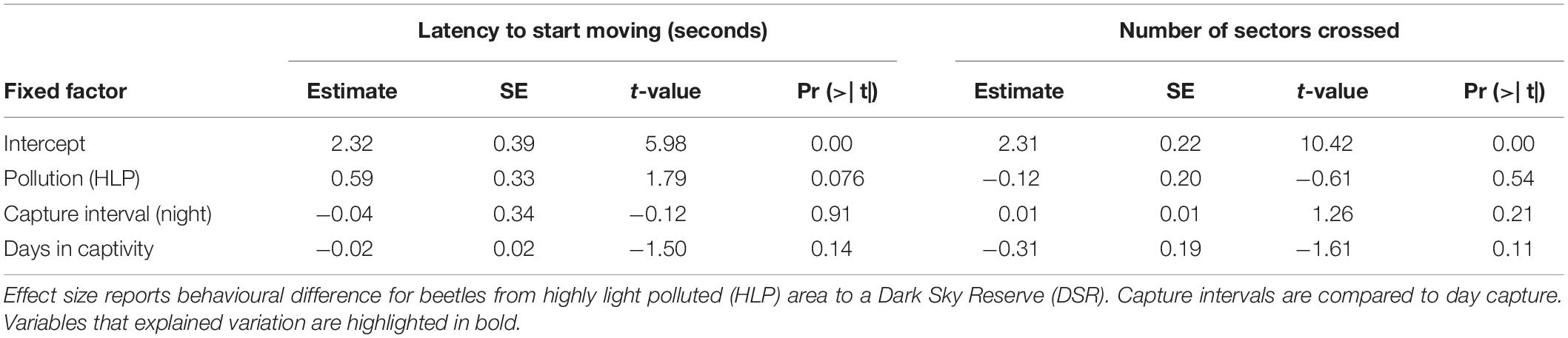
Table 6. General linear distribution model for behavioural variables {latency to start moving [log(seconds)], numbers of sectors crossed} measured in an open field test with 155 beetles C. fuscipes captured at two origins differing in light pollution intensity.
Discussion
Our study confirmed that urban carabid beetles have a reduced attraction to artificial light sources (ALAN), relative to individuals with rural origins. Effects of origin did not differ among the 14 species of three genera investigated, which were all common at both sites of origin. However, different from Altermatt and Ebert (2016) that found different flight-to-light responses in urban and rural moths of the same species, we did not find different responses within the species most common at both origins C. fuscipes.
The species C. fuscipes was the most abundant species in both origins and was also collected both during day and night (approx. 86% during night, 24% during day, see Table 2). This suggests that this species may be very resilient to light pollution. Likewise, the lack of differentiation among species could be seen as evidence that a systematic change in behavioural patterns due to ALAN has already happened and that it has comprehensive implications beyond singular species levels, while resilient and ubiquitous species, such as C. fuscipes, are not strongly affected.
A reduced attraction to light at night of urban insect populations may affect results of light trapping, which is often used to assess the diversity of insects. The use (or addition) of a light-independent survey method should therefore be advised.
Species in human-altered environments (e.g., through the introduction of light, invasive species) have lower population sizes, demonstrating the potentially negative effect of anthropogenic activity on insects (Eisenbeis et al., 2006; Sih et al., 2011), including the fatal attraction to ALAN.
The findings in our study are coherent with the observation of reduction in flight-to-light behaviour in urban moth population seen by Altermatt and Ebert (2016). This suggests that the reduction in light attraction (or even possible avoidance of light sources) can lead to a higher survival rate in urban population by reducing mortality caused by ALAN. Reduction in attraction outweighs potential benefits of light attraction, instead favouring individuals that are not inclined to move towards ALAN (Frank et al., 2006; Altermatt and Ebert, 2016). We also found a reduction of the general activity of urban beetles, compared to rural. The potential underlying mechanism, general mobility reduction (number of crossings or latency to start movement), which was confirmed in one of the two measurements in the open field.
The parameters of the open field highlight the different responses between the genera, as well as a need for more caution in further studies when including both diurnal and nocturnal species in analyses. These are not equally affected by light pollution (Sanders et al., 2021). Here, nocturnal beetles were more active than diurnal ones (Table 3), alas runs were done during daytime and should have been either undertaken in accordance to species-specific activity pattern or both during day and night time. Higher activity in in nocturnal beetles can be caused by the urge to escape direct light exposure to hide under soil or leaves, as it is common for nocturnal carabid beetles over day time (Lindroth and Bangsholt, 1985). Nonetheless, treatment was equal to both cohorts and only partly revealed expected lower activity in beetles from HLP.
General activity decline can constrain migration and exchange between habitat patches on a regional scale (Bennie et al., 2015), thereby restricting gene flow and limiting the inflow of genetic diversity (Kotler, 1984; Eisenbeis et al., 2006; Sih et al., 2011; Hopkins et al., 2018). This can impact persistence of meta-populations in urban green spaces (Bennie et al., 2015) through negatively impacting reproduction success (Gaston and Bennie, 2014). Further, limited genetic exchange between rural and urban habitats can lead to evolutionary differentiation of urban and rural populations with the potential to create new species (Hopkins et al., 2018).
Much recent attention has been paid to light pollution and its manifold and complex impacts on individuals, populations and ecosystems. Our study demonstrates, firstly, that there is a behavioural change in urban population with high potential for adaptation, but with further need to investigate in long-term rearing experiments and in the physiological underlying mechanism; and secondly, that this behavioural change could negatively affect foraging capacity, dispersal and reproduction if lower activity levels are fully confirmed of urban settlers (Lenski, 1984; Hopkins et al., 2018). Previous research shows that rodents have reduced mobility or reduced foraging activity when exposed to increased illumination in open habitats (Kotler, 1984; Bird et al., 2004), but research regarding foraging success in insect under illumination is lacking.
In summary, light pollution can function as an agent of selection (Eisenbeis et al., 2006; Tuomainen and Candolin, 2011) and can shape the behavioural responses towards ALAN in beetle communities. Nonetheless, light pollution is still on the rise in both intensity and in its distribution around the globe (Kyba, 2018; Falchi et al., 2019), highlighting the need for action to develop lightning strategies that minimize the adverse ecological impacts. For example by preventing dark areas from being artificially lit, reduce trespassing of light sources, only illuminating surfaces intended to (by adjusting angle of light, baffles above lamps), changing the spectral composition of used light and further, to raise awareness about the conscious, sustainable use of light in our daily life [see review: Gaston et al. (2012), IDA (2021), Hölker et al. (2010a)]. It is time to turn off the lights.
Data Availability Statement
The raw data supporting the conclusions of this article will be made available by the authors, without undue reservation.
Author Contributions
VK and JE designed the experimental set up and research question. VK performed the animal trapping, experiments and hence, data collection, and organizing of the database. Both authors performed the statistical analyses and contributed to discussion, manuscript revision, read, and approved the submitted version.
Funding
This study was funded by the Deutsche Forschungsgemeinschaft (DFG, German Research Foundation) – Projektnummer 491466077. This study was part of the project “rural-urban coupling” [Ministry for Education and Science BMBF (Bridging in Biodiversity Science (BIBS), package “Rural-urban coupling,” funding number 01LC1501B)].
Conflict of Interest
The authors declare that the research was conducted in the absence of any commercial or financial relationships that could be construed as a potential conflict of interest.
Publisher’s Note
All claims expressed in this article are solely those of the authors and do not necessarily represent those of their affiliated organizations, or those of the publisher, the editors and the reviewers. Any product that may be evaluated in this article, or claim that may be made by its manufacturer, is not guaranteed or endorsed by the publisher.
Acknowledgments
We would like to thank the Biological Station Gülpe of the University of Potsdam for housing us and the carabid beetles and of course, the colleges of the research group “Animal Ecology” at the University of Potsdam. Additionally, we like to express our gratitude to Jens Esser and Michael Schmitt for their Coleoptera expertise, also Sascha Buchholz and Christopher Kyba for support during study planning.
Supplementary Material
The Supplementary Material for this article can be found online at: https://www.frontiersin.org/articles/10.3389/fevo.2022.751288/full#supplementary-material
Footnotes
References
Altermatt, F., and Ebert, D. (2016). Reduced flight-to-light behaviour of moth populations exposed to long-term urban light pollution. Biol. Lett. 12:20160111. doi: 10.1098/rsbl.2016.0111
Bates, D., Maechler, M., Bolker, B., and Walker, S. (2015). Fitting linear mixed-effects models using lme4. J. Stat. Softw. 67, 1–48.
Bennie, J., Duffy, J. P., Davies, T. W., Correa-Cano, M. E., and Gaston, K. J. (2015). Global trends in exposure to light pollution in natural terrestrial ecosystems. Remote Sens. 7, 2715–2730.
Bird, B. L., Branch, L. C., and Miller, D. L. (2004). Effects of coastal lighting on foraging behaviorof beach mice. Conserv. Biol. 18, 1435–1439. doi: 10.1111/j.1523-1739.2004.00349.x
Cinzano, P., Falchi, F., and Elvidge, C. D. (2001). The first world atlas of the artificial night sky brightness. Mon. Not. R. Astron. Soc. 328, 689–707. doi: 10.1046/j.1365-8711.2001.04882.x
Dacke, M., Baird, E., Byrne, M., Scholtz, C. H., and Warrant, E. J. (2013). Dung beetles use the Milky Way for orientation. Curr. Biol. 23, 298–300. doi: 10.1016/j.cub.2012.12.034
Davies, T. W., Bennie, J., and Gaston, K. J. (2012). Street lighting changes the composition of invertebrate communities. Biol. Lett. 8, 764–767. doi: 10.1098/rsbl.2012.0216
Eccard, J. A., Scheffler, I., Franke, S., and Hoffmann, J. (2018). Off-grid: solar powered LED illumination impacts epigeal arthropods. Insect Conserv. Divers. 11, 600–607. doi: 10.1111/icad.12303
Eisenbeis, G., Rich, C., and Longcore, T. (2006). “Artificial night lighting and insects: attraction of insects to streetlamps in a rural setting in Germany,” in Ecological Consequences of Artificial Night Lighting, Vol. 2, eds G. C. Rich and T. Longcore (Washington, DC: Island Press), 191–198.
Falchi, F., Cinzano, P., Duriscoe, D., Kyba, C. C., Elvidge, C. D., Baugh, K., et al. (2016). The new world atlas of artificial night sky brightness. Sci. Adv. 2:e1600377.
Falchi, F., Furgoni, R., Gallaway, T. A., Rybnikova, N. A., Portnov, B. A., Baugh, K., et al. (2019). Light pollution in USA and Europe: the good, the bad and the ugly. J. Environ. Manage. 248:109227. doi: 10.1016/j.jenvman.2019.06.128
Frank, K. D., Rich, C., and Longcore, T. (2006). “Effects of artificial night lighting on moths,” in Ecological Consequences of Artificial Night Lighting, eds C. Rich and T. Longcore (Washington, DC: Island Press), 305–344.
Gaston, K. J., and Bennie, J. (2014). Demographic effects of artificial nighttime lighting on animal populations. Environ. Rev. 22, 323–330.
Gaston, K. J., Davies, T. W., Bennie, J., and Hopkins, J. (2012). Reducing the ecological consequences of night-time light pollution: options and developments. J. Appl. Ecol. 49, 1256–1266. doi: 10.1111/j.1365-2664.2012.02212.x
Grubisic, M., van Grunsven, R. H., Kyba, C. C., Manfrin, A., and Hölker, F. (2018). Insect declines and agroecosystems: does light pollution matter? Ann. Appl. Biol. 173, 180–189.
Hallmann, C. A., Sorg, M., Jongejans, E., Siepel, H., Hofland, N., Schwan, H., et al. (2017). More than 75 percent decline over 27 years in total flying insect biomass in protected areas. PLoS One 12:e0185809. doi: 10.1371/journal.pone.0185809
Hölker, F., Moss, T., Griefahn, B., Kloas, W., Voigt, C. C., Henckel, D., et al. (2010a). The dark side of light: a transdisciplinary research agenda for light pollution policy. Ecol. Soc. 15:13.
Hölker, F., Wolter, C., Perkin, E. K., and Tockner, K. (2010b). Light pollution as a biodiversity threat. Trends Ecol. Evol. 25, 681–682. doi: 10.1016/j.tree.2010.09.007
Hopkins, G. R., Gaston, K. J., Visser, M. E., Elgar, M. A., and Jones, T. M. (2018). Artificial light at night as a driver of evolution across urban–rural landscapes. Front. Ecol. Environ. 16:472–479. doi: 10.1002/fee.1828
IDA (2021). International Dark-Sky Association: Light Pollution Sollution. Available Online at: https://www.darksky.org/light-pollution/light-pollution-solutions/ [Accessed November 1, 2021]
Ineichen, S., and Rüttimann, B. (2012). Impact of artificial light on the distribution of the common European glow-worm, Lampyris noctiluca (Coleoptera: Lampyridae). Lampyrid 2, 31–36.
Jankielsohn, A. (2018). The importance of insects in agricultural ecosystems. Adv. Entomol. 6, 62–73.
Kehoe, R., Frago, E., and Sanders, D. (2021). Cascading extinctions as a hidden driver of insect decline. Ecol. Entomol. 46, 743–756. doi: 10.1111/een.12985
Kielhorn, K.-H. (2005). “Rote liste und gesamtartenliste der laufkäfer (Coleoptera: Carabidae) von Berlin,” (Hrsg.) in Der Landesbeauftragte für Naturschutz und Landschaftspflege/Senatsverwaltung für Stadtentwicklung Berlin: Rote Listen der gefährdeten Pflanzen und Tiere von Berlin CD-ROM.
Kotler, B. P. (1984). Risk of predation and the structure of desert rodent communities. Ecology 65, 689–701. doi: 10.1007/s002449900150
Lenski, R. E. (1984). Food limitation and competition: a field experiment with two Carabus species. J. Anim. Ecol. 53, 203–216. doi: 10.2307/4352
Lindroth, C. H., and Bangsholt, F. (1985). The Carabidae-Coleoptera-Of Fennoscandia and Denmark. Leiden: Brill Archive.
Owens, A. C., and Lewis, S. M. (2018). The impact of artificial light at night on nocturnal insects: a review and synthesis. Ecol. Evol. 8, 11337–11358. doi: 10.1002/ece3.4557
Owens, A. C., Cochard, P., Durrant, J., Farnworth, B., Perkin, E. K., and Seymoure, B. (2020). Light pollution is a driver of insect declines. Biol. Conserv. 241:108259. doi: 10.1016/j.biocon.2019.108259
Powney, G. D., Carvell, C., Edwards, M., Morris, R. K., Roy, H. E., Woodcock, B. A., et al. (2019). Widespread losses of pollinating insects in Britain. Nat. Commun. 10:1018. doi: 10.1038/s41467-019-08974-9
Rich, C., and Longcore, T. (2013). Ecological Consequences of Artificial Night Lighting. Washington, DC: Island Press.
Sanders, D., Frago, E., Kehoe, R., Patterson, C., and Gaston, K. J. (2021). A meta-analysis of biological impacts of artificial light at night. Nat. Ecol. Evol. 5, 74–81. doi: 10.1038/s41559-020-01322-x
Seibold, S., Gossner, M. M., Simons, N. K., Blüthgen, N., Müller, J., Ambarlı, D., et al. (2019). Arthropod decline in grasslands and forests is associated with landscape-level drivers. Nature 574, 671–674. doi: 10.1038/s41586-019-1684-3
Sih, A., Ferrari, M. C., and Harris, D. J. (2011). Evolution and behavioural responses to human-induced rapid environmental change. Evol. Appl. 4, 367–387. doi: 10.1111/j.1752-4571.2010.00166.x
Sotthibandhu, S., and Baker, R. (1979). Celestial orientation by the large yellow underwing moth, Noctua pronuba L. Anim. Behav. 27, 786–800. doi: 10.1016/0003-3472(79)90015-0
Stare, J. (2021). Light Pollution Map. Deneb Geoinformation Solutions. Available Online at: https://www.lightpollutionmap.info/ [Accessed July, 30, 2021]
Szaz, D., Horvath, G., Barta, A., Robertson, B. A., Farkas, A., Egri, A., et al. (2015). Lamp-lit bridges as dual light-traps for the night-swarming mayfly, Ephoron virgo: interaction of polarized and unpolarized light pollution. PLoS One 10:e0121194. doi: 10.1371/journal.pone.0121194
Szentkirályi, F. (2002). Fifty-year-long insect survey in Hungary: T. Jermy’s contributions to light-trapping. Acta Zool. Academ. Sci. Hung. 48, (Suppl. 1), 85–105.
Thiele, H.-U. (1977). Carbid Beetles in Their Environment: A Stud on Habitat Selection by Adaptations in Physiology and Behaviour. Berlin: Springer-Verlag.
Tuomainen, U., and Candolin, U. (2011). Behavioural responses to human-induced environmental change. Biol. Rev. 86, 640–657. doi: 10.1111/j.1469-185x.2010.00164.x
Ugolini, A., Melis, C., and Innocenti, R. (1999). Moon orientation in adult and young sandhoppers. J. Comp. Physiol. A 184, 9–12. doi: 10.1098/rspb.2005.3199
Van Dijk, T. S., and Den Boer, P. (1992). The life histories and population dynamics of two carabid species on a Dutch heathland. Oecologia 90, 340–352. doi: 10.1007/BF00317690
van Langevelde, F., Braamburg-Annegarn, M., Huigens, M. E., Groendijk, R., Poitevin, O., van Deijk, J. R., et al. (2018). Declines in moth populations stress the need for conserving dark nights. Glob. Change Biol. 24, 925–932. doi: 10.1111/gcb.14008
Wilson, J. F., Baker, D., Cheney, J., Cook, M., Ellis, M., Freestone, R., et al. (2018). A role for artificial night-time lighting in long-term changes in populations of 100 widespread macro-moths in UK and Ireland: a citizen-science study. J. Insect Conserv. 22, 189–196. doi: 10.1007/s10841-018-0052-1
Keywords: light pollution, artificial light at night (ALAN), Carabidae beetles, environmental change, Illuminance, solar powered light-emitting diode
Citation: Kaunath V and Eccard JA (2022) Light Attraction in Carabid Beetles: Comparison Among Animals From the Inner City and a Dark Sky Reserve. Front. Ecol. Evol. 10:751288. doi: 10.3389/fevo.2022.751288
Received: 31 July 2021; Accepted: 26 January 2022;
Published: 16 February 2022.
Edited by:
Davide M. Dominoni, University of Glasgow, United KingdomReviewed by:
Janine Bolliger, Swiss Federal Institute for Forest, Snow and Landscape Research (WSL), SwitzerlandBrett Michael Seymoure, Colorado State University, United States
Copyright © 2022 Kaunath and Eccard. This is an open-access article distributed under the terms of the Creative Commons Attribution License (CC BY). The use, distribution or reproduction in other forums is permitted, provided the original author(s) and the copyright owner(s) are credited and that the original publication in this journal is cited, in accordance with accepted academic practice. No use, distribution or reproduction is permitted which does not comply with these terms.
*Correspondence: Vera Kaunath, a2F1bmF0aDFAdW5pLXBvdHNkYW0uZGU=