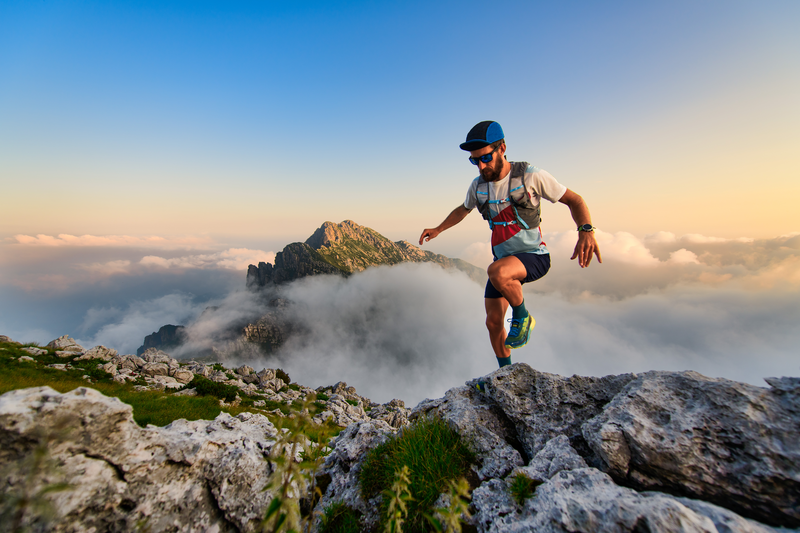
95% of researchers rate our articles as excellent or good
Learn more about the work of our research integrity team to safeguard the quality of each article we publish.
Find out more
ORIGINAL RESEARCH article
Front. Ecol. Evol. , 04 March 2022
Sec. Evolutionary Ecology of Social Behaviour
Volume 10 - 2022 | https://doi.org/10.3389/fevo.2022.727541
This article is part of the Research Topic Social Invertebrates as Models for Non-Kin Cooperation View all 12 articles
Relying on silk can promote sharing, especially when its presence means life and its absence, quick death. In the case of Embioptera, they construct silken tubes and coverings exposed on tree bark in humid and warm environments or in leaf litter and underground in dry habitats. These coverings protect occupants from rain and natural enemies. Of note, adult females are neotenous, wingless and must walk to disperse. Evidence is pulled together from two sources to explore mechanisms that promote the establishment of non-kin groups that typify the neotropical Antipaluria urichi (Clothodidae): (1) a review of relevant information from 40 years of research to identify potential drivers of the facultative colonial system and (2) experimental and observational data exploring how dispersal contributes to group formation. To determine risks of dispersal and decisions of where to settle, adult females were released into the field and their ability to survive in the face of likely predation was monitored. Additional captured dispersers were released onto bark containing silk galleries; their decision to join the silk or to settle was noted. An experiment tested which attributes of trees attract a disperser: vertical or horizontal boles in one test and small, medium, or large boles in another. While walking, experimentally released adult female dispersers experienced a risk of being killed of approximately 25%. Dispersers orient to large diameter trees and join silk of others if encountered. These results align with observations of natural colonies in that adults and late-stage nymphs join existing colonies of non-kin. Experiments further demonstrated that dispersing females orient to vertical and larger diameter tree-like objects, a behavior that matched the distribution of field colonies. The ultimate reason for the observed dispersion pattern is probably because large trees support more expansive epiphytic algae and lichens (the food for this species), although the impact of food resources on dispersion has not been tested. Finally, further research questions and other webspinner species (including parthenogenetic ones) that warrant a closer look are described. Given that this group of primitively social insects, with approximately 1,000 species known, has remained virtually unstudied, one hope is that this report can encourage more exploration.
For a diversity of insects and spiders, ecological factors that can influence differential success of individuals living a solitary life or as part of a group include the costs and risks of dispersal, pressures imposed by predators and parasites, and competition for resources (reviewed in Choe and Crespi, 1997; Costa, 2006). “Basic necessary resources,” sensu Choe and Crespi (1997), include silks because for many insects and spiders these proteins form critical defenses. Despite the value of shared silk, solitary individuals might do well to disperse to seek uncontested food or to avoid parasites that might have built up in the colony. Solitary silk spinners often can quickly construct a new domicile. But are they better off? Sharing the tasks of spinning and silk production and the more extensive silk structure produced by a group might be a better strategy. Possible reasons for remaining part of a colony include greater protection from predators, more efficient prey capture if silk is used as a snare, taking advantage of the spinning by colony-mates and/or avoiding the costs and risks of dispersal. In pholcid spiders, for example, spiderlings grow more quickly and attain larger sizes when they share silk spun by larger conspecifics in the colony (Jakob, 1991). The tendency to share silk by social spiders of the genus Stegodyphus helps to protect individuals from predators as well as more efficiently traps prey (Seibt and Wickler, 1990). A detailed field study of S. dumicola in Namibia showed that arboreal ants could be held at bay by social spiders capable of producing copious sticky silk (Henschel, 1998). However, solitary spiders in that population were better able to avoid a contagious fungal disease that more easily spread in large colonies. Apparently, S. dumicola exists in solitary and social groups because of fluctuating costs and benefits of dispersing vs. remaining in their natal group to share silk abodes. Dispersal tendencies and sociality levels in spiders have also been shown to correlate in an investigation of seven species of Anelosimus (Corcobado et al., 2012). By analyzing morphological traits, the authors found that higher degrees of sociality were negatively correlated with the tendency and ability to disperse.
Populations composed of solitary and colonial reproductives typify another silk-sharing arthropod, insects of the little-known order Embioptera (a.k.a. Embiidina and Embiodea), commonly known as webspinners. The purpose of this report is to identify factors related to the establishment of non-kin groups, the previously demonstrated structure for the neotropical webspinner Antipaluria urichi (Saussure) (Family Clothodidae)—the subject of numerous laboratory and field experiments and censuses (reviewed in Edgerly, 1997, 2018). For this species, evidence points to colony structures that vary from solitary females with their offspring to groups of non-kin adult females that share contiguous silk coverings (Edgerly, 1987a). Field collections of webspinner species over a hundred years demonstrated that some of the estimated 1,500–2,000 species (mostly undescribed, Miller et al., 2012) form colonies of adult females with their young (e.g., Melander, 1903; Bradoo, 1967; Edgerly et al., 2002, and reviewed in Edgerly, 1997; Costa, 2006), whilst others live as single mother-offspring groupings (Edgerly et al., 2002). Indeed, in some species, solitary females are quite aggressive toward others and do not exhibit group-living (Ross, 2000a,b). The phylogenetic pattern and ecological correlates of these different social groupings are not known. This report is multi-faceted, describing basic biology and filling in gaps in knowledge, as follows: (1) a review of the natural history of A. urichi and of research findings related to the cost and benefits of group living, (2) presentation of methods and results of experiments on dispersal behavior of adult females, especially relevant to the question of how non-kin groups form, (3) an analysis of the risk of predation for solitary and colonial adult females, and (4) discussion of avenues for further research to address why A. urichi disperse from their natal colonies and why they often join non-kin groups rather than settle as solitary females raising their young. Lastly, given the lack of knowledge of embiopteran colony structure throughout the range of this cosmopolitan order, a brief summary of lifestyle diversity for webspinners, needed future studies to address missing information, and comparison to other silk-sharing arthropod species will be presented.
For embiopterans, silk functions as protective armor and shapes their lives—morphologically and behaviorally. Sharing silk may have promoted social behaviors in some species who gain an advantage from more expansive coverings. From arboreal bark-dwellers to subterranean crevice-dwellers, webspinners all have the same body shape and basic lifestyle (Figure 1)—a very different scenario when compared to the more hyper-diverse taxa in the Class Insecta. Nymphs and adults alike spin by stepping with their front legs, while releasing dozens of nano-scale silk fibers from modified hair-like ejectors that clothe the bottom of their front tarsi (Büsse et al., 2019). They execute elaborate spin-steps to fashion tubular galleries and sheets of tissue-like waterproof coverings (Osborn Popp et al., 2016; Stokes et al., 2018; Harper et al., 2021) that protect them from rain and predators (Figures 2A–E). Adult females are neotenous (Figures 1, 2F), flexible, and soft-bodied whilst adult males, usually winged and short-lived, sport wings that fold up so they can run backward and forward inside the silk without getting tangled (Ross, 2000a). Embiopterans are also known for the order-wide occurrence of maternal behavior (Ross, 2000b). Adult females vary in how they handle and protect their eggs, but generally, they cluster them—often with elaborate coverings of macerated materials and silk that can act as a shield against the threat of parasitism—and guard them (Edgerly, 1987a,b).
Figure 1. Portraits of Antipaluria urichi. (A) Adult male (winged individual, 1.2 cm long) and female (1.5 cm long) in a lab culture container of dried Live Oak leaves and silk; (B) adult females from five taxonomic families, displaying typical uniform shape and a range of colors (body length not to scale). Families from left to right: Australembiidae, Archembiidae, Embiidae, Oligotomidae, Clothodidae.
Figure 2. Variation in silk domiciles and habitat for webspinners and potential predators of Antipaluria urichi of Trinidad. (A) Haploembia tarsalis and (B) H. solieri silk in California. (C) Pararhagadochir trinitatis silk in Trinidad. (D) Notoligotoma hardyi silk on granite outcrop on Magnetic Island. (E)Metoligotoma incompta silk on Magnetic Island, Queensland. (F) H. tarsalis adult female (1.0 cm in length). (G) A. urichi silk in Trinidad showing thick silk typical of an egg-guarding female. (H) Bay-headed Tanager and Woodcreeper, two birds seen tearing into Antipaluria urichi silk. (I) Gecko preying upon adult female of A. urichi caught outside of her silk. For scale, the webspinner is approximately 1.7 cm in length. Photographs by the author; painting of bird predation by Edward C. Rooks.
Between 1982 and the present day, I along with numerous co-authors have conducted experiments and censused field colonies of A. urichi to determine the extent of their silk coverings and number of occupants (Edgerly, 1987a,b, 1994), types of trees that support them (Edgerly, 1987c), environmental correlates that predict their presence and abundance (Shenoy et al., 2020), cost of spinning silk (Edgerly et al., 2006), how they spin (Edgerly et al., 2002, 2020; Büsse et al., 2015, 2019; McMillan et al., 2016), as well as attributes of their silk and how it acts as waterproofing (Osborn Popp et al., 2016; Stokes et al., 2018; Shenoy et al., 2020; Harper et al., 2021). Related to the occurrence of non-kin groups, the first question posed for my Ph.D. work, under the guidance of the late George Eickwort of Cornell University, was basic: “what type of social behavior do webspinners exhibit?” Before that time, no one had conducted quantitative field experiments, although several observational studies converged on the characterization that embiopterans exhibited maternal care of young (reviewed in Edgerly, 1997; Costa, 2006; Edgerly, 2018). Additionally, evidence of expansive colonies in the more humid tropical regions was especially intriguing (Ross, 1970). Trinidad’s field station, Simla, was the chosen site for my dissertation work because of reports that arboreal colonies of A. urichi (at that time, known as Clothoda urichi) were abundant and easy to find.
As informed by field censuses, A. urichi is characterized as facultatively colonial (Edgerly, 1987a; see below for more details). They feed on epiphytic lichens and algae on vertical surfaces, including large outdoor plant pots, cement walls, road banks and especially trees of a variety of species (Edgerly, 1987c). They continually extend their tissue-like silk to cover new feeding sites during nocturnal activity bouts (Edgerly, 1987b). Adult males are winged (Figure 1A) and they fly from their galleries in the afternoons in search of females (personal observation). The male’s antennae are long and covered with chemoreceptors and pheromonal communication is likely although not investigated for any webspinner species. Males do not feed after reaching maturity and die soon after mating. Females produce an elaborate egg mass averaging 53 eggs (Edgerly, 1987b). They coat each egg with a cement-like material, sticking the eggs to the substrate in organized rows, covering the clustered eggs with gathered, macerated materials and silk, and topping the whole structure with thick silk (Edgerly, 1987b). As demonstrated by detailed time budgets, the mothers stand guard for approximately 6 weeks, helping to prevent egg parasitism by ubiquitous scelionid wasps (Embidobia urichi Ashmead) (Edgerly, 1987b). If the webspinner mother is not present, the wasps can dig through the egg mass covering (0.16 cm thick on average) to reach and parasitize the eggs. Maternal care is necessary to keep the silk intact to avoid parasitism by the wasps and predation by ants of the eggs (Edgerly, 1987b). The outer silk of a domicile constructed by egg-guarding females is relatively thick (Figure 2G). Nymphs rely on their mother’s production of silk, which increases exponentially in area after the eggs hatch because of her emphasis on spinning (Edgerly, 1987b,1988). The young grow faster in her presence (Edgerly, 1988), a phenomenon also known from other webspinner species (Rita, 1993; Choe, 1994). A laboratory experiment on the cost of silk also showed that nymphs attained smaller size as adults on average if they were repeatedly forced to replace stolen silk (Edgerly et al., 2006). A European species (Embia ramburi) is known to collect and retrieve food pieces for their young (LeDoux, 1958) but A. urichi does not display this more elaborate maternal investment. In fact, their colonies deplete food resources under the cover of silk, which is extended to enclose more and more food as the nymphs develop. Central-place foraging habits displayed by other social insects is not adopted by A. urichi and as will be proposed below, may partly explain their dispersal behavior, a focal topic for this report.
The silk of A. urichi is a diffuse cover over resting and foraging sites. These characteristics reflect the point made by Choe and Crespi (1997) in their review of silk-sharing species—that is, individuals living in such a diffuse domicile cannot easily monitor or dominate others in the group. In fact, colonial females share silk but do not appear to cooperate in other ways. Silk coverings found at one field site in the Northern Range Mts. of Trinidad varied from 11 cm2 for a solitary female to 37,000 cm2 for colonial females. One large colony contained 72 individuals, with 24 being adult females. At one location, a survey of 44 colonies found 86% of 138 adult females shared silk with others. While at two other sites, 37% of 57 (Edgerly, 1987a) and 60% of 35 (Edgerly, 1994) adult females were colonial, indicating the commonality and variability of the facultatively colonial structure. Individual colonial females lay significantly more eggs than do solitary females (Edgerly, 1987a). The underlying cause of the difference in the number of eggs laid by solitary and by colonial females is not known. Laboratory experiments on the cost of spinning showed that the number of eggs laid by females that had their silk repeatedly stolen, necessitating replacement, did not differ significantly from those laid by controls (Edgerly et al., 2006). Despite being able to produce more eggs in field colonies, colonial females experience higher rates of egg parasitism and in the end, the number of first instars that emerge do not differ significantly between solitary and colonial females (Edgerly, 1987a). Even though the question of proximate causation remains, higher egg counts appear as a potential benefit and higher parasitism rates appear as a potential cost of group-living.
Webspinner species differ in how much silk they produce in the field and in the lab, even during the short term. One hypothesis is that arboreal species that live exposed on bark surfaces in rainy tropical regions require more silk as cover than those that live in dry habitats under rocks and in leaf litter where substrate materials are stitched together to form protective domiciles (Edgerly et al., 2006). Requiring more silk would be a reason to join others, so that the silk production and spinning task can be shared. Indeed, laboratory experiments comparing silk production and gregarious tendencies for the arboreal A. urichi and for the litter dwelling Metoligotoma incompta (Ross) (previously Australembia incompta; Family Australembiidae) revealed that the arboreal species produced significantly more silk in a short amount of time (Edgerly et al., 2006). Furthermore, when allowed to disperse one at a time into mock habitats of bark and lichens, A. urichi females settled into a contagious dispersion pattern, with an average distance between females of one body length. The litter-dwellers did not spin any silk when placed in petri dishes during a 3-day trial, unless a leaflike object was provided. They also spread out randomly rather than settle near each other when allowed to disperse into a mock habitat. They averaged 1.7 body lengths away from each other and did not share silk. These results are limited in scope because differences in spinning behavior might be due to other underlying conditions, such as differences in food sources, silk proteins and the like. Given the availability of a phylogeny (Miller et al., 2012) and our knowledge of many more species that vary in silk spinning tendencies, a range of species can now be studied to address a similar research question in the future. Nonetheless, A. urichi is a spinner that produces copious silk and is likely to settle near another individual when given the chance. The laboratory results align with the field observations noted above, and with the behaviors described below.
Previously published census work established that natal dispersal is a regular feature of A. urichi life cycle. The occupants of 64 colonies, defined as distinct patches of silk and marked with flagging tape around the field station at Simla, were monitored for residents (including individually marked adult females), presence of eggs, hatch rates, parasitism, evidence of predation and so forth in an area approximately 1,400 m2 (Edgerly, 1987a,b, 1988). During the field season between September and December 1983, 16 unmarked adult females and 10 late-stage nymphs migrated in from elsewhere and settled into the flagged colonies (Edgerly, 1988). New silk galleries also appeared on trees near the previously flagged colonies: 31 established by single adult females and nine by solitary late-stage nymphs. By the time their offspring were approximately half grown, none of the mothers were still present (Edgerly, 1988). Reproductive females with eggs or nymphs were found dead in the silk (n = 3), disappeared for unknown reasons (n = 25), or after predator attacks either by ants or after large holes were torn in the silk above their resting sites (n = 16) (see Table 2 in Edgerly, 1988). They do not display overlapping generations nor have females been seen to lay more than one batch of eggs. Previous work on maternal care of eggs and nymphs (Edgerly, 1987b,1988) supported the conclusion that a female lays one batch of eggs, spends 6 weeks protecting them, and then remains with her nymphs until she dies or disappears. No instance of repeated egg-laying has been observed for this species but the question remains whether it is possible. During the field census, the number and development of nymphs in fifteen colonies that could be closely monitored was recorded. The nymphs slowly disappeared over time, until only two colonies produced adult females in situ (2 in one and 11 in the other) (see Figure 1 in Edgerly, 1988). The regular disappearance of nymphs from their natal galleries and the mere appearance of new silk galleries reflects the potentially high rate of natal dispersal by A. urichi.
Predators, birds and especially ants, cut into silk and kill or catch and carry away their embiopteran prey (Edgerly, 1988, 1994; Figures 2H,I). Because silk functions as a shield for the soft-bodied insects inside, exploring how cuts and tears in the silk accumulate can be used as a measure of predation risk. The two-dimensional silk covering of A. urichi is conspicuous, the area of a colony is easily quantified, and the occupants can be counted. To determine predation rate as a function of silk area and individuals within, I spent 3 weeks monitoring 47 colonies in the rainforest at the Asa Wright Nature Centre in Trinidad, documenting in detailed drawings the cut and torn holes as they accumulated (Edgerly, 1994). Even if holes were ultimately patched by the occupants, scars were still discernible. My assumption was that at least some of these holes reflected the predation attempts like those previously witnessed. At that time, I was interested in whether larger silk expanses attracted more attention than smaller patches to test the encounter effect hypothesis (sensu Turner and Pitcher, 1986). The prediction was that larger silk patches accumulate relatively fewer attacks based on area alone because they are not proportionally more attractive than smaller patches. Most ants will walk over the silk as if it were part of the substrate and not as if it were possible prey, but others do cut in as mentioned. I found that cut holes accumulated less than would be expected based solely on the perimeter of the silk covering. I also discerned that a measure of risk (number of holes per individual per silk perimeter) varied greatly, with smaller colonies experiencing the greatest variability in potential risk per individual: both the lowest and the highest risk levels were in this category. I concluded that individuals dwelling under smaller patches of silk, as is true for solitary females, would experience unpredictable and sometimes very high levels of risk from predators. What I did not test at the time was whether solitary adult females suffered greater risk of attacks than those living in colonies. Because adult females are mostly responsible for spinning and contributing to the expansion of silk, their presence matters more than the total count that includes nymphs. To address the question of why dispersing adult females join others, I re-analyzed the data from Edgerly (1994) for this report to examine how much risk adult females might experience. I sorted them into three groups: solitary adult females, solitary mothers with nymphs, and colonial adult females, which ranged from two to seven at the field site. The reason solitary females with nymphs are considered as a separate group is because once the eggs hatch, the mother expands her silk area exponentially compared to the time she is guarding her eggs (Edgerly, 1988). My prediction is that solitary females (without nymphs) will experience the greatest variability in risk and have less silk on average. They might be completely missed by hunting predators because the silk patch is small. But if they are attacked, the higher risk level (the holes per individual per silk expanse) means they likely will be killed.
This report emphasizes field surveys and natural history to establish context as well as experimental work and quantitative observations. A major intent is to share knowledge of this little-known order of insects while also addressing questions about the dynamics of colony structure. Experiments on the behavior of dispersers and associated risks, described in Edgerly (1987c), have not previously been published. Because of the relevance to how non-kin groups form, the methods and results are presented below.
To observe the process of dispersal, five naturally dispersing adult females were caught walking in the open in the field many kilometers away from Simla and brought back to the field station. Over 2 days, four trials for each female were conducted; each female was allowed to walk out of a petri dish and onto the base of a tree trunk that supported small silk galleries occupied by at least one resident individual A. urichi. The actual number of occupants was not determined because opening the silk to count them would have disturbed the silk. The experiment revealed if dispersers try to settle in already spun silk or if they settle in a spot to spin their own domiciles. Trials were ended when the disperser entered the silk but then left the silk completely (scored as “not joined”) or when agonistic interactions between resident or the disperser stopped for at least 5 min and the disperser remained sitting still in the silk (scored as “joined”). After each trial, the dispersers were collected and returned to their petri dishes. Each female was tested again on a different tree.
Dispersal behavior by these wingless soft-bodied insects without any obvious defenses, except to run fast backward (a unique ability of webspinners), appears particularly risky. To determine how risky, pre-reproductive adult females (n = 33) were released on the ground at the field station and followed. Their fate was recorded as “killed by predator,” lost from view while walking, or settled onto a tree either alone or having joined another’s silk.
To investigate behavior of dispersers in a controlled environment, pre-reproductive adult females (n = 20) were collected in the field, held in petri dishes, and fed for 2 days prior to testing. Their choice of a tree-like habitat was tested in a 9 m2 indoor space wrapped with white cloth to create an arena with diffuse lighting. A hole in the cloth provided viewing by a hidden observer. A vertical stick (1 cm diameter, 30 cm tall) was affixed in the center of the arena and the test objects providing mock habitats for dispersers were arrayed around the arena edges. In the first test, one vertical and four horizontal logs (9 cm diameter, 1 m long) were placed in the corners, evenly spaced and 1 m away from the center vertical stick. The logs were rotated clockwise after each trial. For each trial, a female was released into the center of the arena near the vertical stick. This method was employed because when dispersers were released during field trials, they climbed up a nearby object (such as a vertical grass stem) and turned their heads back and forth before setting off toward a nearby tree. The central vertical stick provided such a climbing post for the dispersers; they all climbed it when released into the arena. In the second experiment, females (n = 15) were given the choice of three differently sized models of vertical logs, made of brown paper (diameters of 7, 11, and 30 cm), again rotated between trials, and placed 1 m away from the central stick. This experiment was replicated three times with the same females tested in random order but without individual identification. A Chi-squared Goodness of Fit test was employed for each trial, with a null hypothesis of random distribution relative to the three choices in each trial and a significance level of P < 0.05. For this and other statistical analyses, JMP Pro 16 software was used by SAS Institute (2021). To gain an understanding of natural dispersion, the presence and absence of colonies on all trees equal to or larger than 10 cm diameter at breast height (DBH—bole diameter measured at approximately 1.4 m from the ground; Cooperrider et al., 1986) was recorded at another site, an abandoned tonka bean plantation on the Arima-Blanchisseuse Road near the Asa Wright Nature Centre.
Relying on data from Edgerly (1994), I conducted non-parametric analysis of variance (Kruskal-Wallis test) to determine if predation risk differed between three types of colonies: solitary adult females (n = 7), solitary adult females with nymphs (n = 7), and colonies with grouped adult females with or without nymphs [n = 6; mean number of adult females = 3.5 ± 0.85 (SE)]. Predation risk was measured as the number of holes cut/perimeter of silk/number of individuals in the silk that accumulated over 3 weeks. Area of silk per colony sorted in the three groupings based on occupants was also compared.
All five released dispersers entered a naturally occurring silk domicile that they discovered while walking up the tree bark. Four of the dispersers joined the occupant of a silk domicile for three trials but did not join in one trial (entered but quickly left). The fifth disperser joined the discovered silk domicile in all four trials. In 14 of the 20 trials the resident of the domicile responded with vigorous shaking as the disperser entered the silk. The agonistic interactions were usually not enough to push out intruders; those dispersers that joined moved away from the resident to settle a short distance away but still within the now-shared silk covering.
Dispersing females released in the field initially climbed onto a vertical stem or protruding leaf litter and turned their heads back and forth, appearing to scan the surroundings. They then dropped off their perches and walked directly to large vertical shapes. In an open grassy area near the field station buildings, they (n = 12) walked quickly at approximately 16 cm per minute for about 6 m toward towering objects: the field houses or a large stand of mahogany trees. Six were killed before reaching their destinations. In the forest, they (n = 21) moved at 20 cm per minute. They walked an average of 3 m before climbing large trees; two were killed on the ground. Of the 19 tree climbers, I was able to follow nine, losing sight of the others; four entered pre-existing silk and five settled on bark to spin on their own. This result reflects the distribution of females in the field: solitary and colonial females exist in the same population. Tree climbers regularly nibbled the surface of the bark, appearing to sample the quality of the habitat. In summary, mortality due to predation for 33 walking females was at least 24% (six by ponerine ants and two by wolf spiders), reflecting the riskiness of walking in the open.
In all trials, females climbed to the highest point of the central stick, turned their heads scanning the room, returned to the floor, and walked directly to one of the objects. In 17 of 20 log orientation trials, females chose the vertical instead of the horizontal logs (Goodness of Fit Test X2 = 38.4; DF = 2; P < 0.005) and position in the room did not matter (Goodness of Fit Test X2 = 0.4; DF = 2; P = 0.5). In the test of size preference, females overwhelmingly preferred the largest log in two trials but split between large and medium sizes in the third trial (Figure 3). The colony dispersion pattern at the field census site again reflected a preference for recruitment to and success on large trees (Figure 4).
Figure 3. Dispersal choices by adult female Antipaluria urichi in the laboratory. Paper cylinders represent trees of three sizes: 7, 11, and 15 cm diameter and 1 m tall. Each tree model was 1 m away from a central stick placed where the disperser would climb to survey the scene. Relative positions are not to scale in the drawing. Numbers represent the number of females choosing each cylinder in the choice test. Trial 1 results shown in red letters (Goodness of Fit Test X2 = 30; DF = 2; P < 0.0001; Trial 2 in blue letters: Goodness of Fit Test X2 = 24.4; DF = 2; P < 0.0001) and Trial 3 in orange letters (Goodness of Fit Test X2 = 4.8; DF = 2; P = 0.09).
Figure 4. Colony dispersion on trees of different sizes in an abandoned Tonkabean plantation in the Northern Range Mts of Trinidad. DBH, diameter at breast height.
Silk coverings for adult females differed in area, with solitary females covered by less silk area than grouped females (Kruskal-Wallis X2 = 10.423; DF = 2; P = 0.005; Figure 5A). The finding that some solitary mothers with nymphs displayed more expansive silk than did egg-guarding mothers reflects their behavior of dramatically increasing silk production after their eggs hatch (Edgerly, 1988). The median risk of predation (number of holes per perimeter of silk per individual) did not vary between solitary, solitary with nymphs or colonial adult females (Krusal-Wallis Test: X2 = 0.7685; DF = 2; P = 0.6810). The variances for risk were significantly different, with solitary females showing the greatest variation (Bartlett’s test: F = 16.1006; DF = 2; P < 0.0001; Figure 5B).
Figure 5. Silk colony sizes and risk of predation for field colonies of Antipaluria urichi. Box plots show medians, quartiles and ranges. Sample sizes: solitary adult females (n = 7), solitary females with nymphs (n = 7), and grouped females (n = 6). (A) Area of silk expanse (cm2) for adult females in the three different colony types. (B) Risk of predation as measured by accumulation of cuts per perimeter of silk covering per individual recorded over 3 weeks in field colonies in the Northern Range Mts of Trinidad.
Dispersal behavior is a focus of research on social behavior because of its role in shaping how groups form and/or if they dissolve. For example, when sexually mature offspring delay dispersal and remain in their natal nest or colony, helping behaviors have evolved in a variety of social animals including birds (Koenig and Dickinson, 2016), ambrosia beetles (Nuotclà et al., 2021), small carpenter bees (Rehan et al., 2014) and social spiders (Henschel, 1998) to name a few. These examples represent groups of kin that cooperate at some level, which contrasts A. urichi colonies that vary from solitary mother-offspring groups to colonial non-kin reproductives that share silk domiciles. My detailed field censuses early on showed that dispersal out of the natal colony is a feature of the life cycle of A. urichi and that aggregations are not composed of overlapping generations of kin (Edgerly, 1988). The reasons that A. urichi disperse must be critical for survival because walking outside of their silk covering is very risky; the reasons they join others to share silk when they do disperse still needs to be resolved.
Dispersing A. urichi tend to walk to large trees and in their rainforest habitat, these trees often support conspecifics, as reflected in the dispersion of colonies of trees shown in Figure 4. If a disperser encounters silk, she will enter it. This behavior can lead to the establishment of non-kin groups if the disperser settles there. Evidence revealed by tracking dispersers in the field suggests that the risk of being outside of silk is high enough that entering silk upon its discovery promotes survival. When experimental dispersers chose to enter another’s domicile residents responded aggressively. Detailed laboratory experiments examining how residents respond to intruders (Dejan et al., 2013) showed that resident adult females signal with various shaking movements when individuals attempt to join their silk. The same response by residents was observed for the field disperser experiment described above. In the 2013 lab experiment, individual pre-reproductive females shook when an intruder entered their silk but quickly stopped signaling and allowed the intruder to settle next to her. Egg-guarding females, in contrast, displayed more vigorous, numerous and varied signals (lunge, shake, snapback, and push-up) directed toward female intruders (nymphs and adults alike) (see Figure 5 in Dejan et al., 2013). Despite the more aggressive responses by these egg-guarders, intruders tended to settle in the silk covering but would stay a short distance from the resident female thus avoiding triggering her aggressive responses. Of interest is the fact that an adult male that entered the silk of an egg-guarder triggered signals but once the male contacted the female she stopped signaling. Females were much more reactive when the intruder was a female.
If one considers the behavioral repertoire just described, it appears that A. urichi females join silk structures for immediate safety. They do not appear to cooperate with the residents except to extend the existing silk structure for their own purposes. Why are residents aggressive when dispersers enter their silk? A possible underlying selective pressure includes higher egg parasitism rates when other nearby egg-layers share silk (Edgerly, 1987a). Untested possibilities include competition for food and risk of cannibalism of eggs and perhaps of vulnerable newly molted nymphs (Edgerly, 1987c). It is not known if colony-mates represent a threat, but as hypothesized by Ross (2000b), cannibalism remains as a possible reason why even the nymphs can be aggressive toward non-kin dispersers that entered their silk abodes.
When silk spinners join together, one might expect that the group would gain an advantage from numbers alone. As described in reviews of the evolution of social behavior (e.g., Costa, 2006; Taborsky et al., 2021), augmented groups can benefit for a variety of reasons including shared vigilance, territory defense or thermoregulation. More adult webspinners in a group can mean a greater expanse of silk, as shown in Figure 5A and in previous reports from field censuses (e.g., Edgerly, 1987a,1994). The hypothesis that grouped adult females do better against predation threats has support only if high variability in the intensity of predator attacks on silk matters, because the median attack rate did not differ for solitary and grouped adult females. Other hypotheses to explain why non-kin groups form remain to be tested, as follows: (1) Females might join others and stay to oviposit because the food is better in particular spots, possibly explaining the higher egg counts for colonial females detected in the field colonies (Edgerly, 1987a). (2) Females join others because more extensive silk protects better against the elements, like heavy tropical rains. Grouped females might be able to recover more quickly after the silk is damaged as has been shown for social spiders that live in larger groups in their rain forest environment (Hoffman and Avilés, 2017). (3) Females join others because their nymphs have a better chance when the silk is more extensive, especially if the mother dies early in their development. Given that nymphs grew more slowly without their mother in a field experiment (Edgerly, 1988) and nymphs achieved smaller sizes on average when their silk was experimentally stolen in the laboratory (Edgerly et al., 2006), a shift in focus to examine offspring success as related to gregarious tendencies seems warranted. (4) Despite contrary evidence from laboratory experiments (Edgerly et al., 2006), females that join others in the field might benefit because they can share in the potentially costly effort to maintain the silk structure, as has been seen in colonial spiders (Uetz and Hieber, 1997). Sharing silk and spinning with others might allow webspinners to lay more eggs.
A 5-month (October through February) field experiment at Simla designed to test the important question of how food resources influence group success and dispersal failed because of persistent predation. Therefore, competition between individuals in colonies of various sizes and how that influences dispersal probability remains untested. The hypothesis was that dispersal by individuals can be triggered by low availability of food resources. If this were true, individuals in colonies established on boles with abundant food (epiphytic algae and lichens on the bark) would remain in place while those with limited food would disperse in search of food. The experimental design also integrated a test of predation as a trigger for dispersal; the high incidence of loss of individuals co-occurring with torn silk in natural colonies around the field station suggested such a connection. During the experiment, adult females placed on uncaged boles were not able to colonize because of high rates of predation. Dozens of attempts failed. Naturally dispersing adult females also showed up on the experimental boles, but they too were attacked. Even within a large exclusion cage designed to exclude predators, ants ultimately found entrances and predation could not be avoided and remained high. Failure of the experiment due to predation supports the hypothesis that predation is a major selective factor for A. urichi. Unfortunately, the roles of competition for food and predation in regulating dispersal and colony structure remain unknown.
For arthropods, silk as a domicile might be costly to produce and/or functions better when voluminous and thick. For insects, silk is mostly composed of simple amino acid building blocks such as glycine, alanine, and serine. This is true for embiopterans as well (Okada et al., 2008; Collin et al., 2009, 2011; Harper et al., 2021). Craig (1997) proposed that such silk proteins are not expensive for an insect to produce. However, the step energy (sensu Peakall and Witt, 1976) required to spin it might incur costs. Therefore, gregariousness by silk spinning insects might have evolved in a variety of lineages, to reduce such costs for immatures and/or for adults. Evidence from A. urichi, as reported above (based on Edgerly, 1988; Edgerly et al., 2006), suggests that nymphs might be more impacted than adults by the costs of spinning. Time budget analysis has shown that investment in silk production and spinning by A. urichi mothers increases after her eggs hatch (Edgerly, 1987b,1988). Joining another adult female could be one mechanism helping her offspring gain support from another’s silk if she were killed early in their development. In contrast to these examples, consider that while caddisflies incur a cost when silk is stolen from them and replacement is required, leaf-rolling gelechiid caterpillars surprisingly fared better when their silk was experimentally taken (Loeffler, 1996). Leoffler suggested that the displaced caterpillars moved onto fresh leaves and therein gained a benefit from better nutrition despite the increased investment in replacement webbing. That finding is interesting in that the natural situation is for larvae to stay within the silk domicile, safe from predation, despite declining food value.
Caterpillars often form groups that share the work of spinning their shelters as described in a review by Costa and Pierce (1997). The authors reported that sociality is widespread within at least 20 families within the order Lepidoptera (Costa and Pierce, 1997, see Table 20.1). Some species are so-called patch-restricted while others are central-place foragers. The former encloses leaves (their food) with silk, which is depleted over time in the same manner as resources are exploited by colonies of embiopterans. Central-place foragers build a shelter and move out periodically to find fresh food and return to their tent to digest, rest and hide. These caterpillars display sophisticated signals, such as trail-marking and recruitment to high quality leaves (Fitzgerald and Peterson, 1983; Fitzgerald and Costa, 1986). The life history characteristics that shape caterpillar social behaviors are strongly influenced by foraging constraints and indeed, the heuristic model presented by Costa and Pierce (1997; see Figure 20-3) emphasized diversity of foraging styles. The three-dimensional tents serve as communication centers for the larvae as they tackle their often difficult and ephemeral food. These traits do not appear to typify webspinners beyond the silk-sharing tendencies. The communication signals we discovered are short-distance messages between egg-guarding females and possible intruders; not signals for an entire diffuse colony of silk-sharers (Dejan et al., 2013). Furthermore, based on my experience and that of Ross (2000b), webspinners from throughout their range can be reared in the laboratory on the same foods: romaine lettuce and lichens. This generalist diet is not true of caterpillars which often are highly specialized foragers. Costa and Pierce’s review did not focus on the relationship of silk to potential predation or parasitism and dispersal risks, the factors I propose as having shaped the group-living behavior of A. urichi.
Costa and Pierce (1997) noted that gregariousness for tent-building caterpillars can evolve in an ecological context where family structure and relatedness are irrelevant. The authors discovered in their review of the literature that no one factor underlies the evolution of group-living for social caterpillars. Super colonies can form when sibling groups merge with others to share silk tents, and where relatedness is quantifiably low, hence the colonies are effectively non-kin groups (Costa and Ross, 2003). Promoting this assemblage, adult females preferentially lay their egg masses next to other egg masses. The offspring spin tents as sib-groups but as they develop, they merge with other groups and form super colonies. The relatedness coefficient for such a colony defined by who shares a tent shifts from 0.5 for young instar larvae to 0.25 for older larvae because of mixing later on (Costa and Ross, 1993).
Costa (2006) produced a marvelously insightful and helpful 767-page tome called “The Other Insect Societies.” Digging into his thoughts about the evolution of social groups, I discovered that webspinners display a selection of his proposed common denominators of group formation; not unexpectedly given that the order Embioptera is featured as one of the chapters. These common denominators are biotic and abiotic ecological pressures, such as predation, parasitism, and environmental challenges. Costa also named thermal challenges as another pressure. I explored the question of thermal challenges for the tropical rainforest A. urichi and two Australian species that live in the hot environment of Magnetic Island in Queensland (Edgerly and Rooks, 2004). Silk domiciles provided little protection against strong solar radiation for the Australian species; instead, they displayed adaptive microhabitat selection to avoid overheating in the field. In our laboratory experiments, we found that effective heat shock proteins, not related to the silk’s ability or lack thereof, prevented death due to heat shock for the Australian species (Edgerly et al., 2005). Antipaluria urichi, in contrast, suffered heat shock and death at comparably lower temperatures, not surprisingly given that the temperature range is narrow and moderate in their environment. My unquantified observations in the field suggested that rainfall, not heat, is an ecological challenge for the tropical rainforest A. urichi that can be solved by their silk covering (Stokes et al., 2018). Their silk functions as a raincoat, protecting the occupants from tropical downpours which would otherwise knock individuals from their bark perches and soak them—a problem that must be avoided given that they die very quickly if wet (personal observations). The question remains whether sharing silk with other individuals enhances the raincoat, which would also function underground where flooding would likely kill the detritivores like Haploembia solieri, Oligotoma nigra, and M. incompta. Costa (2006) also proposed that the common denominators for group-living promote communal oviposition, merging of unrelated groups and the absence of kin discrimination. These features hold true for A. urichi and a few other species for whom field censuses revealed similar facultatively colonial structure of their populations (see reviews Edgerly, 1997; Costa, 2006).
Dependence on silk for protection typifies other arthropods, such as species of Psocoptera and Acari. Some psocids live in enormous colonies, producing a thin silk mesh that can cover entire tree trunks (New, 1985). Little is known, however, about which advantages accrue to individuals in these colonies (reviewed in Costa, 2006). Mites in the genus Schizotetranychus also live in large groups within silk abodes (Saito, 1997). Differences between the mites and webspinners are great, however, making it hard to find common denominators, sensu Costa (2006). The mites are haplodiploid while webspinners are diploid, except for a few polyploids described by Stefani (1953, 1956) and Kelly et al. (2018). The spider mites cooperate in nest building, whereas for A. urichi at least, silk spinning is by individuals that merely join each other’s silk, adding their own to the expanding 2-dimensional cover. The mites also cooperate in brood defense and show overlap of generations. Finally, Saito (1997) concluded in her review that the mite’s complex sociality evolved in the context of kin selection. For other arachnids such as social spiders, kin selection plays a role in facilitating group formation (Avilés, 1997).
One webspinner species (E. ramburi) constructs a nest-like domicile where food is brought by the mother from the outside (LeDoux, 1958). This central-place forager exhibits a behavior seen in other insects that display more complex communication and social behaviors (reviewed in Costa, 2006). Other webspinners are asexual; their behavior varies in unpredictable ways if one assumes that closely related individuals in a family will tend to be gregarious and gain advantages from sharing silk. For example, adult females of the Mediterranean species H. tarsalis (Figure 2D; formerly known as an asexual race of H. solieri, see Stefani, 1956) are aggressive; they fight, repel each other, and do not form groups (Kelly et al., 2018). In contrast, a closely related sexual species, H. solieri forms large aggregations in the same habitat (based on personal observation) and are gregarious in the lab (Kelly et al., 2018). Both species live in leaf litter and in underground burrows in the same habitat. The reasons for the differences in social tendencies for these two species are not known and are worth exploring. In contrast to H. tarsalis, the parthenogenetic Rhagadochir virga (formerly Scelembia virga; Ross, 1961) of Zambia forms large, apparently coordinated groups (personal observation). In laboratory cultures at Santa Clara University, adult females would periodically assemble on the sides of the container as if ready to disperse en masse. They also scattered their eggs in small clumps, did not stand guard but provisioned them with gathered pieces of lichens that perhaps would feed the neonates. The colony also had a lovely odor, like maple syrup. Was this a pheromone coordinating group behaviors? No field studies exist to date for this interesting gregarious species living in a seasonally dry-wet environment.
Predation-dilution effects, feeding facilitation and features of the environment can all play a role. For Embioptera, such a comprehensive review cannot be assembled because of scant information. On a positive note, more scrutiny is now possible as we have a phylogeny (Miller et al., 2012) to assist in making independent contrast tests of hypotheses focused on the evolution of group-living and difference in degree of reliance on silk. Environmental variation is known as well: based on my own experience, I have found species living in arid conditions (Australia), very wet and cool places (Andes of Ecuador), seasonally dry and seasonally wet (Zambia; Thailand), and places that are hospitable all year (tropical rain forests in Ecuador, Trinidad). For a comprehensive review of habitats and habits, see Ross (2000b)—an entomologist responsible for collecting from around the world most of the specimens now held in the collection at the California Academy of Sciences, United States. Some species experience egg parasitism and others do not. Some species suffer from nymphal parasites (Family Sclerogibbidae, Order Hymenoptera) and others do not (Ross, 2000b). Variation in environmental pressures is present and interestingly, as discussed above, except for size and color, morphological variation is not. Embioptera remain as one of the least studied groups of primitively social insects; the avenue is open for interesting research opportunities and further exploration of factors underlying variation in group-living awaits.
The raw data supporting the conclusions of this article will be made available by the authors, without undue reservation.
The author confirms being the sole contributor of this work and has approved it for publication.
The Ph.D. thesis research on dispersion and dispersal behavior in the field was supported by the NSF Dissertation Improvement Grant (BSR-8312897), the National Academy of Science and Sigma Xi Scientific Research Society. Research on predation rates was supported by a Santa Clara University Presidential Research Grant (5-28062).
The author declares that the research was conducted in the absence of any commercial or financial relationships that could be construed as a potential conflict of interest.
All claims expressed in this article are solely those of the authors and do not necessarily represent those of their affiliated organizations, or those of the publisher, the editors and the reviewers. Any product that may be evaluated in this article, or claim that may be made by its manufacturer, is not guaranteed or endorsed by the publisher.
I wish to thank my Ph.D. advisor the late George Eickwort for his suggestion that I conduct research on the little-known order Embioptera. I am grateful for my undergraduate research assistants at Santa Clara University and colleagues from around the world for their many contributions (including techniques in a variety of fields beyond behavior and ecology, that allow us to uncover facts about silk and silk genes, spinning, morphology, genomes, and phylogeny). I thank Edward C. Rooks for helping with field work and photography and for producing the painting of the bird predators. I acknowledge the kindness of the Asa Wright Nature Centre management for allowing me to collect the predation data on their property. I grateful for the endowment for the Michael and Elizabeth Valeriote Chair for supporting my research program at SCU. Michael Taborsky and Glauco Machado provided thoughtful and very helpful comments on an earlier version of this manuscript; these were greatly appreciated.
Avilés, L. (1997). “Causes and consequences of cooperation and permanent-sociality in spiders,” in The Evolution of Social Behavior in Insects and Arachnids, eds J. C. Choe and B. J. Crespi (New York, NY: Cambridge Univ. Press), 476–498. doi: 10.1186/1471-2148-9-257
Bradoo, B. L. (1967). Observations on the life history of Oligotoma ceylonica ceylonica Enderlien (Oligotomidae, Embioptera), commensal in the nest of social spider, Stegodyphus sarasinorum Karsch. J. Bombay Nat. Hist. Soc. 64, 447–454.
Büsse, S., Büscher, T. H., Kelly, E. T., Heepe, L., Edgerly, J. S., and Gorb, S. N. (2019). Pressure-induced silk spinning mechanism in webspinners (Insecta: Embioptera). Soft Matter 15, 9742–9750. doi: 10.1039/c9sm01782h
Büsse, S., Hörnschemeyer, T., Hohu, K., McMillan, D., and Edgerly, J. S. (2015). The spinning apparatus of webspinners–functional-morphology, morphometrics and spinning behaviour. Sci. Rep. 5:9986. doi: 10.1038/srep09986
Choe, J. C. (1994). Communal nesting and subsociality in a webspinner, Anisembia texana (Insecta: Embiidina: Anisembiidae). Anim. Behav. 47, 971–973. doi: 10.1006/anbe.1994.1128
Choe, J. C., and Crespi, B. J. (1997). The Evolution of Social Behavior in Insects and Arachnids. New York, NY: Cambridge Univ. Press. doi: 10.1017/CBO9780511721953
Collin, M. A., Edgerly, J. S., and Hayashi, C. Y. (2011). Comparison of fibroin cDNAs from web spinning insects: insight into silk formation and function. Zoology 114, 239–246. doi: 10.1016/j.zool.2011.01.004
Collin, M. A., Garb, J. E., Edgerly, J. S., and Hayashi, C. Y. (2009). Characterization of silk spun by the embiopteran, Antipaluria urichi. Insect Biochem. Mol. Biol. 39, 75–82. doi: 10.1016/j.ibmb.2008.10.004
Cooperrider, A. Y., Boyd, R. J., and Stuart, H. R. (1986). Inventory and Monitoring of Wildlife Habitat. U.S. Depart. Interior, Bureau of Land Management. Service Center. Denver: U.S. Government Printing Office, 20402.
Corcobado, G., Rodriguez-Gironés, M. A., Moya-Laraño, J., and Avilés, L. (2012). Sociality level correlates with dispersal ability in spiders. Func. Ecol. 26, 794–803.1. doi: 10.1111/j.1365-2435.2012.01996.x
Costa, J. T. (2006). The Other Insect Societies. Cambridge, MA: Harvard Univ. Press. doi: 10.2307/j.ctv22jnr0f
Costa, J. T., and Pierce, N. E. (1997). “Social evolution in the Lepidoptera: ecological context and communication in larval societies,” in The Evolution of Social Behavior in Insects and Arachnids, eds J. C. Choe and B. J. Crespi (New York, NY: Cambridge Univ. Press), 407–442. doi: 10.1017/cbo9780511721953.021
Costa, J. T., and Ross, K. G. (1993). Seasonal decline in intracolony genetic relatedness in eastern tent caterpillars: implications for social evolution. Behav. Ecol. Sociobiol. 32, 47–54. doi: 10.1007/bf00172222
Costa, J. T., and Ross, K. G. (2003). Fitness effects of group merging in a social insect. Proc. R. Soc. Lond. B Biol. Sci. 270, 1697–1702. doi: 10.1098/rspb.2003.2422
Craig, C. L. (1997). Evolution of arthropod silks. Ann. Rev. Ent. 42, 231–267. doi: 10.1146/annurev.ento.42.1.231
Dejan, K. A., Fresquez, J. M., Meyer, A. M., and Edgerly, J. S. (2013). Maternal territoriality achieved through shaking and lunging: an investigation of patterns in associated behaviors and substrate vibrations in a colonial embiopteran, Antipaluria urichi. J. Insect Sci. 13:82. doi: 10.1673/031.013.8201
Edgerly, J. S. (1987a). Colony composition and some costs and benefits of facultatively communal behavior in a Trinidadian webspinner, Clothoda urichi (Embiidina: Clothodidae). Ann. Entomol. Soc. Amer. 80, 29–34. doi: 10.1093/aesa/80.1.29
Edgerly, J. S. (1987b). Maternal behaviour of a webspinner (Order Embiidina). Ecol. Ent. 12, 1–11. doi: 10.1111/j.1365-2311.1987.tb00979.x
Edgerly, J. S. (1987c). Behavioral Ecology of a Primitively Social Webspinner (Embiidina: Clothodidae: Clothoda urichi) (Maternal Care, Host-parasite Interactions, Communal Behavior, Scelionidae, Trinidad). Ithaca, NY: Cornell University. [Ph.D. Thesis].
Edgerly, J. S. (1988). Maternal behaviour of a webspinner (Order Embiidina): mother-nymph associations. Ecol. Ent. 13, 263–272. doi: 10.1111/j.1365-2311.1988.tb00356.x
Edgerly, J. S. (1994). Is group living an antipredator defense in a facultatively communal webspinner (Embiidina: Clothodidae)? J. Insect Behav. 7, 135–147. doi: 10.1007/bf01990076
Edgerly, J. S. (1997). “Life beneath silk walls: a review of the primitively social Embiidina,” in The Evolution of Social Behavior in Insects and Arachnids, eds J. C. Choe and B. J. Crespi (New York, NY: Cambridge Univ. Press), 14–25. doi: 10.1017/cbo9780511721953.003
Edgerly, J. S. (2018). “Biodiversity of Embiodea,” in Insect Biodiversity: Science and Society, eds R. G. Foottit and P. H. Adler (Hoboken: Wiley Online Library), doi: 10.1002/9781118945582.ch9
Edgerly, J. S., Davilla, J. A., and Schoenfeld, N. (2002). Silk spinning behavior and domicile construction in webspinners. J. Insect Behav. 15, 219–242. doi: 10.1023/A:1015437001089
Edgerly, J. S., and Rooks, E. C. (2004). Lichens, sun, and fire: a search for an embiid-environment connection in Australia (Order Embiidina: Australembiidae and Notoligotomidae). Envir. Ent. 33, 907–920. doi: 10.1603/0046-225x-33.4.907
Edgerly, J. S., Sandel, B., Regoli, I., and Okolo, O. (2020). Silk spinning behavior varies from species-specific to individualistic in embioptera: do environmental correlates account for this diversity? Insect Sys. Div. 4:2. doi: 10.1093/isd/ixaa007
Edgerly, J. S., Shenoy, S. M., and Werner, V. G. (2006). Relating the cost of spinning silk to the tendency to share it for three embiids with different lifestyles (Order Embiidina: Clothodidae, Notoligotomidae, and Australembiidae). Envir. Ent. 35, 448–457. doi: 10.1603/0046-225x-35.2.448
Edgerly, J. S., Tadimalla, A., and Dahlhoff, E. P. (2005). Adaptation to thermal stress in lichen-eating webspinners (Embioptera): habitat choice, domicile construction and the potential role of heat shock proteins. Func. Ecol. 19, 255–262. doi: 10.1111/j.1365-2435.2005.00957.x
Fitzgerald, T. D., and Costa, J. T. III (1986). Trail-based communication and foraging behavior of young colonies of forest tent caterpillars (Lepidoptera: Lasiocampidae). Ann. Entomol. Soc. Amer. 79, 999–1007. doi: 10.1093/aesa/79.6.999
Fitzgerald, T. D., and Peterson, S. C. (1983). Elective recruitment by the eastern tent caterpillar (Malacosoma americanum). Anim. Behav. 31, 417–423. doi: 10.1016/S0003-3472(83)80061-X
Harper, J. R., Sripada, N., Kher, P., Whittall, J. B., and Edgerly, J. S. (2021). Interpreting nature’s finest insect silks (Order Embioptera): hydropathy, interrupted repetitive motifs, and fiber-to-film transformation for two neotropical species. Zoology 146:125923. doi: 10.1016/j.zool.2021.125923
Henschel, J. R. (1998). Predation on social and solitary individuals of the spider Stegodyphus dumicola (Araneae, Eresidae). J. Arachnol. 26, 61–69.
Hoffman, C. R., and Avilés, L. (2017). Rain, predators, and spider sociality: a manipulative experiment. Behav. Ecol. 28, 589–596. doi: 10.1093/beheco/arx010
Jakob, E. M. (1991). Costs and benefits of group living for pholcid spiderlings: losing food, saving silk. Anim. Behav. 41, 711–722. doi: 10.1016/S0003-3472(05)80908-X
Kelly, E. T., Whittall, J. B., and Edgerly, J. S. (2018). Resolving two Haploembia (Embioptera: Oligotomidae) cryptic species: molecular data confirms parthenogenetic females can be distinguished by their antisocial behavior. Zootaxa 4504, 225–242. doi: 10.11646/zootaxa.4504.2.4
Koenig, W. D., and Dickinson, J. L. (2016). Cooperative Breeding in Vertebrates: Studies of Ecology, Evolution, and Behavior. Cambridge: Cambridge University Press. doi: 10.1017/CBO9781107338357
LeDoux, A. (1958). Biologie et comportement de l’Embioptere Monotylota ramburi Rims.-Kors. Ann. Sci. Nat. Zool. 20, 515–523.
Loeffler, C. C. (1996). Adaptive trade-offs of leaf folding in Dichomeris caterpillars on goldenrods. Ecol. Ent. 21, 34–40. doi: 10.1111/j.1365-2311.1996.tb00263.x
McMillan, D., Hohu, K., and Edgerly, J. S. (2016). Choreography of silk spinning by webspinners (Insecta: Embioptera) reflects lifestyle and hints at phylogeny. Biol. J. Linn. Soc. 118, 430–442. doi: 10.1111/bij.12749
Melander, A. L. (1903). Notes on the structure and development of Embia texana. Biol. Bull. Mar. Biol. Lab. Woods Hole. 4, 99–118. doi: 10.2307/1535766
Miller, K. B., Hayashi, C., Whiting, M. F., Svenson, G. J., and Edgerly, J. S. (2012). The phylogeny and classification of Embioptera (Insecta). Syst. Ent. 37, 550–570. doi: 10.1111/j.1365-3113.2012.00628.x
New, T. R. (1985). Communal oviposition and egg-brooding in a psocid, Peripsocus niterns (Insecta: Psocoptera) in Chile. J. Nat. Hist. 19, 419–423. doi: 10.1080/00222938500770301
Nuotclà, J. A., Diehl, J. M. C., and Taborsky, M. (2021). Habitat quality determines decisions and fitness in a beetle–fungus mutualism. Front. Ecol. Evol. 9:242. doi: 10.3389/fevo.2021.602672
Okada, S., Weisman, S., Trueman, H. E., Mudie, S. T., Haritos, V. S., and Sutherland, T. D. (2008). An Australian webspinner species makes the finest known insect silk fibers. Int. J. Biol. Macro 43, 271–275. doi: 10.1016/j.ijbiomac.2008.06.007
Osborn Popp, T. M., Addison, J. B., Jordan, J. S., Damle, V. G., Rykaczewski, K., Chang, S. L., et al. (2016). Surface and wetting properties of embiopteran (webspinner) nanofiber silk. Langmuir 32, 4681–4687. doi: 10.1021/acs.langmuir.6b00762
Peakall, D. B., and Witt, P. N. (1976). The energy budget of an orb web-building spider. Comp. Biochem. Physiol. A 54, 187–190. doi: 10.1016/S0300-9629(76)80094-1
Rehan, S. M., Richards, M. H., Adams, M., and Schwarz, M. P. (2014). The costs and benefits of sociality in a facultatively social bee. Anim. Behav. 97, 77–85. doi: 10.1016/j.anbehav.2014.08.021
Rita, C. (1993). Maternal behaviour in a web spinner Pseudembia flava Ross (Embioptera; Insecta). J. Bombay Nat. Hist. Soc. 90, 300–301.
Ross, E. S. (1970). Biosystematics of the Embioptera. Ann. Rev. Ent. 15, 157–172. doi: 10.1146/annurev.en.15.010170.001105
Ross, E. S. (2000a). Embia: contributions to the biosystematics of the insect order Embiidina. Part 1, Origin, relationships and integumental anatomy of the insect order Embiidina. Occas. Papers Calif. Acad. Sci. 149, 1–53.
Ross, E. S. (2000b). Embia: contributions to the biosystematics of the insect order Embiidina. Part 2. A review of the biology of Embiidina. Occas. Papers Calif. Acad. Sci. 149, 1–36.
Saito, Y. (1997). “Sociality and kin selection in Acari,” in The Evolution of Social Behavior in Insects and Arachnids, eds J. C. Choe and B. J. Crespi (New York, NY: Cambridge Univ. Press), 443–457. doi: 10.1017/CBO9780511721953.022
Seibt, U., and Wickler, W. (1990). The protective function of the compact silk nest of social Stegodyphus spiders (Araneae, Eresidae). Oecologia 82, 317–321. doi: 10.1007/BF00317477
Shenoy, S., Ing, K., Barber, R. P. Jr., Rooks, E. C., and Edgerly, J. S. (2020). A multiscale characterization of two tropical embiopteran species: nano-and microscale features of silk, silk-spinning behavior, and environmental correlates of their distributions. Envir. Ent. 49, 1242–1251. doi: 10.1093/ee/nvaa073
Stefani, R. (1953). La fisiologia dell’accoppiamento in Haploembia solieri Ramb. Rend. Accad. Naz. Lincei. 15, 211–216.
Stefani, R. (1956). Il problema della partenogenesi in Haploembia solieri Ramb. (Embioptera-Oligotomidae). Memorie Accad. Naz. Lincei. 8, 126–201.
Stokes, G. Y., DiCicco, E. N., Moore, T. J., Cheng, V. C., Wheeler, K. Y., Soghigian, J., et al. (2018). Structural and wetting properties of nature’s finest silks (order Embioptera). R. Soc. Open Sci. 5:9. doi: 10.1098/rsos.180893
Taborsky, M., Cant, M. A., and Komdeur, J. (2021). The Evolution of Social Behaviour. Cambridge, U.K: Cambridge Univ. Press. doi: 10.1017/9780511894794
Turner, G. F., and Pitcher, T. J. (1986). Attack abatement: a model for group protection by combined avoidance and dilution. Amer. Nat. 128, 228–240. doi: 10.1086/284556
Keywords: silk spinning, social behavior, predator-prey interactions, egg parasitism, habitat selection
Citation: Edgerly JS (2022) Dispersal Risks and Decisions Shape How Non-kin Groups Form in a Tropical Silk-Sharing Webspinner (Insecta: Embioptera). Front. Ecol. Evol. 10:727541. doi: 10.3389/fevo.2022.727541
Received: 18 June 2021; Accepted: 31 January 2022;
Published: 04 March 2022.
Edited by:
Floria M. K. Uy, University of Rochester, United StatesReviewed by:
Glauco Machado, University of São Paulo, BrazilCopyright © 2022 Edgerly. This is an open-access article distributed under the terms of the Creative Commons Attribution License (CC BY). The use, distribution or reproduction in other forums is permitted, provided the original author(s) and the copyright owner(s) are credited and that the original publication in this journal is cited, in accordance with accepted academic practice. No use, distribution or reproduction is permitted which does not comply with these terms.
*Correspondence: Janice S. Edgerly, amVkZ2VybHlyb29rc0BzY3UuZWR1
Disclaimer: All claims expressed in this article are solely those of the authors and do not necessarily represent those of their affiliated organizations, or those of the publisher, the editors and the reviewers. Any product that may be evaluated in this article or claim that may be made by its manufacturer is not guaranteed or endorsed by the publisher.
Research integrity at Frontiers
Learn more about the work of our research integrity team to safeguard the quality of each article we publish.