- 1Graduate School of Life Sciences, Tohoku University, Sendai, Japan
- 2Department of Experimental Limnology, Leibniz-Institute of Freshwater Ecology and Inland Fisheries, Stechlin, Germany
- 3Research Institute for Humanity and Nature, Kyoto, Japan
- 4Graduate School of Agricultural and Life Sciences, The University of Tokyo, Bunkyo, Japan
- 5Fisheries Resources Institute, Japan Fisheries Research and Education Agency, Yokohama, Japan
- 6Department of General Systems Studies, Graduate School of Arts and Sciences, The University of Tokyo, Meguro, Japan
Ecosystem-based disaster risk reduction (Eco-DRR) has attracted increased attention as a sustainable way to achieve both disaster risk reduction and biodiversity conservation, although there have been few quantitative evaluations of the potential impacts of Eco-DRR on biodiversity. Here, we examined the influences of flood hazard and land-use patterns on biodiversity by focusing on the species richness of plants, butterflies and odonates, and the abundance of two frog species in a rural landscape of Wakasa town, Fukui Prefecture, Japan. The direct effect of exposure to flood hazard on the studied taxa was not significant, whereas landscape factors associated with flood hazard significantly influenced either of the taxa in different magnitudes. We then exercised a scenario analysis by replacing urban land-use by non-urban, agricultural land-use (paddy fields in this case) to reduce exposure to flood hazard and projected the impacts on biodiversity. Our results demonstrated that the land-use replacement potentially reduces the risk of flooding by up to 5.19 billion yen (ca. 46 million US$) and, at the same time, positively influences the species richness and abundance, although the ecological impacts are different depending on taxon and spatial location. The land-use replacement was expected to result in the increase of plant richness and abundance of Daruma pond frog at a location by up to 16 and 25%, respectively. On the other hand, butterfly richness at a location was presumed to decrease by until −68%, probably due to their dependence on domestic gardens. The abundance of Japanese wrinkled frog did not show such a clear spatial variation. This study highlights the significance of land-use replacement as an Eco-DRR measure to reduce the disaster risk and conserve biodiversity in the agricultural landscape.
Introduction
Disasters caused by natural hazards have been increasing over the past decades because of urbanization, intensified land use, change of environmental quality and climate change (e.g., Peduzzi et al., 2009; Cui et al., 2019; Williams et al., 2019; Ward et al., 2020). Flooding causes one of the most lethal and economic damages and warrants special consideration (e.g., Degiorgis et al., 2012; Kazakis et al., 2015; Khajehei et al., 2020), so that flood risk management set to be a principal issue frequently in social and economic planning worldwide. Recently much attention has been paid to ecosystem-based disaster risk reduction (Eco-DRR) as an alternative countermeasure for naturel disasters. Disaster risk is usually expressed as the interaction between hazard, exposure and vulnerability (UNISDR, 2009). Eco-DRR utilizes various functions of ecosystems for reducing the risk of disaster events by lowering either or combination of hazard, exposure and vulnerability, as well as for sustainably managing and protecting natural resources provided by ecosystems and biodiversity (Cohen-Shacham et al., 2016; Renaud et al., 2016; Sebesvari et al., 2019). Eco-DRR is defined as “the sustainable management, conservation and restoration of ecosystems to reduce disaster risk, with the aim to achieve sustainable and resilient development” (Estrella and Saalismaa, 2013). Evaluation of the effectiveness of Eco-DRR in various aspects becomes important more and more these days as the demands and attempts of Eco-DRR have been increasing, although there still exist research gaps in various aspects of Eco-DRR in different regions of the world (Sudmeier-Rieux et al., 2021).
The effectiveness of Eco-DRR has been evaluated in previous studies from the points of biophysical, economic, and water quantitative and qualitative impacts, and the advantages of Eco-DRR over other measures have been shown in some cases of previous studies. For example, the Low Impact Development that is an approach to control storm water through the creation of a landscape fostering a natural hydrologic regime has been shown to reduce runoff of surface water by up to 70% and pollutant concentrations by up to >95% (Dietz, 2007; Dietz and Clausen, 2008). Also, floodplain preservation can generate economic benefits to avoid flood damages to property, counting 2.6 million US$ in the case of the East River Watershed, Wisconsin, United States (Kousky et al., 2013) and 7.7 million US$ in St. Louis County, Missouri, United States (Kousky and Walls, 2014). In addition to these advantages, Eco-DRR is expected to give positive effects on ecosystems and biodiversity (Cohen-Shacham et al., 2016; Renaud et al., 2016). Conventional hard-engineering measures of disaster risk reduction could impact ecosystems and biodiversity negatively, but this negative impact can be improved if a win-win relationship between biodiversity and human well-being such as disaster risk reduction is achieved (e.g., Kasada et al., 2017). In fact, coexistence of restoring nature and flood risk control can be achieved and contribute to sustainable countermeasures against flood (Mah, 2011). Areas exposed to higher flooding hazard are considered to experience more frequent flooding, and such areas often used to be wetlands (e.g., riverine floodplains) that frequently harbor high biodiversity (Gibbs, 2000; Tockner and Stanford, 2002). Thus, Eco-DRR that promotes the use of wetlands and floodplain has the potential to contribute to biodiversity conservation. Furthermore, various ecosystem services including provisioning, regulating and cultural ones are also expected to be provided by Eco-DRR (e.g., Cohen-Shacham et al., 2016; Huang et al., 2021).
In spite of the potential contribution of Eco-DRR to biodiversity conservation, there have been limited quantitative evaluations of the impacts of Eco-DRR on biodiversity (IPBES, 2019; Seddon et al., 2020). This may be due to the complex nature of Eco-DRR in how ecosystem-based approaches to reduce risk components (hazard, exposure and vulnerability) can benefit biodiversity conservation. For example, in the case of riverine flood, conserving natural floodplain habitats lowers the exposure of human lives and properties to flood and contributes to biodiversity conservation, whereas flood hazard, which can be reduced by storing storm water in wetlands, also influences biodiversity as it is associated with disturbances that have significant impacts on ecological communities (e.g., Townsent et al., 1997; Pollock et al., 1998). Previous studies have mainly focused on the aspect of habitat conservation or creation of Eco-DRR and revealed the positive impacts on biodiversity (e.g., Liquete et al., 2016; Renaud et al., 2016; Nakamura et al., 2020), although the impacts of Eco-DRR on biodiversity would include other aspects such as the direct effects of hazard on populations and communities of organisms (i.e., disturbance as an ecological process) and the landscape factors that are relevant to animal behavior or meta-population/community, which would be dependent on the exposure to hazard. Then, we hypothesized that the Eco-DRR approach provides overall positive effects on local biodiversity while reducing the potential flood risk, although the effects on local biodiversity would be different depending on species life history and spatial variations in other environmental conditions. This study focused on the direct effects of flood hazard as disturbance and the landscape factors on biodiversity in order to contribute to the understanding of impacts of Eco-DRR on biodiversity.
In this study, we first examined how flood hazard and land-use patterns influence biodiversity independently and interactively in a Japanese rural landscape that is predominated by paddy fields. Rice paddy fields provide spawning and nursery grounds for diverse organisms and thus function as alternative habitats to natural wetlands (Fasola and Ruíz, 1996; Elphick, 2000; Kano et al., 2010; Sesser et al., 2018; Kasahara et al., 2020), although the function is subject to actual agricultural practices (Lawler, 2001; Machado and Maltchik, 2010; Katayama et al., 2011, 2015). We also examined biodiversity in semi-natural grasslands that are formed on the levees in between paddy fields and maintained by periodic mowing (Shinohara et al., 2019). Thus, our studied organisms included plant, butterfly, odonate and frog species that frequently occur in the rural landscape consisting of paddy fields and the associated semi-natural grasslands, although the response to flood hazard and land-use patterns would be different depending on the taxa.
Secondly, we conducted a scenario analysis based on the results of the abovementioned analysis. Scenario analysis can play a significant role in better informing local stakeholders and decision makers (IPBES, 2016), which is an important step to promote the implementation of Eco-DRR on the ground. In our scenario, replacement of urban land-use to agricultural land-use (paddy fields) is considered as a potential measure to reduce the exposure of properties to flood hazard and thus lower the potential flood damages as a measure of Eco-DRR (e.g., Nishihiro et al., 2020; Osawa et al., 2021). Although flood in paddy fields potentially causes loss of agricultural production and damages of agricultural infrastructure, those economic loss is considered to be much less compared to potential loss and damages in urban land-use (Ministry of Land, Infrastructure, Transport and Tourism, Japan [MLIT], 2020a), resulting in the significant reduction of flood risk by the replacement of urban land-use to paddy fields in flood hazard area. Also, land-use planning to reduce exposure to flood hazard is a part of the new national policy of flood risk reduction (Ministry of Land, Infrastructure, Transport and Tourism, Japan [MLIT], 2020b), so that our scenario analysis would contribute to the understanding of consequences of the policy in the studied area. We examined two types of scenarios that assumed population decline or constant population, which was represented as the conversion of urban land-use with agricultural one or the swapping of urban and agricultural land-use, respectively (see details in methods). Replacement of urban land-use to natural floodplain would be ideal in terms of biodiversity conservation, although it might be not realistic and feasible in the actual rural community. Thus, we consider replacement to agricultural land-use (paddy fields) as an alternative. We thus considered two kinds of scenarios and projected the impacts on biodiversity and the risk of flood hazard in the studied rural landscape, although plausibility of the scenarios is a matter of future research. The first scenario is replacement of urban land-use with flood hazard to agricultural land-use to reduce the exposure and damage from flood, called “conversion scenario.” The second one is replacement of urban land-use with flood hazard to agricultural land-use and, in turn, replacement of agricultural land-use without flood hazard to urban land-use. This results in swapping between urban land-use with flood hazard and agricultural one without hazard, called “swapping scenario.”
Materials and methods
Study area, flood hazard and land-use
We studied an area that used to be a floodplain and currently dominated by paddy fields in the southwest part of Fukui Prefecture, western Japan (8 × 10 km; 35°33′N, 135°54′E; Figure 1). We established 72 sampling sites for the biodiversity survey on the basin of the Wakasa-region (Figure 1A). These sites were chosen so that they distributed as uniformly as possible in the whole study area. The mean annual temperature was 15.1°C, and the mean annual precipitation was 2,229 mm (2000–2020), which were recorded in a nearby automated meteorological data acquisition point (35°36′N, 135°55′E) according to the Japan Meteorological Agency (2021). In this region, farmers plant rice in paddy fields once a year, and levees between paddy fields are managed as semi-natural grasslands that are maintained by periodic mowing, which serve as important habitats for plant and insect species (Shinohara et al., 2019).
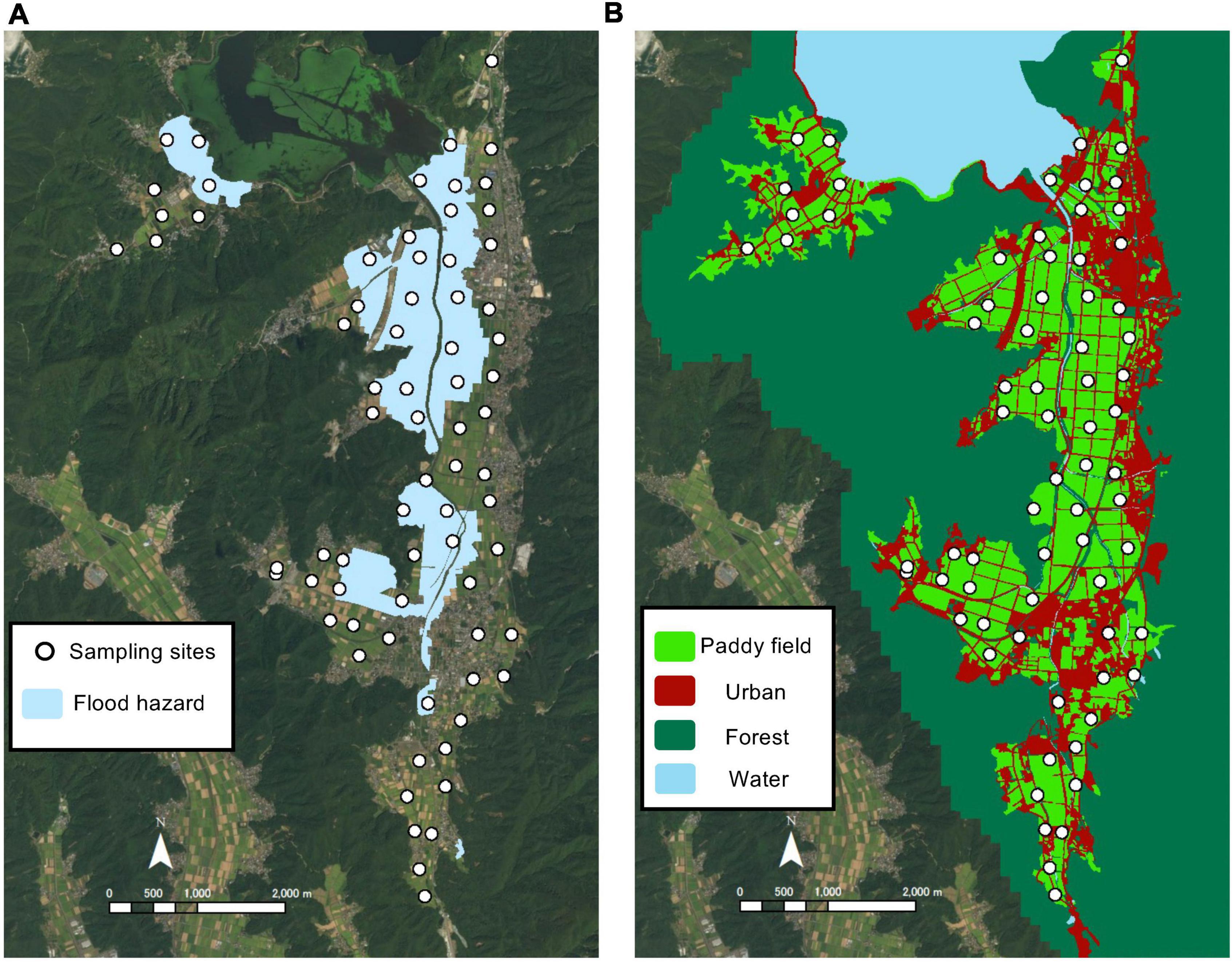
Figure 1. Study area in Wakasa town, Fukui Prefecture, Japan. Panel (A) shows the sampling sites (white circles) and flood hazard (light blue areas). Panel (B) shows the land-use in and around the study area.
We organized flood hazard in the study area (Figure 1A) by assembling actual flooded areas in 1980 to 2015 provided by the Fukui Prefectural government and the flood hazard map obtained from the National Land Numerical Information download service of the Japanese (Ministry of Land, Infrastructure, Transport and Tourism [MLIT], 2012).
The land-use of urban, agriculture (paddy fields), forests and others in the studied area were identified based on aerial photographs (map data: Google) with enough spatial resolution, and the land-use data (polygons) were digitized in the geographical information system (Arc-GIS, Esri).
Biodiversity surveys
Biodiversity in our study refers to richness or abundance of the taxa that frequently occur in the studied rural landscape consisting of paddy fields and the associated semi-natural grasslands, including plant, butterfly, odonate and frog species. We established a transect (2 m × 30 m) on the levees (semi-natural grasslands) of paddy fields at each sampling site. Surveys of the four taxa were conducted three times on each transect in September 2015, May 2016, and September 2016 when the studied organisms were active and relatively easily observed. For subsequent statistical analyses, we pooled all the data from three surveys. The methodologies of field surveys are as follows:
Plant survey
We established and surveyed three plots (each plot size is 0.5 m × 0.5 m), located at regular intervals (∼10m) along each transect. In total, there were 216 plots in 72 transects. We recorded all vascular plant species in each plot. For statistical analyses, we pooled the data from the three plots located in the same transect.
Butterfly, odonate and amphibian survey
Butterfly and odonate species were identified and the number of two amphibian species (Daruma pond frog (Pelophylax porosus) and Japanese wrinkled frog (Glandirana rugosa) were counted by a skilled surveyor at the same time with visual observation for 15 min. between 9 a.m. and 4 p.m. within each transect at a height of less than 3 m under warm, sunny conditions as in the previous studies (Pollard and Yates, 1993; Uchida and Ushimaru, 2014). This protocol was decided according to the preliminary survey and identification of species, and we could detect the organisms well enough to see the differences among transects (Figure 2). We paid careful attention to avoid double counting of individual organisms. We focused on the two dominant amphibian species as other amphibian species were observed only scarcely.
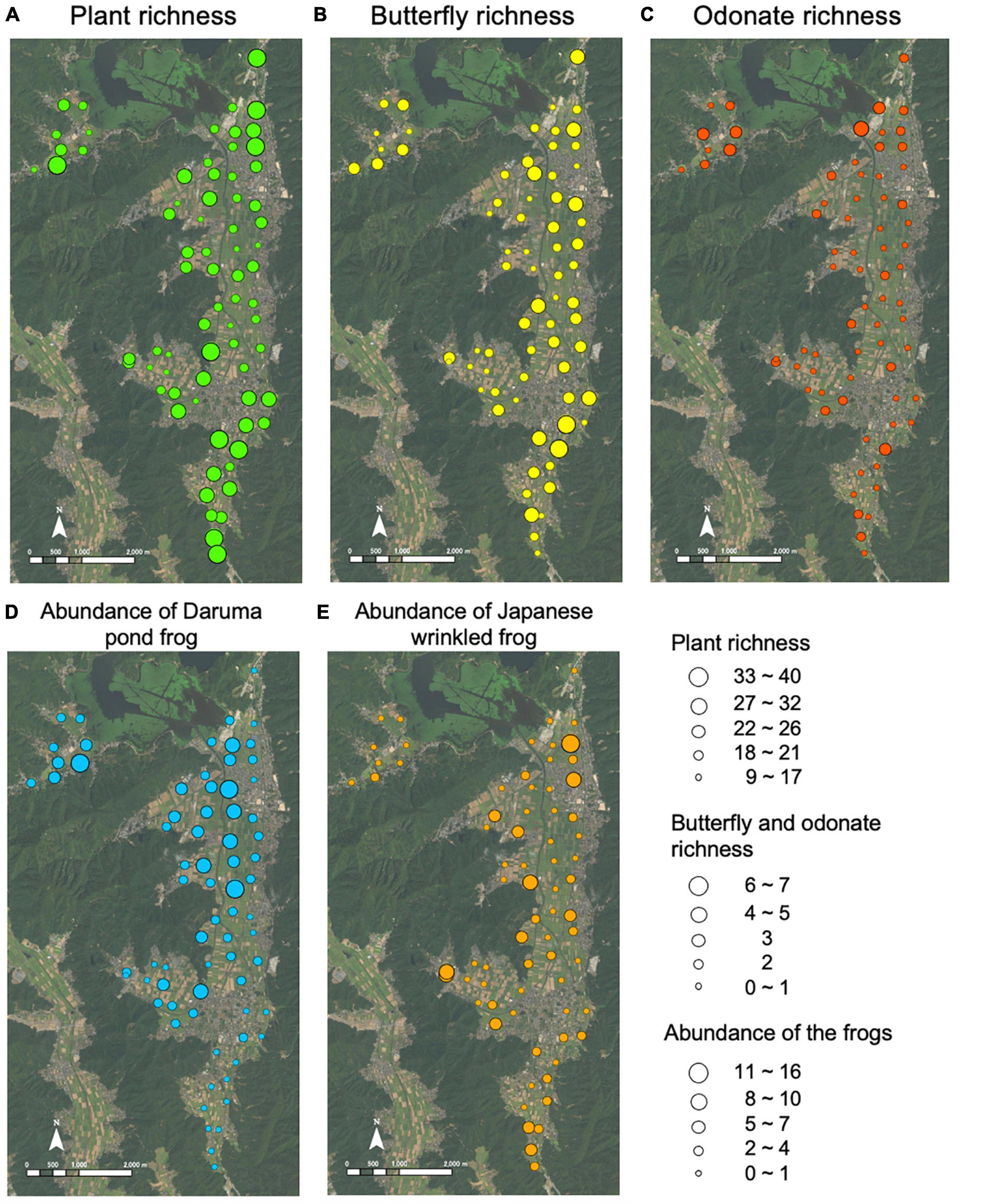
Figure 2. Distribution of species richness of plant (A), butterfly (B), and odonate (C), and abundance of Daruma pond frog (D) and Japanese wrinkled frog (E) in the study area of Wakasa town, Fukui Prefecture, Japan.
Analysis of the relationship among flood hazard, land use and biodiversity
To examine how flood hazard and land-use patterns influences various species inhabiting the study area, we constructed a generalized linear mixed model (GLMM) with a poison distribution. We used plant species richness, butterfly species richness, odonate species richness, and abundance of Daruma pond frog and Japanese wrinkled frog as response variables and created a model for each taxon. To analyze the relationships between biodiversity, flood hazard and landscape factors, we adopted whether or not a sampling site was in the flood hazard, and landscape factors in the area of a circle-shaped buffer created around each sampling site, as potential explanatory variables. The landscape factors consist of a combination of three types of land-use (urban, paddy fields and forests; Figure 1B) and whether or not the land-use is located in the flood hazard, counting six variables of landscape factors in total. Thus, each landscape variable was an area (m2) of a type of landscape, so that the coefficients of landscape factors in the same model can be compared to indicate the magnitude of the influence. We also evaluated a degree of farmland consolidation (three levels) at each sampling site and included it as a random effect in the model. Three levels of farmland consolidation were zero, one time, and two times of the consolidation that have been implemented before our study, whose information was provided by the Wakasa town.
Then, we constructed preliminary models with respect to each of different buffer sizes whose radiuses range from 100 m to 1,000 m with an interval of 100 m to identify the best buffer size for the landscape factors in the model. In this preliminary analysis, we constructed models using all explanatory variables with a different buffer size, and then selected a model with the lowest AIC (see Supplementary Figure 1) to identify the best buffer size for each taxon. After that, we constructed a GLMM for each taxon using the identified best buffer size for the landscape factors. The explanatory variables are six landscape factors (urban, paddy fields and forests × with or without flood hazard) and whether or not the sampling site is in the flood hazard. In total, we constructed 128 models using these explanatory variables with different combinations for each taxon. Models with ΔAIC < 2 were averaged for each taxon using “full-model averaging” as an averaging method according to Lukacs et al. (2010) and Symonds and Moussalli (2011).
In addition, we checked if the residuals of GLMMs showed spatial autocorrelation and found that strong spatial correlation was not evident overall for the taxa examined (Supplementary Figure 2).
Scenario analysis
As a measure of Eco-DRR, replacement of urban land-use to agricultural land-use (paddy fields) was considered in our scenario, as it can reduce the exposure of properties to flood hazard and thus lower the potential flood damages, as well as influencing biodiversity due to the change in land-use patterns. We conducted a scenario analysis based on the results of the GLMM models for each taxon that allowed us to project the change of richness or abundance of the taxa we studied. Due to our sampling and statistical designs, we projected only the local change of richness or abundance, but not the regional diversity or the population size covering the whole study area. In this study, we considered two scenarios. The conversion scenario is gradually replacing urban land-use with flood hazard to agricultural land-use to reduce the exposure and damage from flood. In this case, as some residences are converted, it would not be feasible when the human population is increasing. However, like most municipalities in Japan, this town has been experiencing population decline for the last three decades and the trend is projected to continue in the future (Wakasa town, 2015). In the swapping scenario, which would be more feasible even if the human population is not in the decreasing trend, we gradually swapped urban land-use with flood hazard and agricultural land-use without hazard.
In each scenario, we replaced the land-use in a computer simulation described below and projected the richness or abundance of different taxa and the reduction of potential flood damage as an economic benefit. The simulation procedures of the conversion scenario are as follows:
(1). Choose a polygon of urban land-use with flood hazard randomly and then replace an area of d m2 in the polygon with the same area of agricultural land-use (paddy fields). If the polygon of urban land-use with flood hazard is smaller than d m2, replace all the urban land-use of the polygon with agricultural land-use.
(2). Repeat the procedure 1 until the total replaced area reaches a targeted replacement rate ranged from 10 to 100%.
(3). Project the richness or abundance of each taxon using the newly gained land-use map and the specific GLMM model.
Here, we set the parameter d to 100 m2. We tested other parameter values (10, 50, 300 m2), and confirmed that the results were not different significantly. For the swapping scenario, in the procedure 1, we swapped urban land-use with flood hazard and agricultural land-use without hazard, both of which were randomly selected in the study area. The simulation repeated 100 times at each replacement rate that ranged from 10 to 100% of the total area of urban land-use exposed to flood hazard, by an increment of 10%.
We also calculated the difference in potential flood damage (monetary loss) between before and after the land-use change. The potential flood damage of urban and agriculture land-use was assumed if it was exposed to flood hazard, and the potential monetary loss was calculated based on the actual damages happened in 2013 in the studied municipality. The unit average of the actual damage per 1 m2 for each land-use was obtained from the data of the survey on flood damages collected by the Ministry of Land, Infrastructure, Transport and Tourism [MLIT] (2019). After the simulation of the land-use replacement, we calculated the expected total damage in the study area by multiplying the exposed area of urban and agricultural land-use to flood hazard and the unit average of the damages for respective land-use (8162 JPY m–2 for urban and 29 JPY m–2 for agricultural land-use). It should be noted that actual flood damage may be different from our assumption of potential damage depending on the magnitude of actual flood.
Results
In total, we found 242 plant species, 12 odonate species, 22 butterfly species, and 7 amphibian species in our sampling sites. We also observed the total of 253 and 163 individuals of Daruma pond frog and Japanese wrinkled frog, respectively. A list of all species observed in this study was given in Supplementary Table 1. The richness of plant, butterfly and odonate and abundance of the two frogs showed marked variations in distribution in the study area (Figure 2). The plant richness showed less variations among sampling sites, but still tended to be high at an edge of the forest, where up to forty species were found in the surveyed 0.75 m2 (Figure 2A, coefficient of variation (C.V) = 0.279). Among insects, the butterfly richness spread all over the study area with the maximum of 7 species observed during the survey (Figure 2B, C.V. = 0.575), while the odonate richness was relatively high around the lake (Lake Mikata) with the maximum of 4 species (Figure 2C, C.V. = 0.869). Daruma pond frogs (Pelophylax porosus) seemed to be abundant in the areas with flood hazard with the maximum of 16 individuals observed during the survey (Figure 2D, C.V. = 1.016), while Japanese wrinkled frogs (Glandirana rugosa) did not show such a trend and up to 11 individuals were found (Figure 2E, C.V. = 1.375).
The best buffer sizes of the models explaining the distributions were 700 m for plant richness, 500 m for butterfly richness, 300 m for Daruma pond frog abundance, and 400 m for Japanese wrinkled frog abundance (Supplementary Figure 1). For odonate richness, 1,000 m was the best buffer size in our analysis, although the AIC of the model might be lower with a larger buffer size (Supplementary Figure 1). However, if we use a buffer size larger than 1,000 m, overlapping among buffers becomes too large to lead a meaningful conclusion of the analysis.
The results of the GLMM models showed no significant effect of exposure to flood hazard (whether or not a sampling site was in flood hazard) and several significant effects of landscape factors in explaining the distribution of the studied taxa (Table 1). We found that there was a certain degree of plant richness independent of the environmental parameters we used because the intercept was relatively high (Table 1). Agricultural and urban land-use without flood hazard and forest with flood hazard positively affected the plant richness, although the coefficient was much larger for the forest with flood hazard (Table 1). For the butterfly richness, urban land-use with and without flood hazard had a positive effect with the much higher coefficient for that with flood hazard, whereas forest with flood hazard had a negative one (Table 1). Forest with flood hazard also negatively affected odonate richness, but effects of other landscape factors were not significant (Table 1). For Daruma pond frog, agriculture land-use affected Daruma pond frog positively irrespective of the association with flood hazard, and forest with hazard also showed a positive effect with a higher coefficient compared to those of agriculture land-use (Table 1). On the contrary, forest without flood hazard showed a positive effect on Japanese wrinkled frog in addition to urban land-use without hazard (Table 1). As odonate richness was not significantly influenced by the landscape factors associated with the scenario analysis (urban and agricultural land-use), our scenario of land-use replacement would not lead to a meaningful conclusion for this taxon, so that we excluded odonate richness from the subsequent scenario analysis.
Results of the scenario analyses showed no qualitative difference on the species richness or abundance between the conversion and swapping scenarios (Figure 3). For plant species richness and abundance of the two frogs, slightly positive effects of replacing land-use were detected (Figures 3A,C,D), although the slightly negative effect was shown for butterfly species richness (Figure 3B). For the potential flood damage, we found that the land-use replacement was attributed to reducing the potential damage by 5.19 billion yen (ca. 46 million US$) if we replace urban land-use by agricultural one for all areas with flood hazard (Figure 3E). Although the overall effects of land-use replacement on species richness and abundance were not remarkable, there were large variations in the effects depending on the location in the study area (Figures 4, 5). For the conversion scenario, the effects of land-use replacement were relatively large in the northern locations, especially in those with flood hazard, for plant and butterfly richness and abundance of Daruma pond frog (Figure 4), although abundance of Japanese wrinkled frog did not show such a spatial pattern. After the conversion, plant richness and Daruma pond frog abundance were expected to increase by up to 3% and 25%, respectively, although butterfly richness was expected to decrease by until −67%. The similar spatial patterns were observed for the swapping scenario (Figure 5), although for butterfly richness and abundance of Daruma pond frog, both of positive and negative effects were observed (Figures 5B,D), which was not observed in the conversion scenario. After the swapping, plant richness and Daruma pond frog abundance were expected to increase by up to 16 and 24%, respectively, although butterfly richness was expected to decrease by until −68%.
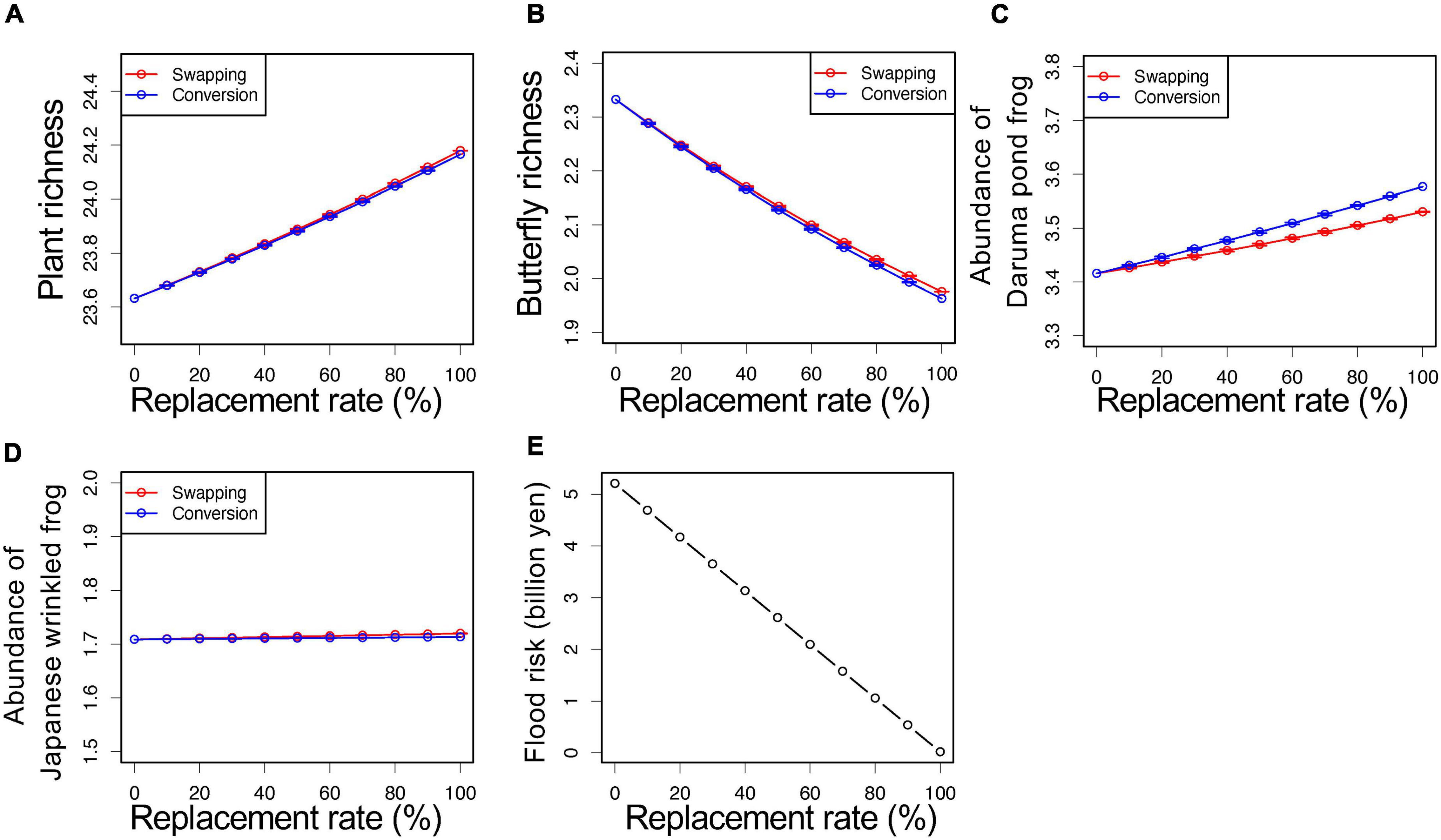
Figure 3. Results of the two scenario analyses of land-use replacement on species richness (plant (A) and butterfly (B)) and abundances of frogs (Daruma pond frog (C) and Japanese wrinkled frog (D)) and the potential flood damage (E). Red lines and blue lines represent the swapping and the conversion scenarios, respectively, with the mean and standard deviation for repeated simulations at each replacement rate. Standard deviation was generally too small to be visible in the figure. Note that the potential flood damage was not different between the two scenarios.
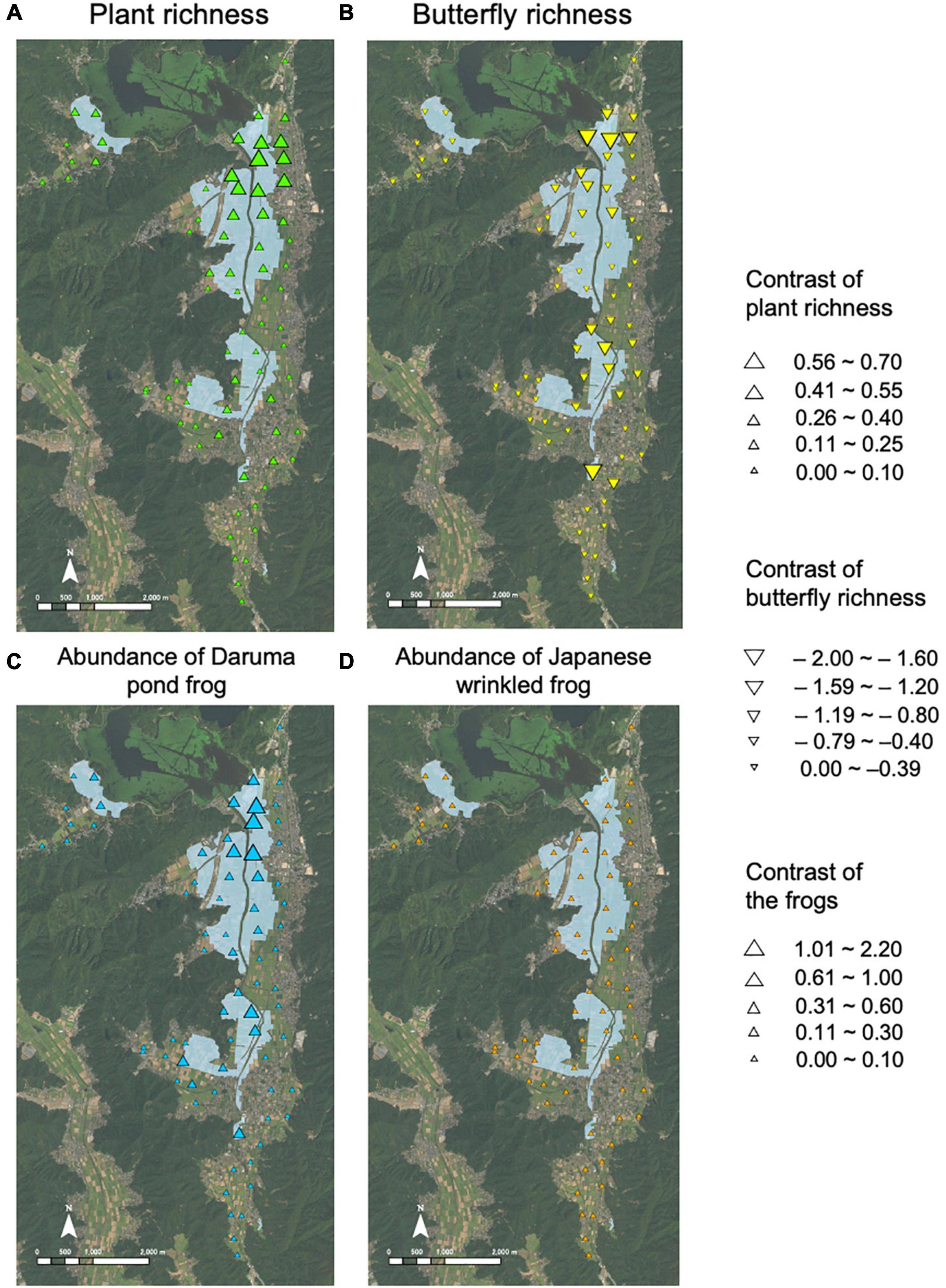
Figure 4. Contrast of plant richness (A), butterfly richness (B), and abundances of Daruma pond frog (C) and Japanese wrinkled frog (D) between before and after the land-use replacement in the conversion scenario. Light blue areas show flood hazard.
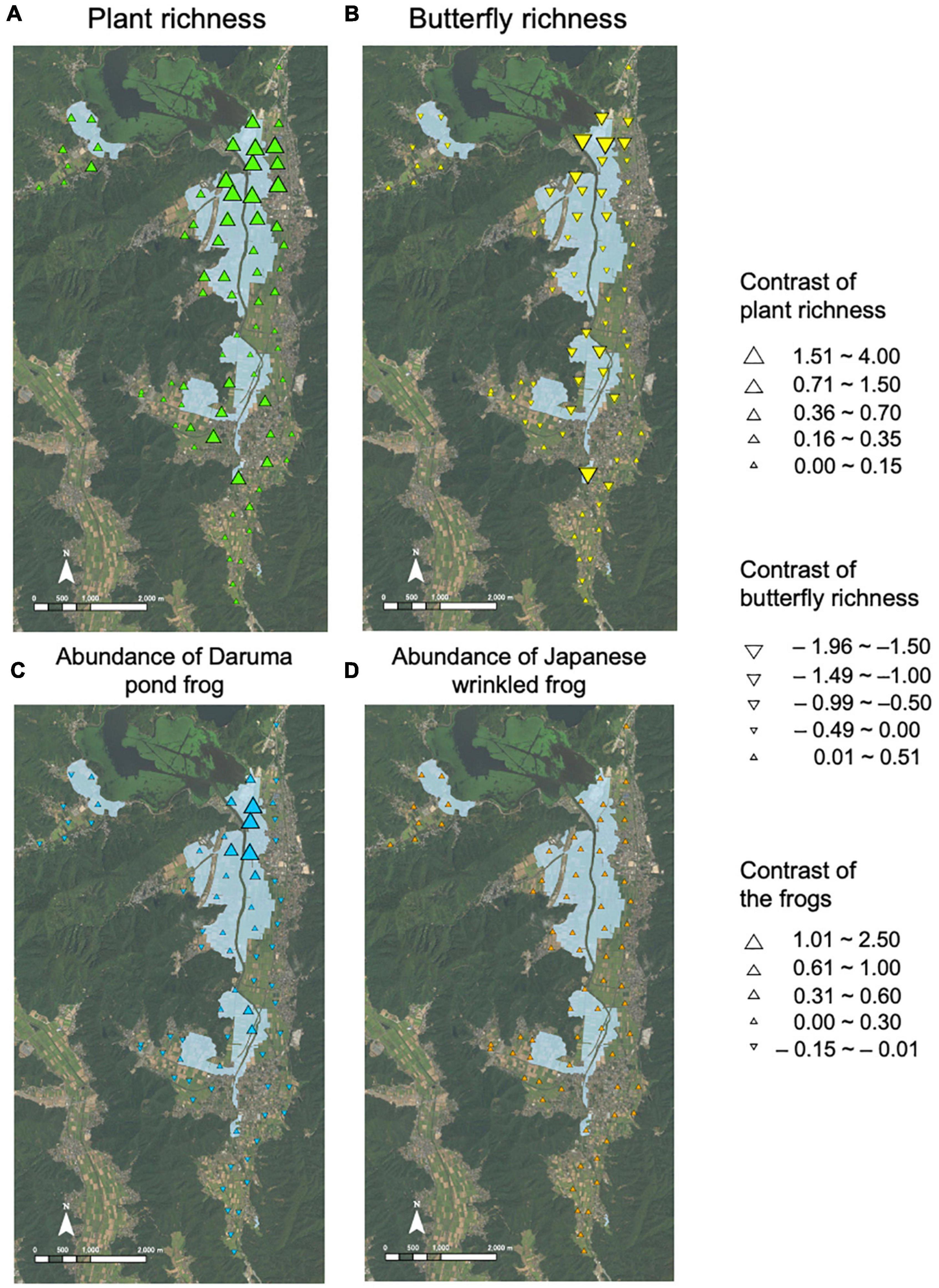
Figure 5. Contrast of plant richness (A), butterfly richness (B), and abundances of Daruma pond frog (C) and Japanese wrinkled frog (D) between before and after the land-use replacement in the swapping scenario. Light blue areas show flood hazard.
Discussion
Our results demonstrated that local biodiversity including species richness and abundance was shaped interactively by land-use patterns and flood hazard in the agricultural landscape dominated by paddy fields. This is in line with ecological literature that has repeatedly shown the importance of both disturbance and land-use in shaping ecological communities (e.g., Townsent et al., 1997; Pollock et al., 1998; Uchida and Ushimaru, 2014; Shinohara et al., 2019). Also, the results have significant implications for multiple functions of Eco-DRR as seen in our scenario analysis. The land-use replacement to reduce exposure of urban land-use to flood hazard affected positively different taxa we studied, as we hypothesized. In the east lakeside areas associated with flood hazard, the plant richness and Daruma pond frog abundance benefited from the land-use replacement than in the other areas (Figures 4, 5), indicating spatial heterogeneity of the effect. Thus, the lakeside areas have potentially high priorities, in which reduction of exposure of urban land-use to flood hazard as a measure of Eco-DRR is expected to result in both disaster risk reduction and biodiversity conservation. The plant richness was positively influenced by the land-use replacement (Figure 3A), because the plant richness was positively correlated with urban land-use without flood hazard and forests with flood hazard (Table 1). Also, creating paddy fields and associated semi-natural grasslands near forests would produce positive effects on plant richness on the grasslands, as in previous studies that showed the importance of a mosaic landscape for species such as birds (Amano et al., 2008) and spiders (Miyashita et al., 2012). As for the two frog species, the land-use replacement was expected to benefit Daruma pond frog more than Japanese wrinkled frog (Figures 3C,D). This should be due to the difference in habitat preference between the two species. Daruma pond frogs occurred around flood hazard areas (Figure 2D), whereas Japanese wrinkled frogs did not show such a trend (Figure 2E). The results suggest that species using habitats more related to flood hazard such as wetlands are more likely to benefit from the Eco-DRR measure of reducing exposure of urban land-use to flood hazard.
Although the land-use replacement showed the positive effects on some taxa, it negatively affected butterfly species. Most of the butterfly species observed in the study area were common species (Supplementary Table 1), and they might be dependent on artificial vegetation grown in domestic gardens. In an urban landscape, sunlight and floral abundance are important factors in determining the diversity of pollinator communities including butterflies (Matteson and Langellotto, 2010) and domestic gardens have an important role in supporting biodiversity (Cameron et al., 2012). In the study area of a rural landscape, there was no tall building, but domestic gardens still might have played an important role to support pollinator species.
Whether the sampling site itself was exposed to flood hazard was not important in shaping local biodiversity in our study (Table 1). This may be related to the fact that our study sites were paddy fields and associated semi-natural grasslands, and that paddy fields experience artificial floods (or irrigation) from spring to summer seasons for rice growing, which is similar timing to that of natural floods caused by the monsoon. Indeed, paddy fields and associated semi-natural grassland provide wet to moist environments, so that they can be an alternative habitat for organisms living in natural wetlands and floodplains (e.g., Kiritani, 2010; Natuhara, 2013). Thus, natural flood itself might have less influence on local biodiversity in the study sites, compared to the landscape factors we considered.
Our results also showed the potential financial benefits of 5.19 billion yen (Figure 3E) if we can replace all urban land-use in the flood hazard areas to agricultural one. This was probably an overestimation because we assumed that all houses in the flood hazard areas were lost and fully damaged if floods actually occurred. The assumption resulted in a linear decline of potential flood damage as the replacement rate increases (Figure 3E). In reality, the flood damage can be variable depending on the local situations including drainage distance, elevation, and flow accumulation (Kousky and Walls, 2014; Kazakis et al., 2015). Recently, technologies of mapping flood hazard have advanced to be able to show very details of flood hazard (e.g., Taki et al., 2013; Motevalli and Vafakhah, 2016; Razavi-Termeh et al., 2018). If we can combine the details of flood hazard obtained by the state-of-the-art techniques with our scenario analysis, we should be able to provide better information about, for example, high priority areas that would be more relevant to the land-use policy and management. Also, our analysis did not account for human damage that is also important for disaster risk reduction. Avoiding exposure to flood hazard by the land-use replacement is expected to reduce human damage as well, although this remains to be considered in the future research.
There was no remarkable distinction in the projected species richness and abundance between the conversion and swapping scenarios (Figure 3). As the difference between the two scenarios was the land-use outside the flood hazard areas, the results suggest that the land-use within the flood hazard areas was more important in shaping local biodiversity than that outside the flood hazard areas. The flood hazard areas were originally natural wetlands and floodplains before paddy fields were constructed in ancient times, and paddy fields provide alternative habitats to species closely associated with natural wetlands (Natuhara, 2013; Katayama et al., 2015). Thus, land-use change from urban to agriculture (paddy fields) within the flood hazard areas might have more influence on local biodiversity than the land-use change outside the flood hazard areas. However, in the conversion scenario, habitat area itself increases in addition to the landscape changes, and this should have large effects on local biodiversity as well, although our analysis did not allow us to examine such effects. In addition, implementing the swapping scenario in reality should be associated with more costs for constructing residence areas than implementing the conversion scenario without such a new construction, although the expected reduction of flood damage is the same between the two scenarios and the conversion scenario assumes the decline of human population. These socio-economic conditions in the local community were also not considered in our scenario analysis, although scenario analysis can be more flexible to include complexities and trade-offs inherent in decision making processes (Sayers et al., 2002; Casal-Campos et al., 2015).
Our scenario analysis considered the replacement from urban to agricultural land-use to reduce exposure to flood hazard. However, it may be more effective for biodiversity conservation to replace to wetlands instead of agriculture land-use. Wetlands not only support high productivity and biodiversity but also provide important ecosystem services including flood regulation (Gibbs, 2000; Woodward and Wui, 2001; Zedler and Kercher, 2005; Everard et al., 2009). Thus, in the context of Eco-DRR, replacing urban land-use to wetlands would be more effective. Nevertheless, the scenario analysis with wetlands was not possible due to the lack of the data sets for wetlands that were very scarce in the study area. It would be an effective approach to restore wetlands even in a small area and then obtain more information of such wetlands to construct an alternative scenario with the land-use replacement to wetlands.
Reconstructing wetlands can be an alternative effective approach that provides multiple ecosystem services (Everard et al., 2012).
We conducted the scenario analysis to quantify the effects on local biodiversity and flood damage reduction if the land-use replacement (either conversion or swapping) to reduce exposure of urban land-use to flood hazard is opted for an actual measure of Eco-DRR. The results suggest that the Eco-DRR approach can dramatically reduce the potential flood risk and provide overall positive effects on local biodiversity, although the effects were different depending on species and spatial location, as we hypothesized. The importance of agricultural land-use in biodiversity conservation would be relevant not only at a local scale, as shown in this study, but also at a broader scale, for example, in conserving an endangered migrating bird like the Oriental White Stork (Ciconia boyciana) that uses the paddy fields as their habitat (Yamada et al., 2019; Tawa and Sagawa, 2021). Thus, land-use planning at a local scale is critically important for both reducing flood risk and conserving local biodiversity, which the implementation of Eco-DRR aims for. Also, it is highly relevant to the global goals and initiatives such as the Sustainable Development Goals, the UN Decade on Ecosystem Restoration, the Sendai Framework for Disaster Risk Reduction, etc. The scenario analysis as we conducted here should provide useful information for quantifying and visualizing the potential outcomes of such land-use planning to support decision-making at a local scale.
Data availability statement
The raw data supporting the conclusions of this article will be made available by the authors, without undue reservation.
Ethics statement
Ethical review and approval was not required for the animal study because we conducted field observations of amphibians without collecting them.
Author contributions
MK, KU, NS, and TY contributed to conception and design of the study. KU and NS conducted field surveys. MK performed the scenario analysis. All authors wrote the manuscript and approved the submission.
Funding
This study was supported by the Environment Research and Technology Development Fund (4-1505) of Environmental Restoration and Conservation Agency, the Research Institute for Humanity and Nature (RIHN: a constituent member of NIHU) Project No. 14200103, and the Grant-in-Aid for JSPS Fellows (19J00864) (to MK).
Acknowledgments
We thank Satoru Itagawa, Yumi Yamada, Mifuyu Ogawa, Jun Ishii, Takuya Yoshida, Masaki Hirano, Keidai Iiyama, and Chinatsu Zakouji for their assistance. We also thank the reviewers and TM for their constructive comments and suggestions.
Conflict of interest
The authors declare that the research was conducted in the absence of any commercial or financial relationships that could be construed as a potential conflict of interest.
The handling editor TM declared a shared affiliation with the authors KU and TY at the time of the review.
Publisher’s note
All claims expressed in this article are solely those of the authors and do not necessarily represent those of their affiliated organizations, or those of the publisher, the editors and the reviewers. Any product that may be evaluated in this article, or claim that may be made by its manufacturer, is not guaranteed or endorsed by the publisher.
Supplementary material
The Supplementary Material for this article can be found online at: https://www.frontiersin.org/articles/10.3389/fevo.2022.699201/full#supplementary-material
References
Amano, T., Kusumoto, Y., Tokuoka, Y., Yamada, S., Kim, E., and Yamamoto, S. (2008). Spatial and temporal variations in the use of rice-paddy dominated landscapes by birds in Japan. Biol. Conserv. 141, 1704–1716. doi: 10.1016/j.biocon.2008.04.012
Cameron, R. W. F., Blanuša, T., Taylor, J. E., Salisbury, A., Halstead, A. J., Henricot, B., et al. (2012). The domestic garden–Its contribution to urban green infrastructure. Urban For. Urban Green. 11, 129–137. doi: 10.1016/j.ufug.2012.01.002
Casal-Campos, A., Fu, G., Butler, D., and Moore, A. (2015). An integrated environmental assessment of green and gray infrastructure strategies for robust decision making. Environ. Sci. Technol. 49, 8307–8314. doi: 10.1021/es506144f
Cohen-Shacham, E., Walters, G., Janzen, C., and Maginnis, S. (2016). Nature-based solutions to address global societal challenges. Gland: IUCN, doi: 10.2305/IUCN.CH.2016.13.en
Cui, Y., Cheng, D., Choi, C. E., Jin, W., Lei, Y., and Kargel, J. S. (2019). The cost of rapid and haphazard urbanization: Lessons learned from the Freetown landslide disaster. Landslides 16, 1167–1176.
Degiorgis, M., Gnecco, M., Gorni, S., Roth, G., Sanguineti, M., and Taramasso, A. C. (2012). Classifiers for the detection of flood-prone areas using remote sensed elevation data. J. Hydrol. 47, 302–315. doi: 10.1016/j.jhydrol.2012.09.006
Dietz, M. E. (2007). Low impact development practices: A review of current research and recommendations for future directions. Water Air Soil Pollut. 186, 351–363. doi: 10.1007/s11270-007-9484-z
Dietz, M. E., and Clausen, J. C. (2008). Stormwater runoff and export changes with development in a traditional and low impact subdivision. J. Environ. Manage. 87, 560–566. doi: 10.1016/j.jenvman.2007.03.026
Elphick, C. S. (2000). Functional equivalency between rice fields and seminatural wetland habitats. Conserv. Biol. 14, 181–191.
Estrella, M., and Saalismaa, N. (2013). “Ecosystem-based DRR: an overview,” in The role of ecosystem management in disaster risk reduction, eds F. Renaud, et al. (New York, NY: UNU Press), 30–31.
Everard, M., Bramley, M., Tatem, K., Appleby, T., and Watts, B. (2009). Flood management: From defence to sustainability. Environ. Liability 17, 35–49.
Everard, M., Harrington, R., and McInnes, R. J. (2012). Facilitating implementation of landscape-scale water management: The integrated constructed wetland concept. Ecosyst. Serv. 2, 27–37. doi: 10.1016/j.ecoser.2012.08.001
Fasola, M., and Ruíz, X. (1996). The value of rice fields as substitutes for natural wetlands for waterbirds in the Mediterranean region. Colon. Waterbirds 19, 122–128.
Gibbs, J. P. (2000). Wetland loss and biodiversity conservation. Conserv. Biol. 14, 314–317. doi: 10.1046/j.1523-1739.2000.98608.x
Huang, W., Hashimoto, S., Yoshida, T., Saito, O., and Taki, K. (2021). Nature-based approach to mitigate flood risk and improve ecosystem services in Shiga. Japan Ecosyst. Serv. 50:101309. doi: 10.1016/j.ecoser.2021.101309
IPBES. (2016). Summary for policymakers of the methodological assessment of scenarios and models of biodiversity and ecosystem services of the intergovernmental science-policy platform on biodiversity and ecosystem services, eds S. Ferrier, et al. (Bonn: IPBES secretariat).
IPBES. (2019). Summary for policymakers of the global assessment report on biodiversity and ecosystem services of the intergovernmental science policy platform on biodiversity and ecosystem services, eds S. Díaz, et al. (Bonn: IPBES secretariat).
Japan Meteorological Agency (2021). Meteorological records. Available onlin at: http://www.data.jma.go.jp/obd/stats/etrn/index.php (Accessed August 27, 2021)
Kano, Y., Kawaguchi, Y., Yamashita, T., and Shimatani, Y. (2010). Distribution of the oriental weatherloach, Misgurnus anguillicaudatus, in paddy fields and its implications for conservation in Sado Island, Japan. Ichthyol. Res. 57, 180–188. doi: 10.1007/s10228-009-0146-6
Kasada, M., Matsuba, M., and Miyashita, T. (2017). Human interest meets biodiversity hotspots: A new systematic approach for urban ecosystem conservation. PLoS One 12:e0172670. doi: 10.1371/journal.pone.0172670
Kasahara, S., Morimoto, G., Kitamura, W., Imanishi, S., and Azuma, N. (2020). Rice fields along the East Asian-Australasian flyway are important habitats for an inland wader’s migration. Sci. Rep. 10:4118.
Katayama, N., Baba, Y. G., Kusumoto, Y., and Tanaka, K. (2015). A review of post-war changes in rice farming and biodiversity in Japan. Agricul. Syst. 132, 73–84. doi: 10.1016/j.agsy.2014.09.001
Katayama, N., Saitoh, D., Amano, T., and Miyashita, T. (2011). Effects of modern drainage systems on the spatial distribution of loach in rice ecosystems. Aquat. Conserv. Marine Freshwater Ecosyst. 21, 156–164.
Kazakis, N., Kougias, I., and Patsialis, T. (2015). Assessment of flood hazard areas at a regional scale using an index-based approach and analytical hierarchy process: Application in rhodope–evros region, Greece. Sci. Total Environ. 538, 555–563. doi: 10.1016/j.scitotenv.2015.08.055
Khajehei, S., Ahmadalipour, A., Shao, W., and Moradkhani, H. (2020). A place-based assessment of flash flood hazard and vulnerability in the contiguous United States. Sci. Rep. 10:448.
Kiritani, K. (2010). A comprehensive list of organisms associated with paddy ecosystems in Japan. Itoshima: Institute of Agriculture and Natural Environment & Machida.
Kousky, C., and Walls, M. A. (2014). Floodplain conservation as a flood mitigation strategy: Examining costs and benefits. Ecol. Econ. 104, 119–128. doi: 10.1016/j.ecolecon.2014.05.001
Kousky, C., Olmstead, S. M., Walls, M. A., and Macauley, M. (2013). Strategically placing green infrastructure: Cost-effective land conservation in the floodplain. Environ. Sci. Technol. 47, 3563–3570. doi: 10.1021/es303938c
Liquete, C., Udias, A., Conte, G., Grizzetti, B., and Masi, F. (2016). Integrated valuation of a nature-based solution for water pollution control. Highlighting hidden benefits. Ecosyst. Serv. 22, 392–401.
Lukacs, P. M., Burnham, K. P., and Anderson, D. R. (2010). Model selection bias and Freedman’s paradox. Ann. Inst. Stat. Math. 62:117. doi: 10.1007/s10463-009-0234-4
Machado, I. F., and Maltchik, L. (2010). Can management practices in rice fields contribute to amphibian conservation in southern Brazilian wetlands? Aquat. Conserv. Marine Freshwater Ecosyst. 20, 39–46.
Mah, D. Y. S. (2011). Restoring nature in engineering structure: A modelling of Kuching bypass floodway. J. Coast. Conserv. 15, 565–571. doi: 10.1007/s11852-011-0145-4
Matteson, K. C., and Langellotto, G. A. (2010). Determinates of inner city butterfly and bee species richness. Urban Ecosyst. 13, 333–347. doi: 10.1007/s11252-010-0122-y
Ministry of Land, Infrastructure, Transport and Tourism [MLIT] (2012). Digital national land information. Available online at: https://nlftp.mlit.go.jp/index.html (Accessed August 27, 2021).
Ministry of Land, Infrastructure, Transport and Tourism [MLIT] (2019). Statistics of floods damage in 2013. Available online at: https://www.mlit.go.jp/river/toukei_chousa/kasen/suigaitoukei/index.html (Accessed August 27, 2021).
Ministry of Land, Infrastructure, Transport and Tourism, Japan [MLIT] (2020a). Flood control economic research manual (draft). Available online : https://www.mlit.go.jp/river/basic_info/seisaku_hyouka/gaiyou/hyouka/r204/chisui.pdf (Accessed August 27, 2021).
Ministry of Land, Infrastructure, Transport and Tourism, Japan [MLIT] (2020b). River basin disaster resilience and sustainability by All. Available online at: https://www.mlit.go.jp/river/kokusai/pdf/pdf21.pdf (Accessed August 27, 2021).
Miyashita, T., Chishiki, Y., and Takagi, S. R. (2012). Landscape heterogeneity at multiple spatial scales enhances spider species richness in an agricultural landscape. Popul. Ecol. 54, 573–581. doi: 10.1007/s10144-012-0329-2
Motevalli, A., and Vafakhah, M. (2016). Flood hazard mapping using synthesis hydraulic and geomorphic properties at watershed scale. Stoch. Environ. Res. Risk Assess. 30, 1889–1900. doi: 10.1007/s00477-016-1305-8
Nakamura, F., Ishiyama, N., Yamanaka, S., Higa, M., Akasaka, T., Kobayashi, Y., et al. (2020). Adaptation to climate change and conservation of biodiversity using green infrastructure. River Res. Appl. 36, 921–933. doi: 10.1002/rra.3576
Natuhara, Y. (2013). Ecosystem services by paddy fields as substitutes of natural wetlands in Japan. Ecol. Eng. 56, 97–106. doi: 10.1016/jecoleng.2012.04.026
Nishihiro, J., Ohtsuki, K., Kohzu, A., Kato, H., Ogasawara, S., Satake, Y., et al. (2020). “Satoyama green-infrastructure”, a measure for climate change adaptation: Potential for multipurpose usage of abandoned paddy on small valley bottoms in the Lake Inbanuma watershed. Ecol. Civil Eng. 22, 175–185. doi: 10.3825/ece.22.175
Osawa, T., Nishida, T., and Oka, T. (2021). Potential of mitigating floodwater damage to residential areas using paddy fields in water storage zones. Int. J. Disaster Risk Reduct. 62:102410. doi: 10.1016/j.ijdrr.2021.102410
Peduzzi, P., Dao, H., Herold, C., and Mouton, F. (2009). Assessing global exposure and vulnerability towards natural hazards: The disaster risk index. Nat. Hazards Earth Syst. Sci. 9, 1149–1159. doi: 10.5194/nhess-9-1149-2009
Pollard, E., and Yates, T. J. (1993). Monitoring butterflies for ecology and conservation; The British butterfly monitoring scheme. New York, NY: Springer-Verlag.
Pollock, M. M., Naiman, R. J., and Hanley, T. A. (1998). Plant species richness in riparian wetlands-a test of biodiversity theory. Ecology 79, 94–105. doi: 10.1890/0012-96581998079[0094:PSRIRW]2.0.CO;2
Razavi-Termeh, S. V., Kornejady, A., Pourghasemi, H. R., and Keesstra, S. (2018). Flood susceptibility mapping using novel ensembles of adaptive neuro fuzzy inference system and metaheuristic algorithms. Sci. Total Environ. 615, 438–451. doi: 10.1016/j.scitotenv.2017.09.262
Renaud, F. G., Sudmeier-Rieux, K., Estrella, M., and Nehren, U. (2016). Ecosystem-based disaster risk reduction and adaptation in practice. Berlin: Springer.
Sayers, P. B., Hall, J. W., and Meadowcroft, I. C. (2002). Towards risk-based flood hazard management in the UK. Proc. Inst. Civil Eng. Civil Eng. 150, 36–42.
Sebesvari, Z., Woelki, J., Walz, Y., Sudmeier-Rieux, K., Sandholz, S., Tol, S., et al. (2019). Opportunities for monitoring green infrastructure and ecosystems in the Sendai framework monitor. Prog. Disaster Sci. 2:100021. doi: 10.1016/j.pdisas.2019.100021
Seddon, N., Chausson, A., Berry, P., Girardin, C. A. J., Smith, A., and Turner, B. (2020). Understanding the value and limits of nature-based solutions to climate change and other global challenges. Phil. Trans. R. Soc. B 375:20190120.
Sesser, K. A., Iglecia, M., Reiter, M. E., Strum, K. M., Hickey, C. M., Kelsey, R., et al. (2018). Waterbird response to variable-timing of drawdown in rice fields after winter-flooding. PLoS One 13:e0204800. doi: 10.1371/journal.pone.0204800
Shinohara, N., Uchida, K., and Yoshida, T. (2019). Contrasting effects of land-use changes on herbivory and pollination networks. Ecol. Evol. 9, 13585–13595. doi: 10.1002/ece3.5814
Sudmeier-Rieux, K., Arce-Mojica, T., Boehmer, H. J., Doswald, N., Emerton, L., Friess, D. A., et al. (2021). Scientific evidence for ecosystem-based disaster risk reduction. Nat. Sustain. 4, 803–810. doi: 10.1038/s41893-021-00732-4
Symonds, M. R. E., and Moussalli, A. (2011). A brief guide to model selection, multimodel inference and model averaging in behavioural ecology using Akaike’s information criterion. Behav. Ecol. Sociobiol. 65, 13–21. doi: 10.1007/s00265-010-1037-6
Taki, K., Matsuda, T., Ukai, E., Nishijima, T., and Egashira, S. (2013). Method for evaluating flood disaster reduction measures in alluvial plains. J. Flood Risk Manage. 6, 210–218. doi: 10.1111/j.1753-318X.2012.01172.x
Tawa, K., and Sagawa, S. (2021). Stable isotopic analysis of stuffed specimens revealed the feeding habits of oriental storks Ciconia boyciana in Japan before their extinction in the wild. J. Ornithol. 162, 193–206. doi: 10.1007/s10336-020-01806-4
Tockner, K., and Stanford, J. (2002). Riverine flood plains: Present state and future trends. Environ. Conserv. 29, 308–330. doi: 10.1017/S037689290200022X
Townsent, C. R., Scarsbrook, M. R., and Dolédec, S. (1997). The intermediate disturbance hypothesis, refugia, and biodiversity in streams. Limnol. Oceanogr. 42, 938–949. doi: 10.4319/lo.1997.42.5.0938
Uchida, K., and Ushimaru, A. (2014). Biodiversity declines due to abandonment and intensification of agricultural lands: Patterns and mechanisms. Ecol. Monogr. 84, 637–658. doi: 10.1890/13-2170.1
Wakasa town (2015). Population vision of the Wakasa town. Available online at: https://www.town.fukui-wakasa.lg.jp/file/page/1377/doc/2.pdf (Accessed August 27, 2021).
Ward, P. J., Blauhut, V., Bloemendaal, N., Daniell, J. E., de Ruiter, M. C., Duncan, M. J., et al. (2020). Natural hazard risk assessments at the global scale. Nat. Hazards Earth Syst. Sci. 20, 1069–1096.
Williams, D. S., Máñez Costa, M., Sutherland, C., Celliers, L., and Scheffran, J. (2019). Vulnerability of informal settlements in the context of rapid urbanization and climate change. Environ. Urban. 31, 157–176.
Woodward, R. T., and Wui, Y. (2001). The economic value of wetland services: A meta-analysis. Ecol. Econ. 37, 257–270. doi: 10.1016/S0921-8009(00)00276-7
Yamada, Y., Itagawa, S., Yoshida, T., Fukushima, M., Ishii, J., Nishigaki, M., et al. (2019). Predicting the distribution of released oriental white stork (Ciconia boyciana) in central Japan. Ecol. Res. 34, 277–285. doi: 10.1111/1440-1703.1063
Keywords: biodiversity conservation, disaster risk reduction, paddy fields, floodplain, scenario analysis, Eco-DRR
Citation: Kasada M, Uchida K, Shinohara N and Yoshida T (2022) Ecosystem-based disaster risk reduction can benefit biodiversity conservation in a Japanese agricultural landscape. Front. Ecol. Evol. 10:699201. doi: 10.3389/fevo.2022.699201
Received: 23 April 2021; Accepted: 22 August 2022;
Published: 07 September 2022.
Edited by:
Tadashi Miyashita, The University of Tokyo, JapanReviewed by:
Nisikawa Usio, Kanazawa University, JapanNaoki Katayama, National Agriculture and Food Research Organization (NARO), Japan
Copyright © 2022 Kasada, Uchida, Shinohara and Yoshida. This is an open-access article distributed under the terms of the Creative Commons Attribution License (CC BY). The use, distribution or reproduction in other forums is permitted, provided the original author(s) and the copyright owner(s) are credited and that the original publication in this journal is cited, in accordance with accepted academic practice. No use, distribution or reproduction is permitted which does not comply with these terms.
*Correspondence: Takehito Yoshida, ty@chikyu.ac.jp
†These authors have contributed equally to this work and share first authorship