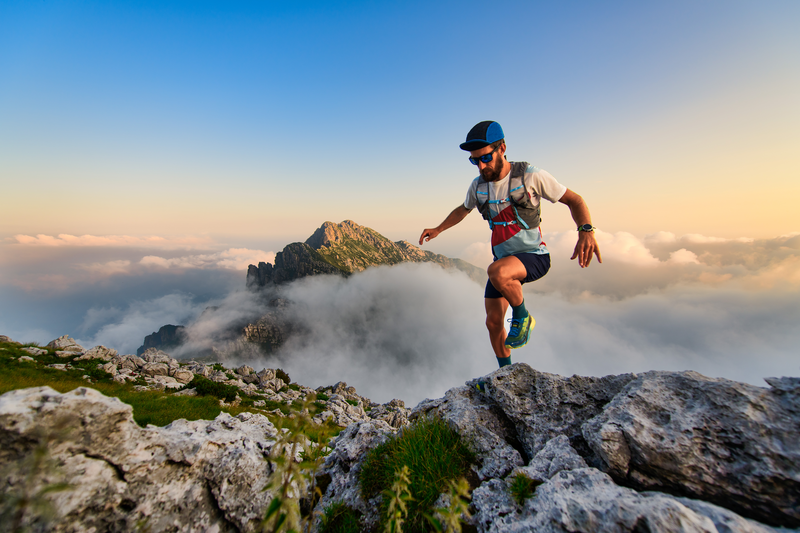
94% of researchers rate our articles as excellent or good
Learn more about the work of our research integrity team to safeguard the quality of each article we publish.
Find out more
ORIGINAL RESEARCH article
Front. Ecol. Evol. , 09 January 2023
Sec. Ecophysiology
Volume 10 - 2022 | https://doi.org/10.3389/fevo.2022.1105729
This article is part of the Research Topic Behavioral and Physiological Adaptations of Mammals and Birds to Anthropogenic Disturbances View all 11 articles
Grazing by large herbivores can potentially affect interspecific interactions between small herbivores by reducing the ecological fitness of animals. Desert rodents are important components in desert ecosystems and indicators of environmental change. Grazing reduces food resources, but rodents can decrease interspecific niche overlap by adaptive behavior. However, the key factors driving rodent behavioral activities and coexistence in the Alxa desert remains unstudied. We monitored population density and behavioral activities of Midday gerbil (Meriones meridianus) and northern three-toed jerboa (Dipus sagitta) in a grazing exclusion experiment in Alxa desert, Inner Mongolia, China, in 2017. We assessed the relationship between environmental factors (such as plant height, density, coverage, rainfall and temperature) and the behavioral activities of two coexisting rodent species. The results showed that: (1) In summer, grazing significantly reduced the activity time of gerbil and jerboa compared to that in grazing exclusion areas (gerbil: F = 5.98, p < 0.05, η2 = 0.22; jerboa: F = 8.57, p < 0.01, η2 = 0.28). Grazing reduced the temporal niche overlap with an obvious shifting of activity peaks between two species. (2) Grazing exclusion enhanced the temporal niche overlap between the two rodent species due to greater food availability which relieved inter-specific competition in each season. (3) Grazing strengthened the sensitivity of rodents to environmental changes in all seasons. These results indicated that grazing affected competition between the rodent species by altering vegetation conditions, which in turn affected the temporal niche and activity patterns of rodents.
Animal behaviour underlies many critical ecological functions, including nutrient cycling, primary productivity, pathogen transmission and habitat provision (Gribben et al., 2009; Barber and Dingemanse, 2010; Palkovacs and Dalton, 2015; Fernandes et al., 2020; Rahelinirina et al., 2021). While every species is inherently linked to ecosystem function, keystone species or ecosystem engineers are far more critical for overall ecosystem function than other species (Wilson et al., 2020). Desert rodents are important components in desert ecosystems and indicators of environmental change (Wu et al., 2016). Human-induced habitat fragmentation has a clear impact on rodents’ behavioral fitness (Goulson and Nicholls, 2022). As one of the most extensive land uses worldwide (Wang et al., 2019), grazing causes habitat loss and reduces wildlife (Loggins et al., 2019), which directly contributes to the reduction of biodiversity. In addition, the behavior of species and their interspecific relationships are altered through the influence of indirect and cascading effects (Azevedo et al., 2018). Grazing may change plant quality and food availability for small mammals, and subsequently may affect their population size, survival, body mass and reproduction (Li et al., 2016). The predation risk hypothesis states that trampling by livestock alters the height and coverage of vegetation, making it easier for predators to hunt, and that prey has greater ability to perceive predation risks in sparse, low vegetation (Pereira et al., 2012). Previous studies have shown that animal behavior changed only when human interference reached a certain threshold level. When animals believe that human activities are neither threatening nor beneficial, chronic or repeated interference can promote animal adaptability and tolerance (Smith et al., 2019).
Human activities, such as overgrazing, can reduce the ecological fitness of animals, and potentially affect interspecific interactions (Li et al., 2021). In this context, how to achieve regional coexistence through ecological niche allocation has become a hot issue in conservation biology and animal ecology in recent years (Bu et al., 2016). Temporal niche allocation for sympatric species is one of the main mechanisms supporting stable species coexistence (Sunarto et al., 2015). Animals can adjust their niche width and range through adaptation or behavioral changes to maximize benefits (Kronfeld-Schor and Dayan, 2003). Recently, a growing number of studies have suggested trade-offs in foraging ecology is a mechanism supporting coexistence (Ziv et al., 1993; Brown, 1996). Different activity patterns mean different evolutionary adaptations. Taxonomically close species possess similar activity patterns (Daan, 1981). Temporal partitioning may occur between distantly related species or even within various functional groups (Shuai et al., 2014). Both theoretical and empirical evidence support the hypothesis that interference competition stimulates temporal niche partitioning between sympatric species (Carothers and Jaksić, 1984; Ziv et al., 1993; Navarro-Castilla et al., 2017; Cepeda–Duque et al., 2021).
Direct human presence and indirect impacts on the surroundings of animals could alter their behaviour via changing population densities, top-down effects, bottom-up effects and effects on the physical environment (Wilson et al., 2020). Inter-specific competition of sympatric species affects their spatiotemporal activities (Odden et al., 2010; Ayala et al., 2021). In addition, many other biotic and abiotic factors may shift individual activity patterns. Such factors include predation risk (Yuan et al., 2017a; Loggins et al., 2019), intraspecific competition (Alanärä et al., 2001), food availability (Tang et al., 2020) and population size (Cui et al., 2015). However, the relative importance of multiple factors acting on rodent species coexistence and the drivers underlying the activity patterns of sympatric species remain unstudied.
Midday gerbil (Meriones meridianus) and northern three-toed jerboa (NTJ, Dipus sagitta) are typical nocturnal dominant rodent species, and coexist in the Alxa desert in China (Wu et al., 2016). They are omnivorous species with similar food habits (Yuan et al., 2018; Yang et al., 2020), and compete for food in the same habitats (Li et al., 2020). Midday gerbil is a quadrupedal forager with food-hoarding habits, while NTJ is a bipedal wanderer with larger home range, and does not store food (Zhao, 1964; Shuai et al., 2016; Song and Liu, 1984). The two species seem to compete, because of similar diets and nocturnal activity. However, they are sympatrically distributed in Alxa desert. These facts seem to violate the niche hypothesis. The climate in the desert is arid and food resources are extremely limited, so there is limited room for compromise in the nutritional niche between Midday gerbils and NTJ. However, the reasons for coexistence of the two species remain unknown. Is the coexistence of the two rodent species caused by rational nighttime allocation? Reasonable time allocation is of great significance to the survival and reproduction of animals (Ebensperger and Hurtado, 2005). Therefore, we hypothesized that: (1) There is strong competition between Midday gerbil and NTJ, and they coexist through temporal niche partitioning. (2) In grazing areas, lower food availability would prolong the activity time of rodents, and decreased vegetation coverage and the expansion of bare land would be beneficial to NTJ, while the vigilance behavior of Midday gerbils in grazing areas would increase. Meanwhile, the NTJ would reduce feeding behavior and increase vigilance in grazing exclusion areas because of dense vegetation. (3) Grazing exclusion would change the key pathways affecting temporal niche and behavioral patterns between sympatric Midday gerbil and NTJ.
This study was conducted in southern Alxa Desert, Inner Mongolia, China, (E104°10′–105°30′, N37°24′–38°25′). Our study area has a continental climate with cold and dry winters and warm summers. Annual precipitation ranges from 75 mm to 215 mm, about 70% of which falls from June to September. The soil is grey desert soil and grey brown soil. The vegetation is sparse, and the plants are mainly xerophytic, super xerophytic and halophytic shrubs. Midday gerbil, NTJ, desert hamsters (Phodopus roborovskii) and Mongolian five-toed jerboa (Orientallactaga sibirica) are dominant small-mammal species (Yang et al., 2020). Other natural enemies include Eurasian eagle owl (Bubo bubo), Marbled polecat (Vormela peregusna), and Corsac fox (Vulpes corsac; Yuan et al., 2018).
In the study area, we established an experimental area using a randomized block design with 3 blocks and 2 treatments (Yuan et al., 2018), grazing exclusion (3 repeated plots) and grazing (3 repeated plots). Each block covered 240 ha and each treatment unit was 60 ha. The distance between each replicate plot is 300–1,000 m. The grazing exclusion area began to be fenced in 1997, and in the grazing plots grazing began in 1995 with a grazing intensity of 1.03 sheep/ha (Yuan et al., 2017a). Before the exclusion areas were set up, the treatment blocks experienced the same grazing events. Grazed and grazing exclusion plots were enclosed by standard sheep fencing (110 cm high) that prevented livestock movement in or out of plots. Other small mammals and natural enemies were allowed to enter and leave the study area freely. We conducted rodent surveys, plant community surveys, and infrared camera monitoring in May, July, September, and October in 2017. According to the local climatic conditions, we defined May as spring, July as summer, September as autumn, and October as early winter.
A 7 × 8 trapping grid (1-ha) at a 15-m inter-trap distance was established at the center of each plot (60 ha). We placed one wire-mesh live trap (42 cm × 17 cm × 13 cm, Guixi Rodent Equipment Co. Ltd.) at each trap station. Traps were baited with fresh peanuts and checked twice (morning and afternoon) each day. We recorded the species name, sex, reproductive status, capture location, and weight (to the nearest 0.10 g) of the captured individuals. Each captured individual was marked with a passive integrated transponder (PIT; 2.12 mm × 8 mm, Guangzhou Ruimai Intelligent Technology Co. Ltd.) tag with a unique identification number (ID) injected under the pelage. In order to prevent rainfall and other sudden events, a wooden box (15 cm × 10 cm × 10 cm) was placed in the live trap to protect the rodents entering the cage (Yuan et al., 2018). The species and quantity of rodents captured every day in each plot were counted. We live-trapped rodents for four consecutive days in each season. The relative population of captured rodents was calculated according to the hundred cage capture rate (Wu et al., 2016).
We randomly selected three 100 m2 plots within each treatment unit to sample shrubs in each season. Within each 100 m2 plot, we randomly placed three 1 m2 quadrats to sample grasses and forbs. We measured the height, cover, density, abundance and biomass of shrubs and herbs (Yuan et al., 2018).
After the rodent survey, the live trap was retrieved and an infrared camera trap experiment was conducted in the same grid. Before camera trapping, five points were randomly selected in each grid. We placed fresh peanut bait at 18:00 daily, checked and weighed the remaining peanuts at 06:00 in the next morning. We then chose the point with the highest number of harvested peanuts, and set up one infrared camera (ERE-E1B, Shenzhen Ereagle Technology Co. Ltd) at the selected point in each grid. In total, there were three camera points in both the grazing exclusion and grazing areas. The camera was fixed at a distance of 30 ~ 40 cm from the ground, so that it could monitor the feeding, handling and vigilant behavior of rodents as much as possible. Camera monitoring continued from 18:00 to 06:00. Camera trapping was conducted for four consecutive days each month. To effectively observe the foraging behavior of rodents, peanuts (about 10 g) were selected as attractants. Other studies on mammals have shown that the use of attractants does not affect abundance and density estimates, distance moved or activity patterns (Preez et al., 2014; Braczkowski et al., 2016). We recorded the shooting time of each video, species, appearance time, departure time, rodent behavior, and duration time of each behavior.
We used the overlap package in R 4.1.2, which was developed specifically for the visualization and activity patterns analysis for camera trapping data. Activity patterns were performed using kernel density estimates which describe the probability of a camera-trap event occurring at any given time (Linkie and Ridout, 2011). We then ran the procedure developed by Ridout and Linkie (2009) to determine the overlap coefficient of activity patterns between different rodent species. Overlap was defined as the area under the curve formed by taking the minimum of the two kernel density estimates at each point in time (Ridout and Linkie, 2009). We used ∆4 for large samples (>75 camera records; Azevedo et al., 2018), and the ∆1 estimator was used when the number of events was less than 50, Confidence intervals were generated using the basic0 estimation for 10,000 bootstrapped samples (Meredith and Ridout, 2017).
A rodent ethogram during foraging was obtained from previous observations (Shang, 2005; Wang et al., 2015; Table 1). We calculated the ratio of a given behavior duration to the total activity time in 1 day, and the duration of the active period (Azlan and Sharma, 2006; Bach et al., 2017). All the data involved in this paper have been Shapiro - Wilk tested, in which activity pattern (p > 0.05, n = 12) and population density (p > 0.05, n = 12) all conform to the normal distribution. In addition, the activity duration have been normalized (log (n + 1)) prior to the analysis. Activity duration and patterns under different grazing treatments and seasonal difference was analyzed with the two-way repeated measures ANOVA using SPSS Statistics. SigmaPlot 12.0 was used for the plot.
We used random forest (RF) to evaluate the relationship of environmental factors with the duration of each behavior. RF analysis was performed to identify the major driving factors and to estimate the importance of the variables. We used percentage increases in mean squared error (MSE) of variables, where a higher MSE% value implied a more important variable. Analysis was conducted using the “rfPermute” package in R (Jiao et al., 2021). Calculation of the correlation coefficient and the visualization of the heat map are all realized through “link ET” (Huang, 2021).
A total of 6,629 videos were shot over the study. The total shooting time was 4,917 min, and the total duration of rodent activity was 1,448 min. Five rodent species, including Midday gerbil, NTJ, desert hamsters, Mongolian five-toed jerboa, and Alashan ground squirrel (Spermophilus alaschanicus) were captured by the cameras.
There were significant seasonal differences in the population(F(3,44) = 9.42, p < 0.01, η2 = 0.36). In the grazing exclusion plots, the population density of Midday gerbil decreased from spring to early winter (F(3,44) = 12.34, p < 0.001), and NTJ population in spring was significantly higher than in other seasons (F(3,44) = 20.64, p < 0.001). The population density of Midday gerbil was significantly higher than that of NTJ in summer (F(3,44) = 3.391, p < 0.05, Figure 1).
Figure 1. Seasonal changes in population densities of Midday gerbil, northern three-toed jerboa. NG represent the grazing exclusion area, RG represent the grazing area. Different capital letters (A and B) indicate that the population density of rodents varied significantly between seasons; different lowercase letters (a and b) indicate significant differences in population density of rodents in the same season.
In the grazing area, there was no significant difference in the population density of Midday gerbil in different seasons (F(3,44) = 0.44, p > 0.05), and the NTJ populations in spring and summer were significantly higher than in autumn and early winter (F(3,44) = 52.36, p < 0.001). The population density of Midday gerbil was significantly higher than that of NTJ in autumn (F(3,44) = 23.874, p < 0.001, Figure 1).
Grazing exclusion promoted higher NTJ population density than in the grazing treatment, both in spring (F(3,44) = 4.314, p < 0.05) and autumn (F(3,44) = 23.874, p < 0.001). Grazing exclusion induced higher population density of Midday gerbil than that in the grazing area in early winter (F(3,44) = 6.086, p < 0.01, Figure 1).
The activity time of the two species had a large overlap, and there were seasonal differences in the daily activity pattern. In spring, the niche overlap index of Midday gerbil with NTJ was higher than other seasons, both in the grazing exclusion and the grazing areas (Δgrazing exclusion = 0.8994, Δgrazing = 0.9549). The activity peak of the two species was concentrated at 20:00. Grazing promoted higher activity intensity of both species compared to the grazing exclusion area (Figures 2A,E). In summer, the niche overlap index of the two species was 0.8890 in the grazing exclusion area (Figure 2B). Grazing induced a clear staggered peak pattern in the daily activity rhythm pattern of the two species, and the overlap index of two species was 0.6329 (Figure 2F). In autumn, the niche overlap index of the two species was 0.8496 in the grazing exclusion area. There was an obvious staggered activity peak pattern in the grazing area (Δ = 0.7957, Figures 2C,G). In early winter, the overlap index of the two species was 0.7195 in the grazing exclusion area (Figure 2D). There was a staggered peak pattern in the grazing area and the niche overlap index of the two species was 0.8329 (Figure 2H).
Figure 2. Daily activity patterns of Midday gerbil and northern three-toed jerboa. The gray area shows the overlap between activities of the two species and the notches at the bottom of each image illustrate the density of camera-trap events. (A–D) represent the daily activity patterns of Midday gerbil and northern three-toed jerboa in the grazing exclusion area in May, July, September and October, respectively. (E–H) represent the daily activity patterns of Midday gerbil and northern three-toed jerboa in the grazing area in May, July, September and October, respectively. The number of independent camera-trap records (n), classification of daily activity patterns, estimates of coefficients of overlapping (Δ) of Midday gerbil with northern three-toed jerboa and 95% confidence intervals (CI) are given in each plate.
There were significant seasonal differences in the activity duration of Midday gerbil and NTJ (Midday gerbil: F(3,44) = 11.87, p < 0.001, η2 = 0.35; NTJ: F(3,44) = 12.26, p < 0.001, η2 = 0.358). In the grazing exclusion area, Midday gerbil had the longest activity duration in spring and autumn (F(3,44) = 2.94, p > 0.05, η2 = 0.31), while the NTJ activity duration reduced in early winter (F(3,44) = 7.39, p < 0.01, η2 = 0.53). In the grazing area, Midday gerbil and NTJ were active longer in spring and autumn than that in other seasons (Midday gerbil: F(3,44) = 6.55, p < 0.01, η2 = 0.49; NTJ: F(3,44) = 4.52, p < 0.05, η2 = 0.40). In summer, the Midday gerbil and NTJ activity duration was significantly longer in the grazing exclusion area than in the grazing area (Midday gerbil: F(1,22) = 5.98, p < 0.05, η2 = 0.22; NTJ: F(1,22) = 8.57, p < 0.01, η2 = 0.28; Figure 3).
Figure 3. Activity duration of Midday gerbil (A), northern three-toed jerboa (B). NG represent the grazing exclusion area, RG represent the grazing area. Different lowercase letters (a and b) indicate that the activity duration of rodents varied significantly between seasons. Asterisk denotes statistically significant differences, *p < 0.05, **p < 0.01.
In the grazing exclusion area, Midday gerbil showed significant foraging behavior in spring and summer (F(3,44) = 6.82, p < 0.01), storing behavior in autumn and early winter (F(3,44) = 10.48, p < 0.01), and most fighting behaviour in autumn (F(3,44) = 7.89, p < 0.01; Table 2). NTJ allocated significantly more time for foraging behavior in spring than that in early winter (F(3,44) = 5.60, p < 0.01), and showed notable vigilance in spring (F(3,44) = 5.20, p < 0.01). There were no significant seasonal differences in storing, feeding and fighting behavior of NTJ among seasons (Storing: F(3,44) = 0.86, p > 0.05; Feeding: F(3,44) = 0.51, p > 0.05; Fighting: F(3,44) = 2.14, p > 0.05; Table 3). In the grazing area, Midday gerbils showed significant foraging behavior and vigilance behaviour in spring and summer (Foraging: F(3,44) = 13.47, p < 0.01; Vigilance: F(3,44) = 3.89, p < 0.05), storing behavior in autumn (F(3,44) = 4.69, p < 0.01), and feeding behavior peaked in spring (F(3,44) = 9.64, p < 0.01; Table 2). In the grazing area, NTJ showed more vigilance behavior in spring (F(3,44) = 4.66, p < 0.01), and no seasonal differences in their other behaviors (Foraging: F(3,44) = 2.42, p > 0.05; Storing: F(3,44) = 1.15, p > 0.05; Feeding: F(3,44) = 0.76, p > 0.05; Vigilance: F(3,44) = 2.16, p > 0.05; Table 3).
In spring, the vigilance behavior of NTJ in the grazing exclusion area was significantly longer than that in the grazing area (F(1,22) = 4.95, p < 0.05). In summer, the storing behavior of NTJ in the grazing exclusion area was significantly longer than that in the grazing area(F(1,22) = 4.63, p < 0.05; Table 3). In autumn, the fighting behavior of Midday gerbil in the grazing exclusion area was significantly longer than that in the grazing area (F(1,22) = 5.99, p < 0.05; Table 2). In early winter, Midday gerbils spent more time on foraging, storing, vigilance and fighting behaviors in the grazing exclusion area than in the grazing area (Foraging: F(3,44) = 5.58, p < 0.05; Storing: F(3,44) = 9.42, p < 0.01; Vigilance: F(3,44) = 4.83, p < 0.05; Fighting: F(3,44) = 11.63, p < 0.01; Table 2).
Environmental humidity (Hum) and precipitation (Pre) reduced the activity overlap of the two dominant rodent species in the desert area (Grazing: RHum = −0.38, p < 0.05; RPre = −0.58, p < 0.001; Grazing exclusion: RHum = −0.67, p < 0.001; RPre = −0.55, p < 0.001; Figure 4). In the grazing exclusion area, environmental factors had non-significant influences on the daily activity rhythm overlap index (Overlap), vigilance behavior of Midday gerbil (M.Vig), and the foraging behavior (D.For), vigilance behavior (D.Vig) and activity duration (D.Dur) of NTJ. In the grazing exclusion area, the key factor affecting animal behaviors was NTJ population density (ROverlap = 0.95, p < 0.001; RM.Vig = 0.51, p < 0.001; RD.For = 0.40, p < 0.01; RD.Vig = 0.46, p < 0.001; RD.Dur = 0.44, p < 0.01; Figure 4A). In the grazing area, environmental factors had non-significant influences on the daily activity rhythm overlap index (Overlap), the behaviors of Midday gerbils (M.For, M.Sto, M.Fee, M.Vig, M.Fig, M.Dur), and the foraging and storing behaviors of NTJ (D.For, D.Sto). Key factors affecting the activity overlap of species were shrub density and coverage. The key factor affecting the behaviors of Midday gerbils was their population size (RM.For = 0.48, p < 0.001; RM.Sto = 0.54, p < 0.001; RM.Fee = 0.52, p < 0.0 1; RM.Vig = 0.43, p < 0.01; RM.Fig = 0.43, p < 0.01; RM.Dur = 0.48, p < 0.01). The population density of Mongolian five-toed jerboa was the key factor affecting vigilance behavior of NTJ (RD.Vig = 0.31, p < 0.05). Grass density was a key factor affecting the storing behavior of NTJ (RD.Vig = 0.48, p < 0.001). Grass height was a key factor affecting the vigilance behavior and activity duration of NTJ (RD.Dur = 0.34, p < 0.05). Rodent activities in grazing areas are affected by more biotic and abiotic factors than activities in grazing exclusion areas (Figure 4B).
Figure 4. Relationship of Midday gerbil and NTJ activities with environmental factors in grazing exclusion areas (A)and Grazing areas (B). The horizontal axis in the figure represents the predictor variable and the vertical axis represents the response variable. The size of the circle represents the importance of the variable. The larger the circle, the stronger the predictive effect of the predictor on the response variable. Different colors represent Spearman correlations. Red represents a negative correlation, blue represents a positive correlation, and the depth of the color represents the size of the correlation. The deeper the color, the stronger the correlation. The bar chart describes the total explanatory value of the predictor variable to the response variable. The variables are: degree of activity overlap of Midday gerbil and north three-toed jerboa (Overlap); Feeding behavior duration of Midday gerbil (M.Fee); Foraging behavior duration of Midday gerbil (M.For); Storage behavior duration of Midday gerbil (M.Sto); Vigilance behavior duration of Midday gerbil (M.Vig); Fighting behavior duration of Midday gerbil (M.Fig); Feeding behavior duration of northern three-toad jerboa (D.Fee); Foraging behavior duration of northern three-tord jerboa (D.For); Feeding behavior duration of northern three-tord jerboa (D.Sto); Vigilance behavior duration of northern three-tord jerboa (D.Vig); Fighting behavior duration of northern three-toed jerboa (D.Fig); Spe, Number of captured rodent species; Population density of Midday gerbil (M.Pop); Population density of northern three-toed jerboa (D.Pop); Population density of Mongolian five-toed jerboa (O.Pop); Population density of desert hamsters (P.Pop); Population density of Alashan ground squirrel (S.Pop); Average height of shrubs (S.Hig); Average shrub density (S.Den); Shrub biomass (S.Bio); Shrub coverage (S.Cov); Number of shrub species (S.Spe); Average height of grass (G.Hig); Average density of grass (G.Den); Grass biomass (G.Bio); Number of grass species (G.Spe); Precipitation (Pre); Temperature (Tem); Hours of sunshine (Sun); Humidity (Hum).
The activity rhythm overlap index reflects the degree of competition and temporal niche separation between two species (Sunarto et al., 2015). The activity patterns of Midday gerbil and NTJ in the grazing exclusion area showed high similarity in different seasons, and the separation degree in the temporal niche between two species was low. Studies in the same study area showed that food availability in the grazing exclusion area was significantly higher than that in the grazing area (Yang et al., 2020). Midday gerbils and NTJ have a similar diet, but higher food availability alleviated the pressure of food competition. Thus, despite high temporal niche overlap of Midday gerbil with NTJ in the grazing exclusion area, they can coexist in the grazing exclusion area due to sufficient food and the lack of large competitors such as livestock. In the grazing areas, the activity patterns of Midday gerbil and NTJ begin to stagger significantly in summer, with a separation of the temporal ecological niches of the two species. This is because summer is a critical period for the growth and development of individual herbivores and determines breeding success (Wang et al., 2021). Interspecific competition among animals is particularly intense in summer, and the presence of competitors can influence the behavioral patterns of species (Ritchie and Johnson, 2010). In contrast, temporal niche separation of species usually occurs in situations where alternative mechanisms of coexistence cannot operate (spatial and trophic niche; Harrington et al., 2009; Anna et al., 2015). To reduced competition, Midday gerbil and NTJ have adopted a strategy of temporal niche separation to achieve coexistence. Therefore, hypothesis (1) was supported, as desert rodents with similar a diet used a strategy of temporal (grazing areas) niche separation to reduce niche overlap and achieve coexistence.
There were seasonal differences in the activity duration of Midday gerbil and NTJ. Their activity durations were significantly higher in spring. In spring, rodents have a higher energy requirement caused by limited food availability (Yang et al., 2020), lower ambient temperatures (Sun, 2006), and frequent reproduction behavior (Wei et al., 1996; Topilko et al., 2022). In summer, the activity duration of Midday gerbil and NTJ was significantly curtailed, and the high temperatures reduced the animals’ foraging efficiency (Plessis et al., 2012). Moreover, food was sufficiently abundant to meet rodents’ energy needs and there was no need to let itself under the risk of predation. Desert rodents maximize their foraging efficiency by reducing the time spent outside their burrows to reduce water loss (Hut et al., 2012) and predation risk, which facilitates their adaptation to arid desert environments (Ji et al., 2021). Due to the higher predation risk from low vegetation coverage for quadrupedal gerbils in the grazing area (Pereira et al., 2012), the activity duration of Midday gerbil in the grazing area in summer was significantly lower than that in the grazing exclusion area. In autumn, there were differences in the activity allocation of the two dominant rodents, because they had different overwintering patterns (Shuai et al., 2016; Yuan et al., 2018; Yang et al., 2020). Midday gerbils are non-hibernators, so they increased food storing behavior and showed confrontation behavior in autumn. NTJ are hibernators (Zhao, 1964). Because they need to store body fat reserves prior to hibernation, and fighting behaviour can be energy draining, so fighting behaviour of NTJ was significantly decreased in autumn. In early winter, the population density of NTJ in the grazing area decreased significantly, while in the grazing exclusion areas it remained at a high level. An increase in population size augments the movement distance and activity duration of mammals (Grove, 2012), The proportion of activity duration spent on each behavior was significantly higher in the grazing exclusion area than in the grazing area. The time allocation strategies of the Midday gerbils and NTJ in the desert area varied with seasons, which not only facilitated their sympatric coexistence, but also enabled them be in a favorable position with regard to natural selection.
Synergies between land use and climate change have more profound negative impacts on biodiversity than single drivers (Brodie, 2016). Our results also demonstrate that rodents respond differently to grazing. Rodent activities in grazing areas had more influencing factors than in grazing exclusion areas. Livestock grazing made desert rodents more sensitive to environmental changes. Both environmental humidity and rainfall had a significant negative effect on the activity overlap of the two rodent species. Midday gerbil and NTJ had different responses to changes in ambient temperature and humidity. Midday gerbil are highly tolerant to rainfall, while NTJ are highly tolerant to high temperature (Wu et al., 2016). The different environmental tolerances of the Midday gerbil and NTJ reduced the overlap between their activities, and facilitated coexistence in the same domain. Our results did not support hypothesis 3, as the key pathways of the effects of grazing on the temporal niche changes and behavioral patterns of sympatric Midday gerbil and NTJ were inconsistent. Based on rodent natural histories, cattle grazing may favor bipedal heteromyids more than quadrupedal species (Jones and Longland, 1999; Wu et al., 2016) because of grazing-induced changes in vegetative structure (Pereira et al., 2012). NTJ and Mongolian five-toed jerboa require similar habitat conditions (Jones and Longland, 1999; Shuai et al., 2016), Therefore, the key factor affecting the activity duration of NTJ in grazing areas was interspecific competition with the Mongolian five-toed jerboa. Grazing expanded the NTJ home range (Yuan et al., 2017b), which then resulted in limited space available for the Midday gerbil in the grazing area. Therefore, the key factor affecting the Midday gerbil behaviors in the grazing area was intraspecific competition. In the grazing exclusion area, the available space for NTJ was limited, so it was most affected by intraspecific competition (Zhang et al., 2022). Therefore, the greatest impact of grazing exclusion on Midday gerbil activity was interspecific competition with NTJ. Previous studies showed that ungulate trampling altered habitat traits and indirectly affected intraspecific competition among rodents (Navarro-Castilla et al., 2017), and intensified intraspecific competition can lead to behavioral adaptation (Murray et al., 2006; Karanth et al., 2017). This is consistent with our results.
Rodent communities in nature are intrinsically dynamic, and some of the dynamics noted during the relatively short duration of our study differed systematically between grazing and grazing exclusion areas, indicating that these animals are sensitive to livestock grazing. There were seasonal differences in the activity pattern and temporal niche of the dominant rodent species in the desert area, and they adjusted their activity strategies according to the habitat characteristics. Grazing increased the competitive pressure on desert rodents, and long-term overgrazing is inevitably unfavorable to species coexistence. Ranchers should formulate suitable grazing regimes based on grass yield to maintain healthy pastures, so that cattle have adequate forage.
The original contributions presented in the study are included in the article further inquiries can be directed to the corresponding authors.
The animal study was reviewed and approved by Research Ethics Review Committee of Inner Mongolian Agricultural University (NND2017012).
XL and SY analyzed the data and wrote the manuscript. LLi, HZ, YJ, LLiu, RZ, SS, and FB collected data and samples in the field. XW and HF mapped out the manuscript. All authors contributed to the article and approved the submitted version.
This experiment was funded by Program for improving the Scientific Research Ability of Youth Teachers of Inner Mongolia Agricultural University, the 2022 Inner Mongolia Autonomous Region Youth Science and Technology Talent Development Plan (NJYT22044), the National Natural Science Foundation of China (32060256, 32060395), Inner Mongolia Autonomous Region Postgraduate Research Innovation Funding Project (B20210160Z), Science and Technology Project of Inner Mongolia Autonomous Region (2021GG0108), Major Science and Technology Project of Inner Mongolia Autonomous Region (2021ZD0006), Grassland Ecological Protection and Restoration Treatment Subsidy.
We would like to thank SW Yang, K Yang, M Ming, N Zhu, DS Wen, QW Guo, and GK Chen for data collection.
The authors declare that the research was conducted in the absence of any commercial or financial relationships that could be construed as a potential conflict of interest.
All claims expressed in this article are solely those of the authors and do not necessarily represent those of their affiliated organizations, or those of the publisher, the editors and the reviewers. Any product that may be evaluated in this article, or claim that may be made by its manufacturer, is not guaranteed or endorsed by the publisher.
Alanärä, A., Burns, M. D., and Metcalfe, N. B. (2001). Intraspecific resource partitioning in brown trout: the temporal distribution of foraging is determined by social rank. J. Anim. Ecol. 70, 980–986. doi: 10.1046/j.0021-8790.2001.00550.x
Anna, W., Andrzej, Z., and Crowther, M. S. (2015). Spatial niche segregation of sympatric stone marten and pine marten – avoidance of competition or selection of optimal habitat? PLoS One 10:e0139852. doi: 10.1371/journal.pone.0139852
Ayala, G. M., Viscarra, M. E., Sarmento, P., Negrões, N., Fonseca, C., and Wallace, R. B. (2021). Activity patterns of jaguar and puma and their main prey in the greater Madidi-Tambopata landscape (Bolivia, Peru). Mammalia 85, 208–219. doi: 10.1515/mammalia-2020-0058
Azevedo, F. C., Lemos, F. G., Freitas-Junior, M. C., Rocha, D. G., and Azevedo, F. C. C. (2018). Puma activity patterns and temporal overlap with prey in a human-modified landscape at southeastern Brazil. J. Zool. 305, 246–255. doi: 10.1111/jzo.12558
Azlan, J. M., and Sharma, D. S. K. (2006). The diversity and activity patterns of wild felids in a secondary forest in peninsular Malaysia. Oryx 40, 36–41. doi: 10.1017/S0030605306000147
Bach, T. H., Chen, J., Hoang, M. D., Beng, K. C., and Nguyen, V. T. (2017). Feeding behavior and activity budget of the southern yellow-cheeked crested gibbons (Nomascus gabriellae) in a lowland tropical forest. Am. J. Primatol. 79:e22667. doi: 10.1002/ajp.22667
Barber, I., and Dingemanse, N. J. (2010). Parasitism and the evolutionary ecology of animal personality. Philos. Trans. R. Soc. B Biol. Sci. 365, 4077–4088. doi: 10.1098/rstb.2010.0182
Braczkowski, A. R., Balme, G. A., Dickman, A., Fattebert, J., Johnson, P., Dickerson, T., et al. (2016). Scent lure effect on camera-trap based leopard density estimates. PLoS One 11:e0151033. doi: 10.1371/journal.pone.0151033
Brodie, J. F. (2016). Synergistic effects of climate change and agricultural land use on mammals. Front. Ecol. Environ. 14, 20–26. doi: 10.1002/16-0110.1
Brown, J. S. (1996). Coevolution and community organization in three habitats. Oikos 75, 193–206. doi: 10.2307/3546243
Bu, H. L., Wang, F., McShea, W., Lu, Z., Wang, D. J., and Li, S. (2016). Spatial co-occurrence and activity patterns of meso-carnivores in the temperate forests of Southwest China. PLoS One 11:e0164271. doi: 10.1371/journal.pone.0164271
Carothers, J. H., and Jaksić, F. M. (1984). Time as a niche difference: the role of interference competition. Oikos 42, 403–406. doi: 10.2307/3544413
Cepeda–Duque, J. C., Gómez–Valencia, B., Alvarez, S., Gutiérrez–Sanabria, D. R., and Lizcano, D. J. (2021). Daily activity pattern of pumas (Puma concolor) and their potential prey in a tropical cloud forest of Colombia. Anim. Biodivers. Conserv. 44, 267–278. doi: 10.32800/abc.2021.44.0267
Cui, Z. W., Wang, Z. L., Zhao, G. L., and Lu, J. Q. (2015). Seasonal variations in activity budget of adult female rhesus macaques (Macaca mulatta) at Mt. Taihangshan area, Jiyuan, China: effects of diet and temperature. Acta Theriol. Sin. 35, 138–146. doi: 10.16829/j.slxb.2015.02.003
Daan, S. (1981). “Adaptive daily strategies in behavior,” in Biological Rhythms. ed. J. Aschoff (Boston, MA: Springer US), 275–298.
Ebensperger, L. A., and Hurtado, M. J. (2005). Seasonal changes in the time budget of degus, Octodon degus. Behaviour 142, 91–112. doi: 10.1163/1568539053627703
Fernandes, D., Bezerra, M., Silva, M., Leal, N., Reis, C., and Almeida, A. (2020). Rodent hosts and flea vectors in Brazilian plague foci: a review. Integr. Zool. 16, 810–819. doi: 10.1111/1749-4877.12480
Goulson, D., and Nicholls, E. (2022). Anthropogenic influences on bee foraging. Science 375, 970–972. doi: 10.1126/science.abn0185
Gribben, P. E., Byers, J. E., Clements, M., McKenzie, L. A., Steinberg, P. D., and Wright, J. T. (2009). Behavioural interactions between ecosystem engineers control community species richness. Ecol. Lett. 12, 1127–1136. doi: 10.1111/j.1461-0248.2009.01366.x
Grove, M. (2012). Space, time, and group size: a model of constraints on primate social foraging. Anim. Behav. 83, 411–419. doi: 10.1016/j.anbehav.2011.11.011
Harrington, L. A., Harrington, A. L., Yamaguchi, N., Thom, M. D., Ferreras, P., Windham, T. R., et al. (2009). The impact of native competitors on an alien invasive: temporal niche shifts to avoid interspecific aggression. Ecology 90, 1207–1216. doi: 10.1890/08-0302.1
Huang, H. (2021). linkET: Everything Is Linkable. R Package Version 0.0.7. Available at: https://github.com/Hy4m/linkET (Accessed June 2, 2022).
Hut, R. A., Kronfeld-Schor, N., van der Vinne, V., and De la Iglesia, H. (2012). In search of a temporal niche: environmental factors. Prog. Brain Res. 199, 281–304. doi: 10.1016/B978-0-444-59427-3.00017-4
Ji, Y., Yuan, S., Fu, H. P., Yang, S. W., Bu, F., Li, X., et al. (2021). Activity strategy and pattern of the Siberian jerboa (Orientallactaga sibirica) in the Alxa desert region, China. PeerJ 9:e10996. doi: 10.7717/peerj.10996
Jiao, S., Lu, Y., and Wei, G. (2021). Soil multitrophic network complexity enhances the link between biodiversity and multifunctionality in agricultural systems. Glob. Chang. Biol. 28, 140–153. doi: 10.1111/gcb.15917
Jones, A. L., and Longland, W. S. (1999). Effects of cattle grazing on salt desert rodent communities. Am. Midl. Nat. 141, 1–11. doi: 10.1674/0003-0031(1999)141[0001:EOCGOS]2.0.CO;2
Karanth, K. U., Srivathsa, A., Vasudev, D., Puri, M., Parameshwaran, R., and Kumar, N. S. (2017). Spatio-temporal interactions facilitate large carnivore sympatry across a resource gradient. Proc. R. Soc. B Biol. Sci. 284:20161860. doi: 10.1098/rspb.2016.1860
Kronfeld-Schor, N., and Dayan, T. (2003). Partitioning of time as an ecological resource. Annu. Rev. Ecol. Evol. Syst. 34, 153–181. doi: 10.1146/annurev.ecolsys.34.011802.132435
Li, Z. L., Duo, L. A., Li, S., and Wang, T. M. (2021). Competition and coexistence among terrestrial mammalian carnivores. Biodivers. Sci. 29, 81–97. doi: 10.17520/biods.2020359
Li, G., Yin, B., Wan, X., Wei, W., Wang, G., Krebs, C. J., et al. (2016). Successive sheep grazing reduces population density of Brandt’s voles in steppe grassland by altering food resources: a large manipulative experiment. Oecologia 180, 149–159. doi: 10.1007/s00442-015-3455-7
Li, X., Yuan, S., Fu, H. P., Wu, X. D., Yang, S. W., Ji, Y., et al. (2020). Activity rhythm and influencing factors of two dominant rodent species in desert region. Acta Theriol. Sin. 40, 585–594. doi: 10.16829/j.slxb.150417
Linkie, M., and Ridout, M. S. (2011). Assessing tiger–prey interactions in Sumatran rainforests. J. Zool. 284, 224–229. doi: 10.1111/j.1469-7998.2011.00801.x
Loggins, A. A., Shrader, A. M., Monadjem, A., and Mccleery, R. A. (2019). Shrub cover homogenizes small mammals' activity and perceived predation risk. Sci. Rep. 9:16857. doi: 10.1038/s41598-019-53071-y
Meredith, M., and Ridout, M. (2017). Package “overlap”: Estimates of coefficient of overlapping for animal activity patterns. Available at: https://cran.r-project.org/web/packages/overlap/overlap.pdf (Accessed October 14, 2022).
Murray, A. L., Barber, A. M., Jenkings, S. H., and Longland, W. S. (2006). Competitive environment affects food-hoarding behavior of Merriam's kangaroo rats (Dipodomys merriami). J. Mammal. 87, 571–578. doi: 10.1644/05-MAMM-A-172R1.1
Navarro-Castilla, Á., Díaz, M., and Barja, I. (2017). Ungulate disturbance mediates behavioral and physiological stress responses in Algerian mice (Mus spretus): a wild exclosure experiment. Hystrix 28, 165–172. doi: 10.4404/hystrix-28.2-12332
Odden, M., Wegge, P., and Fredriksen, T. (2010). Do tigers displace leopards? If so, why? Ecol. Res. 25, 875–881. doi: 10.1007/s11284-010-0723-1
Palkovacs, E. P., and Dalton, C. M. (2015). Ecosystem Consequences of Behavioural Plasticity and Contemporary Evolution. New York: Oxford University Press.
Pereira, J. A., Walker, R. S., and Novaro, A. J. (2012). Effects of livestock on the feeding and spatial ecology of Geoffroy's cat. J. Arid Environ. 76, 36–42. doi: 10.1016/j.jaridenv.2011.08.006
Plessis, K. L. D., Martin, R. O., Hockey, P. A. R., Cunningham, S. J., and Ridley, A. R. (2012). The costs of keeping cool in a warming world: implications of high temperatures for foraging, thermoregulation and body condition of an arid-zone bird. Glob. Chang. Biol. 18, 3063–3070. doi: 10.1111/j.1365-2486.2012.02778.x
Preez, B. D. D., Loveridge, A. J., and Macdonald, D. W. (2014). To bait or not to bait: a comparison of camera-trapping methods for estimating leopard Panthera pardus density. Biol. Conserv. 176, 153–161. doi: 10.1016/j.biocon.2014.05.021
Rahelinirina, S., Scobie, K., Ramasindrazana, B., Andrianaivoarimanana, V., Rasoamalala, F., Randriantseheno, L., et al. (2021). Rodent control to fight plague: field assessment of methods based on rat density reduction. Integr. Zool. 16, 868–885. doi: 10.1111/1749-4877.12529
Ridout, M. S., and Linkie, M. (2009). Estimating overlap of daily activity patterns from camera trap data. J. Agric. Biol. Environ. Stat. 14, 322–337. doi: 10.1198/jabes.2009.08038
Ritchie, E. G., and Johnson, C. N. (2010). Predator interactions, mesopredator release and biodiversity conservation. Ecol. Lett. 12, 982–998. doi: 10.1111/j.1461-0248.2009.01347.x
Shuai, L. Y., Ren, C. L., Cao, C., Song, Y. L., and Zeng, Z. G. (2014). Shifts in activity patterns of Microtus gregalis: a role of competition or temperature? J. Mammal. 95, 960–967. doi: 10.1644/13-mamm-a-303
Shuai, L. Y., Song, Y. L., Kotler, B. P., Embar, K., and Zeng, Z. G. (2016). Foraging behaviour in east Asian desert rodents and its implications on coexistence. Israel J. Ecol. Evol. 62, 171–177. doi: 10.1080/15659801.2015.1045279
Smith, J. A., Duane, T. P., and Wilmers, C. C. (2019). Moving through the matrix: promoting permeability for large carnivores in a human-dominated landscape. Landsc. Urban Plan. 183, 50–58. doi: 10.1016/j.landurbplan.2018.11.003
Song, K., and Liu, R. T. (1984). The ecology of midday gerbil (Meriones meridianus Pallas). Acta Theriol. Sinica 4, 292–300.
Sun, R.Y. (2006). Principles of Animal Ecology. Beijing: Beijing Normal University Publishing Group.
Sunarto, S., Kelly, M. J., Parakkasi, K., and Hutajulu, M. B. (2015). Cat coexistence in Central Sumatra: ecological characteristics, spatial and temporal overlap, and implications for management. J. Zool. 296, 104–115. doi: 10.1111/jzo.12218
Tang, C. B., Wang, G. H., Shi, Z. P., Li, S. Q., Huang, Z. H., Wang, Z. X., et al. (2020). Activity rhythm and time budget of the red-bellied squirrels (Callosciurus erythralus) based on infrared camera data. J. Guangxi Normal Univ. (Nat. Sci. Ed.) 38, 133–139. doi: 10.16088/j.issn.1001-6600.2020.01.018
Topilko, T., Diaz, S. L., Pacheco, C. M., Verny, F., Rousseau, C. V., Kirst, C., et al. (2022). Edinger-Westphal peptidergic neurons enable maternal preparatory nesting. Neuron 110, 1385.e8–1399.e8. doi: 10.1016/j.neuron.2022.01.012
Wang, L., Delgado-Baquerizo, M., Wang, D., Isbell, F., Liu, J., Feng, C., et al. (2019). Diversifying livestock promotes multidiversity and multifunctionality in managed grasslands. Proc. Natl. Acad. Sci. 116, 6187–6192. doi: 10.1073/pnas.1807354116
Wang, H. R., Li, Y. P., Guan, Z. H., Ren, G. P., Yang, X., Huang, Z. P., et al. (2021). Effect of group size on daily travel distance and activity budget of Rhinopithecus bieti during the winter and spring seasons in Mt. Lasha, Yunnan, China. Acta Theriol. Sin. 41, 11–19. doi: 10.16829/j.slxb.150434
Wang, T. T., Ma, C. R. B., Hai, S. Z., and Wang, D. (2015). Construction for the ethogram of brandt's voles (Lasiopodomys brandtii). Acta Agrestia Sin. 23, 646–652. doi: 10.11733/j.issn.1007-0435.2015.03.030
Wei, W. H., Zhou, W. Y., Wang, Q. Y., and Fan, N. C. (1996). Behaviour comparison of plateau zokor between reproductive and non-reproductive periods. Acta Theriol. Sin. 16, 194–201. doi: 10.16829/j.slxb.1996.03.007
Wilson, M. W., Ridlon, A. D., Gaynor, K. M., Gaines, S. D., Stier, A. C., and Halpern, B. S. (2020). Ecological impacts of human-induced animal behaviour change. Ecol. Lett. 23, 1522–1536. doi: 10.1111/ele.13571
Wu, X. D., Yuan, S., Fu, H. P., Zhang, X. D., Zhang, F. S., and Gao, Q. R. (2016). Responses of dominant rodent species to climate change in different disturbed habitats in the Alashan desert. Acta Ecol. Sin. 36, 1765–1773. doi: 10.5846/stxb201408061564
Yang, S. W., Yuan, S., Wu, X. D., Zhang, R., Yue, X. X., Ji, Y., et al. (2020). The effect of grazing on winter survival of midday gerbil (Meriones meridianus) of different genders. Ecol. Evol. 10, 12395–12406. doi: 10.1002/ece3.6870
Yuan, S., Fu, H. P., Wu, X. D., Xing, A., Gan, H. J., and Yue, X. X. (2017a). Response of dominant desert rodent species to grazing disturbances: a structural equation modeling analysis. Acta Ecol. Sin. 37, 4795–4806. doi: 10.5846/stxb201604090650
Yuan, S., Fu, H. P., Wu, X. D., Yang, S. W., and Yue, X. X. (2018). Effects of grazing on the northern three-toed jerboa pre- and post-hibernation: impact of grazing on jerboa overwinter. J. Wildl. Manag. 82, 1588–1597. doi: 10.1002/jwmg.21550
Yuan, S., Yang, S. W., Fu, H. P., Li, Y. N., Wu, X. D., and Yue, X. X. (2017b). The effect of successive grazing on the home range of northern three toed jerboa (Dipus sagitta) in Alax desert. Chin. J. Zool. 52, 964–972. doi: 10.13859/j.cjz.201706005
Zhang, H. T., Fu, H. P., Yang, Y. K., Li, X., Li, L. L., Zhang, Z. R., et al. (2022). Changes and influencing factors of five-toed jerboa’s (Allactaga sibirica) home range under different grazing disturbance in desert area. Chin. J. Grassland 44, 93–100. doi: 10.16742/j.zgcdxb.20210353
Zhao, K. T. (1964). The ecology of northern three toed jerboa (Dipus sagitta Pallas). Chin. J. Zool. 5, 59–62.
Keywords: desert, rodent, grazing exclusion, activity pattern, temporal niche, coexistence mechanisms
Citation: Li X, Yuan S, Li L, Zhang H, Jin Y, Liu L, Zhang R, Bu F, Sun S, Fu H and Wu X (2023) Influence of grazing on the activity pattern and temporal niche of two dominant rodent species in Alxa desert. Front. Ecol. Evol. 10:1105729. doi: 10.3389/fevo.2022.1105729
Received: 23 November 2022; Accepted: 16 December 2022;
Published: 09 January 2023.
Edited by:
Wei Wanrong, China West Normal University, ChinaCopyright © 2023 Li, Yuan, Li, Zhang, Jin, Liu, Zhang, Bu, Sun, Fu and Wu. This is an open-access article distributed under the terms of the Creative Commons Attribution License (CC BY). The use, distribution or reproduction in other forums is permitted, provided the original author(s) and the copyright owner(s) are credited and that the original publication in this journal is cited, in accordance with accepted academic practice. No use, distribution or reproduction is permitted which does not comply with these terms.
*Correspondence: Heping Fu, ✉ ZnVoZXBpbmdAMTI2LmNvbQ==; Xiaodong Wu, ✉ d3V4aWFvZG9uZ19oZ2JAMTYzLmNvbQ==
Disclaimer: All claims expressed in this article are solely those of the authors and do not necessarily represent those of their affiliated organizations, or those of the publisher, the editors and the reviewers. Any product that may be evaluated in this article or claim that may be made by its manufacturer is not guaranteed or endorsed by the publisher.
Research integrity at Frontiers
Learn more about the work of our research integrity team to safeguard the quality of each article we publish.