- 1Cooperative Wildlife Research Laboratory, School of Biological Sciences, Southern Illinois University, Carbondale, IL, United States
- 2Cooperative Wildlife Research Laboratory and Center for Ecology, School of Biological Sciences, Southern Illinois University, Carbondale, IL, United States
Introduction: Migratory songbirds have experienced consistent population declines over the past half-century. An important, though often overlooked, contributor to these declines is the loss and degradation of stopover areas during migration, which are critical for allowing migrants to rest and refuel, and arrive in good condition at breeding and nonbreeding grounds.
Methods: Here, we used publicly available eBird occurrence data to build species distribution models examining habitat suitability of the Dickcissel, a grassland-associated neotropical migrant, during spring and fall migration. Our models included variables representing land cover type, climate, and net primary productivity.
Results: Land cover variables were consistently better predictors of Dickcissel occurrence than climate or net primary productivity, suggesting that land cover type has a stronger impact on stopover habitat suitability than environmental factors. During spring migration, Dickcissel occurrence probability decreased with shrubland cover and precipitation seasonality. During fall migration, occurrence probability increased with proximity to water and human development, and decreased with increasing net primary productivity. We detected positive associations during both spring and fall with annual mean temperature and temperature seasonality, and a negative association with forest cover. Surprisingly, our models detected no strong associations with grassland or agriculture, despite the importance of these habitat types for Dickcissels during the breeding and nonbreeding seasons.
Discussion: Ultimately, our results suggest that Dickcissels exhibit greater flexibility when selecting stopover sites compared to breeding or nonbreeding areas, although their avoidance of forest and shrubland may indicate that they will be negatively impacted by further woody encroachment into open habitats across their migratory routes.
1. Introduction
The past several decades have witnessed an alarming decline of birds, including common species, across North America (Rosenberg et al., 2019). Although multiple factors are responsible, the primary culprits in population declines of birds and other taxa are likely anthropogenic climate and land use change, resulting in loss and degradation of available habitat (Mawdsley et al., 2009; Rosenberg et al., 2016). Migratory birds are particularly at risk, as they rely on suitable habitat availability across widely dispersed locations, and may face threats particular to different regions throughout their annual cycle. Most avian ecology studies are limited to the breeding season, although events during the nonbreeding and migratory stages can have major impacts on bird populations (Sillett and Holmes, 2002; Norris and Marra, 2007). Migration is an influential stage during a bird’s annual cycle, particularly for long distance migrants, as mortality can be high (Netwon, 2007; Diehl et al., 2014; Oppel et al., 2015), and conditions during migration may impact breeding success and overwintering survival via density-dependent compensation and carry-over effects (Norris and Marra, 2007; Harrison et al., 2011; Legagneux et al., 2011). Environmental or land use changes that influence resource availability during migration or delay the arrival to breeding or nonbreeding areas can affect individual and population-level survival and breeding success for the following and subsequent seasons (Norris and Marra, 2007; Sorensen et al., 2009; Harrison et al., 2011; Briedis et al., 2018). Therefore, increasing our understanding of this important stage of a bird’s annual cycle, and its relationship with climate and landcover, is critical for avian conservation.
The Dickcissel (Spiza americana) is an obligate grassland bird that breeds in the tallgrass and mixed-grass North American prairies from Texas to Canada. Although recent population trends are generally stable, Dickcissels experienced severe population declines in the mid-to late-20th century from which they have yet to recover (Sauer et al., 2020; Sousa et al., 2022), and remain vulnerable due to habitat loss and other anthropogenic impacts throughout their annual cycle (Culp et al., 2017). Dickcissels are neotropical migrants, and the majority of the global population spends the nonbreeding season in gregarious flocks in the llanos region of Venezuela (Basili and Temple, 1999). Other nonbreeding areas include northern Columbia and the Pacific coast of Central America and Mexico. Like other neotropical migrants, Dickcissels are especially vulnerable to population declines compared to residents and short-distance migrants due to the variety of threats they may face in different regions throughout their annual cycle. Dickcissels have been negatively impacted by habitat loss and landscape change in their breeding areas (Rickletts et al., 1999; Samson et al., 2004), and persecution by farmers in their nonbreeding grounds (Basili and Temple, 1999), where they are considered an agricultural pest. Dickcissels are a well-studied bird, and much is known about their habitat associations and ecology during the breeding and nonbreeding seasons. However, relatively little is understood about their habitat associations during migration (Sousa et al., 2022).
In this study, we employed species distribution models based on eBird occurrence data to estimate the influence of environmental and landcover characteristics on habitat suitability for Dickcissels during migration. Our goal was to gain insight into how climate, net primary productivity, and landcover type may affect habitat suitability for Dickcissels during spring and fall migration. Dickcissels primarily migrate in large flocks through Central America and Mexico (Sousa et al., 2022), though some birds migrate over the Gulf of Mexico and stop-over in the Caribbean (Lowery, 1946; Bond, 1985). In addition to improving our understanding of Dickcissel migratory stopover habitat, we sought to examine the extent to which Dickcissel habitat associations during spring and fall migration differed. We expected that Dickcissel landcover associations during migration would be similar to those in breeding and nonbreeding areas, including an association with grasslands and agriculture (Basili and Temple, 1999; Fletcher and Koford, 2002; Jacobs et al., 2012; Reiley and Benson, 2019), and avoidance of tree and shrub cover (Hughes et al., 1999; Fletcher and Koford, 2002; Osborne and Sparling, 2013). We predicted that Dickcissel habitat suitability would be positively associated with net primary productivity (NPP), as NPP values generally correlate with greater resource availability for birds (Renfrew et al., 2013; Bridge et al., 2016; Leveau, 2019), including arthropod prey (Fernández-Tízon et al., 2020). Because long-distance migrations are energetically demanding, food availability and quality should have a strong influence on Dickcissel habitat associations during migration. Finally, we predicted that habitat associations during spring migration would differ from those during fall migration. Seasonal changes in the landscape may result in landcover types offering different levels of resources between the spring and fall, and nutrient demands for individuals may vary seasonally (Scott et al., 1994).
2. Materials and methods
2.1. Study area
Migratory routes for Dickcissels vary among individuals, due to the large geographic range of their breeding areas. For instance, individuals that breed farther north may migrate through the midwestern United States before arriving on their breeding grounds in Canada, while individuals that breed near the southern edge of the Dickcissel breeding range may end their spring migration in central Texas. For this study, we chose to limit our analysis to an area encompassing northern South America to the southern portion of the United States, bounded by 35° latitude to the north, 1° latitude to the south, and −70° longitude to the east (Figure 1), which represents the core of most Dickcissel migratory routes. Although limiting our study area in this way reduces our inference into habitat selection patterns in the northern part of some Dickcissels’ migratory range, it improves the chances that our occurrence data represent birds that were actively migrating, and not at their breeding or wintering grounds, when their location was recorded.
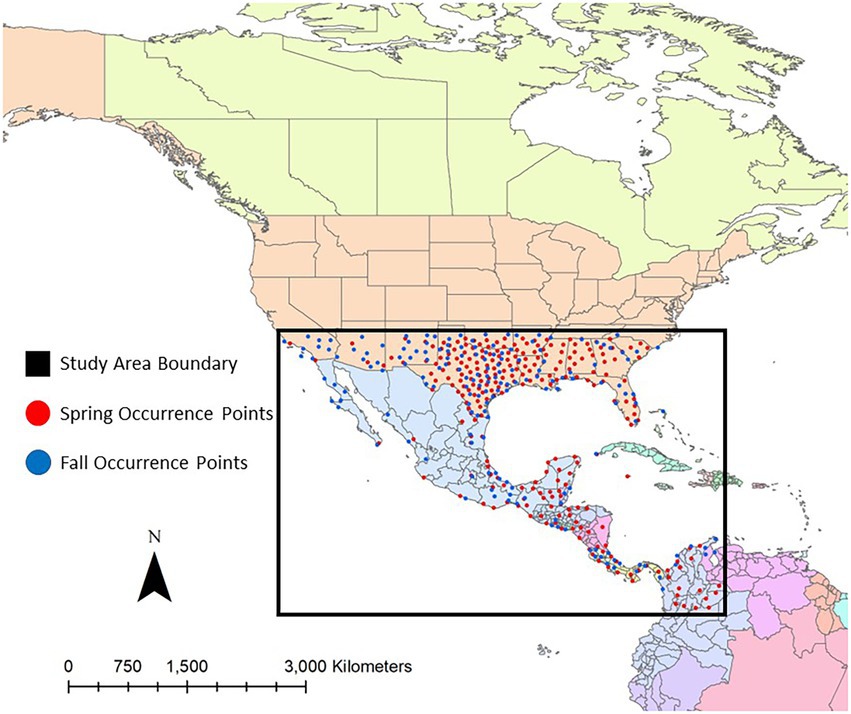
Figure 1. Map of the study area, representing the core of most Dickcissel migratory routes. Dickcissel occurrence points within this study area were used to estimate how habitat suitability for migrating Dickcissels relates to climate and landcover variables. Dickcissel occurrence points during spring migration are represented as red dots, and occurrence points during fall migration are represented as blue dots.
2.2. Occurrence points
Dickcissel locations were obtained from an eBird dataset that spanned 2001 to 2010, accessed via GBIF (Auer et al., 2021). We limited the timeframe of our dataset from 2001 to 2010 so the location data would be more temporally aligned with our land cover raster layers, which are derived from satellite imagery collected from 2000 to 2006 (Tuanmu and Jetz, 2014). To create a dataset of spring migration occurrence points, we truncated the Dickcissel location dataset to only include locations recorded between 25th March and 10th May, the approximate peak of spring migration for Dickcissels (Orians and Paulson, 1969; Basili and Temple, 1999; Larkin et al., 2002). For the fall migration dataset, we only considered locations recorded between September 1 and November 10, representing the approximate peak of fall migration (Sousa et al., 2022). As departure and arrival dates vary for Dickcissels during migration, we limited our occurrence points geographically as well as temporally by excluding any occurrence points that fell outside the bounds of our study area.
We spatially rarefied the spring and fall location data at a 75 km resolution to account for spatial bias in sampling density (Boria et al., 2014; Geldmann et al., 2016), as the activity of eBird users is not consistent throughout the Dickcissel’s migratory range. After spatial rarefying, our spring dataset was reduced from 5,436 to 256 unique locations, and our fall dataset was reduced from 2,112 to 179 unique locations. The final occurrence point dataset is available for download in Supplementary material.
2.3. Environmental and landcover data layers
We used both environmental (climate and NPP) and landcover data layers as predictor variables for our models. Our climate variables included an a priori subset of the bioclimatic variables from WorldClim 2.1 (Fick and Hijmans, 2017): annual mean temperature, temperature seasonality (standard deviation of daily temperature values ×100), maximum temperature of the warmest month, annual precipitation, precipitation of the wettest month, precipitation of the driest month, and precipitation seasonality. These variables are derived from aggregate climate data from 1970 to 2000, and have a spatial resolution of 30 arc-seconds (approximately 1 km). We included an NPP raster (Imhoff et al., 2004) made available by NASA’s Socioeconomic Data and Applications Center (SEDAC). The NPP raster was downscaled in ArcMap 10.6 (ESRI, 2018) to a 30 arc-second resolution using a bilinear resampling method, to be compatible with our other raster layers. Our land cover variables consisted of continuous land cover data layers at 30 arc-second resolutions from EarthEnv.org (Tuanmu and Jetz, 2014). Values for landcover data layers were the estimated percent coverage of the landcover class within each raster pixel, and range from 0 to 100. These data layers include 12 different land cover classes, four of which represent different types of tree cover (evergreen/deciduous needleleaf, evergreen broadleaf, deciduous broadleaf, and mixed/other). We combined these four raster layers in ArcMap by summing the values of overlaying pixels to create one raster layer representing tree cover. The other eight landcover classes included shrubs, herbaceous vegetation, cultivated and managed vegetation, regularly flooded vegetation, urban/built up, snow/ice, barren, and open water. Because we were primarily concerned with Dickcissel habitat selection during migration, and because most eBird users are not documenting Dickcissels that fly over open ocean, we modified the open water raster layer in ArcMap by clipping out all ocean pixels, to create a variable that represented terrestrial water bodies. All data layers were clipped to the extent of the study area in ArcMap 10.6. To reduce covariance among our predictor variables, we first ranked variables based on our a priori belief of their importance. We then created a correlation matrix among all variables. For any variable pairs that were highly correlated (|Pearson’s R| > 0.7), we removed the lower ranked variable. See Table 1 for the final list of predictor variables included in our models.
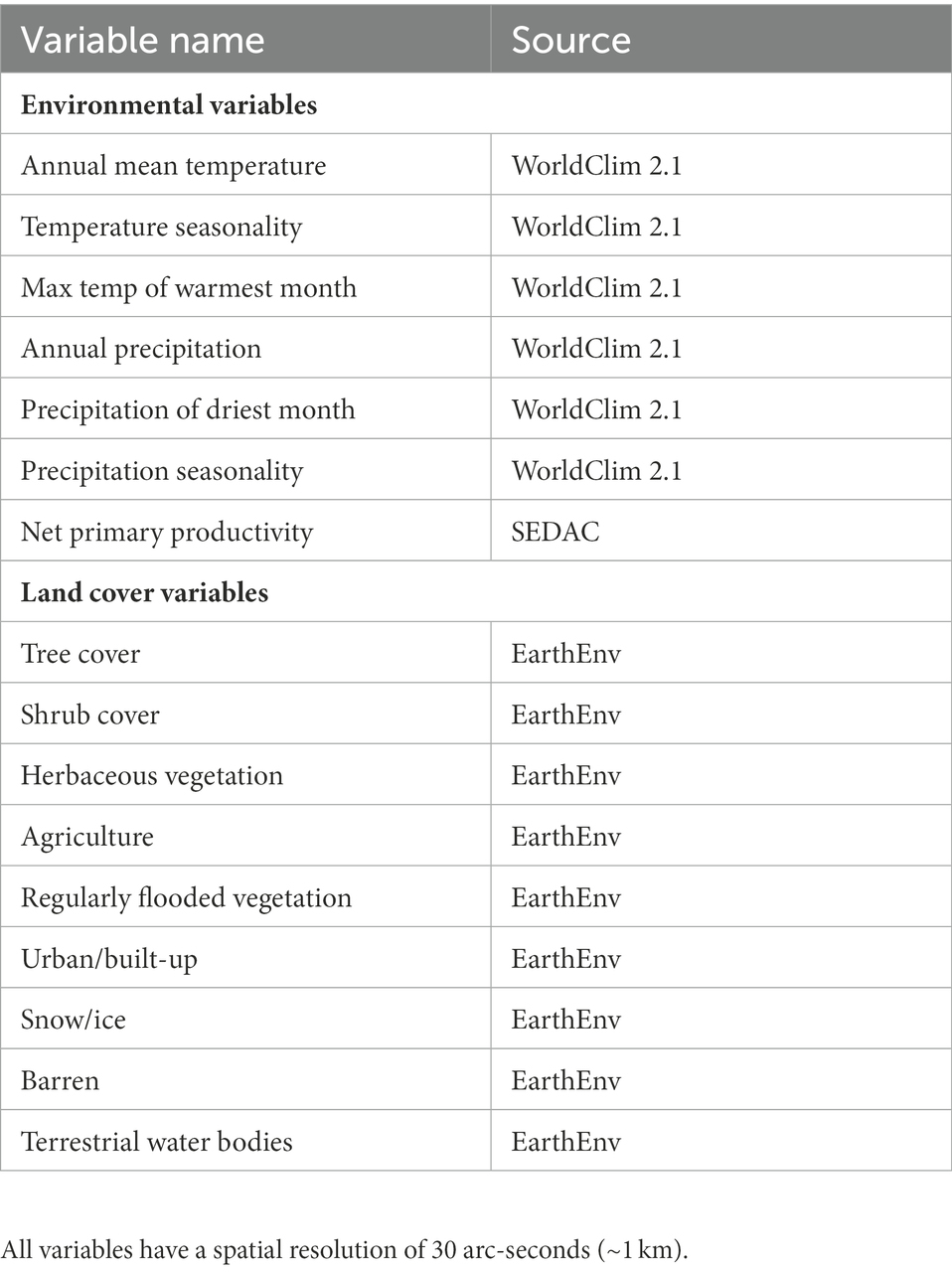
Table 1. Environmental and land cover variables used to build species distribution models for Dickcissels during spring and fall migration.
2.4. Species distribution models
We used species distribution models (SDMs) to estimate the influence of our predictor variables on Dickcissel habitat suitability during migration. We generated SDMs in Maxent 3.4.4 (Phillips et al., 2006) as implemented in SDMtoolbox 2.5 (Brown, 2014; Brown et al., 2017). Maxent builds SDMs from presence-only data by selecting random background points to which the species occurrence points are compared. When building SDMs using presence-only data, it is important to select background points from areas accessible to the species, as failing to do so may artificially inflate model performance or otherwise bias model results (Van DerWal et al., 2009; Barve et al., 2011). Therefore, we limited background point selection to an area representing a 500 km radial buffer around occurrence points, within the confines of the study extent (Figure 1). We chose a large radial buffer size for background point selection due to the high mobility of these birds, which are capable of dispersing widely throughout their migratory range. Maxent selected 10,000 random background locations, the default setting, for modeling. To properly parameterize the SDMs, we evaluated model performance under all combinations of the five Maxent feature classes (linear; linear and quadratic; hinge; linear, quadratic and hinge; linear, quadratic, hinge, and product) and 10 regularization multipliers (0.5 to 5, in increments of 0.5). Models were evaluated through a geographically structured k-fold cross-validation (k = 3), in which occurrence records were divided into three geographically clustered groups. Models were trained with two of the groups and then evaluated with the excluded group until all group combinations were run. We assessed model performance by inspecting, in order of importance, the omission error rate (OER), area under the curve (AUC), and model feature class complexity (Brown et al., 2020). We prioritized OER over AUC because AUC is more susceptible to bias resulting from the lack of true absence points in our dataset: OER calculates the percentage of presence points that were misidentified by the model, whereas AUC considers both misidentified presence points and misidentified absence points (commission errors). The background points generated by Maxent for our dataset are not true absence points, as Dickcissels may have been present in these areas. Despite this shortcoming, AUC remains a useful performance metric for presence-only SDMs, as we still wish to avoid gross commission errors in our models. After assessing model performance, we used the parameters present in the best-performing model to build a final SDM with five replicates, which was converted into a binary (presence-absence) model using the 10% training presence value as the threshold.
To estimate whether environmental variables or land cover type has a greater influence on Dickcissel habitat suitability during migration, we created three different SDMs for both the spring and fall migration datasets: an environment model consisting of all environmental variables, a landcover model consisting of all landcover variables, and an environment + landcover model consisting of all variables (environmental and landcover, Table 1). We estimated the influence of each variable by examining their percent contribution to the final SDM (Coxen et al., 2017). We considered variables with >10% contribution to be important contributors to their model, thus influential variables in determining Dickcissel habitat suitability during migration.
3. Results
3.1. Spring migration
We built three parameter-optimized SDMs for Dickcissels during spring migration, each associating Dickcissel occurrence points with one of three groups of predictor variables: environment, land cover, and all variables together. The land cover model demonstrated the best fit to the data, with an OER of 0.093. Both the environment model and the environment + land cover model had an OER of 0.117 (Table 2). For the land cover model, shrub cover (58.5% contribution to the model) and tree cover (26.3%) were the most important contributors to the model. Response curves for these variables (Figure 2) indicate that habitat suitability for Dickcissels during spring migration decreases with increasing cover of shrubs or trees. For the climate model, the variables with the greatest contribution included precipitation seasonality (56.7%), annual mean temperature (24.4%), and temperature seasonality (16%). Variable response curves suggest that Dickcissel habitat suitability is negatively related to precipitation seasonality, and positively related to temperature seasonality and annual mean temperature (Figure 3). For the environment + land cover model, the most important variables were shrub cover (44.2%), tree cover (19.1%), and temperature seasonality (11.2%). As with the land cover and environment models, Dickcissel habitat suitability was positively related to temperature seasonality and negatively related to forest and shrub cover. Habitat suitability maps for spring migration SDMs are shown in Supplementary Figure S1.
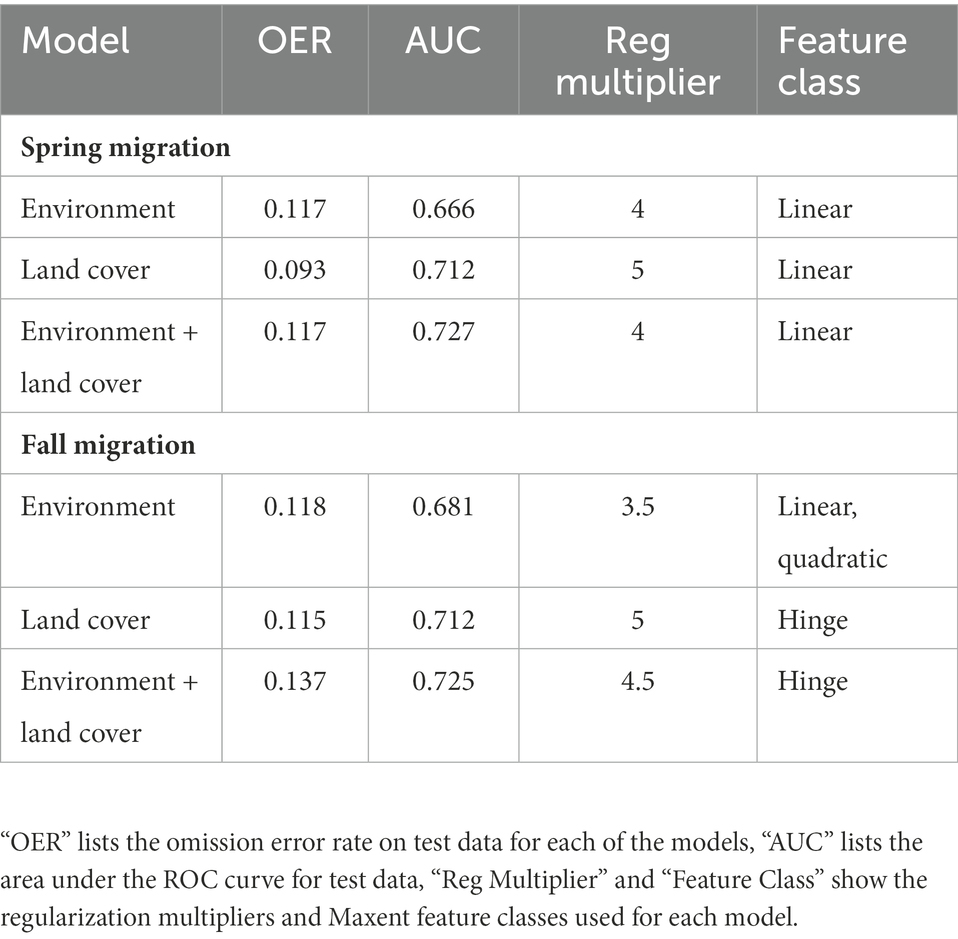
Table 2. Parameter settings and model fit for the final species distribution models using environment, land cover, and environment + land cover variables.
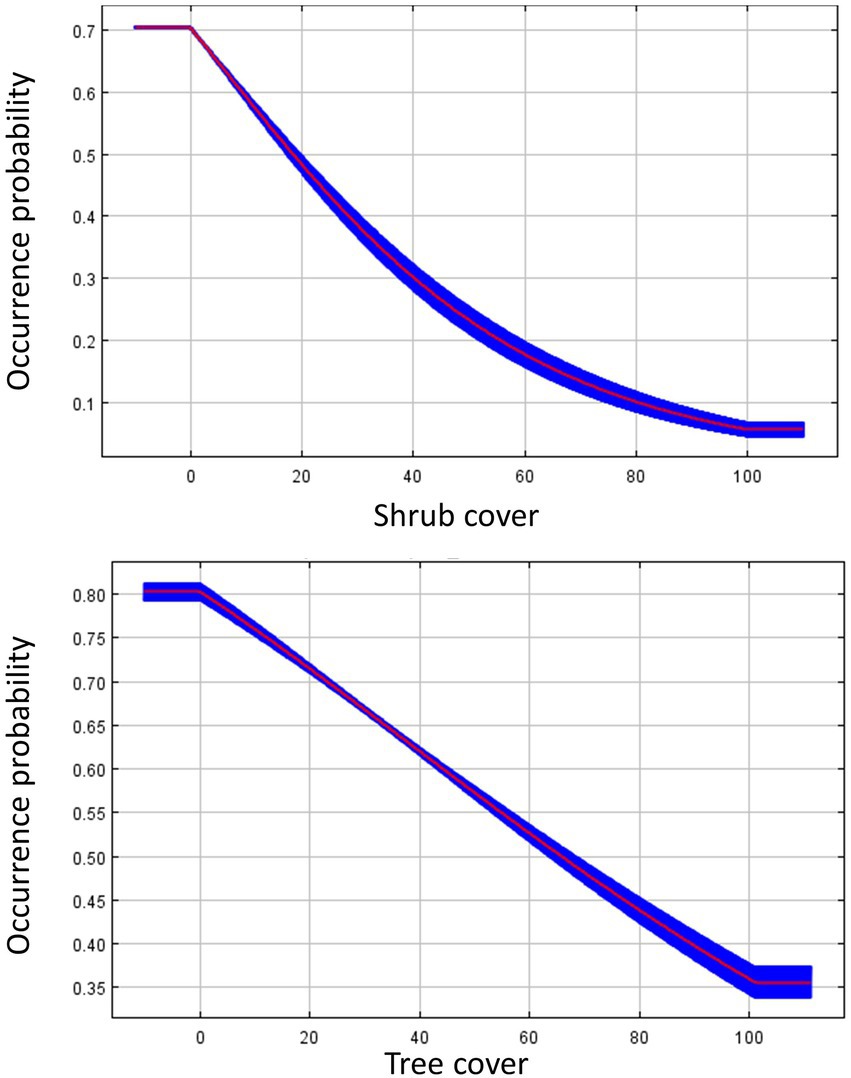
Figure 2. Response curves for influential land cover variables from the species distribution model predicting Dickcissel occurrence during spring migration. The response curves were generated in Maxent, and show how the predicted occurrence probability changes with each variable, while keeping all other variables at their average sample value. The red line plots the average value of the five model replicates, and the blue area plots the variance among the five replicates.
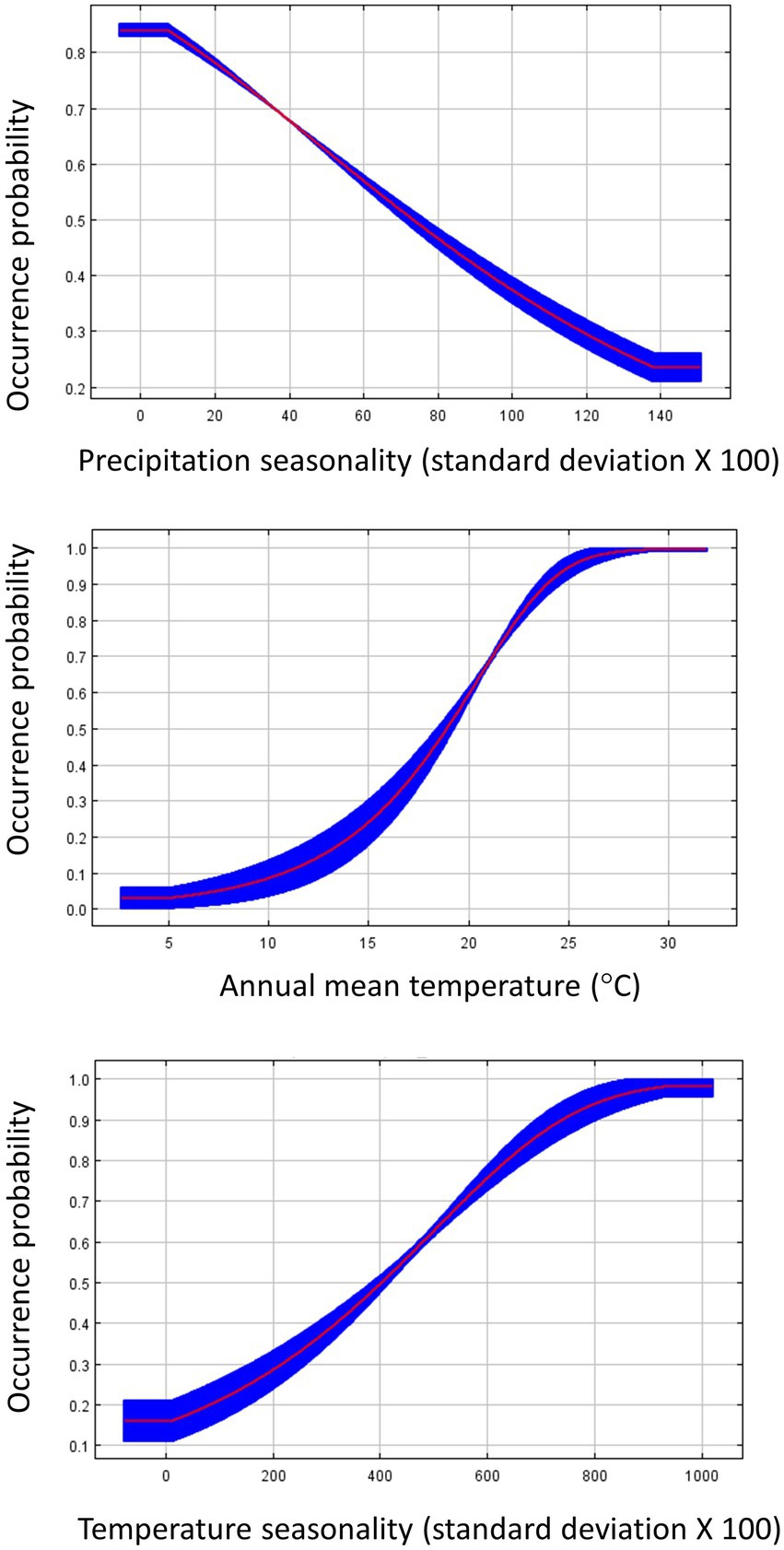
Figure 3. Response curves for influential environmental variables from the species distribution model predicting Dickcissel occurrence during spring migration. The response curves were generated in Maxent, and show how the predicted occurrence probability changes with each variable, while keeping all other variables at their average sample value. The red line plots the average value of the five model replicates, and the blue area plots the variance among the five replicates.
3.2. Fall migration
For our SDMs using fall migration occurrence points, the land cover model again demonstrated the best fit, with an OER of 0.115. The environment model was the second best with an OER of 0.118, while the environment + land cover model had an OER of 0.137 (Table 2). The most important contributors to the land cover model were water bodies (48.3% contribution to the model), urban/built-up areas (35.8%), and tree cover (14.9%). Variable response curves (Figure 4) suggest a preference for areas near water, as Dickcissel occurrence probability increased from 0.55 to >0.95 within 1 km of water. Dickcissel occurrence probability increased in the presence of urban areas and decreased in areas with high tree cover, though the differences in occurrence probability were smaller than that associated with water. The most influential variables for the environment model were NPP (33.6%), temperature seasonality (30.6%), and annual mean temperature (17.2%). Results from this model suggest that habitat suitability during fall migration is negatively related to NPP, and positively related to both annual mean temperature and temperature seasonality (Figure 5). Results from the environment + land cover model were similar to results from the land cover model, with water (45.3%), urban/built-up areas (33.2%), and tree cover (10.9%) being the most influential variables. Response curves were also similar to the land cover model, as Dickcissel occurrence probability increased in the presence of water and urban areas, and decreased in areas of high tree cover. Habitat suitability maps for fall migration SDMs are shown in Supplementary Figure S2.
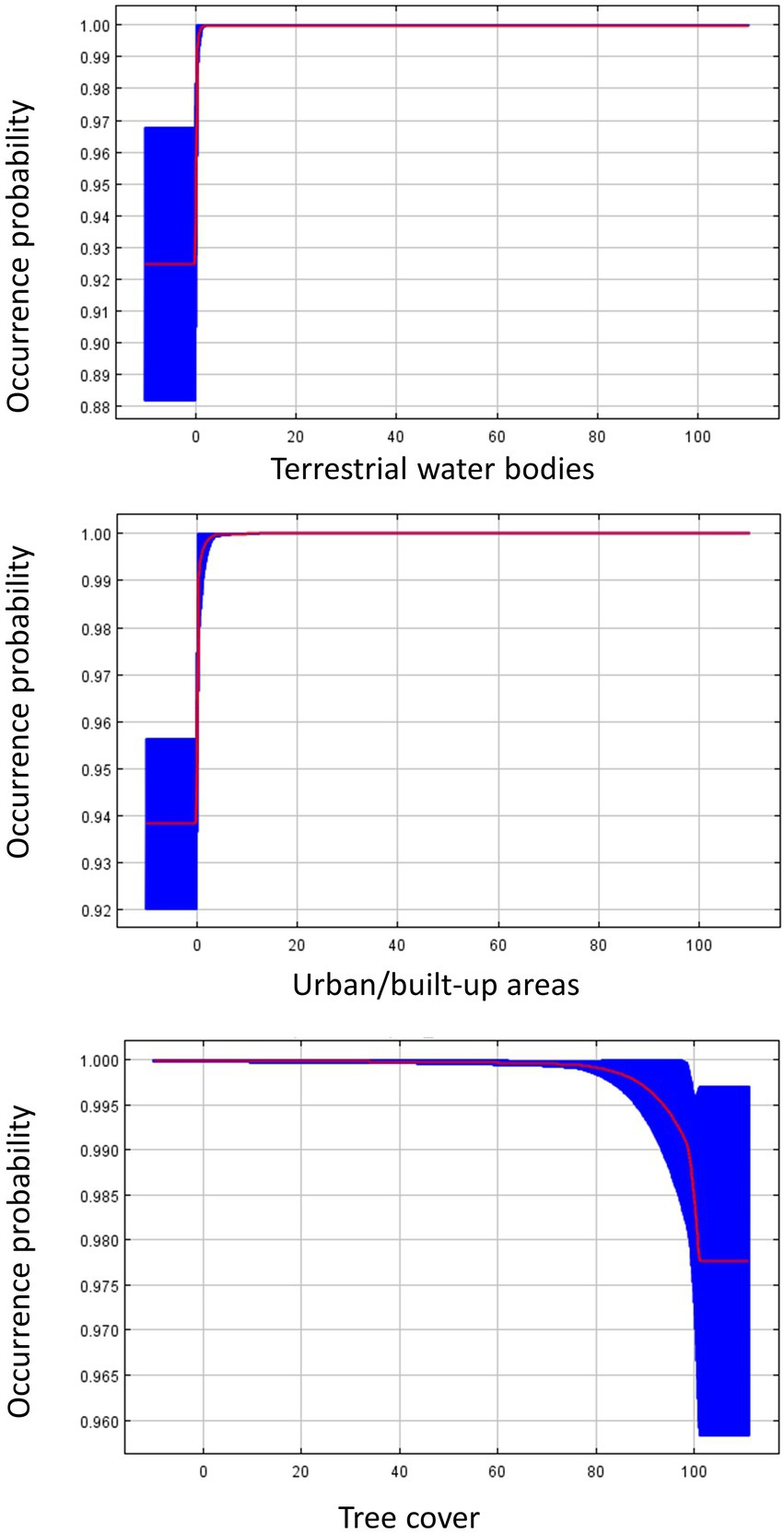
Figure 4. Response curves for influential land cover variables from the species distribution model predicting Dickcissel occurrence during fall migration. The response curves were generated in Maxent, and show how the predicted occurrence probability changes with each variable, while keeping all other variables at their average sample value. The red line plots the average value of the five model replicates, and the blue area plots the variance among the five replicates.
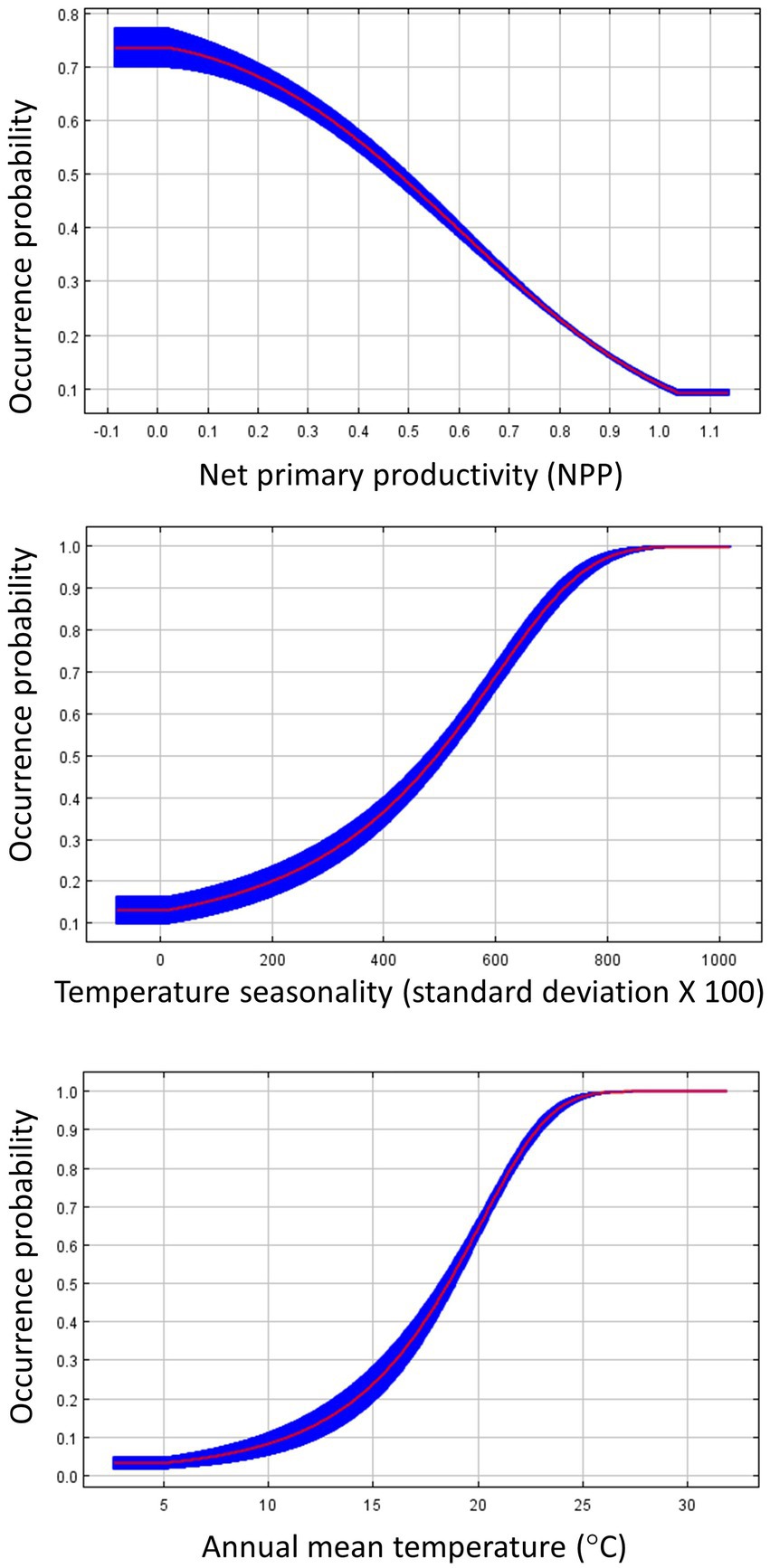
Figure 5. Response curves for influential environmental variables from the species distribution model predicting Dickcissel occurrence during fall migration. The response curves were generated in Maxent, and show how the predicted occurrence probability changes with each variable, while keeping all other variables at their average sample value. The red line plots the average value of the five model replicates, and the blue area plots the variance among the five replicates.
4. Discussion
In this study, we sought to estimate the influence of environmental variables and landcover type on habitat suitability for Dickcissels during migration. While our analysis allows us to detect patterns and estimate habitat relationships across a large study area at a course spatial scale, the 30 arc-second (~1 km) resolution of our raster layers reduced the ability of our models to detect habitat associations at finer scales, which may have influenced our results. Dickcissels are a widely distributed bird that can inhabit a variety of open landscapes, which could have reduced the importance of landcover in predicting Dickcissel occurrence during migration. However, our results suggest that Dickcissel occurrences during spring and fall migration are more highly associated with landcover than climate or NPP, especially during fall migration where no environmental variables contributed substantially to the environment + landcover model. In addition to the landcover models consistently having the lowest OER, the environment models had relatively low AUC (<0.7), suggesting questionable accuracy regarding their ability to identify suitable and unsuitable habitat. Climate has a strong influence on bird distributional ranges and habitat quality, although food availability and shelter from predators, which may be the most important characteristics for migratory bird stopover habitat (Ewert and Hamas, 1996), are determined more by landcover type than by climate within the geographical extent of our study area. Furthermore, while isolated weather events and more short-term weather patterns can have a strong impact on migratory birds (Reside et al., 2010), these are not necessarily reflected in our climate data, which represents long-term trends. The greater influence of landcover compared to environmental variables may indicate that anthropogenic landscape changes will have a greater impact on the migratory stage of these birds than future climate change. As expected, variables governing habitat suitability changed between spring and fall migration, reflecting seasonal changes in resource availability of different landcover types, and behavioral differences of migrating individuals and populations between the spring and the fall (Yong et al., 1998; Horton et al., 2016). However, we documented a consistent negative association with tree cover and positive association with annual mean temperature and temperature seasonality during both spring and fall.
4.1. Spring migration
Of the landcover variables we examined, tree and shrub cover were the most influential during spring migration, and were negatively associated with Dickcissel occurrence probability. This is consistent with Dickcissel habitat preferences in their breeding grounds; however, during the nonbreeding season Dickcissels occasionally use forest patches as roosting sites (Basili and Temple, 1999), though they more frequently roost in sugarcane fields (Basili, 1997). During the breeding season, avoidance of tree and shrub cover is understood to be related to greater densities of nest predators and brood parasites in these areas (Hughes et al., 1999; Jensen and Finck, 2004; Thompson et al., 2014), which are not likely to affect Dickcissels to such an extent during migration. Furthermore, forests may offer more advantageous roosting sites for migrants, with greater protection from predators, than open areas (e.g., Lindström, 1990). Tree and shrub avoidance by migrating Dickcissels may instead relate to foraging preference, as these birds may be more successful foraging for seeds and arthropods in open areas dominated by herbaceous vegetation to which they are accustomed than in forests or shrublands.
Due to the lower accuracy of the environment models, climate and NPP variable results should be interpreted with caution. The spring environment model suggests a tendency for Dickcissel occurrence to associate positively with annual mean temperature and temperature seasonality during spring migration. Higher temperatures may represent greater food resources for migrating Dickcissels via increases in plant and arthropod biomass (Fernández-Tízon et al., 2020; Welti et al., 2020), in addition to reducing cold-related mortalities during migration. Temperature seasonality is distinct from annual mean temperature in that it represents annual variation in temperature, or the difference between high and low temperatures experienced annually. Although we are unsure of the mechanisms driving this association, we suggest that temperature seasonality may function as an index of grassland floral diversity and health, as many grassland-associated plants require sufficiently cold winter temperatures for germination (Greene and Curtis, 1950; Packard and Mutel, 1997), and warm spring and summer temperatures aid in growth and development. The positive association between Dickcissel occurrence and temperature seasonality may also be related to the effect that cooler winter temperatures have on slowing plant decomposition rates (e.g., Webster and Benfield, 1986; Holt, 2008). With winter plant decomposition rates being slower in areas with higher temperature seasonality, there may be more organic material left in the spring to serve as a food base for invertebrates (Eichholz and Elmberg, 2014), meaning more invertebrate prey for migrating Dickcissels.
Although total precipitation was not an informative variable in our SDMs, precipitation seasonality was an important contributor to the spring climate model and was negatively associated with Dickcissel occurrence. High precipitation seasonality corresponds to greater annual variation in precipitation, and may represent areas with a tendency to experience unpredictable weather, or more frequent storm occurrences in landscapes that otherwise receive low to moderate rainfall. Difficult weather events such as storms greatly increase the level of energy expenditure needed to migrate, and can cause massive mortalities in migratory birds (Netwon, 2007; Diehl et al., 2014).
4.2. Fall migration
During fall migration, Dickcissel occurrence probability increased within 1 km of terrestrial water bodies such as rivers and lakes. Riparian habitats can serve as important stopover sites for many migratory birds (e.g., Ewert and Hamas, 1996; Bonter et al., 2008) via increased productivity and the opportunity for birds to efficiently rehydrate. Interestingly, one of the primary advantages of riparian stopover sites is the abundance of emergent insects that hatch on or near water bodies during the spring (Smith et al., 1998, 2007), although we only detected an association with water bodies during fall migration. We suspect that the increased importance of water during fall migration may be related to the higher proportion of naïve juvenile migrants compared to spring migration. Juvenile migrants are weaker flyers than adults (Harel et al., 2016; Rotics et al., 2016; McCabe and Gugleilmo, 2019), thus they may be attracted to water bodies for rehydration opportunities and the beneficial microclimate (Bolund and Hunhammar, 1999). Additionally, the ability of juvenile Dickcissels to use the Earth’s magnetic field for navigation may not yet have developed (Munro et al., 1997; Holland and Helm, 2013), so they may rely on rivers and lakes as landmarks for navigation, resulting in more frequent stops near water bodies (Bonter et al., 2008). The positive association with urban and developed areas during fall migration may also be influenced by the presence of juvenile migrants in the fall occurrence dataset, who may be attracted to these areas by lower apparent predator densities or greater thermal climates. Juveniles may also be more willing than adults to take advantage of potential food sources in urban areas, as they have to stop and forage more frequently than their adult counterparts (McCabe and Gugleilmo, 2019). Alternatively, this association may be a result of sampling bias in our dataset, as eBird sightings are more likely to occur near urban or developed areas where the density of eBird users is higher (Geldmann et al., 2016). The spatial rarefication process we employed minimizes, but does not completely prevent, spatial bias in user sampling effort, especially for our fall migration dataset which had a smaller sample size than spring migration.
Contrary to our predictions, we documented no association with NPP in the spring, and a negative association during fall migration, despite the fact that food availability should generally correlate with NPP. Although several studies have related NPP to bird species richness, it is not always a reliable indicator of bird abundance (Bailey et al., 2004; Leveau, 2019). The 1 km2 scale at which NPP was measured here and in other similar studies may not necessarily reflect finer-scale spatial variation in food resources, or seasonal pulses in food availability that migratory birds have evolved to exploit. Additionally, although NPP was not highly correlated with forest or shrub cover, NPP will generally be higher in these habitat types than in open areas like grasslands or agriculture due to greater plant biomass in habitats dominated by trees, which may have contributed to the negative association between NPP and Dickcissel occurrence probability. Although tree cover was not as influential of a variable in the fall as in the spring, it still outranked NPP in importance for the environment + landcover model. Ultimately, this result may indicate that NPP is not necessarily a reliable indicator of habitat quality or resource availability for Dickcissels or other open field birds during migration.
As with spring migration, Dickcissel occurrence was positively associated with mean annual temperature and temperature seasonality, and negatively associated with forest cover. However, forest cover was not as influential as during spring migration, and our models detected no strong relationship with shrub cover. Taken together with the positive association of water and urban areas, the weaker aversion to tree cover displayed in the fall compared to spring may suggest that Dickcissels are more willing to exploit a variety of habitats during fall migration. This pattern is likely related to the presence of juvenile migrants in the fall who need to stop more frequently and spend more time foraging than adults (McCabe and Gugleilmo, 2019).
4.3. Conclusion
Dickcissel habitat associations during spring and fall migration were distinct from those often documented during their breeding and nonbreeding seasons. Our models indicated no association with grasslands or agriculture, despite the fact that Dickcissels rely heavily on these habitats during breeding and nonbreeding seasons, and large flocks have occasionally been observed in agricultural fields during migration (e.g., Slud, 1964; Monson, 1997). This result may have been influenced by the coarse spatial scale of our raster layers, as bird occurrences in small agricultural fields or grassland patches may have been interpreted by our models as occurring in another landcover type within 1 km. Alternatively, this result suggests that Dickcissels are capable of utilizing a variety of habitats during migration, and are perhaps less reliant on grasslands and agriculture than during other phases of their annual cycle. Migratory birds may generally be more flexible with habitat selection during migration than during other parts of the year, as they are tracking food availability which varies among and within habitats seasonally and annually (Ewert and Hamas, 1996; Parrish, 2000). However, our models detected a strong negative association with shrublands during spring migration, and a consistent avoidance of forests during both the spring and the fall. As woody encroachment in grasslands is common throughout the Americas (Anadón et al., 2014; Londe et al., 2022) our results may indicate that continued woody encroachment into open habitats across Dickcissel migratory routes could negatively impact these birds by reducing the availability of quality stopover sites. Therefore, limiting woody encroachment in grasslands throughout their migratory range may improve the quality and quantity of stopover habitat. Additionally, our results indicate that landcover types apart from grasslands, such as wetlands and riparian habitats, may have conservation value for migrating Dickcissels.
Data availability statement
The original contributions presented in the study are included in the article/Supplementary material, further inquiries can be directed to the corresponding author.
Ethics statement
Ethical review and approval was not required for the animal study because no animals were handled or manipulated for this study; instead, this study analyzes a publicly available dataset of bird occurrence points.
Author contributions
AG designed the study, conducted statistical analyses, and wrote the manuscript. ME edited the manuscript and secured research funding. JB designed the study, assisted with statistical analysis, and edited the manuscript. All authors contributed to the article and approved the submitted version.
Funding
This research was funded by the Illinois Department of Natural Resources via Federal Wildlife Aid Grant W-106-R.
Conflict of interest
The authors declare that the research was conducted in the absence of any commercial or financial relationships that could be construed as a potential conflict of interest.
Publisher’s note
All claims expressed in this article are solely those of the authors and do not necessarily represent those of their affiliated organizations, or those of the publisher, the editors and the reviewers. Any product that may be evaluated in this article, or claim that may be made by its manufacturer, is not guaranteed or endorsed by the publisher.
Supplementary material
The Supplementary material for this article can be found online at: https://www.frontiersin.org/articles/10.3389/fevo.2022.1095188/full#supplementary-material
References
Anadón, J. D., Sala, O. E., Turner, B. L., and Bennett, E. M. (2014). Effect of woody-plant encroachment on livestock production in north and South America. Proc. Natl. Acad. Sci. 111, 12948–12953. doi: 10.1073/pnas.1320585111
Auer, T., Barker, S., Borgmann, K., Charnoky, M., Childs, D., Curtis, J., et al. (2021). EOD – eBird Observation Dataset. Ithaca, NY: Cornell Lab of Ornithology.
Bailey, S.-A., Horner-Devine, M. C., Luck, G., Moore, L. A., Carney, K. M., Anderson, S., et al. (2004). Primary productivity and species richness: relationships among functional guilds, residency groups and vagility classes at multiple spatial scales. Ecography 27, 207–217. doi: 10.1111/j.0906-7590.2004.03631.x
Barve, N., Barve, V., Jiménez-Valverde, A., Lira-Noriega, A., Maher, S. P., Person, A. T., et al. (2011). The crucial role of the accesible área in ecological niche modeling and species distribution modeling. Ecol. Model. 222, 1810–1819. doi: 10.1016/j.ecolmodel.2011.02.011
Basili, G. D. (1997). Continental-scale ecology and conservation of Dickcissels. [dissertation]. [Madison (WI)]: University of Wisconsin.
Basili, G. D., and Temple, S. A. (1999). Winter ecology, behavior, and conservation needs of Dickcissels in Venezuela. Stud. Avian Biol. 19, 289–299.
Bolund, P., and Hunhammar, S. (1999). Ecosystem services in urban areas. Ecol. Econ. 29, 293–301. doi: 10.1016/S0921-8009(99)00013-0
Bonter, D. N., Gauthreax, S. A. Jr., and Donovan, T. M. (2008). Characteristics of important stopover locations for migrating birds: remote sensing with radar in the Great Lakes Basin. Conserv. Biol. 23, 440–448. doi: 10.1111/j.1523-1739.2008.01085.x
Boria, R. A., Olson, L. E., Goodman, S. M., and Anderson, R. P. (2014). Spatial filtering to reduce sampling bias can improve the performance of ecological niche models. Ecol. Model. 275, 73–77. doi: 10.1016/j.ecolmodel.2013.12.012
Bridge, E. S., Ross, J. D., Contina, A. J., and Kelly, J. F. (2016). Do molt-migrant songbirds optimize migration routes based on primary productivity? Behav. Ecol. 27, 784–792. doi: 10.1093/beheco/arv199
Briedis, M., Krist, M., Král, M., Voigt, C. C., and Adamík, P. (2018). Linking events throughout the annual cycle in a migratory bird—non-breeding period buffers accumulation of carry-over effects. Behav. Ecol. Sociobiol. 72:93. doi: 10.1007/s00265-018-2509-3
Brown, J. L. (2014). SDMtoolbox: a python-based GIS toolkit for landscape genetic, biogeographic, and species distribution model analyses. Methods Ecol. Evol. 5, 694–700. doi: 10.1111/2041-210X.12200
Brown, J. L., Bennet, J. R., and French, C. M. (2017). SDMtoolbox 2.0: the next generation python-based GIS toolkit for landscape genetic, biogeographic and species distribution model analyses. PeerJ 5:e4095. doi: 10.7717/peerj.4095
Brown, J. L., Paz, A., Reginato, M., Renata, C. A., Assis, C., Lyra, M., et al. (2020). Seeing the forest through many trees: multi-taxon patterns of phylogenetic diversity in the Atlantic Forest hotspot. Divers. Distrib. 26, 1160–1176. doi: 10.1111/ddi.13116
Coxen, C. L., Frey, J. K., Carleton, S. A., and Collins, D. P. (2017). Species distribution models for a migratory bird based on citizen science and satellite tracking data. Glob. Ecol. Conserv. 11, 298–311. doi: 10.1016/j.gecco.2017.08.001
Culp, L. A., Cohen, E. B., Scarpignato, A. L., Thogmartin, W. E., and Marra, P. P. (2017). Full annual cycle climate change vulnerability assessment for migratory birds. Ecosphere 8:e01565. doi: 10.1002/ecs2.1565
Diehl, R. H., Bates, J. M., Willard, D. E., and Gnoske, T. P. (2014). Bird mortality during nocturnal migration over Lake Michigan: a case study. Wilson J. Ornithol. 126, 19–29. doi: 10.1676/12-191.1
Eichholz, M. W., and Elmberg, J. (2014). Nest site selection by Holarctic waterfowl: a multi-level review. Wild 4, 86–130.
ESRI (2018). ArcMap GIS. Ver. 10.6. Environmental System Research Institute, Inc. Redlands, California
Ewert, D. N., and Hamas, M. J. (1996). “Ecology of migratory landbirds during migration in the Midwest” in Management of Midwestern landscapes for the conservation of Neotropical migratory birds, general technical report NC-187. ed. F. R. Thompson III (St. Paul, MN: USDA Forest Service), 200–208.
Fernández-Tízon, M., Emmenegger, T., Perner, J., and Hahn, S. (2020). Arthropod biomass increase in spring correlates with NDVI in grassland habitat. Sci. Nat. 107:42. doi: 10.1007/s00114-020-01698-7
Fick, S. E., and Hijmans, R. J. (2017). WorldClim 2: new 1km spatial resolution climate surfaces for global land areas. Int. J. Climatol. 37, 4302–4315. doi: 10.1002/joc.5086
Fletcher, R. J., and Koford, R. R. (2002). Habitat and landscape associations of breeding birds in native and restored grasslands. J. Wildl. Manag. 66, 1011–1022. doi: 10.2307/3802933
Geldmann, J., Heilmann-Clausen, J., Holm, T. E., Levinsky, I., Markussen, B., Olsen, K., et al. (2016). What determines spatial bias in citizen science? Exploring four recording schemes with different proficiency requirements. Divers. Distrib. 22, 1139–1149. doi: 10.1111/ddi.12477
Greene, H. C., and Curtis, J. T. (1950). Germination studies of Wisconsin prairie plants. Am. Midl. Nat. 43, 186–194. doi: 10.2307/2421891
Harel, R., Horvitz, N., and Nathan, R. (2016). Adult vultures outperform juveniles in challenging thermal soaring conditions. Sci. Rep. 6:27865. doi: 10.1038/srep27865
Harrison, X. A., Blount, J. D., Inger, R., Norris, D. R., and Bearhop, S. (2011). Carry-over effects as drivers of fitness differences in animals. J. Anim. Ecol. 80, 4–18. doi: 10.1111/j.1365-2656.2010.01740.x
Holland, R. A., and Helm, B. (2013). A strong magnetic pulse affects the precision of departure direction of naturally migrating adult but not juvenile birds. J. R. Soc. Interface 10:20121047. doi: 10.1098/rsif.2012.1047
Holt, R. D. (2008). Theoretical perspectives on resource pulses. Ecology 89, 671–681. doi: 10.1890/07-0348.1
Horton, K. G., Van Doren, B. M., Stepanian, P. M., Farnsworth, A., and Kelly, J. F. (2016). Seasonal difference in landbird migration strategies. Auk 133, 761–769. doi: 10.1642/AUK-16-105.1
Hughes, J. P., Robel, R. J., Kemp, K. E., and Zimmerman, J. L. (1999). Effects of habitat on dickcissel abundance and nest success in conservation reserve program fields in Kansas. J. Wildl. Manag. 63, 523–529. doi: 10.2307/3802638
Imhoff, M. L., Bounoua, L., Ricketts, T., Loucks, C., Harriss, R., and Lawrence, W. T. (2004). HANPP Collection: Global Patterns in Net Primary Productivity (NPP). Palisades, New York: NASA Socioeconomic Data and Applications Center (SEDAC).
Jacobs, R. B., Thompson, F. R. III, Koford, R. R., La Sorte, F. A., Woodward, H. D., and Fitzgerald, J. A. (2012). Habitat and landscape effects on abundance of Missouri’s grassland birds. J. Wildl. Manag. 76, 372–381. doi: 10.1002/jwmg.264
Jensen, W. E., and Finck, E. J. (2004). Edge effects on nesting Dickcissels (Spiza americana) in relation to edge type of remnant tallgrass prairie in Kansas. Am. Midl. Nat. 151, 192–199. doi: 10.1674/0003-0031(2004)151[0192:EEONDS]2.0.CO;2
Larkin, R. P., Evans, W. R., and Diehl, R. H. (2002). Nocturnal flight calls of Dickcissels and Doppler radar echoes over South Texas in spring. J. Field Ornithol. 73, 2–8. doi: 10.1648/0273-8570-73.1.2
Legagneux, P., Fast, P. L. F., Gautheir, G., and Bêty, J. (2011). Manipulating individual state during migration provides evidence for carry-over effects modulated by environmental conditions. Proc. R. Soc. B 279, 876–883. doi: 10.1098/rspb.2011.1351
Leveau, L. M. (2019). Primary productivity and habitat diversity predict bird species richness and composition along urban-rural gradients of Central Argentina. Urban For. Urban Green. 43:126349. doi: 10.1016/j.ufug.2019.05.011
Lindström, Å. (1990). The role of predation risk in stopover habitat selection in migrating bramblings, Fringilla montifringilla. Behav. Ecol. 1, 102–106. doi: 10.1093/beheco/1.2.102
Londe, D., Cady, S., Elmore, R. D., and Fuhlendorf, S. D. (2022). Woody plant encroachment pervasive across three socially and ecologically diverse ecoregions. Ecol. Soc. 27:11. doi: 10.5751/ES-13348-270311
Mawdsley, J. R., O’Malley, R., and Ojima, D. S. (2009). A review of climate-change adaptation strategies for wildlife management and biodiversity conservation. Conserv. Biol. 23, 1080–1089. doi: 10.1111/j.1523-1739.2009.01264.x
McCabe, B. J., and Gugleilmo, C. G. (2019). Migration takes extra guts for juvenile songbirds: energetics and digestive physiology during the first journey. Front. Ecol. Evol. 7:381. doi: 10.3389/fevo.2019.00381
Monson, G. (1997). “A concentration of Dickcissels in Sinaloa, Mexico” in The era of Allan R. Phillips: A festschrift. ed. R. W. Dickerman (Albuquerque, NM: Horizon Communications), 83–85.
Munro, U., Munro, J. A., Phillips, J. B., Wiltschko, R., and Wiltschko, W. (1997). Evidence for a magnetite-based navigational map in birds. Naturwissenschaften 84, 26–28. doi: 10.1007/s001140050343
Netwon, I. (2007). Weather-related mass-mortality events in migrants. Ibis 149, 453–467. doi: 10.1111/j.1474-919X.2007.00704.x
Norris, D. R., and Marra, P. P. (2007). Seasonal interactions, habitat quality, and population dynamics in migratory birds. Condor 109, 535–547. doi: 10.1093/condor/109.3.535
Oppel, S., Dobrev, V., Arkumarev, V., Saravia, V., Bounas, A., Kret, E., et al. (2015). High juvenile mortality during migration in a declining population of a long-distance migratory raptor. Ibis 157, 545–557. doi: 10.1111/ibi.12258
Orians, G. N., and Paulson, D. R. (1969). Notes on costa Rican birds. Condor 71, 426–431. doi: 10.2307/1365741
Osborne, D. C., and Sparling, D. W. (2013). Multi-scale associations of grassland birds in response to cost-share management of conservation reserve program fields in Illinois. J. Wildl. Manag. 77, 920–930. doi: 10.1002/jwmg.553
Packard, S., and Mutel, C. F. (1997). The Tallgrass Restoration Handbook. Island Press, Washington, DC: Island Press.
Parrish, J. D. (2000). Behavioral, energetic, and conservation implications of foraging plasticity during migration. Stud. Avian Biol. 20, 52–70.
Phillips, S. J., Anderson, R. P., and Schapire, R. E. (2006). Maximum entropy modeling of species geographic distributions. Ecol. Model. 190, 231–259. doi: 10.1016/j.ecolmodel.2005.03.026
Reiley, B. M., and Benson, T. J. (2019). Differential effects of landscape composition and patch size on avian habitat use of restored fields in agriculturally fragmented landscapes. Agric. Ecosyst. Environ. 274, 41–51. doi: 10.1016/j.agee.2018.12.017
Renfrew, R. B., Kim, D., Perlut, N., Smith, J., Fox, J., and Marra, P. P. (2013). Phenological matching across hemispheres in a long-distance migratory bird. Divers. Distrib. 19, 1008–1019. doi: 10.1111/ddi.12080
Reside, A. E., Van DerWal, J. J., Kutt, A. S., and Perkins, G. C. (2010). Weather, not climate, defines distributions of vagile bird species. PLoS One 5:e13569. doi: 10.1371/journal.pone.0013569
Rickletts, T. H., Dinerstein, E., Olsen, D. M., Loucks, C. J., Eichbaum, W., DellaSala, D. A., et al. (1999). Terrestrial ecoregions of North America. Washington, DC, and Covello, CA: Island Press.
Rosenberg, K. V., Dokter, A. M., Blancher, P. J., Sauer, J. R., Smith, A. C., Smith, P. A., et al. (2019). Decline of the north American avifauna. Science 366, 120–124. doi: 10.1126/science.aaw1313
Rosenberg, K. V., Kennedy, J. A., Dettmers, R., Ford, R. P., Reynolds, D., Alexander, J. D., et al. (2016). Partners in Flight Landbird Conservation Plan: 2016 revision for Canada and continental United States. McAllen, TX: Partners in Flight Science Committee.
Rotics, S., Kaatz, M., Resheff, Y. S., Turjeman, S. F., Zurell, D., Sapir, N., et al. (2016). The challenges of the first migration: movement and behaviour of juvenile vs. adult white storks with insights regarding juvenile mortality. J. Anim. Ecol. 85, 938–947. doi: 10.1111/1365-2656.12525
Samson, F. B., Knopf, F. L., and Ostlie, W. R. (2004). Great Plains ecosystems: past, present, and future. Wildl. Soc. Bull. 32, 6–15. doi: 10.2193/0091-7648(2004)32[6:GPEPPA]2.0.CO;2
Sauer, J. R., Hines, J. E., and Fallon, J. (2020). The North American Breeding Bird Survey, Results and Analysis 1966–2019. U.S. Geological Survey data release.
Scott, I., Mitchell, P. I., and Evans, P. R. (1994). Seasonal changes in body mass, body composition, and food requirements in wild migratory birds. Proc. Nutr. Soc. 53, 521–531. doi: 10.1079/PNS19940062
Sillett, T. S., and Holmes, R. T. (2002). Variation in survivorship of a migratory songbird throughout its annual cycle. J. Anim. Ecol. 71, 296–308. doi: 10.1046/j.1365-2656.2002.00599.x
Smith, R. J., Hamas, M., Dallman, M., and Ewert, D. (1998). Spatial variation in foraging of the black-throated green warbler along the shoreline of northern Lake Huron. Condor 100, 474–484. doi: 10.2307/1369713
Smith, R. J., Moore, F. R., and May, C. A. (2007). Stopover habitat along the shoreline of northern Lake Huron, Michigan: emergent aquatic insects as a food resource for spring migrating landbirds. Auk 124, 107–121. doi: 10.1093/auk/124.1.107
Sorensen, M. C., Hipfner, J. M., Kyser, T. K., and Norris, D. R. (2009). Carry-over effects in a Pacific seabird: stable isotope evidence that pre-breeding diet quality influences reproductive success. J. Anim. Ecol. 78, 460–467. doi: 10.1111/j.1365-2656.2008.01492.x
Sousa, B. F., Temple, S. A., and Basili, G. D. (2022). “Dickcissel (Spiza americana), version 2.0” in Birds of the world. eds. T. S. Schulenberg and B. K. Keeney (Ithica, NY: Cornell Lab of Ornithology)
Thompson, S. J., Arnold, T. W., and Amundson, C. L. (2014). A multiscale assessment of tree avoidance by prairie birds. Condor 116, 303–315. doi: 10.1650/CONDOR-13-072.1
Tuanmu, M.-N., and Jetz, W. (2014). A global 1-km consensus land-cover product for biodiversity and ecosystem modelling. Glob. Ecol. Biogeogr. 23, 1031–1045. doi: 10.1111/geb.12182
Van DerWal, J., Shoo, L. P., Graham, C., and Williams, S. E. (2009). Selecting pseudo-absence data for presence-only distribution modeling: how far should you stray from what you know? Ecol. Model. 220, 589–594. doi: 10.1016/j.ecolmodel.2008.11.010
Webster, J. R., and Benfield, E. F. (1986). Vascular plant breakdown in freshwater ecosystems. Annu. Rev. Ecol. Evol. Syst. 17, 567–594. doi: 10.1146/annurev.es.17.110186.003031
Welti, E. A. R., Prather, R. M., Sanders, N. J., de Beurs, K. M., and Kaspari, M. (2020). Bottom-up when it is not top-down: predators and plants control biomass of grassland arthropods. J. Anim. Ecol. 89, 1286–1294. doi: 10.1111/1365-2656.13191
Keywords: Dickcissel, habitat suitability, migration, species distribution modeling, Spiza americana
Citation: Glass A, Eichholz MW and Brown JL (2023) Habitat suitability for Dickcissels (Spiza americana) during spring and fall migration: A species distribution modeling approach. Front. Ecol. Evol. 10:1095188. doi: 10.3389/fevo.2022.1095188
Edited by:
Purabi Saikia, Central University of Jharkhand, IndiaReviewed by:
Gianpasquale Chiatante, University of Pavia, ItalyDibyendu Adhikari, National Botanical Research Institute (CSIR), India
Copyright © 2023 Glass, Eichholz and Brown. This is an open-access article distributed under the terms of the Creative Commons Attribution License (CC BY). The use, distribution or reproduction in other forums is permitted, provided the original author(s) and the copyright owner(s) are credited and that the original publication in this journal is cited, in accordance with accepted academic practice. No use, distribution or reproduction is permitted which does not comply with these terms.
*Correspondence: Alex Glass, alex.glass@birdpartners.org