- 1Department of Biology, University of Texas Rio Grande Valley, Edinburg, TX, United States
- 2Department of Entomology, University of California Riverside, Riverside, CA, United States
- 3School of Earth, Environmental and Marine Sciences, University of Texas Rio Grande Valley, Edinburg, TX, United States
- 4Department of Entomology and Plant Pathology, University of Arkansas, Fayetteville, AR, United States
Plants possess physical and chemical defenses which have been found to deter herbivores that feed and oviposit on them. Despite having wide variety of defenses which can be constitutive or induced, plants are attacked and damaged by insects associated with different mouthparts and feeding habits. Since these defenses are costly, trade-offs for growth and defense traits play an important role in warding off the herbivores, with consequences for plant and herbivore growth, development and fitness. Solanum is a diverse and rich genus comprising of over 1,500 species with economic and ecological importance. Although a large number of studies on Solanum species with different herbivores have been carried out to understand plant defenses and herbivore counter defenses, they have primarily focused on pairwise interactions, and a few species of economic and ecological importance. Therefore, a detailed and updated understanding of the integrated defense system (sum of total defenses and trade-offs) is still lacking. Through this review, we take a closer look at the most common plant defense hypotheses, their assumptions and trade-offs and also a comprehensive evaluation of studies that use the genus Solanum as their host plant, and their generalist and specialist herbivores from different feeding guilds. Overall, review emphasizes on using ubiquitous Solanum genus and working toward building an integrated model which can predict defense-fitness-trade-offs in various systems with maximum accuracy and minimum deviations from realistic results.
Background
To protect against herbivores that constantly attack them, plants have evolved defenses that have direct detrimental effects on herbivores affecting their movement, growth, development, feeding, oviposition (Kessler and Baldwin, 2001; Rostás et al., 2003). Plants also harm herbivores indirectly by attracting their natural enemies such as predators and parasitoids (Gols et al., 2008). Both direct and indirect defenses can be constitutive and/or induced (Karban et al., 1997). Constitutive defenses are present regardless of herbivory pressure and consist of surface wax, cuticle, trichomes, and other physical barriers or chemical defenses which deter the establishment of insect pests or affects its growth and development (Gatehouse, 2002). On the other hand, induced defenses are activated as the response to pest attack, which can enhance the plant resistance to the further attack of that insect pest and/or for pests possessing different feeding guilds (Karban and Myers, 1989). Physical defenses, also called structural or mechanical defenses, are the structural modifications that plants possess to deter herbivory. These are also the first line of defense owned by plants which comprise spines, thorns, trichomes and plant waxes. Spines are sharply pointed modified petioles, midribs, veins or/and stipules and provide protection to the plants against herbivory (Hanley et al., 2007; Kariyat et al., 2017). Trichomes are the hair like protuberances from different parts of the plants. These have defensive function against herbivory by acting as a physical barrier, impeding insect movement, delaying or/and deterring herbivore feeding and oviposition, thus making them exposed longer and vulnerable to its predators (Levin, 1973; Dalin et al., 2008; Peiffer et al., 2009; Kaur J. and Kariyat R. R., 2020). Chemical defenses are chemical compounds which are stored in the plant tissues (constitutive) or induced in response to herbivory (induced; Ryan, 1990; Turlings and Benrey, 1998; Sun et al., 2019). These include but are not limited to volatile organic compounds, salicylic, ethylene and jasmonate pathways and various other primary and secondary metabolites including antibiotics, alkaloids, and terpenes, to name a few (Ryan, 1990; Kessler and Baldwin, 2001; Rivard et al., 2004; Howe and Jander, 2008; Degenhardt et al., 2010; Singh et al., 2021). Besides constitutive and induced defenses of plants, tri-trophic interactions of host plants with parasitoids and predators of herbivores also provides them with an additional line of defense. Sometimes the feeding damage done by herbivores to host plants invites herbivore’s natural enemies. Plants release volatiles to attract natural enemies such as predatory mite, Phytoseiulus persimilis (Mesostigmata: Phytoseiidae) as seen in case of tomato plants, Solanum esculentum (Solanaceae) when they are fed by two-spotted spider mite, Tetranychus urticae (Trombidiformes: Tetranychidae; Kennedy, 2003).
Although plants have evolved an astonishing variety of defenses that include physical and chemical defenses, that could be constitutive or induced. Insect herbivores still feed extensively on plants causing continuous damage affecting their fitness. Over a long period of evolutionary history, insect herbivores have evolved a wide array of traits including modifications to their feeding habits and mouth parts. Additionally, to continue their predatory relationship with plants, they also possess elaborate mechanisms and behavioral modifications to avoid, process, sequester, or excrete plant defenses and defensive compounds allowing them to initiate feeding. For instance, herbivores such as caterpillars and beetles having chewing and biting type mouth parts feed on leaves and cause significant photosynthetic tissue loss (Rowell and Simpson, 1992; Wise, 2007). Sucking and piercing type herbivores such as true bugs and aphids. Feeding by sucking and piercing type herbivores such as true bugs and aphids, not only lead to loss of photosynthates from vascular tissues, but also transmit viral diseases (Wisler et al., 2007; Ishikawa and Takahata, 2019). The tiger wing butterfly, Mechanitis isthmia (Lepidoptera: Nymphalidae) have the cooperative feeding and webbing behavior on solanaceous plants covered with dense trichomes as a behavioral adaptation to evade defenses. The young larvae of these butterflies shave the trichomes to feed on leaf tissues and construct silken webs to avoid their piercing by trichomes (Young and Moffett, 1979). Tobacco hornworm, Manduca sexta (Lepidoptera: Sphingidae) feeding on tobacco, Nicotiana spp. (Solanaceae) is adapted to feed and efficiently excrete nicotine, a pyridine alkaloid possessing insecticide properties. Monarch butterfly, Danaus plexippus (Lepidoptera: Nymphalidae) is adapted to feed on, sequester and use toxic cardenolides in milkweed, Asclepias sp. (Apocynaceae) to protect itself against predators (Malcolm, 1994). For more specific details on Solanum herbivores and their feeding habits, see Supplementary Table 1.
Since plant defenses are costly (Baldwin, 1998; Kariyat et al., 2013; Yip et al., 2019; Garcia et al., 2021) taking up already limited resources originally allocated for their growth and reproduction, plants are found to exhibit trade-offs for growth, reproduction, and defense traits (Bazzaz et al., 1987; Kariyat et al., 2013). In general, available plant resources and their sources, i.e., leaves and roots determine the plant growth, commonly measured as the biomass gain over time. This gain in biomass is the result of conversion of primary metabolites into cellular building blocks (Shih, 2018). Where herbivory has a quantifiable negative impact, it leads to the redirection of this energy and matter from growth to defense traits and hence the trade-offs for growth and reproduction comes into play. For natural and unmanaged systems, in which the cost of defense has major effects on the evolutionary trajectory of the species, these trade-offs can have both short- and long-term effects on fitness. However, one of the true measures of plant fitness—the number of successful offspring it can produce in future generations is difficult to measure in such systems, when compared to the agro-ecosystems, where seed set or general plant growth/ biomass can be used as a measure of its fitness and thus, easier to measure (Stireman et al., 2005). To understand the mechanisms governing plant defense trade-offs in both natural and managed ecosystems, with both pairwise and multi-trophic interactions involving biotic and abiotic factors, various hypotheses and theories have been put forward and tested. In this review, we revisit some of the major theories and use Solanum genus as a model to explain the assumptions and trade-off traits, providing suggestions for additional lines of inquiries.
Solanum—The study model
Solanaceae is a diverse plant family consisting of ~90 genera and ~2,700 species which are found in all habitats ranging from dry deserts to wet tropical rainforest and have growth habits ranging from small ephemeral herbs to large perennial trees (Gebhardt, 2016). Among all the genera in Solanaceae, Solanum genus contributes for over 1,500 species in the family (Tchatchouang et al., 2017). This diverse group can be a good study model as it contains economically important food crops such as potato (Solanum tuberosum), eggplant (S. melongena) and cultivated tomato (S. lycopersicum); drug plants including bittersweet nightshade (S. dulcamara), dutch eggplant (S. aculeatissimum) and New Zealand eggplant (S. aviculare); ecologically important weeds including horsenettle (S. carolinense), silverleaf nightshade (S. elaeagnifolium), earleaf nightshade (S. mauritianum), black nightshade (S. nigrum) and American black nightshade (S. americanum); annuals including hairy nightshade (S. sarrachoides) and west Indian nightshade (S. ptychanthum); perennials including horsenettle (S. carolinense) and silverleaf nightshade (S. elaeagnifolium); self-compatible species including pepino (S. muricatum), hairy nightshade (S. villosum); self-incompatible including the ornamental nightshade (S. bulbocastanum) and jasmine nightshade (S. jasminoides; Symon, 1981; Rushing et al., 1985; Blackshaw, 1991; Hermanutz and Weaver, 1996), and species with plasticity in self-incompatibility (S. carolinense, S. elaeagnifolium; Kariyat et al., 2012a). In addition to this, sequenced genomes of species such as tomato (S. lycopersicum) and potato (S. tuberosum; The Potato Genome Sequencing Consortium, 2011; The Tomato Genome Consortium, 2012) makes the genus amenable for genome wide molecular work for conspecifics (Kariyat et al., 2012b).
The Solanum defense phenotype
The high species count, and a wide range of growth habits have led the genus Solanum to evolve tremendous variation in defense traits. The species in the Solanum genus have both constitutive and induced, physical and chemical defenses against herbivory that show variation both inter and intraspecifically, with consequences for trophic interactions. Structural defenses in Solanum mainly consist of trichomes, spines and waxes. An enormous variation in morphology, types, density and dimensions have been reported for trichomes of Solanum (Anjana et al., 2008; Howe and Jander, 2008; Kim et al., 2012; Cho et al., 2017; Kariyat et al., 2018; Watts and Kariyat, 2021a, 2022), with variation for density and types on abaxial and adaxial surfaces, with functional consequences for herbivores (Malakar and Tingey, 2000; Horgan et al., 2007; Kariyat et al., 2013; Kaur I. and Kariyat R. R., 2020; Watts and Kariyat, 2021b), a similar trend of interspecific variation and anti-herbivore defenses functions have also been found for spines (Kariyat et al., 2017), and epicuticular wax (Watts and Kariyat, 2022). Moving past the structural defense barrier in Solanum, the herbivores are then exposed to a gamut of chemical defenses starting from the leaf surface messengers, Ca2+ and Reactive Oxygen Species (ROS). For example., study in tomato have demonstrated that, the oral secretions of tobacco hornworm caterpillars induce ROS, which are then suppressed in the presence of a Ca2+ chelator, suggesting herbivore-associated elicitor induced signaling pathway (Gandhi et al., 2020; further reviewed in detail in Gandhi et al., 2021). Along with oral secretions, excreta or oviposition fluids are also known to act as chemical cues perceived by transmembrane pore proteins in Solanum (Gandhi et al., 2021). More interestingly, the plants of Solanum carolinense has shown trade-offs between constitutive and induced defenses (Kariyat et al., 2013) where the insect suppression reduced the induced resistance significantly more than the constitutive defenses (Coverdale and Agrawal, 2022). The intraspecific variation in Solanum genus also can also lead to differential chemical defense induction (Campbell et al., 2013; Calf et al., 2018; Müller et al., 2020) in herbivores with similar feeding habits. Tomato plants when fed by the corn worm, Helicoverpa zea (Lepidoptera: Noctuidae) altered more metabolites than feeding by tobacco hornworm, and that includes defense related metabolites such as phenolics and precursors of amino acids (Steinbrenner et al., 2011). Also, the alteration and reallocation of primary metabolites and the differential induction of metabolites in different leaves have been documented where tomato plants when damaged by tobacco cutworm, Spodoptera litura (Lepidoptera: Noctuidae) resulted into reallocation of primary metabolites like sugars and amino acids from local to systemic leaves. Differential defense related response was observed as local leaves increased lignin accumulation, and systemic leaves had significant induction of secondary metabolites including chlorogenic acid, caffeic acid and catechin (Kundu et al., 2018). While hormonal signaling pathways and the gene networks (Kariyat et al., 2012b) that mediate them warrants a stand-alone review, in a nutshell—the phytohormone Jasmonic acid is found to play a very important role in plant defense against herbivores (Howe and Jander, 2008). When chewing herbivores damage plants, it leads to activation of Jasmonic acid signaling pathway that leads to both direct toxin induction and also indirect defenses through the induced emission of various herbivore induced plant volatiles (HIPV’s), which can act as antifeedants and also as cues for predators or parasitoids (Kariyat et al., 2012a,b). These defensive volatiles can be produced either locally or systemically and can be flavonoids, terpenoids and alkaloids (Wasternack and Parthier, 1997). For example, on attack by Tuta absoluta, the tomato plants emit volatiles, induced by jasmonic acid which as a result attracts mirid predators (Silva et al., 2018). To mitigate the omnipresent impact of herbivory, plants use trade-offs, and in Solanum, the trade-offs vary by species, breeding status and genetic variation of plants (inbred vs. outbred, maternal families), plant characteristics, herbivore, and environmental conditions as well.
Taken together, we have innumerable studies of plant defenses (Wilkens et al., 1996; Lawrence et al., 2008; Alba et al., 2009; Horgan et al., 2009; Kaplan et al., 2009; Rodríguez-López et al., 2011; Tian et al., 2014; Kundu et al., 2018; Kariyat et al., 2012a,b, 2017, 2018, 2019; Taher et al., 2019; Kaur I. and Kariyat R. R., 2020; Kaur J. and Kariyat R. R., 2020) and trade-offs (Wise and Sacchi, 1996; Cipollini et al., 2004; Walls et al., 2005; Wise et al., 2008; Underwood and Halpern, 2012; Kariyat et al., 2012a,b, 2013) possessed by Solanum plants in case of their interactions with herbivores. However, how defenses and trade-offs function simultaneously in plants to ward-off herbivores has not been recently discussed at length. To address this, in this review, we used Solanum genus as a model to re-explore trade-offs for defenses by plants infested by herbivores varying in their feeding habits and specialization on host plants.
Major hypotheses in plant defenses
Growth-differential balance hypothesis (GDBH)
Proposed by Loomis (1953), this hypothesis states that plant defenses are a result of a trade-off between ‘growth-related processes’ and ‘differentiation-related processes’ in different environments. Growth related processes refers to cell division and enlargement, and differentiation related processes refers to changes in cells leading to their maturation and specialization. For example, in case of interaction of pine trees (Pinaceae) with southern pine beetle, Dendroctonus frontalis (Coleoptera: Curculionidae), spring was found favorable for the beetles to attack their host when most of photosynthates are used for growth-related process of the trees. However, in summer, resin flow (as a result of differentiation) increases and provides resistance to beetles to attack the host (Lorio, 1986). Herms and Mattson (1992) also stated that the plants are in dilemma of increasing their growth to the fullest (growth process), and to maintain defenses (differentiation process) against herbivores and pathogens during the growth period to ensure their survival. The availability of resources also play role in the growth-differential balance. This was supported in the tomato plants (Solanaceae) when the allocation of resources to defense related characters and growth-related characters was evaluated over different levels of resource availability. With low resource allocation, there was low sugar phenolics and plant biomass. With intermediate number of resources, plants had high phenolics but lower growth. In contrary, with high resource availability, plants had high biomass but very low phenolics concentration (Wilkens et al., 1996). This idea was also supported by (Massad et al., 2012) through finding a negative relationship between Flavans, a class of chemical defenses (differentiation process) and biomass accumulation (growth process) in Pentaclethra macroloba (Fabaceae), but only in resource abundant environment. However, Wilkens et al. (1996) conducted an experiment on tomato plants by providing high and low levels of water and light, and contrary to the hypothesis found that the intermediate resource available plants exhibited more growth relative to high resource available plants.
Stamp (2004) states that GDBH is most mature hypothesis of all hypotheses. Although Herms and Mattson (1992) described the framework to test it, Stamp (2004) elaborated it cannot be tested directly and rigorously as in case of most of the other hypotheses. In case it must be tested, considerable efforts and methodology setup is required, which makes extraction of data to validate and test this hypothesis much harder than the other hypotheses. This hypothesis was developed from empirical data of plants growing mostly in temperate systems, and thus whether it is applicable and justifies growth-differentiation in hot and tropical climates is questionable. Hattas et al. (2017) conducted a study on deciduous savanna woody species Combretum apiculatum to explore the fashion of secondary metabolite allocation and whether it is along the line stated by growth-differentiation hypothesis. GDBH could not explain the secondary metabolite allocation in this species, but carbon nutrient balance hypothesis (stated below) could partially explain the allocation of few secondary metabolites.
Another study was conducted by Heckman (2011) to compare and contrast GDBH and optimal defense theory (stated below) with tissue palatability by two different herbivores on seaweeds at two locations in Caribbean Island. GDBH could not predict herbivore relative palatability and thus, was concluded a poor predictor. However, optimal defense hypothesis supported the feeding pattern of herbivores, however it was also concluded a poor predictor. Overall, there are multiple studies which could not agree with GDBH (Barto and Cipollini, 2005; Heckman, 2011; Hattas et al., 2017) and there is much difficulty associated with testing it which makes it unfavorable to be studied in more plant-herbivore systems.
Plant apparency hypothesis (PAH)
Feeny (1976) proposed Plant Apparency Hypothesis stating that the plant will invest more in broadly effective defenses when plants are easily found or are more “apparent” to the herbivores. For example, long lived trees and perennials are present throughout the year, are more apparent to their herbivores and thus invest more in their defenses. On the other hand, short-lived plants and annuals are less apparent plants and thus invest less in defenses and that too against most specialized herbivores. The apparent plants will either develop chemical defenses which are quantitative, or which are digestibility reducers like tannins, and the non-apparent ones will have more of qualitative defenses such as glucosinolates and alkaloids (Rhoades and Cates, 1976). For example, Wiklund (1984) found that the ovipositing females tend to perceive plants with big and colorful flowers as compared to less apparent plants having small flowers. Begon (1984) differentiated the species into r-strategists (high reproduction capacity) and k-strategists (less rate of reproduction but high survival potential) and Coley et al. (1985) suggests that the r-strategist species are the ones that expand quickly and have a brief life cycle, invest in the quality (mobile defenses, which are qualitative defenses and present in low concentrations) of defense compounds rather than the quantity (immobile defenses, found in high concentrations and are inactive metabolically). Coley et al. (1985) also proposed the idea that weeds and herbs contain very toxic low-molecular weight compounds, as these plants are short-lived and hence invest on qualitative compounds. The role of apparency theory was also proposed in accessing the selection of the medicinal herbs by local people (Stepp and Moerman, 2001). De Almeida et al. (2005) on the other hand, when tested the apparency theory on various medicinal plants, found that the plants categorized into k-strategists contained high amounts of quantitative compounds as proposed in the apparency hypothesis.
There are some more studies which contradict with this hypothesis. For example, tannic acid, which was believed to be an important quantitative defense (apparent plants), was reported to fail to inhibit digestion of protein in tobacco hornworm caterpillars (Martin et al., 1987). In some cases, the plants belonging to the same apparency levels are known to have different levels of defenses. Smilanich et al. (2016) did a meta-analysis to conclude whether plant-apparency matters for herbivores and kind of defenses against them. By taking into account 158 published papers, they could conclude that quantitative defenses are majorly present in woody plants and qualitative defenses are primarily present in herbaceous plants, however, quantitative defenses were more impactful against specialist herbivores and qualitative defenses did not differentiate between specialist or generalist herbivores for both apparent and non-apparent plants. Moreover, difficulty to measure apparency as well as the failure of application of this theory to plant species falling into extreme ends of apparency has restricted the further studies in importance of this hypothesis.
Optimal defense theory (ODT)
Mckey (1979) proposed Optimal Defense Theory stating a few principles of resource allocation. Firstly, the plant parts unlikely (roots) to be attacked by herbivores have low constitutive and more induced defenses, and plant parts likely (leaves and reproductive parts) to be attacked by herbivores have more constitutive and less induced defenses. And secondly, plant resources are allocated to the more valuable plant parts which are more exposed to the damage by herbivores. This theory has been found consistent with practical observations in various cases (Keith and Mitchell-Olds, 2017) together with the one done by (Zangerl and Rutledge, 1996) in which artificial damage was done to various plant parts of wild parsnip, Pastinaca sativa (Apiaceae), and the constitutive defenses were highest in leaves and reproductive parts, as these two plant parts were most attacked by herbivores and induced defenses were highest in case of roots, which were least attacked by herbivores. Similarly, Ohnmeiss and Baldwin (2000) had observations consistent with the ODT, but in relation to fitness values of the tissues. Leaves when removed from the bolting stage of plants caused highest fitness ruinous and these leaves were found to have higher constitutive and induced nicotine compared to the leaves of vegetative or flowering plants. Higher constitutive defenses were found consistent with the theory, but higher induced defenses were not. The ODT theory was very appealing to the scientists as it provided early framework to test the cost of defense as well as the genetic expression of plant defense. A meta-analysis done by McCall and Fordyce (2010) also concluded that it can be generally agreed that the chemical defenses are present in higher amounts in the plant parts that can be considered to be of higher value than the other ones.
Along with some studies not completely agreeing with ODT (Barto and Cipollini, 2005; Heckman, 2011), this theory always remained difficult to test due to the difficulty in testing costs of fitness directly. Besides, Pavia et al. (2002) suggested to not to rely completely on originally proposed theories, but to study life history variation among species and populations along with demographic elasticity analysis, and to use it as a tool to put value on fitness on different plant parts. This can further be used to make assumptions and to test those assumptions. Further, in realistic conditions, it can be hard to assign some part of plant as more valuable than the other. This adds another layer of complication to understanding and justifying this theory when response of plants if studied with multiple stresses act on the plants at the same time, especially when the stresses trigger different spatially separate contrasting response pathways in plants. For example, pathogens trigger the immune responses, but abiotic stresses trigger physiological and molecular changes. Thus, Wolinska and Berens (2019) suggested that plant responses should be studied in response to ‘combined stresses’ as seen in nature rather than plant response to just one stress at a time.
Carbon: Nutrient balance hypothesis/environmental constraint hypothesis/carbon nutrient balance model (CNBH)
Bryant et al. (1983) and Tuomi et al. (1988) proposed this hypothesis in which they stated that plants tend to provide defenses against herbivory by aligning the carbon: nitrogen ratio in the environment in such a way that plants growing in nutrient deficient (especially N) soils tend to produce carbon-based defenses and those growing in C deficient (shade, CO2) environment tend to produce N based defenses. Low nutrient adapted plants have slow growth rates and thus respond to herbivory by increased production of thorns (Muckadell, 1962), repellent secondary metabolites and carbon-based defenses such as tannins and terpenes (Mckey, 1979), and carbon deficient plants tend to escape herbivory by rapid growth and accumulating compounds such as alkaloids, cyanogenic glycosides and various other N based compounds. Moreover, in shaded conditions, the leaf palatability tends to increase, due to decrease of carbon-based secondary metabolites (Bryant et al., 1983). Many studies have conducted to test this hypothesis by altering the levels of fertilization as well as giving differential shade and sun conditions. For example, in Ilex vomitoria, the predictions of this hypothesis were supported when the levels of alkaloids were increased many times on application of ammonium nitrate (Palumbo et al., 2007). A study by Price et al. (1989) in which they looked at intraspecific variation of phytochemicals with carbon: nitrogen variation in arroyo willow, Salix lasiolepis (Salicaceae) strongly supported carbon nutrient balance model. However, a strong contradiction of this hypothesis is found in Solanum viarum, where the beetle Gratiana boliviana, was found to cause more damage to the plants growing in full sun as compared to the plants growing in shaded conditions (Kariuki et al., 2016). Most of the studies testing this hypothesis have relied on testing on a few nitrogenous and non-nitrogenous secondary metabolites and not the total metabolites. Along with this, it is also difficult to explain the rapid induction of defense in response to the herbivore damage with the help of carbon nutrient shifts (Stamp, 2003).
There are some studies in which CNBH only partially explains the changes in defense related metabolites. For example, Massad et al. (2012) grew oil bean tree, Pentaclethra macroloba (Fabaceae) under three levels of nitrogen, and trade-offs related to production of saponins and flavans (two classes of defense molecules) were examined by quantifying physiological costs of plant defenses by measuring photosynthetic parameters and plant biomass. They found that flavans declined with nitrogen but saponins increased, thus findings were partially in favor of CNBH.
Although the tests of CNBH are not always in favor of the model predictions (Fajer et al., 1992), Lerdau and Coley (2002) hold an opinion that CNBH is still a useful model for ecological research involving resource allocation as response variable and considering large pool of compounds and biosynthetic pathways of secondary metabolites. However, a comprehensive study by Hamilton et al. (2001) and a strong opinion by Koricheva (2002) lead to almost total rejection and failure of CNBH.
Resource availability hypothesis (RAH)
Coley et al. (1985) proposed Resource Availability Hypothesis which revealed that defenses provided by plants against herbivores depend on the inherent growth rates of plant, which in turn, will depends on the resource availability to the plant. The hypothesis predicts that the species adapted to resource rich environments have faster growth, shorter lifetimes, lower amounts of constitutive defenses and higher inducible defenses. The plant species adapted to resource poor environments have slower growth, longer lifetimes and invest higher in constitutive defenses (Endara and Coley, 2011). Many studies strongly support this hypothesis. A few of those are: An experiment was performed by Fine and Mesones (2011), in which 20 plant species among which some were clay (nutrient rich) specialists and others were white sand (nutrient deficient) specialists, were grown in clay soil and white sand, both with and without herbivores. The clay specialists performed better in both soil types when there were no herbivores, but white sand specialists dominated in white sand and clay specialists dominated in clay soils when herbivores were present, indicating that the white sand specialists did not respond to the protection provided, presumably because of higher constitutive defenses against herbivores. Also, chemical analysis revealed significantly higher tannin: protein ratio in white sand specialists than clay specialists. In tomato cultivars, low levels of phenolics were found to be produced in plants applied with less concentrations of potassium nitrate as compared to the ones with intermediate and high concentrations of fertilizer, showing consistency with growth rate hypothesis (Wilkens et al., 1996). Other few studies conducted in different habitats and with different host plants were also in favor of RAH (Bryant et al., 1983). Massey et al. (2007) also confirmed RAH when tested the 18 grass species for their growth and defense rate and found a negative relationship between them. Endara and Coley (2011) also confirmed through a comprehensive study that RAH can serve as a reliable and a robust concept for predicting plant growth and defense trade-offs. Because of such a high support from empirical data over the years, RAH is considered as the most successful theory to explain and predict growth-defense trade-offs in plants (Gianoli and Salgado-Luarte, 2017; López-Goldar et al., 2020).
Another lesser tested Protein Competition Model (PCM; Jones and Hartley, 1999) hypothesizes the allocation and concentration of total phenols in higher terrestrial plants for slow vs. fast growing species as resource availability changes (Jones and Hartley, 1999). The prediction of PCM fit generally observed prediction and thus, it arguable compliments GDBH and RAH.
With many strongly supporting studies, there are some studies who rejected RAH based on their prediction and results (listed in Endara and Coley, 2011). One of those studies was done by Hernán et al. (2019) in which intra-specific variation in resource availability and plant defenses was examined. RAH has opposite patterns when it comes to intraspecific variations, as seen in some studies (Lamarre et al., 2012; Hahn and Maron, 2016). However, Hernán et al. (2019) found the results as predicted in interspecific examination. This emphasizes the need to better understand RAH, especially for intra-specific variations. Further, López-Goldar et al. (2020) found the predictions of RAH intraspecifically and found a strong positive relationship between resource availability and constitutive defenses, however, no trade-off between constitutive and inducible defenses was found.
Conclusion
As discussed in this review, plant growth defense trade-offs are well illustrated by the diverse members of Solanum genus defending against even more diverse community of insect herbivores (Supplementary Tables 1, 2). There is always a balance that plants strike between growth and defense, governed by maintenance of optimal fitness. There is a wide array of plant defense mechanisms against the herbivores belonging to different types of feeding guilds (Supplementary Table 1), and hence the trade-offs associated with them are also very diverse. The chewing type herbivores induce JA pathways and other related defenses (Figure 1; Redman et al., 2001), but the sap sucking herbivores are known to induce SA pathway defenses (Figure 1; Tan and Liu, 2014). Owing to the active research on the costs of plant defenses, our understanding of the mechanisms governing these trade-offs has been enhanced in past few decades. However, our knowledge is still scant on the economics of cost vs. quality in terms of defense and growth, and there are several issues which still need to be addressed.
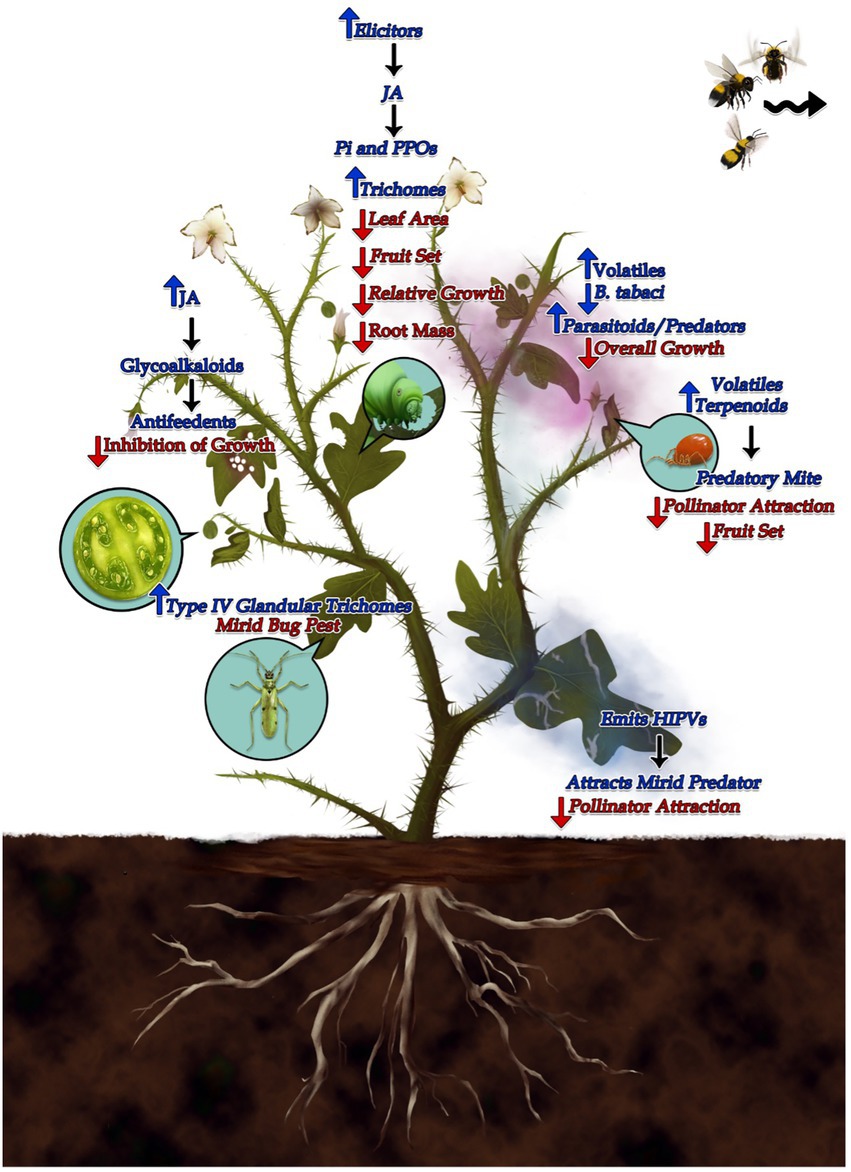
Figure 1. Schematic representation of the plant defense mechanisms to the herbivores belonging to different types of feeding guilds and the trade-offs associated with them. As the plant defenses are expensive, plants display a balance between the allocation of their limited resources for growth and defense. Plants under attack of leaf chewing herbivores generally lead to activation of Jasmonic acid signaling pathways which then emits various Herbivore induced plant volatiles (HIPV’s), which can act as antifeedants and also can act as cues for predators or parasitoids. These defensive volatiles can be produced either locally or systemically and can be flavonoids, terpenoids and alkaloids (Wasternack and Parthier, 1997). For instance, plants of family Solanaceae secrete Glycoalkaloids on infested by Leptinotarsa decemlineata, which acts as antifeedant for these herbivores (Pelletier and Tai, 2001). Tomato plants infested by leaf minor, Tuta absoluta emits Herbivore induced volatiles (HIPV) induced by Jasmonic acid, which attracts three mirid predators, Macrolophus basicornis (Stal), Engytatus varians (Distant), and Campyloneuropsis infumatus (Carvalho; Silva et al., 2018). In another example, on infestation by two-spotted spider mite, Tetranychus urticae, the tomato plants emitted volatile tarpenoids which attracted the predatory mite, Phytoseiulus persimilis (Kant et al., 2004). The defenses induced by Jasmonic acid, comes with a cost. Plants on attack by Manduca sexta, increased the levels of JA induced defense chemicals namely, polyphenol oxidase and peroxidase, however it leads to lower fruit set as well as lesser seed set (Redman et al., 2001). Likewise, plants of Solanum carolinense, when treated with Jasmonic Acid, showed lower relative growth, less root mass along with less aboveground mass (Walls et al., 2005). It was reported that the HIPV’s (Herbivore Induced Plant Volatiles) induced in Solanum peruvianum decreased the pollinator attraction and hence less seed set was observed in plants. Moreover, the bees used those volatiles cues to avoid herbivore infested inflorescences (Kessler et al., 2011). When it comes to phloem feeders, the defense signaling is mainly induced by Salicylic acid pathway. For example, plants under attack of aphids, Myzus persicae deter the attack by Bemisia tabaci and emit volatiles which tend to attract various general as well as aphid specific natural enemies (Tan and Liu, 2014). Interestingly, Datura wrighti exhibit a trade-off between plant resistance to whitefly and susceptibility to mirid bug, Tupiocoris notatus where the glandular trichomes inhibit the colonization of whitefly but increases the oviposition by T. notatus (Van Dam and Hare, 1998).
Most of the hypotheses have not been tested vigorously. Realistically, testing of proposed hypotheses directly and including all components of the hypothesis is near to impossible (Stamp, 2004). Assuming that testing is possible, whole setup required for testing hypotheses required intensive amount of work, place, labor, money etc., to list a few factors which limits the studies and rigorous testing of hypotheses. Another major issue with some hypotheses is the lack of proper definition/equation for different factors being used in defining the hypothesis (Smilanich et al., 2016). For example, some of the constraints in plant apparency hypothesis include: how to differentiate between a specialist and a generalist herbivore? What exactly apparency is? How can it be measured? In most of the studies which tested some hypotheses, a pairwise comparison has been done using a couple of plant species or in contrasting climatic conditions, however whether the same results apply to other systems is not definite (Pavia et al., 2002). Furthermore, some results are justified partially with one hypothesis and partially with the other hypothesis (Heckman, 2011). Some hypotheses apply only to a certain sub-category of plant-insect interaction niche, as seen in case of apparency hypotheses, which focus primarily on comparing defenses of perennial plants/trees with annuals. Although trade-offs deal with costs of fitness, measuring the costs of fitness directly is difficult especially in case of optimal defense hypothesis and thus, can lead to completely different results for experiments performed by two different scientists. In numerous extreme cases, the hypothesis completely fails to explain the defense-growth pattern in plants when attacked by herbivores (Barto and Cipollini, 2005; Heckman, 2011; Hattas et al., 2017). Additionally, none of the studies that we found have investigated trade-offs in case of co-infestation or sequential infection by two or more herbivores.
There is no hypothesis which has been accepted universally so far and is perfect and all of them has their own flaws and contradictions. Scientists over decades suggested amendments to the hypothesis based on different systems and results obtained over the years. These amendments occasionally work and obtained results match the predictions (López-Goldar et al., 2020). Despite the changes, some hypotheses have completely been rejected do its utmost failure, other have been partially accepted with a hope of improvement and some have been widely accepted. For example, Plant apparency hypothesis and carbon nutrient balance hypothesis have been almost completely rejected, growth-differential balance hypothesis and optimal defense hypothesis have been partially accepted, and resource availability has been the most accepted hypothesis as it justifies most of the studies, their predictions and obtained results. And thus, it has been adopted the most and considered to be the best hypotheses to explain defense-fitness-trade-offs in plants.
One of the solutions for several of the above-mentioned issues is to consider pros and cons of these hypotheses and to come up with an integrated hypothesis/equation which can make predictions for defense-fitness-trade-offs in plants with minimum errors and deviations. This hypothesis can then be tested in different systems to validate it over the years. Solanum genus can prove to be perfect for testing proposed/existing hypotheses. Solanum is found in all kinds of habitats and climatic conditions. Most studies tested theories in a chosen climate because of prevalence of the focus plant species in that area. However, with Solanum, because of its ubiquitous prevalence around the globe, comparative studies in different climatic conditions such as dry areas and wetlands can be done. Plethora of insect species with different feeding habits are found on Solanum plants (Supplementary Tables 1–3). Those insects damage different plant parts which makes it easier to test plant response in case damage done by chewing type herbivore and sucking type herbivore. For example, M. sexta feeds primarily on leaves of Solanum, but grubs of Synanthedon rileyana feed primarily on roots (Supplementary Table 1). Thus, studies contrasting growth-defense trade-offs in above-ground and underground plant parts can be done. Solanum genus contains self-pollinated, cross-pollinated, self-compatible and self-incompatible plants, using which study of trade-offs can be expanded in look into phylogenetic trade-offs pattern development across the genus. Solanum genus consisting of tomato, potato and eggplants is already a study model in many labs focusing on different aspects of insect-plant ecology. Most of Solanum species are easy to grow and maintain in controlled conditions. Solanum plants are both annuals and perennials, so theories which require a longer project time such as apparency theory and resource availability theory can also be tested using this genus. Numerous studies have already done been done on Solanum genus as model or/and because of economic value of the focus plant species. Available data and knowledge from those studies makes it easier to quantify genus specific multiple physical and chemical traits for testing defense-fitness-trade-off hypotheses. Overall, Solanum possess numerous properties which can make it a model for testing and studying plant defense-fitness-trade-offs and fill up the knowledge gap discussed in text above.
Author contributions
RK, SW, and SK conceived the idea. SW and SK wrote the first draft. All authors contributed to the article and approved the submitted version.
Funding
This project was supported by Presidential Graduate Fellowship to SW from University of Texas Rio Grande Valley. The publishing was supported by the Open Access Publishing Fund administered through the University of Arkansas Libraries.
Conflict of interest
The authors declare that the research was conducted in the absence of any commercial or financial relationships that could be construed as a potential conflict of interest.
Publisher’s note
All claims expressed in this article are solely those of the authors and do not necessarily represent those of their affiliated organizations, or those of the publisher, the editors and the reviewers. Any product that may be evaluated in this article, or claim that may be made by its manufacturer, is not guaranteed or endorsed by the publisher.
Supplementary material
The Supplementary material for this article can be found online at: https://www.frontiersin.org/articles/10.3389/fevo.2022.1094961/full#supplementary-material
References
Alba, J. M., Montserrat, M., and Fernández-Muñoz, R. (2009). Resistance to the two-spotted spider mite (Tetranychus urticae) by acylsucroses of wild tomato (Solanum pimpinellifolium) trichomes studied in a recombinant inbred line population. Exp. Appl. Acarol. 47, 35–47. doi: 10.1007/s10493-008-9192-4
Anjana, V. N., Kumari, N. O., and Mathew, P. M. (2008). Intra-specific variations of trichomes in the genus solanum L. Biodivers. Conserv., 109–114.
Baldwin, I. T. (1998). Jasmonate-induced responses are costly but benefit plants under attack in native populations. Proc. Natl. Acad. Sci. 95, 8113–8118. doi: 10.1073/pnas.95.14.8113
Barto, E. K., and Cipollini, D. (2005). Testing the optimal defense theory and the growth-differentiation balance hypothesis in Arabidopsis thaliana. Oecologia 146, 169–178. doi: 10.1007/s00442-005-0207-0
Bazzaz, F. A., Chiariello, N. R., Coley, P. D., and Pitelka, L. F. (1987). Allocating resources to reproduction and Defense. Bioscience 37, 58–67. doi: 10.2307/1310178
Begon, M. (1984). “Density and individual fitness: asymmetric competition.” in Evolutionary Ecology, ed. B. Shorrocks (Oxford: Blackwell Sci). 175–94.
Blackshaw, R. E. (1991). Hairy nightshade (Solanum sarrachoides) interference in dry beans (Phaseolus vulgaris). Weed Sci. 39, 48–53. doi: 10.1017/S0043174500057854
Bryant, J. P., Chapin, F. S., and Klein, D. R. (1983). Carbon/nutrient balance of boreal plants in relation to vertebrate herbivory. Oikos 40:357. doi: 10.2307/3544308
Calf, O. W., Huber, H., Peters, J. L., Weinhold, A., and van Dam, N. M. (2018). Glycoalkaloid composition explains variation in slug resistance in Solanum dulcamara. Oecologia 187, 495–506. doi: 10.1007/s00442-018-4064-z
Campbell, S. A., Thaler, J. S., and Kessler, A. (2013). Plant chemistry underlies herbivore-mediated inbreeding depression in nature. Ecol. Lett. 16, 252–260. doi: 10.1111/ele.12036
Cho, K. S., Kwon, M., Cho, J. H., Im, J. S., Park, Y. E., Hong, S. Y., et al. (2017). Characterization of trichome morphology and aphid resistance in cultivated and wild species of potato. Hortic. Environ. Biotechnol. 58, 450–457. doi: 10.1007/s13580-017-0078-4
Cipollini, M. L., Paulk, E., Mink, K., Vaughn, K., and Fischer, T. (2004). Defense Tradeoffs in fleshy fruits: effects of resource variation on growth, reproduction, and fruit secondary chemistry in Solanum carolinense. J. Chem. Ecol. 30, 1–17. doi: 10.1023/B:JOEC.0000013179.45661.68
Coley, P. D., Bryant, J. P., and Chapin, F. S. (1985). Resource availability and plant antiherbivore Defense. Science 230, 895–899. doi: 10.1126/science.230.4728.895
Coverdale, T. C., and Agrawal, A. A. (2022). Experimental insect suppression causes loss of induced, but not constitutive, resistance in Solanum carolinense. Ecology 103:e3786. doi: 10.1002/ecy.3786
Dalin, P., Ågren, J., Björkman, C., Huttunen, P., and Kärkkäinen, K. (2008). “Leaf trichome formation and plant resistance to herbivory” in Induced Plant Resistance Herbivory, ed. Andreas Schaller (Dordrecht: Springer) 89–105.
De Almeida, C. F. C. B. R., Lima, E. S. T. C., Amorim, E. L. C., Maia, M. B. D. S., and Albuquerque, U. P. (2005). Life strategy and chemical composition as predictors of the selection of medicinal plants from the caatinga (Northeast Brazil). J. Arid Environ. 62, 127–142. doi: 10.1016/j.jaridenv.2004.09.020
Degenhardt, D. C., Refi-Hind, S., Stratmann, J. W., and Lincoln, D. E. (2010). Systemin and jasmonic acid regulate constitutive and herbivore-induced systemic volatile emissions in tomato, Solanum lycopersicum. Phytochemistry 71, 2024–2037. doi: 10.1016/j.phytochem.2010.09.010
Endara, M. J., and Coley, P. D. (2011). The resource availability hypothesis revisited: a meta-analysis. Funct. Ecol. 25, 389–398. doi: 10.1111/j.1365-2435.2010.01803.x
Fajer, E. D., Bowers, M. D., and Bazzaz, F. A. (1992). The effect of nutrients and enriched CO2 environments on production of carbon-based allelochemicals in Plantago: a test of the carbon/nutrient balance hypothesis. Am. Nat. 140, 707–723. doi: 10.1086/285436
Feeny, P. (1976). “Plant Apparency and Chemical Defense,” in Biochemical Interaction Between Plants and Insects, eds. J. W. Wallace, R. L. Mansell, J. W. Wallace, and R. L. Mansell Vol 10. (Springer, Boston, MA: Recent Advances in Phytochemistry). doi: 10.1007/978-1-4684-2646-5_1
Fine, P. V., and Mesones, I. (2011). The role of natural enemies in the germination and establishment of Pachira (Malvaceae) trees in the Peruvian Amazon. Biotropica 43, 265–269. doi: 10.1111/j.1744-7429.2011.00778.x
Gandhi, A., Kariyat, R. R., Chappa, C., Tayal, M., and Sahoo, N. (2020). Tobacco hornworm (Manduca sexta) Oral secretion elicits reactive oxygen species in isolated tomato protoplasts. Int. J. Mol. Sci. 21:8297. doi: 10.3390/ijms21218297
Gandhi, A., Kariyat, R., Harikishore, A., Ayati, M., Bhunia, A., and Sahoo, N. (2021). Deciphering the role of ion channels in early Defense Signaling against herbivorous insects. Cells 10:2219. doi: 10.3390/cells10092219
Garcia, A., Martinez, M., Diaz, I., and Santamaria, M. E. (2021). The price of the induced defense against pests: a meta-analysis. Front. Plant Sci. 11:615122. doi: 10.3389/fpls.2020.615122
Gatehouse, J. A. (2002). Plant resistance towards insect herbivores: a dynamic interaction. New Phytol. 156, 145–169. doi: 10.1046/j.1469-8137.2002.00519.x
Gebhardt, C. (2016). The historical role of species from the Solanaceae plant family in genetic research. Theor. Appl. Genet. 129, 2281–2294. doi: 10.1007/s00122-016-2804-1
Gianoli, E., and Salgado-Luarte, C. (2017). Tolerance to herbivory and the resource availability hypothesis. Biol. Lett. 13:20170120. doi: 10.1098/rsbl.2017.0120
Gols, R., Witjes, L. M. A., Loon, J. J. A., Posthumus, M. A., Dicke, M., and Harvey, J. A. (2008). The effect of direct and indirect defenses in two wild brassicaceous plant species on a specialist herbivore and its gregarious endoparasitoid. Entomol. Exp. Appl. 128, 99–108. doi: 10.1111/j.1570-7458.2008.00681.x
Hahn, P. G., and Maron, J. L. (2016). A framework for predicting intraspecific variation in plant defense. Trends Ecol. Evol. 31, 646–656. doi: 10.1016/j.tree.2016.05.007
Hamilton, J. G., Zangerl, A. R., DeLucia, E. H., and Berenbaum, M. R. (2001). The carbon–nutrient balance hypothesis: its rise and fall. Ecol. Lett. 4, 86–95. doi: 10.1046/j.1461-0248.2001.00192.x
Hanley, M. E., Lamont, B. B., Fairbanks, M. M., and Rafferty, C. M. (2007). Plant structural traits and their role in anti-herbivore defence. Perspect. Plant Ecol. Evol. Syst. 8, 157–178. doi: 10.1016/j.ppees.2007.01.001
Hattas, D., Scogings, P. F., and Julkunen-Tiitto, R. (2017). Does the growth differentiation balance hypothesis explain allocation to secondary metabolites in Combretum apiculatum, an African savanna woody species? J. Chem. Ecol. 43, 153–163. doi: 10.1007/s10886-016-0808-6
Heckman, M. L. (2011). A test of optimal defense theory vs. the growth-differentiation balance hypothesis as predictors of seaweed palatability and defenses. Doctoral dissertation Georgia Institute of Technology.
Hermanutz, L. A., and Weaver, S. E. (1996). Agroecotypes or phenotypic plasticity? Comparison of agrestal and ruderal populations of the weed Solanum ptycanthum. Oecologia 105, 271–280. doi: 10.1007/BF00328557
Herms, D. A., and Mattson, W. J. (1992). The dilemma of plants: to grow or defend. Q. Rev. Biol. 67, 283–335. doi: 10.1086/417659
Hernán, G., Castejón, I., Terrados, J., and Tomas, F. (2019). Herbivory and resource availability shift plant defense and herbivore feeding choice in a seagrass system. Oecologia 189, 719–732. doi: 10.1007/s00442-019-04364-6
Horgan, F. G., Quiring, D. T., Lagnaoui, A., and Pelletier, Y. (2007). Variable responses of tuber moth to the leaf trichomes of wild potatoes. Entomol. Exp. Appl. 125, 1–12. doi: 10.1111/j.1570-7458.2007.00590.x
Horgan, F. G., Quiring, D. T., Lagnaoui, A., and Pelletier, Y. (2009). Trade-off between foliage and tuber resistance to Phthorimaea operculella in wild potatoes. Entomol. Exp. Appl. 131, 130–137. doi: 10.1111/j.1570-7458.2009.00842.x
Howe, G. A., and Jander, G. (2008). Plant immunity to insect herbivores. Annu. Rev. Plant Biol. 59, 41–66. doi: 10.1146/annurev.arplant.59.032607.092825
Ishikawa, T., and Takahata, K. (2019). Insect and mite pests of Pepino (Solanum muricatum ait.) in Japan. Biodivers. Data J. 7:36453. doi: 10.3897/BDJ.7.e36453
Jones, C. G., and Hartley, S. E. (1999). A protein competition model of phenolic allocation. Oikos 86, 27–44. doi: 10.2307/3546567
Kant, M. R., Ament, K., Sabelis, M. W., Haring, M. A., and Schuurink, R. C. (2004). Differential timing of spider mite-induced direct and indirect Defenses in tomato plants. Plant Physiol. 135, 483–495. doi: 10.1104/pp.103.038315
Kaplan, I., Dively, G. P., and Denno, R. F. (2009). The costs of anti-herbivore defense traits in agricultural crop plants: a case study involving leafhoppers and trichomes. Ecol. Appl. 19, 864–872. doi: 10.1890/07-1566.1
Karban, R., Agrawal, A., and Mangel, M. (1997). The benefits of induced defenses against herbivores. Ecology 78, 1351–1355. doi: 10.1890/0012-9658(1997)078[1351:TBOIDA]2.0.CO;2
Karban, R., and Myers, J. H. (1989). Induced plant responses to herbivory. Annu. Rev. Ecol. Syst. 20, 331–348. doi: 10.1146/annurev.es.20.110189.001555
Kariuki, E. M., Hix, R. L., Hight, S. D., Reitz, S. R., and Kairo, M. T. (2016). Influence of sun and shade conditions on Gratiana boliviana (coleoptera: Chrysomelidae) abundance and feeding activity on tropical soda apple (Solanaceae) under field conditions. Fla. Entomol. 99, 552–554. doi: 10.1653/024.099.0334
Kariyat, R. R., Balogh, C. M., Moraski, R. P., Moraes, C. M., Mescher, M. C., and Stephenson, A. G. (2013). Constitutive and herbivore-induced structural defenses are compromised by inbreeding in Solanum carolinense (Solanaceae). Am. J. Bot. 100, 1014–1021. doi: 10.3732/ajb.1200612
Kariyat, R. R., Hardison, S. B., Moraes, C. M., and Mescher, M. C. (2017). Plant spines deter herbivory by restricting caterpillar movement. Biol. Lett. 13:20170176. doi: 10.1098/rsbl.2017.0176
Kariyat, R. R., Hardison, S. B., Ryan, A. B., Stephenson, A. G., Moraes, C. M., and Mescher, M. C. (2018). Leaf trichomes affect caterpillar feeding in an instar-specific manner. Commun. Integr. Biol. 11, 1–6. doi: 10.1080/19420889.2018.1486653
Kariyat, R. R., Mauck, K. E., De Moraes, C. M., Stephenson, A. G., and Mescher, M. C. (2012a). Inbreeding alters volatile signalling phenotypes and influences tri-trophic interactions in horsenettle (Solanum carolinense L.). Ecol. Lett. 15, 301–309. doi: 10.1111/j.1461-0248.2011.01738.x
Kariyat, R. R., Mena-Alí, J., Forry, B., Mescher, M. C., Moraes, C. M., and Stephenson, A. G. (2012b). Inbreeding, herbivory, and the transcriptome of Solanum carolinense. Entomol. Exp. Appl. 144, 134–144. doi: 10.1111/j.1570-7458.2012.01269.x
Kariyat, R. R., Raya, C. E., Chavana, J., Cantu, J., Guzman, G., and Sasidharan, L. (2019). Feeding on glandular and non-glandular leaf trichomes negatively affect growth and development in tobacco hornworm (Manduca sexta) caterpillars. Arthropod Plant Interact. 13, 321–333. doi: 10.1007/s11829-019-09678-z
Kaur, I., and Kariyat, R. R. (2020). Eating barbed wire: direct and indirect defensive roles of non-glandular trichomes. Plant Cell Environ. 43, 2015–2018. doi: 10.1111/pce.13828
Kaur, J., and Kariyat, R. R. (2020). “Role of trichomes in plant stress biology” in Evolutionary ecology of plant-herbivore interaction eds. J. Núñez-Farfán and P. Luis Valverde (Cham: Springer), 15–35.
Keith, R. A., and Mitchell-Olds, T. (2017). Testing the optimal defense hypothesis in nature: variation for glucosinolate profiles within plants. PLoS One 12:e0180971. doi: 10.1371/journal.pone.0180971
Kennedy, G. G. (2003). Tomato, pests, parasitoids, and predators: tritrophic interactions involving the genus Lycopersicon. Annu. Rev. Entomol. 48, 51–72. doi: 10.1146/annurev.ento.48.091801.112733
Kessler, A., and Baldwin, I. T. (2001). Defensive function of herbivore-induced plant volatile emissions in nature. Science 291, 2141–2144. doi: 10.1126/science.291.5511.2141
Kessler, A., Halitschke, R., and Poveda, K. (2011). Herbivory-mediated pollinator limitation: negative impacts of induced volatiles on plant-pollinator interactions. Ecology 92, 1769–1780. doi: 10.1890/10-1945.1
Kim, J., Tooker, J. F., Luthe, D. S., Moraes, C. M., and Felton, G. W. (2012). Insect eggs can enhance wound response in plants: a study system of tomato Solanum lycopersicum L. and Helicoverpa zea Boddie. PLoS One 7:e37420. doi: 10.1371/journal.pone.0037420
Koricheva, J. (2002). The carbon-nutrient balance hypothesis is dead; long live the carbon-nutrient balance hypothesis? Oikos 98, 537–539. doi: 10.1034/j.1600-0706.2002.980319.x
Kundu, A., Mishra, S., and Vadassery, J. (2018). Spodoptera litura-mediated chemical defense is differentially modulated in older and younger systemic leaves of Solanum lycopersicum. Planta 248, 981–997. doi: 10.1007/s00425-018-2953-3
Lamarre, G. P., Baraloto, C., Fortunel, C., Dávila, N., Mesones, I., Rios, J. G., et al. (2012). Herbivory, growth rates, and habitat specialization in tropical tree lineages: implications for Amazonian beta-diversity. Ecology 93, S195–S210. doi: 10.1890/11-0397.1
Lawrence, S. D., Novak, N. G., Ju, C. J. T., and Cooke, J. E. K. (2008). Potato, solanum tuberosum, defense against Colorado potato beetle, Leptinotarsa Decemlineata (say): microarray gene expression profiling of potato by Colorado potato beetle regurgitant treatment of wounded leaves. J. Chem. Ecol. 34, 1013–1025. doi: 10.1007/s10886-008-9507-2
Lerdau, M., and Coley, P. D. (2002). Benefits of the carbon-nutrient balance hypothesis. Oikos 98, 534–536. doi: 10.1034/j.1600-0706.2002.980318.x
Levin, D. A. (1973). The role of trichomes in plant Defense. Q. Rev. Biol. 48, 3–15. doi: 10.1086/407484
Loomis, W. E. Growth and differentiation in plants, Iowa State College Press, Ames, IA. (1953). pp. 197–217.
López-Goldar, X., Zas, R., and Sampedro, L. (2020). Resource availability drives microevolutionary patterns of plant defences. Funct. Ecol. 34, 1640–1652. doi: 10.1111/1365-2435.13610
Lorio, P. L. (1986). Growth-differentiation balance: a basis for understanding southern pine beetle-tree interactions. For. Ecol. Manage. 14, 259–273. doi: 10.1016/0378-1127(86)90172-6
Malakar, R., and Tingey, W. M. (2000). Glandular trichomes of Solanum berthaultii and its hybrids with potato deter oviposition and impair growth of potato tuber moth. Entomol. Exp. Appl. 94, 249–257. doi: 10.1046/j.1570-7458.2000.00627.x
Malcolm, S. B. (1994). Milkweeds, monarch butterflies and the ecological significance of cardenolides. Chemoecology 5-6, 101–117. doi: 10.1007/BF01240595
Martin, J. S., Martin, M. M., and Bernays, E. A. (1987). Failure of tannic acid to inhibit digestion or reduce digestibility of plant protein in gut fluids of insect herbivores. J. Chem. Ecol. 13, 605–621. doi: 10.1007/BF01880103
Massad, T. J., Dyer, L. A., and Vega, C. G. (2012). Costs of Defense and a test of the carbon-nutrient balance and growth-differentiation balance hypotheses for two co-occurring classes of plant Defense. PLoS One 7:e47554. doi: 10.1371/journal.pone.0047554
Massey, F. P., Ennos, A. R., and Hartley, S. E. (2007). Grasses and the resource availability hypothesis: the importance of silica-based defences. J. Ecol. 95, 414–424. doi: 10.1111/j.1365-2745.2007.01223.x
McCall, A. C., and Fordyce, J. A. (2010). Can optimal defence theory be used to predict the distribution of plant chemical defences? J. Ecol. 98, 985–992. doi: 10.1111/j.1365-2745.2010.01693.x
Mckey, D. (1979). “The distribution of secondary compounds within plants,” in Herbivores, Their Interaction With Secondary Plant Metabolites, eds. G. A. Rosenthal and D. H. Janzen (New York: Academic Press) 55–133.
Muckadell, M. (1962). Environmental factors in development stages of trees. Tree Growth. Ronald Press, New York, 289–298.
Müller, C., Bräutigam, A., Eilers, E., Junker, R., Schnitzler, J. P., Steppuhn, A., et al. (2020). Ecology and evolution of intraspecific chemodiversity of plants. Res. Ideas Outcomes 6:e49810. doi: 10.3897/rio.6.e49810
Ohnmeiss, T., and Baldwin, I. T. (2000). Optimal defense theory predicts the ontogeny of an induced nicotine defense. Ecology 81, 1765–1783. doi: 10.1890/0012-9658(2000)081[1765:ODTPTO]2.0.CO;2
Palumbo, M. J., Putz, F. E., and Talcott, S. T. (2007). Nitrogen fertilizer and gender effects on the secondary metabolism of yaupon, a caffeine-containing north American holly. Oecologia 151, 1–9. doi: 10.1007/s00442-006-0574-1
Pavia, H., Toth, G. B., and Åberg, P. (2002). Optimal defense theory: elasticity analysis as a tool to predict intraplant variation in defenses. Ecology 83, 891–897. doi: 10.1890/0012-9658(2002)083[0891:ODTEAA]2.0.CO;2
Peiffer, M., Tooker, J. F., Luthe, D. S., and Felton, G. W. (2009). Plants on early alert: glandular trichomes as sensors for insect herbivores. New Phytol. 184, 644–656. doi: 10.1111/j.1469-8137.2009.03002.x
Pelletier, Y., and Tai, G. C. C. (2001). Genotypic variability and mode of action of Colorado potato beetle (coleoptera: Chrysomelidae) resistance in seven solanum species. J. Econ. Entomol. 94, 572–578. doi: 10.1603/0022-0493-94.2.572
Price, P. W., Waring, G. L., Julkunen-Tiitto, R., Tahvanainen, J., Mooney, H. A., and Craig, T. P. (1989). Carbon-nutrient balance hypothesis in within-species phytochemical variation ofSalix lasiolepis. J. Chem. Ecol. 15, 1117–1131. doi: 10.1007/BF01014816
Redman, A. M., Cipollini, D. F., and Schultz, J. C. (2001). Fitness costs of jasmonic acid-induced defense in tomato, Lycopersicon esculentum. Oecologia 126, 380–385. doi: 10.1007/s004420000522
Rhoades, D. F., and Cates, R. G. (1976). “Toward a General Theory of Plant Antiherbivore Chemistry,” in Biochemical Interaction Between Plants and Insects Recent Advances in Phytochemistry, eds. J. W. Wallace and R. L. Mansell Vol. 10 (Boston, MA.: Springer). doi: 10.1007/978-1-4684-2646-5_4
Rivard, D., Cloutier, C., and Michaud, D. (2004). Colorado potato beetles show differential digestive compensatory responses to host plants expressing distinct sets of defense proteins. Arch. Insect Biochem. Physiol. 55, 114–123. doi: 10.1002/arch.10136
Rodríguez-López, M. J., Garzo, E., Bonani, J. P., Fereres, A., Fernández-Muñoz, R., and Moriones, E. (2011). Whitefly resistance traits derived from the wild tomato Solanum pimpinellifolium affect the preference and feeding behavior of Bemisia tabaci and reduce the spread of tomato yellow leaf curl virus. Phytopathology 101, 1191–1201. doi: 10.1094/PHYTO-01-11-0028
Rostás, M., Simon, M., and Hilker, M. (2003). Ecological cross-effects of induced plant responses towards herbivores and phytopathogenic fungi. Basic Appl. Ecol. 4, 43–62. doi: 10.1078/1439-1791-00132
Rowell, C. H. F., and Simpson, S. J. (1992). A peripheral input of thoracic origin inhibits chewing movements in the larvae of Manduca sexta. J. Insect Physiol. 38, 475–483. doi: 10.1016/0022-1910(92)90072-L
Rushing, D. W., Murray, D. S., and Verhalen, L. M. (1985). Weed interference with cotton (Gossypium hirsutum). I. Buffalobur (Solanum rostratum). Weed Sci. 33, 810–814. doi: 10.1017/S0043174500083405
Ryan, C. A. (1990). Protease inhibitors in plants: genes for improving defense against insects and pathogens. Annu. Rev. Phytopathol. 28, 425–449. doi: 10.1146/annurev.py.28.090190.002233
Shih, P. M. (2018). Towards a sustainable bio-based economy: redirecting primary metabolism to new products with plant synthetic biology. Plant Sci. 273, 84–91. doi: 10.1016/j.plantsci.2018.03.012
Silva, D. B., Bueno, V. H. P., Loon, J. J. A., Peñaflor, M. F. G. V., Bento, J. M. S., and Lenteren, J. C. (2018). Attraction of three mirid predators to tomato infested by both the tomato Leaf Mining moth Tuta absoluta and the whitefly Bemisia tabaci. J. Chem. Ecol. 44, 29–39. doi: 10.1007/s10886-017-0909-x
Singh, S., Kaur, I., and Kariyat, R. (2021). The multifunctional roles of polyphenols in plant-herbivore interactions. Int. J. Mol. Sci. 22:1442. doi: 10.3390/ijms22031442
Smilanich, A. M., Fincher, R. M., and Dyer, L. A. (2016). Does plant apparency matter? Thirty years of data provide limited support but reveal clear patterns of the effects of plant chemistry on herbivores. New Phytol. 210, 1044–1057. doi: 10.1111/nph.13875
Stamp, N. (2003). Out of the quagmire of plant defense hypotheses. Q. Rev. Biol. 78, 23–55. doi: 10.1086/367580
Stamp, N. (2004). Can the growth–differentiation balance hypothesis be tested rigorously? Oikos 107, 439–448. doi: 10.1111/j.0030-1299.2004.12039.x
Steinbrenner, A. D., Gómez, S., Osorio, S., Fernie, A. R., and Orians, C. M. (2011). Herbivore-induced changes in tomato (Solanum lycopersicum) primary metabolism: a whole plant perspective. J. Chem. Ecol. 37, 1294–1303. doi: 10.1007/s10886-011-0042-1
Stepp, J., and Moerman, D. E. (2001). The importance of weeds in ethnopharmacology. J. Ethnopharmacol. 75, 19–23. doi: 10.1016/S0378-8741(00)00385-8
Stireman, J. O., Dyer, L. A., and Matlock, R. B. (2005). Top-down forces in managed versus unmanaged habitats. Ecol. Predator-Prey Interact., 303–323.
Sun, R., Jiang, X., Reichelt, M., Gershenzon, J., Subhash Pandit, S., and Giddings Vassã, D. (2019). Tritrophic metabolism of plant chemical defenses and its effects on herbivore and predator performance eLife. 8, e51029. doi: 10.7554/eLife.51029
Symon, D. E. (1981). A revision of the genus solanum in Australia. J. Adelaide Bot. Gardens 4, 1–367.
Taher, D., Rakha, M., Ramasamy, S., and Solberg, S. (2019). Sources of Resistance for Two-spotted Spider Mite (Tetranychus urticae) in Scarlet (Solanum aethiopicum L.) and Gboma (S. macrocarpon L.) Eggplant Germplasms, HortScience horts. 54, 240–245. doi: 10.21273/HORTSCI13669-18
Tan, X. L., and Liu, T. X. (2014). Aphid-induced plant volatiles affect the attractiveness of tomato plants to Bemisia tabaci and associated natural enemies. Entomol. Exp. Appl. 151, 259–269. doi: 10.1111/eea.12190
Tchatchouang, S., Beng, V. P., and Kuete, V. (2017). “Antiemetic African medicinal spices and vegetables” in Medicinal spices and vegetables from Africa, ed. Victor Kuete (Academic Press), 299–313.
The Potato Genome Sequencing Consortium (2011). Genome sequence and analysis of the tuber crop potato. Nature 475, 189–195. doi: 10.1038/nature10158
The Tomato Genome Consortium (2012). The tomato genome sequence provides insights into fleshy fruit evolution. Nature 485, 635–641. doi: 10.1038/nature11119
Tian, D., Peiffer, M., De Moraes, C. M., and Felton, G. W. (2014). Roles of ethylene and jasmonic acid in systemic induced defense in tomato (Solanum lycopersicum) against helicoverpa zea. Planta 239, 577–589. doi: 10.1007/s00425-013-1997-7
Tuomi, J., Niemelä, P., Stuart Chapin, F., Bryant, J. P., and Sirén, S. (1988). Defensive Responses of Trees in Relation to Their Carbon/Nutrient Balance. in Mechanisms of Woody Plant Defenses Against Insects, eds. W. J. Mattson, J. Levieux, and C. Bernard-Dagan (New York, NY.: Springer). doi: 10.1007/978-1-4612-3828-7_3
Turlings, T. C., and Benrey, B. (1998). Effects of plant metabolites on the behavior and development of parasitic wasps. Ecosci. 5, 321–333. doi: 10.1080/11956860.1998.11682472
Underwood, N., and Halpern, S. L. (2012). Insect herbivores, density dependence, and the performance of the perennial herb Solanum carolinense. Ecology 93, 1026–1035. doi: 10.1890/11-1320.1
Van Dam, N. M., and Hare, J. D. (1998). Differences in distribution and performance of two sap-sucking herbivores on glandular and non-glandular Datura wrightii. Ecol. Entomol. 23, 22–32. doi: 10.1046/j.1365-2311.1998.00110.x
Walls, R., Appel, H., Cipollini, M., and Schultz, J. (2005). Fertility, root reserves and the cost of inducible defenses in the perennial plant Solanum carolinense. J. Chem. Ecol. 31, 2263–2288. doi: 10.1007/s10886-005-7101-4
Wasternack, C., and Parthier, B. (1997). Jasmonate-signalled plant gene expression. Trends Plant Sci. 2, 302–307. doi: 10.1016/S1360-1385(97)89952-9
Watts, S., and Kariyat, R. (2021a). Morphological characterization of trichomes shows enormous variation in shape, density and dimensions across the leaves of 14 solanum species. AoB Plants 13:plab071. doi: 10.1093/aobpla/plab071
Watts, S., and Kariyat, R. (2021b). Picking sides: feeding on the abaxial leaf surface is costly for caterpillars. Planta 253, 1–6. doi: 10.1007/s00425-021-03592-6
Watts, S., and Kariyat, R. (2022). Are epicuticular waxes a surface defense comparable to trichomes? A test using two solanum species and a specialist herbivore. Botany. doi: 10.1139/cjb-2021-0206
Wiklund, C. (1984). Egg-laying patterns in butterflies in relation to their phenology and the visual apparency and abundance of their host plants. Oecologia 63, 23–29. doi: 10.1007/BF00379780
Wilkens, R. T., Spoerke, J. M., and Stamp, N. E. (1996). Differential responses of growth and two soluble phenolics of tomato to resource availability. Ecology 77, 247–258. doi: 10.2307/2265674
Wise, M. J. (2007). The herbivores of Solanum carolinense (Horsenettle) in northern Virginia: natural history and damage assessment. Southeast. Nat. 6, 505–522. doi: 10.1656/1528-7092(2007)6[505:THOSCH]2.0.CO;2
Wise, M. J., Cummins, J. J., and Young, C. (2008). Compensation for floral herbivory in Solanum carolinense: identifying mechanisms of tolerance. Evol. Ecol. 22, 19–37. doi: 10.1007/s10682-007-9156-x
Wise, M. J., and Sacchi, C. F. (1996). Impact of two specialist insect herbivores on reproduction of horse nettle, Solanum carolinense. Oecologia 108, 328–337. doi: 10.1007/BF00334658
Wisler, G. C., Li, R. H., Liu, H. Y., Lowry, D. S., and Duffus, J. E. (2007). Tomato chlorosis virus: a new whitefly-transmitted, phloem-limited, Bipartite Closterovirus of Tomato. Phytopathology 88, 402–409. doi: 10.1094/PHYTO1998885402
Wolinska, K. W., and Berens, M. L. (2019). Optimal Defense theory 2.0: tissue-specific stress defense prioritization as an extra layer of complexity. Commun. Integr. Biol. 12, 91–95. doi: 10.1080/19420889.2019.1625661
Yip, E. C., Tooker, J. F., Mescher, M. C., and De Moraes, C. M. (2019). Costs of plant defense priming: exposure to volatile cues from a specialist herbivore increases short-term growth but reduces rhizome production in tall goldenrod (Solidago altissima). BMC Plant Biol. 19, 1–12. doi: 10.1186/s12870-019-1820-0
Young, A. M., and Moffett, M. W. (1979). Behavioral regulatory mechanisms in populations of the butterfly Mechanitis isthmia in Costa Rica: adaptations to host plants in secondary and agricultural habitats (lepidoptera: Nymphalidae: Ithomiinae). Deutsche Entomologische Zeitschrift 26, 21–38.
Keywords: Solanum, herbivory, fitness, defense, predation
Citation: Watts S, Kaur S and Kariyat R (2023) Revisiting plant defense-fitness trade-off hypotheses using Solanum as a model genus. Front. Ecol. Evol. 10:1094961. doi: 10.3389/fevo.2022.1094961
Edited by:
Saumik Basu, Washington State University, United StatesReviewed by:
Rishikesh Ghogare, Texas A&M University, United StatesPritha Kundu, University of Nebraska-Lincoln, United States
Copyright © 2023 Watts, Kaur and Kariyat. This is an open-access article distributed under the terms of the Creative Commons Attribution License (CC BY). The use, distribution or reproduction in other forums is permitted, provided the original author(s) and the copyright owner(s) are credited and that the original publication in this journal is cited, in accordance with accepted academic practice. No use, distribution or reproduction is permitted which does not comply with these terms.
*Correspondence: Rupesh Kariyat, ✉ cmthcml5YXRAdWFyay5lZHU=
†These authors have contributed equally to this work