- College of Agriculture, Anshun University, Anshun, China
The rapid extinction of species is of considerable concern for biodiversity conservation. Identifying the drivers of species diversity and hotspots is beneficial for developing conservation strategies. Studies on insects have mainly focused on terrestrial species and rarely on semiaquatic species. Using 135,208 georeferenced occurrence records of 296 damselflies across North America, their species richness and endemism (represented by weighted endemism) patterns were mapped in a 100 × 100-km grid size, and the effects of environmental variables on species richness and endemism were investigated using generalized linear models and hierarchical partitioning. Subsequently, the top 5% grids with species richness and weighted endemism were separately selected as hotspots and their congruence was evaluated. Finally, species diversity hotspots were identified by integrating two types of hotspot grids, and gap analysis was performed to evaluate their conservation status. Temperature conditions and water availability had the strongest influence on species richness and endemism, respectively. Low congruence among species richness and endemism hotspots was observed. Moreover, four species diversity hotspots were identified, namely, region of the eastern United States and southeastern Canada, southwestern United States, central Mexico, and southernmost North America. Approximately 69.31% of the hotspot grids are not a part of the existing protected areas, presenting a significant conservation gap. The habitats of taxonomic groups should be considered while identifying the most common driving mechanisms of endemism. Strengthening the establishment of protected areas in regions with conservation gaps is urgently needed to promote the conservation of damselflies in North America.
Introduction
Species richness is the most common metric of species diversity and a fundamental measure of community and regional diversity (Gotelli and Colwell, 2001). It also plays an important role in conservation strategies owing to its effectiveness in conserving the highest number of species in small regions (Xu et al., 2017). Endemism is another critical metric of species diversity and is represented by various measures (Linder, 2001; Laffan and Crisp, 2003; Laffan et al., 2013; Guerin et al., 2015). Similar to species richness, endemism has attracted great attention of conservationists (Lamoreux et al., 2006; Laffan et al., 2013; Huang et al., 2016; Noroozi et al., 2019a; Shrestha et al., 2021) because of its inherent properties, such as high extinction risk due to climate change (Sandel et al., 2011). An increasing number of studies have suggested that both species richness and endemism must be considered while developing conservation strategies for species diversity (Zhao et al., 2016; Xu et al., 2017; Yu et al., 2017; Liu et al., 2018a; Xie et al., 2022).
In insects, the most common driving variables of species richness are contemporary climate variables, whereas those of endemism patterns are topographic or historical climate change variables (Schuldt and Assmann, 2009, 2011; Zhao et al., 2020a). Investigating these variables can aid in the understanding of underlying mechanisms that generate species diversity patterns (Jetz and Rahbek, 2002; Schuldt and Assmann, 2009). Studies on simultaneous quantification of determinants of insect species richness and endemism patterns have been mainly conducted in Western Palaearctic and China (Schuldt and Assmann, 2009, 2011; Zhao et al., 2020a) and rarely in other regions, especially at the continental scale. In addition, these studies focused only on terrestrial insects and not on other habitat groups, such as semiaquatic insects. Xu et al. (2015) observed that the most prominent environmental variable of species diversity varied among different habitat groups. Thus, insects from other habitats should be used to validate the findings regarding different underlying mechanisms of species richness and endemism patterns.
Considering the ongoing species extinction crisis and limited conservation resources, priority areas for conservation should be identified. An effective approach for this is to identify “hotspots” (Myers et al., 2000), i.e., regions hosting high species diversity. Interestingly, there is no consensus on the spatial congruence of hotspots for species richness and endemism (Linder, 2001; Orme et al., 2005; Ceballos and Ehrlich, 2006; Lamoreux et al., 2006; Feng et al., 2011; Collen et al., 2014; Daru et al., 2015; Luo et al., 2015); this directly affects the selection of priority areas for conservation. If species richness and endemism hotspots are highly consistent, then any single hotspot type can be considered as a priority area for conservation as it can act as a useful surrogate for another hotspot type (Orme et al., 2005). However, low congruence between species richness and endemism hotspots necessitates the development of other conservation strategies. Identifying priority areas is only the first step in the conservation of species; hence, measures to protect species should also be developed and implemented. Creating protected areas is one of the most effective ways to protect species in situ (Heywood and Dulloo, 2005). Unfortunately, several studies suggest that existing protected areas are ineffective in protecting species and that there is a certain degree of conservation gap (Zhang et al., 2015a; Chi et al., 2017; Noroozi et al., 2019b; Yang et al., 2021). Therefore, existing protected areas should be optimized on an urgent basis.
Damselfly, a small, slender insect species, belongs to the superfamilies Lestidae, Platystictidae, Calopterygidae, and Coenagrionidae in the suborder Zygoptera in the order Odonata (Dijkstra et al., 2013). Damselflies are semiaquatic insects because their larvae live in freshwater habitats and adults live on land. A previous study revealed that damselflies are on the verge of extinction (Clausnitzer et al., 2009), and thus their conservation needs to be increased. Unfortunately, although taxonomic studies on damselflies have markedly increased in the last few decades (e.g., Dumont et al., 2010; Dijkstra et al., 2014; Bybee et al., 2021; Suvorov et al., 2022), studies regarding its species conservation, such as those on the identification of driving factors of species diversity patterns and hotspots, are relatively less, thereby hindering the development of appropriate conservation strategies. Damselflies are widely distributed and are found on every continent except Antarctica. North America is one of the continents with the highest number of damselflies, making it an ideal area for conducting studies on their macroecology and conservation biogeography.
Based on the distribution data of North American damselflies, this study aimed to (1) explore the most prominent factors of species richness and endemism patterns, (2) evaluate the spatial congruence of species richness and endemism hotspots, and (3) identify species diversity hotspots for conservation priority areas and evaluate their conservation status.
Materials and methods
Species distribution data
A total of 135,208 occurrence records of 296 North American damselflies were compiled from the Global Biodiversity Information Facility (GBIF)1 and literature (Ball-Damerow et al., 2015). For the occurrence records obtained from GBIF, only those with geographical coordinates of spatial uncertainty of <10 km were selected. Furthermore, we removed duplicates and ambiguous occurrence records. The species name was standardized using Catalog of Life.2
Species diversity patterns
Species richness and endemism patterns were visualized using a 100 × 100-km grid size. This grid size was chosen because it is ideal for mapping macro patterns of species diversity (Hortal, 2008) and has been widely used in North America (e.g., Wang et al., 2009; Feng et al., 2019). Species richness refers to the total number of species in each grid. Endemism was measured as weighted endemism in each grid (Linder, 2001), which was calculated using Equation 1. The weighted endemism value in a grid is calculated by summing up the weights of each species (inverse of the number of grids occupied by species) within it. Only grids with at least 50% land area were used in subsequent analysis.
where Mi is the number of grids occupied by each species.
Environmental variables
We divided 11 environmental variables into the following four categories: (1) temperature: mean annual temperature (MAT), mean temperature of warmest quarter (MTWQ), and annual potential evapotranspiration (PET); (2) water availability: mean annual precipitation (MAP) and precipitation of warmest quarter (PWQ); (3) habitat heterogeneity: range of the mean annual temperature (MATR) and mean annual precipitation (MAPR), elevation range (ER), and slope (SP), with MATR, MAPR, and ER defined as the difference between their respective maximum and minimum values in a 100 × 100-km grid; and (4) historical climate change: MAT and MAP changes since the last glacial maximum (MATC and MAPC) calculated as MATC = |modernMAT − LGMMAT| and MAPC = |modernMAP − LGMMAP|. Due to uncertainty of the past climate model, MAT and MAP in the last glacial maximum were computed using the mean of the Model for Interdisciplinary Research on Climate Earth system and Community Climate System Model version 4 models.
MAT and MAP in the modern and last glacial maximum, MTWQ, PWQ, and elevation were obtained from the WorldClim database.3 PET was downloaded from the CGIAR-CSI database.4 The resolution of all environmental variables was 30 arc-seconds, except for MAT and MAP in the last glacial maximum (2.5 min). The environmental variable values in a 100 × 100-km grid were computed as the mean of all pixels (30 arc-seconds or 2.5 min) within that grid using ArcGIS 10.7 (ESRI, Redlands, CA, United States)5.
Data analysis
To evaluate the effect of sampling bias on species richness, a linear regression model was developed (Zhao et al., 2020a,b) based on the log10 (x + 1) transformed number of records and species richness in each grid. If the ratio of observed richness to expected richness based on the linear regression in each grid was >70%, it was considered well sampled (Sánchez-Fernández et al., 2008; Romo and García-Barros, 2010). Generalized linear models (GLMs) with quasi-Poisson residuals were used to assess the relationships between species richness/endemism and environment variables (Liu et al., 2017, 2018b) because these models can handle overdispersed problems better than negative binomial residuals (Ver Hoef and Boveng, 2007). First, univariate analysis was performed using GLMs, and coefficients of determination (R2, %) were estimated using Equation 2 (Wang et al., 2011). The significance test of the aforementioned models was recalculated using modified t-tests (Dutilleul et al., 1993) because spatial autocorrelations may inflate the rate of type I errors (Diniz-Filho et al., 2003). Only p < 0.1 was considered significant in GLMs (Liu et al., 2017, 2018b).
Furthermore, hierarchical partitioning was performed to assess the relative importance of environmental variables, identify the most likely causal factors, and effectively alleviate collinearity (Chevan and Sutherland, 1991; Olea et al., 2010). The variance inflation factors of environment variables for species richness and weighted endemism were found to be <18. Before performing hierarchical partitioning for species richness, non-significant environmental variables in univariate analysis were excluded (Table 1). To avoid the influence of different numbers of variables on the results, eight environmental variables were used to assess weighted endemism. PET, MAPC, and SP were excluded because their R2 value was lower than that of other variables (Table 1). The relative role of each environment variable was expressed by its independent effects. The significance of hierarchical partitioning was assessed using a randomization test with a repeated number of 100, and the significance is based on the upper 0.95 confidence limit. Statistical analysis was performed using R 4.2.1 (R Core Team, 2022) with lm and glm functions and car (Fox et al., 2022), hier.part (Walsh and Mac Nally, 2020), and SpatialPack (Osorio et al., 2022) packages.
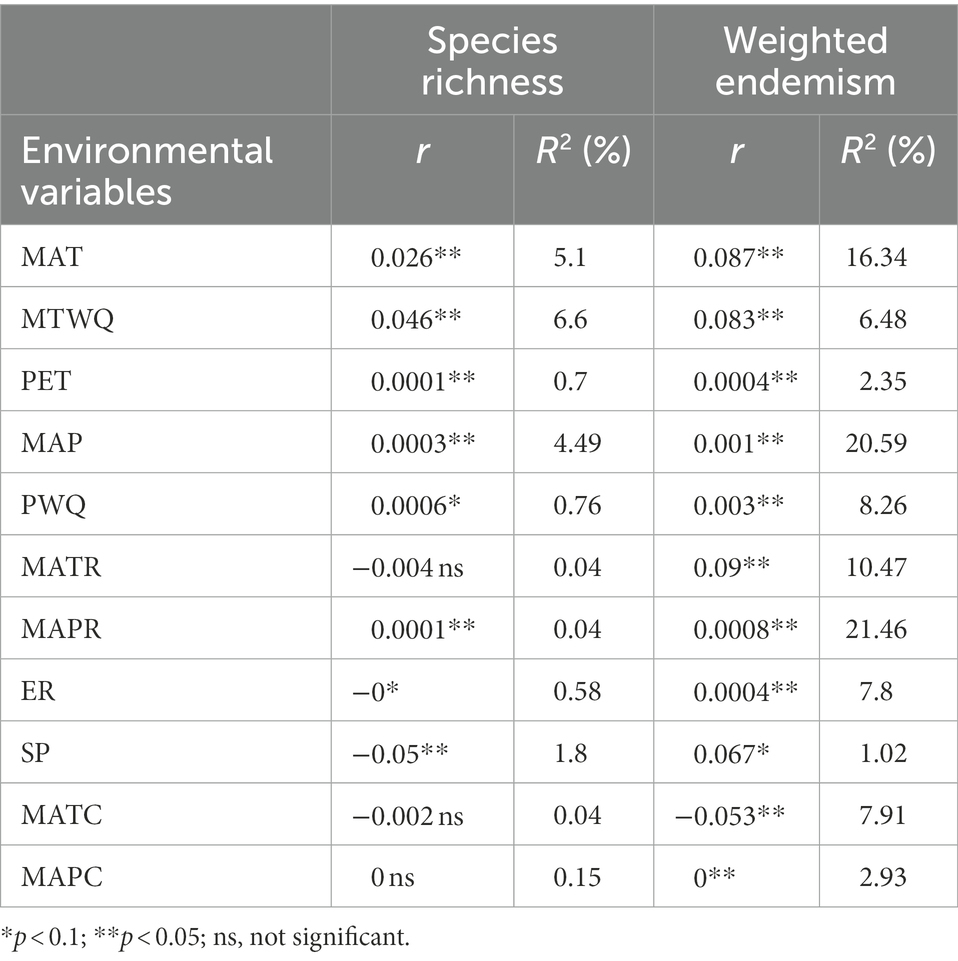
Table 1. Relationship between species richness/weighted endemism and each environmental variable identified using GLMs.
The top 5% grids (most commonly used threshold value) of species richness and weighted endemism were defined as species richness and endemism hotspots, respectively (López-Pujol et al., 2011; Yuan et al., 2014; Zhao et al., 2016; Chi et al., 2017). Two types of hotspots (i.e., total grids of two hotspots) were integrated as final species diversity hotspots, i.e., conservation priority areas (Zhang et al., 2021), due to low spatial congruence among hotspots (see results below). Furthermore, gap analysis was used to assess conservation gaps. We overlaid the species diversity hotspot layer with a protected area layer and defined conservation gaps as grids with a protected area coverage of <10% of the grid area (Xu et al., 2017).
Results
Using linear regression models, we determined that the species richness was >73.67% of the predicted value in each grid (Figure 1). This result indicates a relatively good sampling completeness. High species richness and endemism were consistently observed across central and southern areas of North America (Figures 2A,B).
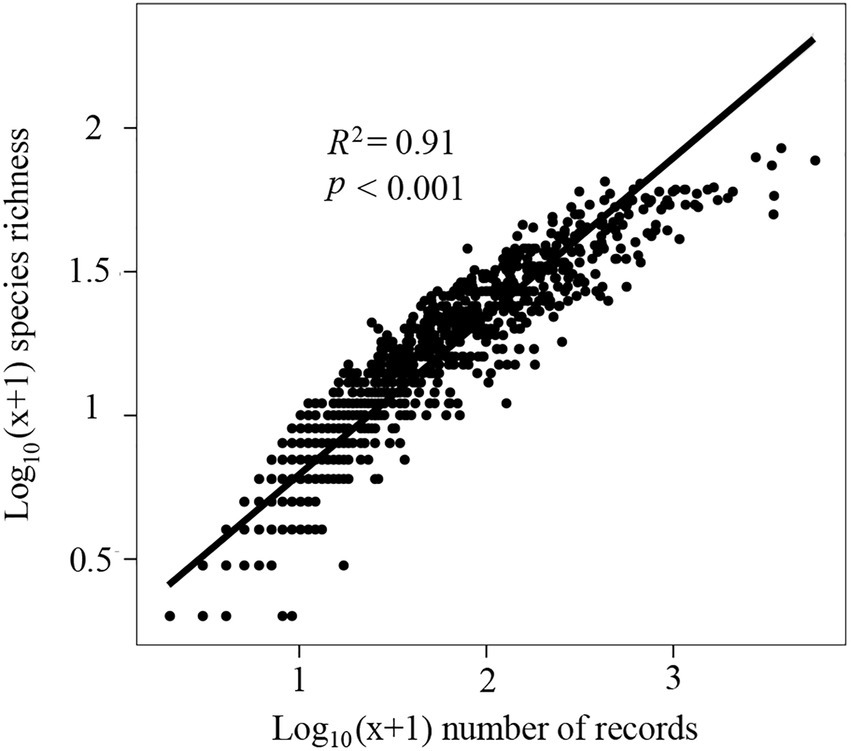
Figure 1. Linear regression (y = 0.55× + 0.243) for log10 (× + 1) transformed number of records and species richness in a 100 × 100-km grid.
The results of GLMs demonstrate that species richness is positively associated with MAT, MTWQ, PET, MAP, PQW, and MAPR but negatively with ER and SP (Table 1). Furthermore, MATR, MATC, and MAPC did not significantly affect species richness (Table 1). Finally, weighted endemism was found to be negatively correlated with MATC but positively with other remaining variables (Table 1).
Hierarchical partitioning analysis revealed that MTWQ, representing temperature, is the most important environmental variable affecting species richness. PWQ, MAP, MAT, and PET showed moderate contribution to species richness, whereas habitat heterogeneity variables (SP, MAPR, and ER) showed relatively low contribution (Figure 3A). The rank order of the effects of environmental variables on weighted endemism was different from that of species richness (Figure 3B). MAP, representing water availability, had the largest influence on weighted endemism, followed by PWQ and MAT. Moreover, MTWQ, MATC, and the three habitat heterogeneity variables—ER, MATR, and MAPR—had a low influence on weighted endemism (Figure 3B).
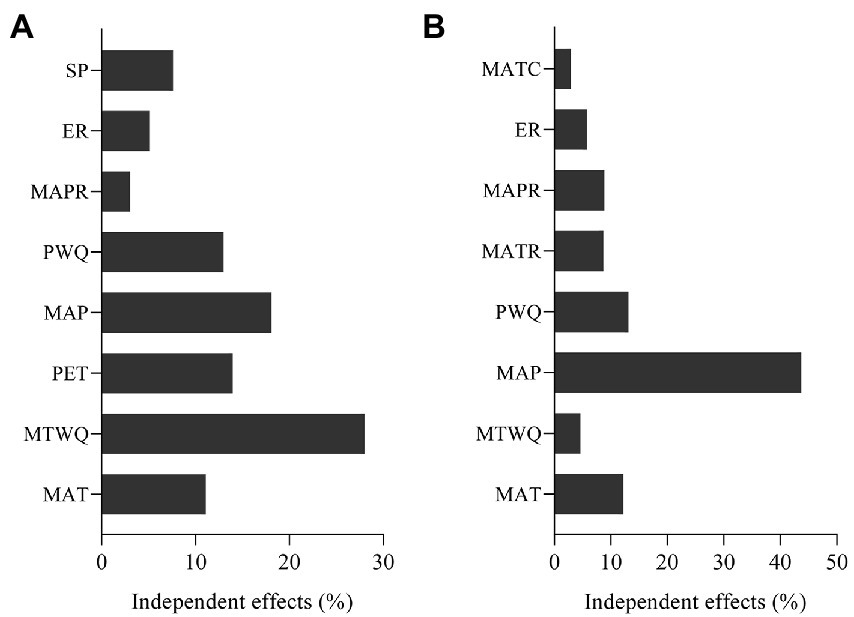
Figure 3. Independent effects of environmental variables for species richness (A) and weighted endemism (B). All environmental variables were significant.
Cumulatively, species richness and endemism hotspots accounted for 88 grids; of these, only 38.63% (34) grids were common to species richness and endemism hotspots and showed low spatial congruence. By integrating species richness and endemism hotspot grids (Figures 4A,B), we obtained four species diversity hotspots (Figure 4C): region of the eastern United States and southeastern Canada (No. 1), southwestern United States (No. 2), central Mexico (No. 3), and southernmost North America (No. 4). Moreover, a large conservation gap was observed (Figure 5). Approximately 69.31% (61) of the total grids were not a part of the existing protected areas. We also found that the number of grids with and without protection in each priority area was different (Table 2).
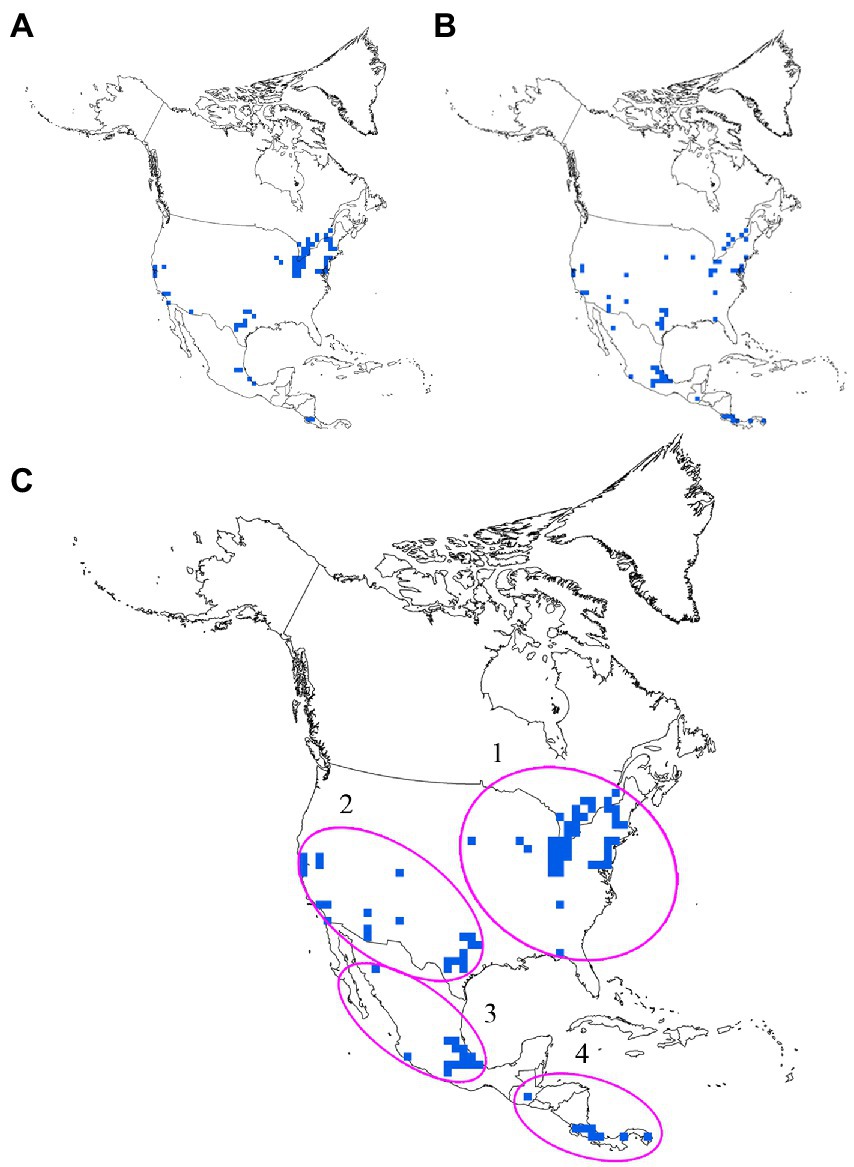
Figure 4. Grids of species richness (A) and weighted endemism (B) hotspots and conservation priority areas (C).
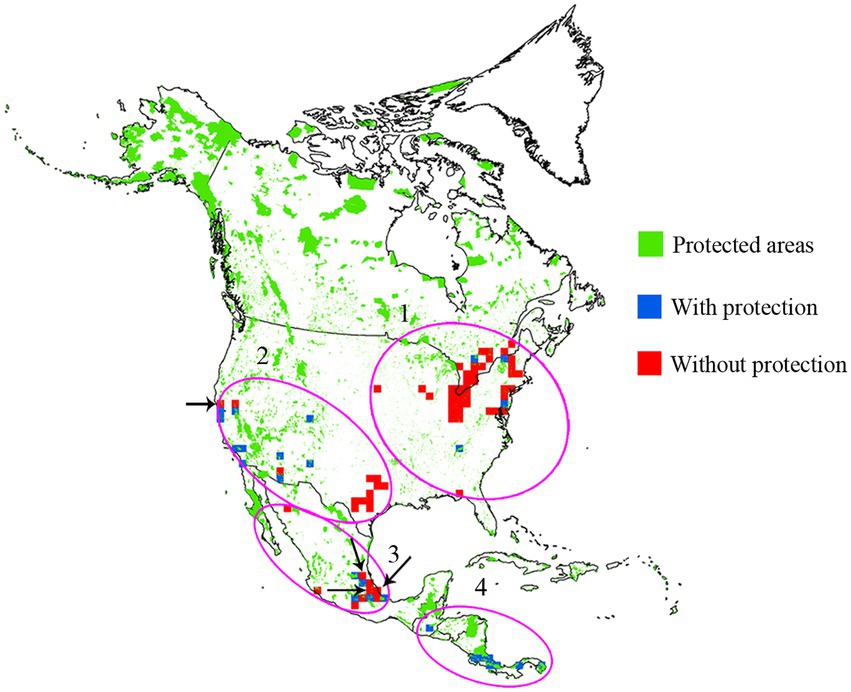
Figure 5. Conservation status of the grids of four species diversity hotspots. The black arrow indicates a grid with threatened species.
Discussion
Our study results indicate that the most prominent environmental factor influencing species richness and endemism of damselflies across North America varies. MTWQ, representing temperature, is the most important environmental variable affecting species richness. Temperature conditions have been reported to improve physiological activities in organisms, such as reproduction (Turner et al., 1987, 1988, 2004), thereby leading to larger population sizes, lower extinction rates, and ultimately higher species richness (Wang et al., 2009; Luo et al., 2012). Some empirical studies have shown the effect of temperatures on physiological activities of damselflies. For example, the rate of development of eggs and larvae increased with an increase in temperature (Rivard et al., 1975; Pickup and Thompson, 1984). In addition, most species exhibited an increase in the number of generations per year in areas with higher temperatures, such as the members of the Calopterygidae and Coenagrionidae families (Corbet et al., 2006). The mortality rate was significantly lower at low temperatures and reached 100% at 15°C (Rivard et al., 1975). In the present study, we observed that central and southern North America with high temperature generated great species richness, whereas northern North America with low temperature resulted in low species richness. Another reason that temperature determines the pattern of species richness is that it controls the species rate (Fine, 2015). In general, higher temperatures lead to higher rates of speciation (Clarke and Gaston, 2006; Brown, 2014). Thus, high species diversity of damselflies in central and southern North America is associated with higher speciation rates induced by high temperatures.
Previous studies on terrestrial insects have determined that endemism patterns are driven by historical climate change or topographic variables (Schuldt and Assmann, 2009, 2011; Zhao et al., 2020a); the reason for this is that endemic species cannot track historical climate change or the role of topographic variables as a historical process, respectively. However, our results clearly indicated that endemism patterns are not controlled by historical climate change or topographic variables but by MAP, which represents water availability (Figure 3). This result is not consistent with that of previous studies and may be largely related to larval habitats. The larvae of damselflies are almost entirely aquatic, and they move to a place near water and develop into adults (Nair, 2011). However, low dispersal capacity of endemic damselfly species (Svenning and Skov, 2007; Schuldt and Assmann, 2009; Zhao et al., 2020a) keeps them close to areas near the water; thus, their endemism is highly associated with water availability. Our results, together with previous findings, imply that the formation mechanisms of endemism patterns between semiaquatic and terrestrial insects are different. Therefore, the habitats of taxonomic groups should be considered before drawing conclusions on the driving mechanisms underlying endemism patterns.
To effectively protect species diversity, conservationists focused on identifying priority areas for conservation (Tang et al., 2006; Zhang et al., 2015b; Huang et al., 2016; Hu et al., 2017; Xie et al., 2021; Xia et al., 2022). Species diversity is measured using several metrics, with species richness and endemism being the most commonly used metrics for developing conservation strategies (Zizka et al., 2019; Noroozi et al., 2019a; Xie et al., 2021; Moreira-Munoz et al., 2022; Qin et al., 2022). The spatial congruence of species richness and endemism hotspots is the basis for identifying priority areas for conservation (Orme et al., 2005) but is rarely evaluated in insects. The results of the present study revealed that the species richness and endemism hotspots of North American damselflies demonstrated a low degree of spatial congruence. This result was consistent with that of previous studies conducted on birds (Orme et al., 2005) and terrestrial vertebrates worldwide (Lamoreux et al., 2006) as well as on plants in Australia (González-Orozco et al., 2011), indicating the low congruence of the species richness and endemism hotspots in different taxonomic groups. Other studies have shown that the low number of grids defining hotspots show low congruence among hotspots (Feng et al., 2011; Zhao et al., 2016); however, this cannot be considered an actual reason. One reasonable explanation for this is that the most vital environmental variable in regulating species richness and endemism patterns differs (representing different formation mechanisms), as shown in the present study. The low congruence among hotspots prompted us to integrate two types of hotspots to determine four species diversity hotspots for damselflies across North America (Figure 4) that contain a large number of species and endemic species. Although a large number of protected areas have been currently established in North America, they have not effectively protected North American damselflies (Figure 5). Three out of the four hotspots had grids with conservation gaps, i.e., the region of the eastern United States and southeastern Canada, southwestern United States, and central Mexico (Figure 5), showing that the existing protected areas of these hotspot areas are insufficient. Furthermore, a previous study demonstrated the distribution patterns of extinction risk for North American damselflies (Rocha-Ortega et al., 2020). In the present study, we identified that the hotspot grids having conservation gaps were located in high extinction regions identified in the abovementioned study. In addition, three threatened species proposed in the IUCN Red List were also found in the hotspot grids with conservation gaps, i.e., Paraphlebia zoe, Lestes simplex, and Ischnura gemina. Previous studies have revealed that habitat suitability areas of Paraphlebia zoe and Ischnura gemina decreased in the future (Sánchez-Guillén et al., 2014; Cuevas-Yáñez et al., 2015), indicating that these species are highly at the risk of extinction. These findings indicate that the pace of establishing protected areas in hotspots areas with conservation gaps should be accelerated.
Conclusion
This study analyzed the key determinants of species diversity, hotspots, and conservation status of North American damselflies. Our results showed that species richness was most strongly affected by temperature. However, endemism patterns were strongly associated with water availability, which is inconsistent with the findings of previous studies on terrestrial insects. Therefore, we propose considering the habitats of taxonomic groups for understanding the mechanisms underlying endemism patterns. Our results also revealed that the low spatial congruence among species richness and endemism hotspots is attributable to differences in their dominant driving mechanisms. Furthermore, four species diversity hotspots (conservation priority areas) were identified; these hotspots should be given the highest attention while developing conservation strategies. This large conservation gap also indicates the need to accelerate the establishment of protected areas.
Data availability statement
The original contributions presented in the study are included in the article/supplementary material, further inquiries can be directed to the corresponding author.
Author contributions
ZZha: manuscript preparing, writing, and revising. XF: data analysis. YZ, YW, and ZZho: distribute data collection. All authors read the text, provided comments, suggestions and corrections, approved the final version, contributed to the article, and approved the submitted version.
Funding
This research was funded by the scientific research platform of Education Department of Guizhou Province (Qianjiaoji [2022] 052).
Conflict of interest
The authors declare that the research was conducted in the absence of any commercial or financial relationships that could be construed as a potential conflict of interest.
Publisher’s note
All claims expressed in this article are solely those of the authors and do not necessarily represent those of their affiliated organizations, or those of the publisher, the editors and the reviewers. Any product that may be evaluated in this article, or claim that may be made by its manufacturer, is not guaranteed or endorsed by the publisher.
Footnotes
References
Ball-Damerow, J. E., Oboyski, P. T., and Resh, V. H. (2015). California dragonfly and damselfly (Odonata) database: temporal and spatial distribution of species records collected over the past century. Zookeys 482, 67–89. doi: 10.3897/zookeys.482.8453
Brown, J. H. (2014). Why are there so many species in the tropics? J. Biogeogr. 41, 8–22. doi: 10.1111/jbi.12228
Bybee, S. M., Kalkman, V. J., Erickson, R. J., Frandsen, P. B., Breinholt, J. W., Suvorov, A., et al. (2021). Phylogeny and classification of Odonata using targeted genomics. Mol. Phylogenet. Evol. 160:107115. doi: 10.1016/j.ympev.2021.107115
Ceballos, G., and Ehrlich, P. R. (2006). Global mammal distributions, biodiversity hotspots, and conservation. P. Natl. Acad. Sci. U. S. A. 103, 19374–19379. doi: 10.1073/pnas.0609334103
Chi, X. L., Zhang, Z. J., Xu, X. T., Zhang, X. B., Zhao, Z. P., Liu, Y. N., et al. (2017). Threatened medicinal plants in China: distributions and conservation priorities. Biol. Conserv. 210, 89–95. doi: 10.1016/j.biocon.2017.04.015
Clarke, A., and Gaston, K. J. (2006). Climate, energy and diversity. P. Roy. Soc. B Biol. Sci. 273, 2257–2266. doi: 10.1098/rspb.2006.3545
Clausnitzer, V., Kalkman, V. J., Ram, M., Collen, B., Baillie, J. E., Bedjanič, M., et al. (2009). Odonata enter the biodiversity crisis debate: the first global assessment of an insect group. Biol. Conserv. 142, 1864–1869. doi: 10.1016/j.biocon.2009.03.028
Collen, B., Whitton, F., Dyer, E. E., Baillie, J. E., Cumberlidge, N., Darwall, W. R., et al. (2014). Global patterns of freshwater species diversity, threat and endemism. Glob. Ecol. Biogeogr. 23, 40–51. doi: 10.1111/geb.12096
Corbet, P. S., Suhlinhg, F., and Sondgerath, D. (2006). Voltinism of Odonata: a review. Int. J. Odonatol. 9, 1–44. doi: 10.1080/13887890.2006.9748261
Cuevas-Yáñez, K., Rivas, M., Muñoz, J., and Córdoba-Aguilar, A. (2015). Conservation status assessment of Paraphlebia damselflies in Mexico. Insect Conserv. Diver. 8, 517–524. doi: 10.1111/icad.12132
Daru, B. H., van Der Bank, M., and Davies, T. J. (2015). Spatial incongruence among hotspots and complementary areas of tree diversity in southern Africa. Divers. Distrib. 21, 769–780. doi: 10.1111/ddi.12290
Dijkstra, K. D. B., Bechly, G., Bybee, S. M., Dow, R. A., Dumont, H. J., Fleck, G., et al. (2013). The classification and diversity of dragonflies and damselflies (Odonata). In: Zhang, Z.Q. (Ed.) animal biodiversity: an outline of higher-level classification and survey of taxonomic richness (Addenda 2013). Zootaxa 3703, 36–45. doi: 10.11646/zootaxa.3703.1.9
Dijkstra, K. D. B., Kalkman, V. J., Dow, R. A., Stokvis, F. R., and Van Tol, J. A. N. (2014). Redefining the damselfly families: a comprehensive molecular phylogeny of Zygoptera (Odonata). Syst. Entomol. 39, 68–96. doi: 10.1111/syen.12035
Diniz-Filho, J. A. F., Bini, L. M., and Hawkins, B. A. (2003). Spatial autocorrelation and red herrings in geographical ecology. Glob. Ecol. Biogeogr. 12, 53–64. doi: 10.1046/j.1466-822X.2003.00322.x
Dumont, H. J., Vierstraete, A., and Vanfleteren, J. R. (2010). A molecular phylogeny of the Odonata (Insecta). Syst. Entomol. 35, 6–18. doi: 10.1111/j.1365-3113.2009.00489.x
Dutilleul, P., Clifford, P., Richardson, S., and Hemon, D. (1993). Modifying the t test for assessing the correlation between two spatial processes. Biometrics 49, 305–314. doi: 10.2307/2532625
Feng, G., Ma, Z., Sandel, B., Mao, L., Normand, S., Ordonez, A., et al. (2019). Species and phylogenetic endemism in angiosperm trees across the Northern Hemisphere are jointly shaped by modern climate and glacial–interglacial climate change. Glob. Ecol. Biogeogr. 28, 1393–1402. doi: 10.1111/geb.12961
Feng, J. M., Zhang, Z., and Nan, R. Y. (2011). Non–congruence among hotspots based on three common diversity measures in Yunnan, south–west China. Plant Ecol. Divers. 4, 353–361. doi: 10.1080/17550874.2012.697204
Fine, P. V. (2015). Ecological and evolutionary drivers of geographic variation in species diversity. Annu. Rev. Ecol. Evol. S. 46, 369–392. doi: 10.1146/annurev-ecolsys-112414-054102
Fox, J., Weisberg, S., Price, B., Adler, D., Bates, D., Baud-Bovy, L., et al. (2022). car: companion to Applied Regression. R package version 3.1–0.
González-Orozco, C. E., Laffan, S. W., and Miller, J. T. (2011). Spatial distribution of species richness and endemism of the genus Acacia in Australia. Aust. J. Bot. 59, 601–609. doi: 10.1071/BT11112
Gotelli, N. J., and Colwell, R. K. (2001). Quantifying biodiversity procedures and pitfalls in the measurement and comparison of species richness. Ecol. Lett. 4, 379–391. doi: 10.1046/j.1461-0248.2001.00230.x
Guerin, G. R., Ruokolainen, L., and Lowe, A. J. (2015). A georeferenced implementation of weighted endemism. Methods Ecol. Evol. 6, 845–852. doi: 10.1111/2041-210X.12361
Heywood, V. H., and Dulloo, M. E. (2005). In situ Conservation of Wild Plant Species: A Critical Global Review of Best Practices. IPGRI Technical Bulletin II. IPGRI, Rome.
Hortal, J. (2008). Uncertainty and the measurement of terrestrial biodiversity gradients. J. Biogeogr. 35, 1335–1336. doi: 10.1111/j.1365-2699.2008.01955.x
Hu, R. C., Wen, C., Gu, Y. Y., Wang, H., Gu, L., Shi, X. Y., et al. (2017). A bird's view of new conservation hotspots in China. Biol. Conserv. 211, 47–55. doi: 10.1016/j.biocon.2017.03.033
Huang, J. H., Huang, J. H., Liu, C., Zhang, J. L., Lu, X. H., and Ma, K. P. (2016). Diversity hotspots and conservation gaps for the Chinese endemic seed flora. Biol. Conserv. 198, 104–112. doi: 10.1016/j.biocon.2016.04.007
Jetz, W., and Rahbek, C. (2002). Geographic range size and determinants of avian species richness. Science 297, 1548–1551. doi: 10.1126/science.1072779
Laffan, S. W., and Crisp, M. D. (2003). Assessing endemism at multiple spatial scales, with an example from the Australian vascular flora. J. Biogeogr. 30, 511–520. doi: 10.1046/j.1365-2699.2003.00875.x
Laffan, S. W., Ramp, D., Roger, E., and Ebach, M. (2013). Using endemism to assess representation of protected areas–the family Myrtaceae in the greater Blue Mountains world heritage area. J. Biogeogr. 40, 570–578. doi: 10.1111/jbi.12001
Lamoreux, J. F., Morrison, J. C., Ricketts, T. H., Olson, D. M., Dinerstein, E., McKnight, M. W., et al. (2006). Global tests of biodiversity concordance and the importance of endemism. Nature 440, 212–214. doi: 10.1038/nature04291
Linder, H. (2001). Plant diversity and endemism in sub-Saharan tropical Africa. J. Biogeogr. 28, 169–182. doi: 10.1046/j.1365-2699.2001.00527.x
Liu, Y. P., Shen, Z. H., Wang, Q. G., Su, X. Y., Zhang, W. J., Shrestha, N., et al. (2017). Determinants of richness patterns differ between rare and common species: implications for Gesneriaceae conservation in China. Divers. Distrib. 23, 235–246. doi: 10.1111/ddi.12523
Liu, B., Zhang, M., Bussmann, W. R., Liu, H. M., Liu, Y. Y., Peng, Y. D., et al. (2018a). Species richness and conservation gap analysis of karst areas: a case study of vascular plants from Guizhou, China. Glob. Ecol. Conserv. 16:e00460. doi: 10.1016/j.gecco.2018.e00460
Liu, Y. P., Su, X. Y., Shrestha, N., Xu, X. T., Wang, S. Y., Li, Y. Q., et al. (2018b). Effects of contemporary environment and quaternary climate change on drylands plant diversity differ between growth forms. Ecography 42, 334–345. doi: 10.1111/ecog.03698
López-Pujol, J., Zhang, F. M., Sun, H. Q., Ying, T. S., and Ge, S. (2011). Centres of plant endemism in China: places for survival or for speciation? J. Biogeogr. 38, 1267–1280. doi: 10.1111/j.1365-2699.2011.02504.x
Luo, Z. H., Tang, S. H., Li, C. W., Fang, H. X., Hu, H. J., Yang, J., et al. (2012). Environmental effects on vertebrate species richness: testing the energy, environmental stability and habitat heterogeneity hypotheses. PLoS One 7:e35514. doi: 10.1371/journal.pone.0035514
Luo, Z. H., Wei, S. C., Zhang, W., Zhao, M., and Wu, H. (2015). Amphibian biodiversity congruence and conservation priorities in China: integrating species richness, endemism, and threat patterns. Biol. Conserv. 191, 650–658. doi: 10.1016/j.biocon.2015.08.028
Moreira-Munoz, A., Palchetti, M. V., Morales-Fierro, V., Duval, V. S., Allesch-Villalobos, R., and Gonzalez-Orozco, C. E. (2022). Diversity and conservation gap analysis of the Solanaceae of southern South America. Front. Plant Sci. 13:854372. doi: 10.3389/fpls.2022.854372
Myers, N., Mittermeier, R. A., Mittermeier, C. G., da Fonseca, G. A., and Kent, J. (2000). Biodiversity hotspots for conservation priorities. Nature 403, 853–858. doi: 10.1038/35002501
Nair, M. V. (2011). Dragonflies & Damselflies of Orissa and Eastern India, Wildlife Organisation, Forest & Environment Department, Orissa.
Noroozi, J., Zare, G., Sherafati, M., Mahmoodi, M., Moser, D., Asgarpour, Z., et al. (2019a). Patterns of endemism in Turkey, the meeting point of three global biodiversity hotspots, based on three diverse families of vascular plants. Front. Ecol. Evol. 7:159. doi: 10.3389/fevo.2019.00159
Noroozi, J., Naqinezhad, A., Talebi, A., Doostmohammadi, M., Plutzar, C., Rumpf, S. B., et al. (2019b). Hotspots of vascular plant endemism in a global biodiversity hotspot in Southwest Asia suffer from significant conservation gaps. Biol. Conserv. 237, 299–307. doi: 10.1016/j.biocon.2019.07.005
Olea, P. P., Mateo-Tomás, P., and de Frutos, Á. (2010). Estimating and modelling bias of the hierarchical partitioning public-domain software: implications in environmental management and conservation. PLoS One 5:e11698. doi: 10.1371/journal.pone.0011698
Orme, C. D., Davies, R. G., Burgess, M., Eigenbrod, F., Pickup, N., Olson, V. A., et al. (2005). Global hotspots of species richness are not congruent with endemism or threat. Nature 436, 1016–1019. doi: 10.1038/nature03850
Osorio, F., Vallejos, R., Cuevas, F., and Mancilla, D. (2022). SpatialPack: tools for assessment the association between two spatial processes. R package version 0.4.
Pickup, J., and Thompson, D. J. (1984). The effects of prey density and temperature on development of larvae of the damselfly Lestes sponsa (Hans.) (Zygoptera: Lestidae). Adv. Odonatolog. 15, 169–176.
Qin, F., Xue, T., Yang, X., Zhang, W., Wu, J., Huang, Y., et al. (2022). Conservation status of threatened land plants in China and priority sites for better conservation targets: distribution patterns and conservation gap analysis. Biodivers. Conserv. 31, 2063–2082. doi: 10.1007/s10531-022-02414-9
R Core Team (2022). R: A Language and Environment for Statistical Computing. R Foundation for Statistical Computing, Vienna, Austria.
Rivard, D., Pilon, J. G., and Thiphrakesone, S. (1975). Effect of constant temperature on egg development of Enallagma boreale selys (Zygoptera: Coenagrionidae). Odonatologica 4, 271–276.
Rocha-Ortega, M., Rodríguez, P., Bried, J., Abbott, J., and Córdoba-Aguilar, A. (2020). Why do bugs perish? Range size and local vulnerability traits as surrogates of Odonata extinction risk. P. Roy. Soc. B. Biol. Sci. 287:20192645. doi: 10.1098/rspb.2019.2645
Romo, H., and García-Barros, E. (2010). Biogeographic regions of the Iberian Peninsula: butterflies as biogeographical indicators. J. Zool. 282, 180–190. doi: 10.1111/j.1469-7998.2010.00730.x
Sánchez-Fernández, D., Lobo, J. M., Abellán, P., Ribera, I., and Millán, A. (2008). Bias in freshwater biodiversity sampling: the case of Iberian water beetles. Divers. Distrib. 14, 754–762. doi: 10.1111/j.1472-4642.2008.00474.x
Sánchez-Guillén, R. A., Munoz, J., Hafernik, J., Tierney, M., Rodriguez-Tapia, G., and Córdoba-Aguilar, A. (2014). Hybridization rate and climate change: are endangered species at risk? J. Insect Conserv. 18, 295–305. doi: 10.1007/s10841-014-9637-5
Sandel, B., Arge, L., Dalsgaard, B., Davies, R. G., Gaston, K. J., Sutherland, W. J., et al. (2011). The influence of late quaternary climate-change velocity on species endemism. Science 334, 660–664. doi: 10.1126/science.1210173
Schuldt, A., and Assmann, T. (2009). Environmental and historical effects on richness and endemism patterns of carabid beetles in the western Palaearctic. Ecography 32, 705–714. doi: 10.1111/j.1600-0587.2009.05763.x
Schuldt, A., and Assmann, T. (2011). Belowground carabid beetle diversity in the western Palaearctic-effects of history and climate on range–restricted taxa (Coleoptera, Carabidae). Zookeys 100, 461–474. doi: 10.3897/zookeys.100.1540
Shrestha, N., Tiwari, A., and Paudel, P. K. (2021). Assessing conservation priorities of endemic seed plants in the central Himalaya (Nepal): a complementarity and phylogenetic diversity approach. Biol. Conserv. 261:109274. doi: 10.1016/j.biocon.2021.109274
Suvorov, A., Scornavacca, C., Fujimoto, M. S., Bodily, P., Clement, M., Crandall, K. A., et al. (2022). Deep ancestral introgression shapes evolutionary history of dragonflies and damselflies. Syst. Biol. 71, 526–546. doi: 10.1093/sysbio/syab063
Svenning, J. C., and Skov, F. (2007). Ice age legacies in the geographical distribution of tree species richness in Europe. Glob. Ecol. Biogeogr. 16, 234–245. doi: 10.1111/j.1466-8238.2006.00280.x
Tang, Z. Y., Wang, Z. H., Zheng, C. Y., and Fang, J. Y. (2006). Biodiversity in China's mountains. Front. Ecol. Environ. 4, 347–352. doi: 10.1890/1540-9295(2006)004[0347:BICM]2.0.CO;2
Turner, J. R. G., Gatehouse, C. M., and Corey, C. A. (1987). Does solar energy control organic diversity? Butterflies, moths and the British climate. Oikos 48, 195–205. doi: 10.2307/3565855
Turner, J. R. G., Lennon, J. J., and Lawrenson, J. A. (1998). British bird species distributions and the energy theory. Nature 335, 539–541. doi: 10.1038/335539a0
Turner, J. R. G. (2004). Explaining the global biodiversity gradient: energy, area, history and natural selection. Basic. Appl. Ecol. 435–448. doi: 10.1016/j.baae.2004.08.004
Ver Hoef, J. M., and Boveng, P. L. (2007). Quasi-poisson vs. negative binomial regression: how should we model overdispersed count data? Ecology 88, 2766–2772. doi: 10.1890/07-0043.1
Walsh, C., and Mac Nally, R. (2020). hier. part: Hierarchical partitioning. R package version 1.0–6.
Wang, Z., Brown, J. H., Tang, Z., and Fang, J. (2009). Temperature dependence, spatial scale, and tree species diversity in eastern Asia and North America. P. Natl. Acad. Sci. U.S.A. 106, 13388–13392. doi: 10.1073/pnas.0905030106
Wang, Z. H., Fang, J. Y., Tang, Z. Y., and Lin, X. (2011). Patterns, determinants and models of woody plant diversity in China. P. Roy. Soc. B. Biol. Sci. 278, 2122–2132. doi: 10.1098/rspb.2010.1897
Xia, C., Huang, Y., Qi, Y., Yang, X., Xue, T., Hu, R., et al. (2022). Developing long–term conservation priority planning for medicinal plants in China by combining conservation status with diversity hotspot analyses and climate change prediction. BMC Biol. 20:89. doi: 10.1186/s12915-022-01285-4
Xie, D., Liu, X. Q., Chen, Y. X., Jiao, D., Lou, J. X., Qiu, X. F., et al. (2021). Distribution and conservation of threatened gymnosperms in China. Glob. Ecol. Conserv. 32:e01915. doi: 10.1016/j.gecco.2021.e01915
Xie, H., Tang, Y., Fu, J., Chi, X., Du, W., Dimitrov, D., et al. (2022). Diversity patterns and conservation gaps of Magnoliaceae species in China. Sci. Total Environ. 813:152665. doi: 10.1016/j.scitotenv.2021.152665
Xu, H. G., Cao, M. C., Wu, J., Cai, L., Ding, H., Lei, J. C., et al. (2015). Determinants of mammal and bird species richness in China based on habitat groups. PLoS One 10:e0143996. doi: 10.1371/journal.pone.0143996
Xu, Y., Shen, Z. H., Ying, L. X., Wang, Z. H., Huang, J. H., Zang, R. G., et al. (2017). Hotspot analyses indicate significant conservation gaps for evergreen broadleaved woody plants in China. Sci. Rep. 7:1859. doi: 10.1038/s41598-017-02098-0
Yang, X. D., Liu, B., Bussmann, R. W., Guan, X., Xu, W. B., Xue, T. T., et al. (2021). Integrated plant diversity hotspots and long–term stable conservation strategies in the unique karst area of southern China under global climate change. Forest Ecol. Manag. 498:119540. doi: 10.1016/j.foreco.2021.119540
Yu, F. Y., Skidmore, A. K., Wang, T. J., Huang, J. H., Ma, K. P., and Groen, T. A. (2017). Rhododendron diversity patterns and priority conservation areas in China. Divers. Distrib. 23, 1143–1156. doi: 10.1111/ddi.12607
Yuan, S., Huang, M., Wang, X. S., Ji, L. Q., and Zhang, Y. L. (2014). Centers of endemism and diversity patterns for typhlocybine leafhoppers (Hemiptera: Cicadellidae: Typhlocybinae) in China. Insect Sci. 21, 523–536. doi: 10.1111/1744-7917.12040
Zhang, Z. J., He, J. S., Li, J. S., and Tang, Z. Y. (2015b). Distribution and conservation of threatened plants in China. Biol. Conserv. 192, 454–460. doi: 10.1016/j.biocon.2015.10.019
Zhang, Y. B., Wang, G. Y., Zhuang, H. F., Wang, L. H., Innes, J. L., and Ma, K. P. (2021). Integrating hotspots for endemic, threatened and rare species supports the identification of priority areas for vascular plants in SW China. Forest Ecol. Manag. 484:118952. doi: 10.1016/j.foreco.2021.118952
Zhang, Z. J., Yan, Y. J., Tian, Y., Li, J. S., He, J. S., and Tang, Z. Y. (2015a). Distribution and conservation of orchid species richness in China. Biol. Conserv. 181, 64–72. doi: 10.1016/j.biocon.2014.10.026
Zhao, Z. X., Jin, B. C., Zhou, Z. X., Yang, L., Long, J. K., and Chen, X. S. (2020a). Determinants of Delphacidae richness and endemism in China. Ecol. Entomol. 45, 1396–1407. doi: 10.1111/een.12924
Zhao, L. N., Li, J. Y., Liu, H. Y., and Qin, H. N. (2016). Distribution, congruence, and hotspots of higher plants in China. Sci. Rep. 6:19080. doi: 10.1038/srep19080
Zhao, Z. X., Yang, L., Long, J. K., Chang, Z. M., Zhou, Z. X., Zhi, Y., et al. (2020b). Testing seven hypotheses to determine what explains the current planthopper (Fulgoridae) geographical and species richness patterns in China. Insects 11:892. doi: 10.3390/insects11120892
Keywords: endemism, environmental variables, hotspots, species richness, Zygoptera
Citation: Zhao Z, Feng X, Zhang Y, Wang Y and Zhou Z (2023) Species diversity, hotspot congruence, and conservation of North American damselflies (Odonata: Zygoptera). Front. Ecol. Evol. 10:1087866. doi: 10.3389/fevo.2022.1087866
Edited by:
Daniel de Paiva Silva, Goiano Federal Institute (IFGOIANO), BrazilReviewed by:
Alex Cordoba-Aguilar, National Autonomous University of Mexico, MexicoKim Medley, Washington University in St. Louis, United States
Copyright © 2023 Zhao, Feng, Zhang, Wang and Zhou. This is an open-access article distributed under the terms of the Creative Commons Attribution License (CC BY). The use, distribution or reproduction in other forums is permitted, provided the original author(s) and the copyright owner(s) are credited and that the original publication in this journal is cited, in accordance with accepted academic practice. No use, distribution or reproduction is permitted which does not comply with these terms.
*Correspondence: Zhengxue Zhao, ✉ enp4NjExMzI0QDE2My5jb20=