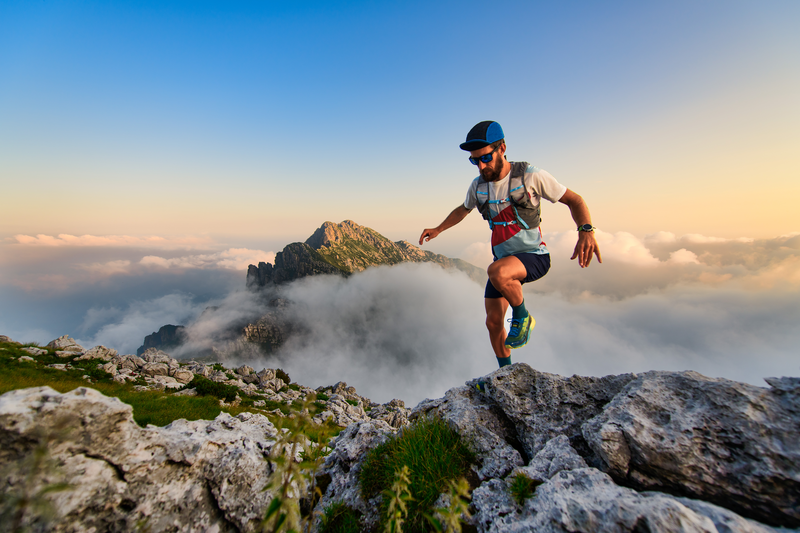
95% of researchers rate our articles as excellent or good
Learn more about the work of our research integrity team to safeguard the quality of each article we publish.
Find out more
REVIEW article
Front. Ecol. Evol. , 14 December 2022
Sec. Evolutionary Developmental Biology
Volume 10 - 2022 | https://doi.org/10.3389/fevo.2022.1082639
This article is part of the Research Topic Reciprocal Illumination and Consilience in Plant Evolutionary-Development View all 8 articles
The Gnetales are one of the most fascinating groups within seed plants. Although the advent of molecular phylogenetics has generated some confidence in their phylogenetic placement of Gnetales within seed plants, their macroevolutionary history still presents many unknowns. Here, we review the reasons for such unknowns, and we focus the discussion on the presence of “long branches” both in their molecular and morphological history. The increased rate of molecular evolution and genome instability as well as the numerous unique traits (both reproductive and vegetative) in the Gnetales have been obstacles to a better understanding of their evolution. Moreover, the fossil record of the Gnetales, though relatively rich, has not yet been properly reviewed and investigated using a phylogenetic framework. Despite these apparent blocks to progress we identify new avenues to enable us to move forward. We suggest that a consilience approach, involving different disciplines such as developmental genetics, paleobotany, molecular phylogenetics, and traditional anatomy and morphology might help to “break” these long branches, leading to a deeper understanding of this mysterious group of plants.
Among the extant groups of gymnosperms [Acrogymnospermae sensu Cantino et al. (2007)], Gnetales are probably the most fascinating as well as the most misunderstood. The three genera Ephedra Tourn. ex L., Welwitschia Hook. f., and Gnetum L. (Figures 1A–C) have been at the center of the debate around the evolution of seed plants since the beginning of evolutionary thought. In particular, they were thought to be one of the keys for understanding the origin and the early evolution of angiosperms (Arber and Parkin, 1907). This focus was renewed after the first cladistic analyses based on morphological data retrieved a clade including Gnetales and flowering plants as well as a few extinct taxa, termed the “Anthophyte hypothesis” (Crane, 1985; Doyle and Donoghue, 1986, 1992; Rothwell and Serbet, 1994; Doyle, 1998).
Figure 1. (A–C) Extant Gnetales, Ephedra (A, Ephedra viridis Coville, White Mountains, California, USA), Welwitschia (B, Welwitschia mirabilis Hook. f., Namibia) and Gnetum (C, Gnetum gnemon L., grown at the Hortus Bergianus, Stockholm, Sweden). (D) Alternative topologies retrieved for the placement of Gnetales within seed plants.
Later analyses based on molecular data, however, undermined the support for the anthophyte hypothesis (Qiu et al., 1999; Donoghue and Doyle, 2000), with such a topology only found in a few analyses with extremely low support (Rydin et al., 2002). Instead, molecular data supported either phylogenies with Gnetales as sister to the other seed plants (“Gnetales sister”) (Rydin et al., 2002; Chen et al., 2016), Gnetales as sister to Pinaceae (“Gnepine”) (Bowe et al., 2000; Chaw et al., 2000; Zhong et al., 2010; Ran et al., 2018; Smith et al., 2020), and Gnetales as sister to cupressophytes (i.e., the rest of the conifers) (“Gnecup”) (Gitzendanner et al., 2018; Li et al., 2019). A few analyses support a topology with Gnetales sister to monophyletic conifers (“Gnetifers”) (Rydin and Korall, 2009; One Thousand Plant Transcriptomes Initiative., 2019; Majeed et al., 2021; Figure 1D). A review of the phylogenetic placement of the Gnetales and an update classification of the gymnosperms have been recently produced (Yang et al., 2022).
It was hoped that a consensus about the phylogenetic placement of the Gnetales among the Acrogymnospermae would “solve” the mystery of their evolutionary history. However, the rejection of the Anthophyte hypothesis and its variations (Crane, 1996; Doyle, 1996; Friis et al., 2007) has only deepened the perceived morphological chasm between Gnetales and their gymnosperm relatives. Moreover, the enormous morphological diversity (disparity) between the extant genera further complicates any attempt at understanding the evolution of morphology and ecology within Gnetales (Ickert-Bond and Renner, 2016).
Here, firstly we review the main issues currently hindering our understanding of the evolution of the Gnetales. Then we propose a multidisciplinary approach to better understand their evolutionary history.
Our understanding of the relationships and evolutionary history of the Gnetales has been hindered by the presence of “long branches” in their evolutionary tree. These long branches are often indications of either long unsampled evolutionary histories, due to extensive extinction and/or a lack of an adequate fossil record; an increase in the rate of molecular or morphological change, potentially associated with phenomena such as neoteny; or a combination of both. In phylogenetic analyses and other phylogenetic applications such as molecular dating or ancestral state reconstruction, long branches pose many challenges. When inferring trees, clades or taxa subtended by long branches might be artificially grouped together [Long-Branch Attraction (Felsenstein, 1978), reviewed in Bergsten (2005)], both when using molecular (Klimov et al., 2018) and morphological data (Coiro et al., 2018). This is particularly apparent when using parsimony but can also arise with other methods. The problem of long-branch attraction is caused by the large among-lineage rate heterogeneity needed to accommodate long branched groups in molecular dating analysis. So even when clock assumptions are relaxed, it might lead to imprecise and even inaccurate estimates of the ages of the resulting dated tree (Sanderson and Doyle, 2001; Crisp et al., 2014). A long, unsampled morphological history makes any attempt at reconstructing ancestral character states uncertain and potentially inaccurate (Finarelli and Flynn, 2006), and prevents understanding of the sequence of origin of apomorphies and related scenarios.
The Gnetales are affected by long-branch issues both in molecular and morphological trees (Figure 2), although the causes of these long branches have not been investigated in detail. Here, we will discuss these issues in both data categories in more detail. Importantly, we suggest a method to break these long branches to overcome this issue.
Figure 2. Phylograms showing the presence of long branches in the Gnetales. Left, molecular phylogram from the concatenated alignment of the 1st- and 2nd-codon positions of 1,308 orthogroups using the partitioned maximum-likelihood method in RAxML, with each gene treated as one partition, from Ran et al. (2018), trimmed to only include acrogymnosperms. Right, morphological phylogram based on the data from Doyle (2006) analyzed using bayesian analysis as in Coiro et al. (2018). Bars indicate the average number of expected molecular and morphological substitutions per site, respectively.
Molecular long branch attraction has been one of the most important confounding factors in the early analyses of molecular data of the seed plants, affecting both angiosperms and Gnetales. Indeed, the Gnetales are subtended by a substantially long branch both in analysis of single or few loci and in genomic-level analyses (Doyle, 1998; Chaw et al., 2000; Rydin et al., 2002; Ran et al., 2018). Long branch attraction between Gnetales and the long-branched outgroups of the seed plants probably generated the so-called “Gnetales-sister” topologies (Figure 1D), as was suggested by methodological incongruence (i.e., when the results of parsimony vs. maximum likelihood for the same dataset result in different placement of long-branched groups) simulation studies, and signal conflicts between the 1st and 2nd codon position vs. the much more variable and often saturated 3rd position (Sanderson et al., 2000; Magallón and Sanderson, 2002; Burleigh and Mathews, 2004).
Long divergence times between the three genera of the Gnetales, and between Gnetales and the other seed plants, may be a cause of the long branches retrieved in molecular studies. However, the timescale for the evolution of Gnetales is higly uncertain. The oldest divergence, that of Gnetales and their closest relatives (Pinaceae or cupressophytes depending on the study), has been variously inferred to be dated between the Carboniferous and the Triassic (Hou et al., 2015; Ran et al., 2018), while the Gnetales crown age has been inferred to be between Late Triassic and the Early Cretaceous (Hou et al., 2015; Ran et al., 2018; Rydin et al., 2021). The divergence between Gnetum and Welwitschia is inferred to have occurred in the Early Cretaceous. The crown age of Gnetum has been inferred to be as young as the Miocene (Won and Renner, 2006) or as old as the Late Cretaceous (Hou et al., 2015). On the other hand, the extant diversity of Ephedra is inferred to be quite young, with ages retrieved between the Oligocene (Loera et al., 2012) and the Pliocene (Ickert-Bond et al., 2009; Rydin et al., 2021). Even though the times of divergence of the Gnetales from the other seed plants are indeed quite old, groups with similarly inferred old ages (i.e., cycads and Ginkgo) do not share the same long branches. The main explanation given for the presence of a long-branch subtending the Gnetales, but not for cycads and Ginkgo, is a shift in the rate of molecular evolution in the Gnetales compared to the other gymnosperms, which persists within the three genera (Drouin et al., 2008; Wang et al., 2015; Ran et al., 2018).
The recent publication of the genome for Gnetum montanum Markgr. further underlines the molecular distinctiveness of the Gnetales: this Gnetum genome presents signs of elimination of repeated sequences, which accumulated freely in the other sequenced gymnosperms genomes, as well as idiosyncratic expansions and reductions of several gene families (Wan et al., 2018). The elevated molecular rates inferred for Gnetum have been hypothesized to have erased the signal of the common genome duplication preceding the origin of the seed plant (the so-called “zeta” duplication event) (Wan et al., 2018). The genome of Welwitschia further stresses the derived state of the Gnetales, showing signs of both an expansion of long terminal repeat-retrotransposons and an idiosyncratic genome duplication that is not shared with Gnetum (Wan et al., 2021). Currently there are no whole genome sequences for Ephedra, limiting our understanding of genome evolution across the Gnetales.
Although a placement of Gnetales within the extant gymnosperms is strongly supported by recent phylogenies, the long branch subtending the Gnetales hinders the resolution of their accurate placement within Acrogymnospermae. The Gnecup topology may have resulted from long-branch attraction between the previously sparsely sampled cupressophytes and Gnetales (Zhong et al., 2010). The Gnepine hypothesis is thought to be the most accurate as it is the most commonly retrieved topology in recent studies, but the morphological gap between Pinaceae and Gnetales is still considerable. The use of molecular data alone may not provide all the evidence for an exact relationship between the conifers and Gnetales. This may be due to the signal erosion linked with the increased rate of evolution in the lineage leading to crown group Gnetales. Other data must then be incorporated into molecular datasets to overcome this signal limitation.
Even though it has received less attention, the presence of a long branch in morphological trees of Gnetales within seed plants (Coiro et al., 2018) is not surprising. Both the morphological uniqueness of the Gnetales within the seed plants, and of the three genera within Gnetales has been clear since the very beginning of evolutionary thought (Chamberlain, 1935). Many gnetalean traits are only shared with the similarly divergent angiosperms. Even within Gnetales, some considered that the three genera did not form a natural group as today they are so disparate and ecologically distinct (e.g., Eames, 1957). The synapomorphies of the Gnetales are both vegetative and reproductive, and they include characters that evolved in other gymnosperm groups, as well as apparently unique traits.
The opposite-decussate phyllotaxis that is typical of Gnetales (Figures 3A–C) is also found in conifers, particularly Cupressus L., Juniperus L., and related genera in Cupressaceae, Agathis Salisb. in Araucariaceae, as well as some genera in the extinct family Cheirolepidiaceae (Watson and Alvin, 1999). However, the combination of opposite-decussate phyllotaxis and long internodes is unknown within other gymnosperms, but is present in many angiosperm groups (e.g., Chloranthaceae, many parasitic plants in Santalales).
Figure 3. Vegetative traits of the Gnetales. (A) Node of Ephedra fragilis Desf., showing opposite leaves (lf) and branches (lb) as well as the main branch. (B) Welwitschia mirabilis, showing the cotyledons (ct) and the two vegetative leaves (lf) (C) Gnetum montanum, showing opposite lateral branches (lb) (courtesy of James Doyle). (D) Epidermis of Gnetum gnemon showing the paracytic stomata (courtesy of Paula Rudall). (E) Schema of vessel of Ephedra monostachya Turcz. redrawn from Thompson (1918), showing the perforations derived from bordered pits.
Gnetum and Welwitschia present many synapomorphic and autapomorphic vegetative traits that are unique within Acrogymnospermae. In Welwitschia, the only vegetative leaves produced during the plant’s life are linear and multiveined with a basal meristem producing new leaf tissue continuously. In Gnetum, leaves have hierarchical venation and free-ending veins, traits only shared with angiosperms. Peculiar vein anastomoses forming a “herringbone” or chevron pattern are present both in cotyledon and vegetative leaves of Welwitschia and cotyledons of Gnetum. Brachyparacytic stomata with mesogenous subsidiary cells are shared by both genera (Figure 3D; Rudall and Rice, 2019), and are only known from angiosperms and (probably) the extinct Bennettitales (Rudall and Bateman, 2019) within seed plants. The presence of vessels in the wood of Gnetales (Figure 3E) is another trait that is only shared with angiosperms within seed plants. Unlike angiosperms though, the unique vessels in Gnetales originate from tracheids with bordered pits (Thompson, 1918; Bailey, 1944, 1953; Carlquist, 1996, 2012).
All three genera of the Gnetales share the presence of a “micropylar tube” formed by an extension of the integument into a hollow tube. A similar structure is only present in the extinct Bennettitales and in seeds assigned to the Erdtmanithecales, and has been considered to be a synapomorphy of a clade including these three groups [the “BEG” clade, (Pedersen et al., 1989; Friis et al., 2007; Mendes et al., 2008)]. However, other authors consider the presence of this structure in Bennettitales and Gnetales to be convergent, based on histological and organographical considerations (Rothwell et al., 2009; Klymiuk et al., 2022). Within fossil taxa, the presence of a micropylar tube is considered an important apomorphy for the assignment of a specimen to the Gnetales (Yang et al., 2005; Friis et al., 2007; Rydin and Friis, 2010).
In the Gnetales, this micropylar tube is enclosed together with the whole ovule by either one envelope in Ephedra and Welwitschia or two in Gnetum (Figure 4). In the former two genera, this structure is clearly formed by the fusion of opposite bracteoles around a terminal ovule (Bierhorst, 1971; Rydin et al., 2010). The nature of the second envelope in Gnetum, though less evident than the clearly bract-like single envelopes of Ephedra or Welwitschia, is also of a bracteolar nature (Thoday, 1921; Rodin and Kapil, 1969; Takaso and Bouman, 1986; Endress, 1996).
Figure 4. Ovulate reproductive structures and diagrams for the Gnetales. (A,B) Welwitschia mirabilis. (C,D) Ephedra viridis. (E,F) Gnetum gnemon. In the three genera micropylar tubes (mt) extend beyond the ovule integument. In the diagrams, black circles represent ovules, crescents represent bract-like structures, and the two concentric circles represent the two envelopes in Gnetum, while x represents the main axis of the cone.
The morphology and development of the megagametophyte is particularly unique within Gnetales. Even though Ephedra has a typical gymnosperm gametophyte, both the egg cell nucleus and the ventral canal nucleus are fertilized creating two separate zygotes (Friedman, 1990a,b). Both Gnetum and Welwitschia evolved extremely divergent morphologies. In Gnetum, the gametophyte has a tetrasporic initiation, and later presents a micropylar side with coenocytic nuclei arranged around a large central vacuole and a chalazal side with dense cytoplasm and numerous nuclei that cellularize early (Figure 5A; Carmichael and Friedman, 1995; Friedman and Carmichael, 1998). During fertilization, both nuclei of the male gametophyte merge with gametophyte cells, leading to two zygotes developing in to separate embryos. The megagametophyte of Welwitschia, even though it shares the tetrasporic initiation present in Gnetum, lacks a central vacuole during its early development. The nuclei of the gametophyte divide to form a dense coenocyte. After that, cell wall formation leads to separate “cells” with multiple nuclei. On the chalazal side, these nuclei fuse leading to a set of polyploid cells. On the micropylar side, the nuclei remain separated, while the cells elongate toward the micropyle forming “prothallial tubes.” Only one nucleus in this prothallial tube participates in the fertilization (Friedman, 2015). Proembryos are cellular in all three genera [or less ambiguously, they are derived from single nucleate cells (Doyle, 1996)], a trait only present in angiosperms outside the Gnetales (Friedman, 1994).
Figure 5. Other reproductive traits of the Gnetales. (A) Scheme of the megagametophyte in Gnetum. Dark circles represent nuclei, while the central vacuole is highlighted in light blue. (B) Polyplicate, boat shaped dispersed fossil gnetalean pollen recovered from the Early Cretaceous Crato Formation, scale bar = 50 um.
The pollen and the male gametophyte are also rather distinct in Gnetales. The pollen is polyplicate and boat-shaped in Ephedra and Welwitschia [being inaperturate in the former (El-Ghazaly et al., 1998) and sulcate in the latter (Rydin and Friis, 2005)] (Figure 5B), while it is echinate, globular, and inaperturate in Gnetum (Gillespie and Nowicke, 1994). The infratectum of the pollen is granular (Doores et al., 2007; Bolinder et al., 2015), another unusual trait present in some angiosperms. Though Ephedra has a rather typical gymnosperm microgametophyte, the number of nuclei is reduced to four in Welwitschia and Gnetum.
Looking at the amount of unique traits present in the Gnetales, it is not surprising that their relationship with conifers only cemented recently. Long branch attraction between the angiosperm and Gnetales branches is at least partially responsible for the retrieval of anthophyte trees in more recent matrices that include the clear synapomorphies between Gnetales and coniferophytes (Coiro et al., 2018).
Though the presence of long branches in the Gnetales does currently represent a big obstacle in understanding of their macroevolutionary history, different strategies can be employed to try to “break” these branches. Here we summarize the evolutionary-developmental tools that can be used to provide new insights into the evolution of the Gnetales. The origin of the unique traits and their homologies with gymnosperm traits can be tested, for example, using information from development and fine anatomy. One clear example is the independent origin of vessel elements found in Gnetales and those of the angiosperms. A thorough investigation of the morphology and developmental trajectory of vessels in Gnetales revealed that these indeed originated through different developmental trajectories, suggesting convergence with angiosperm vessels (Thompson, 1918; Bailey, 1944, 1953; Carlquist, 1996, 2012).
Study of the genetic basis of development can also offer insights into homology and homoplasy of morphological innovations (Müller and Newman, 2005; Chanderbali et al., 2016; Harrison, 2017). Most previous research on the developmental genetics in Gnetales has focused on the known angiosperm regulators of reproductive characters, in particular the MADS-box transcription factor family involved in organ determination and other reproductive processes in the angiosperms (Gramzow and Theissen, 2010). These investigations have shown that MADS-box for the B and C functions do exist in Gnetales, and they are controlled at least partially by the same regulators as in angiosperms (Moyroud et al., 2017). Heterodimerization of MADS-box regulators has also been shown to happen in Gnetum gnemon (Wang et al., 2010). However, many unknowns remain on the function and activities of MADS-box genes in Gnetales, with even the exact number of MADS-box gene families present in the group being uncertain (Gramzow et al., 2014; Chen et al., 2017; Hou et al., 2020).
Even less attention has been paid to the regulation of vegetative aspects of growth in the Gnetales. This is rather surprising, since the continuously growing leaves of Welwitschia and the angiosperm-like leaves of Gnetum represent innovations that are extremely rare within gymnosperms. The long reproductive cycle of Welwitschia and Gnetum hinders their usefulness as models for reverse genetic approaches that have been successful in non-model, non-seed plants (Plackett et al., 2018; Zheng et al., 2022). On the other hand, Ephedra could be amenable as a model system for molecular developmental genetic studies, given their short reproductive cycles and relatively small genomes compared to other gymnosperms (Di Stilio and Ickert-Bond, 2021).
The fossil record could offer a wealth of intermediate forms that would allow us to break the morphological long branch between Gnetales and their closest gymnosperm relatives. However, the fossil record of the Gnetales, though not particularly poor, presents unique challenges. Pre-Cretaceous macrofossil records of Gnetales have been controversial for a long time. The oldest is the cone Palaeognetaleana auspicia Z.Q. Wang from the Permian of China (Wang, 2004). This cone was described as being bisexual, with polyplicate pollen grains, and having a short integumental tube. However, the preservation of this compression fossil does not allow us to resolve its morphology, and the lack of ultrastructural information on the pollen does not allow us to distinguish between gnetalean and convergent morphologies. The Triassic Masculostrobus-Dechellyia from the Chinle formation (USA) was first described as presenting a few characters that might be synapomorphic for the Gnetales, such as Ephedra-type pollen (Equisetosporites chinleana Daugherty), a decussate phyllotaxis, and the presence of two veins entering the leaves (Ash, 1972). However, later reinvestigations have shown that the pollen of Masculostrobus has substantial ultrastructural differences when compared with gnetalean pollen (Pocock and Vasanthy, 1988). Though recent phylogenetic analysis have shown a closer relationship between the Masculostrobus-Dechellyia plant and the crown group coniferophytes (Herrera et al., 2020), a relationship with the total-group Gnetales has not yet been formally tested.
The Piroconites-Bernettia-Desmiophyllum plant, from the Jurassic of Franconia, Germany, has been associated with the Gnetales based on the retrieval of Ephedripites-like pollen in Piroconites kuespertii Gothan (van Konijnenburg-van Cittert, 1992; Doyle, 1996). However, the ovulate scale Bernettia Gothan does not show many characters with gnetalean affinities, suggesting a more distant relationship with the crown group Gnetales (Kustatscher et al., 2016).
The oldest fossil with clear gnetalean affinity is Dayvaultia tetragona Manchester & Crane, a seed-bearing structure preserved as casts and permineralizations from the Late Jurassic Morrison Formation in the USA (Manchester et al., 2021; Figure 6A). This cone comprises two sets of opposite-decussate bracts surrounding six to eight chlamydospermous seeds arranged in an opposite-decussate manner. However, vegetative data for the plant are currently lacking. Another fossil with potential gnetalean affinities, the cone Bassitheca hoodiorum Manchester & Crane, is also retrieved from the Morrison Formation (Manchester et al., 2022). This taxon is represented by a cone with two opposite terminal ovules that seem to be enclosed by bracts. However, the preservation of this material does not currently allow a confident assignment to Gnetales.
Figure 6. Top: examples of macrofossils with gnetalean affinity. (A) Dayvaultia tetragona from the Late Jurassic Morrison Formation, USA, CT scan of the cone (specimen from Smithsonian Natural History Museum, image provided by Steven Manchester). (B) Itajuba yansanae from the Araripe Basin, Early Cretaceous of Brazil, reproductive structure with probable micropylar tube (mt). Image modified from Figure 4 of Ricardi-Branco et al. (2013) under CC-BY 3.0 license (https://creativecommons.org/licenses/by/3.0/), scale bar = 1 mm. (C) Siphonospermum simplex from the Early Cretaceous Yixian Formation. Image modified from Figure 1 of Rydin and Friis (2010) under CC-BY 2.0 license (https://creativecommons.org/licenses/by/2.0/), scale bar = 1 mm. Bottom: (D) map of localities bearing gnetalean macrofossils. Jurassic locality in light blue, Early Cretaceous localities in green.
The macrofossil record of the Gnetales is particularly numerous in Lower Cretaceous rocks (Figures 6B–D; Krassilov and Bugdaeva, 2000; Rong and Yong, 2003; Rydin et al., 2003, 2006; Dilcher et al., 2005; Yang et al., 2005, 2013, 2015, 2017; Kunzmann et al., 2009, 2011; Rydin and Friis, 2010; Wang and Zheng, 2010; Ricardi-Branco et al., 2013; Liu and Wang, 2015; Yang and Ferguson, 2015; Puebla et al., 2017). Most fossils from these strata are preserved either as impressions or compressions that, even when they represent almost complete plants, offer a limited amount of available characters. Others are exquisitely preserved charcoalified mesofossils that are however, limited to dispersed seeds, In both cases, these fossils offer limited information about many of the traits of the original plants. Moreover, many fossil species are only retrieved from a few Lagerstätten. Among these, the Crato Formation from the Aptian of Brazil (Rydin et al., 2003; Dilcher et al., 2005; Kunzmann et al., 2009) and the Yixian Formation from the Aptian-Albian of China (Rydin et al., 2006; Rydin and Friis, 2010; Yang et al., 2013) account for the majority of the gnetalean macrofossil taxa described.
Among the most promising Early Cretaceous fossils, the permineralized female cone Protoephedrites eamesii Rothwell & Stockey from the Valanginian Apple Bay locality, Vancouver Island (Rothwell and Stockey, 2013) bears two opposite ovules preceded by a pair of decussate bracts, borne on axillary shoots of a decussate, bracteate main axis. The structure of this fossil is incredibly similar to the early stage of an Ephedra-like cone as hypothesized by Eames (1952), and could help to break the morphological long branch subtending the Gnetales. Even in this case though, the fossil does not offer any data on the vegetative structures of the parent plant.
During the Cenozoic, very few gnetalean macrofossils have been identified so far. Ephedra miocenica Wodehouse from the Florissant beds (USA) (Wodehouse, 1934), and Ephedra nudicaulis Saporta from the flora of Aix-en-Provence (France) (Saporta de, 1889), are represented only by fragmentary vegetative material, and thus are only tentatively assigned to Ephedra. This lack of record could be driven by niche conservatism within the three genera. Neither the tropical environments currently inhabited by Gnetum, the desert conditions where Welwitschia is currently found [though its fossil relative might have occupied different environmental conditions, see Ribeiro et al. (2021)], nor the seasonally arid conditions favoured by Ephedra are very conducive to fossilization. On the other hand, the reduced vegetative morphology of Ephedra might lead to misidentification of potential fossils. Indeed, fossils previously assigned to Ephedrites johnianus Göpp. & Berendt by Goeppert and Berendt (1845) have been later identified as members of Loranthaceae (Conwentz, 1886; Sadowski et al., 2017).
The pollen record shows a similar pattern to the macrofossil record with high diversity of distribution of gnetalean pollen during the Early Cretaceous (Crane and Lidgard, 1989), see comprehensive summary in Han et al. (2016). The first reliable fossils of Ephedripites Bolchovitina were described by Klaus (1963: Ephedripites primus Klaus) from the upper Permian of Austria and by Wilson (1962 E. corrugatus Wilson) from the Permian Flower Pot Formation (USA). However, no ultrastructural information is known for these grains, putting into question their assignment to the Gnetales. Both sulcate and inaperturate pollen grains (e.g., Jugella Smirnova = syn Welwitschiapites Bolchovitina ex. Potonié and Ephedripites sp.) have been retrieved from the Upper Jurassic Tendaguru Formation of Tanzania (Schrank, 2010). Pollen morphology within ephedraceaous pollen (i.e., polyplicate, inaperturate) (Figure 5B) during the Early Cretaceous seems also to suggest that different pollination modes (i.e., wind, insect and potentially mixed) were present during this time (Bolinder et al., 2016; Hofmann et al., 2022). Even though the abundance of gnetalean pollen declined in the Late Cretaceous, Ephedra-type pollen has a notable Cenozoic record across the globe, including records from the Eocene to the Pliocene of Brazil (Garcia et al., 2016), central Asia (Tang et al., 2011; Yuan et al., 2020), New Zealand (Lee et al., 2012), Patagonia (Palazzesi and Barreda, 2012), India (Ghosh et al., 1963), Turkey (Akkiraz et al., 2008), Taiwan (Shaw, 1998), North America (Wodehouse, 1933; Gray, 1960), Australia (Cookson, 1956), Europe (Potonié, 1958; Krutzsch, 1961; Grímsson et al., 2011). This is in stark contrast with the lack of macrofossils for this genus during the Cenozoic, further complicating the picture of gnetalean evolution.
When considering the fossil record of the Gnetales, a few key questions remain: what would the oldest gnetalean plants have looked like and would we be able to recognize them as such? The group is characterized by a constellation of morphological characters and, as we detail above, with fragmented natures of their macrofossils. Added to this is the overall current spottiness and unevenness of their fossil record. We mainly rely on the preservation of either isolated reproductive structures or more complete plants bearing them to enable identification. We often cannot assign the assorted “sticks” and stems that can be present in a fossil plant assemblage to any group. Some may represent disarticulated ephedroids for instance, but without diagnostic reproductive features or a suite of vegetative characters, we cannot be certain. Until new productive localities are identified we are very reliant on the handful of Late Jurassic and Early Cretaceous Lagerstätten for our understanding of the group, and so as yet we cannot pinpoint their origin nor their ancestral character states.
However, we do note that the pollen record suggests a greater diversity and wider spread of gnetaleans than evidenced just by their macrofossil record. This is not unusual as plants may have grown in areas with limited preservation potential (often areas away from waterways), whereas pollen can be more easily dispersed by wind and transported to locations with improved preservation potential, thus highlighting bias in the fossil record. This gives hope that new localities bearing as yet unknown gnetalean fossils are yet to be discovered. Interestingly, the pollen record and the macrofossil record for the Gnetales start at around the same time and share the Early Cretaceous peak in diversity with the angiosperms (Crane and Lidgard, 1989; Coiro et al., 2019), although with a subsequent dramatic decrease. Does this suggest that the Gnetales had a truly rapid diversification and spread after their origin, but unlike that proposed for the angiosperms, they could not rise to nor compete with their dominance?
Consilience, or convergence of evidence, is a fundamental principle of epistemology (Whewell, 1840) based on the idea that knowledge and science form a coherent unit. It postulates that evidence from independent fields should converge on similar results, and thus statements can be strongly supported by multiple independent lines of evidence even if each line provides only marginal support. This principle is often at the very base of evolutionary investigations: clear examples are the argumentation of “On The Origin of Species” collecting evidence from natural variation, variation under domestications, biogeography, and the fossil record (Darwin, 1859), as well as the so-called “Modern Synthesys,” where support for mendelian, population-based evolutionary mechanisms came from disparate fields. Some authors have advanced the idea that consilience is at the very base of phylogenetic analysis (Kluge, 1983), and it is still considered an important approach to solve evolutionary relationships even in the phylogenomic age (Rota-Stabelli et al., 2011; Fröbius and Funch, 2017; Marlétaz et al., 2019; Ontano et al., 2021).
In the history of gnetalean research, consilience has been fundamental in understanding the evolutionary history of gnetalean traits. The hypothesis of an independent origin of vessels in Gnetales and Angiosperms has been converged on by many lines of evidence, including comparative anatomy, development, morphological-based phylogenetics, and molecular phylogenetics. We believe that a consilience approach, with multiple independent fields and lines of evidence converging on a similar answer, holds extreme promise for the future of the research on Gnetales.
In this light, the problems of the molecular and morphological long branches found in the Gnetales may not be as permanently intractable as first thought. However, issues might need the convergence of different lines of evidence to reach a solid consensus (Figure 7). The long morphological branch could be “cut” by better primary homology assessment and character history informed by developmental genetics, comparative morphology and anatomy, gene duplication histories, and the fossil record. Similarly, the long molecular branch might be better understood by a better temporal framework for the divergence of the genera provided by the fossil record and by a wider sampling of genomes across Gnetales.
Figure 7. Schema showing the promises of a consilience approach, based on convergence of evidence and reciprocal illumination.
Thus, Gnetales still offer many unexplored research paths for the future. Previously only a few exemplary taxa have been sequenced, but we note the increasing ease and speed of sequencing and assembling genomes from non-model, non-crop organisms promises to open further avenues for phylogenomic and evolutionary research. A broader sampling of gnetalean genomes, especially for Ephedra where we currently have a coverage gap, should improve our understanding of their molecular and morphological peculiarities. This, of course, should be integrated with a clearer understanding of the developmental genetics of Gnetales and other gymnosperms, given that their distance from our angiosperm models could reduce the explanatory power of our current regulatory models. Studies looking at the genetic basis of bract development in Ephedra based on transcriptome analyses (Zumajo-Cardona and Ambrose, 2022) and of the genetic network involved in ovule development in Gnetum (Zumajo-Cardona and Ambrose, 2021) have already shown the promises of such approaches in non-model organisms. Developmental genetics could help us to better understand the homologies of vegetative and reproductive structures in Gnetales, and to identify steps in character evolution that can be validated by the fossil record. The potential development of Ephedra as a new model for reverse genetics (Di Stilio and Ickert-Bond, 2021) offers the possibility of an extremely detailed understanding of its genetics and development.
New promising findings are to be made even in a well-understood and studied field such as gnetalean morphology. Recent studies have demonstrated that there is still much to learn about trait variation and its correlation with environmental conditions (Bolinder et al., 2016). Further investigations in Ephedra and particularly in the poorly treated Gnetum could help us to better grasp the patterns of trait evolution in Gnetales, feeding into primary homology assessment within Gnetales and within seed plants as a whole.
A better understanding of the fossil record of Gnetales and the relationships between extant and fossil taxa is also needed to advance our understanding of the group. Very few attempts have been made to place gnetalean fossils on the phylogeny of the extant genera (Rydin et al., 2003), and a few attempts at reconstructing potential character transition patterns have been attempted (Yang, 2014). However, unlike other gymnosperms, no phylogenetic hypotheses have been formally advanced for the whole fossil record. We believe, however, that integrating fossil Gnetales in the phylogeny of the extant species is a necessary step for contributing to our understanding of the timing, character history, and macroevolutionary dynamics of the group. Recent methods and analyses have shown that even fragmentary fossil material, both reproductive and vegetative, can still carry a substantial amount of phylogenetic and evolutionary signal (Coiro et al., 2018; Mongiardino Koch et al., 2021; Woolley et al., 2022). This is particularly true when data are analyzed with techniques that keep in consideration the uncertainty implicit in phylogenetic analyses (Dávalos et al., 2014; Coiro et al., 2018, 2020; Erdei et al., 2019; Klopfstein and Spasojevic, 2019). An alternative path could involve Total-evidence dating, a technique that has shown promise in its application to other plant clades (May et al., 2021).
A reinvestigation of the potential “stem” relatives in the Permian and Triassic could also lead us closer to filling the gap between conifers and Gnetales. Ultrastructure of some of the earliest pollen, as well as a more detailed investigation of the many macrofossils and the test of their relationships in a phylogenetic framework, could help us to follow the thread of early gnetalean evolution in the early diversification of gymnospermous plants (Rothwell and Stockey, 2016; Herrera et al., 2020; Klymiuk et al., 2022).
In conclusion, the future of Gnetales research is still full of unexpected discoveries, and we are confident that the mystery of the origin of this unique group will soon be unveiled.
MC and LJS contributed to the conception of the review. MC, LJS, ER, and C-CH collected references. MC wrote the first draft and prepared the figures. LJS, ER, and C-CH wrote sections of the manuscript. All authors contributed to manuscript revision, read, and approved the submitted version.
MC has been supported by the Austrian Science Fund (FWF), Lise Meitner project number M3168.
We thank Steven Manchester and the Smithsonian Natural History Museum, Paula Rudall, and Xiao-Quan Wang for providing images for the manuscript. James Doyle was thanked for providing images and comments on the manuscript. We also thank two reviewers for their comments that greatly improved the manuscript.
The authors declare that the research was conducted in the absence of any commercial or financial relationships that could be construed as a potential conflict of interest.
All claims expressed in this article are solely those of the authors and do not necessarily represent those of their affiliated organizations, or those of the publisher, the editors and the reviewers. Any product that may be evaluated in this article, or claim that may be made by its manufacturer, is not guaranteed or endorsed by the publisher.
Akkiraz, M. S., Kayseri, M. S., and Akgün, F. (2008). Palaeoecology of coal-bearing Eocene sediments in central anatolia (Turkey) based on quantitative palynological data. Turkish J. Earth Sci. 17, 317–360.
Arber, E. A. N., and Parkin, J. (1907). On the origin of Angiosperms. J Linn. Soc. Lond. Bot. 38, 29–80. doi: 10.1111/j.1095-8339.1907.tb01074.x
Ash, S. R. (1972). Late Triassic plants from the Chinle formation in north-eastern Arizona. Palaeontology 15, 598–618.
Bailey, I. W. (1944). The development of vessels in angiosperms and its significance in morphological research. Am. J. Bot. 31, 421–428. doi: 10.1002/j.1537-2197.1944.tb08053.x
Bailey, I. W. (1953). Evolution of the tracheary tissue of land plants. Am. J. Bot. 40, 4–8. doi: 10.1002/j.1537-2197.1953.tb06441.x
Bergsten, J. (2005). A review of long-branch attraction. Cladistics 21, 163–193. doi: 10.1111/j.1096-0031.2005.00059.x
Bolinder, K., Niklas, K. J., and Rydin, C. (2015). Aerodynamics and pollen ultrastructure in Ephedra. Am. J. Bot. 102, 457–470. doi: 10.3732/ajb.1400517
Bolinder, K., Norbäck Ivarsson, L., Humphreys, A. M., Ickert-Bond, S. M., Han, F., Hoorn, C., et al. (2016). Pollen morphology of Ephedra (Gnetales) and its evolutionary implications. Grana 55, 24–51. doi: 10.1080/00173134.2015.1066424
Bowe, L. M., Coat, G., and DePamphilis, C. W. (2000). Phylogeny of seed plants based on all three genomic compartments: Extant gymnosperms are monophyletic and Gnetales’ closest relatives are conifers. Proc. Natl. Acad. Sci. 97, 4092–4097. doi: 10.1073/pnas.97.8.4092
Burleigh, J. G., and Mathews, S. (2004). Phylogenetic signal in nucleotide data from seed plants: Implications for resolving the seed plant tree of life. Am. J. Bot. 91, 1599–1613. doi: 10.3732/ajb.91.10.1599
Cantino, P. D., Doyle, J. A., Graham, S. W., Judd, W. S., Olmstead, R. G., Soltis, D. E., et al. (2007). Towards a phylogenetic nomenclature of Tracheophyta. Taxon 56, E1–E44. doi: 10.1002/tax.563001
Carlquist, S. (1996). Wood, bark, and stem anatomy of Gnetales: A summary. Int. J. Plant Sci. 157:S58. doi: 10.1086/297404
Carlquist, S. (2012). Wood anatomy of Gnetales in a functional, ecological, and evolutionary context. Aliso A J. Syst. Evolu. Bot. 30, 33–47. doi: 10.5642/aliso.20123001.05
Carmichael, J. S., and Friedman, W. E. (1995). Double Fertilization in Gnetum gnemon: The relationship between the cell cycle and sexual reproduction. Plant cell 7, 1975–1988. doi: 10.1105/tpc.7.12.1975
Chamberlain, C. J. (1935). Gymnosperms. Structure and evolution. Chicago: University of Chicago Press.
Chanderbali, A. S., Berger, B. A., Howarth, D. G., Soltis, P. S., and Soltis, D. E. (2016). Evolving ideas on the origin and evolution of flowers: New perspectives in the genomic era. Genetics 202, 1255–1265. doi: 10.1534/genetics.115.182964
Chaw, S. M., Parkinson, C. L., Cheng, Y., Vincent, T. M., and Palmer, J. D. (2000). Seed plant phylogeny inferred from all three plant genomes: Monophyly of extant gymnosperms and origin of Gnetales from conifers. Proc. Natl. Acad. Sci. 97, 4086–4091. doi: 10.1073/pnas.97.8.4086
Chen, F., Zhang, X., Liu, X., and Zhang, L. (2017). Evolutionary analysis of MIKCc-type MADS-box genes in gymnosperms and angiosperms. Front. Plant Sci. 8:895. doi: 10.3389/fpls.2017.00895
Chen, Z.-D., Yang, T., Lin, L., Lu, L.-M., Li, H.-L., Sun, M., et al. (2016). Tree of life for the genera of Chinese vascular plants. J. Syst. Evolut. 54, 277–306. doi: 10.1111/jse.12219
Coiro, M., Chomicki, G., and Doyle, J. A. (2018). Experimental signal dissection and method sensitivity analyses reaffirm the potential of fossils and morphology in the resolution of the relationship of angiosperms and Gnetales. Paleobiology 44, 490–510. doi: 10.1017/pab.2018.23
Coiro, M., Doyle, J. A., and Hilton, J. (2019). How deep is the conflict between molecular and fossil evidence on the age of angiosperms? New Phytol. 223, 83–99. doi: 10.1111/nph.15708
Coiro, M., Martínez, L. C. A., Upchurch, G. R., and Doyle, J. A. (2020). Evidence for an extinct lineage of angiosperms from the Early Cretaceous of Patagonia and implications for the early radiation of flowering plants. New Phytol. 228, 344–360. doi: 10.1111/nph.16657
Cookson, I. C. (1956). Pollen grains of the Ephedra type in Australian tertiary deposits. Nature 177, 47–48. doi: 10.1038/177047a0
Crane, P. R. (1985). Phylogenetic relationships in seed plants. Cladistics 1, 329–348. doi: 10.1111/j.1096-0031.1985.tb00432.x
Crane, P. R. (1996). The fossil history of the Gnetales. Int. J. Plant Sci. 157, 50–57. doi: 10.1086/297403
Crane, P. R., and Lidgard, S. (1989). Angiosperm diversification and paleolatitudinal gradients in cretaceous floristic diversity. Science 246, 675–678. doi: 10.1126/science.246.4930.675
Crisp, M. D., Hardy, N. B., and Cook, L. G. (2014). Clock model makes a large difference to age estimates of long-stemmed clades with no internal calibration: A test using Australian grasstrees. BMC Evolut. Biol. 14:263. doi: 10.1186/s12862-014-0263-3
Dávalos, L. M., Velazco, P. M., Warsi, O. M., Smits, P. D., and Simmons, N. B. (2014). Integrating incomplete fossils by isolating conflicting signal in saturated and non-independent morphological characters. Syst. Biol. 63, 582–600. doi: 10.1093/sysbio/syu022
Di Stilio, V. S., and Ickert-Bond, S. M. (2021). Ephedra as a gymnosperm evo-devo model lineage. Evolut. Dev. 23, 256–266. doi: 10.1111/ede.12370
Dilcher, D. L., Bernardes-de-Oliveira, M. E. C., Pons, D., and Lott, T. A. (2005). Welwitschiaceae from the Lower Cretaceous of northeastern Brazil. Am. J. Bot. 92, 1294–1310. doi: 10.3732/ajb.92.8.1294
Donoghue, M. J., and Doyle, J. A. (2000). Seed plant phylogeny: Demise of the anthophyte hypothesis? Curr. Biol. 10, R106–R109. doi: 10.1016/S0960-9822(00)00304-3
Doores, A., Osborn, J., and El-Ghazaly, G. (2007). Pollen ontogeny in Ephedra americana (Gnetales). Int. J. Plant 168, 985–997. doi: 10.1086/518839
Doyle, J. A. (1996). Seed plant phylogeny and the relationships of Gnetales. Int. J. Plant Sci. 157, S3–S39. doi: 10.1086/297401
Doyle, J. A. (1998). Molecules, morphology, fossils, and the relationship of angiosperms and Gnetales. Mol. Phylogenet. Evolut. 9, 448–462. doi: 10.1006/mpev.1998.0506
Doyle, J. A. (2006). Seed ferns and the origin of angiosperms. J. Torrey Bot. Soc. 133, 169–209. doi: 10.3159/1095-5674(2006)133[169:SFATOO]2.0.CO;2
Doyle, J. A., and Donoghue, M. J. (1986). Seed plant phylogeny and the origin of angiosperms: An experimental cladistic approach. Bot. Rev. 52, 321–431. doi: 10.1007/BF02861082
Doyle, J. A., and Donoghue, M. J. (1992). Fossils and seed plant phylogeny reanalyzed. Brittonia 44, 89–106. doi: 10.2307/2806826
Drouin, G., Daoud, H., and Xia, J. (2008). Relative rates of synonymous substitutions in the mitochondrial, chloroplast and nuclear genomes of seed plants. Mol. Phylogenet. Evolut. 49, 827–831. doi: 10.1016/j.ympev.2008.09.009
Eames, A. J. (1957). Some aspects of progress in plant morphology during the past fifty years. Am. J. Bot. 44, 100–104. doi: 10.1002/j.1537-2197.1957.tb08216.x
El-Ghazaly, G., Rowley, J., and Hesse, M. (1998). Polarity, aperture condition and germination in pollen grains of Ephedra (Gnetales). Plant Syst. Evolut. 213, 217–231. doi: 10.1007/BF00985202
Endress, P. K. (1996). Structure and function of female and bisexual organ complexes in Gnetales. Int. J. Plant Sci. 157, S113–S125. doi: 10.1086/297407
Erdei, B., Coiro, M., Miller, I., Johnson, K. R., Griffith, M. P., and Murphy, V. (2019). First cycad seedling foliage from the fossil record and inferences for the Cenozoic evolution of cycads. Biol. Lett. 15:20190114. doi: 10.1098/rsbl.2019.0114
Felsenstein, J. (1978). Cases in which parsimony or compatibility methods will be positively misleading. Syst. Biol. 27:401. doi: 10.2307/2412923
Finarelli, J. A., and Flynn, J. J. (2006). Ancestral state reconstruction of body size in the Caniformia (Carnivora, Mammalia): The effects of incorporating data from the fossil record. Syst. Biol. 55, 301–313. doi: 10.1080/10635150500541698
Friedman, W. E. (1990a). Double fertilization in Ephedra, a nonflowering seed plant: Its bearing on the origin of angiosperms. Science 247, 951–954. doi: 10.1126/science.247.4945.951
Friedman, W. E. (1990b). Sexual reproduction in Ephedra nevadensis (Ephedraceae): Further evidence of double fertilization in a nonflowering seed plant. Am. J. Bot. 77, 1582–1598. doi: 10.1002/j.1537-2197.1990.tb11399.x
Friedman, W. E. (1994). The evolution of embryogeny in seed plants and the developmental origin and early history of endosperm. Am. J. Bot. 81, 1468–1486. doi: 10.1002/j.1537-2197.1994.tb15633.x
Friedman, W. E. (2015). Development and evolution of the female gametophyte and fertilization process in Welwitschia mirabilis (Welwitschiaceae). Ame. J. Bot. 102, 312–324. doi: 10.3732/ajb.1400472
Friedman, W. E., and Carmichael, J. S. (1998). Heterochrony and developmental innovation: Evolution of female gametophyte ontogeny in Gnetum, a highly apomorphic seed plant. Evolution 52, 1016–1030. doi: 10.1111/j.1558-5646.1998.tb01830.x
Friis, E. M., Crane, P. R., Pedersen, K. R., Bengtson, S., Donoghue, P. C. J., Grimm, G. W., et al. (2007). Phase-contrast X-ray microtomography links Cretaceous seeds with Gnetales and Bennettitales. Nature 450, 549–552. doi: 10.1038/nature06278
Fröbius, A. C., and Funch, P. (2017). Rotiferan Hox genes give new insights into the evolution of metazoan bodyplans. Nat. Commun. 8, 1–10. doi: 10.1038/s41467-017-00020-w
Garcia, M. J., Premaor, E., Oliveira, P. E. D., Bernardes-de-Oliveira, M. E. C., Dino, R., Antonioli, L., et al. (2016). Cenozoic distribution of Ephedripites Bolkhovitina (1953) ex Potonié (1958) emend. Krutzsch (1961) in Brazil. Grana 55, 52–70. doi: 10.1080/00173134.2015.1119882
Ghosh, A., Banerji, D., and Srivastava, S. (1963). Ephedra-type pollen grains in the Dharamsala (Tertiary) formations, Punjab, India. Nature 198, 408–408. doi: 10.1038/198408a0
Gillespie, L. J., and Nowicke, J. W. (1994). Systematic implications of pollen morphology in Gnetum. Acta Bot. Gallica 141, 131–139. doi: 10.1080/12538078.1994.10515146
Gitzendanner, M. A., Soltis, P. S., Wong, G. K.-S., Ruhfel, B. R., and Soltis, D. E. (2018). Plastid phylogenomic analysis of green plants: A billion years of evolutionary history. Am. J. Bot. 105, 291–301. doi: 10.1002/ajb2.1048
Goeppert, H. R., and Berendt, G. C. (1845). Der bernstein und die in ihm befindlichen pflanzenreste der vorwelt. Berlin: Nicolaische Buchhandlung.
Gramzow, L., and Theissen, G. (2010). A hitchhiker’s guide to the MADS world of plants. Genome Biol. 11, 1–11. doi: 10.1186/gb-2010-11-6-214
Gramzow, L., Weilandt, L., and Theißen, G. (2014). MADS goes genomic in conifers: Towards determining the ancestral set of MADS-box genes in seed plants. Ann. Bot. 114, 1407–1429. doi: 10.1093/aob/mcu066
Gray, J. (1960). Temperate pollen genera in the Eocene (Claiborne) flora. Alabama. Science 132, 808–810. doi: 10.1126/science.132.3430.808
Grímsson, F., Zetter, R., and Baal, C. (2011). Combined LM and SEM study of the middle Miocene (Sarmatian) palynoflora from the Lavanttal Basin, Austria: Part I. Bryophyta, Lycopodiophyta, Pteridophyta, Ginkgophyta, and Gnetophyta. Grana 50, 102–128. doi: 10.1080/00173134.2011.585804
Han, F., Rydin, C., Bolinder, K., Dupont-Nivet, G., Abels, H. A., Koutsodendris, A., et al. (2016). Steppe development on the Northern Tibetan plateau inferred from Paleogene ephedroid pollen. Grana 55, 71–100. doi: 10.1080/00173134.2015.1120343
Harrison, C. J. (2017). Development and genetics in the evolution of land plant body plans. Philos. Trans. R. Soc. B Biol. Sci. 372:20150490. doi: 10.1098/rstb.2015.0490
Herrera, F., Shi, G., Mays, C., Ichinnorov, N., Takahashi, M., Bevitt, J. J., et al. (2020). Reconstructing Krassilovia mongolica supports recognition of a new and unusual group of Mesozoic conifers. PLoS One 15:e0226779. doi: 10.1371/journal.pone.0226779
Hofmann, C.-C., Roberts, E. A., and Seyfullah, L. J. (2022). Diversity of the dispersed Gnetalean pollen record from the Lower Cretaceous Crato Formation, Brazil: Entomophily, harmomegathy and habitat heterogeneity. Cretac. Res. 129:105020. doi: 10.1016/j.cretres.2021.105020
Hou, C., Humphreys, A. M., Thureborn, O., and Rydin, C. (2015). New insights into the evolutionary history of Gnetum (Gnetales). Taxon 64, 239–253. doi: 10.12705/642.12
Hou, C., Li, L., Liu, Z., Su, Y., and Wan, T. (2020). Diversity and expression patterns of MADS-box genes in Gnetum luofuense—implications for functional diversity and evolution. Trop. Plant Biol. 13, 36–49. doi: 10.1007/s12042-019-09247-x
Ickert-Bond, S. M., and Renner, S. S. (2016). The Gnetales: Recent insights on their morphology, reproductive biology, chromosome numbers, biogeography, and divergence times. J. Syst. Evolut. 54, 1–16. doi: 10.1111/jse.12190
Ickert-Bond, S. M., Rydin, C., and Renner, S. S. (2009). A fossil-calibrated relaxed clock for Ephedra indicates an Oligocene age for the divergence of asian and new world clades and miocene dispersal into South America. J. Syst. Evolut. 47, 444–456. doi: 10.1111/j.1759-6831.2009.00053.x
Klimov, P. B., OConnor, B. M., Chetverikov, P. E., Bolton, S. J., Pepato, A. R., Mortazavi, A. L., et al. (2018). Comprehensive phylogeny of acariform mites (Acariformes) provides insights on the origin of the four-legged mites (Eriophyoidea), a long branch. Mol. Phylogenet. Evolut. 119, 105–117. doi: 10.1016/j.ympev.2017.10.017
Klopfstein, S., and Spasojevic, T. (2019). Illustrating phylogenetic placement of fossils using rogueplots: An example from ichneumonid parasitoid wasps (Hymenoptera, Ichneumonidae) and an extensive morphological matrix. PLoS one 14:e0212942. doi: 10.1371/journal.pone.0212942
Kluge, A. G. (1983). “Cladistics and the classification of the great apes,” in New interpretations of ape and human ancestry, (New York, NY: Springer), 151–177. doi: 10.1007/978-1-4684-8854-8_6
Klymiuk, A. A., Rothwell, G. W., and Stockey, R. A. (2022). A novel cupulate seed plant, Xadzigacalix quatsinoensis gen. et sp. nov., provides new insight into the Mesozoic radiation of gymnosperms. Am. J. Bot. 109, 966–985. doi: 10.1002/ajb2.1853
Krassilov, V. A., and Bugdaeva, E. V. (2000). Gnetophyte assemblage from the Early Cretaceous of Transbaikalia. Palaeontograph. Abteil. B 253, 139–151. doi: 10.1127/palb/253/2000/139
Kunzmann, L., Mohr, B. A. R., and Bernardes-de-Oliveira, M. E. C. (2009). Cearania heterophylla gen. nov. et sp. nov., a fossil gymnosperm with affinities to the Gnetales from the Early Cretaceous of northern Gondwana. Rev. Palaeobot. Palynol. 158, 193–212. doi: 10.1016/j.revpalbo.2009.09.001
Kunzmann, L., Mohr, B. A. R., Wilde, V., and Bernardes-de-Oliveira, M. E. C. (2011). A putative gnetalean gymnosperm Cariria orbiculiconiformis gen. nov. et spec. nov. from the Early Cretaceous of northern Gondwana. Rev. Palaeobot. Palynol. 165, 75–95. doi: 10.1016/j.revpalbo.2011.02.005
Kustatscher, E., Van Konijnenburg-van Cittert, J. H., Bauer, K., and Krings, M. (2016). Strobilus organization in the enigmatic gymnosperm Bernettia inopinata from the Jurassic of Germany. Rev. Palaeobot Palynol. 232, 151–161. doi: 10.1016/j.revpalbo.2016.05.003
Lee, D., Conran, J., and Lindqvist, J. (2012). New Zealand Eocene, Oligocene and Miocene macrofossil and pollen records and modern plant distributions in the southern hemisphere. Bot. Rev. 78, 235–260. doi: 10.1007/s12229-012-9102-7
Li, H.-T., Yi, T.-S., Gao, L.-M., Ma, P.-F., Zhang, T., Yang, J.-B., et al. (2019). Origin of angiosperms and the puzzle of the Jurassic gap. Nat. Plants 5:461. doi: 10.1038/s41477-019-0421-0
Liu, Z.-J., and Wang, X. (2015). An enigmatic Ephedra-like fossil lacking micropylar tube from the Lower Cretaceous Yixian Formation of Liaoning, China. Palaeoworld 25, 67–75. doi: 10.1016/j.palwor.2015.07.005
Loera, I., Sosa, V., and Ickert-Bond, S. M. (2012). Diversification in North American arid lands: Niche conservatism, divergence and expansion of habitat explain speciation in the genus Ephedra. Mol. Phylogenet. Evolut. 65, 437–450. doi: 10.1016/j.ympev.2012.06.025
Magallón, S., and Sanderson, M. J. (2002). Relationships among seed plants inferred from highly conserved genes: Sorting conflicting phylogenetic signals among ancient lineages. Am. J. Bot. 89, 1991–2006. doi: 10.3732/ajb.89.12.1991
Majeed, A., Kaur, H., Kaur, A., Das, S., Joseph, J., and Bhardwaj, P. (2021). Codon usage pattern in Gnetales evolved in close accordance with the Gnetifer hypothesis. Bot. J. Linn. Soc. 196, 423–436. doi: 10.1093/botlinnean/boab006
Manchester, S. R., Zhang, X., Hotton, C. L., Wing, S. L., and Crane, P. R. (2021). Distinctive quadrangular seed-bearing structures of gnetalean affinity from the Late Jurassic Morrison Formation of Utah, USA. J. Syst. Palaeontol. 19, 743–760. doi: 10.1080/14772019.2021.1968522
Manchester, S. R., Zhang, X., Hotton, C. L., Wing, S., and Crane, P. R. (2022). Two-seeded cones of probable gnetalean affinity from the Morrison Formation (Late Jurassic) of Utah and Colorado. USA. Acta Palaeobot. 62, 77–92.
Marlétaz, F., Peijnenburg, K. T., Goto, T., Satoh, N., and Rokhsar, D. S. (2019). A new spiralian phylogeny places the enigmatic arrow worms among gnathiferans. Curr. Biol. 29, 312–318. doi: 10.1016/j.cub.2018.11.042
May, M. R., Contreras, D. L., Sundue, M. A., Nagalingum, N. S., Looy, C. V., and Rothfels, C. J. (2021). Inferring the total-evidence timescale of marattialean fern evolution in the face of model sensitivity. Syst. Biol. 70, 1232–1255. doi: 10.1093/sysbio/syab020
Mendes, M. M., Friis, E. M., and Pais, J. (2008). Erdtmanispermum juncalense sp. nov., a new species of the extinct order Erdtmanithecales from the Early Cretaceous (probably Berriasian) of Portugal. Review of Palaeobotany and Palynology 149, 50–56. doi: 10.1016/j.revpalbo.2007.10.005
Mongiardino Koch, N., Garwood, R. J., and Parry, L. A. (2021). Fossils improve phylogenetic analyses of morphological characters. Proc. R. Soc. B 288:20210044. doi: 10.1098/rspb.2021.0044
Moyroud, E., Monniaux, M., Thévenon, E., Dumas, R., Scutt, C. P., Frohlich, M. W., et al. (2017). A link between LEAFY and B-gene homologues in Welwitschia mirabilis sheds light on ancestral mechanisms prefiguring floral development. New Phytol. 216, 469–481. doi: 10.1111/nph.14483
Müller, G. B., and Newman, S. A. (2005). The innovation triad: An EvoDevo agenda. J. Exp. Zool. Part B Mol. Dev. Evolut. 304, 487–503. doi: 10.1002/jez.b.21081
One Thousand Plant Transcriptomes Initiative. (2019). One thousand plant transcriptomes and the phylogenomics of green plants. Nature 574, 679–685. doi: 10.1038/s41586-019-1693-2
Ontano, A. Z., Gainett, G., Aharon, S., Ballesteros, J. A., Benavides, L. R., Corbett, K. F., et al. (2021). Taxonomic sampling and rare genomic changes overcome long-branch attraction in the phylogenetic placement of pseudoscorpions. Mol. Biol. Evolut. 38, 2446–2467. doi: 10.1093/molbev/msab038
Palazzesi, L., and Barreda, V. (2012). Fossil pollen records reveal a late rise of open-habitat ecosystems in Patagonia. Nat. Commun. 3, 1–6. doi: 10.1038/ncomms2299
Pedersen, K., Crane, P. R., and Friis, E. (1989). Pollen organs and seeds with Eucommiidites pollen. Grana 28, 37–41. doi: 10.1080/00173138909427441
Plackett, A. R., Conway, S. J., Hazelton, K. D. H., Rabbinowitsch, E. H., Langdale, J. A., and Di Stilio, V. S. (2018). LEAFY maintains apical stem cell activity during shoot development in the fern Ceratopteris richardii. Elife 7:e39625. doi: 10.7554/eLife.39625
Pocock, S. A., and Vasanthy, G. (1988). Cornetipollis reticulata, a new pollen with angiospermid features from Upper Triassic (Carnian) sediments of Arizona (USA), with notes on Equisetosporites. Rev. Palaeobot. Palynol. 55, 337–356. doi: 10.1016/0034-6667(88)90092-9
Potonié, R. (1958). Synopsis der Gattungen der sporae dispersae. II. teil: Sporites (nachträge), saccites, Aletes, Praecolpates, Polyplicates, Monocolpates. Alexander Doweld. Moscow: Alexander Doweld.
Puebla, G. G., Iglesias, A., Gómez, M. A., and Prámparo, M. B. (2017). Fossil record of Ephedra in the Lower Cretaceous (Aptian), Argentina. J. Plant Res. 130, 975–988. doi: 10.1007/s10265-017-0953-1
Qiu, Y., Lee, J., Bernasconi-quadroni, F., Soltis, D. E., Soltis, P. S., Zanis, M., et al. (1999). The earliest angiosperms: Evidence from mitochondrial, plastid and nuclear genomes. Nature 402, 404–407. doi: 10.1038/46536
Ran, J.-H., Shen, T.-T., Wang, M.-M., and Wang, X.-Q. (2018). Phylogenomics resolves the deep phylogeny of seed plants and indicates partial convergent or homoplastic evolution between Gnetales and angiosperms. Proc. R. Soc. B Biol. Sci. 285:20181012. doi: 10.1098/rspb.2018.1012
Ribeiro, A. C., Ribeiro, G. C., Varejão, F. G., Battirola, L. D., Pessoa, E. M., Simões, M. G., et al. (2021). Towards an actualistic view of the Crato Konservat-lagerstätte paleoenvironment: A new hypothesis as an Early Cretaceous (Aptian) equatorial and semi-arid wetland. Earth Sci. Rev. 216:103573. doi: 10.1016/j.earscirev.2021.103573
Ricardi-Branco, F., Torres, M., Tavares, S. S., Carvalho, I. D. S., Tavares, P. G. E., and Campos, A. C. A. (2013). “Itajuba yansanae Gen. and Sp. nov. of Gnetales, Araripe Basin (Albian-Aptian) in Northeast Brazil,” in Climate change and regional/local responses, (Rijeka: IntechOpen), 187–205. doi: 10.5772/55704
Rodin, R., and Kapil, R. (1969). Comparative anatomy of the seed coats of Gnetum and their probable evolution. Ame. J. Bot. 56, 420–431. doi: 10.1002/j.1537-2197.1969.tb07553.x
Rong, T. J., and Yong, Y. (2003). Alloephedra xingxuei gen. et sp. nov., an Early Cretaceous member of Ephedraceae from Dalazi Formation in Yanji Basin, Jilin province of China. Gu Sheng wu xue bao= Acta Palaeontol. Sin. 42, 208–215.
Rota-Stabelli, O., Campbell, L., Brinkmann, H., Edgecombe, G. D., Longhorn, S. J., Peterson, K. J., et al. (2011). A congruent solution to arthropod phylogeny: Phylogenomics, microRNAs and morphology support monophyletic Mandibulata. Proc. R. Soc. B Biol. Sci. 278, 298–306. doi: 10.1098/rspb.2010.0590
Rothwell, G. W., and Serbet, R. (1994). Lignophyte phylogeny and the evolution of spermatophytes: A numerical cladistic analysis. Syst. Bot. 19, 443–482. doi: 10.2307/2419767
Rothwell, G. W., and Stockey, R. A. (2013). Evolution and phylogeny of gnetophytes: Evidence from the anatomically preserved seed cone Protoephedrites eamesii gen. et sp. nov. and the seeds of several bennettitalean species. Int. J. Plant Sci. 174, 511–529. doi: 10.1086/668688
Rothwell, G. W., and Stockey, R. A. (2016). Phylogenetic diversification of Early Cretaceous seed plants: The compound seed cone of Doylea tetrahedrasperma. Am. J. Bot. 103, 923–937. doi: 10.3732/ajb.1600030
Rothwell, G. W., Crepet, W. L., and Stockey, R. A. (2009). Is the anthophyte hypothesis alive and well? New evidence from the reproductive structures of Bennettitales. Am. J. Bot. 96, 296–322. doi: 10.3732/ajb.0800209
Rudall, P. J., and Bateman, R. M. (2019). Leaf surface development and the plant fossil record: Stomatal patterning in Bennettitales. Biol. Rev. 94, 1179–1194. doi: 10.1111/brv.12497
Rudall, P. J., and Rice, C. L. (2019). Epidermal patterning and stomatal development in Gnetales. Ann. Bot. 124, 149–164. doi: 10.1093/aob/mcz053
Rydin, C., and Friis, E. M. (2005). Pollen germination in Welwitschia mirabilis Hook. f.: Differences between the polyplicate pollen producing genera of the Gnetales. Grana 44, 137–141. doi: 10.1080/00173130500230459
Rydin, C., and Friis, E. M. (2010). A new Early Cretaceous relative of Gnetales: Siphonospermum simplex gen. et sp. nov. from the Yixian formation of northeast China. BMC Evolut. Biol. 10:183. doi: 10.1186/1471-2148-10-183
Rydin, C., and Korall, P. (2009). Evolutionary relationships in Ephedra (Gnetales), with implications for seed plant phylogeny. Int. J. Plant Sci. 170, 1031–1043. doi: 10.1086/605116
Rydin, C., Blokzijl, R., Thureborn, O., and Wikström, N. (2021). Node ages, relationships, and phylogenomic incongruence in an ancient gymnosperm lineage – Phylogeny of Ephedra revisited. TAXON 70, 701–719. doi: 10.1002/tax.12493
Rydin, C., Källersjö, M., and Friis, E. M. (2002). Seed plant relationships and the systematic position of Gnetales based on nuclear and chloroplast DNA: Conflicting data, rooting problems, and the monophyly of conifers. Int. J. Plant Sci. 163, 197–214. doi: 10.1086/338321
Rydin, C., Khodabandeh, A., and Endress, P. K. (2010). The female reproductive unit of Ephedra (Gnetales): Comparative morphology and evolutionary perspectives. Bot. J. Linn. Soc. 163, 387–430.
Rydin, C., Mohr, B., and Friis, E. M. (2003). Cratonia cotyledon gen. et sp. nov.: A unique Cretaceous seedling related to Welwitschia. Proceed. R. Soc. Lond. Ser. B: Biol. Sci. 270, S29–S32. doi: 10.1098/rsbl.2003.0044
Rydin, C., Wu, S., and Friis, E. (2006). Liaoxia Cao et SQ Wu (Gnetales): Ephedroids from the Early Cretaceous Yixian Formation in Liaoning, northeastern China. Plant Syst. Evol. 262, 239–265. doi: 10.1007/s00606-006-0481-2
Sadowski, E.-M., Seyfullah, L. J., Wilson, C. A., Calvin, C. L., and Schmidt, A. R. (2017). Diverse early dwarf mistletoes (Arceuthobium), ecological keystones of the Eocene Baltic amber biota. Am. J. Bot. 104, 694–718. doi: 10.3732/ajb.1600390
Sanderson, M. J., and Doyle, J. A. (2001). Sources of error and confidence intervals in estimating the age of angiosperms from rbcL and 18S rDNA data. Am. J. Bot. 88, 1499–1516. doi: 10.2307/3558458
Sanderson, M., Wojciechowski, M., Hu, J.-M., Khan, T. S., and Brady, S. (2000). Error, bias, and long-branch attraction in data for two chloroplast photosystem genes in seed plants. Mol. Biol. Evol. 17, 782–797. doi: 10.1093/oxfordjournals.molbev.a026357
Saporta de, G. (1889). Dernières adjonctions à la flore fossile d’Aix-en-Provence. Ann. Sci. nat. Bot. 10, 1–192.
Schrank, E. (2010). Pollen and spores from the Tendaguru beds, Upper Jurassic and Lower Cretaceous of southeast Tanzania: Palynostratigraphical and paleoecological implications. Palynology 34, 3–42.
Smith, S. A., Walker-Hale, N., Walker, J. F., and Brown, J. W. (2020). Phylogenetic conflicts, combinability, and deep phylogenomics in plants. Syst. Biol. 69, 579–592. doi: 10.1093/sysbio/syz078
Takaso, T., and Bouman, F. (1986). Ovule and seed ontogeny in Gnetum gnemon L. Botan. magazine= Shokubutsu-gaku-zasshi 99, 241–266. doi: 10.1007/BF02489542
Tang, Z., Ding, Z., White, P. D., Dong, X., Ji, J., Jiang, H., et al. (2011). Late Cenozoic central asian drying inferred from a palynological record from the northern Tian Shan. Earth Planet. Sci. Lett. 302, 439–447. doi: 10.1016/j.epsl.2010.12.042
Thoday, M. (1921). Anatomy of the ovule and seed in Gnetum gnemon, with notes on Gnetum funiculare. Ann. Bot. 35, 37–53. doi: 10.1093/oxfordjournals.aob.a089746
Thompson, W. (1918). Independent evolution of vessels in Gnetales and angiosperms. Bot. Gazette 65, 83–90. doi: 10.1086/332191
van Konijnenburg-van Cittert, J. H. (1992). An enigmatic Liassic microsporophyll, yielding Ephedripites pollen. Rev. Palaeobot. Palynol. 71, 239–254. doi: 10.1016/0034-6667(92)90165-D
Wan, T., Liu, Z., Leitch, I. J., Xin, H., Maggs-Kölling, G., Gong, Y., et al. (2021). The Welwitschia genome reveals a unique biology underpinning extreme longevity in deserts. Nat. Commun. 12, 1–15. doi: 10.1038/s41467-021-24528-4
Wan, T., Liu, Z.-M., Li, L.-F., Leitch, A. R., Leitch, I. J., Lohaus, R., et al. (2018). A genome for gnetophytes and early evolution of seed plants. Nat. Plants 4, 82–89. doi: 10.1038/s41477-017-0097-2
Wang, B., Jiang, B., Zhou, Y., Su, Y., and Wang, T. (2015). Higher substitution rates and lower dN/dS for the plastid genes in Gnetales than other gymnosperms. Biochem. Syst. Ecol. 59, 278–287. doi: 10.1016/j.bse.2015.02.009
Wang, X., and Zheng, S. (2010). Whole fossil plants of Ephedra and their implications on the morphology, ecology and evolution of Ephedraceae (Gnetales). Chin. Sci. Bull. 55, 1511–1519. doi: 10.1007/s11434-010-3069-8
Wang, Y.-Q., Melzer, R., and Theißen, G. (2010). Molecular interactions of orthologues of floral homeotic proteins from the gymnosperm Gnetum gnemon provide a clue to the evolutionary origin of ‘floral quartets.’. Plant J. 64, 177–190. doi: 10.1111/j.1365-313X.2010.04325.x
Wang, Z.-Q. (2004). A new Permian gnetalean cone as fossil evidence for supporting current molecular phylogeny. Ann. Bot. 94, 281–288. doi: 10.1093/aob/mch138
Watson, J., and Alvin, K. L. (1999). The cheirolepidiaceous conifers Frenelopsis occidentalis Heer and Watsoniocladus valdensis (Seward) in the Wealden of Germany. Cretac. Res. 20, 315–326. doi: 10.1006/cres.1999.0152
Whewell, W. (1840). The philosophy of the inductive sciences: Founded upon their history. Rocky Mount, NC: JW Parker.
Wilson, L. R. (1962). Permian plant microfossils from the Flowerpot formation, Greer Country, Oklahoma. Oklahoma Geol. Surv. Circ. 49, 1–50.
Wodehouse, R. P. (1933). Tertiary pollen-II The oil shales of the Eocene Green River formation. Bull. Torrey Bot. Club 60, 479–524. doi: 10.2307/2480586
Won, H., and Renner, S. S. (2006). Dating dispersal and radiation in the gymnosperm Gnetum (Gnetales)–clock calibration when outgroup relationships are uncertain. Syst. Biol. 55, 610–622. doi: 10.1080/10635150600812619
Woolley, C. H., Thompson, J. R., Wu, Y.-H., Bottjer, D. J., and Smith, N. D. (2022). A biased fossil record can preserve reliable phylogenetic signal. Paleobiology 48, 480–495. doi: 10.1017/pab.2021.45
Yang, Y. (2014). A systematic classification of Ephedraceae: Living and fossil. Phytotaxa 158, 283–290. doi: 10.11646/phytotaxa.158.3.8
Yang, Y., and Ferguson, D. K. (2015). Macrofossil evidence unveiling evolution and ecology of early Ephedraceae. Perspect. Plant Ecol. Evol. Syst. 17, 331–346. doi: 10.1016/j.ppees.2015.06.006
Yang, Y., Ferguson, D. K., Liu, B., Mao, K.-S., Gao, L.-M., Zhang, S.-Z., et al. (2022). Recent advances on phylogenomics of gymnosperms and an updated classification. Plant Diver. 44, 340–350. doi: 10.1016/j.pld.2022.05.003
Yang, Y., Geng, B.-Y., Dilcher, D. L., Chen, Z.-D., and Lott, T. A. (2005). Morphology and affinities of an Early Cretaceous Ephedra (Ephedraceae) from China. Am. J. Bot. 92, 231–241. doi: 10.3732/ajb.92.2.231
Yang, Y., Lin, L., and Ferguson, D. K. (2015). Parallel evolution of leaf morphology in gnetophytes. Organ. Diversit Evol. 15, 651–662. doi: 10.1007/s13127-015-0226-6
Yang, Y., Lin, L., and Wang, Q. (2013). Chengia laxispicata gen. et sp. nov., a new ephedroid plant from the Early Cretaceous Yixian Formation of western Liaoning, Northeast China: Evolutionary, taxonomic, and biogeographic implications. BMC Evol. Biol. 13:72. doi: 10.1186/1471-2148-13-72
Yang, Y., Lin, L.-B., Ferguson, D. K., Zhang, S.-Z., and Wan, T. (2017). A new gnetalean macrofossil from the Early Cretaceous and its evolutionary significance. Cretac. Res. 74, 56–64. doi: 10.1016/j.cretres.2017.02.007
Yuan, Q., Barbolini, N., Rydin, C., Gao, D.-L., Wei, H.-C., Fan, Q.-S., et al. (2020). Aridification signatures from fossil pollen indicate a drying climate in east-central Tibet during the late Eocene. Clim. Past 16, 2255–2273. doi: 10.5194/cp-16-2255-2020
Zheng, B., Xing, K., Zhang, J., Liu, H., Ali, K., Li, W., et al. (2022). Evolutionary analysis and functional identification of ancient brassinosteroid receptors in Ceratopteris richardii. Int. J. Mol. Sci. 23:6795. doi: 10.3390/ijms23126795
Zhong, B., Yonezawa, T., Zhong, Y., and Hasegawa, M. (2010). The position of gnetales among seed plants: Overcoming pitfalls of chloroplast phylogenomics. Mol. Biol. Evol. 27, 2855–2863. doi: 10.1093/molbev/msq170
Zumajo-Cardona, C., and Ambrose, B. A. (2021). Deciphering the evolution of the ovule genetic network through expression analyses in Gnetum gnemon. Ann. Bot. 128, 217–230. doi: 10.1093/aob/mcab059
Keywords: phylogeny, development, Ephedra, Welwitschia, Gnetum, seed plants
Citation: Coiro M, Roberts EA, Hofmann C-C and Seyfullah LJ (2022) Cutting the long branches: Consilience as a path to unearth the evolutionary history of Gnetales. Front. Ecol. Evol. 10:1082639. doi: 10.3389/fevo.2022.1082639
Received: 28 October 2022; Accepted: 25 November 2022;
Published: 14 December 2022.
Edited by:
Ricard Albalat, University of Barcelona, SpainReviewed by:
Chen Hou, Guangdong Academy of Forestry, ChinaCopyright © 2022 Coiro, Roberts, Hofmann and Seyfullah. This is an open-access article distributed under the terms of the Creative Commons Attribution License (CC BY). The use, distribution or reproduction in other forums is permitted, provided the original author(s) and the copyright owner(s) are credited and that the original publication in this journal is cited, in accordance with accepted academic practice. No use, distribution or reproduction is permitted which does not comply with these terms.
*Correspondence: Mario Coiro, bWFyLmNvaXJvQGdtYWlsLmNvbQ==
Disclaimer: All claims expressed in this article are solely those of the authors and do not necessarily represent those of their affiliated organizations, or those of the publisher, the editors and the reviewers. Any product that may be evaluated in this article or claim that may be made by its manufacturer is not guaranteed or endorsed by the publisher.
Research integrity at Frontiers
Learn more about the work of our research integrity team to safeguard the quality of each article we publish.