- 1Department of Environmental Science and Conservation Biology, United Graduate School of Agricultural Sciences, Kagoshima University, Kagoshima, Japan
- 2Organization for Regional Collaboration, University of the Ryukyus, Okinawa, Japan
- 3Graduate School of Education, University of the Ryukyus, Okinawa, Japan
- 4Faculty of Agriculture, University of the Ryukyus, Okinawa, Japan
- 5Hokkaido Forest Research Station, Field Science Education and Research Center, Kyoto University, Hokkaido, Japan
Selfish genetic elements (SGEs) increase their transmission efficiency relative to the rest of the individual genome, which is often deleterious to individual fitness. Theoretical studies have suggested that intragenomic conflict over the sex ratio distortion between SGEs and the rest of the genome should lead to the evolution of sex-determining systems. However, in insects, there are relatively few studies other than those on Dipterans, which makes it difficult to understand the role of SGEs in the evolution of insect sex determination. This is partially due to the difficulties in observing SGEs under field conditions. The effect of SGEs is often masked by the counter-evolution of the resistance genes. Interpopulation cross-breeding experiments are effective to detect the SGEs and their resistance genes. If these populations have different SGEs and resistance genes, cross-breeding experiments reveal their existence by collapsing the evolutionary antagonistic state. The Ryukyu drywood termites Neotermes sugioi, distributed in the Ryukyu Islands, show male-biased sex ratios in pseudergates, nymphs, alates and soldiers both in Okinawa and Ishigaki Islands, but different degrees of bias have been reported between the islands. Male-specific microsatellite alleles have been reported in this species, which allowed us to identify the sex of the eggs and young larvae. In this study, we used the microsatellite locus with male-specific alleles to investigate the primary sex ratio of field colonies on Okinawa and Ishigaki islands and the sex ratio of offspring obtained through cross-breeding experiments between the islands. The primary sex ratios of field colonies were male-biased in Okinawa but not in Ishigaki. Cross-breeding experiments showed that Okinawa males tend to have a male-biased sex ratio in their offspring, but Ishigaki males do not. This result is consistent with the hypothesis that the male bias in this species is caused by SGEs, even though termites are phylogenetically distant from Diptera. Accumulation of knowledge on genetic conflicts in a wide range of taxa might be an important step toward elucidating the mechanisms of diversification of sex determination systems in insects.
1. Introduction
Selfish genetic elements (SGEs) escape from Mendelian laws of inheritance by enhancing their own transmission to the next generation relative to the rest of the individual genome, which is often deleterious to individual fitness (Werren et al., 1988). An extraordinarily biased sex ratio can occur when SGEs exist near the sex determination locus. Theoretical studies have shown that intragenomic conflict over sex ratio distortion between SGEs and the rest of the genome leads to the origin of sex chromosomes and evolution of sex-determining systems (Werren and Beukeboom, 1998; Kozielska et al., 2009; Úbeda et al., 2015). Examples of sex ratio bias due to SGEs have been found in a wide range of taxa in eukaryotes (Jaenike, 2001; Burt and Trivers, 2006), but there are relatively few studies on the bias due to SGEs in insects other than Dipterans. This makes it difficult to understand the role of SGEs in the evolution of insect sex determination. Detection of SGEs in field conditions is often difficult because the counter-evolution of resistance genes masks the effect of SGEs (extraordinarily sex ratio biases) and leads to stabilization in an evolutionary antagonistic state (Jaenike, 2001; Burt and Trivers, 2006; Werren, 2011). Thus, SGEs causing sex ratio biases may be widespread in insects other than Dipterans but have not been found due to the counter-evolution of resistance genes. Interpopulation cross-breeding experiments are effective to detect SGEs and their resistance genes. Cross-breeding experiments reveal their existence by collapsing the evolutionary antagonistic state if these populations have different SGEs and their resistance genes (Jaenike, 2001; Burt and Trivers, 2006; Lindholm et al., 2016).
In termites, the XY sex determination system is thought to be widely conserved (Jankásek et al., 2021), where the sex ratio of eggs is expected to be 1:1 according to Mendel’s law. However, some species show sex ratio bias, the cause of which remains unknown in many cases (Neoh and Lee, 2011). Miyaguni et al. (2021) showed that in the Ryukyu dry-wood termite N. sugioi, recently separated from N. koshunensis (Yashiro et al., 2019), numerical sex ratios were biased toward males in pseudergates, nymphs, alates and soldiers (including pre-soldiers) on all islands examined (Figure 1A). In Okinawa population, the sex ratio of alate biomass, as an index of the sex allocation ratio, was biased also toward males. Such a male-biased sex ratio is inconsistent with the prediction of Fisher’s sex allocation theory, and therefore the possibility of SGEs has not been ruled out. In some mosquitoes and stalk-eyed flies, SGEs are present in the Y-chromosome and biases the sex ratio during spermatogenesis (Wood and Newton, 1991; Presgraves et al., 1997). In these species, resistance genes are developed on the X-chromosome in populations where SGEs are present, whereas there are populations where neither selfish nor resistance genes are present. If the biased sex ratio is caused by SGEs, a stronger deviation from an equal ratio would be detected in the sex ratio of eggs obtained via interpopulation cross-breeding compared to the sex ratio of eggs from intrapopulation cross-breeding. The males of F1 individuals born from these interpopulation crosses have a Y-chromosome with a SGEs and an X-chromosome without a resistance gene; therefore, the sex ratio of sperm formed by F1 males is extremely biased (Wood and Newton, 1991; Presgraves et al., 1997). In summary, the presence of SGEs is verified by examining the sex ratio of the F2 generation. It is difficult to complete the experiment to determine whether the sex ratio is biased at this point of spermatogenesis as termites, which are social insects, take several years to obtain their second generation of alates. In this study, we focused on the differences in investment sex ratios among islands and examined whether their primary sex ratios were biased, at least at the time of oviposition. It is often difficult to determine the sex of eggs. However, male-specific microsatellite alleles have been reported in this species, which allow us to identify the sex of eggs (Agarie et al., 2020).
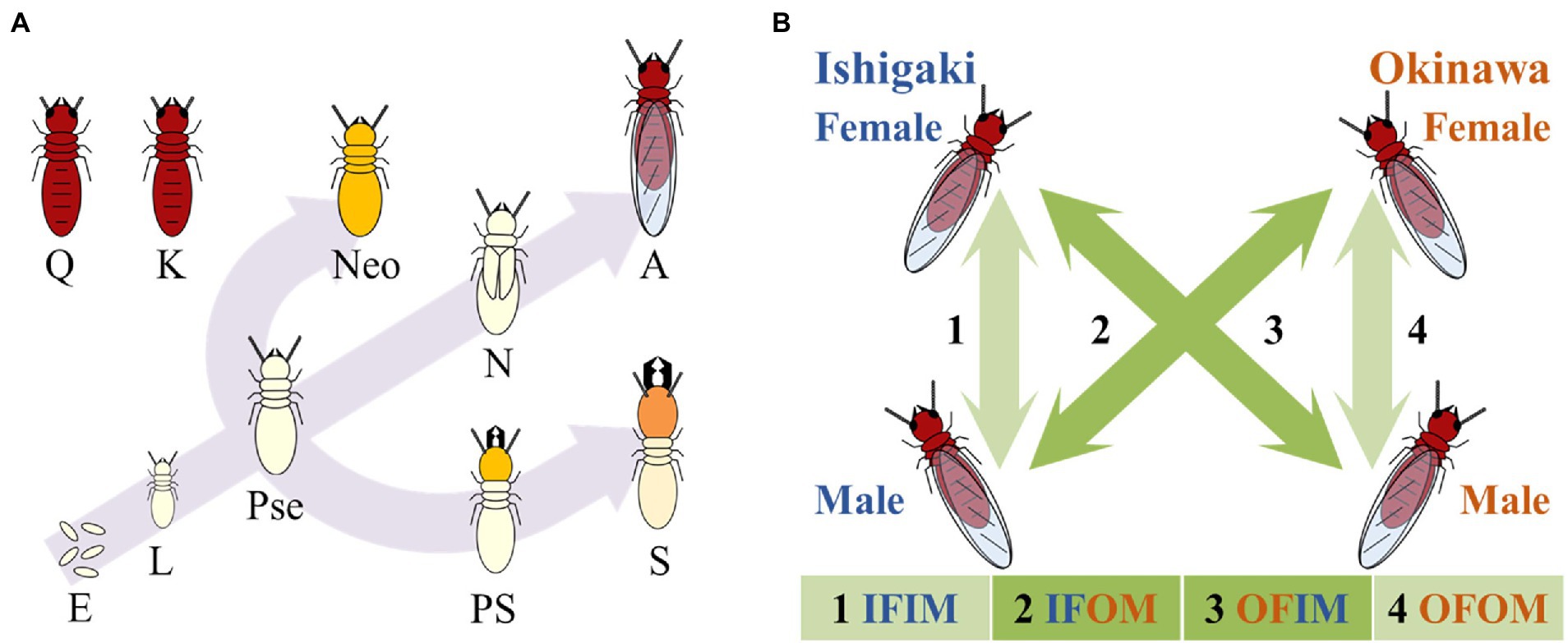
Figure 1. Caste developmental pathway in Neotermes sugioi and the scheme of cross-breeding experiment in Okinawa and Ishigaki population performed in this study. (A) Caste-developmental pathway in N. sugioi. Q: primary queen, K: primary king, Neo: neotenic (producing males only), E: egg, L: larva, Pse: pseudergates, N: nymph, A: alate, PS: pre-soldier, S: soldier. (B) Cross-breeding experiment in Okinawa and Ishigaki populations performed in this study. Mating pattern 1 and 4 are intra-population, 2 and 3 are inter-population. The cross-breeding experiments performed four mating patterns: Ishigaki male and female (IFIM), Okinawa male and Ishigaki female (IFOM), Ishigaki male and Okinawa female (OFIM), and Okinawa male and female (OFOM).
To clarify the primary sex ratio in field populations, we analyzed microsatellites with male-specific alleles for eggs from the Okinawa and Ishigaki populations. To explore the existence of SGEs causing the male-biased sex ratio of eggs, we performed microsatellite analysis of the eggs produced via cross-breeding between Okinawa and Ishigaki populations, where sex ratios of alates were different in a previous study (Miyaguni et al., 2021). Based on these results, we discussed the relationship between SGEs and biased sex ratios in termites, and suggested that this species can be an important material for studying the relationship between sex ratios and SGEs in termites, and may provide a new steppingstone to elucidate the evolutionary process of the sex determination system in insects.
2. Materials and methods
The Ryukyu drywood termite N. sugioi (new Neotermes species recently separated from N. koshunensis; Yashiro et al., 2019), belongs to the Kalotermitidae family, and is distributed in the Ryukyu Islands of Japan. In N. sugioi, we can discriminate the sex even at the egg and 1st instar larval stages using previously reported male-specific alleles at the microsatellite locus (NK12-1; Agarie et al., 2020). Neotermes sugioi has a unique reproductive system. Generally, in termites including N. sugioi, a colony is founded by a monogamous pair of alates which become the primary king and the primary queen (Roisin, 2000; Sugio et al., 2020; Figure 1A). After the death of one of the founders, one of their offspring develops into a neotenic reproductive (secondary king or queen; Roisin, 2000). However, all neotenics produced in N. sugioi colonies are male (Miyaguni et al., 2013; Sugio et al., 2020).
2.1. Sampling of eggs in field colonies
From 2018 to 2020, we collected colonies of N. sugioi from the Okinawa Islands (n = 9) and Ishigaki Islands (n = 9) in the Ryukyu Archipelago, Japan (Supplementary Table 1). These islands are separated by the sea and more than 400 km apart. All colonies were brought to our laboratory at University of the Ryukyus and their eggs were preserved in 99.5% ethanol for subsequent genetic analyses.
2.2. Sampling and cross-breeding experiments among Okinawa and Ishigaki populations
We sampled eight mature colonies of N. sugioi each from the two populations (Okinawa and Ishigaki islands) from late April to early June from 2017 to 20 (Supplementary Table 2). They were maintained in a plastic box (221 × 141 × 37 mm or 270 × 190 × 51 mm) with damp chips of sliced pine wood at 27°C in our laboratory until the nymphs molted and the alates matured. In July, female and male alates that molted in a plastic box were used for cross-breeding experiments according to the following four mating patterns (Figure 1B; Supplementary Table 2): Okinawa males and females (OFOM, the number of established colonies, n, was 213), Ishigaki males and females (IFIM, n = 340), Okinawa males and Ishigaki females (IFOM, n = 316), and Ishigaki males and Okinawa females (OFIM, n = 222). Each pair of alates was kept in 35-mm Petri dishes containing layers of a filter paper and two damp chips of pine wood (Kobayashi and Miyaguni, 2016) at 27°C for 90–120 days and checked every 30 days. When larvae were found, they were sampled from the dish and preserved in 99.5% ethanol for subsequent genetic analysis. All individuals and eggs that survived at the end of the experiment were preserved in 99.5% ethanol for the subsequent genetic analysis, except for the colonies in which one founder died before the end of the experiment. Finally, the numbers of colonies we genotyped were 43 for OFOM, 43 for IFIM, 56 for IFOM, and 49 for OFIM (Supplementary Table 2).
2.3. Sex discrimination using male-specific alleles
Eggs in field colonies (76–99 eggs per colony) and offspring (eggs or 1st instar larvae; 4–6 individuals per mating pair) produced in each cross-breeding pair were genotyped to discriminate their sex by the male-specific microsatellite allele. Since there are multiple male-specific alleles in this species (Agarie et al., 2020), it is possible that previously undiscovered male-specific alleles may be found in this experiment. As a precaution, the parents (alates) were also genotyped (Supplementary Table 3). A leg of each alate or the full body of an egg or a larva was crushed in 200 μl tubes, to which 0.5 μl of proteinase K (20 mg/ml) and 100 μl of 10% Chelex solution (10 mM Tris–HCl, 1 mM EDTA; pH 8.0) were added; the mixture was incubated at 35°C overnight. Subsequently, the mixture was boiled at 95°C for 15 min to inactivate the proteinase K. After brief centrifugation, a supernatant was used as the template DNA. Each of the 15.2 μl reaction mixtures contained 5 pmol of each primer and 1 pmol of dyed reverse primer (Supplementary Table 4), 1 μl of dNTP mix (10 mM each), 3 μl of 25 mM MgCl2, 3 μl of 5 × buffer, 0.1 μl of KAPATaq EXtra DNA Polymerase (NIPPON Genetics; 5 U/μl), and 1 μl template DNA. The reactions were run according to the PCR cycle, which consisted of an initial denaturation step at 94°C for 2 min, followed by 35 cycles of 30 s at 94°C, 30 s at 60°C, 30 s at 72°C, and one step at 72°C for 1 min to complete the extension at the end. The length of PCR products was checked using the SeqStudio Genetic Analyzer (Applied Biosystems) with 0.5 μl of GeneScan 600 LIZ dye Size Standard v2.0.
2.4. Statistical analysis
We performed a generalized linear model (GLM) analysis with a binomial error term and a logit link function to compare the observed primary sex ratios of the Okinawa and Ishigaki populations with the equal ratio. We also analyzed whether the population of origin of alate used in the cross-breeding experiments affected the sex ratio of the offspring using GLM with a binomial error term and a logit link function. Free software R ver. 4.0.2 (R Development Core Team 2020) was used for analyses. We also analyzed the correlation between the presence of a neotenic king and the colony-level sex ratio in N. sugioi, using the previously published field data on the reproductive structure and sex ratios of pseudergates in Okinawa population from May to August (Miyaguni et al., 2021). The data included colonies with a neotenic king and a primary queen (7 colonies). Then, we compared their sex ratios with those of the colonies headed by a primary king and a primary queen (36 colonies) by Welch’s t-test (Supplementary Table 5). Note that the previous study (Sugio et al., 2020) showed the sex-ratio of alate stage did not seasonally fluctuate.
3. Results
3.1. Primary sex ratio in field populations
The mean primary sex ratios, that is, the proportions of individuals bearing one of the male-specific alleles at the microsatellite locus NK12-1, were 0.6213 and 0.4838 in the Okinawa and Ishigaki populations, respectively. The 95% confidence interval (CI) did not include 0.5 in seven out of nine colonies in the Okinawa population, whereas the CIs of all colonies in the Ishigaki population covered 0.5 (Table 1). The GLM analysis showed that the primary sex ratio of the Okinawa population was biased toward males (z = 6.825, p < 0.001), but that of the Ishigaki population was not biased (z = −0.918, p = 0.359).
3.2. Cross-breeding experiments among Okinawa and Ishigaki populations
The mean sex ratio of offspring (i.e., the proportion of individuals bearing one of the male-specific alleles at NK12-1) obtained via cross-breeding was 0.451 (SD = 0.232, n = 43; GLM analysis with Wald test, coefficient = −0.2195, SE = 0.1280, z = −1.715, p = 0.086) in IFIM, 0.579 (SD = 0.223, n = 56; GLM analysis with Wald test, coefficient = 0.3042, SE = 0.1135, z = 2.681, p = 0.007) in IFOM, 0.395 (SD = 0.268, n = 49; GLM analysis with Wald test, coefficient = −0.4084, SE = 0.1214, z = −3.365, p < 0.001) in OFIM, and 0.577 (SD = 0.229, n = 43; GLM analysis with Wald test, coefficient = 0.3133, SE = 0.1278, z = 2.452, p = 0.014) in OFOM. The sex ratio tended to be different among the patterns of cross-breeding (Figure 2; GLM analysis with likelihood ratio test, df = 3, deviance = 27.866, residual df = 187, residual deviance = 296.78, p < 0.001). In particular, Okinawa males tended to have male-biased sex ratios in their offspring compared with that of Ishigaki males (Figure 2; Table 2). Based on the genotypes (Supplementary Table 4), some offspring (six offspring in four founding colonies) were thought to be produced via parthenogenesis, which suggests that the proportion of males in the sexually produced offspring is more biased toward males than the analyzed sex ratio, including both the parthenogenetically and sexually produced offspring.
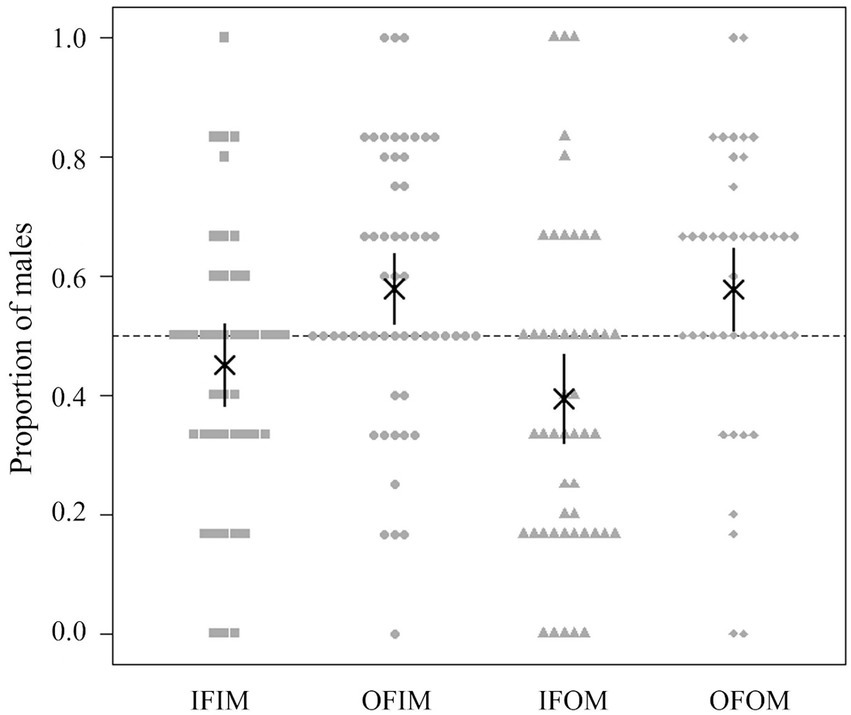
Figure 2. Proportion of male offspring obtained from the cross-breeding experiments. The asterisk indicates the mean for each mating pattern, the vertical line shows the 95% confidence interval, and each gray circle corresponds to the proportion of male offspring obtained from each mating pattern: IFIM, IFOM, OFIM, and OFOM.
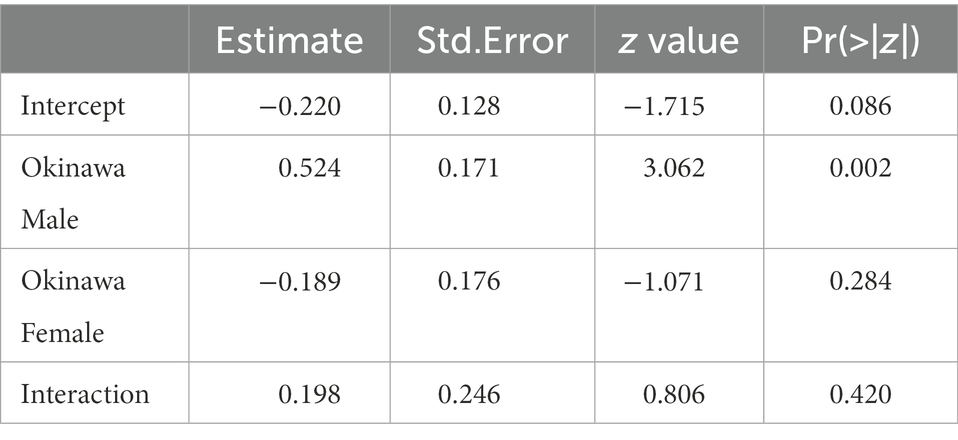
Table 2. Summary of GLM analysis for the effects of male and female natal populations and their interaction term on proportion of males in their offspring obtained through cross-breeding experiments.
4. Discussion
The cross-breeding experiments showed different patterns of sex ratio depending on the mating pattern: Okinawa males tended to have a male-biased sex ratio in their offspring, but Ishigaki males did not (Figure 2; Table 2). In fact, the primary sex ratio was biased toward males in Okinawa but not in Ishigaki (Table 1). These results suggest that Okinawa males tend to produce more sperm with Y-chromosomes than those with X-chromosomes. The Ryukyu drywood termite N. sugioi is thought to have an XY sex determination system (Agarie et al., 2020). In this case, spermatogenesis is important when considering the mechanism that biases the primary sex ratio. In some Dipterans, selfish genetic elements are known to result in overproduction of sperm with a Y-chromosome during spermatogenesis (due to the meiotic drive). We can consistently explain our results by assuming the existence of a similar selfish element in N. sugioi. Future detailed studies on functional genes near male-specific alleles will contribute to revealing the mechanisms that produce sex ratio bias and its evolutionary processes.
The hypothesis that SGEs cause a biased sex ratio in N. sugioi can be tested by examining either the spermatogenesis process or the sex ratio of the F2 generation. The former provides direct evidence by detecting functional genes in the process of spermatogenesis, thereby increasing the number of sperm with the Y-chromosome. The latter approach assumes that sex-ratio distorter and resistance genes are developed in the Okinawan population but not in the Ishigaki population. In this case, there would be a stronger male bias in the F2 generation produced by male individuals obtained from crosses between Okinawan males and Ishigaki females. As it takes several years to obtain alates from artificially founded colonies, no sex ratio data for the F2 generation were available in this study.
In this study, the Ishigaki population showed unbiased primary numerical sex ratios throughout 2018 and 2020 (Table 1). Furthermore, in the cross-breeding experiment, the males did not tend to bias the numerical sex ratio of offspring toward males (Figure 2). Based on these results, one can predict an equal numerical sex ratio in the Ishigaki population. However, Miyaguni et al. (2021) showed male-biased numerical sex ratios in older developmental stages (pseudergates, nymphs, alates) both in Okinawa and Ishigaki populations. One hypothesis to solve this discrepancy is the annual and seasonal variation in the primary sex ratio of the Ishigaki population. Such seasonal variation in the primary sex ratio was suggested to occur in some termites, in which all workers and soldiers are of a particular sex and the opposed sex seems to be produced only during certain seasons (Roisin, 1992; Roisin, 2001; Lee et al., 2017). Furthermore, in Cavitermes tuberosus, the sex ratio of eggs varies widely from colony to colony presumably related to the seasonal sex ratio variation (min-max: 0.431–0.700; Hellemans et al., 2019). Also, in N. sugioi the primary sex ratio might change seasonally (in this study, we sampled colonies from May to June), and data on the primary sex ratio in other seasons for Ishigaki population is required to test this hypothesis. Another hypothesis for this discrepancy is the sexual difference in the developmental speed. Larvae hatch from eggs become alates through pseudergates and nymphs (Figure 1A). Colonies release mature alates out of nests during the swarming season to establish new colony (Roisin, 2000; Sugio et al., 2020; Figure 1A). If males take longer to develop to alates than females during this developmental process, the sex ratio of colony can be biased toward males, even if they were born at an equal primary sex ratio. This hypothesis is also to be investigated.
Neotermes species, such as N. connexus, N. papua and N. sugioi, have the male-specific neotenic system (i.e., female neotenics are absent; Myles and Chang, 1984; Roisin and Pasteels, 1991; Miyaguni et al., 2013). Moreover, a male-biased sex ratio in some colony members was found in N. connexus, N. papua and N. sugioi (Myles and Chang, 1984; Roisin and Pasteels, 1991; Miyaguni et al., 2021). Other species, such as N. jouteli (Nagin, 1972; Jones et al., 1988), which are termites with the bisexual neotenic system (producing neotenics both in males and females), show an equal sex ratio. Understanding the relationship between this unique neotenic system and the male-biased sex ratio in Neotermes termites is an intriguing research topic. In N. sugioi, no difference in the sex ratio was found between field colonies headed by a primary-queen-and-primary-king pair and those headed by a primary-queen-and-neotenic-king pair (Supplementary Table 5). The causal relationship between the male-specific neotenic system and the male-biased sex ratio is puzzling and requires more information of Neotermes species for deeper understandings.
In Kalotermitidae, some species show male-biased sex ratios that are not well explained by the sex allocation theory (Nagin, 1972; Myles and Chang, 1984; Roisin, 2000; Neoh and Lee, 2011; Huang et al., 2012; Miyaguni et al., 2021). This study showed a male-biased sex ratio at the egg stage in N. sugioi, thereby suggesting the presence of SGEs on the Y-chromosome. A focus on the genetic conflict caused by SGEs may explain the unresolved sex ratio biases in termites. A better understanding of the molecular basis of termite sex determination is required to elucidate the relationship between termite sex ratio bias and SGE. In termites, sex-linked genetic markers have been reported in three species, including N. sugioi (Fournier et al., 2016; Hayashi et al., 2017; Agarie et al., 2020). Moreover, dsx, which is a conserved gene for sex determination in insects, has also been identified in several species (Miyazaki et al., 2021). However, the genetic cascade underlying termite sex determination remains unclear. More molecular biological studies will be required in the Ryukyu drywood termite N. sugioi, because this termite is a useful model for exploring the relationship between sex determination, SGEs, and sex ratio bias. In addition, in males of some termites including the genus Neotermes multiple autosomes are translocated with sex chromosomes to form a complex “chain” or “ring” that is inherited as a single linkage group, while in females, chromosomes are translocated independently (Syren and Luykx, 1977; Luykx, 1990; Martins and Mesa, 1995; Bergamaschi et al., 2007). The formation of such a chromosome complex can change the effective sex-linked fraction of the genome and have a strong impact on the pattern of gene transmission (Roisin, 2001; Yashiro et al., 2021), which may also be involved in the proximate mechanism of the biased primary sex ratio. The presence of this phenomenon is not yet surveyed in N. sugioi. Future chromosomic and cytological approaches will also be required.
Insects show a tremendous diversity of sex-determining mechanisms both within and among taxonomic orders (Bachtrog et al., 2014; Blackmon et al., 2017), and intragenomic conflicts caused by SGEs are likely to explain the diversification of sex-determination systems (Werren and Beukeboom, 1998; Kozielska et al., 2009; Úbeda et al., 2015; Palmer et al., 2019). However, there are few examples of intragenomic conflicts that cause evolutionary transitions in sex determination systems (Palmer et al., 2019), and such studies have focused on a few taxa in Diptera. Our results suggest that SGEs distort sex ratios, even in termites that are phylogenetically distant from well-studied taxa. Identifying the genes responsible for the biased sex ratio in this species and comparing them across populations may shed light on not only the evolutionary process of biased sex ratio at the gene level but also on the diversification process of sex-determination mechanisms. Accumulation of knowledge on intragenomic conflicts in a wide range of species might be an important step toward elucidating the mechanisms of diversification of sex determination systems in insects.
Data availability statement
The original contributions presented in the study are included in the article/Supplementary material, further inquiries can be directed to the corresponding author.
Author contributions
AA, YM, and KK: study conception and design. AA and YM: sampling termites. AA and KK: genotyping and writing original draft. YM, KK and KT: funding acquisition. KS, KT, and KK: project administration and supervision. All authors contributed to the article and approved the submitted version.
Funding
This work was supported by JSPS KAKENHI no. 22H02702 to KT, no. 21H02205 to YM and KK, and by no. 17H05048 and ISHIZUE 2020 of Kyoto University Research Development Program to KK. Also, United Graduate School of Agricultural Sciences Research Grant and The University of Ryukyus Foundation Research Grant to AA.
Acknowledgments
We would like to thank H. Tatsuta for insightful discussions and H. Nakahara for technical assistance with genotyping. We would also like to thank Editage (www.editage.com) for English language editing.
Conflict of interest
The authors declare that the research was conducted in the absence of any commercial or financial relationships that could be construed as a potential conflict of interest.
Publisher’s note
All claims expressed in this article are solely those of the authors and do not necessarily represent those of their affiliated organizations, or those of the publisher, the editors and the reviewers. Any product that may be evaluated in this article, or claim that may be made by its manufacturer, is not guaranteed or endorsed by the publisher.
Supplementary material
The Supplementary material for this article can be found online at: https://www.frontiersin.org/articles/10.3389/fevo.2022.1081043/full#supplementary-material
References
Agarie, A., Miyaguni, Y., Sugio, K., and Kobayashi, K. (2020). Male-specific alleles in the Ryukyu drywood termite Neotermes sugioi. Ecol. Evol. 10, 9584–9587. doi: 10.1002/ece3.6671
Bachtrog, D., Judith, E. M., Catherine, L. P., Mark, K., Sarah, P. O., Tia, L. A., et al. (2014). Sex determination: why so many ways of doing it? PLoS Biol. 12, e1001899–e1001813. doi: 10.1371/journal.pbio.1001899
Bergamaschi, S., Dawes-Gromadzki, T. Z., Scali, V., Marini, M., and Mantovani, B. (2007). Karyology, mitochondrial DNA and the phylogeny of Australian termites. Chromosom. Res. 15, 735–753. doi: 10.1007/s10577-007-1158-6
Blackmon, H., Ross, L., and Bachtrog, D. (2017). Sex determination, sex chromosomes, and karyotype evolution in insects. J. Hered. 108, 78–93. doi: 10.1093/jhered/esw047
Burt, A., and Trivers, R. (2006). Genes in Conflict: The Biology of Selfish Genetic Elements. Cambridge, MA: Harvard University Press.
Fournier, D., Hellemans, S., Hanus, R., and Roisin, Y. (2016). Facultative asexual reproduction and genetic diversity of populations in the Humivorous termite Cavitermes Tuberosus. Proc. Royal Soc. B. 283, 1–8. doi: 10.1098/rspb.2016.0196
Hayashi, Y., Oguchi, K., Yamaguchi, K., Kitade, O., Maekawa, K., Miura, T., et al. (2017). Male-specific molecular genetic markers in the Japanese subterranean termite Reticulitermes Speratus. Insectes Soci. 64, 357–364. doi: 10.1007/s00040-017-0553-z
Hellemans, S., Kaczmarek, N., Marynowska, M., Calusinska, M., Roisin, Y., and Fournier, D. (2019). Bacteriome-associated Wolbachia of the parthenogenetic termite Cavitermes tuberosus. FEMS Microbiol. Ecol. 95, 1–13. doi: 10.1093/femsec/fiy235
Huang, Z.-Y., Pan, J.-C., Zhong, J.-H., Qian, X., Tan, W-Y., Li, Z.-Q., et al. (2012). Sex ratios of the dryword termite Cryptotermes domesticus. Chin. J. Appl. Entomol. 49, 969–975.
Jaenike, J. (2001). Sex chromosome meiotic drive. Annu. Rev. Ecol. Syst. 32, 25–49. doi: 10.1146/annurev.ecolsys.32.081501.113958
Jankásek, M., Varadínová, Z. K., and Stáhlavský, F. (2021). Blattodea karyotype database. Eur. J. Entomol. 118, 192–199. doi: 10.14411/eje.2021.020
Jones, S. C., La Fage, J. P., and Howard, R. W. (1988). Isopteran sex ratios: phylogenetic trends. Sociobiology 14, 89–156.
Kobayashi, K., and Miyaguni, Y. (2016). Facultative parthenogenesis in the Ryukyu drywood termite Neotermes koshunensis. Sci. Rep. 6:30712. doi: 10.1038/srep30712
Kozielska, M., Weissing, F. J., Beukeboom, L., and Pen, I. (2009). Segregation distortion and the evolution of sex-determining mechanisms. Heredity 104, 100–112. doi: 10.1038/hdy.2009.104
Lee, T. R. C., Bourguignon, T., and Lo, N. (2017). Female-only workers and soldiers in Schedorhinotermes intermedius are not produced by parthenogenesis. Insect. Soc. 64, 133–139. doi: 10.1007/s00040-016-0523-x
Lindholm, A. K., Dyer, K. A., Firman, R. C., Fishman, L., Forstmeier, W., Holman, L., et al. (2016). The ecology and evolutionary dynamics of meiotic drive. Trends Ecol. Evol. 31, 315–326. doi: 10.1016/j.tree.2016.02.001
Luykx, P. (1990). A cytogenetic survey of 25 species of lower termites from Australia. Genome 33, 80–88. doi: 10.1139/g90-013
Martins, V. G., and Mesa, A. (1995). Two permanent linear chains of sex chromosomes in Neotermes fulvescens and karyotypes of two other neotropical Kalotermitidae species (Insecta, Isoptera). Genome 38, 958–967. doi: 10.1139/g95-126
Miyaguni, Y., Agarie, A., Sugio, K., Tsuji, K., and Kobayashi, K. (2021). Caste development and sex ratio of the Ryukyu drywood termite Neotermes sugioi and its potential mechanisms. Sci. Rep. 11, 15037–15038. doi: 10.1038/s41598-021-94505-w
Miyaguni, Y., Sugio, K., and Tsuji, K. (2013). The unusual neotenic system of the Asian dry wood termite, Neotermes koshunensis (Isoptera: Kalotermitidae). Sociobiology 60, 65–68. doi: 10.13102/sociobiology.v60i1.65-68
Miyazaki, S., Fujiwara, K., Kai, K., Masuoka, Y., Gotoh, H., Niimi, T., et al. (2021). Evolutionary transition of Doublesex regulation from sex-specific splicing to male-specific transcription in termites. Sci. Rep. 11, 1–12. doi: 10.1038/s41598-021-95423-7
Myles, T. G., and Chang, F. (1984). The caste system and caste mechanisms of Neotermes connexus (Isoptera: Kalotermitidae). Sociobiology 9, 163–319.
Nagin, B. R. (1972). Caste determination in Neotermes jouteli (banks). Insect. Soc. 19, 39–61. doi: 10.1007/BF02223214
Neoh, K. B., and Lee, C. Y. (2011). Developmental stages and caste composition of a mature and incipient colony of the drywood termite, Cryptotermes dudleyi (Isoptera: Kalotermitidae). J. Econ. Entomol. 104, 622–628. doi: 10.1603/EC10346
Palmer, D. H., Rogers, T. F., Dean, R., and Wright, A. E. (2019). How to identify sex chromosomes and their turnover. Mol. Ecol. 28, 4709–4724. doi: 10.1111/mec.15245
Presgraves, D. C., Severance, E., and Wilkinson, G. S. (1997). Sex chromosome meiotic drive in stalk-eyed flies. Genetics 147, 1169–1180. doi: 10.1093/genetics/147.3.1169
Roisin, Y. (1992). Development of non-reproductive castes in the neotropical termite genera Cornitermes, Embiratermes, and Rhynchotermes. Insect. Soc. 39, 313–324. doi: 10.1007/BF01323951
Roisin, Y. (2000). “Diversity and evolution of caste patterns” in Termites: Evolution, Sociality, Symbioses, Ecology. eds. T. Abe, D. E. Bignell and M. Higashi (Dordrecht: Kluwer Academic Press), 95–119.
Roisin, Y. (2001). Caste sex ratios, sex linkage, and reproductive strategies in termites. Insect. Soc. 48, 224–230. doi: 10.1007/PL00001770
Roisin, Y., and Pasteels, J. M. (1991). Sex ratio and asymmetry between the sexes in the production of replacement reproductive in the termite, Neotermes papua (Desneux). Ethol. Ecol. Evol. 3, 327–335. doi: 10.1080/08927014.1991.9525361
Sugio, K., Miyaguni, Y., and Yoshimura, T. (2020). Colony structure and caste distribution in living trees of the Ryukyu drywood termite, Neotermes sugioi (Blattodea: Kalotermitidae) in Okinawa Island. J. Asia Pac. Entomol. 23, 853–862. doi: 10.1016/j.aspen.2020.07.013
Syren, R. M., and Luykx, P. (1977). Permanent segmental interchange complex in the termite Incisitermes schwarzi. Nature 266, 167–168. doi: 10.1038/266167a0
Úbeda, F., Patten, M. M., and Wild, G. (2015). On the origin of sex chromosomes from meiotic drive. Proc. Royal Soc. B. 282:20141932. doi: 10.1098/rspb.2014.1932
Werren, J. H. (2011). Selfish genetic elements, genetic conflict, and evolutionary innovation. Proc. Natl. Acad. Sci. U. S. A. 108, 10863–10870. doi: 10.1073/pnas.1102343108
Werren, J. H., and Beukeboom, L. W. (1998). Sex determination, sex ratios and genetic conflict. Ann. Rev. Ecol. Syst. 29, 233–261. doi: 10.1146/annurev.ecolsys.29.1.233
Werren, J. H., Nur, U., and Wu, C. I. (1988). Selfish genetic elements. Trends Ecol. Evol. 3, 297–302. doi: 10.1016/0169-5347(88)90105-X
Wood, R. J., and Newton, M. E. (1991). Sex-ratio distortion caused by meiotic drive in mosquitoes. Am. Nat. 137, 379–391. doi: 10.1086/285171
Yashiro, T., Takematsu, Y., Ogawa, N., and Matsuura, K. (2019). Taxonomic assessment of the termite genus Neotermes (Isoptera: Kalotermitidae) in the Ryukyu-Taiwan Island arc, with description of a new species. Zootaxa 4604, 549–561. doi: 10.11646/zootaxa.4604.3.10
Keywords: termite, sex ratio, selfish genetic elements, genetic conflict, sex determining system
Citation: Agarie A, Miyaguni Y, Sugio K, Tsuji K and Kobayashi K (2023) Male’s influence on the primary sex ratio bias in Ryukyu drywood termite. Front. Ecol. Evol. 10:1081043. doi: 10.3389/fevo.2022.1081043
Edited by:
Silvio Erler, Julius Kühn-Institut—Braunschweig, GermanyReviewed by:
Simon Hellemans, Okinawa Institute of Science and Technology Graduate University, JapanRobert Hanus, Academy of Sciences of the Czech Republic (ASCR), Czechia
Copyright © 2023 Agarie, Miyaguni, Sugio, Tsuji and Kobayashi. This is an open-access article distributed under the terms of the Creative Commons Attribution License (CC BY). The use, distribution or reproduction in other forums is permitted, provided the original author(s) and the copyright owner(s) are credited and that the original publication in this journal is cited, in accordance with accepted academic practice. No use, distribution or reproduction is permitted which does not comply with these terms.
*Correspondence: Ayaka Agarie, YXlhbW9jaGk0NTUxQGdtYWlsLmNvbQ==