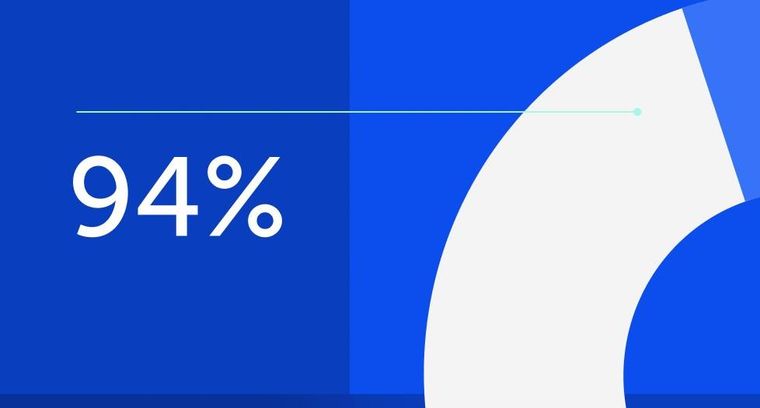
94% of researchers rate our articles as excellent or good
Learn more about the work of our research integrity team to safeguard the quality of each article we publish.
Find out more
ORIGINAL RESEARCH article
Front. Ecol. Evol., 21 December 2022
Sec. Population, Community, and Ecosystem Dynamics
Volume 10 - 2022 | https://doi.org/10.3389/fevo.2022.1080599
This article is part of the Research TopicUntangling the Complexity: Recent Advances in Understanding Interactions Between Plants, Pathogens and Herbivorous InsectsView all 5 articles
In the present study, field surveys were conducted to detect potential associational susceptibility of crop plants (potato, alfalfa and maize) caused by Canadian goldenrod, Solidago canadensis (L.), one of the most common invasive weeds in Central and Eastern Europe, via plant associated virus vectoring aphids. Assessments were made in two major agricultural land types: crops grown under high input vs. low-input (LIF) conditions, with and without fertilizers and pesticides. The two most frequent aphid species, found both on Canadian goldenrod and crop plants, were the leaf-curling plum aphid, Brachycaudus helichrysi Kaltenbach and the glasshouse-potato aphid, Aulacorthum solani (Kaltenbach). Plant viruses in both weed and crop plants were identified using high-throughput sequencing of small RNAs. Peroxidase (POD) enzyme activity was tested in weed and crop plants to connect aphids feeding processes in weeds and crops. In addition, conceptual modeling was used to detect direct relationships between viruses and other crops. The Canadian goldenrod density was only relevant in the LIF regime. Furthermore, its association with B. helichrysi and associational susceptibility was detected only in LIF. In total, 18 viruses comprising 17 plant and one insect virus were detected in Canadian goldenrod, of which 11 were also detected in potato and alfalfa crops. POD activity was high and correlated with high aphid density in both weed and crop plants, suggesting a direct associational susceptibility between these plants through aphid infestation and viral transmission.
Over the past 25 years studies performed on invasive weeds in Central Europe have recorded 435 alien species within 82 plant families. One of the most frequently found and identified as highly invasive is Canadian goldenrod, Solidago canadensis L., which occurs in a wide variety of habitats, including the verges of main roads, along railway tracks and verges, arable lands and even gardens, parks and cemeteries (Anastasiu and Negrean, 2005). Although weed control strategies exists in European countries, including mulching, tilling and burning as well as chemical control and crop management approaches, i.e., crop rotation (Uchino et al., 2012; Mabuza et al., 2013), the areas encroached upon by this plant in Central Europe are generally increasing (Tunaitienė et al., 2015; Pacanoski, 2017).
Beside the problems of invasion and pollen expansion caused by Canadian goldenrod, the latter causing severe allergies within the human population, another important issue is the plant’s suitability as a host for local pest aphid species. Several local sap-feeding pest insect populations, especially aphids, readily use Canadian goldenrod as a summer host; in the case of aphids, these may create so-called ‘associational susceptibility’ (AS) with local crops, such that the aphids transmit pathogenic plant viruses from this reservoir host plant to nearby or adjacent crop plants, factors rarely considered when the importance of an invasive weed are considered. To date, the fact that invasive weeds may act as virus reservoirs and hence pose a potentially serious threat via local migrating winged aphid species in terms of virus vectoring has yet to be tested under different crop management systems (Bell, 1983; Popkin et al., 2017). Having said that, several local aphid species, notably the leaf-curling plum aphid, Brachycaudus helichrysi Kaltenbach and the glasshouse-potato aphid, Aulacorthum solani (Kaltenbach), have already been tested to determine whether invasive weeds such as annual fleabane, Stenactis (= Erigeron) annua (L.) and Canadian horseweed, Erigeron (= Conyza) canadensis can act as summer ‘reservoir’ hosts for pest aphids, thereby allowing transmission of pathogenic crop plant viruses from these plants to adjoining crop plants (Szabó et al., 2019, 2020).
Clearly, direct and indirect interactions between plants in close proximity due to aphid infestation and viral transmission exists and the influence of one plant (i.e., an invasive weed in the present case) to another plant or plants (i.e., crop plants) may increase AS or decrease ‘associational resistance (AR)’, according to the potential importance of the focal herbivore abundances connecting these particular plants (Barbosa et al., 2009). Another important factor is the aspect on habitat diversity (i.e., diverse habitat surrounded by natural landscape mosaics) and conventional management systems (intensive crop management involving high chemical input), both of which may influence these relations between new invasive weeds and existing crop plants (Steffan-Dewenter et al., 2001).
To date, only a relatively few aspects of these plant-aphid-plant relationships have so far been investigated, although one such aspect is the induction of volatiles by plants when these are injured, injuries influencing the probability of detection of neighboring plants (i.e., crops) by winged pest aphids, as originally demonstrated by Quiroz et al. (1997). These authors noted that undamaged wheat attracted higher numbers of aphids than that already infested by aphids. Another aspect is that some stress enzymes such as peroxidase (POD) are induced when plant cells are injured, the infected plants being perceived by attacking aphids as lower quality hosts, at least for a short while. In this way, POD enzyme activation is a good indicator of AS, i.e., high POD activity is correlated with high levels of infestation (Argandoña et al., 2001; Chaman et al., 2001; War et al., 2012; Scully et al., 2016).
Considering these various aspects, the main aims of the present study were to: (i) assess the population density of Canadian goldenrod in two differently managed agricultural crops field systems, i.e., with fertilizers and chemical pesticide input (high-input fields, HIF) and without significant management (low-input fields, LIF), respectively; (ii) assess the local abundance of aphids adapting to Canadian goldenrod along with actual detection of infection of these plants via POD activity; and (iii), compare and contrast the aphid population densities in adjacent crop plants along with detection of common plant viruses occurring within weed and crops, respectively The overarching hypothesis we wished to test was whether Canadian goldenrod forms associational susceptibility of crop plants via local aphids within a short time frame and if so, thereby represents a potentially serious threat as a plant pathogenic viral reservoir within the European agroecosystem.
Field assessment of weeds and associated aphids were conducted during the crop growing season from spring to autumn over a three-year period (2015–17) in Transylvania, Romania. Surveys were performed under the two aforementioned management regimes (low vs. high input fields), purposely selected to reflect similar geographic and climatic regions. The low-input (LIF) fields were characterized by traditionally managed fields of the Old Saxon region, some 7,440 km2 in area at an altitude of 230–800 m above sea level (a.s.l.), the HIF by large monocultures with a low density of natural vegetation, situated about 5,500 km2 in area at an altitude of 220–750 m a.s.l. (Szabó et al., 2019, 2020). Around 200 km separated the areas with no direct connections between them via main roads or railways.
Canadian goldenrod, S. canadensis (L.) is native to north-eastern and north-central America, grows in a diverse range of habitats, and is one of the most aggressive invasive weeds in Europe, resulting in significant crop losses in newly invaded areas (Anastasiu and Negrean, 2005; Abhilasha et al., 2008; Fenesi et al., 2015).
The weed assessment method here used has earlier been detailed by Szabó et al. (2019, 2020). In brief, two sample areas each of around 10m2 were selected in both LIF and HIF regions. Inside each, 10 smaller 1×1 m subplots were assessed and the exact coverage of all plants (invasive and other weeds) identified. Bi-weekly assessments were made in the same subplots from the end of May until June during the 2-year vegetation growing period.
Canadian goldenrod together with aphid colonies were collected and stored at −20°C. Aphids were counted in the laboratory and species identified according to Blackman and Eastop (2000) and Blackman (2010). For each sample collection period, 100 samples were collected per transect and management system, i.e., 400 samples/management system/collection data = total 1,600 samples/year. A similar sample collection system was followed for crop plants around each weed transect. Because only potato, alfalfa and maize were cultivated, samples from these crops were collected and tested for viruses. All plant material was stored at 70°C prior to virus identification.
The two native and locally-distributed aphid species investigated, the leaf-curling plum aphid, B. helichrysi Kaltenbach, and the foxglove aphid, A. solani (Kaltenbach), are both considered to be highly polyphagous (Blackman and Eastop, 2000; Blackman, 2010), and as such, important virus vectors. In B. helichrysi, hosts include members of the Asteraceae, e.g., Chrysanthemum, as well as Prunus spp., Citrus, Fragaria, Medicago, Solanum, Trifolium and also maize (Popkin et al., 2017). The most important viruses transmitted are Beet mild yellowing virus, Potato virus Y and Sharka virus (= Plum Pox virus; Isac et al., 1998). Hosts of A. solani include several crop plants, e.g., celery, carrots, cucurbits, peppers, tomato, tobacco, tulip bulbs, and legumes (Jandricic et al., 2014), the aphid transmitting Potato viruses A, Y, and X, Potato leaf roll virus, Bean yellow mosaic virus, Cucumber mosaic virus, Soybean dwarf virus and Turnip yellows virus (Jandricic et al., 2010, 2014).
Analyses were made according to White and Kaper (1989) and Szabó et al. (2020). Material from both weed and crops was separately homogenized in an ice-cold mortar in 650 μl extraction buffer (100 mM glycine, pH 9.0, 100 mM NaCl, 10 mM EDTA, 2% SDS and 1% sodium lauroylsarcosine). Samples were mixed with phenol, centrifuged for 5 min., whereafter the aqueous phase was treated with a mixture of phenol, chloroform and isoamyl-alcohol (25:24:1). Following further treatment using chloroform: isoamyl-alcohol (24:1), the solution was precipitated with ethanol and re-suspended in sterilized water (White and Kaper, 1989; Szabó et al., 2020). Polyacrylamide gels from RNA pools were used for small RNA High Throughput Screening (HTS), separately for samples related to weed, crops, area and collection times. This approach allowed detection of any plant viruses present in samples at any given time during the survey. When investigating field crops belonging to different plant families (potato, alfalfa and maize) and also when the origin of cultivars was uncertain, their virus patterns were pooled. These pools were used for small RNA library preparation (2 libraries, one for weed and one for crop plants) using Truseq Small RNA Library Preparation Kit (Illumina, USA; Czotter et al., 2018). Thereafter, all samples were sequenced using HiScan2000 by UD Genomed (Debrecen, Hungary) 50 bp, single end procedures (Supplementary Figure S1). Fastq files of the sequenced libraries were deposited in the Gene Expression Omnibus (GEO) repository1, accessible via series accession number GSE132755 (Szabó et al., 2019, 2020). Aphids from each plants (weed and crop) were also tested for viruses; the insects were carefully collected from plants during feeding, and stored in absolute ethanol together with the plant portion containing the aphids’ stylets, prior to virus testing.
The analyses were made in 2022. For POD enzyme analysis, weed and crop plant leaf samples were collected each year and date simultaneously with the aphid samples collected from the various fields and transects. In this way, aphid-plant interactions through high POD activity could be directly tested. Leaves and stems from the top of the plants containing aphids were sampled from Canadian goldenrod (n = 10 / 1 m2 sub-transect) and crop plants (n = 7 / 1 m2 sub-transect), using 20 samples from each transect (Supplementary Table S1), and stored at −20°C prior to enzyme extraction and activity assays. For this, ~ 400 mg of leaves were homogenized in 1 ml 50 mM phosphate buffer, pH 7.0, in a high-speed benchtop homogenizer (MP Biomedicals). The concentration of enzyme extract was determined using the Bradford method (1976; Szabó et al., 2019), whereafter POD activity was analyzed according to Németh et al. (2009). The increase in absorbance at λ 480 nm was followed in a spectrophotometer for 5 min., with POD activity separately defined as an absorbance change of 0.01 units.min −1 at both 5 and 10 μmol min−1. mg protein.−1
Besides the fact that only potato, alfalfa and maize crops were present in both years of the assessment of crops and aphids detected around stands of Canadian goldenrod, some viruses other than those infecting these crops were also identified. In light of this, a detailed survey of the scientific literature was performed to assess the virus infection potential of crops studied and also other cash crops. All viruses detected in Canadian goldenrod and the aforementioned crops were analysed and scientific references searched using WoS (Web of Science) for the years 2000–22 inclusive. From this, all possible crop plants with potential as aphid hosts in Europe (i.e., in which viral symptoms had at one time been found) were noted.
Firstly, for the Canadian goldenrod data, a mean coverage value in each 1 m2 sub-quadrat was computed, involving averaging all the species relative abundance data from each 1 × 1 m plot per sampling date. As goldenrod was only present in low abundance under HIF conditions, its average coverage of 0.8% did not allow further analyses, so only data from LIF was considered. Next, analyses of inter-annual differences in coverage were compared using MANOVA, whereupon the mean values of weed coverage for one 1 m2 quadrat (40 data / field type / collection dates) were used. No significant difference in coverage were detected between the 2 years of the survey (p = 0.34); therefore cumulated and averaged data were used for analyses.
Thereafter, aphid abundances were assessed on both weeds and crop plants. Aphid abundance on Canadian goldenrod did not meet the assumption of normality so that the Kruskal-Wallis test was used, followed by the Mann–Whitney U test to compare the abundance of the two aphid species’ on this host plant. Aphid density analyses of crop plants were performed by considering only the abundance of B. helichrysi. This was because the abundance of A. solani was too low in crop plants (except potato), so that no statistics were possible using this data set. As the B. helichrysi aphid data was normally distributed, ANOVA followed by Tukey testing were performed to compare abundances between treatments and crop plants. All analyses were done using R version 3.0.1 (R Core Team, 2013). Other aphid species were also detected, but in small numbers (e.g., Macrosiphum spp.), hence were not included in the analyses.
Virus diagnosis was determined by small RNA HTS for weed and crops. For bioinformatics analysis, CLC Genomic Workbench was used. For plant virus diagnostics two strategies were followed. Firstly, longer contigs from the non-redundant reads were built and the CLC assembler (de novo assembly) used. Next, directly mapped contigs were made using Reference Genomes of those viruses, which were characterized at least with one contig in any of the libraries (CLC Genomic workbench).Virus incidence in samples was claimed if at least two parameters (virus specific contig and/or normalized redundant virus specific read count) was >> 200, and/or coverage of the virus genome was > > 60%; (Szabó et al., 2019, 2020). Because some viruses detected from weeds could not be directly associated with those detected in crops in close proximity to Canadian goldenrod, a detailed analyses of scientific references was made to associate these viruses with other potential crops present in the area.
POD activity values for weed and crop plants at 5 and 10 μmol min−1 were compared between years (n = 20 / year) using MANOVA, with values from 1 m2 sub-transects per sampling period considered. Because no significant year effect was observed (p = 0.83), data between years were averaged and used for further analyses. Similarly with weed data, where only samples from LIF were considered. POD enzyme data at 5 and 10 μmol min−1. mg protein−1 unit were analyzed separately and compared between assessment data, when aphids abundances were the highest in weed and crop plants (June 05–15), and also when aphid abundance decreased (between June 15 and 24) each year (Supplementary Table S1). Because data were normally distributed, a complete randomized factorial ANOVA of POD specific activity values (5 and 10 μmol min−1. mg protein−1 unit) was used for comparison. The Tukey test with p < 0.01 and LSMEAN (Minimal quadratic means) test were performed using the statistic package SAS. For analyses, the average POD quantity / 20 plants / 1 m2 sub-transect were used. Linear correlation analyses between POD activity level at both 5 and 10 μmol min−1. mg protein−1 unit and aphid species (B. helichrysi and A solani) abundances on Canadian goldenrod were computed for the same assessment period using the SPSS package, version 3.14.
The whole analyses was performed in 2022. To link virus infections with crop plants, four major factors influencing infections were considered: (1) the weed-aphid species interactions identified as in our assessments; (2), plant viruses identified in both weed and crop plants using new generation small RNA assessment; (3), virus incidences observed and identified suggesting a direct link between weeds and crop plants via aphid-viral interactions; and (4), management strategies formulated to control virus infections. From these, only papers dealing with at least three factors were considered. To link factors, the number of searches that connected these factors, i.e., weed-virus-crops (other than those assessed by us) were considered. Of 44 papers searched between 2000 and 2022, a strong link was found between factors connecting Canadian goldenrod and crop plants, such information being indicative of viral transmission in at least one direction (i.e., virus transfer from weed to crop via aphids; Reference list, Supplementary online materials). Strong and influential links were considered between two factors when at least 10 searches were found that connected these in one direction (and marked in Figure 1 as conceptual representations). This was done by placing the factors (weed, aphids and crop plants) in a Sankey diagram representing direct contact. The method used is rather similar to those used for factors modeling within the DPI (Driving force–Pressure–Impact) framework, reporting different environmental issues facilitating interrelations between these and human and/or social factors (Gabrielsen and Bosch, 2003).
Figure 1. Sankey diagram representing direct contact between Canadian goldenrod and crop plants due to plant pathogenic virus transmission via B. helichrysi. To link virus infections with crop plants, data from scientific references were collected and four major factors that influence infections were considered: 1, the same weed-aphid species interactions identified as in our assessments; 2, plant viruses identified in both weed and crop plants using new generation small RNA assessment; 3, virus incidences were observed and were identified as a direct link between weeds and crop plants through aphids; and 4, Management strategies were formulated to control virus infections. The model fits with factors modeling within the DPI (Driving force–Pressure–Impact) framework (Gabrielsen and Bosch, 2003).
Canadian goldenrod was present in LIF fields at an average coverage of 2.5%. In contrast, at the other site with high input management, its average coverage in transects was 0.8%; thus samples from this site were not used to test for the presence of viruses. A higher density of B. helichrysi was detected on goldenrod (U1–40 = 3.4, p < 0.01) compared with A. solani. Other species (Macrosiphum spp) were observed at low density (12 individuals) on S. canadensis. Considering aphids on crop plants, the abundance of B. helichrysi was higher on alfalfa and potato and significantly lower on maize (F1–40 = 4.5 p < 0.01; Figure 2A), while the abundance of A. solani was always low so that no statistics were possible, but a higher abundance on potato was clearly observed (Figure 2B).
Figure 2. Brachycaudus helichrysi (A) and Aulacorthum solani (B) abundance on crop plants. Data for B. helichrysi from goldenrod were compared using Kruskal-Wallis followed by Mann–Whitney U tests. Data from crop plants were compared using ANOVA followed by Tukey testing. Bars represent standard errors. No statistics were done for A. solani because of low abundances.
The small RNA libraries sequencing resulted in more than 21 million raw reads for crop plants and more than 17 million for Canadian goldenrod. After trimming and quality control, > > 20 million raw reads were obtained for crops and > > 16 million for weeds (Table 1). Overall, the number of plant viruses detected in Canadian goldenrod from LIF was 18, of which 17 were plant viruses, and one an insect virus. Of the plant viruses detected, 15 were RNA and two DNA viruses (Table 2). Altogether 11 viruses were found to be directly associated with crop plants assessed during the study, mostly alfalfa and potato, whereas no particular virus associations were discovered with maize. The vast majority of the viruses detected were associated with plants from the family Solanaceae; all viruses that infect these plants (Potato virus Y, V, S, M, X, Wild potato mosaic virus and Bidens mosaic virus isolate SP01) were found in goldenrod and in potato crops all over the LIF area sampled. Furthermore, two viruses infecting sweet pepper and potato were detected (Pepper severe mosaic virus and Pepper yellow mosaic virus). Bean leaf roll virus and the Lucerne transient streak virus, both infecting plants of the family Fabaceae, were also detected in goldenrod and alfalfa at all sampling sites (Table 2). Amongst other viruses associated with Canadian goldenrod, some were highly plant pathogenic, including PPV (Plum Pox Virus), Sunflower ring blotch virus, Sunflower chlorotic mottle virus and Tobacco vein clearing virus. Two viruses associated with sweet-potato may be considered important in terms of potential virus vectoring (Sweet potato virus G isolate and Sweet potato mastrevirus), both present in goldenrod, although its presence in host plants other than sweet potato has yet to be assessed. The presence of the insect virus (Helicoverpa zea nudivirus-2) in Canadian goldenrod is a new finding, with no previous confirmed record of its occurrence in Europe (Table 2).
While considerable efforts were made to extract and detect these plant viruses from aphid stylets collected from weeds and crop plants, this proved technically impossible in our particular study due to the low titer of virus present.
Upon assessing POD enzyme activity, this was found to be detectable at both 5 and 10 μmol min−1. mg protein−1 unit, its activity increasing when aphids feeding was at its highest on the sampled plant (June 05–15 each year; Figure 3). A very similar trend was observed in crop plants. Furthermore, a high positive correlation between aphids abundance and POD activity level was detected on weeds (Figure 3), along with a very similar trend in crop plants (a Rho value of <0.79 at 5, and < 0.8 at 10 μmol min−1. mg protein−1 for B. helichrysi on potato, and < 0.77 at 5, and < 0.82 at 10 μmol min−1. mg protein−1 on alfalfa). Because of the low density of A. solani on crops, no comparison were made.
Figure 3. POD enzyme activity values at 5 and 10 μmol min−1. mg protein−1 unit in Canadian goldenrod. Data were compared using complete randomized factorial ANOVA of POD specific activity values. The Tukey test with p < 0.01 and LSMEAN (Minimal quadratic means) test were performed using the statistic package SAS. The average POD quantity / 20 plants / 1 m2 sub-transect were used. Linear correlation analyses between POD activity level at both 5 and 10 μmol min−1. mg protein−1 unit and aphid species (B. helichrysi and A solani) abundances on Canadian goldenrod were computed for the same assessment period.
Conceptual analyses of viruses identified in the present assessment revealed that the most vulnerable crops appeared to be members of the Solanaceae. In the case of Canadian goldenrod, which belongs to the family Asteracae, this is currently expanding its range in mainland Europe, followed next by species from the family Fabaceae, e.g., alfalfa. In addition, the possibility of virus infection via aphid transmission from goldenrod to Helianthus, Chenopodiaceae and also Stone fruits (peaches, plums, cherries, etc.) are high. As Sweet potato cultivation is also expanding in Europe, viral infection of this crop is likely when Canadian goldenrod is present (Figure 1).
As shown in this study, a clear associational susceptibility of crop plants potato and alfalfa in the presence of Canadian goldenrod occurs as a direct result of local aphids acting as vectors of plant pathogenic viruses, here B. helichrysi and A. Solani. These aphids are also important because of their ability to infect other crops too, such as Helianthus, Chenopodiaceae and Stone fruits. It is also apparent that in the case of conventional management when weed and pest control is enacted (i.e., HIF), the abundance of Canadian goldenrod is low, such that its potential as a reservoir of plant pathogenic viruses is insignificant. In contrast, when no adequate management efforts are made (i.e., LIF), even at low density, this weed is potentially important in terms of aphid borne viral transmission to adjacent agricultural crops.
Several factors seem to positively influence the associational susceptibility of crop plants caused by Canadian goldenrod. The likelihood of detection of neighboring plants by aphids, including settling, feeding and asexual propagation, and the successional availability that can directly sustain aphid populations and influence the survival of local colonies on these plants, seemingly include natural habitat diversity, and lack of any management, including any chemical (pesticide/herbicide) application under the LIF regime conditions used. Therefore, the general perception about lack of any management (more specifically weed management) inside protected areas needs to be reconsidered. In this particular scenario, the close proximity and high population density of virus vectoring B. helichrysi infesting Canadian goldenrod and their (mainly) aerial dispersal to adjacent crop plants (here alfalfa and potato) clearly influenced the associational susceptibility observed (Figure 2). Colonization experiments with this aphid species also demonstrated that other invasive weed species (Stenactis annua and Erigeron canadensis) are suitable hosts for this aphid species, while its colonization from these weeds toward maize, alfalfa and potato has earlier been demonstrated (Szabó et al., 2019).
While we made determined efforts to extract and identify viruses from the stylets of aphids attacking either weeds or crop plants, such efforts proved unsuccessful, undoubtedly due to the titer of the various viruses within aphid stylets being very low, whereas it is much amplified in the host plant itself, allowing detection. Indeed, our previous colonization experiments (Szabó et al., 2019) clearly demonstrated that B. helichrysi colonizing crop plants from nearby weeds involves the transmission of plant pathogenic virus by these insects.
High POD activity on goldenrod, as here shown to be correlated with the density of infesting aphids on weeds and nearby crop plants, argues that goldenrod is a high quality host for B. helichrysi, supporting large aphid populations. This in turn suggests that a significantly higher stress is caused by aphids feeding on goldenrod and on crop plants too, a conjecture, if true, further supporting the virus transmission potential of this aphid from weeds to crops (Figure 3). That plant-induced POD activity increases as a consequence of sap-feeding insect activity was first revealed by Felton et al. (1989), reviewed by War et al. (2012). Other similar studies have also revealed the anti-herbivore role of POD, arising, as it appears to do, as a consequence of the herbivore defense-inducing signal molecules systemin and methyl jasmonate (MeJA), which in turn trigger higher POD activity levels in – for example – tomato leaves (Constabel et al., 1995; Constabel and Barbehenn, 2008; Mai et al., 2016). Hence, evidence exists that the increased level of POD is associated with intensive feeding by aphids, resulting in injury of the plant’s cells (Dicke, 1998; Argandoña et al., 2001; Balog et al., 2017). Other similar studies on barley, Hordeum vulgare L, also report that infestation of the plant by the greenbug (aphid), Schizaphis graminum (Rondani) increased the total soluble POD activity, which was also associated with a maximum level of hydrogen peroxide activity (H2O2), after around 20 min of insect feeding (Argandoña et al., 2001). Taken together, these findings strongly suggest that the increase of POD activity in both weed and crop plants is directly associated with the processes of aphid feeding, while similar viruses detected in goldenrod and crop plants (potato and alfalfa in this cases) reflect the direct transfer of virus/es from goldenrod to crops.
Conceptual analyses of viruses identified in this study also revealed that the most vulnerable crops in Europe in relation to invasive Canadian goldenrod are plant species of the family Solanaceae, followed by species of Fabaceae. In addition, the possibility of virus infection to species of Helianthus, Chenopodiaceae and Stone fruits are high (Figure 1). As for the Lepidoptera-infecting Helicoverpa zea nudivirus 2, this has not been reported from Europe before (EPPO listings; www.eppo.int). Further analyses are necessary to test its origin in Canadian goldenrod, along with its possible effect on local lepidopteran species. The type and nature of the viruses as here detected using small RNA HTS could be supported in future studies by the use of RT-PCR.
In conclusion, our findings show that even when low-input management farming systems are widely studied, and conservation protocols implemented in such areas (Akeroyd and Page, 2011; Fischer et al., 2012; Mikulcak et al., 2013), the total abandonment or absence of proper weed management may cause serious AS of some crops and hence create difficulties in terms of aphid-borne viral incidence due to increased local aphid virus vectoring. These areas are considered as low-cost (i.e., no or low management costs) effective systems with high biodiversity and cultural values (Hartel et al., 2013). Even so, damage to crops produced by virus infections transmitted by aphids infesting goldenrod may exceed the costs of any environmentally-friendly weed control. If this is so, new weed management systems and new research is necessary to evaluate the effect/s of weeds as virus reservoirs, even under low management regimes, as well as in many other ecosystems in Europe where invasive weed problems are on the increase.
The original contributions presented in the study are included in the article/Supplementary material, further inquiries can be directed to the corresponding author.
All applicable international, national, and/or institutional guidelines for the care and use of animals were followed. The article does not contain any studies with human participants performed by any of the authors.
A-KS, AB, and JB perceived and designed the experiment. A-KS and SA performed the small RNA HTS. A-KS, JB, HDL, AM, and AB performed the data collections. A-KS, AB, HDL, CF, and AM performed the data analyses, and wrote the paper. All authors contributed to the article and approved the submitted version.
Virus and enzyme activity assessments were funded by the UEFISCDI Romania, project PN-III-P4-PCE-2021-0543.
The authors are grateful to all those, master students and local people, who helped in various scientific assessments and supported our field research.
The authors declare that the research was conducted in the absence of any commercial or financial relationships that could be construed as a potential conflict of interest.
All claims expressed in this article are solely those of the authors and do not necessarily represent those of their affiliated organizations, or those of the publisher, the editors and the reviewers. Any product that may be evaluated in this article, or claim that may be made by its manufacturer, is not guaranteed or endorsed by the publisher.
The Supplementary material for this article can be found online at: https://www.frontiersin.org/articles/10.3389/fevo.2022.1080599/full#supplementary-material
Abhilasha, D., Quintana, N., Vivanco, J., and Joshi, J. (2008). Do allelopathic compounds in invasive Solidago canadensis s.l. restrain the native European flora? J. Ecol. 96, 993–1001. doi: 10.1111/j.1365-2745.2008.01413.x
Akeroyd, J., and Page, N. (2011). Conservation of high nature value (HNV) grassland in a farmed landscape in Transylvania, Romania. Contrib. Bot. 46, 57–71.
Anastasiu, P., and Negrean, G. (2005). Alien plants in Romania (I). Analele Stiintifice ale Universitatii" Al. I. Cuza" din Iasi 51:87.
Argandoña, V. H., Chaman, M., Cardemil, L., Muñoz, O., Zúñiga, G. E., and Corcuera, L. J. (2001). Ethylene production and peroxidase activity in aphid-infested barley. J. Chem. Ecol. 27, 53–68. doi: 10.1023/A:1005615932694
Balog, A., Loxdale, H. D., Bálint, J., Benedek, K., Szabó, K.-A., Jánosi-Rancz, K.-T., et al. (2017). The arbuscular mycorrhizal fungus Rhizophagus irregularis affects arthropod colonization on sweet pepper in both the field and greenhouse. J. Pest. Sci. 90, 935–946. doi: 10.1007/s10340-017-0844-1
Barbosa, P., Hines, J., Kaplan, I., Martinson, H., Szczepaniec, A., and Szendrei, Z. (2009). Associational resistance and associational susceptibility: having right or wrong neighbors. Annu. Rev. Ecol. Evol. Syst. 40, 1–20. doi: 10.1146/annurev.ecolsys.110308.120242
Bell, A. C. (1983). The life-history of the leaf-curling plum aphid Brachycaudus helichrysi in Northern Ireland and its ability to transmit potato virus YC(AB). Ann. Appl. Biol. 102, 1–6. doi: 10.1111/j.1744-7348.1983.tb02660.x
Blackman, R., and Eastop, V. (2000). Aphids on the world’s crops: an identification and information guide. Available at: http://eu.wiley.com/WileyCDA/WileyTitle/productCd-0471851914.html (Accessed March 10, 2016).
Bradford, M. M. (1976). A rapid and sensitive method for the quantitation of microgram quantities of protein utilizing the principle of protein-dye binding. Anal. Biochem. 72, 248–254. doi: 10.1016/0003-2697(76)90527-3
Chaman, M. E., Corcuera, L. J., Zúñiga, G. E., Cardemil, L., and Argandoña, V. H. (2001). Induction of soluble and cell wall peroxidases by aphid infestation in barley. J. Agric. Food Chem. 49, 2249–2253. doi: 10.1021/jf0011440
Constabel, C. P., and Barbehenn, R. (2008). “Defensive roles of polyphenol oxidase in plants,” in Induced Plant Resistance to Herbivory. ed. A. Schaller (Dordrecht: Springer), 253–270.
Constabel, C. P., Bergey, D. R., and Ryan, C. A. (1995). Systemin activates synthesis of wound-inducible tomato leaf polyphenol oxidase via the octadecanoid defense signaling pathway. PNAS 92, 407–411. doi: 10.1073/pnas.92.2.407
Czotter, N., Molnár, J., Pesti, R., Demián, E., Baráth, D., Varga, T., et al. (2018). “Use of siRNAs for diagnosis of viruses associated to Woody plants in nurseries and stock collections” in Viral Metagenomics: Methods and Protocols Methods in Molecular biology. eds. V. Pantaleo and M. Chiumenti (New York, NY: Springer), 115–130.
Dicke, M. (1998). Induced responses to herbivory by R. Karban and I.T. Baldwin. Trends Ecol. Evol. 13:83. doi: 10.1016/S0169-5347(97)01267-6
Felton, G. W., Donato, K., Vecchio, R. J. D., and Duffey, S. S. (1989). Activation of plant foliar oxidases by insect feeding reduces nutritive quality of foliage for noctuid herbivores. J. Chem. Ecol. 15, 2667–2694. doi: 10.1007/BF01014725
Fenesi, A., Geréd, J., Meiners, S. J., Tóthmérész, B., Török, P., and Ruprecht, E. (2015). Does disturbance enhance the competitive effect of the invasive Solidago canadensis on the performance of two native grasses? Biol. Invasions 17, 3303–3315. doi: 10.1007/s10530-015-0954-8
Fischer, J., Hartel, T., and Kuemmerle, T. (2012). Conservation policy in traditional farming landscapes. Conserv. Lett. 5, 167–175. doi: 10.1111/j.1755-263X.2012.00227.x
Gabrielsen, P., and Bosch, P. (2003). Environmental Indicators: Typology and Use in Reporting. Copenhagen: European Environment Agency, 20.
Hartel, T., Dorresteijn, I., Klein, C., Máthé, O., Moga, C. I., Öllerer, K., et al. (2013). Wood-pastures in a traditional rural region of Eastern Europe: characteristics, management and status. Biol. Conserv. 166, 267–275. doi: 10.1016/j.biocon.2013.06.020
Isac, M., Preda, S., and Marcu, M. (1998). Aphid species--vectors of plum pox virus. Acta. Virol. 42, 233–234.
Jandricic, S. E., Mattson, N. S., Wraight, S. P., and Sanderson, J. P. (2014). Within-plant distribution of Aulacorthum solani (Hemiptera: Aphididae), on various greenhouse plants with implications for control. J. Econ. Entomol. 107, 697–707. doi: 10.1603/EC13428
Jandricic, S. E., Wraight, S. P., Bennett, K. C., and Sanderson, J. P. (2010). Developmental times and life Table statistics of Aulacorthum solani (Hemiptera: Aphididae) at six constant temperatures, with recommendations on the application of temperature-dependent development models. Environ. Entomol. 39, 1631–1642. doi: 10.1603/EN09351
Mabuza, M. L., Sithole, M. M., Wale, E., Ortmann, G. F., and Darroch, M. A. G. (2013). Factors influencing the use of alternative land cultivation technologies in Swaziland: implications for smallholder farming on customary Swazi nation land. Land Use Policy 33, 71–80. doi: 10.1016/j.landusepol.2012.12.009
Mai, V. C., Tran, N. T., and Nguyen, D. S. (2016). The involvement of peroxidases in soybean seedlings’ defense against infestation of cowpea aphid. Arthropod Plant Interact. 10, 283–292. doi: 10.1007/s11829-016-9424-1
Mikulcak, F., Newig, J., Milcu, A. I., Hartel, T., and Fischer, J. (2013). Integrating rural development and biodiversity conservation in Central Romania. Environ. Conserv. 40, 129–137. doi: 10.1017/S0376892912000392
Németh, Z., Pozsgai-Harsányi, M., Gálos, B., and Albert, L. (2009). Stress sensitivity of correlation between POD and PPO activities in plants. Acta Silvatica et Lignaria Hungarica 5, 27–45.
Pacanoski, Z. (2017). Current situation with invasive Erigeron annuus (l.) Pers. (daisy fleabane) in the republic of Macedonia. EPPO Bull. 47, 118–124. doi: 10.1111/epp.12368
Popkin, M., Piffaretti, J., Clamens, A.-L., Qiao, G.-X., Chen, J., Vitalis, R., et al. (2017). Large-scale phylogeographic study of the cosmopolitan aphid pest Brachycaudus helichrysi reveals host plant associated lineages that evolved in allopatry. Biol. J. Linn. Soc. 120, 102–114. doi: 10.1111/bij.12869
Quiroz, A., Pettersson, J., Pickett, J., Wadhams, L., and Niemeyer, H. (1997). Semiochemicals mediating spacing behavior of bird cherry-oat aphid, Rhopalosiphum padi feeding on cereals. J. Chem. Ecol. 23, 2599–2607. doi: 10.1023/B:JOEC.0000006669.34845.0d
R Core Team (2013). R: A Language and Environment for Statistical Computing. R Foundation for Statistical Computing, Vienna, Austria. ISBN 3-900051-07-0, URL http://www.R-project.org/
Scully, E. D., Donze-Reiner, T., Wang, H., Eickhoff, T. E., Baxendale, F., Twigg, P., et al. (2016). Identification of an orthologous clade of peroxidases that respond to feeding by greenbugs (Schizaphis graminum) in C4 grasses. Funct. Plant Biol. 43, 1134–1148. doi: 10.1071/FP16104
Steffan-Dewenter, I., Münzenberg, U., and Tscharntke, T. (2001). Pollination, seed set and seed predation on a landscape scale. Proc. R. Soc. Lond. Ser. B: Biol. Sci. 268, 1685–1690. doi: 10.1098/rspb.2001.1737
Szabó, A.-K., Kiss, J., Bálint, J., Kőszeghi, S., Loxdale, H. D., and Balog, A. (2019). Low and high input agricultural fields have different effects on pest aphid abundance via different invasive alien weed species. NeoBiota 43, 27–45. doi: 10.3897/neobiota.43.31553
Szabó, A.-K., Várallyay, É., Demian, E., Hegyi, A., Galbács, Z. N., Kiss, J., et al. (2020). Local aphid species infestation on invasive weeds affects virus infection of nearest crops under different management systems – a preliminary study. Front. Plant Sci. 11:684. doi: 10.3389/fpls.2020.00684
Tunaitienė, V., Naugžemys, D., Patamsytė, J., and Žvingila, D. (2015). Gradient of genetic diversity of erigeron Annuus in the part of invasive European range. Bot. Lithuanica 21, 81–88. doi: 10.1515/botlit-2015-0011
Uchino, H., Iwama, K., Jitsuyama, Y., Ichiyama, K., Sugiura, E., Yudate, T., et al. (2012). Effect of interseeding cover crops and fertilization on weed suppression under an organic and rotational cropping system: 1. Stability of weed suppression over years and main crops of potato, maize and soybean. Field Crop Res. 127, 9–16. doi: 10.1016/j.fcr.2011.10.007
War, A. R., Paulraj, M. G., Ahmad, T., Buhroo, A. A., Hussain, B., Ignacimuthu, S., et al. (2012). Mechanisms of plant defense against insect herbivores. Plant Signal. Behav. 7, 1306–1320. doi: 10.4161/psb.21663
Keywords: invasive weeds, virus vector aphids, vegetable crops, cropping systems, weed management
Citation: Szabó A-K, Bálint J, Molnár A, Aszalos SE, Fora CG, Loxdale HD and Balog A (2022) Associational susceptibility of crop plants caused by the invasive weed Canadian goldenrod, Solidago canadensis, via local aphid species. Front. Ecol. Evol. 10:1080599. doi: 10.3389/fevo.2022.1080599
Received: 26 October 2022; Accepted: 07 December 2022;
Published: 21 December 2022.
Edited by:
Sayanta Bera, University of Maryland, United StatesReviewed by:
Shirin Parizad, University of Idaho, United StatesCopyright © 2022 Szabó, Bálint, Molnár, Aszalos, Fora, Loxdale and Balog. This is an open-access article distributed under the terms of the Creative Commons Attribution License (CC BY). The use, distribution or reproduction in other forums is permitted, provided the original author(s) and the copyright owner(s) are credited and that the original publication in this journal is cited, in accordance with accepted academic practice. No use, distribution or reproduction is permitted which does not comply with these terms.
*Correspondence: Attila-Károly Szabó, c3phYm9fYXRhQHlhaG9vLmNvbQ==; Adalbert Balog, YWRhbGJlcnQuYmFsb2dAbXMuc2FwaWVudGlhLnJv
Disclaimer: All claims expressed in this article are solely those of the authors and do not necessarily represent those of their affiliated organizations, or those of the publisher, the editors and the reviewers. Any product that may be evaluated in this article or claim that may be made by its manufacturer is not guaranteed or endorsed by the publisher.
Research integrity at Frontiers
Learn more about the work of our research integrity team to safeguard the quality of each article we publish.