- 1Department of Environmental Sciences, COMSATS University Islamabad (CUI), Abbottabad, Pakistan
- 2College of Engineering and Science, Victoria University, Melbourne, VIC, Australia
- 3Center of Excellence in Water Resources Engineering, University of Engineering and Technology, Lahore, Pakistan
- 4Department of Development Studies, COMSATS University Islamabad (CUI), Abbottabad, Pakistan
- 5Department of Environmental Sciences, Kohsar University Murree, Murree, Pakistan
- 6Arid Land Research Center, Tottori University, Tottori, Japan
- 7College of Engineering, IT & Environment, Charles Darwin University, Darwin, NT, Australia
- 8Department of Botany, Kohsar University Murree, Murree, Pakistan
- 9College of Overseas Education, Fujian Agriculture and Forestry University, Fuzhou, China
Productivity of an orchard generally depends upon the fertility of the soil and the nutrient requirements of the fruit trees. Phosphorus (P) extractability from soils influences the P sorption, release patterns, and P bioavailability. A study was carried out to investigate P extractability via seven extraction methods in relation to soil properties in three fruit orchards. In total, 10 soil samples were collected from each fruit orchard, namely, citrus (Citrus sinensis L.), loquat (Eriobotrya japonica L.), and guava (Psidium guajava L.), located in similar ecological conditions to the Haripur district of Pakistan. Available P in the soil was extracted using deionized H2O, CaCl2, Mehlich 1, Bray 1, Olsen, HCl, and DTPA methods. Selected soil properties [pH, electrical conductivity (EC), soil organic matter (SOM)], texture, cation exchange capacity (CEC), macronutrients, and micronutrients were also determined. Soils sampled from orchards indicated significant differences in soil properties. Orchards have sequestered more amount of C stock in soil than without an orchard. The extractability of P from soils was profoundly affected by P extraction methods. The average amount of extractable P was relatively higher in those soils where the total amount of P was also higher. These methods extracted different pools of soil P with varying P concentrations regulated by the soil properties. Phosphorus amounts extracted were varied in the order of HCl > DTPA > Mehlich 1 > Bray 1 > Olsen > CaCl2 > water. Among orchards, a higher amount of P was found in soils of loquat followed by citrus and guava orchards. Regardless of the method, subsurface soil got a lower concentration of extractable P than surface soil in all orchards. The extractable P was highly associated with soil properties. DTPA extractable P was related to SOM soil clay content and CEC by R2 values of 0.83, 0.87, and 0.78, respectively. Most of the extraction methods were positively correlated with each other. This study indicated that SOM inputs and turnover associated with orchard trees exhibited a substantial quantity of extractable P in soils. Predicting available P in relation to its bioavailability using these methods in contrasting soils is required.
1 Introduction
Fertilization of orchards generally depends on the fertility of the soil and the nutrient requirements of the fruit trees. Fruit plants require essential nutrients such as nitrogen (N), phosphorus (P), and potassium (K) for their growth and fruit production. Regular replenishment of nutrient elements is required for the quality of fruit trees. Soil nutrients play a vital role when present in the soil in an adequate quantity for healthy fruit trees. Nitrogen is required for the vigorous vegetative and floral growth of trees (Gul et al., 2006). Phosphorus is important for the normal cell division, growth, and establishment of sugar-phosphate (Zhang et al., 2019). Soil P is an essential nutrient for plant growth and biomass production (Smith et al., 2015). The provision of P is essential for living organisms as it involves major processes of metabolism, e.g., energy transfer in the form of adenosine triphosphate (ATP). During the process of photosynthesis, plants depend on P nutrients to get energy (Ruttenberg, 2003). Therefore, the importance of P for plants is highly recognized. The optimal quantity of macronutrients is essential for quality fruit production (Aggelopoulou et al., 2011). The deficiency of P in soils retards the growth and productivity of fruit trees (Nazarkiewicz and Kaniuczak, 2012). The quality of fruit production is also associated with the availability of P (Li et al., 2021). Phosphorus is essential for the physiological and biochemical processes of plants (Simpson et al., 2011).
The quality of soil is regulated by both physical and chemical properties of soils and their mutual interactions (Carter, 2002). The availability of nutrients from soil depends upon the soil properties in the rhizosphere (Jiang et al., 2009). These interactions are essential for the balance of the agroecosystem (Cataldo et al., 2021). The physical indicators (e.g., aggregate stability, soil texture, bulk density, and soil porosity) and chemical indicators [e.g., soil organic carbon (SOC), electrical conductivity (EC), pH, and cation exchange capacity (CEC)] for the quality of soil have been reported (Zornoza et al., 2015). The productivity of soil depends on several soil physicochemical properties. For instance, soil texture influences soil biophysical properties and is interrelated with soil fertility and soil quality (Upadhyay and Raghubanshi, 2020). The soil pH affects the bioavailability of nutrients (Berbecea et al., 2011). The optimum soil pH controls the microbial activities in the soil and enhances the availability of mineral nutrients (Jarociński, 2005). Plants absorb P in the form of orthophosphate ions H2PO4– and HPO42– (Becquer et al., 2014). The uptake of phosphate decreases as the pH of the soil solution increases (White, 2012).
Besides soil properties, orchard production is also controlled by plant nutrients in the soil (Zia et al., 2006). The deficiency of plant nutrients is a major constraint to the productivity and sustainability of soils (Chaudhari et al., 2012). Poor soil fertility leads to low fruit productivity (Kai et al., 2016). Optimal soil fertility with adequate nutrients is required for better yield (Hoying et al., 2004; Brunetto et al., 2015). The concentration of P in the soil is affected by the interaction of soil properties (Hinsinger, 2013; Messiga et al., 2015). The livelihood and nutritional security of farmers depend on fruit production; therefore, the restoration of the soil is important for the orchard sustainability (Tejada et al., 2006). Nutritional deficiencies in horticultural gardens are common (Urade et al., 2019). The soil of the fruit trees needs to be analyzed for enhancing nutrient management. Average yields of fruit crops in Pakistan are lower than potential yields due to the imbalanced proportion of plant nutrients in soil despite the favorable climatic conditions (Zia et al., 2006). Measurement of available P in soil is required for efficient P use. Phosphorus application to soils is generally based on soil analyses, for which different soil P extraction methods have been used (Wuenscher et al., 2015). In less developed soils, the ranges of average total P are 200–800 mg/kg (Nwoke et al., 2003). Phosphorus characterization in soils is important because P forms influence P desorption/release patterns and their bioavailability. This also depends on the precise measurement of the phyto-availability of P from soil. The average amount of organic P has been reported as 30–65% of the total P (Condron and Tiessen, 2005; Bibi et al., 2022). In soil, major forms of P are as follows: (1) dissolved P in soil water, (2) sorbed P of clay/mineral surfaces or Al and Fe oxides, (3) P in the primary phosphate minerals, and (4) P in living organisms and organic substances. Therefore, numerous extraction methods of soil P have been developed depending on the extracting agents and soil properties. Different methods have been reported for the extraction of distinct pools of soil P, and the extractability of a given pool has been influenced by the soil properties to different extents (Zehetner et al., 2018).
Applications of chemical P fertilizers and animal manure to agricultural land improve the soil P fertility (Shen et al., 2011). Phosphorus in soils is supplied from the weathering process of the primary mineral apatite (Zhou et al., 2018) and the application of both inorganic and organic fertilizers. A small portion of total P in soils is available for agricultural plants and microorganisms (Sayers et al., 2008) because most of the P is strongly bound in soil particles and partially weathered material or occluded in secondary minerals. Application of chemical fertilizers and good crop and soil management can optimize P availability. The relationships between extractable P and plant-available P are predicted by keeping in view soil properties and P forms (Delgado and Torrent, 2001). In total, 16 methods of extraction for the available P have been reported (Tóth et al., 2014). Estimating available P in soils is important for sustainable nutrient management. Therefore, investigating P fertility in soils is desirable for the productivity of fruit orchards. Studies on the availability of P from orchard soil in relation to soil properties for better yield potential and quality of fruit in the Haripur district of Pakistan are lacking. The quality of soil is associated with the mutual interactions of the physicochemical and biological properties of soils (Messiga et al., 2015). Soil quality and restoration of plant nutrients in fruit orchards are important for sustainable productivity.
Several P extraction methods have been utilized to extract the inorganic form of P, which is also considered bioavailable. Soil properties apparently influence the extraction of P from soils; therefore, the availability of P from soil differs with an extracting agent (Haque et al., 2013). Moreover, an extraction method needs to be identified, which may accurately reflect the available P in soils of different land uses. The interrelation of P extractability from soils and major soil properties in field conditions of orchards has not been sufficiently reported. The study hypothesized the differences in P extraction from soils of three orchards. Research on the availability of P in relation to soil properties is necessary for better yield potential of orchards. Therefore, the objective of the study was to investigate P extractability using seven extraction methods in relation to soil properties under the field conditions of three fruit orchards. Investigation of available P in an agroecosystem is important to adopt appropriate fertilizer use and soil management. This study may serve as a guideline for the efficient and sustainable management of fruit production. This would provide an opportunity to attain social and economic security for the poorly resourced farmers of the region.
2 Materials and methods
2.1 Soil sampling and analyses
The soil was sampled from three types of fruit orchards, namely, citrus (Citrus sinensis L.), loquat (Eriobotrya japonica L.), and guava (Psidium guajava L.), located in the Haripur district of Hazara Division, Pakistan (Figure 1). These orchards were selected because of their abundance in the area. The weather of Haripur has relatively high temperatures and precipitation is evenly distributed throughout the year. The average rainfall is less than 550 mm. The average temperature in summer and winter ranges between 2 and 40°C. Loquat and citrus species are evergreen trees, and guava is a deciduous fruit species. These orchards were 12–15 years of age. In each orchard, 10 random subplots (5 m × 5 m) were selected for soil samples from the two layers of soil (0–25 and 25–50 cm depths) as composite soil samples (Sumera et al., 2022). There were ten soil samples from each orchard collected separately from both soil depths. The experiment was a factorial (3 types of orchards × 2 soil depths), resulting in 6 experimental units arranged into a completely randomized block design. There were three fruit orchards. Thus, the total number of soil samples was 60. The land features have been properly considered during the soil sampling. These soil samples were mixed appropriately in plastic bags. Composite soil samples were taken from the adjoining fields of each orchard as control soil. The control fields have been cultivated with cereal crops (wheat and maize rotation) for a longer period. The topography of the orchard and crop land was flat. Soil samples were collected randomly from each core with an auger and kept in polythene bags under moist conditions. The materials (stones, granules, plant parts, and leaves) were carefully removed from soil samples. Samples were air-dried, ground, and passed via a 2-mm sieve. There were three types of orchards and two layers of soil. The adjacent field of the orchard was considered as a control treatment.
Soil samples were analyzed for physical and chemical properties, i.e., pH, EC, organic matter, texture (sand, silt, and clay), potassium (K), magnesium (Mg), calcium (Ca), and sodium (Na). The soil organic matter (SOM) was calculated by the dry combustion method (Cambardella et al., 2001). Total N content was determined using the Kjeldahl method. The pH was determined using a pH meter (Model: HANNA HI 8520) and EC by electrical conductivity meter (Model: 4320 JENWAY) at a ratio of 1:5 of soil and water (Chi and Wang, 2010). Soil texture was determined using a hydrometric method (Huluka and Miller, 2014). Soil bulk density was estimated by a core method (Throop et al., 2012) using the mass of soil (g) in relation to the volume of soil (cm3). Concentrations of total macro- and micro-elements (P, Ca, Mg, K, Zn, Cu, Mn, and Fe) in soil were determined after digesting the soil samples in (1:3) mixture of perchloric acid (HClO4) and nitric (HNO3) acid. The contents were determined using an atomic absorption spectrophotometer (AAS) (Model: Analyst 700, Perkin Elmer) (Miller et al., 2013). Calcium, Mg, and K were extracted by ammonium acetate and these exchangeable cations were determined using AAS.
The total carbon stock for the 0- to 30-cm soil layer was estimated based on a hectare.
where C = carbon (t ha–1); BD soil = soil bulk density (g cm–3); Depth soil = soil depth.
In this equation, C is expressed as a decimal fraction, e.g., 2.2% carbon is expressed as 0.022 in the equation.
2.2 Phosphorus extraction methods
(1) Available P content from soil was extracted using deionized water. During this method, 10 g of soil was added to 50 ml of deionized water. Samples were shaken for 1 h and centrifuged at 10,000 rpm for 10 min and filtered via a 0.22-μm filter paper. The water extraction method determines dissolved or readily soluble P forms in soil (Zehetner and Wenzel, 2000). (2) Calcium chloride (CaCl2): the CaCl2 extraction was prepared following Houba et al. (2000). This is based on the principle of a salt solution (dilute) making some ion exchange process, but it depends on the dissolution of P in extraction solution (Wuenscher et al., 2015). (3) Olsen method: in this method, 0.5 M sodium bicarbonate (NaHCO3) solution (at 8.5 pH) was used for the extraction of P from the soil (Olsen et al., 1954; Otabbong et al., 2009). (4) Mehlich 1 method: for this method, a mixture of 0.05 M hydrochloric acid (HCl) + 0.025 M sulfuric acid (H2SO4) was used to dissolve Fe and Al phosphates and adsorbed P (Mehlich, 1953). (5) By Bray and Kurtz P 1 method: P contents were extracted with extracting solution of 0.025 M HCl in 0.03 M NH4F (Bray and Kurtz, 1945; Sims, 2000). (6) Hydrochloric acid (HCl) method: this method dissolved the inorganic P using 0.5 mol/L HCl (Pagliari and Laboski, 2012). (7) DTPA extractable P method: in this procedure, the P associated with apatite was dissolved and aluminum (Al) and iron (Fe) bound P was extracted (Kuo, 1996; Memon, 2008), using 1 M ammonium bicarbonate (NH4HCO3) + 0.005 M diethylene triamine penta acetic acid (DTPA) solution (at pH 7.6) (Tan, 1995). During each procedure, the soil samples were shaken and centrifuged at 10,000 rpm for 10 min, and then, the supernatants were filtered through a 0.22-μm filter paper. The samples were analyzed for P contents using the phosphomolybdate blue color method via a spectrophotometer (Model: LI-UV-7000) at 710 nm (Murphy and Riley, 1962).
For the determination of the total concentration of P, the soil sample was digested in a mixture (1:3) of perchloric (HClO4) and nitric (HNO3) acids. For wet digestion, a soil sample weighing 5 g was transferred into a flask, treated with 24 ml of concentrated HNO3, and left to digest overnight. After adding 8 ml of HCIO4, the flask was heated gently until the digestion of soil material. Upon cooling, contents were filtered through Whatman No. 40 filter paper and the desired volume was diluted with deionized water. The samples were analyzed for P contents using the phosphomolybdate blue color method via a spectrophotometer (Model: LI-UV-7000) at 710 nm (Murphy and Riley, 1962).
Data were statistically analyzed using a StatView software (SAS, 1999). The analysis of variance (ANOVA) technique was used to differentiate soil properties among three orchards, and the level of significance was determined via the least significant difference (LSD) value at p < 0.05. A summary of a two-way analysis of variance for orchard effects on soil properties is also given (Table 5). The relationship of extractable P using the above methods with selected soil properties was determined using regression plot analysis.
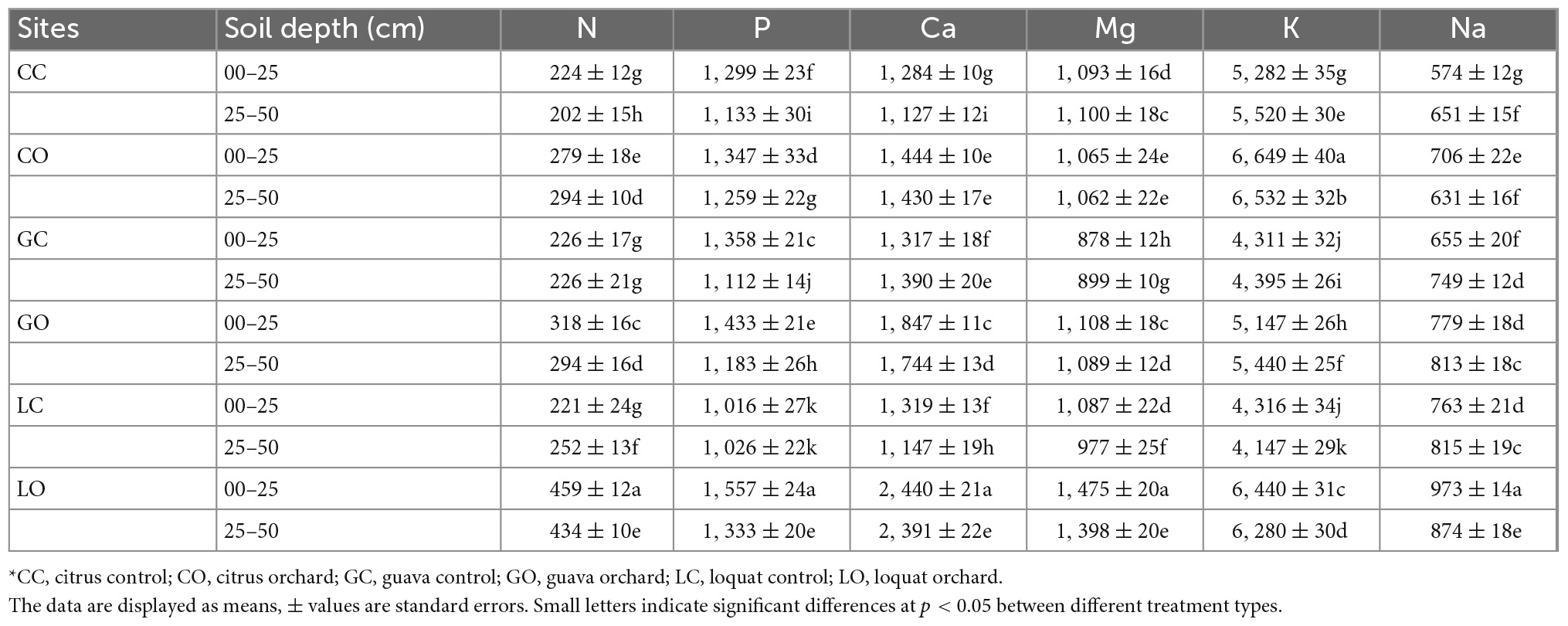
Table 2. Changes in nutrients concentrations (mg kg–1) in soils as influenced by three types of fruit orchards*.
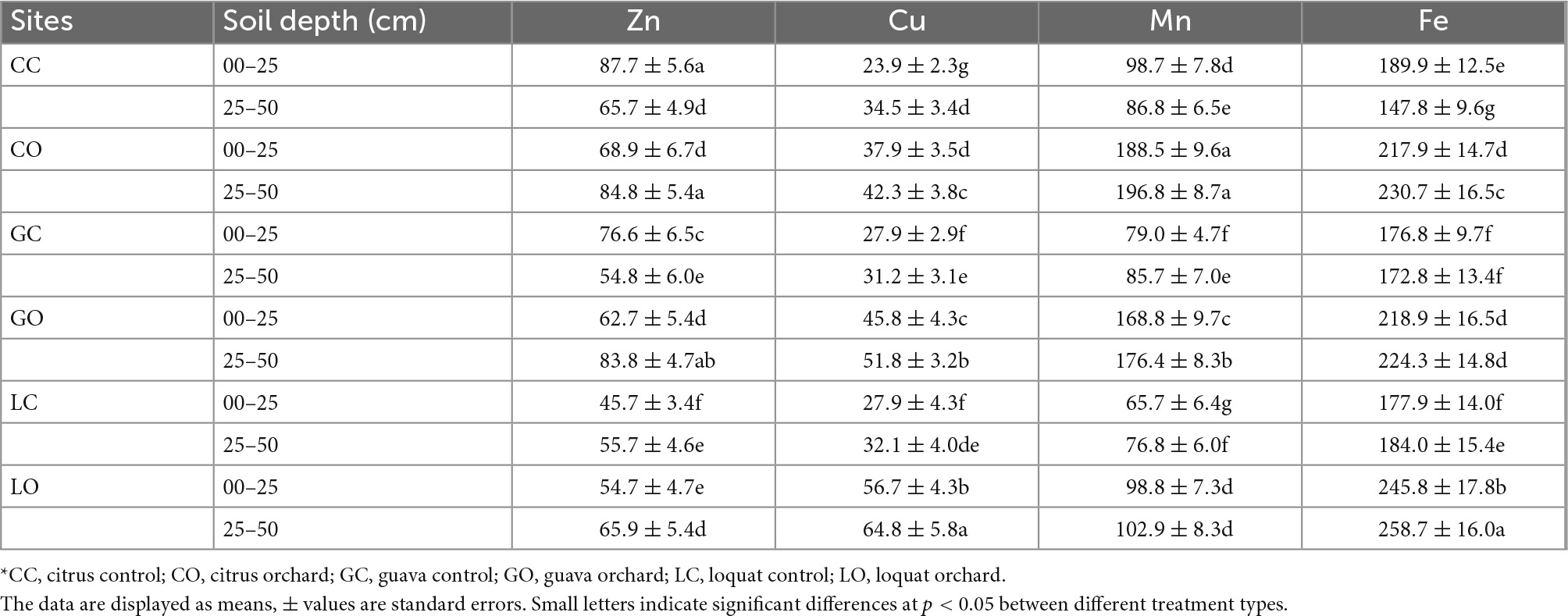
Table 3. Changes in trace elements concentrations (mg kg–1) in soils as influenced by three types of fruit orchards*.
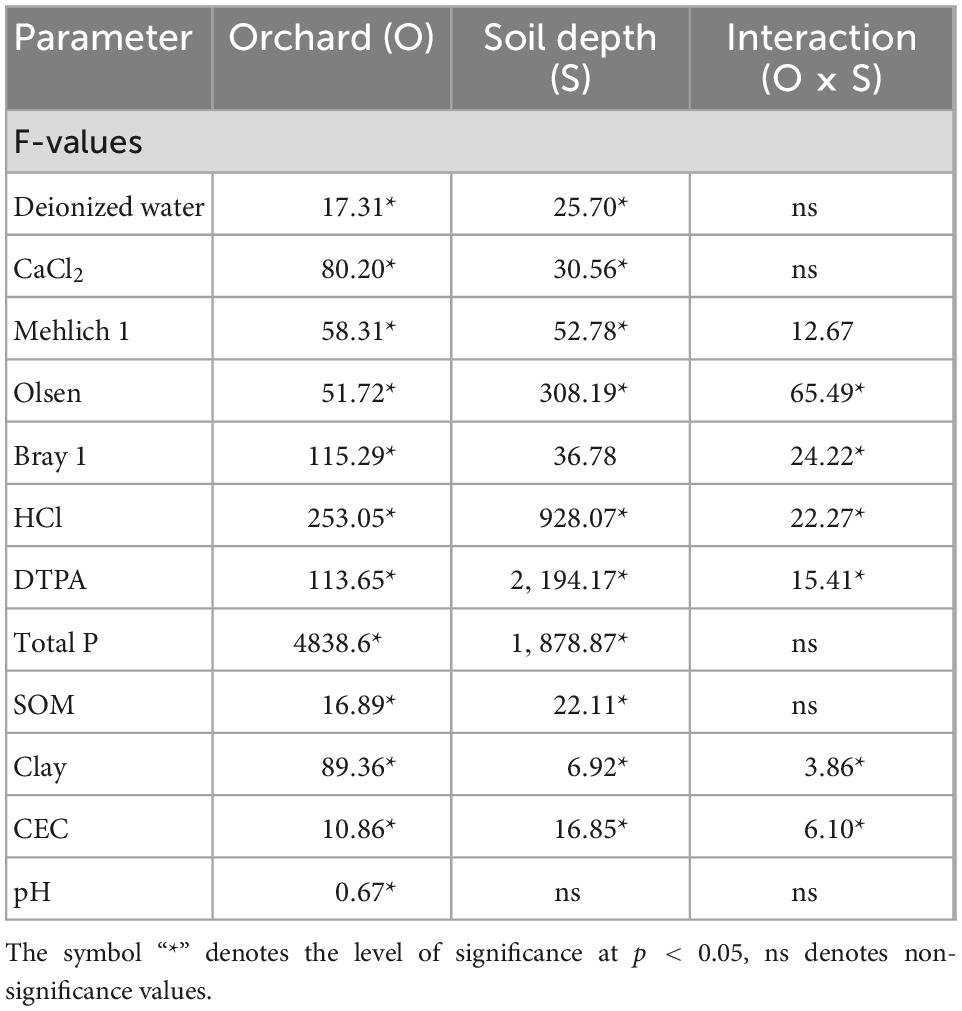
Table 5. Summary of the two-way analysis of variance (ANOVA) for the effect of orchards on soil properties.
3 Results
3.1 Description of soil properties
Soil samples collected from different orchards showed significant differences among most of the soil properties (p < 0.05) (Tables 1–3). The different uses of land with fruit orchards significantly affected the soil properties when compared to the control soil, i.e., without an orchard. The orchard trees brought tangible changes in the chemical composition depending on the nature of the soil and the type of orchard. A higher amount of SOM was observed in orchard soil as compared to control soil irrespective of the fruit orchard. Fallen leaf litter of citrus, guava, and loquat orchards have accumulated 2.6, 1.9, and 3.1 times more SOM in the surface soil (0–25 cm) than in the control fields. In subsurface soil (25–50 cm), the SOM contents were 3.3, 2.4, and 3.9 times higher than the respective control soil of fruit orchards. Orchard soil accumulated a substantial amount of C stock (t ha–1) when compared to the respective control fields (Figure 2). Loquat and citrus trees have apparently sequestered more amount of C stock than the guava orchard after comparing with the control soil. This phenomenon could be associated with higher biomass, plant growth pattern, and litter inputs in soil CEC of soil, which was higher in soil planted with loquat trees followed by citrus and guava trees. The bulk density of soil was lower in orchard soil with a higher amount of SOM. The orchard soil exhibited slightly higher pH values than the control soil. The pH of the surface soil was higher than subsurface soil, regardless of the tree species. Under the orchard conditions, SOM, CEC, and essential plant nutrients were found higher in the topsoil as compared to the subsoil.
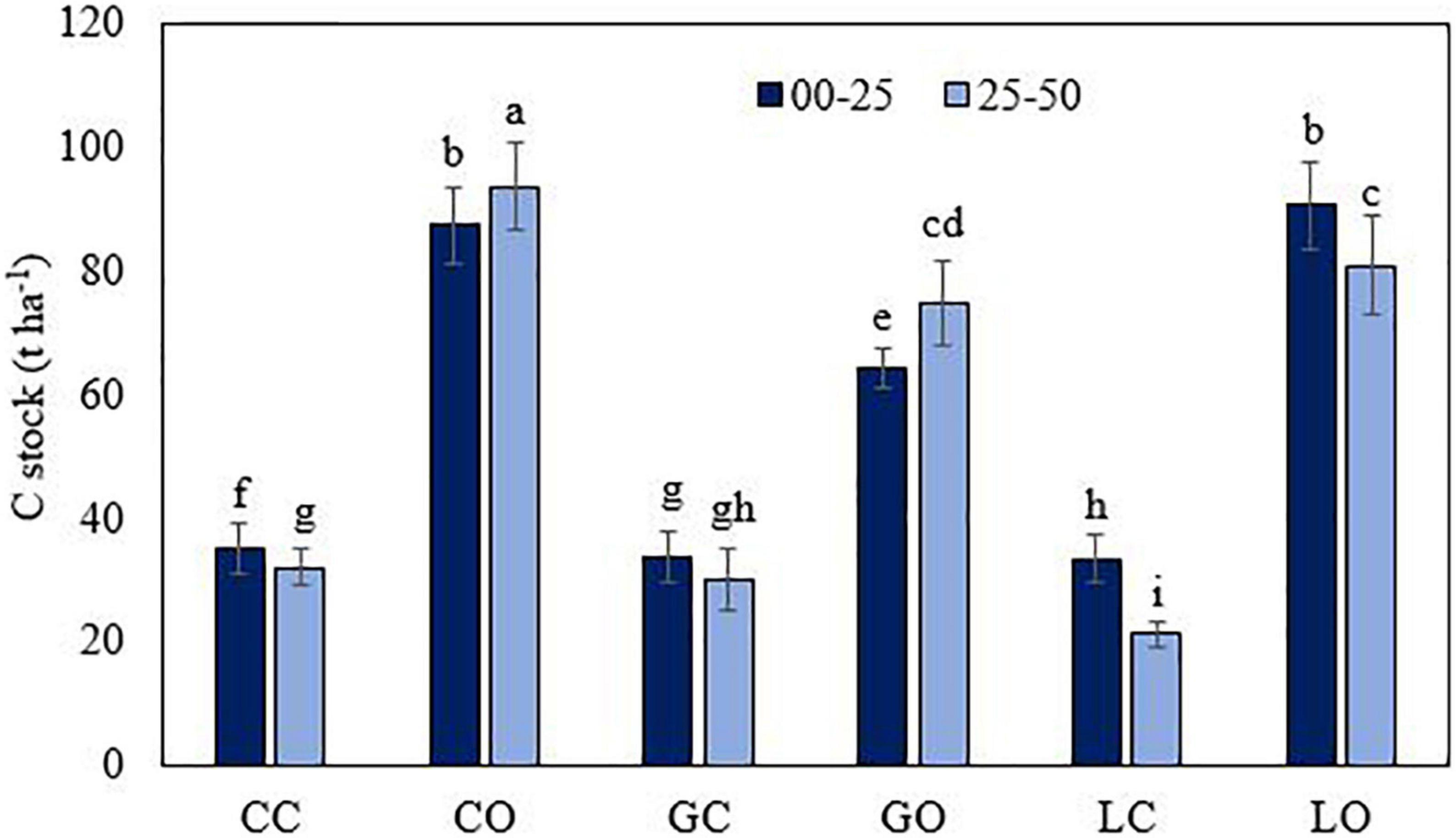
Figure 2. Carbon stock in soil (0–30 cm layer) as affected by three types of orchards. CC, citrus control; CO, citrus orchard; GC, guava control; GO, guava orchard; LC, loquat control; LO, loquat orchard. “±” Values are standard errors. Significant differences between the treatments at p ≤ 0.05 are indicated by different letters.
Nutrient concentrations (N, P, Ca, Mg, and K) were higher in soils under horticultural trees. The concentrations of nutrients were found as loquat > citrus > guava (Tables 2, 3). Total P contents were enhanced by citrus, guava, and loquat orchards by 9.3, 18.6, and 53.2%, respectively, in the surface soil as compared to respective control soils. The contents were increased in subsoils under the orchard conditions by 11.1, 13.7, and 29.9%, respectively. Total N contents were enhanced by citrus, guava, and loquat orchards by 24.5, 40.1, and 107.6%, respectively, in the surface soil. The N contents were increased in subsurface soils under these orchards by 45.5, 30.0, and 72.2%, respectively. The cationic elements were differentiated in soils among tree species as K > Ca > Mg > Na. Micronutrients in soils were found in the order of Cu < Zn < Mn < Fe (Table 3). Manganese concentration was higher in the soil of the citrus orchard followed by guava and loquat. Copper was higher in the soil after being cultivated with the loquat species and lower in the soil of citrus. Iron concentration was achieved higher in soil under loquat and citrus compared with guava. Zinc was substantially higher in the citrus orchard followed by guava when compared to the soil samples of the loquat orchard.
3.2 Phosphorus extraction methods
The average amount of extractable P was found significantly higher (p < 0.05) in those soils of fruit orchards where the total amount of P was higher. The amounts of extractable P via different extractants were varied in the order of HCl > DTPA > Mehlich 1 > Bray 1 > Olsen > CaCl2 > water (Figures 3–7). Almost all extractants exhibited an identical pattern of P release from soil samples, i.e., higher from orchard soil and lower from control soil. The widest range between the lowest and highest P value was found for Mehlich 1, where the highest P value was 579 mg kg–1 and the lowest value was 268 mg kg–1 (Figure 4A). The narrowest range was observed for the HCl extraction among soils, where the maximum extracted P was 549 mg kg–1, and the minimum value was 425 mg kg–1 (Figure 5B). Irrespective of the extraction method used, the average amount of extractable P was found higher in soils in the order of loquat > citrus > guava orchard. Water extractable P concentrations in the surface soil were achieved as 223, 183, and 181 mg kg–1 in loquat, guava, and citrus orchards, respectively. Adjacent control fields of these orchards exhibited water extractable P in the topsoil (0–25 cm) as 104, 129, and 160 mg kg–1, respectively. The lowest extractable P concentrations in soils were found for the water extraction method, and the highest amounts were extracted by the method used for total P. The P concentrations extracted with CaCl2 in the surface soils were obtained in the control fields of citrus, guava, and loquat as 145, 167, and 164 mg kg–1, respectively. CaCl2 extractable P concentrations in soils sampled from these orchards were 212, 228, and 242 mg kg–1, respectively. Mehlich 1 P was higher in the soil of loquat followed by guava and citrus orchards. Olsen P differed in the order of guava < citrus < loquat orchard. Bray 1 P was enhanced in the surface soil by 6.8, 26.2, and 119.2% in the fields of citrus, guava, and loquat orchards, respectively, when compared to control fields (Figure 5A). Irrespective of the extraction method, subsurface soils gave a lower amount of extractable P than surface soils in all fruit orchards. Extractable P of HCl in soil was increased from 452 to 487 mg kg–1 by citrus plants, from 447 to 528 mg kg–1 by guava, and from 452 to 549 mg kg–1 by loquat orchard as compared to control soil. Similarly, DTPA extractable P concentrations were also higher in soil under fruit trees as compared to control soil (Figure 6). Among the soils, the loquat orchard substantially increased the extractable P in the soils as compared to other orchards. This could indicate that P accumulation in soil occurred due to the addition of P from the plant litter. The extractable P was highly correlated with the SOM, soil texture, and CEC of soils. This also suggested the transformation of organic P into inorganic form in organic matter due to microbial activities. The correlation matrix showed that most of the extraction methods were correlated positively (Table 4). The result indicates that the ability of P extraction was different for different methods, but the pattern of P release from soil was similar. This is in agreement with the findings of Haque et al. (2013). A summary of the two-way analysis of variance for orchards’ effects on soil properties is given in Table 5.
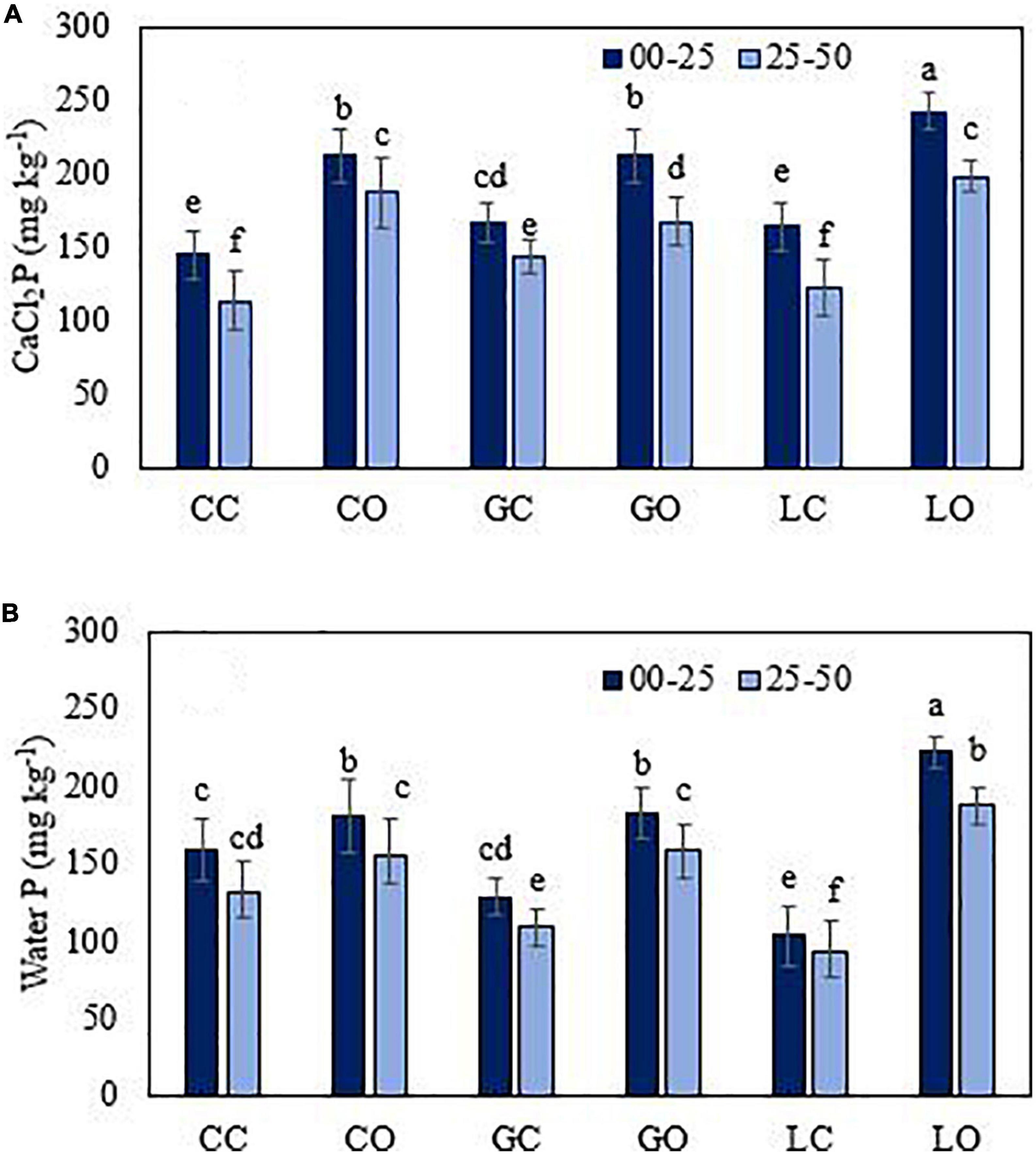
Figure 3. CaCl2 (A) and water (B) extractable P from soil under three fruit orchards. CC, citrus control; CO, citrus orchard; GC, guava control; GO, guava orchard; LC, loquat control; LO, loquat orchard. “±” Values are standard errors. Significant differences between the treatments at p ≤ 0.05 are indicated by different letters.
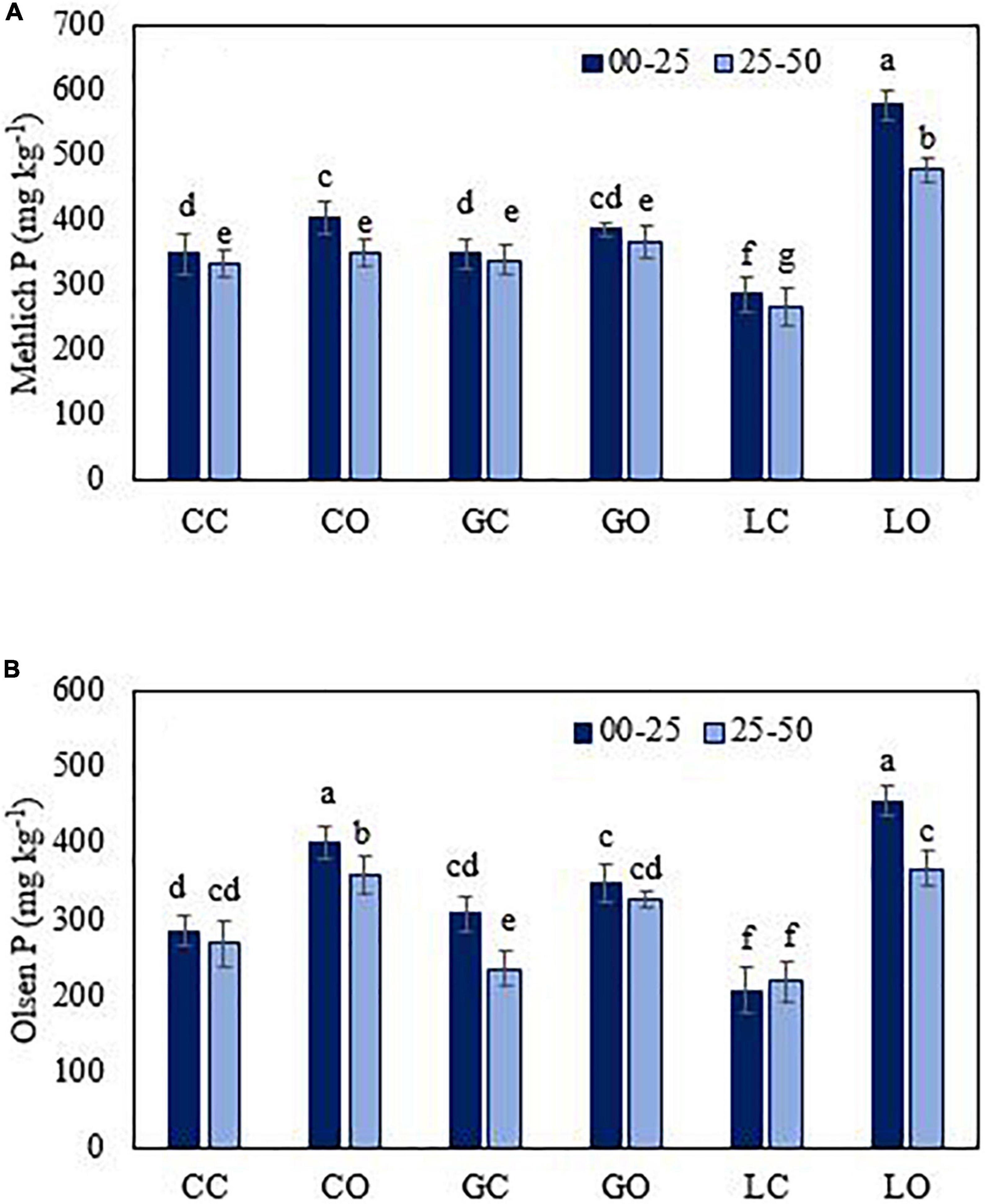
Figure 4. Mehlich 1 (A) and Olsen (B) extractable P from soil under three fruit orchards. CC, citrus control; CO, citrus orchard; GC, guava control; GO, guava orchard; LC, loquat control; LO, loquat orchard. “±” Values are standard errors. Significant differences between the treatments at p ≤ 0.05 are indicated by different letters.
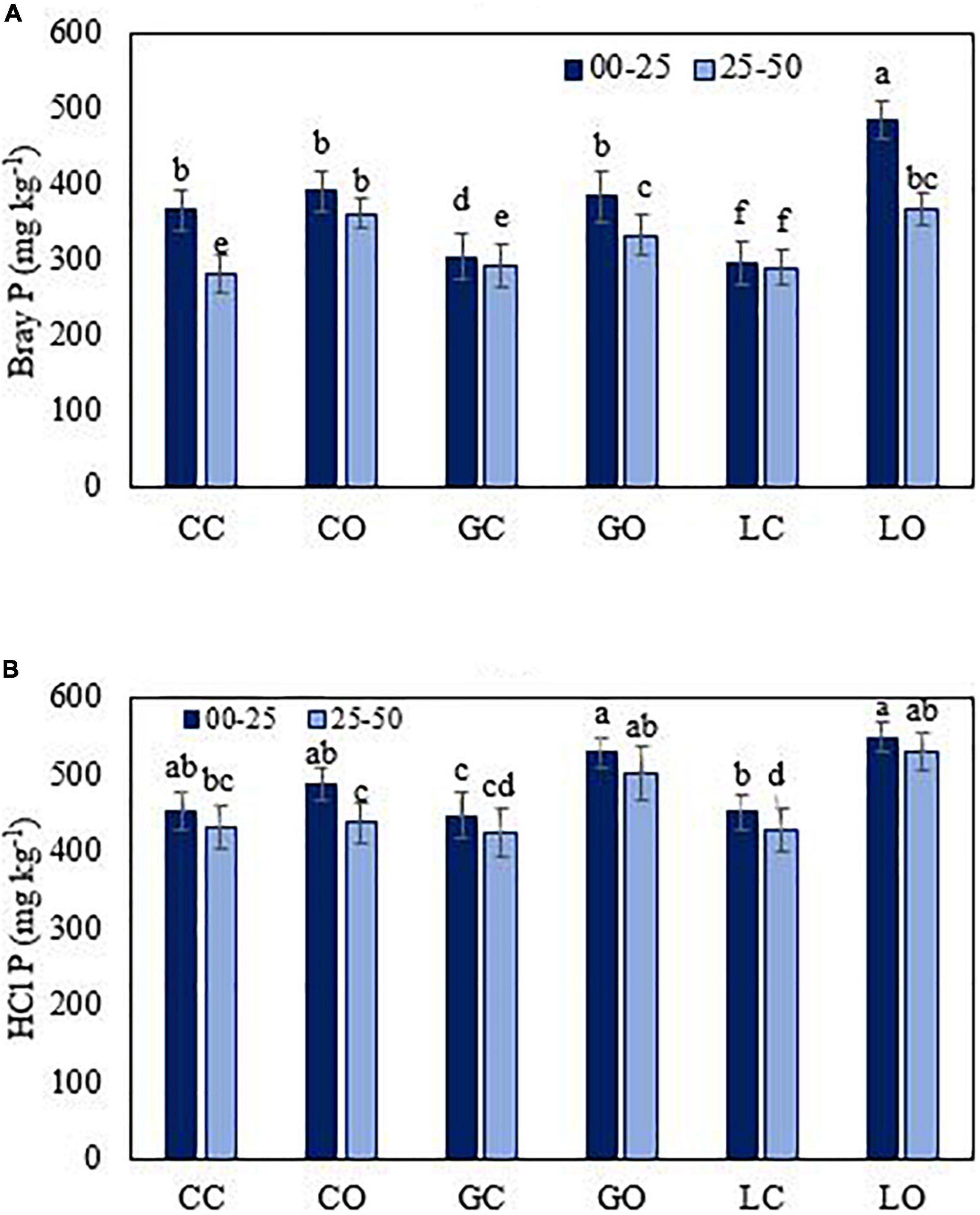
Figure 5. Bray 1 (A) and HCl (B) extractable P from soil under three fruit orchards. CC, citrus control; CO, citrus orchard; GC, guava control; GO, guava orchard; LC, loquat control; LO, loquat orchard. “±” Values are standard errors. Significant differences between the treatments at p ≤ 0.05 are indicated by different letters.
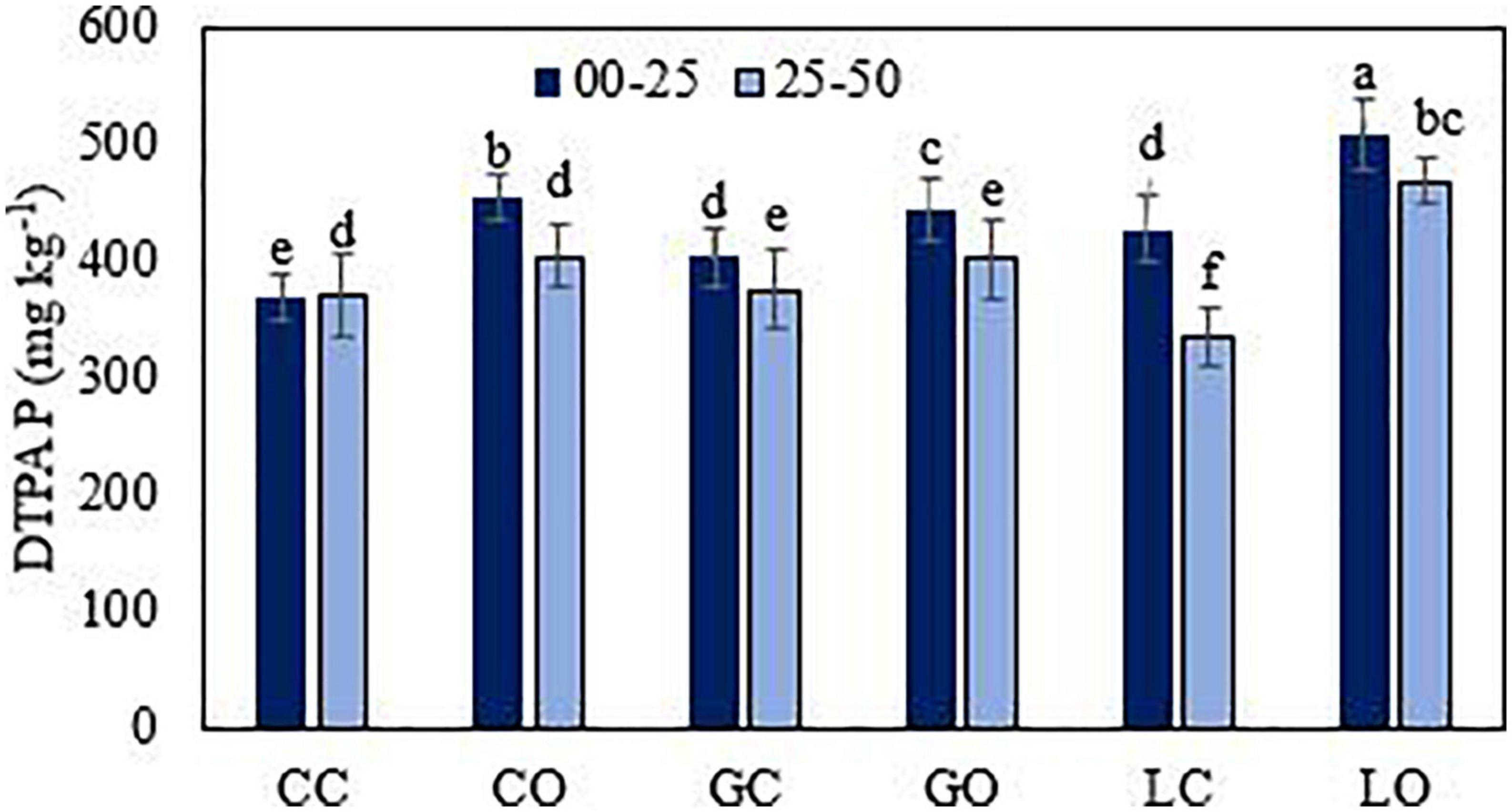
Figure 6. Diethylene triamine penta acetic acid extractable P from soil under three fruit orchards. CC, citrus control; CO, citrus orchard; GC, guava control; GO, guava orchard; LC, loquat control; LO, loquat orchard. “±” Values are standard errors. Significant differences between the treatments at p ≤ 0.05 are indicated by different letters.
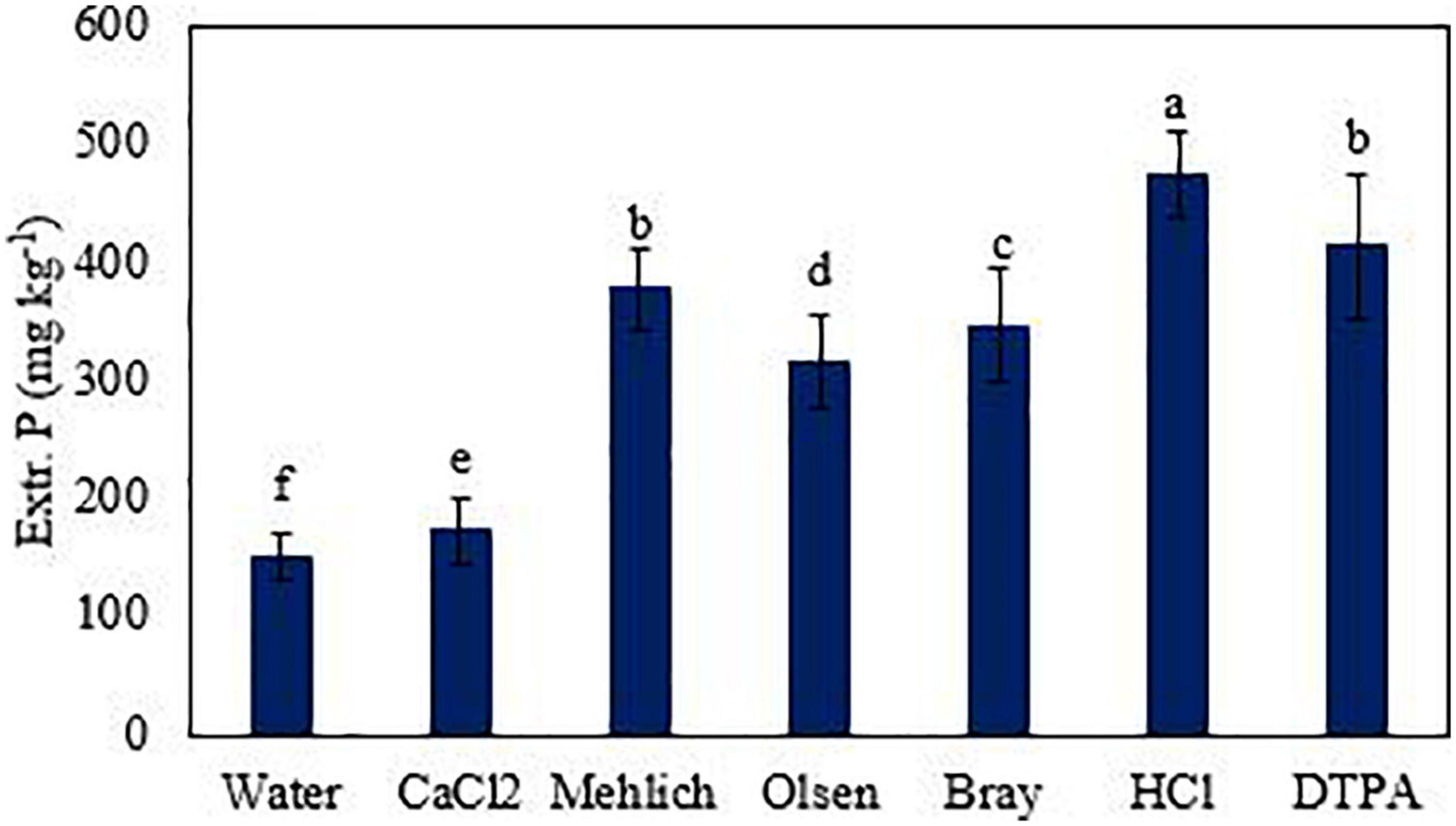
Figure 7. Extractable P across all soil samples (0–25 cm) using different extraction methods. Significant differences between the treatments at p ≤ 0.05 are indicated by small letters.
4 Discussion
In total, seven extraction methods were compared for the extractability of P from orchard soils. Phosphorus amounts extracted through different extractants were varied in the order of HCl > DTPA > Mehlich 1 > Bray 1 > Olsen > CaCl2 > water. Due to the various extraction procedures utilized for available P in the soil, these methods extracted varied P concentrations depending on the soil properties. The efficiency for the extractability of P among all extraction methods significantly differed in soils. This suggests that the extracting efficiency of the contrasting methods is strongly affected by the presence of available P in orchard-covered soils. The differences among the P extraction methods have been related to the extracting agents that may extract P fractions depending on the soil components involved in the P sorption process (Haque et al., 2013). The extractable P in soils was reported as Olsen > Mehlich-3 > Bray-1 by Haque et al. (2013). Diversified methods (i.e., acid oxalate, Bray, and Mehlich 3 methods) have been utilized to analyze available soil P, due to the different soil conditions and their properties as well as different cropping patterns (Khaledian et al., 2018). The extraction methods based on water solubility and ion exchange (using neutral salt solutions) have been considered weaker for the P extraction from soils at pH 6, where P retention in soils was relatively low, whereas calcium acetate lactate, Mehlich 3, Bray II, dithionite, oxalate, and total P methods were reported as the strong P extraction methods for calcareous soils (Zehetner et al., 2018).
In this study, higher levels of inorganic P (Pi) were released from the soil samples of the loquat and citrus orchards. These differences in the P extraction from soils are largely attributed to the biomass pattern of trees, soil fertility, and controlling factors of P transformation in the soil. The occurrence of extractable P in soils may depend on the quantity and quality of leaf litter fall. This could predict that the quantity and quality of litter fall from orchards have affected the decomposition rate of litter and play a significant role in the P dynamics in soils. Wuenscher et al. (2015) also reported the differential extractability of P with different extraction methods and soil properties. Each extracting agent has a different ability to extract the amount of soil P because the reagent is targeted for different pools of soil P (Zhang et al., 2004). Phosphorus in soils may transform and be partially available, while the rest amount of P may either adsorb or precipitate in soils. The presence of total amount of P may not guarantee its availability in the soil. Ahmad et al. (1999) reported that in soils, P may be fixed on the surface of Ca/Mg carbonates as well as in the Ca/Mg phosphate compounds and converted the presence of P contents into insoluble or less soluble and thus retard the P bioavailability. Zhao et al. (2007) reported that the mineralization of organic P and the decomposition of litter were the main sources of available P and associated biological processes.
The available P could be predicted using a number of relatively stable soil properties such as soil texture and soil properties that could change over time periods, i.e., pH, extractable Ca, and total organic carbon (Khaledian et al., 2018). This makes a rapid assessment of P availability. The extractability of P was well correlated with clay percentage and organic matter contents in the soils. Soil texture affects the chemical characteristics of soil, including the formation of Al-organic matter bonded stable P and the leaching of P from soils (Sugihara et al., 2012), which may be related to the available P. Clay content was significantly related to the amount of P in the tested models because P can adsorb on the surfaces of clay minerals (Shen et al., 2011). Soil texture is important in determining P leaching from soils and influence the hydrology of soil (Negassa and Leinweber, 2009). The values of extractable P were related to the total amount of soil P. When the different extraction methods were correlated with each other, this showed that most extraction methods were correlated positively. However, the extent of correlation differs substantially among the P extraction methods used. Zheng and Zhang (2012) reported that texture and distribution of particle size heavily influenced soil P extraction in soils. This study revealed that the significant increase in soil P occurred under the influence of fruit tree species as compared to control soil (i.e., without an orchard). The differences in Pi concentrations in soil under long-term tree plantation may regulate P dynamics in soils. Chen et al. (2008) reported enhanced mineralization of organic P and available P as a consequence of afforestation. Abdu (2006) also reported that the prevailing soil properties, i.e., pH and CaCO3 content, soil texture, mineralogical composition, total surface area, organic matter content, and the presence of Fe and Al(hydr-) oxides substantially affected the solubility, availability, and extractability of P in the soil environment.
Soil organic matter has been considered an important component of soil quality. The amount of C in the soil was markedly affected by the presence of tree species. The C stock in soils differed by orchard species as loquat > citrus > guava. A higher amount of SOC was observed in the soil of a natural forest due to the higher density of the vegetation cover, deeper root distribution, greater size of the trees, larger biomass production, and litter inputs in the soil (Bakhshandeh et al., 2019). The extractable amount of P in the soil under the influence of different tree species followed almost a similar trend such as SOM. This indicates that total C contents in the soil could be linked to the P extractability in the soil. Organic matter contents in soil were also correlated with extractable P via seven extraction methods. Soil OC provides binding sites and influences P availability in soil (Kang et al., 2009), and changes in the organic P can regulate the P bioavailability from soils (Shen et al., 2011). Total P was well related to the amount of extractable P and clay contents, respectively. Yang et al. (2013) reported organic P as a major part of the soil P pool. A weak and inconsistent relationship of extractable P was found with the soil pH during the study.
The changes in the organic P indicated that organic matter inputs associated with tree growth influenced the P dynamics in soil (Chirino-Valle et al., 2016). Mehvish et al. (2011) reported the significance of macronutrients and micronutrients for the improvement of tree health, fruit yield, and quality. Various extraction methods have been reported for evaluating the availability of nutrients from soils (Ure and Davidson, 2002; Naidu et al., 2003). Menon et al. (1989) reported a positive relationship between P extracted with HCl and soil texture. Fernandes et al. (1999) reported that P extracted by Olsen, Fe-oxide Pi, and cation and anion exchange membranes was less dependent on the soil texture. Pueyo et al. (2004) recommended the 0.01 M CaCl2 extraction method due to its feasibility for elemental analysis. This study inferred that fallen plant litter brought tangible changes in the P composition depending on the nature of the soil and the magnitude of SOM.
Plant nutrients were found in higher concentrations in orchards and the concentrations of K, Ca, and Mg in soil samples varied with the kind of plantation. Total K, Ca, and Mg were higher in soil afforested with loquat and citrus trees and lower in the soil sampled from guava. Sufficient amounts of these nutrients in orchard soils could be attributed to the higher values of SOM (>1%) when compared to the control agricultural soil. This research indicated that the soil quality could be attributed to the type of orchard, growth pattern, amount of litter falls, and sequestration of SOM in the soil. Plant biomass production and nutrient cycling due to vegetation influenced the soil properties and plant nutrient availability (Chen et al., 2003). Different tree species have varying effects on nutrient cycling due to the differences in tree size, litterfall, and litter chemical composition (Mlambo et al., 2005). Zeraatpisheh et al. (2020) reported the spatial variations in soil properties and terrain attributes as a possible approach to delineating soil management zone for citrus plantations. Moreover, the higher correlation between soil properties and terrain attributes revealed considerable spatial variability, and a site-specific nutrient management system has been suggested (Zeraatpisheh et al., 2020).
The EC of orchard soils was found higher, which decreased significantly in control soils. An increasing trend in the soil EC was reported after the addition of organic materials of varying nature (Sarwar et al., 2003). Soils planted with orchard tree species were slightly more alkaline than those of adjacent control fields due to the higher concentrations of base cations. The increase in the base elements is likely to alter reactions on exchange sites, soil process rates, and the composition of the soil biotic community (Reich et al., 2005).
5 Conclusion
Land use has a significant influence on the available phosphorus (P). Loquat and citrus trees improved the soil quality more than guava species. SOM, CEC, and essential nutrients have been enhanced under the orchard system. Tree Plant species have varied effects on the P extractability from the soil. Hydrochloric acid (HCl) and diethylenetriamine pentaacetate (DTPA) extractants gave more amount of extractable P than other methods. Extractable P was markedly associated with the total amount of soil P. Topsoil showed more P concentrations than subsoil in all fruit orchards. Orchards have sequestered more amount of C stock than soil without an orchard. A higher amount of C stock in orchard soil as compared to control could be associated with higher inputs of leaf litter. The extractability P was well correlated with soil properties such as clay and organic matter contents in the soil. Therefore, we suggest the application of organic matter in soils for better orchard management. This study could help to address the issues related to P fertility and environmental management. More studies are required on the P extractability in relation to the bioavailability using different extraction methods in contrasting soils for sustainable P management.
Data availability statement
The original contributions presented in this study are included in the article/supplementary material, further inquiries can be directed to the corresponding author.
Author contributions
MI, FU, QM, MS, and PA: experiment design. SB, MI, ZH, AA, and AH: soil sampling and experiment administration. MM, AN, MI, PA, FU, and QM: data analysis. SB, MT, MI, ZH, and MM: writing — original draft preparation, data verification, and proofreading. AN, NG, QM, and MS: proofreading. All authors have read and agreed to the published version of the manuscript.
Acknowledgments
The authors acknowledge Charles Darwin University, Australia for its support.
Conflict of interest
The authors declare that the research was conducted in the absence of any commercial or financial relationships that could be construed as a potential conflict of interest.
Publisher’s note
All claims expressed in this article are solely those of the authors and do not necessarily represent those of their affiliated organizations, or those of the publisher, the editors and the reviewers. Any product that may be evaluated in this article, or claim that may be made by its manufacturer, is not guaranteed or endorsed by the publisher.
References
Abdu, N. (2006). Soil-phosphorus extraction methodologies: A review. Afr. J. Agric. Res. 1, 159–161.
Aggelopoulou, K., Pateras, D., Fountas, S., Gemtos, T., and Nanos, G. (2011). Soil spatial variability and site-specific fertilization maps in an apple orchard. Precis. Agric. 12, 118–129. doi: 10.1007/s11119-010-9161-x
Ahmad, N., Razzaq, A., and Yaseen, M. (1999). Phosphate adsorption/desorption in seven soil series of Pakistan. Pak. J. Biol. Sci. 2, 897–899. doi: 10.3923/pjbs.1999.897.899
Bakhshandeh, E., Hossieni, M., Zeraatpisheh, M., and Francaviglia, R. (2019). Land use change effects on soil quality and biological fertility: A case study in northern Iran. Eur. J. Soil Biol. 95:103119. doi: 10.1016/j.ejsobi.2019.103119
Becquer, A., Trap, J., Irshad, U., Ali, M., and Claude, P. (2014). From soil to plant, the journey of P through trophic relationships and ectomycorrhizal association. Front. Plant Sci. 5:548. doi: 10.3389/fpls.2014.00548
Berbecea, A., Radulov, I., Sala, F., Crista, F., and Lato, A. (2011). Interrelation between metal availability, soil pH and mineral fertilization. Res. J. Agric. Sci. 43, 19–22.
Bibi, S., Irshad, M., Mohiuddin, M., Sher, S., Tariq, M., and Ng, A. (2022). Distribution of phosphorus fractions in orchard soils in relation to soil properties and foliar P contents. Sustainability 14:3966. doi: 10.3390/su14073966
Bray, R., and Kurtz, L. (1945). Determination of total, organic, and available forms of phosphorus in soils. Soil Sci. 59, 39–46. doi: 10.1097/00010694-194501000-00006
Brunetto, G., Melo, G., Toselli, M., Quartieri, M., and Tagliavini, M. (2015). The role of mineral nutrition on yields and fruit quality in grapevine, pear and apple. Rev. Brasileira Fruticult. 37, 1089–1104. doi: 10.1590/0100-2945-103/15
Cambardella, C., Gajda, A., Doran, J., Wienhold, B., Kettler, T., and Lal, R. (2001). “Estimation of particulate and total organic matter by weight loss-on-ignition,” in Assessment methods for soil carbon, eds J. Kimble, R. Follett, and B. Stewart (Boca Raton, FL: CRC press), 349–359.
Carter, M. (2002). Soil quality for sustainable land management: Organic matter and aggregation interactions that maintain soil functions. Agron. J. 94, 38–47. doi: 10.2134/agronj2002.3800
Cataldo, E., Fucile, M., and Mattii, G. (2021). A review: Soil management, sustainable strategies and approaches to improve the quality of modern viticulture. Agronomy 11:2359. doi: 10.3390/agronomy11112359
Chaudhari, P., Ahire, D., and Ahire, V. (2012). Correlation between Physico-chemical properties and available nutrients in sandy loam soils of Haridwar. J. Chem. Biol. Phys. Sci. 2:1493.
Chen, C., Condron, L., and Xu, Z. (2008). Impacts of grassland afforestation with coniferous trees on soil phosphorus dynamics and associated microbial processes: A review. For. Ecol. Manage. 255, 396–409. doi: 10.1016/j.foreco.2007.10.040
Chen, C., Condron, L., Davis, M., and Sherlock, R. (2003). Seasonal changes in soil phosphorus and associated microbial properties under adjacent grassland and forest in New Zealand. For. Ecol. Manage. 177, 539–557. doi: 10.1016/S0378-1127(02)00450-4
Chi, C., and Wang, Z. (2010). Characterizing salt-affected soils of Songnen plain using saturated paste and 1: 5 soil-to-water extraction methods. Arid Land. Res. Manage. 24, 1–11. doi: 10.1080/15324980903439362
Chirino-Valle, I., Davis, M., and Condron, L. (2016). Impact of different tree species on soil phosphorus immediately following grassland afforestation. J. Soil Sci. Plant Nutr. 16, 477–489. doi: 10.4067/S0718-95162016005000040
Condron, L., and Tiessen, H. (2005). “Interactions of organic phosphorus in terrestrial ecosystems,” in Organic phosphorus in the environment, eds B. Turner, E. Frossard, and D. Baldwin (Wallingford: CAB International), 295–307. doi: 10.1079/9780851998220.0295
Delgado, A., and Torrent, J. (2001). Comparison of soil extraction procedures for estimating phosphorus release potential of agricultural soils. Commun. Soil. Sci. Plant Anal. 32, 87–105. doi: 10.1081/CSS-100102995
Fernandes, M., Indiati, R., Countinho, J., and Buondonno, A. (1999). Soil properties affecting phosphorus extraction from Portuguese soils by conventional and innovative methods. Commun. Soil Sci. Plant Anal. 30, 921–936. doi: 10.1080/00103629909370257
Gul, H., Khattak, A., and Amin, N. (2006). Accelerating the growth of Araucaria heterophylla seedlings through different gibberellic acid concentrations and nitrogen levels. J. Agric. Biol. Sci. 1, 25–29.
Haque, E., Kashem, A., and Osman, K. (2013). Extractable phosphorus contents of some soils of Bangladesh and their correlation with phosphorus concentration in rice leaves. Open J. Soil Sci. 3, 115–122. doi: 10.4236/ojss.2013.32013
Hinsinger, P. (2013). “Plant-induced changes in soil processes and properties,” in Soil conditions and plant growth, eds P. Gregory and S. Nortcliff (Oxford: Blackwell Publishing Ltd). doi: 10.1002/9781118337295.ch10
Houba, V., Temminghoff, E., Gaikhorst, G., and Van Vark, W. (2000). Soil analysis procedures using 0.01 M calcium chloride as extraction reagent. Commun. Soil Sci. Plant Anal. 31, 1299–1396. doi: 10.1080/00103620009370514
Hoying, S., Fargione, M., and Iungerman, K. (2004). Diagnosing apple tree nutritional status: Leaf analysis interpretation and deficiency symptoms. N.Y. Fruit Q. 12, 16–19.
Huluka, G., and Miller, R. (2014). Particle size determination by hydrometer method. Southern Cooperative Ser. Bull. 419, 180–184.
Jarociński, B. (2005). Assessment of orchard soils with respect to essential mineral contents. Zesz Nauk Inst Sad Kwiac 13, 17–29.
Jiang, Y., Zhang, Y., Zhou, D., Qin, Y., and Liang, W. (2009). Profile distribution of micronutrients in an aquic brown soil as affected by land use. Plant Soil Environ. 55, 468–476. doi: 10.17221/57/2009-PSE
Kai, T., Mukai, M., Araki, K., Adhikari, D., and Kubo, M. (2016). Analysis of chemical and biological soil properties in organically and conventionally fertilized apple orchards. J. Agric. Chem. Environ. 5, 92–99. doi: 10.4236/jacen.2016.52010
Kang, J., Hesterberg, D., and Osmond, D. (2009). Soil organic matter effects on phosphorus sorption: A path analysis. Soil Sci. Soc. Am. J. 73, 360–366. doi: 10.2136/sssaj2008.0113
Khaledian, Y., Quinton, J., Brevik, E., Pereira, P., and Zeraatpisheh, M. (2018). Developing global pedotransfer functions to estimate available soil phosphorus. Sci. Total Environ. 644, 1110–1116. doi: 10.1016/j.scitotenv.2018.06.394
Kuo, S. (1996). “Phosphorus,” in Methods of soil analysis. Part 3. SSSA Book Ser. 5, ed. D. L. Sparks (Madison, WI: SSSA), 869–919. doi: 10.2136/sssabookser5.3.c32
Li, Z., Qiu, Q., Chen, Y., Lin, D., Huang, J., and Huang, T. (2021). Metabolite alteration in response to low phosphorus stress in developing tomato fruits. Plant Physiol. Biochem. 159, 234–243. doi: 10.1016/j.plaphy.2020.12.023
Mehlich, A. (1953). Determination of P, Ca, Mg, K, Na, and NH4. North Carolina soil test division (Mimeo 1953). Raleigh NC: North Carolina Department of Agriculture, 23–89.
Mehvish, F., Khan, S., Razzaq, A., Nawaz, I., and Haq, I. (2011). Fertility status of guava orchards in Kohat District of Pakistan. J. Soil Sci. Environ. Manage. 3, 260–268.
Memon, M. (2008). Role of Fe-oxides for predicting phosphorus sorption in calcareous soils. Karlsruhe: KIT Scientific Publishing.
Menon, R., Chien, S., Hammondm, L., and Henao, J. (1989). Modified techniques for preparing paper strips for the new Pi soil test for phosphorus. Fert. Res. 19, 85–91. doi: 10.1007/BF01054679
Messiga, A., Ziadi, N., Mollier, A., Parent, L., Schneider, A., and Morel, C. (2015). Process-based mass-balance modeling of soil phosphorus availability: Testing different scenarios in a long-term maize monoculture. Geoderma 243-244, 41–49. doi: 10.1016/j.geoderma.2014.12.009
Miller, R., Gavlak, R., and Horneck, D. (2013). Soil, plant and water reference methods for the western region. WREP-125, 4th Edn. Fort Collins, CO: Colorado State University.
Mlambo, D., Nyathi, P., and Mapaure, I. (2005). Influence of Colophospermum mopane on surface soil properties and under-storey vegetation in a southern African savanna. For. Ecol. Manage. 212, 394–404. doi: 10.1016/j.foreco.2005.03.022
Murphy, J., and Riley, J. (1962). A modified single solution method for determination of phosphate in natural waters. Anal. Chim. Acta 27, 31–36. doi: 10.1016/S0003-2670(00)88444-5
Naidu, R., Gupta, V., Rogers, S., Kookana, R., Bolan, N., and Adriano, D. (2003). “Bioavailability of metals in the soil plant environment and its potential role in risk assessment,” in Bioavailability toxicity and risk relationships in ecosystems, eds R. Naidu, S. Rogers, V. V. S. R. Gupta, R. S. Kookana, N. S. Bolan, and D. Adriano (Enfield, NH: Science Publishers).
Nazarkiewicz, M., and Kaniuczak, J. (2012). The influence of liming and mineral fertilization on the content of available forms of phosphorus, potassium and magnesium in Haplic Luvisols. Soil Sci. Annu. 63, 49–54. doi: 10.2478/v10239-012-0011-6
Negassa, W., and Leinweber, P. (2009). How does the Hedley sequential phosphorus fractionation reflect impacts of land use and management on soil phosphorus: A review. J. Plant Nutr. Soil Sci. 172, 305–325. doi: 10.1002/jpln.200800223
Nwoke, O., Vanlauwe, B., Diels, J., Sanginga, N., Osonubi, O., and Merckx, R. (2003). Assessment of labile phosphorus fractions and adsorption characteristics in relation to soil properties of West African savanna soils. Agric. Ecosys. Environ. 100, 285–294. doi: 10.1016/S0167-8809(03)00186-5
Olsen, S., Cole, C., Watanabe, F., and Dean, L. (1954). Estimation of available phosphorus in soils by extraction with sodium bicarbonate. USDA Circ. 939. Washington, DC: US Government Printing Office.
Otabbong, E., Börling, K., Kätterer, T., and Mattsson, L. (2009). Compatibility of the ammonium lactate (AL) and sodium bicarbonate (Olsen) methods for determining available phosphorus in Swedish soils. Acta Agric. Scand. Sec. B Soil Plant Sci. 59, 373–378. doi: 10.1080/09064710902777091
Pagliari, P., and Laboski, C. (2012). Investigation of the inorganic and organic phosphorus forms in animal manure. J. Environ. Qual. 41, 901–910. doi: 10.2134/jeq2011.0451
Pueyo, M., Lopez-Sanchez, J., and Rauret, G. (2004). Assessment of CaCl2, NaNO3, NH4NO3 extraction procedure for the study of Cd, Cu, Pb and Zn extractability in contaminated soils. Analyt. Chim. Acta 504, 217–226. doi: 10.1016/j.aca.2003.10.047
Reich, P., Oleksyn, J., Modrzynski, J., Mrozinski, P., Hobbie, S., Eissenstat, D., et al. (2005). Linking litter calcium, earthworms and soil properties: A common garden test with 14 tree species. Ecol. Lett. 8, 811–818. doi: 10.1111/j.1461-0248.2005.00779.x
Ruttenberg, K. (2003). The global phosphorus cycle. TrGeo 8:682. doi: 10.1016/B0-08-043751-6/08153-6
Sarwar, G., Hussain, N., Mujeeb, F., Schmeisky, H., and Hassan, G. (2003). Biocompost application for the improvement of soil characteristics and dry matter yield of Lolium perenne (Grass). Asian J. Plant Sci. 2, 237–241. doi: 10.3923/ajps.2003.237.241
Sayers, J., Johnston, A., and Curtin, D. (2008). Efficiency of soil and fertilizer phosphorus use: Reconciling changing concepts of soil phosphorus behaviour with agronomic information. Rome: FAO Fertilizer and Plant Nutrition Bulletin, Food and Agriculture Organization of the United Nations.
Shen, J., Yuan, L., Zhang, J., Li, H., Bai, Z., Chen, X., et al. (2011). Phosphorus dynamics: From soil to plant. Plant Physiol. 156, 997–1005. doi: 10.1104/pp.111.175232
Simpson, R., Oberson, A., Culvenor, R., Ryan, M., Veneklaas, E., Lambers, H., et al. (2011). Strategies and agronomic interventions to improve the phosphorus-use efficiency of farming systems. Plant Soil 349, 89–120. doi: 10.1007/s11104-011-0880-1
Sims, J. (2000). “Soil test phosphorus: Bray and Kurtz P-1,” in Methods of phosphorus analysis for soils, sediments, residuals, and waters, ed. G. Pierzynski (Raleigh: North Carolina State University), 13–14.
Smith, D., Francesconi, W., Livingston, S., and Huang, C. (2015). Phosphorus losses from monitored fields with conservation practices in the Lake Erie Basin, USA. Ambio 44, 319–331. doi: 10.1007/s13280-014-0624-6
Sugihara, S., Funakawa, S., Nishigaki, M., Kilasara, M., and Kosaki, T. (2012). Dynamics of fractionated P and P budget in soil under different land management in two Tanzanian croplands with contrasting soil textures. Agric. Ecosyst. Environ. 162, 101–107. doi: 10.1016/j.agee.2012.07.019
Sumera, B., Irshad, M., Mohiuddin, M., Sher, S., Tariq, M., and Anne, W. (2022). Distribution of phosphorus fractions in orchard soils in relation to soil properties and foliar P contents. Sustainability 14, 3966.
Tan, K. (1995). “Determination of macro-elements,” in Soil sampling, preparation, and analysis, ed. K. H. Tan (New York, NY: Marcel Dekker, Inc), 135–188.
Tejada, M., Hernandez, M., and Garcia, C. (2006). Application of two organic amendments on soil restoration: Effects on the soil biological properties. J. Environ. Qual. 35, 1010–1017. doi: 10.2134/jeq2005.0460
Throop, H., Archer, S., Monger, H., and Waltman, S. (2012). When bulk density methods matter: Implications for estimating soil organic carbon pools in rocky soils. J. Arid Environ. 77, 66–71. doi: 10.1016/j.jaridenv.2011.08.020
Tóth, G., Guicharnaud, R., Tóth, B., and Hermann, T. (2014). Phosphorus levels in croplands of the European Union with implications for P fertilizer use. Eur. J. Agron. 55, 42–52. doi: 10.1016/j.eja.2013.12.008
Upadhyay, S., and Raghubanshi, A. (2020). “Chapter 16 - Determinants of soil carbon dynamics in urban ecosystems,” in Urban ecology, emerging patterns and social-ecological systems, eds P. Verma, R. Singh, P. Singh, and A. Raghubanshi (Amsterdam: Elsevier), 299–314. doi: 10.1016/B978-0-12-820730-7.00016-1
Urade, P., Jadhav, A., and Dheware, R. (2019). Studies on physico-chemical properties of tamarind orchard soils of Latur district. J. Pharm. Phytochem. 8, 2738–2740. doi: 10.20546/ijcmas.2019.802.076
Ure, A., and Davidson, C. (2002). “Chemical speciation in soils and related materials by selective chemical extraction,” in Chemical speciation in the environment, 2nd Edn, eds A. M. Ure and D. M. Davidson (London: Blackwell Science Ltd), 265–300. doi: 10.1002/9780470988312.ch10
White, P. (2012). “Ion uptake mechanisms of individual cells and roots: Short-distance transport,” in Marschner’s mineral nutrition of higher plants, ed. P. Marschner (London: Academic Press), 7–47. doi: 10.1016/B978-0-12-384905-2.00002-9
Wuenscher, R., Unterfrauner, H., Peticzka, R., and Zehetner, F. (2015). A comparison of 14 soil phosphorus extraction methods applied to 50 agricultural soils from Central Europe. Plant Soil Environ. 61, 86–96. doi: 10.17221/932/2014-PSE
Yang, X., Post, W., Thorton, P., and Jain, A. (2013). The distribution of soil phosphorous for global biogeochemical modeling. Biogeosciences 10, 2525–2537. doi: 10.5194/bg-10-2525-2013
Zehetner, F., and Wenzel, W. (2000). Nickel and copper sorption in acid forest soils. Soil Sci. 165, 463–472. doi: 10.1097/00010694-200006000-00002
Zehetner, F., Wuenscher, R., Peticzka, R., and Unterfrauner, H. (2018). Correlation of extractable soil phosphorus (P) with plant P uptake: 14 extraction methods applied to 50 agricultural soils from Central Europe. Plant Soil Environ. 64, 192–201. doi: 10.17221/70/2018-PSE
Zeraatpisheh, M., Bakhshandeh, E., Emadi, M., Li, T., and Xu, M. (2020). Integration of PCA and fuzzy clustering for delineation of soil management zones and cost-efficiency analysis in a citrus plantation. Sustainability 12:5809. doi: 10.3390/su12145809
Zhang, M., Wright, R., Heaney, D., and Vanderwel, D. (2004). Comparison of different phosphorus extraction and determination methods using manured soils. Canad. J. Soil Sci. 84, 469–475. doi: 10.4141/S02-023
Zhang, S., Chen, Y., Xie, Z., Zhang, H., Lin, L., and Wang, D. (2019). Unraveling the molecular mechanism of the response to changing ambient phosphorus in the dinoflagellate Alexandrium catenella with quantitative proteomics. J. Proteom. 196, 141–149. doi: 10.1016/j.jprot.2018.11.004
Zhao, Q., Zeng, D., Lee, D., He, X., He, Z., Fan, Z., et al. (2007). Effects of Pinus sylvestris var. mongolica afforestation on soil phosphorus status of the Keerqin Sandy Lands in China. J. Arid Environ. 69, 569–582. doi: 10.1016/j.jaridenv.2006.11.004
Zheng, Z., and Zhang, T. (2012). “Soil phosphorus tests and transformation analysis to quantify plant availability: A review,” in Soil fertility improvement and integrated nutrient management – a global perspective, ed. J. Whalen (Rijeka: InTech), 19–36. doi: 10.5772/29904
Zhou, J., Bing, H., Wu, Y., Sun, H., and Wang, J. (2018). Weathering of primary mineral phosphate in the early stages of ecosystem development in the Hailuogou Glacier foreland chronosequence. Eur. J. Soil Sci. 69, 450–461. doi: 10.1111/ejss.12536
Zia, M., Ahmad, R., Khaliq, I., Ahmad, A., and Irshad, M. (2006). Micronutrients status and management in orchards soils: Applied aspects. Soil Environ. 25, 6–16.
Keywords: soil quality (SQ), orchard species, nutrient, arid region, phosphorus
Citation: Bibi S, Irshad M, Ullah F, Mahmood Q, Shahzad M, Tariq MAUR, Hussain Z, Mohiuddin M, An P, Ng AWM, Abbasi A, Hina A and Gonzalez NCT (2023) Phosphorus extractability in relation to soil properties in different fields of fruit orchards under similar ecological conditions of Pakistan. Front. Ecol. Evol. 10:1077270. doi: 10.3389/fevo.2022.1077270
Received: 22 October 2022; Accepted: 29 November 2022;
Published: 04 January 2023.
Edited by:
Awais Shakoor, Universitat de Lleida, SpainReviewed by:
Saeed Rehman, South China University of Technology, ChinaVarucha Misra, Indian Institute of Sugarcane Research (ICAR), India
Yasir Majeed, Gansu Agricultural University, China
Copyright © 2023 Bibi, Irshad, Ullah, Mahmood, Shahzad, Tariq, Hussain, Mohiuddin, An, Ng, Abbasi, Hina and Gonzalez. This is an open-access article distributed under the terms of the Creative Commons Attribution License (CC BY). The use, distribution or reproduction in other forums is permitted, provided the original author(s) and the copyright owner(s) are credited and that the original publication in this journal is cited, in accordance with accepted academic practice. No use, distribution or reproduction is permitted which does not comply with these terms.
*Correspondence: A. W. M. Ng, ✉ anne.ng@cdu.edu.au