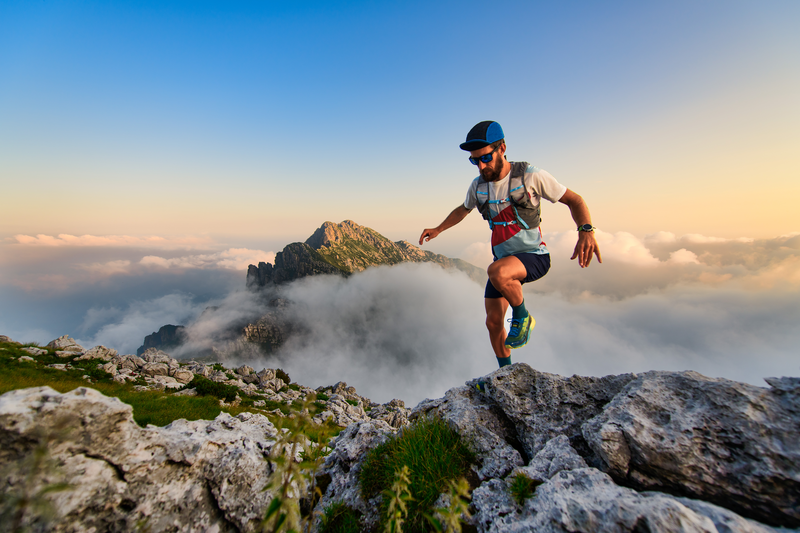
94% of researchers rate our articles as excellent or good
Learn more about the work of our research integrity team to safeguard the quality of each article we publish.
Find out more
ORIGINAL RESEARCH article
Front. Ecol. Evol. , 01 February 2023
Sec. Chemical Ecology
Volume 10 - 2022 | https://doi.org/10.3389/fevo.2022.1070859
This article is part of the Research Topic Women in Chemical Ecology 2022 View all 8 articles
Plants perceive and respond to herbivore insect eggs. Upon egg deposition on leaves, a strong hypersensitive response (HR)-like cell death can be activated leading to egg desiccation and/or dropping. In Brassica spp., including many crops, the HR-like mechanism against eggs of cabbage white butterflies (Pieris spp.) is poorly understood. Using two Brassica species, the crop B. rapa and its wild relative B. nigra, we studied the cellular and molecular plant response to Pieris brassicae eggs and characterized potential insect egg-associated molecular patterns (EAMPs) inducing HR-like cell death. We found that eggs of P. brassicae induced typical hallmarks of early immune responses, such as callose deposition, production of reactive oxygen species and cell death in B. nigra and B. rapa leaf tissue, also in plants that did not express HR-like cell death. However, elevated levels of ethylene production and upregulation of salicylic acid-responsive genes were only detected in a B. nigra accession expressing HR-like cell death. Eggs and egg wash from P. brassicae contains compounds that induced such responses, but the eggs of the generalist moth Mamestra brassicae did not. Furthermore, wash made from hatched Pieris eggs, egg glue, and accessory reproductive glands (ARG) that produce this glue, induced HR-like cell death, whereas washes from unfertilized eggs dissected from the ovaries or removal of the glue from eggs resulted in no or a reduced response. This suggests that there is one or multiple egg associated molecular pattern (EAMP) located in the egg glue a that teresponse in B. nigra is specific to Pieris species. Lastly, our results indicate that the EAMP is neither lipidic nor proteinaceous. Our study expands the knowledge on the mechanism of Brassica-Pieris-egg interaction and is a step closer toward identification of EAMPs in Pieris egg glue and corresponding receptor(s) in Brassica.
Plants rely on an immune system that regulates the perception of attackers and subsequent activation of inducible defenses (Wilkinson et al., 2019). Perception involves detection of pathogen-derived effector proteins and of molecular patterns, which can derive from different organisms, such as microbes (MAMPs) or herbivores (HAMPs; Gust et al., 2017; Stahl et al., 2018; Van der Burgh and Joosten, 2019). Perception is followed by an early signaling cascade including rapid ion-flux changes and production of reactive oxygen species (ROS; Couto and Zipfel, 2016). Induced defenses typically include reinforcement of extracellular barriers, for example by callose deposition, the production of antimicrobial or insecticidal metabolites and proteins, and a localized, rapid cell death response, the hypersensitive response (Cui et al., 2015; Couto and Zipfel, 2016; Campos et al., 2018; Balint-Kurti, 2019; Dalio et al., 2020). Most in-depth studies on activation of the plant immune system have been performed on interactions with plant pathogens. So far, 119 HAMPs and/or effectors mostly from oral secretions of piercing sucking and chewing herbivores, have been identified, but pattern recognition receptors for HAMPs are just starting to be discovered (Erb and Reymond, 2019; Steinbrenner et al., 2020).
The detection by plants of herbivore eggs deposited on plant tissue is remarkable, as eggs are immobile and seemingly harmless structures. However, insect eggs will turn into feeding larvae and thus pose a future threat to the plant. Upon detection, plants can mount defenses against eggs that range from plant-mediated desiccation of eggs, egg dropping, egg crushing and the production of ovicidal substances (Hilker and Fatouros, 2015, 2016). Eggs of cabbage white butterflies (Pieris spp.) trigger necrotic lesions in leaves of the black mustard, Brassica nigra that can result in egg-killing by desiccating and/or dropping off singly laid Pieris eggs (Shapiro and DeVay, 1987; Griese et al., 2017). As the egg-induced plant phenotype resembles an HR, it has been referred to as hypersensitive response-like (“HR-like”; Fatouros et al., 2012). Oviposition by Pieris butterflies has been shown to induce HR-like cell death in several other plants of the Brassicaceae family, although the severity of the response varies between, and within species (Pashalidou et al., 2015; Griese et al., 2020, 2021; Groux et al., 2021; Bassetti et al., 2022).
Besides HR-like cell death, eggs of different insect species induce immune responses similar to pattern-triggered immunity (PTI), including callose deposition, accumulation of ROS and SA, and transcriptome changes of several defense genes, including PATHOGENESIS-RELATED PROTEIN 1 (PR1) in A. thaliana (Little et al., 2007; Bruessow et al., 2010; Gouhier-Darimont et al., 2013; Reymond, 2013; Lortzing et al., 2020). These early signaling defense responses to eggs seem to be conserved across different plant species (Lortzing et al., 2020). Expression of PR1 was also induced in leaves of B. nigra underneath P. brassicae and P. rapae eggs (Fatouros et al., 2014, 2015). Whether brassicaceous species that are natural hosts of Pieris spp., including B. nigra and B. rapa, respond with a general immune response, including ROS, callose and expression of different defense genes, to insect eggs, and whether there is genetic variation for this response, is largely unknown.
So far, few studies have identified EAMPs that activate defense against insect eggs (Reymond, 2013; Hilker and Fatouros, 2015; Stahl et al., 2018). In some studies, secretions surrounding eggs were sufficient to elicit defense responses in plants and a few elicitors have been isolated from these secretions (Hilker et al., 2005; Tamiru et al., 2011; Salerno et al., 2013). Most EAMPs were found to be organic compounds of low-molecular weight associated with the eggs, while recently a first proteinaceous compound, diprionin, was found as an elicitor of pine defense against sawfly eggs (Hundacker et al., 2021). In P. brassicae, egg-enveloping secretions are produced by the females’ accessory reproductive gland (ARG) and form a glue-like structure between the eggs and leaves (Beament and Lal, 1957; Fatouros et al., 2012). Treatment of Brassica plants with extracts from ARGs has shown to induce HR-like cell death and plant chemical cues attracting egg parasitoids, and to prime plants for future larval attack (Fatouros et al., 2008, 2009, 2015; Paniagua Voirol et al., 2020). Anti-aphrodisiacs transferred from the butterfly male to the female during mating were shown to be present in minute amounts in the ARG secretion and were suggested as potential elicitors (Fatouros et al., 2008, 2009). However, glands from unmated females induced HR-like, and therefore another, female-derived elicitor is likely to play a role (Fatouros et al., 2015). In A. thaliana, egg-derived phosphatidylcholines were found to induce production of H2O2, SA and result in cell death as shown by trypan blue staining (Stahl et al., 2020). It is still unclear which compounds from eggs of Pieris spp. and Anthocharis cardamines are detected by Brassica spp. and close relatives that result in HR-like activation, and whether these reside in the eggs themselves or in the secretions surrounding the eggs (Griese et al., 2021).
In this study, we developed and implemented a method to characterize the compounds from Pieris spp. eggs and egg-enveloping secretions. We specifically addressed: (1) the cellular and molecular response of two Brassica species, (2) the specificity of these responses by comparing eggs and egg washes of Pieris with the generalist moth M. brassicae, (3) the origin of EAMP inducing HR-like cell death, and (4) the chemical nature and stability of the elicitor.
Black mustard (B. nigra L.) accessions WUR-01 and WUR-02 used, originated from plants that were collected near the river Rhine in Wageningen, Netherlands (N51.96, E05.68). Brassica rapa L. genotypes used in this study (L58, R-o-18, RC-144) were obtained from the Laboratory of Plant Breeding (WUR). Plants were grown in a greenhouse (18 ± 5°C, 50–70% RH, L16, D8) and were used when three to five weeks old.
Pieris brassicae L. (Lepidoptera: Pieridae) was reared on Brussels sprouts plants (Brassica oleracea var. gemmifera cv. Cyrus) in a climate room (21 ± 1°C, 50%–70% RH, L16: D8) at the Laboratory of Entomology, Wageningen University. Virgin adult females were obtained by isolating female butterflies immediately after eclosion. Otherwise, twenty females and males could mate in a large cage (60 × 60 × 90 cm) and females were used for oviposition in experiments or oviposition on filter paper (grade 3 hw, Sartorius, Germany) for egg wash production (see section below). The cabbage moth Mamestra brassicae L. (Lepidoptera: Noctuidae) was reared on Brussels sprouts plants in a climate room (21 ± 1°C, 50%–70% RH, L16: D8) at the Laboratory of Entomology, Wageningen University.
The protocol for preparation of egg washes has been newly developed to prepare egg wash free from leaf-surface related chemical compounds which enables us to conduct chemical analysis and identify possible EAMPs. Full procedure is described in Supplementary Data (Supplementary Figure S1). Pieris brassicae eggs were collected on filter paper pinned underneath a B. oleracea leaf in a cage containing 20 mated females. Egg clutches laid on the paper were cut out and submersed in 1 mL 2-(N-morpholino)ethanesulfonic acid (Mes) buffer per 400 eggs, overnight (16 h) without disturbance. Previously, we have tested different buffers and found that using Mes buffer resulted in the most consistent plant response (data not shown). The solution (egg wash) was pipetted into a new tube the next morning.
To obtain egg-enveloping secretions for wash of only egg glue, 1-day old P. brassicae eggs were collected as above, counted, and then carefully removed from the paper using a brush. The spots of secretions underneath eggs were then cut out and washed overnight (16 h) in 1 mL 20 mM Mes buffer (pH 5.7) per 400 eggs. A wash of the pieces of the same filter paper without secretions was used as control.
To remove egg-enveloping secretions from eggs, eggs on paper were submersed in 1 mL per 400 eggs of either a solution of 1% bleach and 2% Tween-20 or in 250 mM NaPO4 pH 9.0 for 30 min (two different treatments that were shown to remove egg glue; Jacobs et al., 2013). After treatment, eggs were rinsed once with 1 mL MILLI-Q® water (Sigma-Aldrich, MO, United States), hereafter “MQ water,” and then 1 mL Mes buffer and then washed overnight (16 h) in 20 mM Mes buffer pH 5.7. Eggs that were used to compare where egg-enveloping secretions were not removed, were submersed in Mes for 30 min, rinsed in MQ and Mes, and then washed.
To study the induction by eggs of different ages, eggs were collected as above on paper, and then kept at room temperature until they were washed at the end of each day, until they hatched (6–7 days after oviposition). After hatching, young caterpillars were carefully removed using a brush. Empty eggshells and associated secretions (on paper) were then washed overnight (16 h).
To collect unfertilized eggs for egg wash, filter paper was pinned underneath a B. nigra leaf of a plant placed in a cage with 10 virgin adult females and then prepared in a similar way as fertilized eggs.
Paper sheets with M. brassicae eggs were obtained from a rearing population from the Laboratory of Entomology (WUR). To obtain egg wash, eggs were counted, spots with eggs were cut out and washed in 1 mL 20 mM Mes buffer (pH 5.7) per 400 eggs. The wash was pipetted off the next morning and frozen at −20°C until use.
For all experiments testing the effects of treating plants with egg washes, 10 μL egg wash (i.e., an equivalent of 25 eggs) was pipetted on the abaxial side of the fourth or fifth emerged leaf of 3–4 weeks old B. nigra plants, unless otherwise specified. Symptoms induced by egg wash were scored 4 days after treatment. To quantify severity, a scoring system from 0 to 4 was adapted from Griese et al. (2017). Severity score 0: no visual response; score 1: brown spots underneath eggs or egg wash spot, only visible at abaxial side leaf; score 2: cell death also visible at adaxial side of leaf, spot smaller than 2 mm diameter, score 3: cell death the size of egg wash spot, and score 4: spreading lesion beyond spot of treatment (Supplementary Figure S1). Score 0 and 1 are classified as “non-HR,” score 2–4 are classified as “HR”.
For experiments with oviposition on plants, one mated female P. brassicae butterfly was placed in a cage with a B. nigra or B. rapa plant and removed when ~10–25 eggs were laid. HR-like cell death was scored 4 days after oviposition, using the same scoring system as with egg wash. For experiments with M. brassicae, five mated female moths were placed together with a B. nigra plant in a cage to allow egg deposition overnight (16 h) and removed in the morning. No visible cell death was observed under M. brassicae eggs as was also reported in our previous studies (Fatouros et al., 2012; Griese et al., 2021).
For histochemical staining of plant tissue underneath eggs, P. brassicae egg-laden plants were sampled 24, 48, and 72 h after oviposition by taking a 10 mm diameter leaf disk of the area surrounding the eggs. Similarly this was done with plants treated with egg wash. Eggs were carefully removed with a brush before proceeding with histochemical staining. Pictures of the leaf disks were taken with a Dino-Lite digital microscope (AnMo Electronics Corporation, Taiwan) before staining (with eggs) and after staining (without eggs). As cell death is generally preceded by production of reactive oxygen species (ROS) such as hydrogen peroxide and superoxide anion (Torres, 2010), we visualized ROS with different histochemical stainings. 3,3′-diaminobenzidine (DAB; Sigma-Aldrich, MO, United States) was used to stain hydrogen peroxide (H2O2). Leaf disks (10 mm Ø) were submersed in 1 mg/mL DAB solution and samples were incubated for 30–60 min in the dark. Nitroblue tetrazolium chloride (NBT; Sigma-Aldrich, MO, United States) was used to stain superoxide radical O2•−. For this leaf disks (10 mm Ø) were submersed in 0.2% NBT with 50 mM sodium phosphate buffer (pH 7.5) and samples were incubated 30–60 min in the dark. For visualization of cell death, leaves were submersed in 0.08% trypan blue solution (Sigma-Aldrich, MO, United States), overnight (16 h). For all three stainings, destaining of leaves was performed with 96% ethanol. Pictures of leaf disks before staining (with eggs) and after staining (without eggs) were taken with a Dino-Lite digital microscope (AnMo Electronics Corporation, Taiwan). For staining of callose, leaf disks were first destained and then submersed in 0.01% aniline blue (Sigma-Aldrich, MO, United States) with 150 mM K2HPO4 and imaged after at least 2 h of incubation using a DAPI filter on a fluorescence microscope equipped with a DS-5MC camera and NIS elements AR 2.30 software.
In female butterflies, eggs are produced in the ovaries, pass through the common oviduct and are fertilized by sperm released from the bursa copulatrix (BC) into the vagina. Before being expelled through the ovipore, the eggs are covered by secretions released from the accessory reproductive gland (ARG), a paired gland that contains egg-enveloping secretions and glue-like material to attach eggs to leaves (Supplementary Figure S2). For dissection of tissues from the female reproductive tract, namely ARGs and BCs or eggs from the ovarian tubules (hereafter termed “ovarian eggs”), mated P. brassicae females were obtained by pairing a virgin female and virgin male 1 day after eclosion, which were kept together until mating was observed. Virgin females were obtained by keeping them separately shortly after eclosion. Three to four days after eclosion, mated and virgin P. brassicae females were killed by beheading using a scalpel and then dissected. ARGs, BC and ovarian eggs were dissected from females under a stereomicroscope (optical magnification 20×) in 20 mM Mes buffer. Dissected structures were washed overnight (16 h) in 20 mM Mes pH 5.7 using 50 μL per each ARG, 100 μL buffer per each BC or 5 μL buffer per egg. Solution was pipetted off the next morning and frozen until use (modified after Fatouros et al., 2015).
To characterize the chemical nature of EAMP in the egg wash we exposed it to different treatments. Untreated egg wash was used as a control. To study the stability of the elicitor, the egg wash was heated and frozen. For heating, egg wash was made as described above, collected in microcentifuge tubes and boiled in a water bath for 30 min at 95°C. For freezing treatment, egg wash was frozen at −20°C and then thawed for 1 h at room temperature, then frozen again. Freezing–thawing cycles were repeated up to four times, after which, all washes were used to treat plants. To study if the elicitor was an intact protein, a proteinase treatment was performed, a stock of 20 mg/mL Proteinase K (Qiagen, Cat No./ID: 19133) was diluted 100× in 100 μL egg wash (10% volume, pH 7.2) and incubated with egg wash for 30 min at 37°C.
Phosphatidylcholines (PCs) were recently found to be the main components of P. brassicae egg extracts that show a bioactivity in A. thaliana Col-0 resulting in induction of SA and PR1 marker gene as well as ROS accumulation and cell death formation (Stahl et al., 2020). Thus, we tested whether the same PCs used in Stahl et al. (2020) may be associated with development of HR-like cell death by B. nigra. Phospholipids were ordered from Avanti Polar Lipids (Alabaster, Alabama, United States). Specifically, PC(16:1/16:1), 1,2-dipalmitoleoyl-sn-glycero-3-phosphocholine, catalog no. 850358 and PC(18:1/18:1), 1,2-dioleoyl-sn-glycero-3-phosphocholine, catalog no. 850357 were used for experiments. Phospholipid stock solutions were made in 100% MeOH. For phospholipid application, the MeOH was evaporated under a nitrogen-flux and the phospholipids were dissolved in MQ water with 1% DMSO, 0.5% glycerol, 0.1% Tween 20 by sonication. Phospholipids were applied on B. nigra leaves at different concentrations (1, 5 and 10 μg/μL) that were previously shown to have biological activity in A. thaliana (Stahl et al., 2020). An MQ water solution of 1% DMSO, 0.5% glycerol and 0.1% Tween 20 was used as control.
Ethylene production is regularly measured to characterize the induction of early plant immune response by biotic stresses (Fan et al., 2017). Ethylene was measured as previously published (Oome et al., 2014). To measure the plant production of ethylene, in B. nigra, ten plants for each accession (with and without HR-like phenotype) were used. For B. rapa, three plants of each genotype were used. For each plant, leaf disks (3 mm Ø) were sampled from mature leaves of untreated plants and incubated overnight (16 h) in demineralised water. Subsequently, three leaf disks for each biological replicate (a single plant) were randomly chosen and incubated for 5 h in air tight glass vials with in either 400 μL of 20 mM Mes pH 5.7 or 400 μL egg wash (400 eggs/mL in same Mes buffer). After incubation, 1 mL of the headspace of each sample was taken to measure ethylene concentration on a Focus gas chromatograph (Thermo Electron S.p.A., Milan, Italy) equipped with an FID detector and a RT-QPLOT column, 15 m × 0.53 mm ID (Restek, Bellefonte, PA, United States). The system was calibrated with a certified gas of 1.01 μL L−1 (1 ppm) ethylene in synthetic air (Linde Gas Benelux B.V., Schiedam, Netherlands). After sampling leaf disks for the ethylene assay, plants were also treated with P. brassicae egg wash to determine their HR-like cell death phenotype.
To compare gene expression induced by eggs and egg wash, B. nigra plants were treated with either 10 μL of egg wash at lower concentration (400 eggs/mL in Mes buffer) or oviposited with an egg clutch of 10 eggs. Six leaf disks (Ø 6 mm) were sampled with a leaf puncher directly next to the eggs or the egg wash-treated spot at 0, 3, 6, 24, and 48 h, as done in previous research (Fatouros et al., 2014; Griese et al., 2021). For each timepoint, four plants were sampled individually and considered biological replicates. Brassica rapa plants were treated with three single eggs on a single leaf of each plant. Leaf disks (Ø 6 mm) were then harvested next to the eggs at 0, 3, 6, 24, and 96 h after treatment. For each timepoint, three plants were sampled individually and considered biological replicates.
To compare gene expression between P. brassicae and M. brassicae egg wash, B. nigra plants were treated with 10 μL of either egg wash (400 eggs/mL in Mes buffer) or a negative control (Mes buffer). Six leaf disks (Ø 6 mm) were sampled directly next to the egg wash-treated spot 24 h after treatment. Four plants were used for each treatment as biological replicates.
To compare the gene expression of two B. nigra accessions with contrasting ability to develop HR-like cell death, egg wash at higher concentration (~1,000 eggs/mL in demineralised water) was used. Experimental design consisted of two treatments (egg wash, control), two B. nigra accessions (WUR-01 without HR, and WUR-02, developing HR), and three time points after treatment (6, 24, and 48 h after treatment). For each treatment combination, egg wash or control were applied with two droplets of 5 μL on the abaxial side of a single leaf. Leaf disks (Ø 6 mm) were harvested at each time point on the treatment spots and disks from the same treatment on a leaf were pooled. For each treatment combination, a total of 15 plants were used and groups of 3 plants with similar treatments were pooled to compose a total of 5 biological replicates. For each gene expression experiment, samples were snap frozen in liquid nitrogen and stored at −80°C until use.
We investigated to which extent the difference in HR-like phenotype between B. nigra accessions WUR-01 and WUR-02 was related to activation of different phytohormones associated with plant immunity. Thus, we quantified expression of genes induced by SA, such as ICS1, PR1, and PR2 (Little et al., 2007), and genes regulated by JA, i.e., MYC2, VSP1, and VSP2 (Reymond et al., 2004). For experiments on gene expression induced by eggs or egg wash in both B. nigra and B. rapa, RNA extraction was performed according to Oñate-Sánchez and Vicente-Carbajosa (2008). For experiments on gene expression induced by egg wash in two B. nigra accessions, RNA extraction was performed with Direct-zol RNA Miniprep Kit (Zymo Research, CA, United States) following the manufacturer’s protocol. For preparation of cDNA of all experiments, 1 μg of RNA was reverse-transcribed using SensiFAST cDNA synthesis kit (Bioline, United Kingdom) according to the manufacturer’s instructions. Real time qRT-PCR reactions were performed using SensiFAST SYBR No-ROX Kit (Bioline, United Kingdom) in 10 μL reaction volumes, containing 3 μL cDNA and 500 nM of primers on a CFX96 Touch Real-Time PCR Detection System (Bio-Rad, CA, United States). The following qRT-PCR program was used: 95°C for 2 min followed by 40 cycles of 95°C for 5 s; primer-specific annealing temperature for 5 s and 72°C for 10 s, with data collection at 72°C, followed by a melt curve analysis. Relative gene expression was calculated with the ΔΔCq method, using GAPDH as reference gene. Primers sequences are available in Supplementary materials (Supplementary Table S1).
All data analysis was carried out in R (R Core Team, 2020). Scoring of HR-like cell death in severity categories were analyzed with a non-parametric method (Kruskal–Wallis test) on HR scores (0, 1, 2, 3, and 4) and different treatments were included as categorical fixed factors. For all other statistical analyses involving comparison of mean values (gene expression, ethylene production), the choice of parametric or non-parametric methods was made after checking the assumptions of normality (Shapiro–Wilk normality test) and homogeneity of variances (Fligner–Killeen test) on the raw data. As parametric methods, Student’s T-test, Welch T-test and ANOVAs followed by Tukey’s honestly significant difference test were used. As non-parametric method, Kruskal–Wallis test followed by pairwise Wilcoxon rank sum test was used. Gene expression data from qRT-PCR were calculated with the ΔΔCt method (Livak and Schmittgen, 2001) and were analyzed on log2-transformed data (parametric test) or on raw data (non-parametric test) specifying time points or treatments as factors.
We first investigated cellular responses against Pieris brassicae oviposition in its natural host, the black mustard Brassica nigra. Reactive oxygen species (ROS) such as superoxide anion (O2•−) and hydrogen peroxide (H2O2) accumulated underneath eggs at 24 h (Figure 1A). At the same time point, aniline blue staining in B. nigra revealed also callose deposition underneath eggs (Figure 1A). Further, the occurrence of cell death in plant tissue under the eggs was investigated by staining with trypan blue (TB), showing that cell death occurred 72 h after oviposition (Figure 1B). Occasionally, a few TB-stained cells were visible at 48 h (not shown). In B. nigra, we regularly observed a strong, macroscopically visible HR-like cell death that spreads beyond the egg site and stops 96 h after oviposition (Figure 1C). Cellular responses against P. brassicae oviposition were also investigated in B. rapa. ROS accumulation and cell death formation were also detected in B. rapa at 24 h and 72 h after oviposition, respectively (Supplementary Figures S2A,B). Although these early cellular responses appeared similar to what was observed in B. nigra, the macroscopically visible HR-like cell death developed by B. rapa appeared as black necrotic spots that never spread beyond the egg site (Supplementary Figure S2C).
Figure 1. Plant immunity responses induced in Brassica nigra by Pieris brassicae eggs (Bn). (A) B. nigra leaf 24 h after oviposition with accumulation of O2•− (NBT staining), H2O2 (DAB staining) and callose deposition (aniline blue staining). (B) B. nigra leaf 72 h after oviposition showing cell death (trypan blue staining). (C) Fully developed HR-like cell death that is macroscopically visible at 96 h after oviposition. A few eggs were removed from the clutch to show the cell death underneath. Stainings were repeated multiple times with similar results. Scale bars = 1 mm.
To isolate potential EAMPs and to quickly screen plants for HR-like cell death, we developed a method to dissolve egg-enveloping secretions from P. brassicae eggs (Methods section; Supplementary Figure S1). We then compared visually whether the treatment of leaves with egg wash induced a similar HR-like cell death as the butterfly eggs (Figure 2A). As a control, leaves were treated with only the buffer (no eggs were washed). Symptoms induced by eggs or egg wash were scored after 4 days, and HR-like frequency (proportion of plants showing HR-like) and HR-like severity (mean score of induced symptoms) were compared between the two treatments in B. nigra. HR-like severity after oviposition by eggs or treatment with egg wash did not differ (Kruskal–Wallis: χ2 = 1.33, df = 1, p = 0.24; Figure 2B; Supplementary Table S2).
Figure 2. Plant responses induced by Pieris brassicae eggs and egg wash in Brassica nigra. (A) Picture of responses induced by eggs and egg wash next to each other on a leaf (microscopic image): both eggs and egg wash induce HR-like necrotic spots. (B) Quantification of severity of symptoms induced by egg wash and eggs. Shown is the fraction of plants that were scored in a specific class for each treatment (N = 32 plants for both treatments; Kruskal–Wallis, p > 0.05). ns = no significant difference. (C) BnPR1 expression in plants after egg deposition (red box plots) or treatment with egg wash (blue box plots). Gene expression was measured by qRT-PCR and normalized to the housekeeping gene BnGADPH. Each treatment consisted of 3–4 biological replicates. Expression at each time point was compared to the 0 h timepoint (Kruskal–Wallis, p < 0.05 for eggs and p < 0.05 for egg wash). (D) Ethylene production in parts per million (ppm) by plants treated with egg wash. (Student’s T-test, p < 0.001). Each treatment consisted of 10 biological replicates. The height of the boxes in C-D represents the first to the third quartile of the range; the horizontal line within the box is the median; the whiskers indicate the data minimum and maximum; and dots represent data points. Asterisk indicates p < 0.05, *** <0.001.
We compared expression of SA-marker gene PR1 in B. nigra oviposited on by P. brassicae butterflies or treated with egg wash. PR1 was significantly upregulated both after oviposition and after treatment with egg wash. In B. nigra, PR1 expression increased at 6 h and was significantly upregulated at 24 h and 48 h after egg deposition (Kruskal–Wallis: χ2 = 12.23, df = 3, p = 0.015; Figure 2C). No significant differences in PR1 expression were found between B. nigra plants treated with eggs or egg wash (Figure 2C; Supplementary Table S3). Similarly, in B. rapa, PR1 expression was significantly upregulated by egg deposition at 24 h and showed further increase at 96 h (Kruskal–Wallis: χ2 = 11.18, df = 4, p = 0.024; Supplementary Figure S2D).
Next, we tested whether egg wash induced ethylene. Brassica nigra leaves responded with higher ethylene production after incubation with egg wash compared to incubation with control Mes buffer (Figure 2D; Supplementary Table S4). In addition, there was a significant difference in ethylene produced between plants with contrasting HR-like phenotypes. Plants responding with stronger HR-like cell death (score 2 or higher), produced a significantly higher amount of ethylene after incubation with egg wash than plants with no HR-like cell death (Student’s T-test = −4.087, df = 18, p < 0.001; Figure 2D; Supplementary Table S4). Similarly, B. rapa plants developing HR-like cell death, also showed a higher ethylene production upon incubation of leaves with P. brassicae egg wash (Student’s T-test = −3.876, df = 4, p = 0.018; Supplementary Figure S3E). These results suggest that there is an early detection response in plants after contact with egg wash that will ultimately lead to cell death.
All SA marker genes were upregulated in B. nigra plants upon egg wash treatment compared to control. However, the magnitude of expression of SA-related genes was significantly different between the two B. nigra accessions at different time points (Figure 3). In contrast, relative expression of JA-related genes was generally very low and not significantly different between two accessions. SA-marker BnICS1 was significantly expressed at higher levels in plants that showed a visible HR already at 6 h after treatment (Welch’s T-test = −4.901, df = 5.3, p = 0.003). Both, BnPR1 and BnPR2 showed increased expression across the time points, although with a different magnitude between the two accessions. Both genes were already expressed at higher level at 6 h in plants that showed a visible HR (Welch’s T-test = −2.932, df = 5.3, p = 0.03 and Welch’s T-test = −4.850, df = 5.8, p = 0.003 respectively), and the difference with plants not expressing HR increased up to 5-fold (24 h) and 20-fold (48 h) for BnPR1 and 4-fold (24 h) and 5-fold (48 h) for BnPR2. The expression of JA-related genes showed more stable profiles across time points, with a small peak at 24 h in all treatments, but with no significant differences between the two accessions (MYC2, Welch’s T-test = −1.549, df = 5.8 p > 0.05; VSP1, Welch’s T-test = −0.141, df = 3 p > 0.05; VSP2, Welch’s T-test = −0.301, df = 3.5 p > 0.05).
Figure 3. Expression of SA- and JA-related defense genes upon P. brassicae egg wash treatment in two different B. nigra accessions. Gene expression was measured with two treatments (egg wash, control), two accessions (no HR and HR) and at three time points (6, 24, and 48 h after treatment). HR phenotype of each plant was scored after sampling for RNA isolation. Gene expression was measured by qPCR-RT and normalized to housekeeping gene BnGADPH. Expression levels were calculated at each time point relative to control treatment (water droplets). Each treatment consisted of 4–5 biological replicates and is reported as boxplots. The height of the boxes in represents the first to the third quartile of the range; the horizontal line within the box is the median; the whiskers indicate the data minimum and maximum; and dots represent data points. At each time point, gene expression between the two accessions was compared (Welch’s T-test). Asterisks indicate different p-values within the same time point: ** <0.01, *** <0.001, no asterisks indicate no significant difference.
Staining of leaves showed that plant cells did not die underneath M. brassicae eggs as leaves did not stain with trypan blue (Figures 4A,B). Further, M. brassicae eggs induced O2•− production in some plants, but weaker than Pieris eggs (Figures 4C,D). While P. brassicae egg wash induced the expression of PR1 after 24 h (ANOVA followed by Tukey, p < 0.01), PR1 expression induced by M. brassicae egg wash was not different from the control treatment (Figure 4C; Supplementary Table S5). In addition, incubation with M. brassicae egg wash induced lower ethylene production compared to P. brassicae egg wash (Kruskal–Wallis: χ2 = 21.36, df = 2, p < 0.001; Figure 4D), comparable to the ethylene levels previously observed in B. nigra plants lacking a visible cell death (Figure 2D). Overall, eggs of M. brassicae did not induce cell death and only a weak ROS and ethylene response in B. nigra.
Figure 4. Responses to eggs and egg wash of M. brassicae in B. nigra. (A) Leaf of B. nigra oviposited on by M. brassicae moth by NBT showing light O2•− accumulation underneath M. brassicae eggs. (B) Leaf stained by trypan blue showing no cell death underneath M. brassicae eggs. (C) BnPR1 expression in leaf tissue treated with control solution, P. brassicae wash or M. brassicae wash. Different letters indicate significant differences in mean PR1 expression (ANOVA followed by Tukey, p < 0.01). (D) Ethylene production in B. nigra leaf in response to egg washes. The height of the boxes in C,D represents the first to the third quartile of the range; the horizontal line within the box is the median; the whiskers indicate the data minimum and maximum; and dots represent data points. Values that have no accompanying letters in common differ significantly in production of ethylene (Kruskal–Wallis followed by pairwise Wilcoxon test, p < 0.001). Scale bars = 1 mm.
There was no significant difference in eliciting activity of eggs of increasing age, from one-day-old, to five-day-old eggs, although HR-like severity decreased slightly. Egg wash was also prepared from eggshells and secretions that remained on the paper after caterpillars hatched, and this still induced HR-like symptoms (Kruskal–Wallis: χ2 = 3.20, df = 5, p > 0.1; Figure 5A; Supplementary Table S6).
Figure 5. Proportion of HR-like cell death induced by washes made of eggs, washes made of tissues of the reproductive tract of Pieris brassicae, and washes of eggs with and without glue, in Brassica nigra. (A–D) (A) Egg washes made of eggs of different ages or eggshells and egg glue remaining on filter paper. N plants = 13–26 (B) Egg wash of dissected structures of reproductive tract. N plants = 5. (C) Egg wash of eggs of mated females versus virgin females. N plants = 8–24 (D) Wash of eggs, glue alone (in filter paper) or wash of eggs with egg-enveloping secretions removed. For glue removal, two treatments were used, either a 1% bleach wash or wash with NaPO4. N plants = 8–16. Values that have no accompanying letters in common differ significantly (Kruskal–Wallis).
A wash of dissected accessory reproductive glands (ARGs) induced HR-like cell death, similar to the positive control P. brassicae egg wash (Figure 5B). On the contrary, neither a wash of mature but unfertilized eggs dissected from the ovary (“ovarian eggs”) nor a wash of the sperm-containing bursa copulatrix (BC) induced symptoms (Figure 5B). HR-like severity was significantly higher in plants treated with egg wash or a wash of ARG, compared to wash of ovarian eggs or bursa copulatrix (Kruskal–Wallis: χ2 = 19.833, df = 4, p < 0.001; Supplementary Table S6).
A wash from unfertilized, deposited eggs (containing secretions from the ARG) from virgin butterflies induced a similar response as wash from fertilized, deposited eggs of mated females (Figure 5C). There was no significant effect of the mating status of the female (mated or virgin) on the frequency of HR-like cell death elicited or on HR severity (Kruskal–Wallis: χ2 = 2.61, df = 1, p > 0.1). In addition, the wash of ARGs from virgin females induced strong symptoms similar to those of mated females (Figure 5B). These results show that egg fertilization is not necessary for the induction of the HR-like cell death in B. nigra, and that an EAMP resides in the ARG, and is female-derived.
Finally, both egg wash and wash of glue alone induced a severe HR-like cell death, and HR-like severity was significantly lower when B. nigra was treated with a wash of eggs from which the glue was removed (Kruskal–Wallis: H = 26.60, df = 3, p < 0.001; Figure 5D; Supplementary Table S6).
None of the egg wash treatments conducted, i.e., freezing and thawing, proteinase K, or boiling, had an effect on its bioactivity. That the elicitor does not lose the capability to induce HR in B. nigra leaves after these treatments, indicates that it is a small, stable, water-soluble molecule that is likely not a protein (Figure 6; Supplementary Table S7).
Figure 6. Proportion of HR-like cell death induced by differently treated egg washes made of Pieris brassicae eggs in Brassica nigra. (A,B) Severity of HR-like symptoms induced in plants by the different washes. (A) Egg washes that were subjected to increasing amount of freeze–thaw cycles. N plants/treatment = 24–32. NS (B) Egg washes subjected to boiling (95°C for 30 min) or incubated with proteinase K. N plants/treatment = 9. NS, not significant, Kruskal–Wallis test.
Lastly, we tested whether two phosphatidylcholines (PC) could also induce a HR-like responses in B. nigra. We did not observe any visible cell death upon treatment of B. nigra with neither PC16:1/PC16:1 nor PC18:1/PC18:1 (Figure 7; Supplementary Table S8).
Figure 7. Phosphatidylcholines (PC) does not induce HR-like cell death in B. nigra plants unlike P. brassicae egg wash. Brassica nigra (SF48-O1) plants were treated with phosphatidylcholines PC(16:1/16:1) and PC(18:1/18:1) or with egg wash (EW). PCs were solubilized in 1% DMSO, 0.5% Glycerol and 0.1% Tween and were applied at different concentrations (1, 5, and 10 μg/μL). A solution of 1% DMSO, 0.5% Glycerol and 0.1% Tween was used as control (ctl). Egg wash400 was prepared with 400 eggs/mL, egg wash1000 was prepared with 1,000–1,200 eggs/1 mL. All treatments were applied on the same leaf, with two leaves per plant, on 15 biological replicates. Scale bar = 1.
In this study, we show that HR-like inducing EAMPs are in the egg-enveloping secretions that are produced in female ARGs. Our results indicate that the EAMP is unlikely of lipidic or proteinaceous nature but an organic compound of low-molecular weight. When plants are treated with egg wash, HR-like cell death is induced in responsive plant genotypes, together with upregulation of SA-responsive genes and elevated levels of ethylene suggesting that the HR-like cell death is mediated by one or both phytohormones. In addition, deposition of eggs leads to ROS and callose deposition, also in plant genotypes that do not show HR-like cell death. All phenotypes observed were shared between the two Brassica species that vary in cell death severity and are specific to eggs and egg wash of the specialist P. brassicae.
The increasing knowledge on plant defense mechanisms to eggs of herbivores suggests that plants can specifically recognize and respond to egg deposition, presumably via the detection of EAMPs. However, very few EAMPs have been identified, and those that have so far, are organic compounds of low-molecular weight associated with the eggs (Reymond, 2013; Hilker and Fatouros, 2015; Stahl et al., 2018). Only recently a novel type of EAMP, a proteinaceous compound, diprionin, was found as an elicitor of pine defense against sawfly eggs (Hundacker et al., 2021). Diprionin is an annexin-like protein which is released with the egg-associated secretions of the sawfly Diprion pini into needles of pines. When pine twigs were treated with diprionin they emitted similar quantities of the sesquiterpene (E)-β-farnesene that is known to attract egg parasitoids like when laden with sawfly eggs (Hundacker et al., 2021).
Our study reveals that the EAMP associated with P. brassicae eggs likely belongs to the first category of non-proteinaceous molecules. Neither boiling nor treatment of the egg wash with proteinase K had an effect on elicitation of HR-like. Recently, phosphatidylcholines (PCs) were identified as EAMPs of the P. brassicae egg-induced response in A. thaliana. Active PCs were mainly containing C16- and C18-fatty acyl chains and treatment with the latter resulted in SA, H2O2, induction of PR1 and trypan blue staining (Stahl et al., 2020). PCs are components of cell membranes. Earlier, phospholipids of Sogatella furcifera Horváth were also found to induce an ovicidal response in rice (Yang J. O. et al., 2014). Our results suggest that the EAMPs in P. brassicae eggs that induce HR-like cell death in B. nigra are other compounds than PCs. First, the B. nigra response is specific to Pieris eggs and egg wash and is absent in response to other lepidopteran eggs. Second, given our method used of washing eggs in a water-like buffer, lipids are not expected to be present in (high amounts in) the wash. Finally, as PCs are present in membranes, ovarian eggs should also induce the response. Indeed, ovarian eggs alone induced PR1 expression in A. thaliana (Little et al., 2007) while we showed that ovarian eggs did not induce HR-like cell death in B. nigra.
When washing eggs, mainly compounds from outside of the eggs and from egg-enveloping secretions are dissolved. We present evidence that at least one Pieris-specific EAMP is in these secretions that envelop the eggs: (i) wash of glue on filter paper is sufficient to induce the HR-like cell death, (ii) when the secretions are removed from eggs, and eggs are then washed, HR-like cell death in plants is diminished, and (iii) a wash of ARGs, the organs where secretions are produced, is also sufficient to induce HR-like cell death. As the egg surface and egg-exterior associated secretions are in direct contact with the leaves, it could be expected that plants evolve to detect elicitors in egg-enveloping secretions. It resembles the natural situation of leaf-egg interaction, more so than, for example, crushing of eggs (Little et al., 2007; Bruessow et al., 2010) or crushing of adults (Doss et al., 2000; Yang Y. et al., 2014). Previously, a male-derived anti-aphrodisiac compound transferred during mating to the female ARG, benzyl cyanide, was suggested as potential elicitor (Fatouros et al., 2008). We find no evidence for a male-derived elicitor: eggs and ARGs of virgin P. brassicae butterflies induced HR-like cell death in an equal manner as mated butterflies. We thus hypothesize that at least one HR-inducing EAMP is present in the egg-enveloping secretions and ARGs and is female-derived. Chemical analysis of egg wash and glands is currently carried out to identify this EAMP.
Plant responses to insect eggs are similar to responses to (microbial) pathogens, and include SA and ROS accumulation, callose deposition, defense gene expression and cell death (Reymond, 2013). For example, callose deposition was shown to be associated with lesions following pathogen invasion or autoimmune responses (Koga et al., 1988; Dietrich et al., 1994). We observed the deposition of callose in B. nigra associated with lesions induced by P. brassicae eggs. Natural variation in strength of egg-induced cell death was found in several brassicaceous species, including B. nigra (Pashalidou et al., 2015; Griese et al., 2021; Groux et al., 2021). Here, we show that plants that do not express a strong HR-like cell death, still responded with ROS accumulation and cell death as showed by trypan blue staining. Similarly, in S. dulcamara, variation exists for egg-induced chlorosis, and a genotype that did not respond with chlorosis and on which egg hatching rate of S. exigua was not reduced, still accumulated SA after oviposition (Geuss et al., 2017). We thus hypothesize that Pieris eggs generally induce an immune response in all plants of B. rapa and B. nigra, that is only in some plants accompanied by a stronger cell death response. In pathogen-induced HR, cell death can often be uncoupled from (preceding) biochemical and molecular changes and the two processes can be genetically dissected (Künstler et al., 2016). In that case, cell death is dispensable for resistance. However, in egg-induced HR, previous studies show that the stronger the HR-like cell death, the higher egg mortality (Fatouros et al., 2014; Griese et al., 2017, 2020, 2021).
In A. thaliana, PR1 expression was also found in response to crushed egg extracts of different insects (Bruessow et al., 2010; Stahl et al., 2020). In B. nigra, there was no cell death underneath M. brassicae eggs, and neither induction of PR1 nor ethylene production in response to M. brassicae egg wash. Our results suggest that cell death, ethylene production and gene expression, at least in B. nigra, are specific to P. brassicae eggs, and we expect the response to be activated after detection of a Pierinae-specific elicitor. This hypothesis is strengthened by our previous work which showed Pierinae-specific induction of PR1 in B. nigra (Griese et al., 2021). It is possible that a mild cellular defense-like response is activated against a general insect-derived EAMP, for example PCs, while the strong HR-like is activated only by EAMPs specifically derived from Pieris spp. in those plants that can detect these. The production of ethylene after incubation with egg wash only in plants that show a strong HR-like cell death (and not in non-HR plants), points to this effect. Mapping efforts in B. nigra plants, can reveal whether the genetic variation in plants is for the HR-like cell death and/or for the detection of a Pieris-specific elicitor.
Molecular patterns that are detected by plants are thought to be structurally conserved molecules (Van der Burgh and Joosten, 2019). The presence of EAMPs in the glue suggests that their function could be a structural component of the glue, or a compound with an essential function to the fertilized eggs. Many proteins are found in glue of insect eggs (Li et al., 2008), and egg glue of P. brassicae was described to consist of proteins and unsaturated lipoids (Beament and Lal, 1957). In addition, the ARG secretions could contain molecules that are produced by the parents and/or microbial symbionts to protect the vulnerable egg, for example compounds with antimicrobial activity (Flórez et al., 2018). Further identification of EAMPs can lead to new research in this direction.
In summary, we present a method to obtain EAMPs from insect eggs, and using this method, show that the EAMP inducing the HR-like cell death response is in the egg glue, derived from the female ARG. We furthermore assess the chemical nature and specificity of these elicitors and the molecular response of Brassica plants to Pieris and other eggs. The obtained knowledge paves the way for future studies on identification of EAMPs in Pieris egg glue, and the corresponding receptor genes in Brassica plants.
The datasets presented in this study can be found in the data repository Zenodo at doi.org/10.5281/zenodo.7194716.
LC, NB, JL, RM, and NF planned and designed the research. LC, NB, and PV performed the experiments and/or analyzed the data. LC, NB, and NF wrote the manuscript with contributions by PV, RM, JL, and MS. All authors contributed to the article and approved the submitted version.
This research was made possible by support of the Dutch Technology Foundation TTW, which is part of Netherlands Organisation for Scientific Research (NWO) (NWO/TTW VIDI grant 14854 to N.E.F.).
We are grateful to the employees of Unifarm (WUR) for rearing and caring of the plants used in the experiment. We thank Pieter Rouweler, André Gidding, and the late Frans van Aggelen for rearing of Pieris brassicae and Mamestra brassicae. We thank Martijn Flipsen, Gabriella Bukovinszkine’Kiss, Klaas Bouwmeester, Femke van Doesburg, Ewan van Eijden and Arjen van der Peppel for assistance with some of the experiments. Guusje Bonnema and Erik Poelman are acknowledged for seeds of B. rapa and B. nigra, respectively.
The authors declare that the research was conducted in the absence of any commercial or financial relationships that could be construed as a potential conflict of interest.
All claims expressed in this article are solely those of the authors and do not necessarily represent those of their affiliated organizations, or those of the publisher, the editors and the reviewers. Any product that may be evaluated in this article, or claim that may be made by its manufacturer, is not guaranteed or endorsed by the publisher.
The Supplementary material for this article can be found at: https://www.frontiersin.org/articles/10.3389/fevo.2022.1070859/full#Supplementary-material
Balint-Kurti, P. (2019). The plant hypersensitive response: concepts, control and consequences. Mol. Plant Pathol. 20, 1163–1178. doi: 10.1111/mpp.12821
Bassetti, N., Caarls, L., Bukovinszkine’kiss, G., El-Soda, M., Van Veen, J., Bouwmeester, K., et al. (2022). Genetic analysis reveals three novel QTLs underpinning a butterfly egg-induced hypersensitive response-like cell death in Brassica rapa. BMC Plant Biol. 22:140. doi: 10.1186/s12870-022-03522-y
Beament, J. W. L., and Lal, R. (1957). Penetration through the egg-shell of Pieris brassicae (L.). Bull. Entomol. Res. 48, 109–125. doi: 10.1017/S0007485300054134
Bruessow, F., Gouhier-Darimont, C., Buchala, A., Metraux, J.-P., and Reymond, P. (2010). Insect eggs suppress plant defence against chewing herbivores. Plant J. 62, 876–885. doi: 10.1111/j.1365-313X.2010.04200.x
Campos, M. L., De Souza, C. M., De Oliveira, K. B. S., Dias, S. C., and Franco, O. L. (2018). The role of antimicrobial peptides in plant immunity. J. Exp. Bot. 69, 4997–5011. doi: 10.1093/jxb/ery294
Couto, D., and Zipfel, C. (2016). Regulation of pattern recognition receptor signalling in plants. Nat. Rev. Immunol. 16, 537–552. doi: 10.1038/nri.2016.77
Cui, H., Tsuda, K., and Parker, J. E. (2015). Effector-triggered immunity: from pathogen perception to robust defense. Annu. Rev. Plant Biol. 66, 487–511. doi: 10.1146/annurev-arplant-050213-040012
Dalio, R. J. D., Paschoal, D., Arena, G. D., Magalhães, D. M., Oliveira, T. S., Merfa, M. V., et al. (2020). Hypersensitive response: from NLR pathogen recognition to cell death response. Ann. Appl. Biol. 178, 268–280. doi: 10.1111/aab.12657
Dietrich, R. A., Delaney, T. P., Uknes, S. J., Ward, E. R., Ryals, J. A., and Dangl, J. L. (1994). Arabidopsis mutants simulating disease resistance response. Cells 77, 565–577. doi: 10.1016/0092-8674(94)90218-6
Doss, R. P., Oliver, J. E., Proebsting, W. M., Potter, S. W., Kuy, S., Clement, S. L., et al. (2000). Bruchins: insect-derived plant regulators that stimulate neoplasm formation. Proc. Natl. Acad. Sci. U. S. A. 97, 6218–6223. doi: 10.1073/pnas.110054697
Erb, M., and Reymond, P. (2019). Molecular interactions between plants and insect herbivores. Annu. Rev. Plant Biol. 70, 527–557. doi: 10.1146/annurev-arplant-050718-095910
Fan, L., Chae, E., Gust, A. A., and Nürnberger, T. (2017). Isolation of novel MAMP-like activities and identification of cognate pattern recognition receptors in Arabidopsis thaliana using next-generation sequencing (NGS)–based mapping. Current Protocols in Plant Biology 2, 173–189. doi: 10.1002/cppb.20056
Fatouros, N. E., Broekgaarden, C., Bukovinszkine'kiss, G., Van Loon, J. J. A., Mumm, R., Huigens, M. E., et al. (2008). Male-derived butterfly anti-aphrodisiac mediates induced indirect plant defense. Proc. Natl. Acad. Sci. 105, 10033–10038. doi: 10.1073/pnas.0707809105
Fatouros, N. E., Lucas-Barbosa, D., Weldegergis, B. T., Pashalidou, F. G., Van Loon, J. J. A., Dicke, M., et al. (2012). Plant volatiles induced by herbivore egg deposition affect insects of different trophic levels. PLoS One 7:e43607. doi: 10.1371/journal.pone.0043607
Fatouros, N. E., Paniagua Voirol, L. R., Drizou, F., Doan, Q. T., Pineda, A., Frago, E., et al. (2015). Role of large cabbage white butterfly male-derived compounds in elicitation of direct and indirect egg-killing defenses in the black mustard. Front. Plant Sci. 6:794. doi: 10.3389/fpls.2015.00794
Fatouros, N. E., Pashalidou, F. G., Aponte Cordero, W. V., Van Loon, J. J. A., Mumm, R., Dicke, M., et al. (2009). Anti-aphrodisiac compounds of male butterflies increase the risk of egg parasitoid attack by inducing plant synomone production. J. Chem. Ecol. 35, 1373–1381. doi: 10.1007/s10886-009-9714-5
Fatouros, N. E., Pineda, A., Huigens, M. E., Broekgaarden, C., Shimwela, M. M., Figueroa Candia, I. A., et al. (2014). Synergistic effects of direct and indirect defences on herbivore egg survival in a wild crucifer. Proc. R. Soc. Biol. 281:20141254. doi: 10.1098/rspb.2014.1254
Flórez, L. V., Scherlach, K., Miller, I. J., Rodrigues, A., Kwan, J. C., Hertweck, C., et al. (2018). An antifungal polyketide associated with horizontally acquired genes supports symbiont-mediated defense in Lagria villosa beetles. Nat. Commun. 9:2478. doi: 10.1038/s41467-018-04955-6
Geuss, D., Stelzer, S., Lortzing, T., and Steppuhn, A. (2017). Solanum dulcamara response to eggs of an Arabidopsis thaliana herbivore comprises ovicidal hydrogen peroxide production. Plant Cell Environ. 40, 2663–2677. doi: 10.1111/pce.13015
Gouhier-Darimont, C., Schmiesing, A., Bonnet, C., Lassueur, S., and Reymond, P. (2013). Signalling of Arabidopsis thaliana response to Pieris brassicae eggs shares similarities with PAMP-triggered immunity. J. Exp. Bot. 64, 665–674. doi: 10.1093/jxb/ers362
Griese, E., Caarls, L., Bassetti, N., Mohammadin, S., Verbaarschot, P., Bukovinszkine’kiss, G., et al. (2021). Insect egg-killing: a new front on the evolutionary arms-race between brassicaceous plants and pierid butterflies. New Phytol. 230, 341–353. doi: 10.1111/nph.17145
Griese, E., Dicke, M., Hilker, M., and Fatouros, N. E. (2017). Plant response to butterfly eggs: inducibility, severity and success of egg-killing leaf necrosis depends on plant genotype and egg clustering. Sci. Rep. 7:7316. doi: 10.1038/s41598-017-06704-z
Griese, E., Pineda, A., Pashalidou, F. G., Iradi, E. P., Hilker, M., Dicke, M., et al. (2020). Plant responses to butterfly oviposition partly explain preference–performance relationships on different brassicaceous species. Oecologia 192, 463–475. doi: 10.1007/s00442-019-04590-y
Groux, R., Stahl, E., Gouhier-Darimont, C., Kerdaffrec, E., Jimenez-Sandoval, P., Santiago, J., et al. (2021). Arabidopsis natural variation in insect egg-induced cell death reveals a role for LECTIN RECEPTOR KINASE-I.1. Plant Physiol. 185, 240–255. doi: 10.1093/plphys/kiaa022
Gust, A. A., Pruitt, R., and Nürnberger, T. (2017). Sensing danger: key to activating plant immunity. Trends Plant Sci. 22, 779–791. doi: 10.1016/j.tplants.2017.07.005
Hilker, M., and Fatouros, N. E. (2015). Plant responses to insect egg deposition. Annu. Rev. Entomol. 60, 493–515. doi: 10.1146/annurev-ento-010814-020620
Hilker, M., and Fatouros, N. E. (2016). Resisting the onset of herbivore attack: plants perceive and respond to insect eggs. Curr. Opin. Plant Biol. 32, 9–16. doi: 10.1016/j.pbi.2016.05.003
Hilker, M., Stein, C., Schröder, R., Varama, M., and Mumm, R. (2005). Insect egg deposition induces defence responses in Pinus sylvestris: characterisation of the elicitor. J. Exp. Biol. 208, 1849–1854. doi: 10.1242/jeb.01578
Hundacker, J., Bittner, N., Weise, C., Bröhan, G., Varama, M., and Hilker, M. (2021). Pine defense against eggs of an herbivorous sawfly is elicited by an annexin-like protein present in egg-associated secretion. Plant Cell Environ. 45, 1033–1048. doi: 10.1111/pce.14211
Jacobs, C. G. C., Rezende, G. L., Lamers, G. E. M., and Van Der Zee, M. (2013). The extraembryonic serosa protects the insect egg against desiccation. Proc. R. Soc. B Biol. Sci. 280:20131082. doi: 10.1098/rspb.2013.1082
Koga, H., Zeyen, R., Bushnell, W. R., and Ahlstrand, G. (1988). Hypersensitive cell death, autofluorescence, and insoluble silicon accumulation in barley leaf epidermal cells under attack by Erysiphe graminis f. sp. hordei. Physiol. Mol. Plant Pathol. 32, 395–409. doi: 10.1016/S0885-5765(88)80033-X
Künstler, A., Bacsó, R., Gullner, G., Hafez, Y. M., and Király, L. (2016). Staying alive – is cell death dispensable for plant disease resistance during the hypersensitive response? Physiol. Mol. Plant Pathol. 93, 75–84. doi: 10.1016/j.pmpp.2016.01.003
Li, D., Huson, M. G., and Graham, L. D. (2008). Proteinaceous adhesive secretions from insects, and in particular the egg attachment glue of Opodiphthera sp. moths. Arch. Insect Biochem. Physiol. 69, 85–105. doi: 10.1002/arch.20267
Little, D., Gouhier-Darimont, C., Bruessow, F., and Reymond, P. (2007). Oviposition by pierid butterflies triggers defense responses in Arabidopsis. Plant Physiol. 143, 784–800. doi: 10.1104/pp.106.090837
Livak, K. J., and Schmittgen, T. D. (2001). Analysis of relative gene expression data using real-time quantitative PCR and the 2-ΔΔCT method. Methods 25, 402–408. doi: 10.1006/meth.2001.1262
Lortzing, T., Kunze, R., Steppuhn, A., Hilker, M., and Lortzing, V. (2020). Arabidopsis, tobacco, nightshade and elm take insect eggs as herbivore alarm and show similar transcriptomic alarm responses. Sci. Rep. 10:16281. doi: 10.1038/s41598-020-72955-y
Oñate-Sánchez, L., and Vicente-Carbajosa, J. (2008). DNA-free RNA isolation protocols for Arabidopsis thaliana, including seeds and siliques. BMC. Res. Notes 1:93. doi: 10.1186/1756-0500-1-93
Oome, S., Raaymakers, T. M., Cabral, A., Samwel, S., Böhm, H., Albert, I., et al. (2014). Nep1-like proteins from three kingdoms of life act as a microbe-associated molecular pattern in Arabidopsis. Proc. Natl. Acad. Sci. U. S. A. 111, 16955–16960. doi: 10.1073/pnas.1410031111
Paniagua Voirol, L. R., Valsamakis, G., Lortzing, V., Weinhold, A., Johnston, P. R., Fatouros, N., et al. (2020). Plant responses to insect eggs are not induced by egg-associated microbes, but by a secretion attached to the eggs. Plant Cell Environ. 43, 1815–1826. doi: 10.1111/pce.13746
Pashalidou, F. G., Fatouros, N. E., Van Loon, J. J. A., Dicke, M., and Gols, R. (2015). Plant-mediated effects of butterfly egg deposition on subsequent caterpillar and pupal development, across different species of wild Brassicaceae. Ecol. Entomol. 40, 444–450. doi: 10.1111/een.12208
R Core Team (2020). R: A Language and Environment for Statistical Computing. Vienna, Austria: R Foundation for Statistical Computing.
Reymond, P. (2013). Perception, signaling and molecular basis of oviposition-mediated plant responses. Planta 238, 247–258. doi: 10.1007/s00425-013-1908-y
Reymond, P., Bodenhausen, N., Van Poecke, R. M. P., Krishnamurthy, V., Dicke, M., and Farmer, E. E. (2004). A conserved transcriptional pattern in response to a specialist and a generalist herbivore. Plant Cell 16, 3132–3147. doi: 10.1105/tpc.104.026120
Salerno, G., De Santis, F., Iacovone, A., Bin, F., and Conti, E. (2013). Short-range cues mediate parasitoid searching behavior on maize: the role of oviposition-induced plant synomones. Biol. Control 64, 247–254. doi: 10.1016/j.biocontrol.2012.12.004
Shapiro, A. M., and Devay, J. E. (1987). Hypersensitivity reaction of Brassica nigra L. (Cruciferae) kills eggs of Pieris butterflies (lepidoptera: Pieridae). Oecologia 71, 631–632. doi: 10.1007/BF00379310
Stahl, E., Brillatz, T., Queiroz, E. F., Marcourt, L., Schmiesing, A., Hilfiker, O., et al. (2020). Phosphatidylcholines from Pieris brassicae eggs activate an immune response in Arabidopsis. elife 9:e60293. doi: 10.7554/eLife.60293
Stahl, E., Hilfiker, O., and Reymond, P. (2018). Plant-arthropod interactions: who is the winner? Plant J. 93, 703–728. doi: 10.1111/tpj.13773
Steinbrenner, A. D., Munoz-Amatriain, M., Chaparro, A. F., Jessica Montserrat, A.-V., Lo, S., Okuda, S., et al. (2020). A receptor-like protein mediates plant immune responses to herbivore-associated molecular patterns. Proc. Natl. Acad. Sci. U. S. A. 117, 31510–31518. doi: 10.1073/pnas.2018415117
Tamiru, A., Bruce, T. J. A., Woodcock, C. M., Caulfield, J. C., Midega, C. A. O., Ogol, C. K. P. O., et al. (2011). Maize landraces recruit egg and larval parasitoids in response to egg deposition by a herbivore. Ecol. Lett. 14, 1075–1083. doi: 10.1111/j.1461-0248.2011.01674.x
Torres, M. A. (2010). ROS in biotic interactions. Physiol. Plant. 138, 414–429. doi: 10.1111/j.1399-3054.2009.01326.x
Van Der Burgh, A. M., and Joosten, M. H. A. J. (2019). Plant immunity: thinking outside and inside the box. Trends Plant Sci. 24, 587–601. doi: 10.1016/j.tplants.2019.04.009
Wilkinson, S. W., Mageroslashy, M. H., Lopez Sanchez, A., Smith, L. M., Furci, L., Cotton, T. E. A., et al. (2019). Surviving in a hostile world: plant strategies to resist pests and diseases. Annu. Rev. Phytopathol. 57, 505–529. doi: 10.1146/annurev-phyto-082718-095959
Yang, J. O., Nakayama, N., Toda, K., Tebayashi, S., and Kim, C. S. (2014). Structural determination of elicitors in Sogatella furcifera (Horváth) that induce japonica rice plant varieties (Oryza sativa L.) to produce an ovicidal substance against S. furcifera eggs. Bioscience. Biotechnol. Biochem. 78, 937–942. doi: 10.1080/09168451.2014.917266
Yang, Y., Xu, J., Leng, Y., Xiong, G., Hu, J., Zhang, G., et al. (2014). Quantitative trait loci identification, fine mapping and gene expression profiling for ovicidal response to whitebacked planthopper (Sogatella furcifera Horvath) in rice (Oryza sativa L.). BMC Plant Biol. 14:145. doi: 10.1186/1471-2229-14-145
Keywords: Pieris, cabbage, cell death, plant-insect interaction, oviposition-induced response, egg-associated molecular pattern
Citation: Caarls L, Bassetti N, Verbaarschot P, Mumm R, van Loon JJA, Schranz ME and Fatouros NE (2023) Hypersensitive-like response in Brassica plants is specifically induced by molecules from egg-associated secretions of cabbage white butterflies. Front. Ecol. Evol. 10:1070859. doi: 10.3389/fevo.2022.1070859
Received: 15 October 2022; Accepted: 19 December 2022;
Published: 01 February 2023.
Edited by:
Maria L. Pappas, Democritus University of Thrace, GreeceReviewed by:
Junji Takabayashi, Kyoto University, JapanCopyright © 2023 Caarls, Bassetti, Verbaarschot, Mumm, van Loon, Schranz and Fatouros. This is an open-access article distributed under the terms of the Creative Commons Attribution License (CC BY). The use, distribution or reproduction in other forums is permitted, provided the original author(s) and the copyright owner(s) are credited and that the original publication in this journal is cited, in accordance with accepted academic practice. No use, distribution or reproduction is permitted which does not comply with these terms.
*Correspondence: Lotte Caarls, bG90dGUuY2FhcmxzQHd1ci5ubA==; Nina E. Fatouros,
bmluYS5mYXRvdXJvc0B3dXIubmw=
†Present address: Lotte Caarls, Plant Breeding, Wageningen University and Research, Wageningen, Netherlands
Disclaimer: All claims expressed in this article are solely those of the authors and do not necessarily represent those of their affiliated organizations, or those of the publisher, the editors and the reviewers. Any product that may be evaluated in this article or claim that may be made by its manufacturer is not guaranteed or endorsed by the publisher.
Research integrity at Frontiers
Learn more about the work of our research integrity team to safeguard the quality of each article we publish.