- 1Department of Public Health, Experimental and Forensic Medicine, University of Pavia, Pavia, Italy
- 2CRIAMS-Sport Medicine Centre Voghera, University of Pavia, Pavia, Italy
- 3Department of Sport Science, Medical Section, University of Innsbruck, Innsbruck, Austria
One may ask if the transition to bipedalism from the condition of quadrupedalism, which occurred about 7 million years ago, has been the cause or consequence of a series of fundamental physiological muscular aspects including the cost of locomotion, a crucial determinant of endurance, which has been found to be significantly lower in humans than in apes. This issue strictly links to unsolved issues regarding the significance of several muscular structural and functional adaptations, classically attributed to bipedalism and its associated locomotions, which we cannot simply attribute to the acquisition of the upright position and which may directly or indirectly contribute to the observed changes in muscle energetics that make the modern human an exceptional endurance walker and runner compared to quadrupedals.
Economy of locomotion, the potentially selective force for the development of bipedalism in hominins?
Bipedal walking evolved as a unique feature in the early hominins, very likely soon after diverging of chimpanzee and human lineages (Bramble and Lieberman, 2004; Zollikofer et al., 2005). It was long hypothesized that bipedalism is associated with the advantage of reduced walking energetics when compared to other ape-like ancestors (Rodman and McHenry, 1980). Beside others, Sockol and colleagues tested this hypothesis by comparing locomotor energetics in humans and adult chimpanzees and attempted to establish links between energy costs, anatomy and gait, supported by concomitant recordings of metabolic, kinematic, and kinetic data (Sockol et al., 2007).
Actually, these authors demonstrated greater mass-specific cost of transport (as expected for body size) for chimpanzees compared to humans; energy costs of human walking only amounted to about 75% of chimpanzee locomotion (Sockol et al., 2007). The cost of transport was measured as oxygen consumption per kg of body mass per meter of locomotion (VO2/kg/m), and cost of locomotion as oxygen consumption per kg of body mass per second (VO2/kg/s). Cost of locomotion was considered as a function of contact time (the duration of foot-ground contact for one step) and the volume of muscle activated per unit of ground force during locomotion (Roberts et al., 1998). The (mass-specific) cost of locomotion increased with walking speed, linearly in chimpanzees and curvilinearly in humans (Sockol et al., 2007; Pontzer et al., 2014). In contrast, the cost of transport in humans varied considerably with the walking speed (U-shaped relation), reaching an optimum (minimum energy costs) at a pace of about 1.3 m/s (about 4.7 km/h), while a slight linear increase was observed in chimpanzee when quadrupedally walking, with an about 2-fold energy expenditure compared to humans at the speed of 1.3 m/s (Pontzer, 2017).
Low energy cost of walking (Figure 1A) results from the “inverted pendulum type” of moving (Figure 1B), that diminishes muscle-force and work demands during single support; energy costs are primarily resulting from the redirection of the center of body mass in the transition between steps (Kuo et al., 2005). When bipedal walking of chimpanzees was compared with human walking, the latter activated smaller muscle volumes per unit of ground force and used longer contact times than chimpanzees (Sockol et al., 2007). When human (bipedal) walking was compared with quadrupedal chimpanzees, chimpanzees had slightly longer contact times but activated much more muscle per unit of ground force than humans, explaining the lower energy costs for locomotion in humans (Sockol et al., 2007). The shorter contact times and increased ground reaction force (during bipedal walking) are consequences of the shorter hind limbs of chimpanzees compared to humans (Hoyt et al., 2000). Thus, increased bipedal locomotion cost in chimpanzee are resulting from the generation of larger ground forces at a faster rate (Pontzer, 2007). In contrast to chimpanzee, the upright posture of the walking human decreases the volume of muscle activated per unit of ground force because the ground reaction force vector is closer situated to the hip and knee joints (Pontzer, 2007; Sockol et al., 2007). Provided that locomotor economy represents a selective force to develop bipedalism in early hominins, one might expect that their lower limbs should be longer and the ischia of their pelvis more dorsally projecting (Sockol et al., 2007). However, findings from australopithecines suggest that they walked with greater knee and hip flexion (associated with larger energy costs) compared to modern humans (Susman et al., 1984). Thus, it remains to be established whether the energetic advantage related to bipedalism was key in the evolution of hominins.
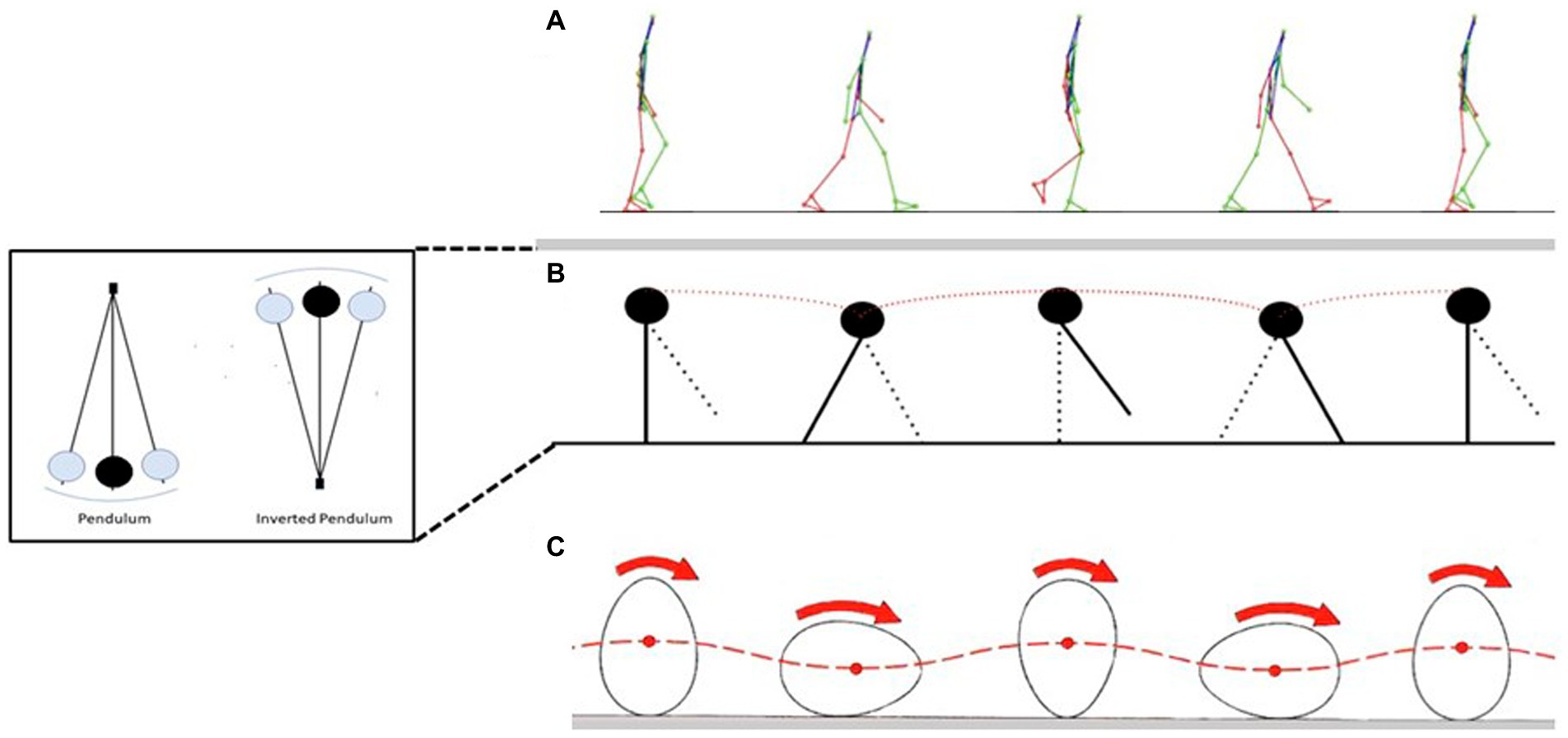
Figure 1. Gait cycle (A) depiction of the gait cycle from the single support of one foot to the next one. In the middle you can see: double support (second figure), single contralateral support and double support; when one limb is in single support, the other is in the swing phase. (B) “The inverted pendulum” the locomotor paradigm of the gait. The center of mass moves on arcs of circumference during the ground support phase as would a pendulum with a fulcrum on the floor (inset). In walking, the fulcrum on the ground is given by the foot, while the bar on which the center of mass is attached is the lower limb. The center of mass is at the apex of the trajectory during single support (the lower limb is extended and perpendicular to the ground), at the lower end during double support. (C) “The egg that rolls” the first paradigm used to describe the gait cycle. It can be seen how the center of mass of the egg moves from a higher to a lower position as the egg rotates [modified from Pavei (2019), with permission].
Apes like chimpanzees can sprint rapidly but perform poor in endurance running (defined as running at a given speed for a prolonged time period, e.g., 1 h) compared to humans, whose endurance running capability range from 2.3 in recreational to 6.5 m/s in elite runners (Cavanagh and Kram, 1989). Only few scientists have considered running as a mode of locomotion that could have influenced the evolution of humans (Bramble and Lieberman, 2004).
As aerobic running performance primarily depends on the individual peak oxygen uptake (VO2peak) during maximal running, endurance capabilities between different species are compared by the use of the running speed at VO2peak (Weibel et al., 2004). This measure depends on the volume of exercising muscles, which is lower in bipedalism (i.e., when forelimbs are removed) than quadrupedalism. Due to the larger hind limb muscles and similar running economy in humans compared to chimpanzees, humans have higher VO2peak speed values (Richard Taylor et al., 1981; Pontzer, 2017).
The economy of running does not much differ between humans and chimpanzees, because energy expenditure increases due to more flexed knees and hips when humans switch from walking to running at about 2.3–2.5 m/s (Usherwood, 2013; Pontzer et al., 2014). At these speeds, running becomes more economical than walking because tendons and ligaments store elastic energy during the braking part of the stance phase, which is followed by energy release through recoil during the subsequent propulsive phase (mass-spring mechanism; Cavagna et al., 1976). This mechanism has little effect on energy savings during walking, but may save up to 50% of the metabolic cost when running (Alexander, 1991). Walking at an optimal speed is much more economical than running, but the energy expenditure remains stable across a broad range of speeds during aerobic running (Bramble and Lieberman, 2004).
Sustained running is associated with extreme mechanical and thermoregulatory challenges, which considerably exceed those during prolonged walking. Thus, adaptations like expanded joint surfaces in the spine, hip, and legs, combined with effective cooling mechanisms (e.g., sweating) are essential for the toleration of those challenges (Bramble and Lieberman, 2004). Moreover, characteristics like shortening of forearms and decoupling of the head and the pectoral girdle, have contributed to the extraordinary endurance running capabilities of humans (Bramble and Lieberman, 2004). Nevertheless, the relevance of endurance running in the evolution of Homo remains to be elucidated.
Bipedalism and muscle evolution: A complex interaction
The gradual emergence of bipedalism represents a fundamental turning point in understanding the functional anatomy of modern man. It has been known since Darwin’s time that bipedalism was already a distinctive element of the hominid family, from which Homo Sapiens originated (Darwin, 1859). It is therefore not surprising that in the early hominids, the presence of bipedalism of sorts, although incomplete and not exclusive, suggests a musculoskeletal reorganization was needed to acquire the upright position and for locomotion.
Although studying adaptations to bipedalism in ancient and generally partial and incomplete skeletal finds is complex, palaeoanthropology also uses the comparative skeletal anatomy between the human being and the closest living ascendants. In particular, the model commonly used for this purpose includes the great apes, who are members of the subfamily Homininae. In this context, the Panini tribes include the four species of great apes: the western gorillas (Gorilla gorilla), eastern gorillas (Gorilla beringei), chimpanzees (Pan troglodytes), and bonobos (Pan paniscus; Roskov et al., 2015). From the comparison of the skeletal segments, it emerges that a series of elements of anatomical specialization accompanied the acquisition of the upright position being its cause and consequence simultaneously. The adaptations of the skull and the postcranial elements are thus distinguished (Mounier, 2018). In this comparative analysis, the most critical anatomical cranial feature directly related to bipedalism involves the spatial position of the foramen magnum, namely its allocation in the context of the occipital bone, and the fact that, physiologically, the foramen is the element of continuity between the cranial cavity and the vertebral canal both in the bipedal and in the quadruped.
In Homo sapiens, the reciprocal spatial relationship between the foramen magnum and the spine defines how the acquisition of the upright position has determined a different allocation compared to the ascending primates with the primary purpose of maintaining in axis the cranial and the spinal content. Thus, the foramen is found in a lower position, and the cerebrum is superior to the spine in the Homo sapiens. At the same time, it maintains a posterior position in the primates in which the encephalon is anteriorized. For the anatomic-topographical evaluation of the layout of the foramen in the context of the skull, an index of great importance is the measurement of the angle between the foramen magnum and the orbital plane. This angle is more significant in Homo sapiens and the ancestor Australopithecus than, for example, that found in the Pan troglodytes (Diogo et al., 2017).
The position of the foramen magnum is a conditioning factor in the anchoring of the neck muscles such as the longissimus capitis inserted proximally in the posterior margin of the mastoid process and temporal bone and the semispinalis capitis inserted proximally between the nuchal lines of the occipital bone, both resulting in a lower anchorage in man compared to the monkey (Table 1). Even the post-cranial skeleton carries sign of the passage to the bipedalism as evidenced by the presence of the vertebral column curvatures already in the Australopithecus and hominid Sahelanthropus tchadensis (Zollikofer et al., 2005).

Table 1. Some distinctive anatomical features of the skull in apes and humans to be considered in the process of evolution to bipedalism.
We need to emphasize that for both the development and its correlation to the upright position, the absent at birth, secondary curvatures of the spine, namely the cervical and lumbar are associated, from a temporal point of view to the walking of the infant and, therefore, to the action of the gravitational load in conditions of bipedalism as well as to a consequence of the pelvic extension at birth. This observation supports the concept that the evolution toward bipedalism was highlighted by the development of lumbar lordosis, which represents a clear phenotypic expression of the gravitational load of the lumbosacral tract in an upright position (Sparrey et al., 2014).
Further confirmation would seem to lie in the results reported in that series of studies where small quadruped mammals are forced into an upright position (Yamada, 1962). In general, this forced change produces a process of adaptation with the appearance of a certain degree of lumbar lordosis. This evidence, of course, does not represent the proof of the causal element of lumbar lordosis in man (Reichmann and Lewin, 1971), where other concomitant mechanisms may also be assumed, especially concerning some evidence of the very early onset of curvature at birth that is not compatible with neither a process of albeit rapid adaptation due to bipedalism and walking nor the fascinating evidence of a process of adaptation in the newborn kept for a long time in the supine decubitus.
From this point of view, a hypothesis of great interest is that it is the delivery itself to contribute to the formation of lumbar lordosis also as a result of the temporal term of the condition of forced kyphosis in utero. The anatomical organization of the pelvis also reflects a different posture and locomotion in the bipedal and quadruped. The adaptation process has produced a superior-inferiorly shortened and anterolaterally enlarged pelvis, easily distinguishable from the superior-inferiorly particularly elongated pelvis of the monkey, which is associated with a lower bicondylar angle compared to that of humans. Another feature can be added to these distinctive characteristics and that is the presence in humans of a wider birth canal as a consequence of the volumetric expansion of the brain during the evolutionary process. Interestingly the pelvis of the Australopithecus (Lucy) has no intermediate characteristics between those observables in the great apes and those featured in the Homo Sapiens as if to consolidate the doubts on the Lamarckian theory of evolution. Lucy’s pelvis appears, in fact, further enlarged latero-laterally and associated with a greater bicondylar angle: both of these anatomical features suggest perfect bipedalism, although different from the human one (Tague and Lovejoy, 1986).
The distinctive features of the pelvis of a biped reflect the mechanical characteristics of locomotion, which are well distinguishable in the quadruped (for review: Special Issue, 2017). Terrestrial locomotion requires generating a reaction force with the ground in the opposite direction of the movement. This process follows that of extending the limbs between the ground and the mass center of the individual. The extension with elongation of the limb determines a reaction to the ground that pushes the torso in the direction produced by the angle between the limb and the ground. In quadrupedal primates, the center of gravity is anterior to the hind limbs, which determines a significant horizontal component of the reaction to the ground.
Since the knee and hip joints are remarkably flexed at the start of the gait cycle, the extension of both is prolonged and powerful and efficiently moves the center of mass with minimal vertical displacement (D’Août et al., 2001, 2004). In bipedalism, the center of mass assumes a higher position than the breech stance. The moment you begin to walk, the knee extension and ankle rotation produce a reaction to the ground with a vertical component which leads us to conclude the gait cycle on the tip of the foot. To advance, we must then move the center of mass in front of the leg. Therefore, the posterior limb is elongated to react to the ground while the contralateral oscillates anteriorly to avoid the fall of the trunk.
In contrast to the walking of the quadruped, in the biped, the horizontal component of the reaction to the ground is limited because it is mostly directed vertically and also because the driving limb is already close to the extension limit because of the practically extended hip joint and that of the knee almost extended to its maximum (Cavagna, 2017). This condition determines a marked displacement of the center of mass that can be described with the model of the “egg rolling” (Figure 1C) or the “inverted pendulum” (Figure 1B). In the latter, the center of mass moves on arcs of circumference exactly like a pendulum with a fulcrum on the floor, at the apex of the trajectory described during the single support and at the minimum during the double support. From a biomechanical point of view, therefore, bipedal locomotion can be synthesized in some substantial elements such as the presence of adduct and extended lower limbs, a minimal pelvic rotational component, and a vertically oriented trunk and a vertical reaction to the biphasic ground (heel and toe).
Conversely, in quadrupedal locomotion, the lower limbs will be flexed and abducted, the pelvic rotational component will be evident, the trunk will retain a front tilt, and the ground reaction force will have a monophasic vertical component (D’Aout et al., 2004; Finestone et al., 2018). Considering these apparent differences, some recent experimental works of great interest studied the human step’s biomechanics while maintaining the trunk’s bending and comparing it to the bipedal one of the chimpanzee. The most recent results in this sense show that the bending of the trunk determines the maintenance of some biomechanical elements typical of the human species, such as the limited pelvic rotation on the transverse plane, while others, such as the angular pelvic variations on the sagittal plane, recall the bipedalism of the monkey (Johnson et al., 2022).
If, therefore, the conclusion is that the anatomic-skeletal features and the pelvis biomechanics reflect the mechanics of bipedal and quadrupedal locomotion, one may wonder whether the evolution of the pelvic muscles follows directly the biomechanical needs imposed by the passage in an upright position. From this point of view, the gluteus muscle and, in particular, the gluteus maximus represents a paradigmatic example that requires us to evaluate the morpho-functional modifications of the muscles beyond bipedalism per se in the context of the functional activities associated with it.
In this sense, the first step in attempting an interpretation is to draw on the anatomical comparison between man and quadrupedal primates. In the evolutionary process, there have been remarkable changes related to muscle size, patterns of origin, and insertion of the gluteus muscle. In monkeys, this muscle has two distinct compartments for origin and insertion. The cranial part, called gluteus maximum proprius, originates from the gluteal aponeurosis, the sacroiliac ligament, the sacrum’s dorsal face and the upper part of the coccyx and inserts into the iliotibial tract. The caudal part, called gluteus ischiofemoralis, is the most represented and originates from the ischial tuberosity to insert on the lateral face of the femur up to the lateral condyle. The evolutionary process has led to the disappearance of the gluteus ischiofemoralis and a significant enlargement of the proprius muscle. Anatomical studies show that the muscle weight/body weight ratio is significantly higher in humans than in monkeys (about 1.6 times). That muscle mass constitutes about 18% of that associated with the pelvis (in the monkey, between 11.7% and 13.3%; Lieberman et al., 2006).
Since the time of Cuvier (1835) (Cuvier et al., 1846), anatomists have speculated on the role of the gluteus maximum in maintaining the upright position. In this sense, several studies have investigated the function of stabilizing the hip and controlling torso flexion relative to the support leg during walking. These speculations have proved largely false in several respects. In fact, the involvement of the buttock in the upright position and the path has been denied mainly by a set of electromyographic studies that have demonstrated almost zero activation level in these conditions, especially in the locomotion in the plane. If, therefore, the morpho-structural evolution of this muscle does not seem directly linked to the assumption of bipedalism, one can ask what the functional demand that imposed such a significant anatomical change is. Electromyographic studies have shown an increasing level of activation of the gluteus maximum when taking a step with a flexed hip and a flexed knee (Joseph and Williams, 1957; Zimmermann et al., 1994), which is a biomechanical condition, that takes place in climbing and stepping or as a result of flexion of the torso (Kozma et al., 2018). It, therefore, seems that from an evolutionary point of view, the morphological reorganization may correlate with the need to maintain non-locomotive behaviors such as climbing and the need to stabilize the trunk in flexion in the performance of daily activities like standing from a sitting position or walking uphill, especially in unstable conditions, and in the performance of activities with a flexed trunk. This change, together with others, may have contributed to the overall reduction of the energy cost of locomotion at least during walking.
The correlated locomotor behaviors such as running are also fundamental (Lieberman et al., 2006). When running, a bending of the trunk of about 10 degrees is maintained, during which there is a high level of activation of the muscle, especially in its cranial part, both in the final phase of the swing and the initial part of the stance, emphasizing a role of the gluteus maximum in the stabilization of the trunk that tends to flex anteriorly in these phases.
The strange case of the gluteus muscle shows that a fundamental approach for the knowledge of the adaptive process to the upright position must pass through both the anatomical and functional skeletal and muscular study, with the analysis of the contribution also in non-walking behaviors that have probably produced, a secondary adaptive process when bipedalism has been acquired.
So, recent and fascinating acquisitions highlight how muscular elements such as fibularis tertius (Olewnik, 2019), classically attributed to the function of walking in an upright position because it is responsible for a movement of eversion, dorsiflexion and lifting of the foot, plus is active during the swing phase of the gait, are also found in primates with a prevalent quadrupedal gait, emphasizing that a simplistic approach to the issue is not sufficient to explain the anatomy and muscle function and energetics in modern man (Diogo et al., 2017).
Data availability statement
The original contributions presented in the study are included in the article/supplementary material, further inquiries can be directed to the corresponding author.
Author contributions
GD’A and MB: drafting and critical revision. All authors contributed to the article and approved the submitted version.
Conflict of interest
The authors declare that the research was conducted in the absence of any commercial or financial relationships that could be construed as a potential conflict of interest.
Publisher’s note
All claims expressed in this article are solely those of the authors and do not necessarily represent those of their affiliated organizations, or those of the publisher, the editors and the reviewers. Any product that may be evaluated in this article, or claim that may be made by its manufacturer, is not guaranteed or endorsed by the publisher.
References
Alexander, R. M. (1991). Energy-saving mechanisms in walking and running. J. Exp. Biol. 160, 55–69. doi: 10.1242/jeb.160.1.55
Bramble, D. M., and Lieberman, D. E. (2004). Endurance running and the evolution of homo. Nature 432, 345–352. doi: 10.1038/nature03052
Cavagna, G., (2017). Physiological Aspects of Legged Terrestrial Locomotion. Springer International Publishing, Cham.
Cavagna, G. A., Thys, H., and Zamboni, A. (1976). The sources of external work in level walking and running. J. Physiol. 262, 639–657. doi: 10.1113/jphysiol.1976.sp011613
Cavanagh, P. R., and Kram, R. (1989). Stride length in distance running: velocity, body dimensions, and added mass effects. Med. Sci. Sports Exerc. 21, 467–479.
Cuvier, G. B., Duméril, C., and Duvernoy, G. L., (1846). Leçons d’anatomie comparée de G. Cuvier. Crochard et Cie, Paris.
D’Août, K., Aerts, P., de Clercq, D., Schoonaert, K., Vereecke, E., and van Elsacker, L. (2001). Studying bonobo (pan paniscus) locomotion using an integrated setup in a zoo environment: preliminary results. Primatologie 4, 191–206.
D’Aout, K., Vereecke, E., Schoonaert, K., de Clercq, D., van Elsacker, L., and Aerts, P. (2004). Locomotion in bonobos (pan paniscus): differences and similarities between bipedal and quadrupedal terrestrial walking, and a comparison with other locomotor modes. J. Anat. 204, 353–361. doi: 10.1111/j.0021-8782.2004.00292.x
Diogo, R., Potau, J. M., Pastor, J. F., de Paz, F. J., Arias-Martorell, J., Turcotte, C., et al. (2017). Photographic and Descriptive Musculoskeletal Atlas of Bonobos, with Notes on the Attachments, Variations, Innervation, Synonymy and Weight of the Muscles. New York, NY: Springer.
Finestone, E. M., Brown, M. H., Ross, S. R., and Pontzer, H. (2018). Great ape walking kinematics: implications for hominoid evolution. Am. J. Phys. Anthropol. 166, 43–55. doi: 10.1002/ajpa.23397
Hoyt, D. F., Wickler, S. J., and Cogger, E. A. (2000). Time of contact and step length: the effect of limb length, running speed, load carrying and incline. J. Exp. Biol. 203, 221–227. doi: 10.1242/jeb.203.2.221
Johnson, R. T., O’Neill, M. C., and Umberger, B. R. (2022). The effects of posture on the three-dimensional gait mechanics of human walking in comparison with walking in bipedal chimpanzees. J. Exp. Biol. 225:eb243272. doi: 10.1242/jeb.243272
Joseph, J., and Williams, P. L. (1957). Electromyography of certain hip muscles. J. Anat. 91, 286–294.
Kozma, E. E., Webb, N. M., Harcourt-Smith, W. E. H., Raichlen, D. A., D’Août, K., Brown, M. H., et al. (2018). Hip extensor mechanics and the evolution of walking and climbing capabilities in humans, apes, and fossil hominins. Proc. Natl. Acad. Sci. U. S. A. 115, 4134–4139. doi: 10.1073/pnas.1715120115
Kuo, A. D., Donelan, J. M., and Ruina, A. (2005). Energetic consequences of walking like an inverted pendulum: step-to-step transitions. Exerc. Sport Sci. Rev. 33, 88–97. doi: 10.1097/00003677-200504000-00006
Lieberman, D. E., Raichlen, D. A., Pontzer, H., Bramble, D. M., and Cutright-Smith, E. (2006). The human gluteus maximus and its role in running. J. Exp. Biol. 209, 2143–2155. doi: 10.1242/jeb.02255
Mounier, A. (2018). “Anatomy, evolution of human,” in The International Encyclopedia of Anthropology. ed. H. Callan (Hoboken, New Jersey (USA): Wiley), 1–14.
Olewnik, Ł. (2019). Fibularis Tertius: anatomical study and review of the literature. Clin. Anat. 32, 1082–1093. doi: 10.1002/ca.23449
Pavei, G. (2019). “Biomechanics of walking, running, swimming and cycling,” in Physical Activity: Physiology, Adaptations to Exercise, Prevention, Sports Therapy and Nutrition. ed. G. Dantona (Gudo Visconti (MI): Poletto), 216.
Pontzer, H. (2007). Predicting the energy cost of terrestrial locomotion: a test of the LiMb model in humans and quadrupeds. J. Exp. Biol. 210, 484–494. doi: 10.1242/jeb.02662
Pontzer, H. (2017). Economy and endurance in human evolution. Curr. Biol. 27, R613–R621. doi: 10.1016/j.cub.2017.05.031
Pontzer, H., Raichlen, D. A., and Rodman, P. S. (2014). Bipedal and quadrupedal locomotion in chimpanzees. J. Hum. Evol. 66, 64–82. doi: 10.1016/j.jhevol.2013.10.002
Reichmann, S., and Lewin, T. (1971). The development of the lumbar lordosis. Archiv Orthopedische Unfall-Chirurgie 69, 275–285. doi: 10.1007/BF00416265
Richard Taylor, C., Maloiy, G. M. O., Weibel, E. R., Langman, V. A., Kamau, J. M. Z., Seeherman, H. J., et al. (1981). Design of the mammalian respiratory system. III. Scaling maximum aerobic capacity to body mass: wild and domestic mammals. Respir. Physiol. 44, 25–37. doi: 10.1016/0034-5687(81)90075-X
Roberts, T. J., Chen, M. S., and Taylor, C. R. (1998). Energetics of bipedal running. II. Limb design and running mechanics. J. Exp. Biol. 201, 2753–2762. doi: 10.1242/jeb.201.19.2753
Rodman, P. S., and McHenry, H. M. (1980). Bioenergetics and the origin of hominid bipedalism. Am. J. Phys. Anthropol. 52, 103–106. doi: 10.1002/ajpa.1330520113
Roskov, Y., Abucay, L., Orrell, T., Nicolson, D., Kunze, T., Culham, A., et al. (eds.) (2015). Species 2000 & ITIS Catalogue of Life, 2015 Annual Checklist. Species 2000: Naturalis, Leiden, the Netherlands.
Sockol, M. D., Raichlen, D. A., and Pontzer, H. (2007). Chimpanzee locomotor energetics and the origin of human bipedalism. Proc. Natl. Acad. Sci. U. S. A. 104, 12265–12269. doi: 10.1073/pnas.0703267104
Sparrey, C. J., Bailey, J. F., Safaee, M., Clark, A. J., Lafage, V., Schwab, F., et al. (2014). Etiology of lumbar lordosis and its pathophysiology: a review of the evolution of lumbar lordosis, and the mechanics and biology of lumbar degeneration. Neurosurg. Focus. 36:E1. doi: 10.3171/2014.1.FOCUS13551
Special Issue (2017). The human pelvis: anatomy, development and function. Anat. Rec. (Hoboken) 300, 615–775.
Susman, R. L., Stern, J. T., and Jungers, W. L. (1984). Arboreality and Bipedality in the Hadar hominids. Folia Primatol. 43, 113–156. doi: 10.1159/000156176
Tague, R. G., and Lovejoy, C. O. (1986). The obstetric pelvis of a.L. 288-1 (Lucy). J. Hum. Evol. 15, 237–255. doi: 10.1016/S0047-2484(86)80052-5
Usherwood, J. R. (2013). Constraints on muscle performance provide a novel explanation for the scaling of posture in terrestrial animals. Biol. Lett. 9:20130414. doi: 10.1098/rsbl.2013.0414
Weibel, E. R., Bacigalupe, L. D., Schmitt, B., and Hoppeler, H. (2004). Allometric scaling of maximal metabolic rate in mammals: muscle aerobic capacity as determinant factor. Respir. Physiol. Neurobiol. 140, 115–132. doi: 10.1016/j.resp.2004.01.006
Yamada, K. (1962). The dynamics of experimental posture. Experimental study of intervertebral disk herniation in bipedal animals. Clin. Orthop. 25, 20–31.
Zimmermann, C. L., Cook, T. M., Bravard, M. S., Hansen, M. M., Honomichl, R. T., Karns, S. T., et al. (1994). Effects of stair-stepping exercise direction and cadence on EMG activity of selected lower extremity muscle groups. J. Orthop. Sports Phys. Ther. 19, 173–180. doi: 10.2519/jospt.1994.19.3.173
Keywords: skeletal muscle, muscle energetics, evolution, walking economy, posture
Citation: D’Antona G and Burtscher M (2022) Muscle endurance: Is bipedalism the cause? Front. Ecol. Evol. 10:1067396. doi: 10.3389/fevo.2022.1067396
Edited by:
Juan Jesus Tena, Spanish National Research Council (CSIC), SpainReviewed by:
Takamitsu Arakawa, Kobe University, JapanCopyright © 2022 D’Antona and Burtscher. This is an open-access article distributed under the terms of the Creative Commons Attribution License (CC BY). The use, distribution or reproduction in other forums is permitted, provided the original author(s) and the copyright owner(s) are credited and that the original publication in this journal is cited, in accordance with accepted academic practice. No use, distribution or reproduction is permitted which does not comply with these terms.
*Correspondence: Giuseppe D’Antona, gdantona@unipv.it