- 1Key Laboratory of Adaptation and Evolution of Plateau Biota, Northwest Institute of Plateau Biology, Chinese Academy of Sciences, Xining, China
- 2Sanjiangyuan Grassland Ecosystem National Observation and Research Station, Xining, China
- 3Research Center for Environment Protection and Water and Soil Conservation, China Academy of Transportation Sciences, Beijing, China
- 4Qinghai Province Key Laboratory of Animal Ecological Genomics, Xining, China
During the last two decades, numerous studies have shown the effects of traffic noise on animal vocal communication. However, studies on the influences of traffic noise on wildlife behavior and physiology are scarce. In the present study, we experimentally manipulated the traffic noise exposure of plateau pika, a native small mammal widely distributed in the alpine meadow of Qinghai-Tibet Plateau, to explore the effects of traffic noise exposure on its behavior and physiology. We showed that noise exposure increased the pika’s exploration and cortisol concentration (CORT) but decreased the resting metabolic rate (RMR). In addition, the relationships between RMR and exploration or CORT appeared under traffic noise treatment. This study suggests that traffic noise plays a large role in the behavior and physiology of plateau pikas and may have a long-term negative effect on the fitness of rodent populations. Generalizing these non-lethal effects to different taxa is crucial for the conservation and management of biodiversity in this increasingly noisy world.
Introduction
From the last century, worldwide human population growth dramatically influenced the Earth through urbanization and industrialization (Sih et al., 2011). Transportation construction developed in an unprecedented scale, which helped us live a more convenient life. Transportation has become a fundamental element of human well-being. Although about 80% of Earth’s terrestrial surface remains roadless, more than half is located in <1 km2 buffer to roads (Ibisch et al., 2016). Simultaneously, traffic brings significant impacts, which are usually negative, on native species and ecosystems; such impacts include natural landscape change (Fahrig and Rytwinski, 2009; Kati et al., 2020), biological invasion (Iacarella et al., 2020), habitat fragmentation (Ware et al., 2015), biodiversity loss (Secco et al., 2022). Following a huge anthropogenic road-building program, road ecology is now increasingly being studied globally (Ibisch et al., 2016). Road and traffic can affect terrestrial animal populations via fragmenting them into smaller sub-populations, which are more vulnerable to local extinction, increasing mortality directly or decreasing habitat amount and quality (Rytwinski and Fahrig, 2015). Around 194 million birds and 29 million mammals may be killed each year on European roads (Grilo et al., 2020); with the increase in traffic density, roadkills consisting of mammals and birds increased in Southern Spain (Canal et al., 2019); road reduced the natural area and space use of jaguars (Panthera onca) and wolf (Canis lupus), which prefer to cross low-speed and low-traffic-volume roads (Cerqueira et al., 2021; Dennehy et al., 2021); forest road can serve as barriers that impede movements and space use of red squirrel (Tamiasciurus hadsonicus grahamensis; Chen and Koprowski, 2016). Traffic also decreases animals’ accessibility to food, mate, and resources, thus reducing the reproductive fitness and threatening population persistence (Fahrig and Rytwinski, 2009; Rytwinski and Fahrig, 2015; Secco et al., 2022). In 2050, a 60% increase of global road length or 25 million kilometers of new-building roadway is predicted compared with that in 2010 (Dulac, 2013). Therefore, road ecology should pay more attention to the effects of traffic on the biodiversity and conservation in the world.
In the last two decades, an increasing number of studies demonstrated that traffic noise has become one of the most important noise pollutions and has significant effects on wildlife (Halfwerk et al., 2011). The impact of traffic noise has been expended to variety of taxa, such as frogs (Troïanowski et al., 2017), bats (Berthinussen and Altringham, 2012), and songbird (Potvin and MacDougall-Shackleton, 2015). Traffic noise has negative effects on animals, i.e., disturbing their acoustic communication, reducing their reproductive success, embryo survival rate, and nestling growth, increasing stress hormone level, and influencing the population maintenance (Crino et al., 2013; Caorsi et al., 2017). Birds show behavioral flexibility under noise and adjust their song features to adapt to the variable-noise environment (Slabbekoorn, 2013). Bischoff’s treefrog (Boana bischoffi) moves away from the noise source and decreases its advertisement call rate and dominant frequency (Caorsi et al., 2017), whereas the European treefrog (Hyla arborea) increases corticosterone levels and decreases immune function under traffic noise (Troïanowski et al., 2017). Shipping noise drives right whale (Eubalaena spp.) to shift their call frequencies, whereas male fin whales (Balaenoptera physalus) modify their song characteristics (Pirotta et al., 2019). However, most studies on noise effects on animals focus on the acoustic communicating species, with approximately two-thirds of such research mentioning songbirds and marine mammals (Shannon et al., 2016); a limited number of studies centered on non-lethal effects of traffic noise on terrestrial mammals (Naidenko et al., 2021), especially on small mammals such as rodents. Rodents represent around 2,277 (42%) of the world’s mammalian species with different life forms (Wilson and Reeder, 2005), and they are widely distributed in variable environments of the Earth. Studying the effects of traffic noise on rodents is crucial for their conservation and management.
The transportation construction has developed rapidly in China. By 2018, more than 5,280,700 km of highways, including 117,000 km of national expressways and 40,000 km of high-speed railways, have been built (Ministry of Transport of China, 2022). In 2035, the lengths of railway and expressway are predicted to reach 70,000 and 160,000 km, respectively (Xinhua News Agency, 2021). Sanjiangyuan National Park is one of the first batch of National Parks and also the largest National Park in China, occupying an area of 190,700 km2. This park is a biodiversity hotspot with more than 270 wild animals; thus, balancing the ecological conservation and livelihood development is a crucial issue in Sanjiangyuan National Park (Cheng et al., 2020). Qinghai-Tibet highway and railway, which cross the Sanjiangyuan National Park, are major human and transportation routes and are thus also extremely busy traffic channels. The streams of traffic produce noise and light pollution along traffic channels. It has demonstrated that both richness and abundances of ground-dwelling birds was decreased near the Qinghai-Tibet highway and railway (Li et al., 2010), and the behaviors of Tibetan antelope were obviously affected by the highway (Ru et al., 2022). Plateau pikas are native small Lagomorphs that are widely distributed in the alpine meadow of Qinghai-Tibet Plateau (Smith and Xie, 2008; Wei et al., 2022). They are regarded as keystone species in the ecosystem of the plateau (Wei et al., 2019), and commonly found near the highway slope (Zhang et al., 2021). Previous studies have demonstrated that the Qinghai-Tibet highway and railway have changed the local rodent community structure (Yang et al., 2006) and restricted plateau pika’s genetic exchange, leading to several genetic differentiations (Zhou et al., 2006). However, the knowledge of how traffic noise affects the plateau pikas is limited. This study aimed to test whether traffic noise affects the plateau pika’s behavioral and physiological traits and their correlations. We predicted that (i) chronic exposure to traffic noise induces stress in plateau pika; (ii) traffic noise increases pika’s exploration and metabolism; (iii) the correlations between behavior and physiology may change under traffic noise.
Materials and methods
Experiment animals
Plateau pikas were live-trapped using the using nooses anchored at burrow entrances with chopsticks (see details in the Qu et al., 2018) at the alpine meadow of Geermu, Qinghai in 10th-12th August 2019, brought back and raised in the animal laboratory at the Northwest Institute of Plateau Biology, Chinese Academy of Sciences. The trap site was about 2.0 km away from the road to ensure that the plateau pikas were not exposed to traffic noise in the natural environment.
Plateau pikas were maintained in separate polypropylene cages (47 cm × 35 cm × 20 cm) under natural light cycle condition, and the ambient temperature was kept 23 ± 1°C. Their gender was tested, and ID information were marked on the cages. The pikas were fed standard rabbit pellet (Beijing Ke Ao Food, Co., China) and pure water ad libitum. They were kept in the laboratory and adapted for 2 weeks. Then, 16 adult males and 12 adult females were used for the following experiment.
Traffic noise preparation and broadcasting
We recorded the traffic noise for playback experiments at Qinghai-Tibet highway (G109) at about 3,002 milestone. Recordings were collected 20 m away from the edge of the paved road at a height of 1 m, where plateau pikas are frequently found, on August 15th, 2019 from 09:00 am to 12:00 am, and lasted for 3 h. A total of 1,430 vehicles were recorded during this period. We selected such date and time because they represented the normal vehicle flux during the season, according to the records of the local department of transportation. A portable sound level meter (DELIXI 2201, 0.1 dB precision) was used to measure the mean amplitude (dB) of the traffic noise, and SONY PCM–D100 recorder was utilized to obtain sounds. From the 2 h recording, a 30 min segment was selected and normalized to a mean amplitude level using Praat v 6.2 software.
Experimental procedure
The behavior and physiology of all pikas were measured at the start of the experiment in early September. Then, the pikas were randomly divided into two treatment groups (“treat” for short), i.e., “Noise” and “Control” groups, and housed in separate but adjoining rooms (18 m2) with the same conditions. In the “Noise” group room, traffic noise was broadcasted. In the “Control” group room, a silent broadcast was conducted during the whole experiment. The treatment lasted for 30 days. At the end of the experiment, the behavior and physiology of all pikas were measured once again using the same procedure.
Traffic noise was broadcasted using an amplified loudspeaker connected to a radio at the center of the “Noise” group room, with all the cages spread around the room to ensure that all the pikas heard the same noise. The noise was loop broadcasted during daytime from 07:00 am to 19:00 pm at 80 ± 2 dB sound pressure level to simulate the natural acoustic environment. Although a certain traffic noise was observed at night in G109, we did not broadcast it in the experiment because pikas are inactive and live underground overnight (Zong and Xia, 1987).
Behavioral and physiological measurement
We conducted behavioral and physiological assays in early September and early October 2019, i.e., “Pro” and “Post” (“trial” for short) the traffic noise treatments, respectively. The behavioral and physiological parameters were tested in the following sequence:
Heart-rate test
A digital voice recorder (Sony PCM-D100) was placed on the left thorax of a pika to record the heart rate. Individuals were held gently by an experimenter, and the heart rate was measured for 30 s by another experimenter. The intervals between two adjacent heartbeats at 12 randomly selected points were detected from each audio file using Praat v 6.2 software. Previous studies demonstrated that this approach is feasible for the heart-rate measurement of plateau pikas (Qu et al., 2018).
Open-field test
After the heart-rate test, pikas were gently transferred to an open-field arena. The arena was a black acrylic box (50 cm × 50 cm × 50 cm) with a white floor. We recorded the behavior exhibited by each pika for 2 min using a digital camera that was fixed above the arena using a tripod. Then, the pika was removed from the arena, and its ID and gender were recorded. After each trial, the arena was cleaned using 75% alcohol and air-dried for the subsequent trial. Using EthoVision XT 9.0 (Noldus Inc., Beijing, China) software, we measured the distances covered by the pika for 2 min as a proxy of exploration (Réale et al., 2007).
Resting metabolic rate (RMR)
We used an 8-channel FMS (Sable Systems International, Henderson, NV, United States) portable respiratory metabolism system to measure the RMR at 27°C using a biochemical incubator. Oxygen consumption per hour (mL O2/[g h]) was expressed as the index of RMR. After the pikas had acclimatized in the chamber for 30 min, the RMR of plateau pikas was measured. Four rounds of metabolism were measured in 120 min, with each round lasting for 30 min. We obtained the average of the lowest consecutive records as the RMR (Zhu et al., 2021).
Fecal cortisol concentration (CORT)
After each test, fresh feces were immediately collected and transferred to a −20°C refrigerator. Fecal CORT was measured using rabbit cortisol enzyme-linked immunosorbent assay kit following the operation manual (Shanghai DUMA Biotechnology Co., Ltd., China). The assay sensitivity was 1.0 ng/ml with the degree of confidence above 99.8%. The intra-and inter-assay coefficients of variance were 10.3 and 6.8%, respectively.
Statistical analysis
We calculated the repeatability of the heart rate, exploration, RMR, and CORT as an intra-class correlation coefficient from one-way ANOVA with treat as a fixed factor using the “rptR” package (Stoffel et al., 2017). Linear mixed-effects models (LMMs) were used to test the potential effect of traffic noise on these behavioral and physiological traits. We assessed the existence of correlations between the heart rate, exploration, RMR, and CORT of each individual from different treatment and trial groups by Spearman rank correlations using the “Hmisc” package. The LMM allows the estimation of the effects of explanatory variables and their interactions as fixed effects, with ID as random effects. LMMs were fitted using the “lmer” package, and “lmerTest” package was used to obtain the summary table and p-values for LMMs via Satterthwaite’s degrees of freedom method (Kuznetsova et al., 2017).
R 4.2.1 software (R Development Core Team, 2022) was used for all statistical analyses.
Ethical statement
The animal study was reviewed and approved by The Ethics Committee of Northwest Institute of Plateau Biology, Chinese Academy of Sciences (NWIPB-20190727). The procedure for capturing, feeding, and operating on the plateau pikas followed the guidelines of the ethical approval standard of the committee. No effect of transport and experiment was observed on pika mortality.
Results
Repeatability and correlations
According to the variance proportions explained by between-individual variations, the behavioral and physiological traits of plateau pikas were repeatable (Table 1). The repeatability of heart rate and RMR showed similar patterns (Radj = 0.333 [0.099, 0.707], Radj = 0.333 [0.120, 0.698], respectively), followed by CORT (Radj = 0.321 [0.025, 0.667]) and exploration (Radj = 0.303 [0.068, 0.673]), which suggest that all behavioral and physiological traits measured were medium repeatable over time. At the start of the experiment, all traits were not significantly correlated with each other in the two groups. However, at the end of the experiment, exploration and CORT were significantly positively correlated, and RMR was significantly negatively correlated with exploration or CORT in the Noise group (p < 0.001; Figure 1A). However, all traits were not significantly correlated with each other in the Control group (Figure 1B).
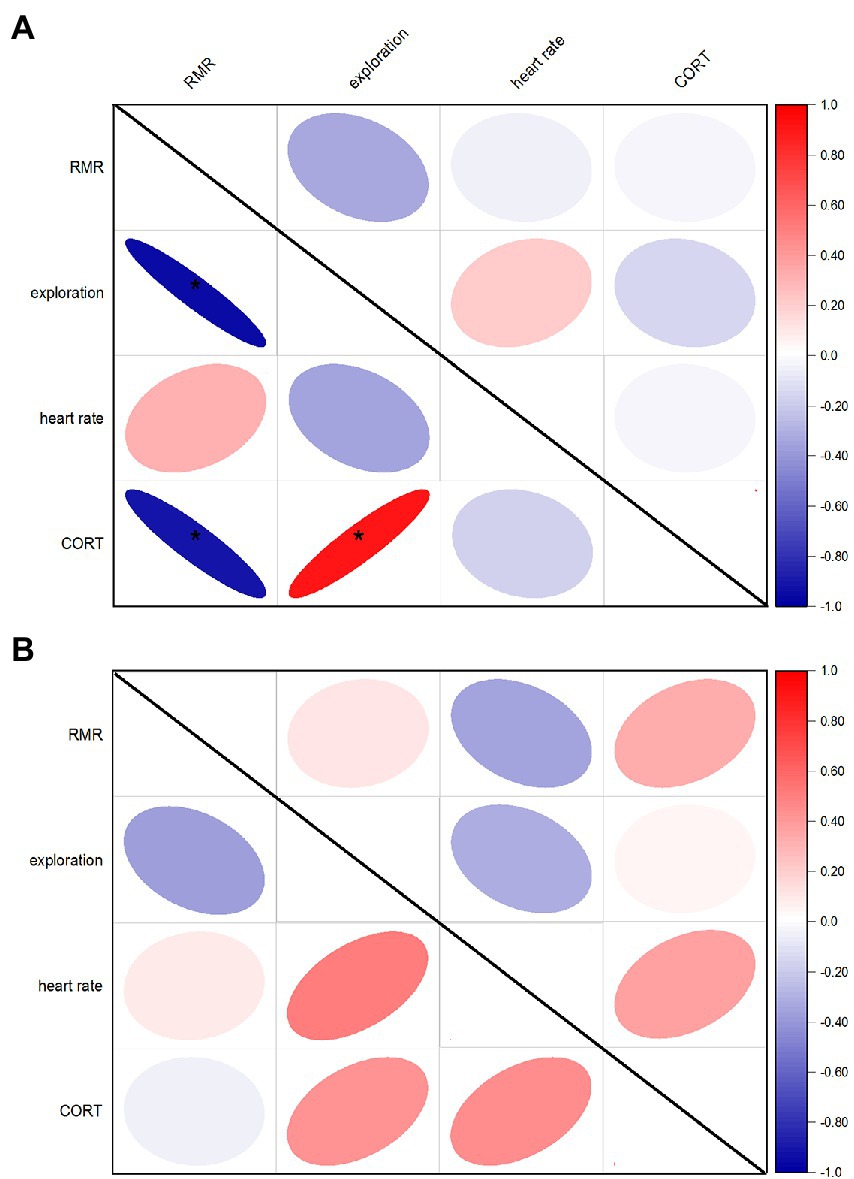
Figure 1. Correlations between behavioral and physiological traits pre and post experiment [(A): Noise; (B): Control]. Upper and under the diagonal indicated pre and post experiments, respectively. *Suggests significant correlations between two traits.
Main and interaction effects
Before the experiments, the heart rates of plateau pikas were in the range of 140.70–413.82 beats per min, with an average of 342.22 ± 2.13 beats per min. At the end of the experiment, the average exploration of the Noise and Control groups changed to 383.60 ± 4.24 and 372.93 ± 2.67 beats per min, respectively. Treatment, trial, and their interaction and gender had no significant effects on heart rate (Figure 2A; Table 2).
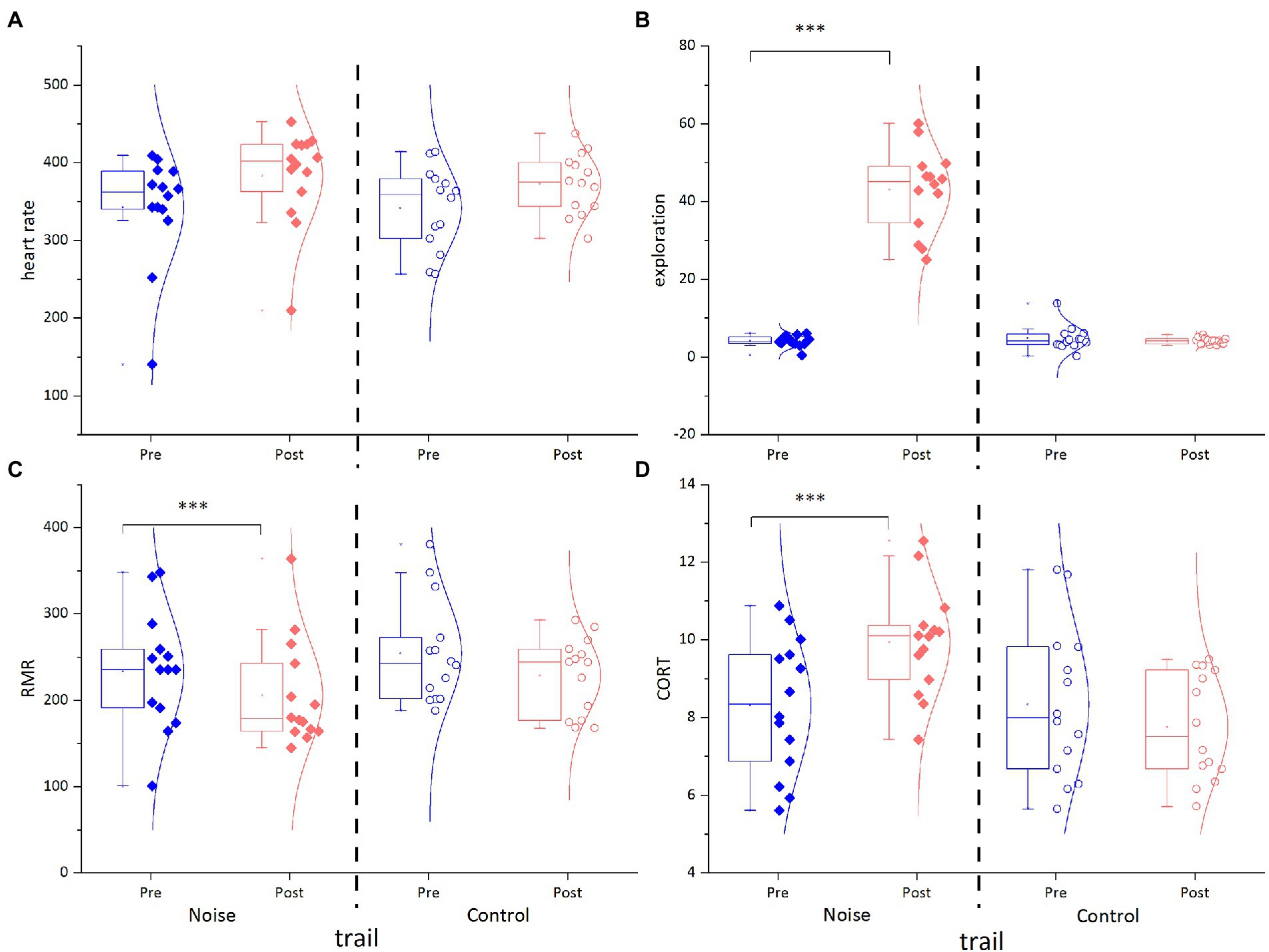
Figure 2. Effect of treatment on behavioral and physiological traits pre and post experiment in the Noise and Control groups. (A) Heart rate under handling, (B) exploration, (C) RMR, and (D) fecal CORT.
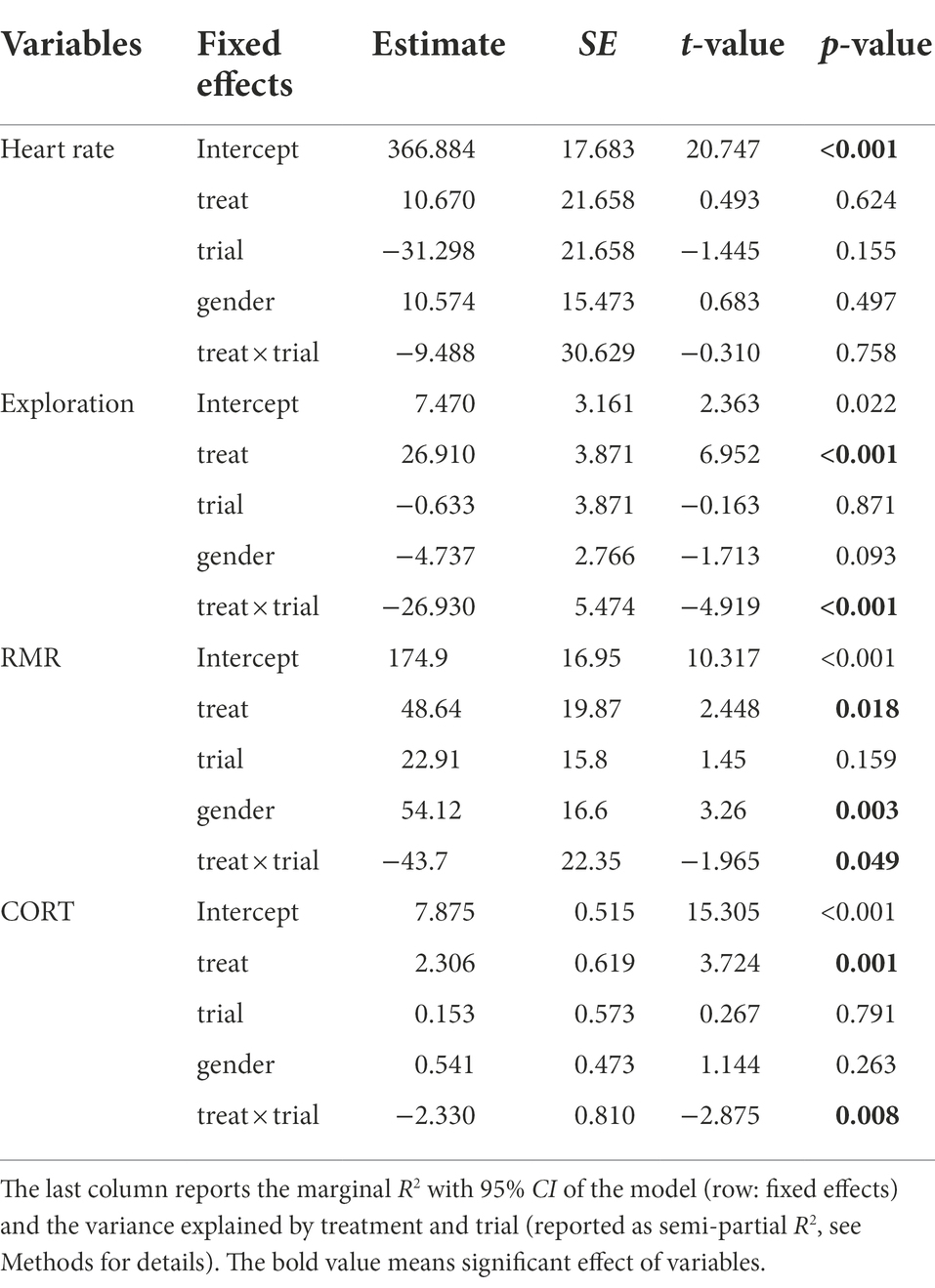
Table 2. Results from the LMM analyses with traffic noise and time as fixed effects and ID as the random effect.
Before the start of experiments, the exploration ranged from 0.50 to 6.09, with an average of 4.44 ± 0.08. By contrast, at the end of the experiment, the average exploration of Noise and Control groups changed to 42.93 ± 0.76 and 4.13 ± 0.06, respectively. The treatment and its interaction with the trial had significant effects on exploration (Figure 2B; Table 2).
Before the experiments, the RMR of plateau pikas ranged from 100.83 ml O2/[g·h] to 348.01 ml O2/[g·h], with the mean of 244.07 ± 2.26 ml O2/[g·h]. At the end of the experiment, the mean RMR of the Noise and Control groups changed to 205.83 ± 4.40 and 228.74 ± 3.17 ml O2/[g·h], respectively. Although the trial had no significant effects on RMR, treatment, gender, and the interaction between treatment and trial had significant effects on RMR (Figure 2C; Table 2).
Similarly, before the start of the experiment, the CORT of plateau pikas ranged from 5.612 ng/mg to 11.799 ng/mg, with a mean of 8.32 ± 0.06 ng/mg. At the end of the experiment, the mean CORT of the Noise and Control groups changed to 9.88 ± 0.06 and 7.76 ± 0.09 ng/mg, respectively. The treatment and its interaction with trial had significant effects on CORT (Figure 2D; Table 2).
Discussion
The present study aimed to examine whether traffic noise has effects on the behavioral and physiological traits of plateau pikas. We observed significant increases in exploration and CORT but a significant decrease in the RMR of plateau pikas. Correlations between several traits were detected after exposure to traffic noise compared with the controls, supporting our prediction that traffic noise constitutes a chronic stressor to rodents. The results of one-month experimental studies focusing on the effects of artificial noise on rodent behavior and physiology (e.g., cortisol) were consistent with those of previous research (Cui et al., 2016, 2018).
Test sequence: Habituation to trial
When wild animals face repeated stimulus, their behavior may be modified, a process called habituation (Rankin et al., 2009). Heart rate and RMR tests involve the capture and handling of subjects, which may elicit significant stress responses (Careau et al., 2008). In this study, although neither behavioral (i.e., exploration) nor physiological traits (heart rate, RMR, and CORT) were singly affected by trial, exploration, RMR, and CORT were interactively affected by trial and traffic noise, suggesting that plateau pikas habituated to the experimental trial, which may weaken the impacts of traffic noise, as shown by the decreases or homogenizations in the exploration, RMR, and CORT of the Control group (Figure 2). In this scenario, we still detected the significant effects of traffic noise on exploration, RMR, and CORT, suggesting the crucial effects of traffic noise on pikas.
Numerous studies have reported the high repeatability estimate for animal behavior over time and/or context environment, which is termed personality (Réale et al., 2010). When animals face external challenges or disturbances, they may reflect behavioral variations, which can be explained through individual consistency and plasticity (Clermont et al., 2019; Couchoux et al., 2021). Using meta-analysis, Bell et al. (2009) observed a mean repeatability of 0.37; our previous study showed that the repeatability of exploration and CORT ranged from 0.34 to 0.63 in the natural population of plateau pikas (Qu et al., 2018). In the present study, the repeatability of exploration, heart rate, RMR, and CORT was around 0.32, indicating that plateau pikas showed lower behavioral and physiological consistency, but higher plasticity under traffic noise stress compared with the natural environment. Plasticity benefits animals to alter their behavior and physiology to better cope with variable environments. For example, urban birds show behavioral plasticity of the acoustic phenotype to increase their survival and reproduction rate during the urbanization process following artificial noise (Slabbekoorn, 2013). A number of insect species show behavioral plasticity in foraging, reproduction, habitat choice, and modified social behavior to cope with artificial rapid environmental changes (van Baaren and Candolin, 2018). This study demonstrated that rodents can change their behavior and physiology to mitigate the potential adverse effects of traffic noise.
Behavioral, physiological variations and correlations
Heart rate under handling indicates the individual physiological index of coping style and reflects the response of the animal autonomic nervous system to predation risk or fearful stimuli rather than disturbance stress (Koolhaas et al., 2010). Our previous studies demonstrated that in the natural population, the heart rate and personality were correlated in plateau pikas, where the predation risk is high (Qu et al., 2018; Wei et al., 2020), as pikas are the primary prey for almost all predators on the Qinghai-Tibetan Plateau (Harris et al., 2014). In this study, the heart rate was consistent at the start and end of the experiment, indicating that plateau pikas maintained a stable response to human handling, regardless of whether they were exposed to traffic noise exposure; thus, artificial traffic noise may have different affecting mechanisms for wildlife compared with predation risk, and animals show variable coping styles to predation and artificial challenges (Francis and Barber, 2013).
As an external stressor, noise can hyperactivate the sympathetic autonomic nervous system and activate the hypothalamic–pituitary–adrenal axis, which increase the organism CORT and finally alter metabolism (Schmidt et al., 2013). Two weeks of noise enhanced the blood glucose and CORT levels and varied the glucose metabolism and gut microbiota composition in rats (Cui et al., 2016). Noise exposure also increased the corticosterone level of male treefrog but decreased their immune function (Troïanowski et al., 2017). Our results showed that at the end of the experiment, the CORT of plateau pikas in the Noise group was significantly higher than that at the start, whereas no significant variations in the CORT pre and post experiment were detected in the Control group. Thus, traffic noise caused evident stress to the pikas, and the significant interactions between treatment and trial on CORT suggested that pikas might have accustomed to the experimental environment over 1 month, which relieved the potential stress from an unfamiliar environment. Exploration reflects an animal’s response to space, and it is associated with anxiety; anxious animals consistently show heightened exploration (Karl et al., 2006). In this study, the increased CORT and exploration suggest that persistent traffic noise is an interference pressure resource to pikas, and it affects their hormone sensitivity and anxiety, finally influencing their body conditions.
Metabolism is a proximate mechanism underlying behavioral and physiological variations (Careau et al., 2008). According to the Pace-of-life Syndrome (POLS) hypothesis, animal life history, behavior, and physiology are correlated, and their coupling is used to cope with external challenges (Réale et al., 2010; Boulton et al., 2015; Houslay et al., 2017). In this study, at the start of the experiment in both groups and at the end of experiment in the Control group, no significant correlations were detected among traits, whereas significant correlations between exploration, RMR, and CORT appeared in the Noise group at the end of the experiment, suggesting that traffic noise promoted the correlations among traits, that is, the occurrence of coping style. Although the presence of personality traits has been found in different external stresses, such as predation risk (Dingemanse et al., 2007, 2009) and artificial harvest (Biro and Post, 2008), we emphasized for the first time a significant relationship among behavior, RMR, and CORT induced by traffic noise.
Two distinct conceptual models explain the relationships between animal metabolism and behavior (Careau et al., 2008). The performance model refers to the situation in which a high metabolic rate requires more active behavioral traits. Thus, exploration, aggressiveness and boldness should positively correlate with energy metabolism. A positive correlation was detected between boldness and metabolism in wild-caught orb-weaving spider (Larinioides cornutus; Shearer and Pruitt, 2014) and fall field crickets (Gryllus pennsylvanicus; Careau et al., 2019). By contrast, the allocation model argues that with individuals having to allocate a fixed amount of energy to maintain their normal metabolism, limited energy is expended in the behaviors associated with activity, exploration, etc. The active mosquitofish (Gambusia holbrooki) has a low RMR, which suggests that high activity rates require individuals to allocate less energy toward maintenance (Biro et al., 2020). In this study, the decrease of RMR, as well as negative relationship between RMR and exploration in the Noise group at the end of experiment, provided evidence for the allocation model of energy management. Under ad libitum food conditions in the laboratory, traffic noise caused trade-offs between RMR and exploration. The relationships between behavior and metabolism are not always stable (Mathot et al., 2019). For instance, the risk-taking behavior (i.e., boldness) and basal metabolic rate of the great tit (Parus major) changed from positive to negative in two consecutive years (Mathot et al., 2015). Under artificial light stimulus, the common toad (Bufo bufo) allocates less energy on activities but more energy on RMR (Touzot et al., 2019). Similarly, given that hormone production is a high-energy-consuming process, the negative relationships between CORT and RMR occurred under noise exposure conditions, which is consistent with the result of Guenther et al. (2014), who observed negative correlations between CORT and RMR in juvenile and mature cavies (Cavia aperea).
Conclusion
This study provided experimental evidence of the effects of chronic noise exposure on the behavior and physiology of wild rodents. Our results indicate that traffic noise exposure altered the exploration, metabolism, and CORT and their correlations in plateau pikas. The behavioral and physiological effects of traffic noise are currently overlooked in rodents, and their ecological consequences on the conservation and management are unclear. Our study showed that they should receive more attention, and traffic noise should be regarded as a potential influencing factor when assessing the effects of transportation on ecosystems. In the future, generalizing the non-lethal effects of traffic noise to different taxa, especially non-flagship or endangered species, is also crucial for the conservation and management of the whole ecosystem in this increasingly noisy world.
Data availability statement
The original contributions presented in the study are included in the article/Supplementary material, further inquiries can be directed to the corresponding author.
Ethics statement
The animal study was reviewed and approved by The Ethics Committee of Northwest Institute of Plateau Biology, Chinese Academy of Sciences (NWIPB-20190727). Written informed consent was obtained from the owners for the participation of their animals in this study.
Author contributions
JQ and YW conceived the study. HZ, YY, and LZ completed the majority of the experiments, and collected samples. JQ wrote the manuscript. YW reviewed the manuscript. All authors contributed to the articles and approved the final manuscript.
Funding
This work was supported by the Second Tibetan Plateau Scientific Expedition and Research Program (STEP; grant no. 2021QZKK0203), Strategic Priority Research Program of Chinese Academy of Sciences (XDA26020201), CAS “Light of West China” for Interdisciplinary Innovation Team, Qinghai Province Natural Sciences Foundation (2021-ZJ-929), and Joint Grant from Chinese Academy of Sciences-People’s Government of Qinghai Province on Sanjiangyuan National Park (LHZX-2020-01).
Acknowledgments
We thank Yourong Lai for his help in the fieldwork. We express our sincere gratitude to editors and reviewers for their time and effort.
Conflict of interest
The authors declare that the research was conducted in the absence of any commercial or financial relationships that could be construed as a potential conflict of interest.
Publisher’s note
All claims expressed in this article are solely those of the authors and do not necessarily represent those of their affiliated organizations, or those of the publisher, the editors and the reviewers. Any product that may be evaluated in this article, or claim that may be made by its manufacturer, is not guaranteed or endorsed by the publisher.
Supplementary material
The Supplementary material for this article can be found online at: https://www.frontiersin.org/articles/10.3389/fevo.2022.1065966/full#supplementary-material
References
Bell, A. M., Hankison, S. J., and Laskowski, K. L. (2009). The repeatability of behaviour: a meta-analysis. Anim. Behav. 77, 771–783. doi: 10.1016/j.anbehav.2008.12.022
Berthinussen, A., and Altringham, J. (2012). The effect of a major road on bat activity and diversity. J. Appl. Ecol. 49, 82–89. doi: 10.1111/j.1365-2664.2011.02068.x
Biro, P. A., and Post, J. R. (2008). Rapid depletion of genotypes with fast growth and bold personality traits from harvested fish populations. Proc. Natl. Acad. Sci. U. S. A. 105, 2919–2922. doi: 10.1073/pnas.0708159105
Biro, P. A., Thomas, F., Ujvari, B., Adriaenssens, B., and Beckmann, C. (2020). Spontaneous activity rates and resting metabolism: support for the allocation model of energy management at the among-individual level. Ethology 126, 32–39. doi: 10.1111/eth.12957
Boulton, K., Couto, E., Grimmer, A. J., Earley, R. L., Canario, A. V. M., Wilson, A. J., et al. (2015). How integrated are behavioral and endocrine stress response traits? A repeated measures approach to testing the stress-coping style model. Ecol. Evol. 5, 618–633. doi: 10.1002/ece3.1395
Canal, D., Camacho, C., Martín, B., de Lucas, M., and Ferrer, M. (2019). Fine-scale determinants of vertebrate roadkills across a biodiversity hotspot in southern Spain. Biodivers. Conserv. 28, 3239–3256. doi: 10.1007/s10531-019-01817-5
Caorsi, V. Z., Both, C., Cechin, S., Antunes, R., and Borges-Martins, M. (2017). Effects of traffic noise on the calling behavior of two Neotropical hylid frogs. PLoS One 12:e0183342. doi: 10.1371/journal.pone.0183342
Careau, V., Beauchamp, P. P., Bouchard, S., and Morand-Ferron, J. (2019). Energy metabolism and personality in wild-caught fall field crickets. Physiol. Behav. 199, 173–181. doi: 10.1016/j.physbeh.2018.11.023
Careau, V., Thomas, D., Humphries, M. M., and Réale, D. (2008). Energy metabolism and animal personality. Oikos 117, 641–653. doi: 10.1111/j.0030-1299.2008.16513.x
Cerqueira, R. C., de Rivera, O. R., Jaeger, J. A. G., and Grilo, C. (2021). Direct and indirect effects of roads on space use by jaguars in Brazil. Sci. Rep. 11:22617. doi: 10.1038/s41598-021-01936-6
Chen, H. L., and Koprowski, J. L. (2016). Barrier effects of roads on an endangered forest obligate: influences of traffic, road edges, and gaps. Biol. Conserv. 199, 33–40. doi: 10.1016/j.biocon.2016.03.017
Cheng, Q., Cheng, X., Ma, K., Zhao, X., and Qu, J. (2020). Offering the win-win solutions between ecological conservation and livelihood development: national parks in Qinghai, China. Geogr. Sustain. 1, 251–255. doi: 10.1016/j.geosus.2020.10.001
Clermont, J., Réale, D., Lindsay, M. È., and Giroux, J. F. (2019). Plasticity, state-dependency, and individual consistency in Canada goose nest defense behavior. Behav. Ecol. Sociobiol. 73:66. doi: 10.1007/s00265-019-2681-0
Couchoux, C., Garant, D., Aubert, M., Clermont, J., and Réale, D. (2021). Behavioral variation in natural contests: integrating plasticity and personality. Behav. Ecol. 32, 277–285. doi: 10.1093/beheco/araa127
Crino, O. L., Johnson, E. E., Blickley, J. L., Patricelli, G. L., and Breuner, C. W. (2013). Effects of experimentally elevated traffic noise on nestling white-crowned sparrow stress physiology, immune function and life history. J. Exp. Biol. 216, 2055–2062. doi: 10.1242/jeb.081109
Cui, B., Gai, Z., She, X., Wang, R., and Xi, Z. (2016). Effects of chronic noise on glucose metabolism and gut microbiota-host inflammatory homeostasis in rats. Sci. Rep. 6:36693. doi: 10.1038/srep36693
Cui, B., Su, D., Li, W., She, X., Zhang, M., Wang, R., et al. (2018). Effects of chronic noise exposure on the microbiome-gut-brain axis in senescence-accelerated prone mice: implications for Alzheimer’s disease. J. Neuroinflammation 15:190. doi: 10.1186/s12974-018-1223-4
Dennehy, E., Llaneza, L., and López-Bao, J. V. (2021). Contrasting wolf responses to different paved roads and traffic volume levels. Biodivers. Conserv. 30, 3133–3150. doi: 10.1007/s10531-021-02239-y
Dingemanse, N. J., Van der Plas, F., Wright, J., Réale, D., Schrama, M., Roff, D. A., et al. (2009). Individual experience and evolutionary history of predation affect expression of heritable variation in fish personality and morphology. Proc. R. Soc. B Biol. Sci. 276, 1285–1293. doi: 10.1098/rspb.2008.1555
Dingemanse, N. J., Wright, J., Kazem, A. J. N., Thomas, D. K., Hickling, R., and Dawnay, N. (2007). Behavioural syndromes differ predictably between 12 populations of three-spined stickleback. J. Anim. Ecol. 76, 1128–1138. doi: 10.1111/j.1365-2656.2007.01284.x
Dulac, J. (2013). Global Land Transport Infrastructure Requirements. Paris: International Energy Agency 20, 2014
Fahrig, L., and Rytwinski, T. (2009). Effects of roads on animal abundance: an empirical review and synthesis. Ecol. Soc. 14:21. doi: 10.5751/ES-02815-140121
Francis, C. D., and Barber, J. R. (2013). A framework for understanding noise impacts on wildlife: an urgent conservation priority. Front. Ecol. Environ. 11, 305–313. doi: 10.1890/120183
Grilo, C., Koroleva, E., Andrášik, R., Bíl, M., and González-Suárez, M. (2020). Roadkill risk and population vulnerability in European birds and mammals. Front. Ecol. Environ. 18, 323–328. doi: 10.1002/fee.2216
Guenther, A., Finkemeier, M. A., and Trillmich, F. (2014). The ontogeny of personality in the wild Guinea pig. Anim. Behav. 90, 131–139. doi: 10.1016/j.anbehav.2014.01.032
Halfwerk, W., Holleman, L. J. M., Lessells, C. M., and Slabbekoorn, H. (2011). Negative impact of traffic noise on avian reproductive success. J. Appl. Ecol. 48, 210–219. doi: 10.1111/j.1365-2664.2010.01914.x
Harris, R. B., Jiake, Z., Yinqiu, J., Kai, Z., Chunyan, Y., and Yu, D. W. (2014). Evidence that the Tibetan fox is an obligate predator of the plateau pika: conservation implications. J. Mammal. 95, 1207–1221. doi: 10.1644/14-MAMM-A-021
Houslay, T. M., Vierbuchen, M., Grimmer, A. J., Young, A. J., and Wilson, A. J. (2017). Testing the stability of behavioural coping style across stress contexts in the Trinidadian guppy. Funct. Ecol. 32, 424–438. doi: 10.1111/1365-2435.12981
Iacarella, J. C., Burke, L., Davidson, I. C., DiBacco, C., Therriault, T. W., and Dunham, A. (2020). Unwanted networks: vessel traffic heightens the risk of invasions in marine protected areas. Biol. Conserv. 245:108553. doi: 10.1016/j.biocon.2020.108553
Ibisch, P. L., Hoffmann, M. T., Kreft, S., Peer, G., Kati, V., Biber-Freudenberger, L., et al. (2016). A global map of roadless areas and their conservation status. Science 354, 1423–1427. doi: 10.1126/science.aaf7166
Karl, T., Burne, T. H. J., and Herzog, H. (2006). Effect of Y1 receptor deficiency on motor activity, exploration, and anxiety. Behav. Brain Res. 167, 87–93. doi: 10.1016/j.bbr.2005.08.019
Kati, V., Kassara, C., Psaralexi, M., Tzortzakaki, O., Petridou, M., Galani, A., et al. (2020). Conservation policy under a roadless perspective: minimizing fragmentation in Greece. Biol. Conserv. 252:108828. doi: 10.1016/j.biocon.2020.108828
Koolhaas, J. M., de Boer, S. F., Coppens, C. M., and Buwalda, B. (2010). Neuroendocrinology of coping styles: towards understanding the biology of individual variation. Front. Neuroendocrinol. 31, 307–321. doi: 10.1016/j.yfrne.2010.04.001
Kuznetsova, A., Brockhoff, P. B., and Christensen, R. H. B. (2017). Lmer test package: tests in linear mixed effects models. J. Stat. Softw. 82, 1–26. doi: 10.18637/jss.v082.i13
Li, Z., Ge, C., Li, J., Li, Y., Xu, A., Zhou, K., et al. (2010). Ground-dwelling birds near the Qinghai-Tibet highway and railway. Transp. Res. Part D: Transp. Environ. 15, 525–528. doi: 10.1016/j.trd.2010.07.004
Mathot, K. J., Dingemanse, N. J., and Nakagawa, S. (2019). The covariance between metabolic rate and behaviour varies across behaviours and thermal types: meta-analytic insights. Biol. Rev. 94, 1056–1074. doi: 10.1111/brv.12491
Mathot, K. J., Nicolaus, M., Araya-Ajoy, Y. G., Dingemanse, N. J., and Kempenaers, B. (2015). Does metabolic rate predict risk-taking behaviour? A field experiment in a wild passerine bird. Funct. Ecol. 29, 239–249. doi: 10.1111/1365-2435.12318
Ministry of Transport of China. (2022). Available at: https://xxgk.mot.gov.cn/2020/jigou/zhghs/202205/t20220524_3656659.html
Naidenko, S., Chistopolova, M., Hernandez-Blanco, J. A., Erofeeva, M., and Rozhnov, V. (2021). The effect of highway on spatial distribution and daily activity of mammals. Transp. Res. Part D: Transp. Environ. 94:102808. doi: 10.1016/j.trd.2021.102808
Pirotta, V., Grech, A., Jonsen, I. D., Laurance, W. F., and Harcourt, R. G. (2019). Consequences of global shipping traffic for marine giants. Front. Ecol. Environ. 17, 39–47. doi: 10.1002/fee.1987
Potvin, D. A., and MacDougall-Shackleton, S. A. (2015). Traffic noise affects embryo mortality and nestling growth rates in captive zebra finches. J. Exp. Zool. A Ecol. Genet. Physiol. 323, 722–730. doi: 10.1002/jez.1965
Qu, J., Fletcher, Q. E., Réale, D., Li, W., and Zhang, Y. (2018). Independence between coping style and stress reactivity in plateau pika. Physiol. Behav. 197, 1–8. doi: 10.1016/j.physbeh.2018.09.007
R Development Core Team (2022). R: A Language and Environment for Statistical Computing. [Computer Software]. R Foundation for Statistical Computing, Vienna, Austria. Available at: http://www.R-project.org
Rankin, C. H., Abrams, T., Barry, R. J., Bhatnagar, S., Clayton, D. F., Colombo, J., et al. (2009). Habituation revisited: an updated and revised description of the behavioral characteristics of habituation. Neurobiol. Learn. Mem. 92, 135–138. doi: 10.1016/j.nlm.2008.09.012
Réale, D., Garant, D., Humphries, M. M., Bergeron, P., Careau, V., and Montiglio, P. O. (2010). Personality and the emergence of the pace-of-life syndrome concept at the population level. Philos. Trans. R. Soc. Lond. B. Biol. Sci. 365, 4051–4063. doi: 10.1098/rstb.2010.0208
Réale, D., Reader, S. M., Sol, D., McDougall, P. T., and Dingemanse, N. J. (2007). Integrating animal temperament within ecology and evolution. Biol. Rev. 82, 291–318. doi: 10.1111/j.1469-185X.2007.00010.x
Ru, H., Xu, J., and Jiang, S. (2022). Experimental observation and analysis of traffic impact on Tibetan antelopes on the Qinghai-Tibet highway. Adv. Civil Eng. 2022, 1–10. doi: 10.1155/2022/1226781
Rytwinski, T., and Fahrig, L. (2015). “The impacts of roads and traffic on terrestrial animal populations,” in Handbook of Road Ecology. eds. R. van der Ree, D. J. Smith and C. Grilo, 237–246.
Schmidt, F. P., Basner, M., Kröger, G., Weck, S., Schnorbus, B., Muttray, A., et al. (2013). Effect of nighttime aircraft noise exposure on endothelial function and stress hormone release in healthy adults. Eur. Heart J. 34, 3508–3514. doi: 10.1093/eurheartj/eht269
Secco, H., da Costa, V. O., Guerreiro, M., and Gonçalves, P. R. (2022). Evaluating impacts of road expansion on porcupines in a biodiversity hotspot. Transp. Res. Part D: Transp. Environ. 102:103151. doi: 10.1016/j.trd.2021.103151
Shannon, G., McKenna, M. F., Angeloni, L. M., Crooks, K. R., Fristrup, K. M., Brown, E., et al. (2016). A synthesis of two decades of research documenting the effects of noise on wildlife. Biol. Rev. 91, 982–1005. doi: 10.1111/brv.12207
Shearer, T. A., and Pruitt, J. N. (2014). Individual differences in boldness positively correlate with heart rate in orb-weaving spiders of genus Larinioides. Curr. Zool. 60, 387–391. doi: 10.1093/czoolo/60.3.387
Sih, A., Ferrari, M. C., and Harris, D. J. (2011). Evolution and behavioural responses to human-induced rapid environmental change. Evol. Appl. 4, 367–387. doi: 10.1111/j.1752-4571.2010.00166.x
Slabbekoorn, H. (2013). Songs of the city: noise-dependent spectral plasticity in the acoustic phenotype of urban birds. Anim. Behav. 85, 1089–1099. doi: 10.1016/j.anbehav.2013.01.021
Smith, A. T., and Xie, Y. (2008). A Guide to the Mammals of China. Princeton, New Jersey: Princeton University Press.
Stoffel, M. A., Nakagawa, S., and Schielzeth, H. (2017). Rpt R: repeatability estimation and variance decomposition by generalized linear mixed-effects models. Methods Ecol. Evol. 8, 1639–1644. doi: 10.1111/2041-210X.12797
Touzot, M., Teulier, L., Lengagne, T., Secondi, J., Théry, M., Libourel, P. A., et al. (2019). Artificial light at night disturbs the activity and energy allocation of the common toad during the breeding period. Conservation. Physiology 7:coz002. doi: 10.1093/conphys/coz002
Troïanowski, M., Mondy, N., Dumet, A., Arcanjo, C., and Lengagne, T. (2017). Effects of traffic noise on tree frog stress levels, immunity, and color signaling. Conserv. Biol. 31, 1132–1140. doi: 10.1111/cobi.12893
Van Baaren, J., and Candolin, U. (2018). Plasticity in a changing world: behavioural responses to human perturbations. Curr. Opin. Insect Sci. 27, 21–25. doi: 10.1016/j.cois.2018.02.003
Ware, H. E., McClure, C. J. W., Carlisle, J. D., and Barber, J. R. (2015). A phantom road experiment reveals traffic noise is an invisible source of habitat degradation. Proc. Natl. Acad. Sci. U. S. A. 112, 12105–12109. doi: 10.1073/pnas.1504710112
Wei, W., Knops, J. M., and Zhang, W. (2019). The impact of plateau pikas (Ochotona curzoniae) on alpine grassland vegetation and soil is not uniform within the home range of pika families. Plant Ecol. Divers. 12, 417–426. doi: 10.1080/17550874.2019.1628113
Wei, W., Zhen, Q., Deng, J., Yue, H., Qin, M., and Oosthuizen, M. (2022). Grazing during the grassland Greenup period promotes plant species richness in alpine grassland in winter pastures. Front. Plant Sci. 13:973662. doi: 10.3389/fpls.2022.973662
Wei, W., Zhen, Q., Tang, Z., and Oosthuizen, M. K. (2020). Risk assessment in the plateau pika (Ochotona curzoniae): intensity of behavioral response differs with predator species. BMC Ecol. 20, 41–48. doi: 10.1186/s12898-020-00309-3
Wilson, D. E., and Reeder, D. M. (2005). Mammal Species of the World: A Taxonomic and Geographic Reference. Baltimore, Maryland: Johns Hopkins University Press.
Xinhua News Agency (2021). Available at: http://www.gov.cn/zhengce/2021-02/24/content_5588654.htm
Yang, S. M., Huai, H. Y., Zhang, Y. L., Yin, B. F., Zhou, L., and Wei, W. H. (2006). Effects of railway traffic on the community structure of rodents in warm steppe along the Qinghai-Tibet railway. Acta Theriol. Sin. 26, 267–273.
Zhang, Y., Gao, Q., Ganjurjav, H., Dong, S., Zheng, Q., Ma, Y., et al. (2021). Grazing exclusion changed the complexity and keystone species of alpine meadows on the Qinghai-Tibetan plateau. Front. Ecol. Evol. 9:638157. doi: 10.3389/fevo.2021.638157
Zhou, L., Yin, B., Yang, S., Huai, H., Li, S., Zhang, Y., et al. (2006). Effects of Qinghai-Tibet highway on genetic differentiation of plateau pika (Ochotona curzoniae). Acta Ecol. Sin. 26, 3572–3577.
Zhu, H. J., Zhong, L., Li, J., Wang, S. Q., and Qu, J. P. (2021). Differential expression of metabolism related genes in plateau pika (Ochotona curzoniae) at different altitudes of Qinghai-Tibet plateau. Front. Genet. 12:2875. doi: 10.3389/fgene.2021.784811
Keywords: behavior, cortisol, metabolism, road ecology, Qinghai-Tibet Plateau, rodent, small mammal, non-lethal effect
Citation: Qu J, Wang Y, Kong Y, Zhu H, Yu Y and Zhong L (2022) Effect of chronic traffic noise on behavior and physiology of plateau pikas (Ochotona curzoniae). Front. Ecol. Evol. 10:1065966. doi: 10.3389/fevo.2022.1065966
Edited by:
Wei Wanrong, China West Normal University, ChinaReviewed by:
Zheng Gang Guo, Lanzhou University, ChinaJunhu Su, Gansu Agricultural University, China
Copyright © 2022 Qu, Wang, Kong, Zhu, Yu and Zhong. This is an open-access article distributed under the terms of the Creative Commons Attribution License (CC BY). The use, distribution or reproduction in other forums is permitted, provided the original author(s) and the copyright owner(s) are credited and that the original publication in this journal is cited, in accordance with accepted academic practice. No use, distribution or reproduction is permitted which does not comply with these terms.
*Correspondence: Jiapeng Qu, jpqu@nwipb.cas.cn; Yun Wang, wangyun80314@163.com