- 1State Key Laboratory of Freshwater Ecology and Biotechnology, Institute of Hydrobiology, Chinese Academy of Sciences, Wuhan, Hubei, China
- 2University of Chinese Academy of Sciences, Beijing, China
- 3Laboratoire Évolution et Diversité Biologique (EDB), Université de Toulouse, Toulouse, France
- 4National Research Centre for Freshwater Fisheries Engineering, Ministry of Sciences and Technology, Wuhan, Hubei, China
Lake littoral zones with abundant submerged vegetation are considered to harbor diverse biotic communities and additionally serve as spawning grounds for important fishery resources. In recent decades, due to various human activities submerged vegetation in lakes have declined dramatically resulting in the function loss of spawning grounds. In order to enhance the recruitment of lacustrine fish (mainly Carassius auratus and Cyprinus carpio) and the proliferation of fishery resources, we explored a method of constructing artificial spawning ground based on artificial spawning substrate in lake. The results of present study indicate that the artificial substrates with complex and dense three-dimensional structure which have enough space to enable material exchange and oxygen circulation could be efficient to enhance fish recruitment. The water temperature of spawning peaks of artificial substrates were within the range of 20 ~ 25°C, and the water temperature provides a basis for the deployment period of artificial spawning grounds. The spawning substrate in the upper layer of water column was more attractive for fish to spawn. The above results provide guidance for the deployment of artificial spawning grounds from both temporal and spatial scales. Observations on the behavior of aggregations found that spawning activities mainly occurred at night and in the early morning. The abundance of larger individuals (>20 cm), as potential spawning groups, increased during this period. The above findings are informative to the construction and management of artificial spawning ground in lakes.
1. Introduction
Submerged vegetation in lake littoral zones is often considered to provide important structure habitat for many invertebrate and fish species (Pawlikowski and Kornijów, 2019; Bolduc et al., 2020). It has been extensively verified that the decline of submerged vegetation is an important cause of loss of aquatic biodiversity and degradation of ecosystem function in lakes (von Nordheim et al., 2018; Pawlikowski and Kornijów, 2019). The decline in structural complexity of submerged vegetation may directly compromise the nursery functions of many invertebrate and fish species, including spawning, foraging, and overwintering (Heck et al., 2003; Knaepkens et al., 2004; Churchill et al., 2016). One of the most important ecological functions of aquatic vegetation is to provide spawning substrates for a wide range of phytophilic spawners (Heck et al., 2003; Miano et al., 2019). Although the function of supporting spawning is common knowledge in aquatic ecology, this important ecosystem service has been rarely studied in details and receives little attention in current lake ecosystem management programs (Marsh et al., 2022). However, fish dependent on submerged vegetation for spawning are important component of the food web and important fishery resources, such as cyprinids in lakes (such as common carp, Cyprinus carpio; and crucian carp, Carassius auratus). The fecundity of phytophilic cyprinids in lakes is thought to be closely dependent on the availability of submerged vegetation (Liu and He, 1992).
However, lakes have suffered a significant loss of submerged vegetation in the past decades due to human activities such as reclamation of paddy fields and aquaculture ponds, anthropogenic regulation of water levels, over-stocking of herbivorous fish, urbanization, and eutrophication (Du et al., 2011; Wang et al., 2022). These human activities have led to an increase in water turbidity and resulting sharp shrinkage in the distribution of submerged vegetation and shift in species composition from diverse species to homogeneous and/or seasonal species such as Potamogeton crispus (Liu and Wang, 2010; Zhang et al., 2018). In this regard, the impairment of natural spawning ground for phytophilic spawners in lakes is strongly associated with the decline of submerged vegetation in the littoral zones (Kanstinger et al., 2018). However, restoration of submerged vegetation is a lengthy and expensive process and is often not feasible in hypereutrophic lakes (Zeng et al., 2017; Slagle and Allen, 2018). In recent years, restoration of degraded fish spawning habitats has become a priority practice for fisheries development and biodiversity conservation (Palm et al., 2007; Manny et al., 2014; Riebe et al., 2014). Specifically, artificial spawning substrates such as artificial macrophytes, shrubs and nets and so on have been used as medias to assist fish spawning and improve spawning habitat quality to increase spawning and restore fish populations (Gillet and Dubois, 1995; Nash et al., 1999; Lehtonen et al., 2006; Čech et al., 2012; Crane and Farrell, 2013; Malinovskyi et al., 2018).
Nowadays, the effectiveness of artificial spawning substrates in enhancing fish recruitment and the construction and management of artificial spawning grounds are increasingly being studied. Among them, the selection of artificial spawning substrate materials and structures is the most widely reported (Gillet and Dubois, 1995; Nash et al., 1999; Lehtonen et al., 2006; Čech et al., 2012; Crane and Farrell, 2013; Malinovskyi et al., 2018; von Nordheim et al., 2018). Complex and rigid substrates are the better choice for constructing artificial spawning grounds (Gillet and Dubois, 1995; Nash et al., 1999). Artificial materials are superior to natural materials in providing a healthy incubation environment for eggs, especially the provision of adequate oxygen (Čech et al., 2012; Malinovskyi et al., 2018; von Nordheim et al., 2018). Environmental factors such as water temperature, depth, waves, flow velocity, and sunshine duration can all affect the efficiency of artificial spawning substrates (Gillet and Dubois, 1995; Nash et al., 1999; Čech et al., 2012). Therefore, it is very important to determine the timing and location of artificial spawning grounds. Proper placement and regular maintenance can maximize the effectiveness of artificial spawning substrates (Nash et al., 1999). Artificial spawning substrate is an ideal material for studying fish reproduction behavior, and imaging sonar is considered to be the most effective, safe and environment-friendly observation method for fish reproduction behavior (Pavlov et al., 2009; Langkau et al., 2016; Smith et al., 2021). The reproductive behavior of fish is a guideline for developing strategies for the protection and management of artificial spawning grounds. However, very little knowledge exists about the effects of artificial spawning substrates on lacustrine phytophilic spawners.
This study aimed to (1) according to the effects of spawning substrates of different structures and materials on egg adhesion and egg hatching, explore effective materials for constructing artificial spawning grounds; (2) according to the influence of water depth and water temperature on the effect of artificial spawning substrates, explore the effective deployment period and water column position of artificial spawning grounds; (3) according to the diurnal rhythm patterns and spawning population composition of fish in artificial spawning grounds, explore the effective management and protection strategies on spawning population of artificial spawning grounds. The results of this field study can provide insights in managing these phytophilic spawning populations and can guide habitat restoration or conservation efforts in lakes.
2. Materials and methods
2.1. Study area
The study was carried out in Liangs Lake (Figure 1, 114.34 ~ 114.85°E; 30.04 ~ 30.43°N) which is a typical shallow lake with a surface area of 271 km2 in the Yangtze River basin. It is the also largest impounded lake in Hubei Province and extensively covered with macrophytes (such as Potamogeton maackianus, Vallisneria natans) until the extreme flood in the Yangtze River basin in 2010 (Wang et al., 2019).
2.2. Artificial substrates
Three types of artificial substrates with different morphologic complexity and material were used in current study (Figure 2A; Figure A1 in Appendix). These artificial substrates were palm sheet (PAS; length: 450 mm, width: 300 mm), polyethylene filament (PEF; diameter: 0.3 mm, length: 350 mm) and polyethylene net (PEN; mesh size: 2.5 mm, length: 300 mm, width was 75 mm), respectively. PAS is a kind of natural material and has the highest complexity of three materials. The structure of PEF in water is more complicated than that of PEN, although they are all man-made materials. Each type of artificial substrate was used to make three-layers of circular spawning nests (diameter: 300 mm; height: 1500 mm; vertical interval between each layer: 500 mm). Each layer of circular spawning nest was consisted of four bundles of the same substrates fixed at four corners on a steel ring. One bundle of PAS has 3–4 pieces, PEF has about 1,000 filaments, PEN has 6–8 pieces. Each unit of three-layers of circular spawning nests was installed with a colored float and steel weight. The vertical height between the spawning nests from top to bottom and water surface were 200 mm, 700 mm, 1,200 mm, respectively (Figure 2A).
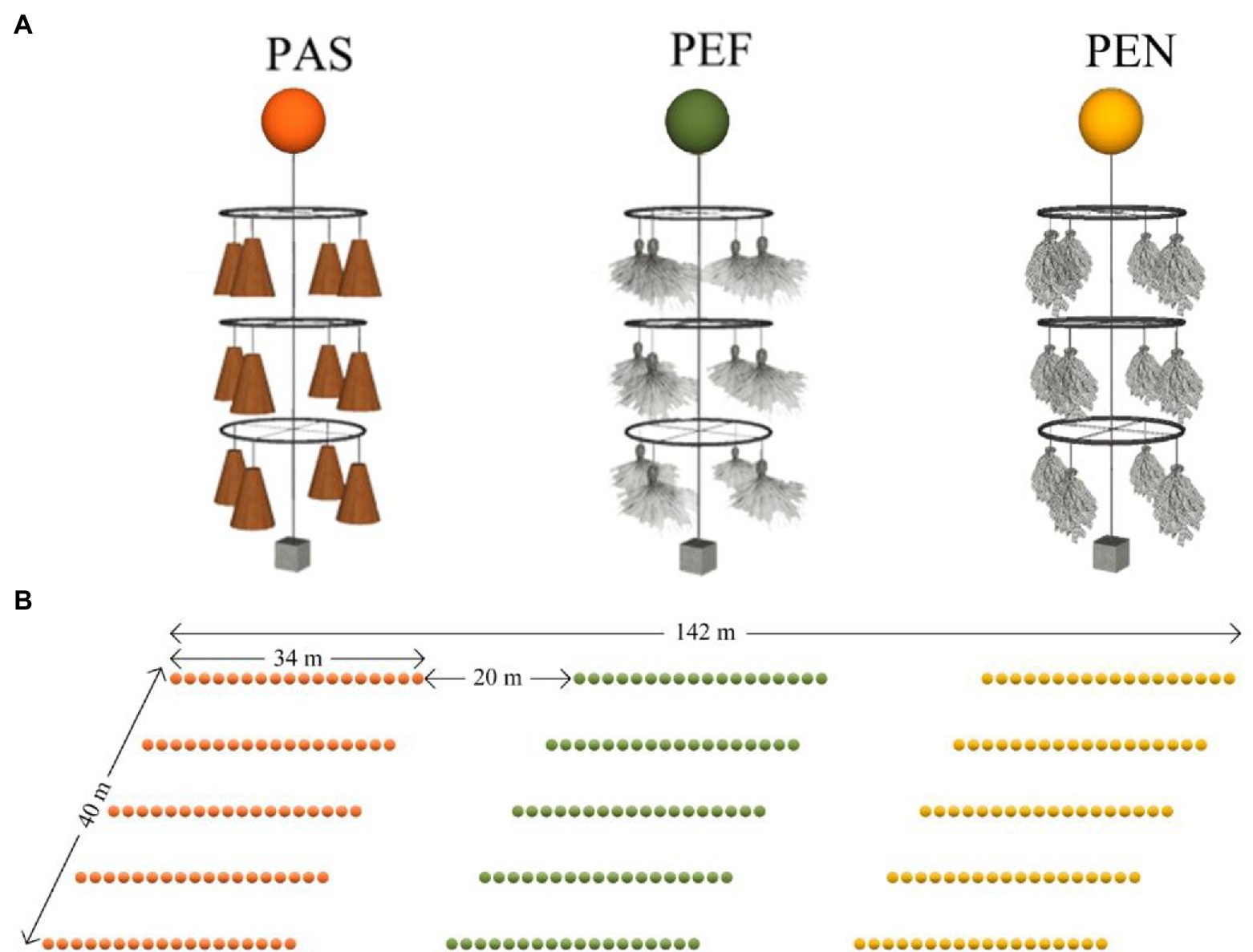
Figure 2. The structure of artificial spawning substrates (A) and arrangement of artificial spawning grounds (B). PAS, palm sheet; PEF, polyethylene filament; PEN, polyethylene net.
The artificial spawning grounds were deployed in the littoral zone with an average water depth of 2.5 m in Liangzi Lake on March 21, 2018. For each type of artificial spawning substrate, there are 90 units of artificial spawning nets which were arranged in 5 lines at 10 m intervals. In total, 18 units of the same artificial spawning substrates were connected at 2 m intervals between each unit using 20 mm diameter nylon rope following the water depth contour in Liangzi lake. Moreover, there was an interval of 20 m between adjacent types of artificial spawning grounds (Figure 2B). It should be noted that, in practical applications, the artificial spawning substrate must exist in the form of artificial spawning grounds, that is, it will not exist as a separate unit. Therefore, this study chose to build three artificial spawning grounds in similar locations with relatively homogeneous water environment. The difference between these three is only the difference of the spawning substrate material used. And there is a certain interval between the spawning grounds of different materials. In this way, the difference of artificial spawning substrates of different materials in practical application (used in combination to construct spawning grounds rather than isolated) can be studied. Therefore, this study did not adopt the method of random arrangement of artificial spawning matrix units of different materials.
2.3. Sampling strategy
The in-situ monitoring experiment of spawning activities was conducted daily between March 21 and June 7, 2018. According to records, the breeding of common carp and crucian carp in the Liangzi lake starts in April and basically ends in June. The main spawning period of the studied species was therefore basically included in the study period. Although the spawning time of a few of their individuals may be delayed until the end of June, but the high temperature will make the number of spawning eggs very small (Department of Ichthyology, Institute of Hydrobiology, CAS, 1976). Viable and dead eggs on the three types of spawning substrates were counted daily, which could be determined by the natural transparency of originally vital eggs and cloudiness of dead eggs (white color) (von Nordheim et al., 2018). Egg retention was randomly inspected daily on one unit of spawning nests along the five lines, hence there were five replicates for each type of artificial spawning ground. And the exact same relative position was sampled in 1 day in the spawning grounds composed of three materials, so as to ensure the comparability of the data of artificial spawning substrates made of different materials. Three layers of circular spawning nests from top to bottom were counted, respectively. The specific method of egg counting is to take a fixed number of pieces or filaments spawning substrates out of the water surface and count the attachment of eggs on the spot. To verify field data and reduce counting errors, digital images of sampled substrates on every surface were taken, and the viable and dead eggs were counted with an imaging software Image J (version 1.38x, Wayne Rasband, National Institute of Health, 2008). The sampled surface area of each material was calculated.
A certain number of viable eggs were sampled together with spawning substrates and used for hatching experiment to estimate hatchability when the spawning peak occurred in the field. Incubation experiments are carried out at the station near the lake and use lake water for incubation to ensure the environment is close to the lake environment. Subsequently, the hatched fry was reared for 1 week to calculate survival rate. When the fry has grown to a size that can identify the species, they are sampled and identified under a microscope based on morphology. According to microscopic identification, the fry hatched from eggs attached to the artificial substrates were dominated by crucian carp (Carassius auratus) and common carp (Cyprinus carpio).
2.4. Spawning monitoring
Dual-frequency identification sonar (DIDSON, ARIS Explorer 3,000, Sound Metrics) was used to observe the spawning behavior, monitor the spawning peak and measure the body length of spawning groups on the artificial spawning grounds. It is worth mentioning that this method cannot identify species. DIDSON operates at two frequencies, providing two modes. Identification mode, which provides high resolution (operating frequency is 3.0 MHz) and low range (nominal effective range is 5 m). Detection mode, which provides lower resolution (operating frequency is 1.8 MHz) and high range (nominal effective range is 15 m). In this study, we used the detection mode to broaden the observational range in the artificial spawning ground. The beam array was 128 beams at the low-frequency setting, with beamwidth of 0.3°H by 14°V. This array of beams is assembled into a sector along the narrow dimension of the beams to constitute a 30° × 15° field of view. The downrange resolution is 0.003 m. The max frame rate is 4–15 frames/s. The probe was about 20 cm below the surface and the field of vision was horizontal. In this way, the probe was at the same water depth as the upper rings. Through early surveys, the PAS was the favored artificial spawning substrates. Therefore, the observation site of DIDSON was arranged in the PAS spawning ground. The probe was fixed on a boat and the observation distance was set to 0.7 ~ 11.5 m. The direction of the probe was almost coincided with the first row of the PAS spawning ground. As the result, there were 5 rings of artificial spawning substrates in the monitor vision of DIDSON. The spawning peak and body length of spawning groups were monitored by DIDSON during April 8 to April 12, 2018. From the perspective of the whole study period, the spawning monitoring period was in the third spawning peak (Figure 3). Although the spawning intensity of fish at each spawning peak was different, according to the distributison of fish spawning periods in Liangzi Lake, common carp and crucian carp were still the main spawning species during this monitoring period (Department of Ichthyology, Institute of Hydrobiology, CAS, 1976). Therefore, the fish behavior and spawning assemblage composition observed during the monitoring period are representative of the study.
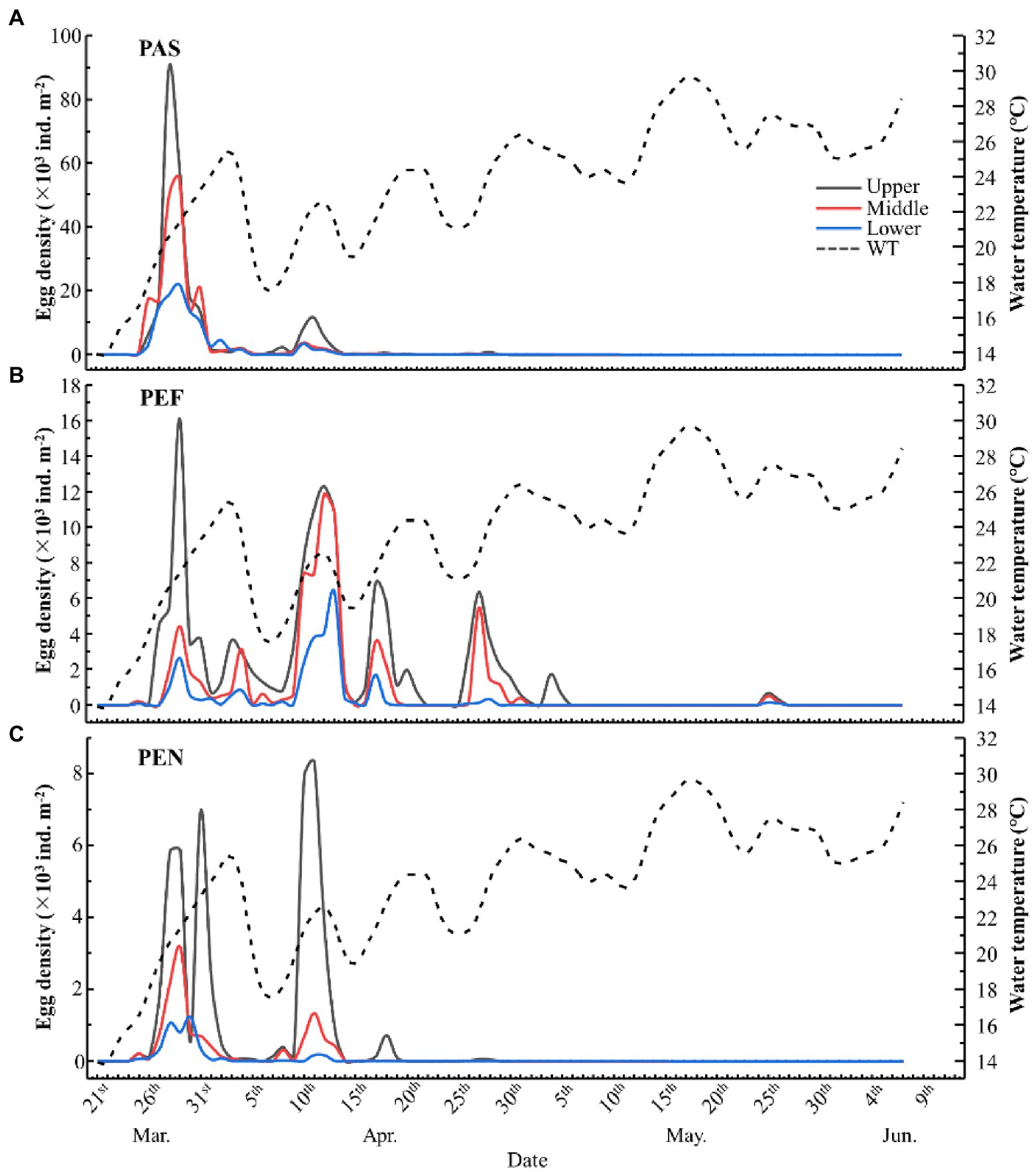
Figure 3. The relationship between daily water temperature and egg density among substrate groups and water layers. PAS, palm sheet; PEF, polyethylene filament; PEN, polyethylene net.
2.5. Water temperature monitoring
To study the relationship between fish spawning behavior and water temperature, water temperature of artificial spawning ground was recorded every 2 h under 0.5 m from the water surface using water temperature recorders (HOBO, Onset Computer Corporation) during study period.
2.6. Data analyses
2.6.1. Egg deposition and mortality
Egg deposition was determined by counting the eggs attached to the artificial substrate and measuring the surface are of artificial substrate. Each artificial spawning substrate had five replicates in each water layer (upper, middle, and lower). Egg density on artificial spawning substrate was calculated by the following equation:
The percentage of dead eggs attached on the artificial substrate was calculated by the following equation:
The pairwise comparisons of daily egg density and egg mortality among different artificial substrates and water layers were conducted when the spawning events were observed.
2.6.2. Hatching rate and survival rate
In total, 22, 20 and 26 batches (about 100 eggs per batch) of eggs from PAS, PEN and PEF were used in hatching experiment, respectively. The initial number of eggs in each batch () were reared until hatch where surviving fry were enumerated to determine egg hatch number (). Initial numbers of fry () were enumerated after 7 days to determine the 7-day survival rate (). The hatching rate (the probability of eggs hatching successfully), 7-day survival rate (the probability of hatched fry surviving for 7 days) and the total survival rate (the probability of eggs hatching into fry and surviving for 7 days) could be calculated as follow:
2.6.3. Fish length of spawning group
Spawning fish were enumerated and measured from DIDSON data analyzed using ARISFish (version 2.6.2, Sound Metrics, 2019) software. Fish observed by the DIDSON were measured and counted from 6:30 AM on April 9 to 6:30 AM on April 10 when the spawning peaks occurred.
2.6.4. Statistics
Statistical analyses were performed using SPSS (version 19.0, Statistical Package for Social Sciences (SPSS), 2010), R (version 3.5.2, R Core Team, 2018), and Origin Pro (version 2017C, Origin Lab Corporation, 2017). For egg density and egg mortality, pairwise comparisons between values of all substrate materials (PAS, PEF, PEN) of same water layers were conducted with the pairwise Mann–Whitney U-test (nonparametric method chosen due to nonnormal distribution of data). The same pairwise comparisons were conducted between values of all water layers (Lower, Middle, Upper) of same substrate materials. For hatching rate and survival rate of eggs, pairwise Mann–Whitney U-test were conducted to compare the difference of substrate materials. Paired test was applied to eliminate the effect of different sampling batches (different sampling data and locations in spawning ground). The p values of pairwise comparisons were adjusted by Bonferroni correction. The significance level for all statistical analyses within the study was set to p < 0.05.
The range of water temperatures during the study period in which fish spawn most vigorously was tested. Average the egg density of all samples and water layers for each material each day, and divide the data into three groups according to the water temperature of the day: < 20°C, 20–25°C, >25°C. Kruskal–Wallis test was conducted to test if there were significant differences between the different temperature groups.
3. Results
3.1. Effects of different materials on egg adhesion and hatching
In terms of the averaged egg density on the three types of substrates, PAS was the most preferred spawning substrate, followed by PEF (not significant with PAS, p > 0.05; Figure 4A) and PEN has the lowest egg density (significant with PAS and PEF, p < 0.05; Figure 4A). However, the egg mortality of PAS was significantly higher than PEF and PEN (p < 0.05; Figure 4C).
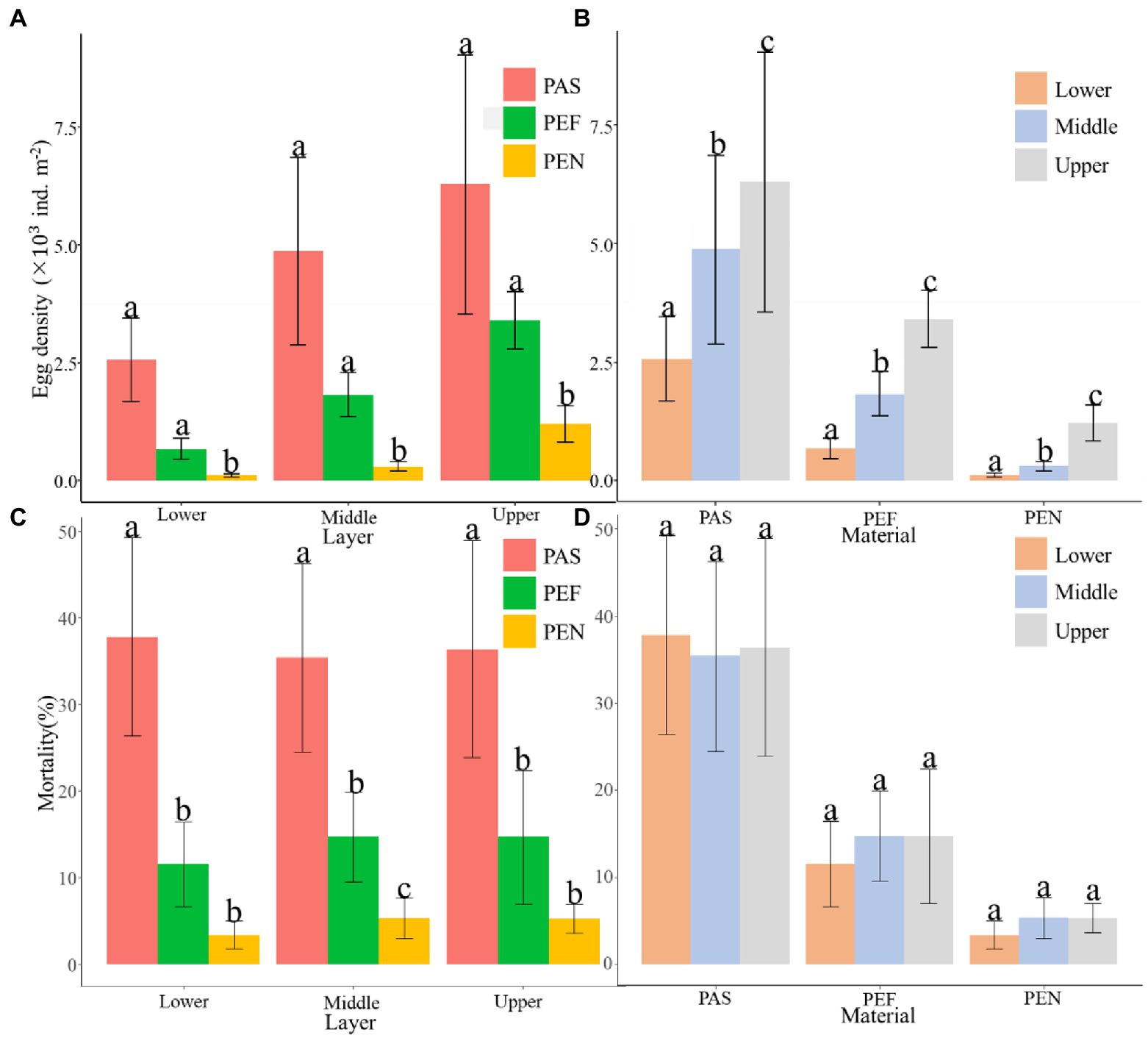
Figure 4. The comparison of (A) egg density between different materials; (B) egg density between different water layers; (C) egg mortality between different materials; (D) egg mortality between different water layers. PAS, palm sheet; PEF, polyethylene filament; PEN, polyethylene net. Meanwhile, More spawning peaks were observed on PEF (five peaks: March 26 ~ April 1, April 3 ~ April 4, April 8 ~ April 13, April 16 ~ April 20 and April 26 ~ April 30), and followed by PEN and PAS (two peaks: March 26 ~ April 1 and April 8 ~ April 13; Figure 3).
In incubation experiments of three different materials, the hatching rate of egg, seven-day survival rate of fry and total survival rate of egg on PEN were significantly lower than PAS and PEF (p < 0.05). There was no significant difference between PAS and PEF (p > 0.05; Figure 5).
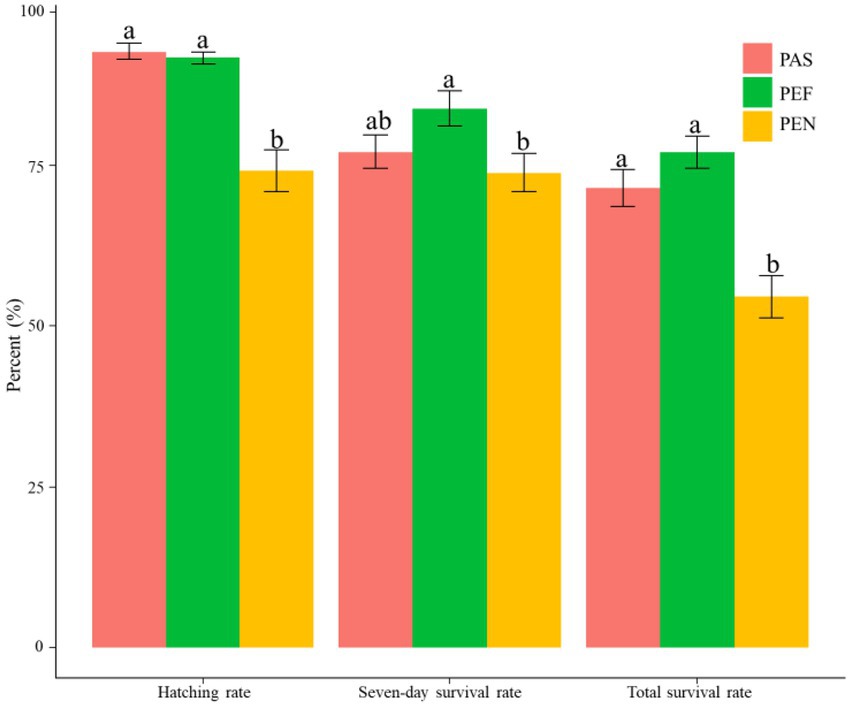
Figure 5. The comparison of hatching rate and survival rate among substrate groups. PAS, palm sheet; PEF, polyethylene filament; PEN, polyethylene net.
3.2. Influence of water depth and temperature on artificial spawning substrates
Different water layers apparently influence the density of attached eggs on artificial spawning substrates. In all types of artificial spawning grounds, the upper water layer was the most preferred spawning water layer, followed by the middle and lower water layer (all significant between each layer, p < 0.05; Figure 4B). However, there was no significant difference in egg mortality among the three water layers (p > 0.05; Figure 4D).
Changes in water temperature also affect the effectiveness of artificial spawning substrates. The daily water temperature of the artificial spawning grounds in Liangzi Lake were recorded and the corresponding water temperature for the spawning peaks were delineated in Figure 3. According to the results of Kruskal-Wallis test, the egg densities on the three materials in the water temperature range of 20 ~ 25°C were significantly (p < 0.05) greater than those in other temperature ranges (< 20°C and > 25°C). In addition, spawning peaks always occurred during periods of temperature increase, and visible declines of water temperature were commonly observed prior to the occurrence of spawning peaks (Figure 3).
3.3. Diurnal rhythm patterns of spawning and population composition of fish in artificial spawning grounds
For diurnal rhythm patterns of spawning, 22 spawning events were recorded by DIDSON during 12:00 on April 8 to 12:00 on April 12, 2018. 9.1, 72.7, 18.2 and 0.0% of spawning events in the artificial spawning grounds occurred during 18:00–00:00 (sunset to midnight), 00:00 ~ 06:00 (midnight to sunrise), 06:00–12:00 (sunrise to midday) and 12:00–18:00 (midday to sunset) respectively (Figure 6).
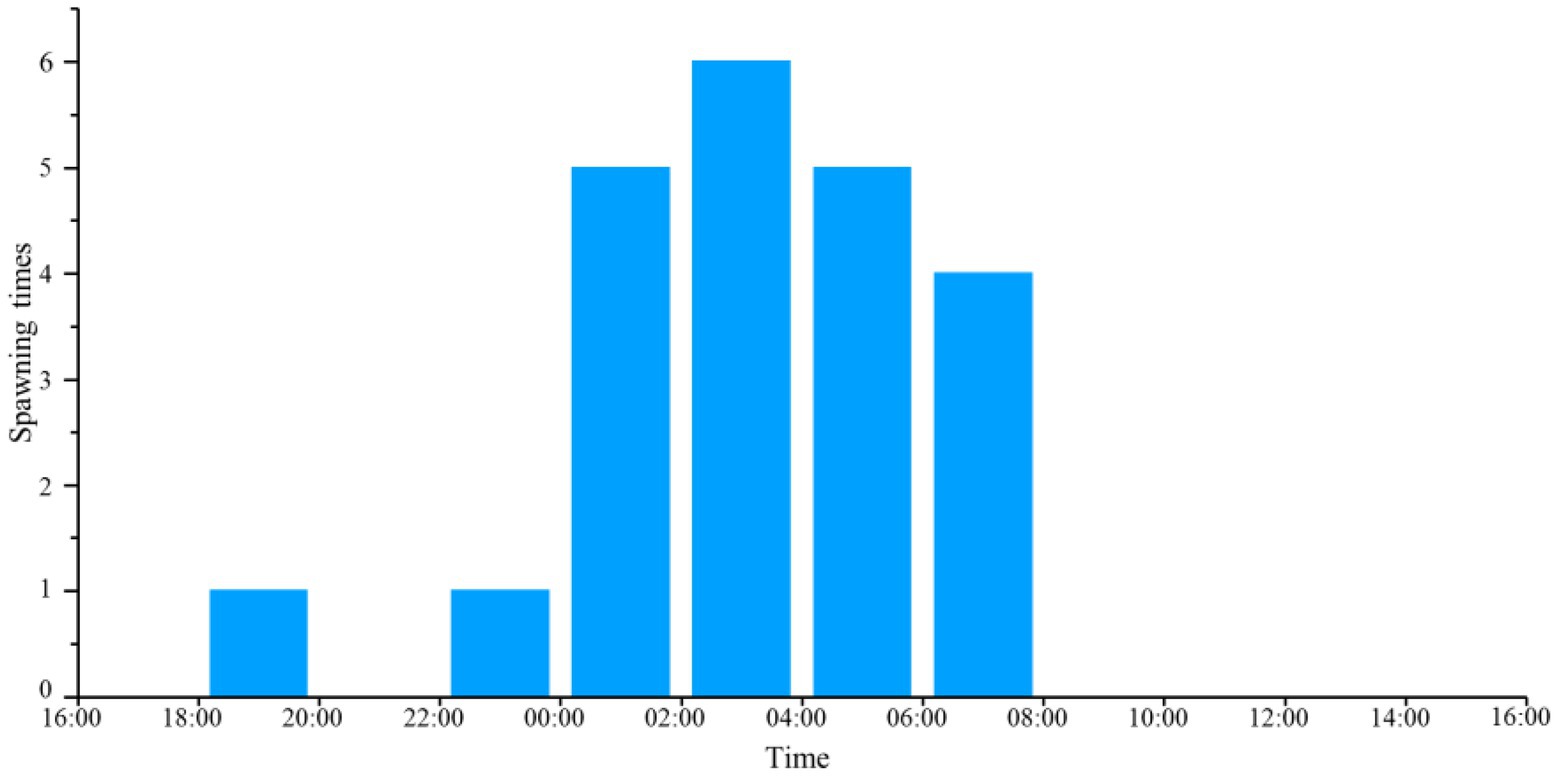
Figure 6. The temporal distribution of spawning occurrences in a full day. Spawning times represents the number of fish spawning observed by DIDSON.
A total of 13,924 fish were measured by DIDSON during this period (Figure 7). The fish length mainly ranged from 5 to 20 cm accounting for 79.24% of the total measured fish. Fish with a body size of less than 15 cm dominated during 07:30–19:30. In the remaining 12 h, the proportion of fish larger than 15 cm increased. Especially 23:30–05:30, which coincides with the time with the most spawning events in Figure 6. Meanwhile, the number of fish larger than 20 cm and 30 cm were also increased during 19:30 to 06:30, especially in the mid night (Figure 8). When almost all females of crucian carp (Carassius auratus) and common carp (Cyprinus carpio) reach sexual maturity, their body lengths are 15 cm and 30 cm, respectively, (Liu and He, 1992).
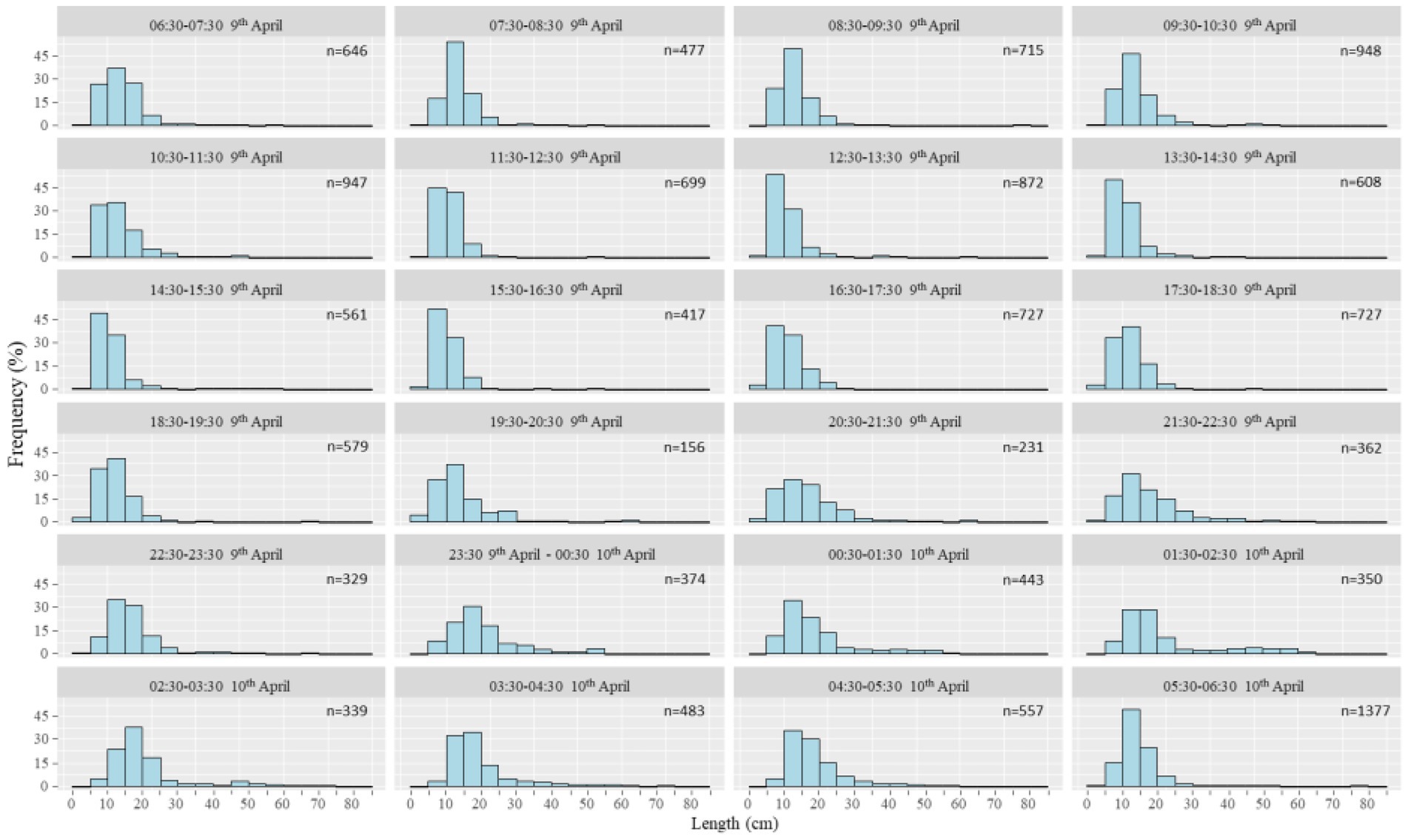
Figure 7. The temporal distribution of fish number and body length in a full day. The distribution of body length of spawning fish was depicted by hour in a whole day.
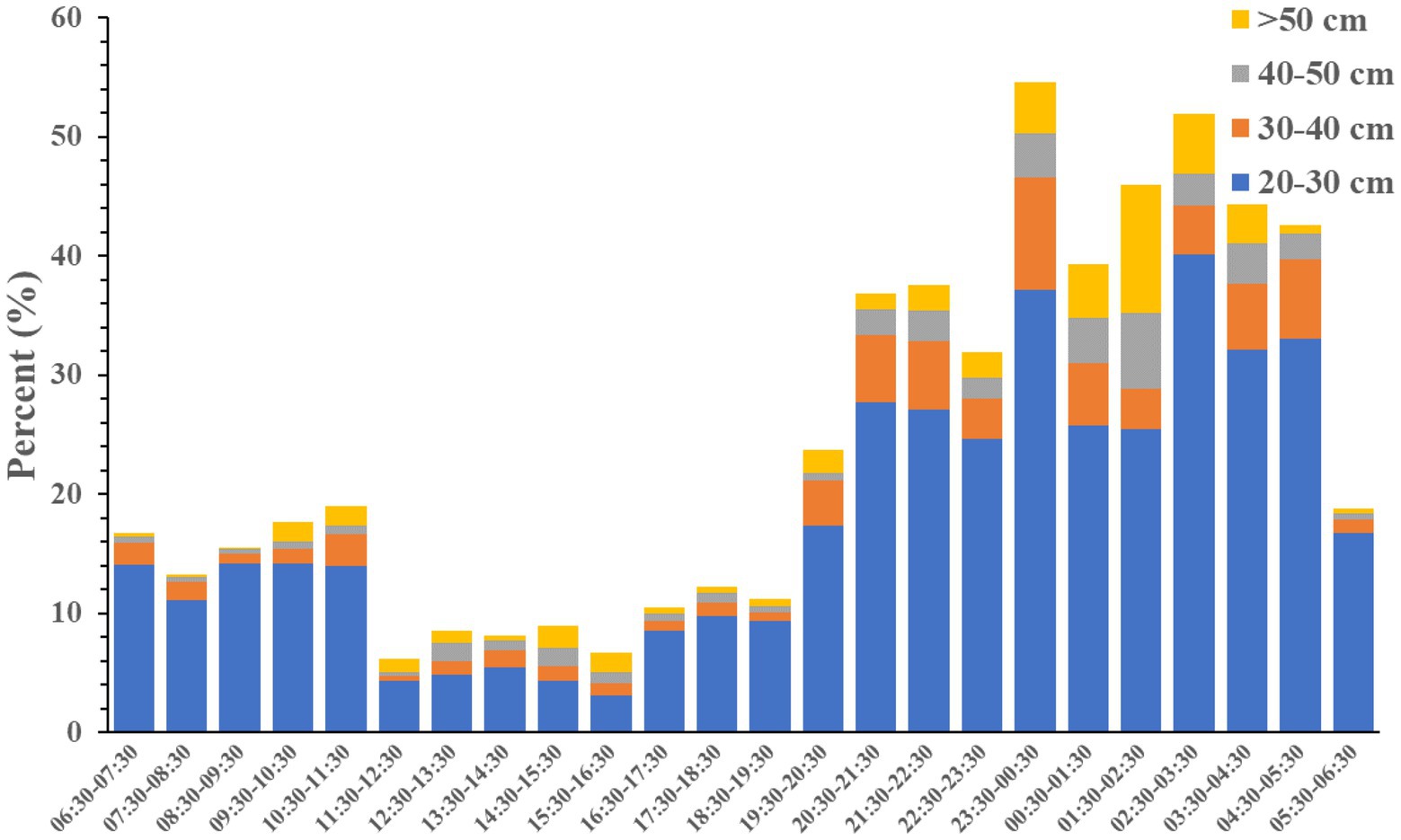
Figure 8. The temporal distribution of the percent contribution of the number of different body length group to the total number of fish in a full day.
4. Discussion
This study systematically explored artificial spawning substrates as a means of artificially assisting fish proliferation. The advantages and disadvantages of three artificial spawning substrates in the construction of artificial spawning grounds were studied. PAS had the highest egg density and also the highest egg mortality. PEF has intermediate egg density and egg mortality, but has the advantage of consistently attracting fish to spawn. PEN has the lowest egg density due to its simple structure and has a lower hatchability in hatching experiments. The position of artificial spawning substrate in the water column will affect the density of attached eggs but has no effect on the mortality of eggs. There is an optimum temperature for fish to use artificial spawning grounds, and the time for constructing spawning grounds should follow this basis. Monitoring of fish spawning assemblage and behavior determined the likely size range of spawning individuals and the timing of spawning behavior.
4.1. Explore effective materials and structure for constructing artificial spawning grounds
In recent decades, rehabilitation of degraded aquatic habitats has been one of the important approaches for fisheries management and biodiversity conservation (Slagle and Allen, 2018; Whiterod et al., 2021). Artificial spawning substrates may be a promising tool for habitat enhancement in degraded ecosystems such as lakes (Knaepkens et al., 2004; Malinovskyi et al., 2018). Therefore, the effectiveness of materials and structures of artificial spawning substrates has been a topic of discussion by numerous researchers (Gillet and Dubois, 1995; Nash et al., 1999; Lehtonen et al., 2006; Čech et al., 2012; Crane and Farrell, 2013; Malinovskyi et al., 2018). Dense and complex three-dimensional structures are more attractive for fish spawning than loose and simple two-dimensional structures, and differences in structure appear to be more important than the material itself (Gillet and Dubois, 1995; Čech et al., 2012; Crane and Farrell, 2013). The results of present study are consistent with this theory. PAS attracts more fish to spawn because it has the densest structure among the three selected substrates. On the other hand, although PEF and PEN were made of same materials, the complex three-dimensional structure of PEF formed in water is significantly more attractive to fish spawning than the simple two-dimensional network structure of PEN.
Egg mortality is another important indicator to assess the efficacy of artificial substrates supporting the recruitment of fish populations (Malinovskyi et al., 2018; von Nordheim et al., 2018). The main reasons for the death of eggs attached to the substrate are the inhibition of oxygen supply and metabolite processing by multi-layered and clustering eggs (Čech et al., 2012; von Nordheim et al., 2018), and the infection of fungi (Malinovskyi et al., 2018; von Nordheim et al., 2018). Both of these above reasons obviously lead to PAS having the highest egg mortality. Excessive density of attached eggs results in hypoxia of eggs on PAS, while degradation of natural materials may generate a suitable medium for fungal infection (Zarski et al., 2015), further increasing the egg mortality. In contrast, the egg mortality of PEF and PEN was significantly lower than that of PAS, which reflects the advantage of artificial synthetic materials in preventing water body from being polluted by organic matter (Malinovskyi et al., 2018). Although not significant, PEF still had a higher egg mortality than PEN due to higher egg density.
PEF exhibited the most enduring attraction to fish, as it had the most spawning peaks and its egg density was at a relative high level from the beginning to the end of the spawning period. In contrast, the egg density on PAS declined rapidly despite having the highest level at the beginning of the spawning period. This is because natural materials degrade and produce organic contamination when introduced into the water, which may prevent fish from accessing the substrate (Nash et al., 1999; Malinovskyi et al., 2018). In addition, Nash et al. (1999) proposed that artificial spawning substrates require regular maintenance (cleaning or replacement) to maintain their effectiveness in attracting fish.
The hatching rate profiles among the three substrates were normal compared with previous studies (Peng et al., 2016). However, the hatching rate of PEN was slightly lower than the other two substrates. In hatching experiments, only healthy eggs were selected and incubated under the same conditions along with the substrate. Therefore, only the different attachment patterns of the eggs on the substrate affected the hatchability. Previous studies have shown that eggs were scattered on more structurally complex substrates, while eggs on less complex substrates adjoined side-by-side, which may block oxygen supply and metabolite processing during egg hatching (von Nordheim et al., 2018).
4.2. Explore effective deployment period and water column position of artificial spawning grounds
The recruitment efficiency of artificial spawning substrates for fish depends on the deployment period of artificial spawning grounds (Gillet and Dubois, 1995). Setting too early can make maintenance and cleaning of artificial spawning grounds cumbersome, while setting too late can miss spawning peaks (Nash et al., 1999). The deployment period of the artificial spawning ground needs to be consistent with the spawning time of fish, which depends on the water temperature (Malinovskyi et al., 2018). The condition for triggering fish spawning is that the water temperature threshold is reached, so it is feasible to determine the deployment period of artificial spawning grounds according to the water temperature (Gillet and Dubois, 1995). At the same time, mastering the relationship between spawning peak and water temperature is conducive to the monitoring and maintenance of artificial spawning grounds (Nash et al., 1999).
Under natural conditions, common carp begin to spawn when the water temperature rises to 17°C, generally spawn in large batches at water temperature of 18 ~ 21°C and basically stops when the water temperature is above 26°C. The spawning temperature of crucian carp is very similar to those of common carp (Liu and He, 1992). In this study, the water temperature of spawning peaks on three types of artificial substrates were almost within the range of 20 ~ 25°C. Therefore, this water temperature range is within the suitable range for spawning for both species. In addition, spawning peaks mainly occurred during the rising phases of water temperature after dramatic declines due to strong wind or heavy rainfall. Our results are in line with Malinovskyi et al. (2018) who suggested a strong relationship between fish spawning and water temperature rise under natural condition. Meanwhile, Gillet and Dubois (1995) suggested sudden drop in water temperature slows spawning intensity.
Previous studies have found that the choice of artificial spawning substrate by fish is related to its location in the water column (Gillet and Dubois, 1995; Knaepkens et al., 2004; Čech et al., 2012). There are differences in the selection of different species of fish. For example, in Europe, where artificial spawning substrates are widely used, perch and pike spawn on the bottom of the water, and the roach spawns near the surface (Gillet and Dubois, 1995). Common carp and crucian carp are phytophilic spawner, and they choose submerged plants for spawning under natural conditions (Liu and He, 1992). Santos et al. (2008) pointed out that the position of the artificial substrate in the water column may influence fish selection more than the structure of the artificial substrate. In our study, the spawning substrate in the upper layer of the water column (200 mm from the water surface) was more attractive for fish to spawn. Determining the optimal water column location for artificial spawning substrates is critical to the effectiveness of spawning ground arrangements.
4.3. Explore effective management and protection strategies on spawning population of artificial spawning grounds
To formulate management strategies for efficient operation of artificial spawning grounds, it is necessary to study the behavior of spawning population. The artificial spawning substrate is an ideal material for studying spawning behavior (Gillet and Dubois, 1995). The observation of fish spawning activity in the artificial spawning grounds has been subject to difficulties that cannot be solved by traditional methods such as diving observation, underwater video and passive acoustic because these methods are either affected by turbidity in lakes or do not enable continuous 24-h monitoring, and therefore all have significant deficiencies (Zhadan et al., 2018; Grabowski et al., 2020; Rastoin-Laplane et al., 2020). In this study, DIDSON enables full day observation (especially at night) of fish spawning and diminishes anthropogenic disturbances to fish spawning. It provides a new attempt to observe spawning behaviors in artificial structures (Pavlov et al., 2009; Langkau et al., 2016; Smith et al., 2021). Mastering the circadian rhythm of spawning and the composition of spawning populations is conducive to formulating protection and management strategies for artificial spawning grounds.
DIDSON monitoring results revealed that spawning was focused between 18.00 and 08.00 h, especially between 00.00 and 08.00 h in Liangzi Lake. These findings are consistent with previous studies that spawning usually occurs at night and early morning (Schlumberger and Proteau, 1996; Malinovskyi et al., 2018). And this has also been confirmed in previous observations using DIDSON (Pavlov et al., 2009; Langkau et al., 2016). In respect to the spawning aggregations, small-sized fish (<20 cm) consistently predominated in the artificial spawning grounds, which may be related to the miniaturization of fish due to overfishing in Liangzi Lake (Xie et al., 2001; Ye et al., 2006; Wang et al., 2019). Large-sized fish (> 20 cm) were found mainly from 19.30 to 06.30 h. These findings are generally in line with previous study that large fish utilize artificial structures only after sunset (Santos et al., 2008).
The present research is of great significance to the management of artificial spawning grounds and the protection of spawning populations. Human disturbance to spawning grounds should be reduced during the spawning time of fish, especially to prevent poaching in artificial spawning grounds. Targeted protection on larger individuals of spawning aggregations can maintain adequate numbers of parents in artificial spawning grounds. In our observations, small individual fish always aggregated around the substrate after spawning behavior occurred. Although it cannot be concluded from DIDSON observations, but some species of small individuals in the lake have the potential to feed on fish eggs (Jin et al., 1996). Therefore, the selective removal of these small individual species can also have a protective effect on artificial spawning grounds.
4.4. Exploration in the application of artificial spawning substrate
Despite these promising results, applications in artificial spawning substrates remain explorable. The contradiction between multi-layered eggs caused by high egg density attached on substrates and high egg mortality is obvious in the present study. This may be due to the insufficient number and size of artificial spawning substrates in this experiment to support the recruitment of lacustrine fish (Malinovskyi et al., 2018). Hence, further research should be undertaken to determine the minimum scale of artificial spawning grounds. Meanwhile, artificial spawning grounds also require regular maintenance and cleaning (Nash et al., 1999). While these issues may be mitigated by setting the optimum deployment period of artificial spawning grounds based on water temperature, a range of management deadlines still needs to be explored in practice. Structure-specific spawning substrates could be selectively used by specific taxa group of fish (Santos et al., 2008; Rastoin-Laplane et al., 2020). Therefore, based on the principle of mimicking natural spawning grounds (Winfield, 1986), there is abundant room for further development of artificial spawning substrates that support the recruitment of more species.
Despite our use of DIDSON, there are still limitations in observing fish behavior in artificial spawning grounds. In the present study, DIDSON observations were specific to PAS only, as this substrate is most attractive to fish spawners. However, differences in fish spawning behavior between all materials were not investigated. DIDSON does not directly identify species. It is even more impossible to distinguish whether the individual in the field of view has been observed, so repeated observations may be unavoidable (Foote, 2009).
Although this study proves that the artificial spawning grounds are beneficial to increase fishery resources as a means of assisting fish proliferation, there are still potential risks and deficiencies in their use that need attention. From the perspective of environmental pollution, the selection of artificial spawning substrate materials needs to be cautious. The pollution of freshwater environment by microplastics has become a hot topic (Li et al., 2020), so more consideration should be given to natural and degradable materials. In addition, it is particularly important that artificial spawning grounds can never replace the role of natural aquatic plants in the ecosystem. Although artificial substrates can provide more spawning grounds for fish, they cannot provide other ecological functions of aquatic vegetation, such as water purification, providing nursery and food for fish (Heck et al., 2003; Knaepkens et al., 2004; Churchill et al., 2016). Even this complex artificial structure can cause fish to fall into the trap. Therefore, lake ecosystem managers need to clearly understand that artificial spawning grounds are a compromise to increase fishery resources in the case of natural habitat loss. To truly restore fish resources and ecological environment, it is fundamental to protect and restore natural habitats.
5. Conclusion
In the context of ongoing global degradation of submerged vegetation in littoral zone in lakes, the restoration of aquatic habitats such as spawning grounds is imperative. The results of present study indicate that the artificial substrates with complex and dense three-dimensional structure which have enough space to enable material exchange and oxygen circulation could be used to enhance fish recruitment in lakes. Meanwhile, this study also provides guidance on the restoration engineering parameters including the material and structure of artificial spawning substrates, deployment time and water column position of artificial spawning grounds, management and protection strategies on spawning population in lakes. In general, the results confirm the effectiveness of artificial substrates as suitable spawning substrates for fish. Therefore, artificial substrates may be an important tool in fisheries enhancement in lakes. However, this study proposes that the artificial spawning substrate is only a compromise of artificially assisted fish proliferation, and cannot replace the natural fish habitat.
Data availability statement
Raw data are available upon request to the authors.
Author contributions
KF: methodology, software, investigation, formal analysis, data curation, writing—original draft, writing—review and editing, and visualization. JY: resources. YZ and JQ: investigation. JL, ZL, and SL: writing—review and editing. QW: conceptualization, methodology, investigation, formal analysis, data curation, writing—review and editing, supervision, project administration, formal analysis, and funding acquisition. All authors contributed to the article and approved the submitted version.
Funding
This work was financially supported by the National Key Research and Development Program of China (no. 2019YFD0900602), National Natural Science Foundation of China (no. 32002396), Youth Innovation Promotion Association CAS (no. 2022344).
Acknowledgments
The contribution of S. S. De Silva from School of Life and Environmental Sciences, Deakin University was made when on a Visiting Professorship under the auspices of the CAS, tenable at the Institute of Hydrobiology, Wuhan.
Conflict of interest
The authors declare that the research was conducted in the absence of any commercial or financial relationships that could be construed as a potential conflict of interest.
Publisher’s note
All claims expressed in this article are solely those of the authors and do not necessarily represent those of their affiliated organizations, or those of the publisher, the editors and the reviewers. Any product that may be evaluated in this article, or claim that may be made by its manufacturer, is not guaranteed or endorsed by the publisher.
Supplementary material
The Supplementary material for this article can be found online at: https://www.frontiersin.org/articles/10.3389/fevo.2022.1062612/full#supplementary-material
References
Bolduc, P., Bertolo, A., Hudon, C., and Pinel-Alloul, B. (2020). Submerged aquatic vegetation cover and complexity drive crustacean zooplankton community structure in a large fluvial lake: an in situ approach. J. Great Lakes Res. 46, 767–779. doi: 10.1016/j.jglr.2019.12.011
Čech, M., Vejřík, L., Peterka, J., Říha, M., Muška, M., Jůza, T., et al. (2012). The use of artificial spawning substrates in order to understand the factors influencing the spawning site selection, depth of egg strands deposition and hatching time of perch (Perca fluviatilis L.). J. Limnol. 71, 18–179. doi: 10.4081/jlimnol.2012.e18
Churchill, R. T. J., Schummer, M. L., Petrie, S. A., and Henry, H. A. L. (2016). Long-term changes in distribution and abundance of submerged aquatic vegetation and dreissenid mussels in long Point Bay, Lake Erie. J. Great Lakes Res. 42, 1060–1069. doi: 10.1016/j.jglr.2016.07.012
Crane, D. P., and Farrell, J. M. (2013). Spawning substrate size, shape, and siltation influence walleye egg retention. N. Am. J. Fish Manag. 33, 329–337. doi: 10.1080/02755947.2012.760504
Department of Ichthyology, Institute of Hydrobiology, CAS (1976). Fishes of the Yangtze River. Beijing: Science Press.
Du, Y., Xue, H., Wu, S., Ling, F., Xiao, F., and Wei, X. (2011). Lake area changes in the middle Yangtze region of China over the 20th century. J. Environ. Manag. 92, 1248–1255. doi: 10.1016/j.jenvman.2010.12.007
Foote, K. G. (2009). “Acoustic methods: brief review and prospects for advancing fisheries research” in The Future of Fisheries Science in North America. eds. R. J. Beamish and B. J. Rothschild (Berlin: Springer), 313–343.
Gillet, C., and Dubois, J. P. (1995). A survey of the spawning of perch (Perca fluviatilis), pike (Esox lucius), and roach (Rutilus rutilus), using artificial spawning substrates in lakes. Hydrobiologia 300-301, 409–415. doi: 10.1007/BF00024482
Grabowski, T., Young, S. P., and Cott, P. A. (2020). Looking for love under the ice: using passive acoustics to detect burbot (Lota lota: Gadidae) spawning activity. Freshw. Biol. 65, 37–44. doi: 10.1111/fwb.13314
Heck, K. L., Hays, G., and Orth, R. J. (2003). Critical evaluation of the nursery role hypothesis for seagrass meadows. Mar. Ecol. Prog. Ser. 253, 123–136. doi: 10.3354/meps253123
Jin, K., Shi, W., Yu, X., Hu, H., and Li, W. (1996). The preliminary observation on eight small freshwater fishes eating adhesive fish eggs. J. Dal. Fish. Coll. 11, 24–30.
Kanstinger, P., Beher, J., Grenzdörffer, G., Hammer, C., Huebert, K. B., Stepputis, D., et al. (2018). What is left? Macrophyte meadows and Atlantic herring (Clupea harengus) spawning sites in the Greifswalder Bodden, Baltic Sea. Estuar. Coast. Shelf Sci. 201, 72–81. doi: 10.1016/j.ecss.2016.03.004
Knaepkens, G., Bruyndoncx, L., Coeck, J., and Eens, M. (2004). Spawning habitat enhancement in the European bullhead (Cottus gobio), an endangered freshwater fish in degraded lowland rivers. Biodivers. Conserv. 13, 2443–2452. doi: 10.1023/B:BIOC.0000048448.17230.40
Langkau, M. C., Clavé, D., Schmidt, M. B., and Borcherding, J. (2016). Spawning behaviour of Allis shad Alosa alosa: new insights based on imaging sonar data. J. Fish Biol. 88, 2263–2274. doi: 10.1111/jfb.12978
Lehtonen, H., Lappalainen, J., Kervinen, J., and Fontell, E. (2006). Spatial distribution of spawning sites of pikeperch (Sander lucioperca L.) in a highly eutrophic clay-turbid lake - implications for management. J. Appl. Ichthyol. 22, 540–542. doi: 10.1111/j.1439-0426.2006.00721.x
Li, C., Busquets, R., and Campos, L. C. (2020). Assessment of microplastics in freshwater systems: a review. Sci. Total Environ. 707:135578. doi: 10.1016/j.scitotenv.2019.135578
Liu, X., and Wang, H. (2010). Estimation of minimum area requirement of river-connected lakes for fish diversity conservation in the Yangtze River floodplain. Divers. Distrib. 16, 932–940. doi: 10.1111/j.1472-4642.2010.00706.x
Malinovskyi, O., Veselý, L., Blecha, M., Křišťan, J., and Policar, T. (2018). The substrate selection and spawning behaviour of pikeperch Sander lucioperca L. broodstock under pond conditions. Aquac. Res. 49, 3541–3547. doi: 10.1111/are.13819
Manny, B. A., Roseman, E. F., Kennedy, G., Boase, J. C., Craig, J. M., Bennion, D. H., et al. (2014). A scientific basis for restoring fish spawning habitat in the St. Clair and Detroit Rivers of the Laurentian Great Lakes. Restor. Ecol. 23, 149–156. doi: 10.1111/rec.12159
Marsh, J. E., Lauridsen, R. B., Gregory, S. D., Kratina, P., Scott, L. J., Cooling, D., et al. (2022). High summer macrophyte cover increases abundance, growth, and feeding of juvenile Atlantic salmon. Ecol. Appl. 32:e02492. doi: 10.1002/eap.2492
Miano, A. J., Leblanc, J. P., and Farrell, J. M. (2019). Laboratory evaluation of spawning substrate type on potential egg predation by round goby (Neogobius melanostomus). J. Great Lakes Res. 45, 390–393. doi: 10.1016/j.jglr.2019.02.002
Nash, K. T., Hendry, K., and Cragg-Hine, D. (1999). The use of brushwood bundles as fish spawning media. Fish. Manag. Ecol. 6, 349–356. doi: 10.1046/j.1365-2400.1999.00153.x
National Institute of Health. (2008). ImageJ Version 1.38x, Wayne Rasband. USA: National Institute of Health. Available at: https://imagej.nih.gov/ij/
Origin Lab Corporation. (2017). Origin Pro, version 2017C. Northampton, Massachusetts, USA. Available at: https://www.originlab.com/
Palm, D., Brännäs, E., Lepori, F., Nilsson, K., and Stridsman, S. (2007). The influence of spawning habitat restoration on juvenile brown trout (Salmo trutta) density. Can. J. Fish. Aquat. Sci. 64, 509–515. doi: 10.1139/f07-027
Peng, P., Li, Y. F., and Li, X. H. (2016). Effect evaluation of artificial fishnest on common carp (Cyprinus carpio) in Xijiang river. Freshwater Fisheries (In Chinese with English abstract) 46, 45–49. doi: 10.3969/j.issn.1000-6907.2016.06.008
Pavlov, D. S., Borisenko, E. S., and Pashin, V. M. (2009). Investigations of spawning migration and assessment of abundance of the Kamchatka steelhead (Parasalmo mykiss) from the Utkholok River by means of Didson dual-frequency identification sonar. J. Ichthyol. 49, 1042–1064. doi: 10.1134/S0032945209110046
Pawlikowski, K., and Kornijów, R. (2019). Role of macrophytes in structuring littoral habitats in the Vistula lagoon (southern Baltic Sea). Oceanologia 61, 26–37. doi: 10.1016/j.oceano.2018.05.003
R Core Team. (2018). R: a language and environment for statistical computing, reference index version 3.5.2. Vienna, Austria: R Foundation for Statistical Computing. Available at: https://www.R-project.org/
Rastoin-Laplane, E., Goetze, J., Harvey, E. S., Acuna-Marrero, D., Fernique, P., and Salinas-de-Leon, P. (2020). A diver operated stereo-video approach for characterizing reef fish spawning aggregations: the Galapagos marine reserve as case study. Estuarine Coast. Shelf Sci. 243:106629. doi: 10.1016/j.ecss.2020.106629
Riebe, C. S., Sklar, L. S., Overstreet, B. T., and Wooster, J. K. (2014). Optimal reproduction in salmon spawning substrates linked to grain size and fish length. Water Resour. Res. 50, 898–918. doi: 10.1002/2013WR014231
Santos, L. N., Araújo, F. G., and Brotto, D. S. (2008). Artificial structures as tools for fish habitat rehabilitation in a neotropical reservoir. Aquat. Conserv. Mar. Freshwat. Ecosyst. 18, 896–908. doi: 10.1002/aqc.931
Schlumberger, O., and Proteau, J. P. (1996). Reproduction of pike-perch (Stizostedion lucioperca) in captivity. J. Appl. Ichthyol. 12, 149–152. doi: 10.1111/j.1439-0426.1996.tb00080.x
Slagle, Z. J., and Allen, M. S. (2018). Should we plant macrophytes? Restored habitat use by the fish community of Lake Apopka, Florida. Lake Reserv. Manag. 34, 296–305. doi: 10.1080/10402381.2018.1443179
Smith, C. S., Paxton, A. B., Donaher, S. E., Kochan, D. P., Neylan, I. P., Pfeifer, T., et al. (2021). Acoustic camera and net surveys reveal that nursery enhancement at living shorelines may be restricted to the marsh platform. Ecol. Eng. 166:106232. doi: 10.1016/j.ecoleng.2021.106232
Sound Metrics. (2019). ARISFish Software User Guide version 2.6.2. Bellevue, WA: Sound Metrics. Available at: https://www.soundmetrics.com/
Statistical Package for Social Sciences (SPSS). (2010). Version 19.0. Armonk, NY: SPSS Inc, IBM Corporation. Available at: https://www-01.ibm.com/software/analytic/spss/
von Nordheim, L., Kotterba, P., Moll, D., and Polte, P. (2018). Impact of spawning substrate complexity on egg survival of Atlantic herring (Clupea harengus, L.) in the Baltic Sea. Estuar. Coasts 41, 549–559. doi: 10.1007/s12237-017-0283-5
Wang, Q., Li, Z., Lin, M., Ye, S., Li, W., Guo, C., et al. (2019). A changed post-flood management strategy enables the culture-based fishery of the Liangzi Lake, Yangtze River basin, China, to remain economically and environmentally viable. Fish. Manag. Ecol. 26, 548–557. doi: 10.1111/fme.12386
Wang, H., Wang, P., Xu, C., Sun, Y., Shi, L., Zhou, L., et al. (2022). Can the “10-year fishing ban” rescue biodiversity of the Yangtze River? Innovation 3:100235. doi: 10.1016/j.xinn.2022.100235
Whiterod, N. S., Brown, L., Bachmann, M., Farrington, L., and Vilizzi, L. (2021). Long and lasting: spatial patterns and temporal trends in a fish community responding to landscape-scale hydrological restoration of a coastal freshwater wetland complex. Landsc. Ecol. 36, 1511–1532. doi: 10.1007/s10980-021-01219-5
Winfield, I. J. (1986). The influence of simulated aquatic macrophytes on the zooplankton consumption rate of juvenile roach, Rutilus rutilus, Rudd, Scardinius erythrophthalmus, and perch, Perca fluviatilis. J. Fish Biol. 29, 37–48. doi: 10.1111/j.1095-8649.1986.tb04997.x
Xie, S., Cui, Y., and Li, Z. (2001). Small fish communities in two regions of the Liangzi Lake, China, with or without submersed macrophytes. J. Appl. Ichthyol. 17, 89–92. doi: 10.1046/j.1439-0426.2001.00248.x
Ye, S., Li, Z., Lek-Ang, S., Feng, G., Lek, S., and Cao, W. (2006). Community structure of small fishes in a shallow macrophytic Lake (Niushan lake) along the middle reach of the Yangtze River, China. Aquat. Living Resour. 19, 349–359. doi: 10.1051/alr:2007005
Zarski, D., Horvath, A., Held, J. A., and Kucharczyk, D. (2015). “Artificial reproduction of percid fishes” in Biology and Culture of Percid Fishes. eds. P. Kestemont, K. Dabrowski, and R. C. Summerfelt (Dordrecht: Springer) doi: 10.1007/978-94-017-7227-3_4
Zeng, L., He, F., Dai, Z. G., Xu, D., Liu, B. Y., Zhou, Q. H., et al. (2017). Effect of submerged macrophyte restoration on improving aquatic ecosystem in a subtropical, shallow lake. Ecol. Eng. 106, 578–587. doi: 10.1016/j.ecoleng.2017.05.018
Zhadan, P. M., Vaschenko, M. A., and Ryazanov, S. D. (2018). Assessing the effect of environmental factors on the spawning activity of the sea urchin Strongylocentrotus intermedius through video recording observations. Mar. Ecol. Prog. Ser. 588, 101–119. doi: 10.3354/meps12436
Keywords: artificial spawning ground, Liangzi Lake, spawning behavior, phytophilic spawners, fishery resources, habitat restoration
Citation: Feng K, Yuan J, Zhang Y, Qian J, Liu J, Li Z, Lek S and Wang Q (2023) Application of artificial spawning substrates to support lacustrine fish recruitment and fisheries enhancement in a Chinese lake. Front. Ecol. Evol. 10:1062612. doi: 10.3389/fevo.2022.1062612
Edited by:
Naicheng Wu, Ningbo University, ChinaReviewed by:
José Lino Vieira De Oliveira Costa, University of Lisbon, PortugalBinsong Jin, Hangzhou Normal University, China
Copyright © 2023 Feng, Yuan, Zhang, Qian, Liu, Li, Lek and Wang. This is an open-access article distributed under the terms of the Creative Commons Attribution License (CC BY). The use, distribution or reproduction in other forums is permitted, provided the original author(s) and the copyright owner(s) are credited and that the original publication in this journal is cited, in accordance with accepted academic practice. No use, distribution or reproduction is permitted which does not comply with these terms.
*Correspondence: Qidong Wang, ✉ d2FuZ3FkQGloYi5hYy5jbg==