- 1Department of Geosciences, University of Cincinnati, Cincinnati, OH, United States
- 2Arctic National Wildlife Refuge, U.S. Fish and Wildlife Service, Fairbanks, AK, United States
- 3Department of Geosciences, University of Alaska Fairbanks and University of Alaska Museum, Fairbanks, AK, United States
Caribou (Rangifer tarandus) have among the longest annual migrations of any terrestrial mammal as they move from winter ranges to spring calving grounds. Biomonitoring records indicate broad consistencies in calving geography across the last several decades, but how long have herds used particular calving grounds? Furthermore, how representative are modern patterns of calving geography to periods that pre-date recent climatic perturbations and increased anthropogenic stresses? While modern ecological datasets are not long enough to address these questions, bones from past generations of caribou lying on the tundra provide unique opportunities to study historical calving geography. This is possible because female caribou shed their antlers within days of giving birth, releasing a skeletal indicator of calving. Today, the Coastal Plain of the Arctic National Wildlife Refuge (Alaska) is a key calving ground for the Porcupine Caribou Herd (PCH). To test the duration across which caribou have used this area as a calving ground, we radiocarbon dated three highly weathered female antlers collected from tundra surfaces on the Coastal Plain. Calibrated radiocarbon dates indicate that these antlers were shed between ~1,600 and more than 3,000 calendar years ago. The antiquity of these shed antlers provides the first physical evidence of calving activity on the PCH calving grounds from previous millennia, substantiating the long ecological legacy of the Coastal Plain as a caribou calving ground. Comparisons to published lake core records also reveal that dates of two of the antlers correspond to periods with average summer temperatures that were warmer than has been typical during the last several decades of biomonitoring. This finding expands the range of climatic settings in which caribou are known to use the current PCH calving grounds and suggests that the Coastal Plain of the Arctic Refuge may remain an important caribou calving ground during at least portions of predicted future warming. Discarded skeletal materials provide opportunities to assess the historical states of living populations, including aspects of reproductive biology and migration. Particularly in high-latitude settings, these insights can extend across millennia and offer rare glimpses into the past that can inform current and future management policies.
Introduction
For migratory populations of caribou (Rangifer tarandus), a key characteristic of their ecology and population biology is the annual journey to areas where they give birth in the spring: their calving grounds (Griffith et al., 2002; Nicholson et al., 2016; Cameron et al., 2020). Migrations to and from the calving grounds represent significant energy expenditures, but have multiple hypothesized benefits that increase calf survival and maintain the population. These include calving in areas with reduced predator pressure, abundant early-greening spring vegetation, and that are in close proximity to habitats used for insect avoidance later in the spring/summer (Griffith et al., 2002; Russell and Gunn, 2019). Thus, maintaining access to spring calving grounds and associated migration routes are top management and conservation priorities that are generally considered critical for maintaining herd viability (Griffith et al., 2002; Gunn et al., 2009; Russell and Gunn, 2019; Joly et al., 2021a). However, migratory patterns of caribou and their seasonal landscape use are sensitive to a variety of factors including annual weather patterns, larger-scale shifts in climate, changes in herd size, development of roads and industrial infrastructure, and a variety of other ecological and anthropogenic influences (Skoog, 1968; Russell et al., 1993; Cameron et al., 2005, 2020; Hinkes et al., 2005; Vistnes and Nellemann, 2008; Vors and Boyce, 2009; Joly et al., 2011). Over the last several decades of climatic and ecological changes across the Arctic, herds such as the Porcupine Caribou Herd (PCH) have demonstrated high annual variability in calving ground geography (Griffith et al., 2002; Cameron et al., 2005; Caikoski, 2020). As biologists and wildlife agencies establish conservation and management goals designed to insulate herds from future ecological stresses, several fundamental questions remain unanswered. How long have herds (or caribou more broadly) utilized particular calving grounds and how well do contemporary patterns of seasonal landscape use and caribou migration correspond to those under different regimes of climate and anthropogenic influences? While the timescales inherent to these questions far outstrip those available from traditional ecological datasets, antlers shed from female caribou provide useful proxies for assessing historical calving ground geography (Miller and Barry, 1992; Miller et al., 2013, 2021). This is possible because female caribou shed their antlers around the time they give birth (Espmark, 1971; Bubenik et al., 1997) and those antlers can persist for hundreds to thousands of years on Arctic landscapes (Sutcliffe and Blake, 2000; Stewart and England, 2008; Le Moullec et al., 2019; Miller and Simpson, 2022). Here, by radiocarbon dating antlers collected from tundra surfaces in Alaska, we test the long-term persistence of calving activity on the modern calving grounds of the PCH. In so doing, we document calving activity on the Coastal Plain of the Arctic National Wildlife Refuge across more than 3,000 years.
Shed female caribou antlers lying on landscape surfaces provide evidence of past calving activity. This is possible because female caribou, like their male counterparts, annually grow and shed antlers (Espmark, 1971; Bergerud, 1976). However, the schedules of antler growth and shedding are different between males and females (Bergerud, 1976; Bubenik et al., 1997). Females generally grow antlers in the spring/summer and maintain their antlers until shedding them within days of giving birth in the spring (Espmark, 1971; Bergerud, 1976; Whitten, 1995). Males, on the other hand, shed their antlers in the late fall and early winter (Bergerud, 1976). Because calving is highly synchronized among caribou and migratory caribou travel in large herds to the calving grounds (103 to 104 individuals), annual inputs of shed female caribou antlers can accumulate over time to produce large concentrations of shed antlers (~1,000 antlers/km2, Cameron and Whitten, 1979; Griffith et al., 2002; Joly et al., 2011; Miller et al., 2013). As antlers slowly decay across decades to centuries or more, they become increasingly weathered (Meldgaard, 1986; Sutcliffe and Blake, 2000; Miller et al., 2021; Miller and Simpson, 2022). Because progressive changes in weathering provide useful information about their time-since-shed, accumulations of shed antlers offer opportunities to evaluate changes in seasonal landscape use, including calving activity, across space and through time (Behrensmeyer, 1978; Miller, 2012; Miller et al., 2013, 2021).
Today, the Coastal Plain of the Arctic Refuge primarily serves as a calving ground for the PCH (Figure 1; Griffith et al., 2002). The Coastal Plain is a flat region of tundra between the north side of the Brooks Range and the coast of the Beaufort Sea (Griffith et al., 2002; Jorgenson et al., 2002). The PCH calve throughout most of the Coastal Plain of the Arctic Refuge and Ivvavik National Park, Canada, immediately east of the Arctic Refuge (Fancy and Whitten, 1991; Griffith et al., 2002). The PCH is currently one of the largest caribou herds in the world (~218,000 individuals in 2017; Caikoski, 2020) and has one of the longest annual migrations of any terrestrial mammal (~1,350 km straight-line round-trip distance; Teitelbaum et al., 2015; Joly et al., 2019), which takes them between their winter range and spring calving grounds. The PCH has been studied for decades by academic, state, territorial, and federal wildlife biologists and an important aspect of that work has been assessing variability in calving ground geography through time (Russell et al., 1993, 2005; Griffith et al., 2002; Russell and Gunn, 2019; Caikoski, 2020). Evaluating the drivers behind annual variation and/or long-term consistency in calving geography has been a research focus due, in part, to industry and political interests in developing portions of their calving ground for hydrocarbon extraction (Whitten, 1994; Griffith et al., 2002; Gunn et al., 2009; Russell and Gunn, 2019). Referred to as the “1002 Area,” this portion of Coastal Plain was set aside by the U.S. Congress in 1980 for potential development (Udall, 1980). Petroleum lease sales were eventually sold in 2020 following a congressional mandate (Brady, 2017), though as of 2022, infrastructure to support hydrocarbon extraction has not been developed.
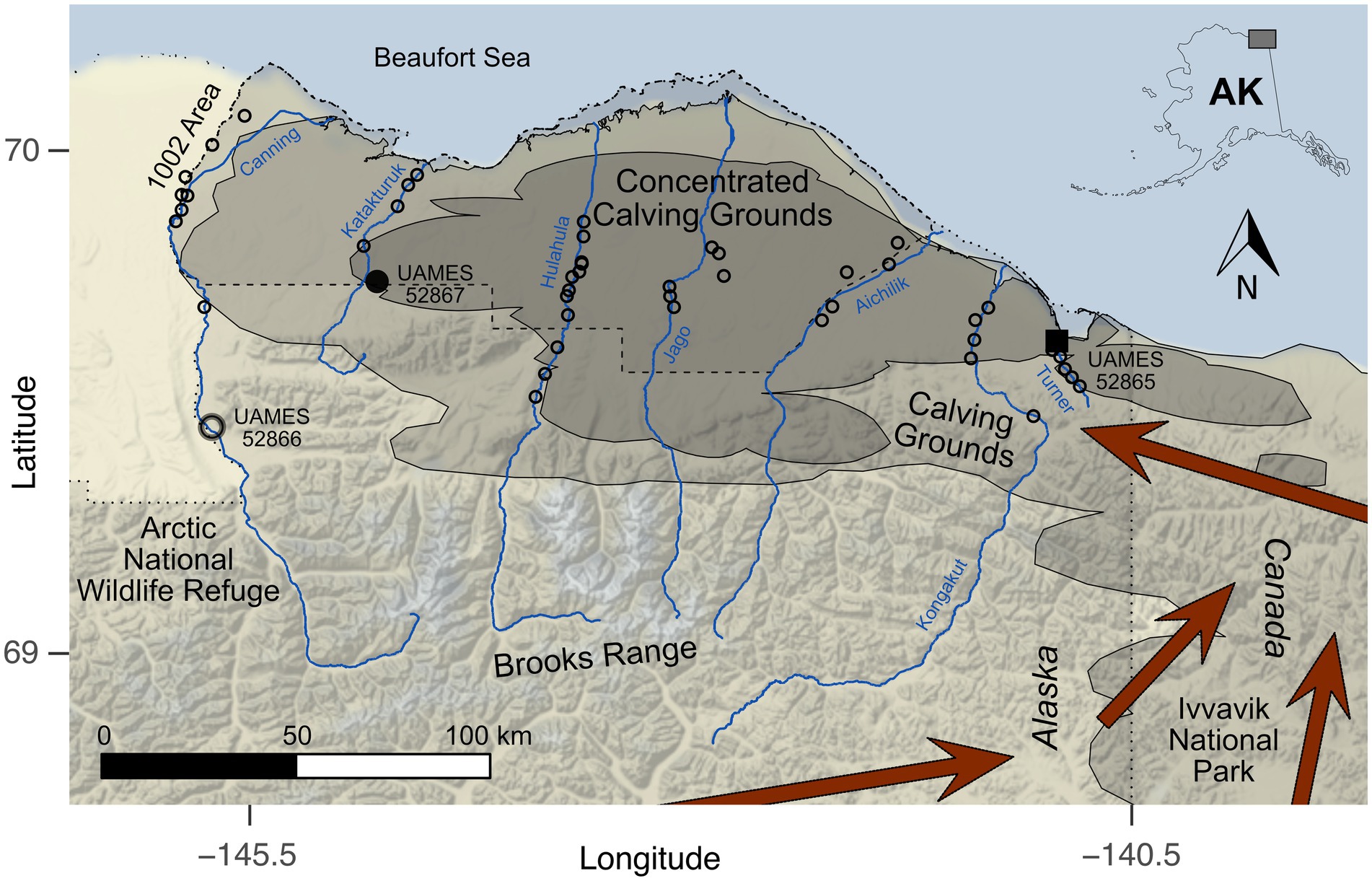
Figure 1. Map of the calving grounds (Alaskan) and nearby environments for the Porcupine Caribou Herd (PCH). Demarcation of the PCH calving grounds (lightly shaded region) and concentrated calving grounds (darker shaded region) are based on monitoring records (Griffith et al., 2002; Caikoski, 2020). Boundary of the Arctic National Wildlife Refuge and the “1002 Area” identified by dotted and dashed lines, respectively. Blue lines identify rivers used as transportation corridors during antler surveys: Canning, Katakturuk, Hulahula, Jago, Aichilik, Kongakut, and Turner. Locations of radiocarbon dated antlers identified by a large open circle (UAMES 52866), a large filled circle (UAMES 52867), and a large filled square (UAMES 52865). Locations of additional antler surveys are shown as small open circles. Dark red arrows identify generalized major spring migration routes to the calving grounds (Fancy et al., 1989).
Calving ground geography for migratory caribou is a tale of two temporal scales. Survey data often show high annual variability in calving locations, with the centers of annual calving grounds differing from year to year across dozens to over 100 km (Griffith et al., 2002; Baltensperger and Joly, 2019; Cameron et al., 2020). At the same time, those annual shifts occur within a broader area that defines an overall “calving ground,” which is often stable across decadal timescales (Griffith et al., 2002; Cameron et al., 2020; Joly et al., 2021b). But what about longer timescales? The vast majority of data on calving grounds of migratory caribou are from the 1980s onward, with sparser data from the 1970s, 1960s, and earlier (Skoog, 1968; Whitten et al., 1984, 1992; Fancy et al., 1989; Fancy and Whitten, 1991; Bergerud, 1996; Griffith et al., 2002; Burch, 2012; Porcupine Caribou Management Board [PCMB], 2016; Benson, 2019). Consequently, these records overlap periods of increasing climate change and anthropogenic pressures. This issue is highlighted by the Central Arctic Herd, which mostly calves to the west of the Arctic Refuge. The Central Arctic Herd was recognized as a herd only in the mid-1970s, but its geographic range had been under development for petroleum resources (including the Trans-Alaska Pipeline) since the 1960s (Cameron and Whitten, 1979; Fancy, 1983; Cameron et al., 1992, 2005; Lenart, 2015; Nicholson et al., 2016; Johnson et al., 2020). Caribou ecology and seasonal landscape use are also influenced by shifts in climate and associated environmental changes, which have been particularly severe in the Arctic (Griffith et al., 2002; Arctic Climate Impact Assessment [ACIA], 2004; Joly et al., 2011; Pearson et al., 2013; Rantanen et al., 2022). Yet, again, observations of calving geography, particularly more standardized monitoring programs that started in the 1970s and 1980s, were initiated after Arctic climates had already started changing. In some cases, records from early European explorers and hunters provide glimpses into historical migrations and calving ground geographies, but such insights are generally based on limited observations with poor spatial resolution (Burch Jr., 2012; Benson, 2019). Oral histories and Traditional Knowledge of Native Alaskans and Canadians are another source of historical insight, though groups such as the Gwich’in traditionally stayed away from the calving grounds and so have restricted knowledge of their historical geographies (Benson, 2019). Although challenging to establish, expanding the timescales across which we study caribou calving geography and migration can help us evaluate the biological significance of modern calving grounds and test for changes associated with recent climatic and anthropogenic perturbations (Miller et al., 2021). Here, we use ancient antlers to expand the time series with which we assess calving ground geography for the PCH and to test how well contemporary patterns of seasonal landscape use are reflected in the deeper past.
Materials and methods
Antlers used for this study came from broader sampling efforts to study historical caribou landscape use (Miller et al., 2013, 2021; Miller and Simpson, 2022). Shed antlers reported here were found exposed on landscape surfaces on the Coastal Plain of the Arctic Refuge in the summers of 2011, 2012, and 2018. Two of the antlers were discovered during standardized surveys of well-drained, cobble-rich tundra habitats with abundant Dryas, a low-growing evergreen dwarf shrub (i.e., Dryas river terraces, sensu Jorgenson et al., 2002; Jorgenson and Walker, 2018). One of the antlers came from a gravel bar that was discovered while walking between surveys. To sample Dryas terraces across the Coastal Plain of the Arctic Refuge, we traveled along major river systems that bisect the Coastal Plain from south to north (Miller et al., 2013, 2021). Targeting Dryas terrace habitats, we conducted 50 antler surveys near the Canning, Katakturuk, Hulahula, Jago, Aichilik, Kongakut, and Turner Rivers (Figure 1). Using each river, we sampled from the northern foothills of the Brooks Range to as close to the Beaufort Sea as was logistically possible. Across the region, we sampled areas that are currently within the PCH calving grounds as well as areas not currently known to support calving. Survey sites were accessed by a combination of fixed-wing aircraft, helicopter, or by rafting down rivers to survey locations. Because vegetation on Dryas terraces is sparse and/or low-growing (with few tussocks, willows, or other vegetation that would be visually obstructive), surveyors had clear views of the ground and any antlers in the area (Miller et al., 2013, 2021). All antlers evaluated here were at least partially exposed on landscape surfaces. Surveys and collections were done with permission from, and in collaboration with, the Arctic National Wildlife Refuge (United States Fish and Wildlife Service). The antlers are accessioned into the University of Alaska Museum Earth Sciences Collection (UAMES) at the University of Alaska Fairbanks.
Shed antlers can be discriminated from antlers introduced due to death by the presence of an exposed antler-skull attachment surface (Miller, 2012; Miller et al., 2013). That “pedicle attachment surface” becomes smooth and rounded as the antler detaches from the skull during the cell death processes that lead to shedding (Lincoln and Tyler, 1994; Bubenik et al., 1997). To differentiate female antlers from male antlers, we took advantage of known differences in surface areas between the pedicle attachment surfaces of males and females (females are generally smaller, Miller et al., 2013, 2021). Previous work using museum specimens with known sex has shown that while there is some overlap between pedicle surface areas of older females and younger males, such overlap is limited. Thus, surface area of the pedicle attachment surface offers a useful method for differentiating antler sex for most caribou antlers found on the Coastal Plain (Miller et al., 2013, 2021). Pedicle attachments are approximately elliptical, thus we estimated surface area using the long axis (A1) and short axis (A2) of the pedicle attachment surface (measured using IP67 Absolute Mitutoyo digital calipers) and the standard formula for estimating surface area of an ellipse: π * (½ * A1) * (½ * A2).
Based on the known size frequency distributions of pedicle surface areas of male and female antlers, we used a previously developed Monte Carlo method to calculate the probability (mean and 95% confidence interval) that each antler evaluated here was female (Miller et al., 2013, 2021). The surface areas of all antler pedicle attachments in this study were quite small, indicating the antlers were definitively female. We measured the length of each specimen by following the curvature of the antler fragment using a flexible plastic measuring tape.
We used Accelerator Mass Spectrometry (AMS) radiocarbon dating to estimate when each antler was grown, which is roughly synchronous with when the antler was shed. Radiocarbon dating measures the amount of 14C in a sample relative to that of atmospheric radiocarbon in 1950 and can be used to estimate the ages when biological specimens formed across roughly the last 50,000 years (Hajdas et al., 2021). To radiocarbon date the antlers, we sampled bone from the proximal portion of the main antler beam. This region has dense cortical bone and should be most resistant to diagenetic processes and infiltration of younger carbon (Miller et al., 2021). Antlers were cut using a low-speed Dremel with a diamond cutting wheel. Samples were broken into small chips and sent to the Keck-Carbon Cycle AMS Facility at the University of California, Irvine or the Center for Accelerator Mass Spectrometry at Lawrence Livermore National Laboratory for chemical pretreatment and AMS radiocarbon dating. Collagen was prepared using standard treatments for demineralization and humic acid removal (Beaumont et al., 2010; Crowley et al., 2010), ultrafiltered, and lyophilized. For the one sample sent to the Keck-Carbon Cycle AMS Facility (UAMES 52867), lipids were removed by sonicating antler chips in a 2:1 chloroform/methanol solution for 30 min prior to demineralization. This was repeated with a fresh chloroform/methanol solution until the solution was no longer cloudy. The sample was then sonicated for 30 min in methanol and 30 min in Milli-Q water. The sonicator bath was cooled to keep the temperature below 60°C. For the two antlers dated at Lawrence Livermore, lipids were not removed prior to dating. To account for isotopic fractionation, the δ13C of a sample must first be normalized to a standard prior to calculating its radiocarbon age (Stuiver and Polach, 1977; Hajdas et al., 2021). We previously reported no difference in δ13C values for antlers with or without lipid removal (p = 0.81, Miller et al., 2021), suggesting differences in preparation should be of negligible concern for specimen radiocarbon dates and their comparisons. To evaluate the quality of collagen preservation, and thus the reliability of the radiocarbon dates, we measured the atomic C:N ratios of each specimen, which are generally between 3.1 and 3.5 for well-preserved specimens (DeNiro, 1985; Ambrose, 1990; van Klinken, 1999). We calibrated radiocarbon dates to calendar years using IntCal20 (Reimer et al., 2020) and the “rcarbon” package (version 1.4.2, Crema and Bevan, 2021) in R (version 4.1.1, R Core Team, 2021). Calibrated radiocarbon dates produce a probability distribution of possible ages. We summarized each antler’s calibrated age as the weighted-median of its age distribution along with the range (minimum and maximum ages) of the 2-sigma 95% confidence interval of that distribution (Telford et al., 2004; Crema and Bevan, 2021).
Results
Antler specimens described below came from near three rivers: Canning, Katakturuk, and Turner. Each specimen had pedicle attachment surfaces that were intact and calibrated radiocarbon dates older than 1,000 calendar years before present (BP; where “present” is standardized to 1950). C:N ratios (ranging from 3.18 to 3.27; Table 1) are all within the expected range for well-preserved collagen (DeNiro, 1985; Ambrose, 1990; van Klinken, 1999). Each antler is described below.
UAMES 52867 (Figure 1, large filled circle; Figure 2A) was recovered during a standardized antler survey on a Dryas terrace in a slightly upland region near the Katakturuk River. The antler fragment extends approximately 151 mm from the pedicle attachment surface and includes part of the brow tine. The pedicle attachment surface is small and confidently female (93.2%; Table 1). The pedicle attachment surface is well defined and smooth, indicating that the antler was shed during the life of the animal. When discovered, it was more than half buried in mineral-rich soils that were adjacent to the mound of a ground squirrel (Urocitellus parryii). The antler’s exterior surfaces are well-weathered and have a fibrous texture (Figure 2A). Portions of the antler show signs of chemical maceration and dissolution. Lichen development on the antler is minimal, though moss was attached to the antler along many of the surfaces that were in contact with the soil. The weighted median calibrated date is 3,157 cal years BP (2-sigma range: 3,218–3,072 cal years BP; Table 2).
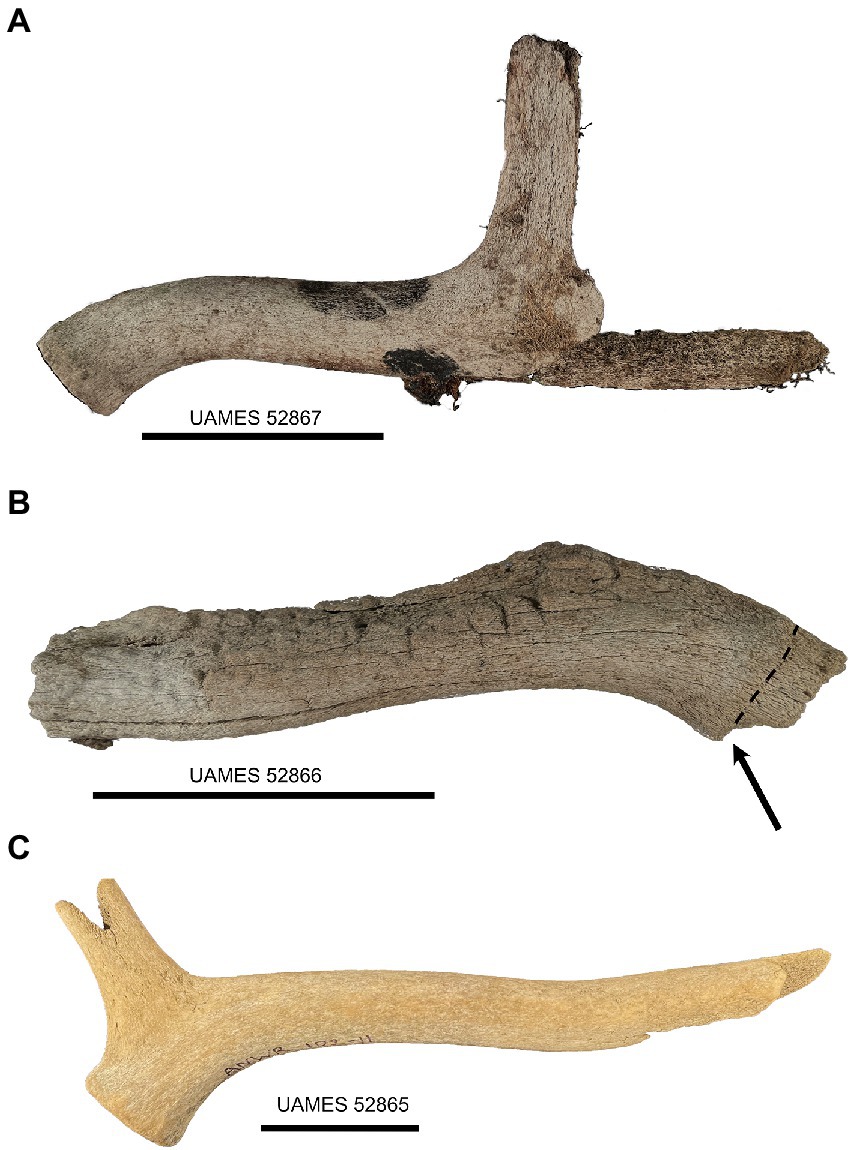
Figure 2. Plates of radiocarbon dated antlers. (A) UAMES 52867. (B) UAMES 52866; dashed line identifies well-delimited pedicle attachment surface; arrow identifies exposed attachment surface. (C) UAMES 52865. Scale bars are 5 cm.
UAMES 52866 (Figure 1, large open circle; Figure 2B) was found during a standardized antler survey on a Dryas terrace in an upland (near foothills) region near the Canning River. This antler fragment was discovered lying entirely exposed on the tundra. However, it was adjacent to a region that had been dug into (likely by a grizzly bear; Ursus arctos horribilis), so previous and perhaps relatively recent exhumation of the specimen cannot be excluded. Surfaces of the antler are acid etched with mm-scale curvilinear grooves consistent with damage by roots. No observable lichens were present. The antler fragment is 149 mm long and there is no indication of either brow or bez tines. UAMES 52866 has a small antler attachment surface and is confidently female (94.5%; Table 1). The antler includes a small portion of the cranial pedicle, indicating this antler was not shed during life. However, the pedicle attachment surface is well delineated (Figure 2B, dashed line) and portions of the pedicle are cleanly removed from the attachment surface (Figure 2B, arrow). Some areas at the pedicle attachment surface are not pristinely preserved and it is difficult to evaluate some of this specimen’s anatomy. The median calibrated date for UAMES 52866 is 2,581 cal years BP (2-sigma range: 2,714–2,494 cal years BP; Table 2).
UAMES 52865 (Figure 1, filled square; Figure 2C) was discovered while the team was walking between surveys along the Turner River. This specimen was found on a sand bar near the active portion of the channel. The antler fragment is 113 mm long and includes portions of a brow tine and the main antler beam. The antler is moderately rounded, indicating exposure to fluvial processes. The antler is also hollowed-out in some regions, which is also likely due to exposure to fluvial processes. Hollowing occurs along the main beam, brow tine, and into the base of the antler near the pedicle attachment surface. While the pedicle attachment surface is river-rounded, it is generally well-preserved and well-defined, indicating the antler was naturally shed. The pedicle attachment surface is small and confidently female (92.3%; Table 1). No observable lichens are present. While the original shedding location for this antler is unknown, the low gradient and sinuous nature of the Turner River suggests it was unlikely to have been transported over long distances. The Turner is also short (<20 km straight-line distance between its head waters and the Beaufort Sea) and the entire river courses through what are currently the calving grounds of the PCH. Thus, any degree of fluvial transportation is unlikely to substantially modify the spatial accuracy with which this specimen is used as a calving ground indicator. The calibrated radiocarbon date for this specimen is 1,629 cal years BP (2-sigma range: 1,704–1,549 cal years BP; Table 2).
Discussion
Ancient antlers highlight millennial-scale consistencies in calving ground geography
The antiquity (1,629 and 3,157 cal years BP) of the shed female caribou antlers recovered from the Coastal Plain of the Arctic Refuge, paired with the geography of the PCH calving grounds today (Figure 1; Griffith et al., 2002; Caikoski, 2020) indicates that caribou calves have been born in this region across at least several millennia. This historical contextualization provides a reference for the long-term importance of caribou calving habitats north of the Brooks Range. Additionally, the shed antlers were recovered from within what is currently recognized as the “concentrated calving grounds” in Alaska (Figure 1). The concentrated calving grounds, originally defined based on calving observations between 1983 and 2001, are portions of the overall calving grounds where females give birth in greater-than-average spatial concentrations (Griffith et al., 2002). Based on these higher frequencies of use, and data suggesting higher survivorship for calves born in portions of the concentrated calving grounds, preserving access to these high-quality calving habitats is a priority for PCH conservation and management (Griffith et al., 2002; Russell and Gunn, 2019). Our limited sample size prevents a detailed evaluation of the spatial distribution of ancient antlers, but recovering antlers from what are currently the concentrated calving grounds (and only from the concentrated calving grounds) that were shed by female caribou thousands of years ago bolsters the interpretation that the concentrated calving areas of the PCH delimit core caribou calving habitats.
Today, the foothills of the Brooks Range are used by caribou as gateways to the calving grounds, but they are not focal calving areas. This may be at least partially due to increased concentrations of mammalian predators in the highlands (Whitten et al., 1984, 1992; Fancy et al., 1989; Fancy and Whitten, 1991; Griffith et al., 2002). A lack of calving is particularly notable along the highlands near the western margin of the Arctic Refuge (Figure 1; near UAMES 52866). This behavioral pattern seems to be reflected by UAMES 52866, the skeletal remains of a female caribou that died in upland habitats near the Canning River over 2,500 years ago and preserves characteristics consistent with her traveling to the calving grounds. This antler fragment is still partially attached to a portion of the antler pedicle and is the only non-shed antler reported here. It is also the only antler found in the foothills of the Brooks Range (i.e., not from the lowland Coastal Plain). Apparent bone decalcification at the pedicle-antler attachment is consistent with death near the time she would have shed the antler. Thus, she was possibly within days to weeks of giving birth and was likely on her way to the calving grounds. While acknowledging our small sample size, it is notable that both shed antlers reported here were recovered from known calving grounds, while the single not-yet shed antler was recovered from an area that does not support calving today. Taken together, we find similarity between migration and calving ground patterns observed today (e.g., Griffith et al., 2002; Caikoski, 2020) and proxies of those behaviors from millennia ago. Our results are also consistent with oral histories of the Gwich’in people, which indicate that many patterns of landscape use observable today have historical roots, including calving grounds north of the Brooks Range (Benson, 2019).
Could the antlers (both shed and not-yet shed) have come from females that were not impregnated or experienced miscarriages prior to reaching the calving grounds? This seems unlikely, given their proximity to today’s calving grounds and the greater variability in when and where such nonparturient female caribou shed their antlers. Nonparturient females shed their antlers over a wide interval (across one or more months) in the late winter to earliest spring (Espmark, 1971; Bergerud, 1976; Bubenik et al., 1997). For the PCH, this would translate to antlers cast across nearly 100 km (perhaps more) of the migration on landscapes that could be more than 100 km farther south and/or east from the calving grounds sampled here (Fancy et al., 1989; Griffith et al., 2002). Some shifts in migratory patterns would not be unexpected across millennia. However, if the antlers dated here came from nonparturient caribou, this would suggest a dramatic reorganization of today’s migratory pathway, including either (1) arrival north of the Brooks Range up to several months earlier than observed today (and prior to green-up; Griffith et al., 2002) or (2) transition of the population to a non-migratory state. While additional work is required to understand the drivers of migration and changes in migratory dynamics among individuals or entire populations (Bergerud, 1996; Baltensperger and Joly, 2019; Joly et al., 2021b), invoking such a high level of migratory reorganization would be far less parsimonious than the more likely interpretation that these millennially-aged antlers were from parturient females that were on (UAMES 52867, UAMES 52865) or approaching (UAMES 52866) their calving grounds.
Beyond expanding the timescales across which calving behaviors can be inferred, millennially-aged antlers also provide insight into the diversity of climate settings in which the Arctic Refuge’s Coastal Plain has been used as a calving ground. Local climate records from the late Holocene are not available, but nearby lake cores from Hanging and Trout Lakes (Yukon, Canada; ~200 km from the Turner River) provide temperature reconstructions based on assemblages of fossil midges and floral community reconstructions based on pollen analyses (Cwynar, 1982; Kurek et al., 2009; Irvine et al., 2012; Kaufman et al., 2016). Starting with the oldest antler, ~3,000 cal years BP, both temperature records indicate mean July temperatures (summer temperatures) that were similar or slightly cooler than average modern summers (Kurek et al., 2009; Irvine et al., 2012; Kaufman et al., 2016). Both proxy records show an increase of ~1°C in summer temperatures by ~2,500 cal years BP (the age of the non-shed antler). By ~1,600 cal years BP, summer temperatures were similarly high, ranging between ~0.5 and > 1°C above the ~3,000 cal years BP starting point. While error bars on these estimates are large, the trajectories of temperature changes are consistent between cores (Kurek et al., 2009; Irvine et al., 2012; Kaufman et al., 2016). Neither records shows dramatic changes in floral community between 1,000 and 3,000 years ago, though these very recent portions of the records were not the main research foci. Antlers shed in the PCH calving grounds thousands of years ago indicate that the Coastal Plain can serve as a calving ground across a variety of climatic settings, including those with warmer summer temperatures than observed in recent decades. While the predicted trajectories of future climate change generally fall outside baselines established by climate records from the last several millennia (Arctic Climate Impact Assessment [ACIA], 2004; Kaufman et al., 2020; IPCC, 2022), it seems likely that the Coastal Plain of the Arctic Refuge will remain a viable calving ground for at least the next stages of the warming Arctic. But across how much warming may current patterns of caribou migration persist and what will ultimately drive future changes, if they occur? Pairing analyses of sediment cores at higher temporal resolutions with continued sampling of antler records may provide informative tests for how previous shifts in temperature and floral communities impacted the geographic distribution of caribou calving grounds and other aspects of caribou migration. In this way, historical proxies could provide more detailed guidance and expectations for how to manage caribou amidst future ecological change.
Inefficient recycling of antlers contributes to a redistribution of limiting nutrients across Arctic habitats
Vertebrates are important vectors of nutrient redistribution in a variety of biological systems (Doughty et al., 2013, 2016; Doughty, 2017), but the redistribution of nutrients driven by antler growth and shedding of migratory caribou is underappreciated. Antlers are composed of bone apatite and, as such, are rich in bioavailable calcium and phosphorous. Phosphorous, in particular, is a limiting nutrient in many settings, including Arctic ecosystems (Chapin et al., 1978; Weintraub, 2011). Due to distinct seasonal ranges, migratory caribou grow antlers in different areas from where they are shed (Miller et al., 2021). This is true for both male and female caribou, which grow and shed antlers according to different schedules (Espmark, 1971; Bergerud, 1976; Bubenik et al., 1997). Caribou in the PCH are in a near constant state of movement, which is broadly true for individuals in migratory herds (Fancy et al., 1989; Griffith et al., 2002; Joly et al., 2021a). An exception to this comes immediately following the birth of their calves (generally around June 1 for the PCH; Griffith et al., 2002), after which daily movement rates of female caribou are dramatically reduced. But daily travel increases quickly as calves gain strength (Fancy and Whitten, 1991; Griffith et al., 2002) and some calves are traveling as much as 90 km/day by the end of June and early July (Griffith et al., 2002). Overall, Porcupine Caribou stay on their calving grounds between ~1 to up to ~2 months. Due to their high mobility, females that successfully calved in the spring will start to grow new antlers at least 10s of kilometers away from those calving locations. Antlers will continue to grow for the next several months, peaking in growth rate during the summer and completing antler mineralization by the fall, when they are far (>100 km) from the calving grounds (Bergerud, 1976; Fancy et al., 1989; Griffith et al., 2002). Caribou also mobilize their own skeletal resources to help build antlers (Baksi and Newbrey, 1989; Baxter et al., 1999). Thus, mineral resources contributing to antlers come from a complex mixture of geographies that are largely or entirely allochthonous to the location where they are eventually shed.
A formal calculation of the impact of this nutrient redistribution is beyond the scope of this paper, but we can at least establish some basic expectations of this phosphorous conveyor belt. The size of the PCH continuously fluctuates, but it was last estimated at 218,000 individuals (Caikoski, 2020). While herd demographics change through time, ~82% of the herd are adults (Caikoski, 2020), ~52% of herd adults are female (Caikoski, 2020) and the vast majority (95%) of female caribou grow antlers each year (Cronin et al., 2003). Thus, in the last several years, we can expect ~176,615 female antlers introduced to the system each year. The size of female caribou antlers change with ontogeny, but if an average female caribou antler is ~300 grams (Thomas and Barry, 2010) and phosphorous accounts for approximately 84 mg/g of cervid antlers (Nowicka et al., 2006), that equates to ~4,451 kg of phosphorous redistributed annually in the form of antlers of PCH females. While the majority of these inputs will fall onto the calving grounds, the complete picture of mineral redistribution is more complex because females that do not become pregnant or miscarry their calves will shed their antlers earlier than pregnant females, when they are at different points along their migration (Bergerud, 1976).
What becomes of all the phosphorous and calcium introduced to the calving grounds by shed antlers? On the Coastal Plain, phosphorous availability fluctuates seasonally for both plants and the vertebrates that feed on them (Chapin et al., 1978; Weintraub, 2011; Oster et al., 2018). For example, during early spring, the daily calcium requirements for a lactating female caribou are not easily obtainable from available graminoid forage on the spring ranges of the PCH. By late spring and summer, available forage is essentially unable to accommodate the daily phosphorus requirements for a lactating female (Oster et al., 2018). Antlers and other bones provide an alternative source of both phosphorus and calcium and, as documented in other settings, are gnawed on by a variety of Arctic mammals, including caribou and rodents (McCabe, 1957; Gordon, 1976; Fernandez-Jalvo and Andrews, 2016). As bones weather and decay, they will also release calcium and phosphorous into nearby soils. More detailed analyses of antler loss rates due to weathering and consumption by local mammals will be critical to estimating the rates by which key nutrients are returned to the system. But high concentrations of antlers on Coastal Plain calving grounds (>1,000 antlers/km2, Miller et al., 2013) paired with the persistence of antlers on these same landscapes for up to thousands of years indicates that caribou calving grounds act as a sink for phosphorous, calcium, and other nutrients locked away in shed antlers.
Slow bone decomposition produces time-rich datasets for conservation paleobiological applications
Recovering millennially-aged antlers lying on the Coastal Plain of the Arctic Refuge highlights the slow weathering and recycling rates of bones and other biological materials in high-latitude, cold-weather environments. Bones and antlers of similar antiquity have been previously recovered from landscapes on Ellesmere Island, Svalbard, Greenland, and Antarctica, all of which have been used to explore the historical presence, geographic distribution, and population biology of caribou and other species (Olson and Broecker, 1961; Nichols, 1968; Meldgaard, 1986; Sutcliffe and Blake, 2000; Koch et al., 2019; Le Moullec et al., 2019). The consistency with which millennially-aged bones have been collected from the surfaces of Arctic and Antarctic landscapes is good news for those interested in assessing the historical states of large mammals in these settings. However, while Arctic and Antarctic settings consistently yield bones from deep antiquity, the rates of bone recycling in lower latitudes are faster and yield narrower temporal perspectives (Miller and Simpson, 2022).
Bone weathering and loss rates are controlled by a variety of factors, including soil moisture, temperature, UV-incidence, and cycles such as wet-dry and freeze–thaw (Behrensmeyer, 1978; Tappen, 1994; Fiorillo, 1995; Todisco and Monchot, 2008; Behrensmeyer and Miller, 2012; Pokines et al., 2016, 2018; Stokes et al., 2020). Damage to bones due to inadvertent modification and/or direct consumption by carnivores, rodents, and ungulates can also play important roles (Pobiner, 2008; Fernandez-Jalvo and Andrews, 2016; Pobiner et al., 2020). In temperate North America (Yellowstone National Park, United States), bones of large mammals (e.g., elk, horse; Cervus canadensis, Equus ferus caballus) may persist for hundreds of years on landscape surfaces (Miller, 2011; Miller and Simpson, 2022). But in Amboseli National Park (Kenya), bones of large mammals may persist for only several decades (Behrensmeyer, 1978; Behrensmeyer and Miller, 2012). Clearly the depth of historical insight available from accumulations of bones in Arctic, temperate, and tropical settings is very different, which means the conservation and management-related questions we ask of these accumulations must be temporally appropriate. Unfortunately, the number of ecosystems that may benefit from the historical ecological data available in bone accumulations is far larger than the number of sites for which we have estimated the duration of bone persistence. A recent study (Miller and Simpson, 2022), however, which included antler UAMES 52867, found that the duration of maximum bone persistence (logged) in different environments is closely linked to that environment’s mean annual temperature (p < 0.01, r2adjust = 0.95). Using this relationship, it is possible to estimate the likely depth of historical insight available from bones at a given locality. While estimating maximum bone persistence using a single variable may be an oversimplification, it can serve as a useful starting point for establishing initial expectations for the temporal richnesses of bone accumulations that are waiting to be studied on different landscapes.
Conclusion
Today, the PCH gives birth to their calves on the Coastal Plain of the Arctic National Wildlife Refuge (Alaska) and Ivvavik National Park (Yukon, Canada). While Traditional knowledge of the Gwich’in people and historical records of early European explorers and trappers indicate similarity in landscape use across at least hundreds of years (Burch Jr., 2012; Benson, 2019), the long-term continuity of contemporary PCH calving grounds remains unknown. Female caribou shed their antlers within days of giving birth and serve as a useful proxy for historical calving activity (Espmark, 1971; Whitten, 1995; Miller et al., 2021). Radiocarbon dates of antlers found on the PCH calving grounds reveal shed antlers as old as 1,629 and 3,157 cal years BP. The antiquity of these shed antlers provides the first physical evidence of calving activity from previous millennia and substantiates the long ecological legacy of the Coastal Plain as a caribou calving ground. Some of the ancient antlers evaluated here also come from periods when summer temperatures were higher than recent decades, indicating that the PCH calving ground may be viable for at least the next few stages of projected climate warming. Millennial-aged antlers have been recovered from multiple high latitude settings in the northern and southern hemispheres (Miller and Simpson, 2022), highlighting the extended ecological histories available from bone accumulations in cold-weather environments. Extended persistence of antlers on Arctic caribou calving grounds also indicates that recycling nutrients from caribou antlers (phosphorus, calcium) is highly inefficient. Paired with large annual inputs of antlers to the calving grounds (105 antlers per year), we also find that caribou calving grounds are an underappreciated sink for nutrients that are important to both plant and animal communities. Evaluating antlers (and other bones) on modern landscapes provides important opportunities to evaluate historical states of living populations and to gain new insight into the biological intricacies of even well-studied ecosystems.
Data availability statement
The original contributions presented in the study are included in the article, further inquiries can be directed to the corresponding author.
Author contributions
JM designed the study, conducted fieldwork, prepared the specimens, analyzed the data, and wrote the manuscript. EW conducted fieldwork and edited the manuscript. PD provided logistical support, curated the specimens, and edited the manuscript. All authors contributed to the article and approved the submitted version.
Funding
This work was supported by funding from the U.S. Fish and Wildlife Service (Arctic National Wildlife Refuge), The National Geographic Society (9133-12 to JM), a Christine Stevens Wildlife Award from the Wildlife Society (JM), the University of Cincinnati Office of Research, and the National Science Foundation (DEB-2135479 to JM).
Acknowledgments
We thank all the people who supported and contributed to the fieldwork for this study, including Dave Payer, Steve Arthur, Steve Berendzen, Janet Jorgenson, Greta Burkart, Joshua Rose, Melissa Werle, and Alfredo Soto, as well as our pilots Hollis Twitchell, Dave Sowards, and Roger Kaye. We also thank our two reviewers and editor for their helpful comments on prior drafts of the manuscript.
Conflict of interest
The authors declare that the research was conducted in the absence of any commercial or financial relationships that could be construed as a potential conflict of interest.
Publisher’s note
All claims expressed in this article are solely those of the authors and do not necessarily represent those of their affiliated organizations, or those of the publisher, the editors and the reviewers. Any product that may be evaluated in this article, or claim that may be made by its manufacturer, is not guaranteed or endorsed by the publisher.
References
Ambrose, S. H. (1990). Preparation and characterization of bone and tooth collagen for isotopic analysis. J. Archaeol. Sci. 17, 431–451. doi: 10.1016/0305-4403(90)90007-R
Arctic Climate Impact Assessment [ACIA] (2004). Impacts of a warming Arctic: Arctic climate impact assessment. New York, NY: Cambridge University Press.
Baksi, S. N., and Newbrey, J. W. (1989). Bone metabolism during antler growth in female reindeer. Calcif. Tissue Int. 45, 314–317. doi: 10.1007/BF02556025
Baltensperger, A. P., and Joly, K. (2019). Using seasonal landscape models to predict space use and migratory patterns of an arctic ungulate. Mov. Ecol. 7:18. doi: 10.1186/s40462-019-0162-8
Baxter, B. J., Andrews, R. N., and Barrell, G. K. (1999). Bone turnover associated with antler growth in red deer (Cervus elaphus). Anat. Rec. 256, 14–19. doi: 10.1002/(SICI)1097-0185
Beaumont, W., Beverly, R., Southon, J., and Taylor, R. E. (2010). Nuclear instruments and methods in physics research B. Nucl. Inst. Methods Phys. Res. B 268, 906–909. doi: 10.1016/j.nimb.2009.10.061
Behrensmeyer, A. K. (1978). Taphonomic and ecologic information from bone weathering. Paleobiology 4, 150–162. doi: 10.1017/S0094837300005820
Behrensmeyer, A. K., and Miller, J. H. (2012). “Building links between ecology and paleontology using taphonomic studies of recent vertebrate communities,” in Paleontology in Ecology and Conservation Springer Earth System Sciences. ed. J. Louys (Berlin: Springer), 69–91.
Benson, K. (2019). Gwich’in Knowledge of Porcupine Caribou: State of Current Knowledge and Gaps Assessment. Fort McPherson, NT: Department of Cultural Heritage, Gwich’in Tribal Council.
Bergerud, A. T. (1976). The annual antler cycle in Newfoundland caribou. Can. Field-Nat. 90, 449–463.
Bergerud, A. T. (1996). Evolving perspectives on caribou population dynamics, have we got it right yet? Rangifer 16, 95–116. doi: 10.7557/2.16.4.1225
Brady, K. (2017). H.R.1. An act to provide for reconciliation pursuant to titles II and V of the concurrent resolution on the budget for fiscal year 2018. 115th Congress of the United States of America.
Bubenik, G. A., Schams, D., White, R. J., and Rowell, J. (1997). Seasonal levels of reproductive hormones and their relationship to the antler cycle of male and female reindeer (Rangifer tarandus). Comp. Biochem. Physiol. B 116, 269–277. doi: 10.1016/S0305-0491(97)00183-1
Burch, E. S. Jr. (2012). Caribou Herds of Northwest Alaska 1850–2000. eds. I. Krupnik and J. Dau (Fairbanks, AK: University of Alaska Press).
Caikoski, J. (2020). Porcupine Caribou herd management report and plan, game management units 25A, 25B, 25D, and 26C: report period 1 July 2012–30 June 2017, and plan period 1 July 2017–30 June 2022. Juneau, AK: Alaska Department of Fish and Game, Species Management Report and Plan ADF&G/DWC/SMR&P-2020-22.
Cameron, M. D., Joly, K., Breed, G. A., Mulder, C. P. H., and Kielland, K. (2020). Pronounced fidelity and selection for average conditions of calving area suggestive of spatial memory in a highly migratory ungulate. Front. Ecol. Evol. 8:564567. doi: 10.3389/fevo.2020.564567
Cameron, R. D., Reed, D. J., Dau, J., and Smith, W. (1992). Redistribution of calving caribou in response to oil field development on the Arctic slope of Alaska. Arctic 45, 338–342. doi: 10.14430/arctic1412
Cameron, R. D., Smith, W. T., White, R. G., and Griffith, B. (2005). Central Arctic Caribou and petroleum development: distributional, nutritional, and reproductive implications. Arctic 58, 1–9. doi: 10.14430/arctic382
Cameron, R. D., and Whitten, K. R. (1979). Seasonal movements and sexual segregation of caribou determined by aerial survey. Arctic 43, 626–633. doi: 10.2307/3808740
Chapin, F. S., Barsdate, R. J., Barèl, D., and Barel, D. (1978). Phosphorus cycling in Alaskan coastal tundra: a hypothesis for the regulation of nutrient cycling. Oikos 31:189. doi: 10.2307/3543562
Crema, E. R., and Bevan, A. (2021). Inference from large sets of radiocarbon dates: software and methods. Radiocarbon 63, 23–39. doi: 10.1017/RDC.2020.95
Cronin, M. A., Haskell, S. P., and Ballard, W. B. (2003). The frequency of antlerless female caribou and reindeer in Alaska. Rangifer 23:67. doi: 10.7557/2.23.2.362
Crowley, B. E., Carter, M. L., Karpanty, S. M., Zihlman, A. L., Koch, P. L., and Dominy, N. J. (2010). Stable carbon and nitrogen isotope enrichment in primate tissues. Oecologia 164, 611–626. doi: 10.1007/s00442-010-1701-6
Cwynar, L. C. (1982). A late-quaternary vegetation history from Hanging Lake, northern Yukon. Ecol. Monogr. 52, 1–24. doi: 10.2307/2937342
DeNiro, M. J. (1985). Postmortem preservation and alteration of in vivo bone collagen isotope ratios in relation to palaeodietary reconstruction. Nature 317, 806–809. doi: 10.1038/317806a0
Doughty, C. E. (2017). Herbivores increase the global availability of nutrients over millions of years. Nat. Ecol. Evol. 1, 1–8. doi: 10.1038/s41559-017-0341-1
Doughty, C. E., Roman, J., Faurby, S., Wolf, A., Haque, A., Bakker, E. S., et al. (2016). Global nutrient transport in a world of giants. Proc. Natl. Acad. Sci. U. S. A. 113, 868–873. doi: 10.1073/pnas.1502549112
Doughty, C. E., Wolf, A., and Malhi, Y. (2013). The legacy of the Pleistocene megafauna extinctions on nutrient availability in Amazonia. Nat. Geosci. 6, 761–764. doi: 10.1038/ngeo1895
Espmark, Y. (1971). Antler shedding in relation to parturition in female reindeer. J. Wildl. Manag. 35, 175–177.
Fancy, S. G. (1983). Movements and activity budgets of caribou near oil drilling sites in the Sagavanirktok River floodplain, Alaska. Arctic 36, 193–197. doi: 10.2307/40509571
Fancy, S. G., Pank, L. F., Whitten, K. R., and Regelin, W. L. (1989). Seasonal movements of caribou in arctic Alaska as determined by satellite. Can. J. Zool. 67, 644–650. doi: 10.1139/z89-093
Fancy, S. G., and Whitten, K. R. (1991). Selection of calving sites by porcupine herd Caribou. Can. J. Zool. 69, 1736–1743. doi: 10.1139/z91-242
Fernandez-Jalvo, Y., and Andrews, P. (2016). Atlas of Taphonomic Identifications. New York, NY: Springer.
Fiorillo, A. R. (1995). The possible influence of low temperature on bone weathering in Curecanti National Recreation Area, Southwest Colorado. Cur. Res. Pleist. 12, 69–71.
Gordon, B. C. (1976). “Antler pseudo-tools made by caribou” in Symposium on Primitive Art and Technology (Calgary, AB: Chacmool (University of Calgary Students’ Association Publication)), 121–128.
Griffith, B., Douglas, D. C., Walsh, N. E., Young, D. D., McCabe, T. R., Russell, D. E., et al. (2002). “Section 3: the porcupine Caribou herd,” in Arctic Refuge Coastal Plain Terrestrial Wildlife Research Summaries. Biological Science Report USGS/BRD/BSR-2002-0001. eds. D. C. Douglas, P. E. Reynolds, and E. Rhode (Reston, VA: United States Geological Survey), 8–37.
Gunn, A., Russell, D., White, R. G., and Kofinas, G. (2009). Facing a future of change: wild migratory caribou and reindeer. Arctic 62, iii–vi. doi: 10.2307/40513303
Hajdas, I., Ascough, P., Garnett, M. H., Fallon, S. J., Pearson, C. L., Quarta, G., et al. (2021). Radiocarbon dating. Nat. Rev. Meth. Primers 1:62. doi: 10.1038/s43586-021-00058-7
Hinkes, M. T., Collins, G. H., and Van Daele, L. J. (2005). Influence of population growth on caribou herd identity, calving ground fidelity, and behavior. J. Wildl. Manag. 69, 1147–1162.
IPCC (2022). Climate Change 2022: Impacts, Adaptation, and Vulnerability. Contribution of Working Group II to the Sixth Assessment Report of the Intergovernmental Panel on Climate Change. eds. H.-O. Pörtner, D. C. Roberts, M. Tignor, E. S. Poloczanska, K. Mintenbeck, and A. Alegría, et al. (Cambridge: Cambridge University Press).
Irvine, F., Cwynar, L. C., Vermaire, J. C., and Rees, A. B. H. (2012). Midge-inferred temperature reconstructions and vegetation change over the last ~15,000 years from Trout Lake, northern Yukon territory, Eastern Beringia. J. Paleolimnol. 48, 133–146. doi: 10.1007/s10933-012-9612-7
Johnson, H. E., Golden, T. S., Adams, L. G., Gustine, D. D., and Lenart, E. A. (2020). Caribou use of habitat near energy development in Arctic Alaska. J. Wildl. Manag. 84, 401–412. doi: 10.1002/jwmg.21809
Joly, K., Gunn, A., Côté, S. D., Panzacchi, M., Adamczewski, J., Suitor, M. J., et al. (2021a). Caribou and reindeer migrations in the changing Arctic. Anim. Migr. 8, 156–167. doi: 10.1515/ami-2020-0110
Joly, K., Gurarie, E., Hansen, D. A., and Cameron, M. D. (2021b). Seasonal patterns of spatial fidelity and temporal consistency in the distribution and movements of a migratory ungulate. Ecol. Evol. 11, 8183–8200. doi: 10.1002/ece3.7650
Joly, K., Gurarie, E., Sorum, M. S., Kaczensky, P., Cameron, M. D., Jakes, A. F., et al. (2019). Longest terrestrial migrations and movements around the world. Sci. Rep. 9, 1–10. doi: 10.1038/s41598-019-51884-5
Joly, K., Klein, D. R., Verbyla, D. L., Rupp, T. S., and Chapin, F. S. (2011). Linkages between large-scale climate patterns and the dynamics of Arctic caribou populations. Ecography 34, 345–352. doi: 10.1111/j.1600-0587.2010.06377.x
Jorgenson, J. C., Joria, P. E., and Douglas, D. C. (2002). “Section 2: land cover,” in Arctic Refuge Coastal Plain Terrestrial Wildlife Research Summaries. Biological Science Report USGS/BRD/BSR-2002-0001 (Reston, VA: United States Geological Survey), 4–7.
Jorgenson, J. C., and Walker, D. A. (2018). Land Cover and Vegetation Map, Arctic National Wildlife Refuge. Oak Ridge, TN: ORNL DAAC.
Kaufman, D. S., Axford, Y. L., Henderson, A. C. G., McKay, N. P., Oswald, W. W., Saenger, C., et al. (2016). Holocene climate changes in eastern Beringia (NW North America) – a systematic review of multi-proxy evidence. Quat. Sci. Rev. 147, 312–339. doi: 10.1016/j.quascirev.2015.10.021
Kaufman, D., McKay, N., Routson, C., Erb, M., Dätwyler, C., Sommer, P. S., et al. (2020). Holocene global mean surface temperature, a multi-method reconstruction approach. Sci. Data 7:201. doi: 10.1038/s41597-020-0530-7
Koch, P. L., Hall, B. L., Bruyn, M., Hoelzel, A. R., Baroni, C., and Salvatore, M. C. (2019). Mummified and skeletal southern elephant seals (Mirounga leonina) from the Victoria Land Coast, Ross Sea Antarctica. Mar. Mam. Sci. 35, 934–956. doi: 10.1111/mms.12581
Kurek, J., Cwynar, L. C., and Vermaire, J. C. (2009). A late quaternary paleotemperature record from Hanging Lake, northern Yukon Territory, Eastern Beringia. Quat. Res. 72, 246–257. doi: 10.1016/j.yqres.2009.04.007
Le Moullec, M., Pedersen, Å. Ø., Stien, A., Rosvold, J., and Hansen, B. B. (2019). A century of conservation: the ongoing recovery of Svalbard reindeer. J. Wildl. Manag. 83, 1676–1686. doi: 10.1002/jwmg.21761
Lenart, E. A. (2015). “Chapter 18: units 26B and 26C caribou,” in Caribou Management Report of Survey and Inventory Activities 1 July 2012–30 June 2014. eds. P. Harper and L. A. McCarthy (Juneau, AK: Alaska Department of Fish and Game, Species Management Report ADF&G/DWC/SMR-2015-4), 1–38.
Lincoln, G. A., and Tyler, N. J. C. (1994). Role of gonadal hormones in the regulation of the seasonal antler cycle in female reindeer, Rangifer tarandus. J. Reprod. Rertil. 101, 129–138.
McCabe, R. A. (1957). Observations on the disappearance of shed Caribou antlers. J. Mammal. 38:275. doi: 10.2307/1376336
Meldgaard, M. (1986). The Greenland caribou - zoogeography, taxonomy, and population dynamics. Meddelelser om Gronland 20, 1–89.
Miller, J. H. (2011). Ghosts of Yellowstone: multi-decadal histories of wildlife populations captured by bones on a modern landscape. PLoS One 6:e18057. doi: 10.1371/journal.pone.0018057.g004
Miller, J. H. (2012). Spatial fidelity of skeletal remains: elk wintering and calving grounds revealed by bones on the Yellowstone landscape. Ecology 93, 2474–2482. doi: 10.1890/12-0272.1
Miller, F. L., and Barry, S. J. (1992). Nonrandom distribution of antlers cast by Peary caribou bulls, Melville Island, Northwest Territories. Arctic 45, 252–257.
Miller, J. H., Crowley, B. E., Bataille, C. P., Wald, E., Kelly, A., Gaetano, M., et al. (2021). Historical landscape use of migratory caribou: new insights from old antlers. Front. Ecol. Evol. 8:590837. doi: 10.3389/fevo.2020.590837
Miller, J. H., Druckenmiller, P., and Bahn, V. (2013). Antlers on the Arctic refuge: capturing multi-generational patterns of calving ground use from bones on the landscape. Proc. R. Soc. B 280:20130275. doi: 10.1126/science.1064539
Miller, J. H., and Simpson, C. (2022). When did mammoths go extinct? Nature 612, E1–E3. doi: 10.1038/s41586-022-05416-3
Nicholson, K. L., Arthur, S. M., Horne, J. S., Garton, E. O., and Del Vecchio, P. A. (2016). Modeling caribou movements: seasonal ranges and migration routes of the Central Arctic herd. PLoS One 11, e0150333–e0150320. doi: 10.1371/journal.pone.0150333
Nowicka, W., Machoy, Z., Gutowska, I., Noceń, I., Piotrowska, S., and Chlubek, D. (2006). Contents of calcium, magnesium, and phosphorus in antlers and cranial bones of the European red deer (Cervus elaphus) from different regions in western Poland. Polish. J. Environ. Stud. 15, 297–301.
Olson, E. A., and Broecker, W. S. (1961). Lamont natural radiocarbon measurements VII. Radiocarbon 3, 141–175. doi: 10.1017/S0033822200020919
Oster, K. W., Barboza, P. S., Gustine, D. D., Joly, K., and Shively, R. D. (2018). Mineral constraints on arctic caribou (Rangifer tarandus): a spatial and phenological perspective. Ecosphere 9, e02160–e02118. doi: 10.1002/ecs2.2160
Pearson, R. G., Phillips, S. J., Loranty, M. M., Beck, P. S. A., Damoulas, T., Knight, S. J., et al. (2013). Shifts in Arctic vegetation and associated feedbacks under climate change. Nature Clim. Change 3, 673–677. doi: 10.1038/nclimate1858
Pobiner, B., Dumouchel, L., and Parkinson, J. (2020). A new semi-quantitative method for coding carnivore chewing damage with an applition to modern African lion-damaged bones. PALAIOS 35, 302–315. doi: 10.2110/palo.2019.095
Pokines, J. T., Faillace, K., Berger, J., Pirtle, D., Sharpe, M., Curtis, A., et al. (2018). The effects of repeated wet-dry cycles as a component of bone weathering. J. Archaeol. Sci. Rep. 17, 433–441. doi: 10.1016/j.jasrep.2017.11.025
Pokines, J. T., King, R. E., Graham, D. D., Costello, A. K., Adams, D. M., Pendray, J. M., et al. (2016). The effects of experimental freeze-thaw cycles to bone as a component of subaerial weathering. J. Archaeol. Sci. Rep. 6, 594–602. doi: 10.1016/j.jasrep.2016.03.023
Porcupine Caribou Management Board [PCMB] (2016). Porcupine Caribou Annual Summary Report 2016. Whitehorse, YT: Porcupine Caribou Management Board.
R Core Team (2021). R: A Language and Environment for Statistical Computing. R Foundation for Statistical Computing, Vienna, Austria.
Rantanen, M., Karpechko, A. Y., Lipponen, A., Nordling, K., Hyvärinen, O., Ruosteenoja, K., et al. (2022). The Arctic has warmed nearly four times faster than the globe since 1979. Commun. Earth Environ. 3:168. doi: 10.1038/s43247-022-00498-3
Reimer, P. J., Austin, W. E. N., Bard, E., Bayliss, A., Blackwell, P. G., Bronk Ramsey, C., et al. (2020). The IntCal20 northern hemisphere radiocarbon age calibration curve (0–55 cal kBP). Radiocarbon 62, 725–757. doi: 10.1017/RDC.2020.41
Russell, D., and Gunn, A. (2019). Vulnerability analysis of the porcupine Caribou herd to potential development of the 1002 lands in the Arctic National Wildlife Refuge, Alaska. Report prepared for: Environment Yukon, Canadian Wildlife Service, and GNWT Department of Environment and Natural Resources.
Russell, D. E., Martell, A. M., and Nixon, W. A. C. (1993). Range ecology of the porcupine Caribou herd in Canada. Rangifer 8, 1–168. doi: 10.7557/2.13.5.1057
Russell, D. E., White, R. G., and Daniel, C. J. (2005). Energetics of the Porcupine Caribou Herd: a computer simulation model. Ottawa, ON: Canadian Wildlife Service.
Skoog, R. O. (1968). Ecology of the Caribou (Rangifer tarandus granti) in Alaska (Ph.D. Dissertation). Berkeley, CA: University of California Berkeley.
Stewart, T. G., and England, J. (2008). An early Holocene caribou antler from northern Ellesmere Island, Canadian Arctic archipelago. Boreas 15, 25–31. doi: 10.1111/j.1502-3885.1986.tb00739.x
Stokes, S., Márquez-Grant, N., and Greenwood, C. (2020). Establishing a minimum PMI for bone sun bleaching in a UK environment with a controlled desert-simulated comparison. Int. J. Legal Med. 134, 2297–2306. doi: 10.1007/s00414-020-02385-y
Stuiver, M., and Polach, H. A. (1977). Discussion reporting of 14C data. Radiocarbon 19, 355–363. doi: 10.1017/S0033822200003672
Sutcliffe, A. J., and Blake, W. (2000). Biological activity on a decaying caribou antler at cape Herschel, Ellesmere Island, Nunavut, high Arctic Canada. Polar Rec. 36, 233–246.
Tappen, M. (1994). Bone weathering in the tropical rain forest. J. Archaeol. Sci. 21, 667–673. doi: 10.1006/jasc.1994.1066
Teitelbaum, C. S., Fagan, W. F., Fleming, C. H., Dressler, G., Calabrese, J. M., Leimgruber, P., et al. (2015). How far to go? Determinants of migration distance in land mammals. Ecol. Lett. 18, 545–552. doi: 10.1111/ele.12435
Telford, R. J., Heegaard, E., and Birks, H. J. B. (2004). The intercept is a poor estimate of a calibrated radiocarbon age. Holocene 14, 296–298. doi: 10.1191/0959683604hl707fa
Thomas, D., and Barry, S. (2010). Antler mass of barren-ground caribou relative to body condition and pregnancy rate. Arctic 58, 241–246. doi: 10.14430/arctic425
Todisco, D., and Monchot, H. (2008). Bone weathering in a periglacial environment: the Tayara site (KbFk-7), Qikirtaq Island, Nunavik (Canada). Arctic 61, 87–101. doi: 10.2307/40513184
Udall, M. (1980). “H.R.39. Alaska national interest lands conservation act,” in 96th congress of the United States of America, Washington, DC.
van Klinken, G. J. (1999). Bone collagen quality indicators for palaeodietary and radiocarbon measurements. J. Archaeol. Sci. 26, 687–695. doi: 10.1006/jasc.1998.0385
Vistnes, I., and Nellemann, C. (2008). The matter of spatial and temporal scales: a review of reindeer and caribou response to human activity. Polar Biol. 31, 399–407. doi: 10.1007/s00300-007-0377-9
Vors, L. S., and Boyce, M. S. (2009). Global declines of caribou and reindeer. Glob. Change Biol. 15, 2626–2633. doi: 10.1111/j.1365-2486.2009.01974.x
Weintraub, M. N. (2011). “Biological phosphorus cycling in arctic and alpine soils” in Phosphorus in Action Soil Biology. eds. E. Bünemann, A. Oberson, and E. Frossard (Berlin, Heidelberg: Springer Berlin Heidelberg), 295–316.
Whitten, K. R. (1994). “International cooperation in managing the Porcupine Caribou Herd.” in 74th Annual Conference Western Association of Fish and Wildlife Agencies. Anchorage, Alaska. 64–68.
Whitten, K. R. (1995). Antler loss and udder distention in relation to parturition in caribou. J. Wildl. Manag. 59, 273–277.
Whitten, K. R., Garner, G. W., and Mauer, F. J. (1984). Calving Distribution, Initial Productivity, and Neonatal Mortality of the Porcupine Caribou Herd, 1983. Alaska Department of Fish and Game and Arctic National Wildlife Refuge.
Keywords: taphonomy, radiocarbon (14C) dating, time-averaging, seasonal landscape use, migration, caribou (Rangifer tarandus)
Citation: Miller JH, Wald EJ and Druckenmiller P (2023) Shed female caribou antlers extend records of calving activity on the Arctic National Wildlife Refuge by millennia. Front. Ecol. Evol. 10:1059456. doi: 10.3389/fevo.2022.1059456
Edited by:
G. Lynn Wingard, United States Geological Survey (USGS), United StatesReviewed by:
Robert S. Feranec, New York State Museum, United StatesDean Cluff, Government of the Northwest Territories, Canada
Copyright © 2023 Miller, Wald and Druckenmiller. This is an open-access article distributed under the terms of the Creative Commons Attribution License (CC BY). The use, distribution or reproduction in other forums is permitted, provided the original author(s) and the copyright owner(s) are credited and that the original publication in this journal is cited, in accordance with accepted academic practice. No use, distribution or reproduction is permitted which does not comply with these terms.
*Correspondence: Joshua H. Miller, ✉ am9zaC5taWxsZXJAdWMuZWR1
†Present address: Eric J. Wald,National Park Service, Arctic Network, Inventory and Monitoring Division, Fairbanks, AK, United States