- 1Department of Biology, Mount Allison University, Sackville, NB, Canada
- 2Canadian Wildlife Service, Environment and Climate Change Canada, Sackville, NB, Canada
- 3Prairie and Northern Wildlife Research Centre, Science and Technology Branch, Environment and Climate Change Canada, Saskatoon, SK, Canada
Semipalmated Sandpipers (Calidris pusilla) are Arctic-breeding shorebirds that use staging sites in Atlantic Canada during their annual migration to South America. The Bay of Fundy has long been recognized as a critical staging site for migrating Semipalmated Sandpipers and supports a large prey base. The diet of adult sandpipers in the Bay is flexible but the diet of juveniles, which arrive later, is not well documented. Comparatively little is known about the prey base and how it is utilized by sandpipers at sites in Atlantic Canada outside the Bay. Plasma metabolite measures can provide useful insight to assess habitat quality for sandpipers and have not yet been measured in Semipalmated Sandpipers in Atlantic Canada. To address these knowledge gaps we sampled shorebird habitat to estimate invertebrate availability in the Bay of Fundy and the Northumberland Strait. Concurrently, we collected blood samples from adult and juvenile sandpipers for analysis of plasma metabolite levels and isotopic estimates of dietary niche in both regions. We found that sites on the Northumberland Strait hosted a more diverse and variable prey base than sites within the Bay of Fundy, and that sandpipers were selective when foraging there, appearing to prefer bivalves. Juveniles may occupy a broader dietary niche than adults along the Northumberland Strait, though appear to gain weight as efficiently. Sandpipers sampled along the Northumberland Strait had higher plasma triglyceride concentrations than those within the Bay of Fundy, which may suggest differences in fattening rate or dietary fat intake. Sandpipers that had lower triglyceride concentrations on the Northumberland Strait were more likely to move into the Bay of Fundy, while sandpipers with high triglyceride values tended to remain on the Strait. These data suggest that sandpipers made movement decisions within the region depending on their physiological state. Our results suggest adult and juvenile Semipalmated Sandpipers successfully use a variety of staging habitats in Atlantic Canada. This is an encouraging finding for sandpiper conservation in the region, but also indicates that maintaining access to a broad variety of staging habitats is critical, supporting calls for stronger conservation measures throughout the region.
1 Introduction
Shorebird populations throughout the western hemisphere are in decline. This is especially true for long distance migrants (North American Bird Conservation Initiative Canada, 2019), which are known to stage at a number of sites to refuel during their migration (Warnock, 2010; Ma et al., 2013). Birds choose staging sites based on a variety of factors including food availability (Hicklin and Smith, 1984; Goss-Custard et al., 1991; Ydenberg et al., 2002; Hamilton et al., 2003; Sprague et al., 2008) and site safety (Ydenberg et al., 2002; Sprague et al., 2008). High-quality staging sites are thus essential for successful migration (McGowan et al., 2011) and maintaining the integrity of these sites is a conservation priority. Often these are coastal sites that are experiencing erosion and habitat loss, risking their future availability for shorebird use (Galbraith et al., 2002). For species in which individuals stage at just one or two sites to refuel during their migration, particular areas can take on even greater importance [e.g., Delaware Bay for Red Knots (Calidris canutus rufa); Morrison and Harrington, 1992]. The rate of refueling at these sites can determine migration speed and success (Lyons et al., 2008), thus a healthy prey base and safe environment are essential.
Fat is the main energy source used on migration, as it has the most energy per unit mass (Ramenofsky, 1990; McWilliams et al., 2004). Thus, an ability to gain fat efficiently at staging sites is critical to successful migration. Previous studies have found a positive correlation between plasma triglyceride concentrations and fattening rates (Jenni-Eiermann and Jenni, 1994; Williams et al., 1999), making triglyceride measures a good index of fattening rates and potentially site quality (Lyons et al., 2008). In contrast, when energy use is higher than intake, lipids are burned as an alternative fuel source (Ramenofsky, 1990). β-OH-butyrate is indicative of fat catabolism and can therefore indicate fasting, restrictive feeding, or long endurance flights (Jenni-Eiermann and Jenni, 1994; Stevens, 1996). Previous studies have examined plasma metabolite levels in migrating juvenile and adult shorebirds. Guglielmo et al. (2002) found no difference in plasma triglyceride levels between adult and juvenile Western Sandpipers (Calidris mauri) during staging, but there was some evidence that juveniles may have higher β-OH-butyrate levels than adults. Another study in the prairie pothole region found that adult Semipalmated Sandpipers (Calidris pusilla) had higher plasma triglyceride levels and lower β-OH-butyrate levels than juveniles (Thomas and Swanson, 2013).
Semipalmated Sandpipers migrate annually from their Arctic breeding grounds to winter in South America. The species has experienced widespread declines, with losses observed on breeding, staging, and non-breeding sites (Jehl, 2007; Andres et al., 2012; Gratto-Trevor et al., 2012b; Morrison et al., 2012). The Bay of Fundy, Canada, is a critical staging location on southbound migration for eastern and central breeding Semipalmated Sandpipers (Hicklin, 1987; Gratto-Trevor et al., 2012a). Recent work has found adult sandpipers using the Bay of Fundy have high within and between-year fidelity, returning to the same areas of the Bay each year (Neima et al., 2020). Additionally, the behavior, movement and diet of adult sandpipers in this habitat are well understood (e.g., MacDonald et al., 2012; Quinn and Hamilton, 2012; Neima et al., 2022). Less is known about the use of these habitats by juvenile Semipalmated Sandpipers, which may need additional time or resources to gain sufficient weight for a successful southbound migration. Further, sites outside the Bay of Fundy, which are also used by Semipalmated Sandpipers and host a diverse assemblage of other staging shorebird taxa, have received little attention.
Juvenile Semipalmated Sandpipers have lower survivorship than adults, which may be contributing to population declines (Hitchcock and Gratto-Trevor, 1997). Anderson et al. (2019) found that juvenile shorebirds had lower fuel loads and were less likely than adults to be detected south of James Bay, possibly suggesting higher mortality on southbound migration. Reduced survivorship among juveniles may be due to inefficient foraging, or insufficient fat stores to complete migration. Juvenile Semipalmated Sandpipers use a broader variety of sites than adults while staging in Atlantic Canada (Linhart et al., in press). This could lead to differences in prey consumption and slower fattening rates as they may burn more energy while traveling between sites. In some songbird species juveniles are forced to forage in less profitable areas, resulting in increased stopover duration to gain sufficient weight (Woodrey, 2000; Moore et al., 2003). Recent work in the region has found juveniles stay ∼3 days longer than adults, adjusted for timing of migration (Mann et al., 2017; Linhart et al., in press), though the reason for this longer staging period needs more investigation. Additionally, juvenile sandpipers migrate through the region later than adults (Gratto et al., 1984; Hicklin, 1987; Dunn et al., 1988), which may reduce or change prey available to them.
Staging strategies of Semipalmated Sandpipers appear to vary depending on the sites they use. Recent work in Atlantic Canada found sandpipers tagged in the Bay of Fundy remained there, while birds tagged at a site outside the Bay would either choose to move into the Bay, or to remain outside the Bay for the duration of their stay in the area (Neima et al., 2020; Linhart et al., in press). Birds using sites outside the Bay had a significantly shorter length of stay than those using the Bay of Fundy, staying on average ∼7 days less (Linhart et al., in press). This may be due to differences in migratory strategy, or it could be because birds using sites outside the Bay are able to gain weight more efficiently than those using the Bay of Fundy, possibly because they use a different prey base.
To assess these possibilities, and to better understand staging by birds in Atlantic Canada, we examined resource availability and use and metabolic state of adult and juvenile Semipalmated Sandpipers in the Bay of Fundy and on the Northumberland Strait, a coastal body of water separated from the Bay of Fundy by approximately 50 km. We hypothesized that diets would vary between adult and juvenile birds, and between our two study regions (Bay and Strait). Given that the Bay of Fundy is known to host an abundant invertebrate community consisting of high quality prey (Quinn and Hamilton, 2012; Gerwing et al., 2015; Quinn et al., 2017) and the majority of Semipalmated Sandpipers in Atlantic Canada use the Bay during migration, we predicted that sandpipers there would have higher plasma triglyceride values (signifying fattening rate) than those using small staging sites on the Northumberland Strait. In that event, the increased length of stay observed in the Bay (Linhart et al., in press) may be related to higher site quality (sensu Herbert et al., 2022). We also predicted that birds choosing to transfer from the Northumberland Strait into the Bay of Fundy would be those that needed to move to higher quality habitat to gain sufficient weight. Finally, we predicted that juvenile birds, which use a wider range of habitat (Linhart et al., in press) and are generally inexperienced may exhibit lower plasma triglyceride levels but higher β-OH-butyrate levels as a result of increased movement within the region. This study provides an opportunity to assess habitat quality through a lens of physiological response and helps us to understand the conservation value and utility of small staging areas in this region. Further, investigation of metabolic markers in adult and juvenile Semipalmated Sandpipers in Atlantic Canada may help us to better understand the implications of observed age-related differences in movements and resource use.
2 Materials and methods
2.1 Study area
We collected data at two coastal marine sites in the Northumberland Strait and Bay of Fundy (Figure 1). On the Northumberland Strait, we captured birds and collected invertebrate samples at Petit Cap (46.1791°N, −64.1398°W) in 2018 and 2019. We also collected invertebrate samples at another site, Cape Jourimain (46.1544°N, −63.8397°W), in 2019 (Figure 1). Petit Cap is a 1.25 km dynamic barrier beach with coarse sandy sediment and ∼0.5 km of sand and mudflats at low tide, while Cape Jourimain is a 1.3 km beach with a gradual slope bordering an extensive salt marsh complex, and ∼0.4 km of beach exposed at low tide. In the Bay of Fundy, we captured birds at Johnson’s Mills (adjacent to the Grande Anse mudflat) and collected invertebrate samples at both Grande Anse (45.8339°N, −64.5119°W) and Pecks Cove (45.7567°N, −64.4891°W) in both years (Figure 1). The Grande Anse mudflat extends ∼2 km from shore and is largely uniform with a high sediment water content and sediment penetrability (MacDonald et al., 2012; Gerwing et al., 2015). Pecks Cove is smaller, the sediment is firmer and has a lower water content than Grand Anse (Gerwing et al., 2015). The tidal flat extends ∼850 m from shore, and there are deep ravines across the flats.
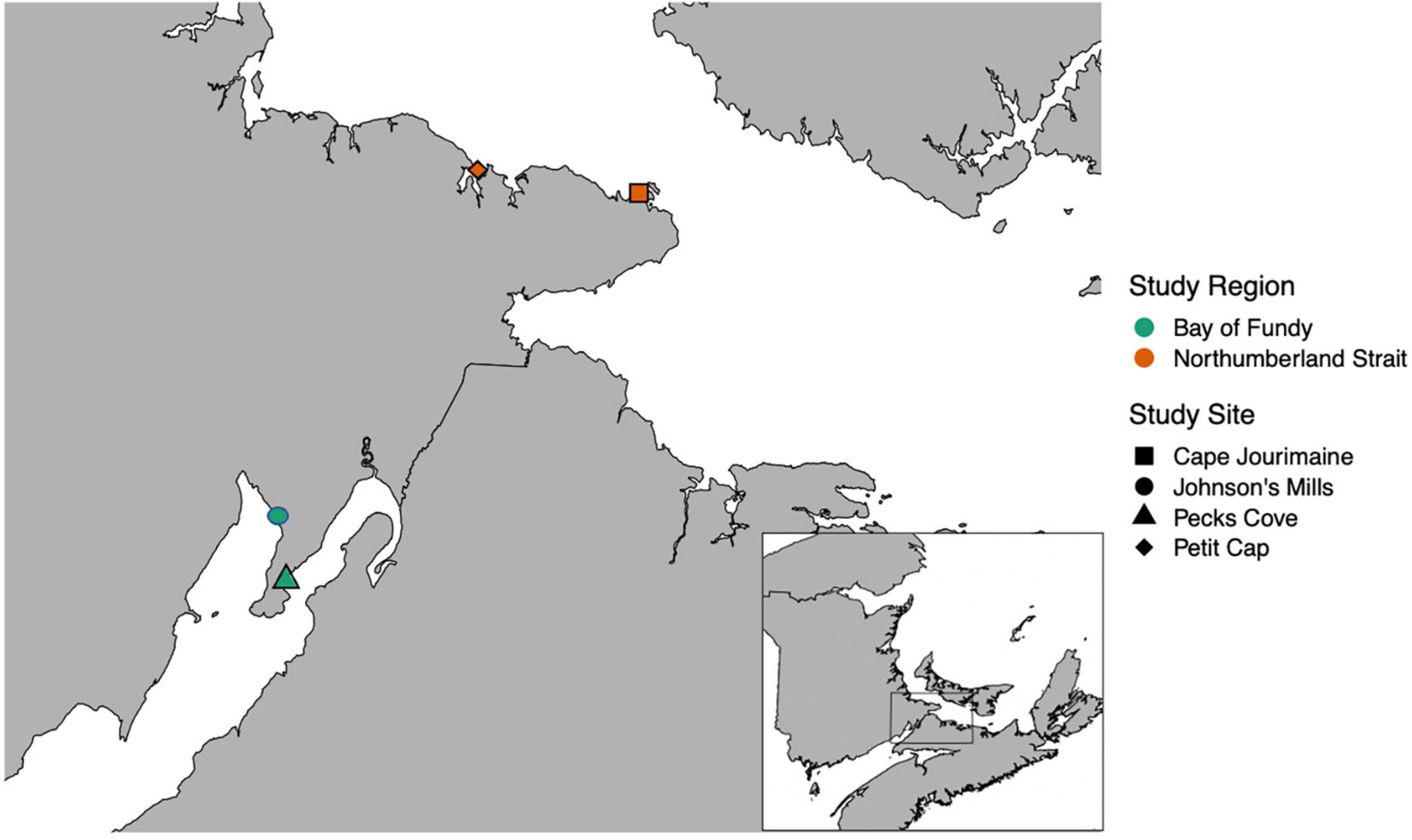
Figure 1. Map of study sites in both regions: the Northumberland Strait (orange), and the Bay of Fundy (green). Semipalmated Sandpiper captures occurred at Johnson’s Mills (adjacent to Grand Anse) (green circle) and Petit Cap (orange diamond). Habitat transects were completed at all four sites in 2019. In 2018 Cape Jourimain was not sampled.
2.2 Bird capture and radio-telemetry
We captured birds at Johnson’s Mills and Petit Cap during southbound migration from late July through mid-September in 2018 and 2019 (Table 1). On the Northumberland Strait we used mist nets deployed during low tides in the evening and at night. In the Bay of Fundy we used a Fundy pull trap (Hicklin et al., 1989) to capture birds roosting during high tide, as the high density of birds and speed of tidal movements made mist netting inappropriate in most circumstances. Upon capture, birds received individually numbered metal leg bands on the upper left leg and white alpha-numeric flags on the upper right leg. We recorded morphometric data including mass (±0.1 g), flattened straightened wing chord (±1 mm), bill (culmen to tip), and tarsus length (±0.1 mm) for each bird. Birds were aged as hatch year (HY), second year (SY), or after second year (ASY) following Gratto-Trevor (2004) and Pyle (2008). We attached digitally encoded 0.67-g Lotek VHF nanotags (NTQB-3-2, Lotek Wireless Inc., Newmarket, ON, Canada) to a subset of light (<30 g) HY (hereafter referred to as juvenile) and AHY (hereafter referred to as adult) birds at both sites. Details of tagging are provided in Linhart et al. (in press) and are similar to Neima et al. (2020).
We collected blood from the brachial vein of birds using 27-gauge needles and heparinized capillary tubes. A maximum of 140 μl of blood was collected from each bird. Blood was transferred from the capillary tubes to Eppendorf tubes in the field and kept on ice until it could be centrifuged in the lab, a maximum of 6 h after it was drawn. Samples were centrifuged at 10,000 RPM for 1 min (mySPIN12 Mini Centrifuge, Thermo Scientific) to separate plasma and red blood cells. We pipetted off the plasma into separate tubes and stored samples at −20°C until used for analyses. We also collected a drop of blood from tagged birds on Whatman FTA cards (Whatman®, Marlborough, MA, USA) for molecular sexing. Blood used for plasma metabolite assays was collected within 20 min of capture to minimize the impact of handling stress (Guglielmo et al., 2002). Our average bleed time (time after capture) was 9.5 min (±4.5 min) for these birds. Blood was also collected from heavy birds (>32 g) for stable isotope analyses to estimate diet. Stable isotopes in blood plasma reflect the diet of birds for approximately the previous 6 days (Hobson and Clark, 1993). We chose heavy birds to ensure samples reflected the local diet of a bird that had likely been present in the region for at least 1 week (Quinn and Hamilton, 2012), as there is a positive relationship between fuel loads and length of stay in the region (Linhart et al., in press), and Semipalmated Sandpipers are known to arrive in the region light and roughly double their weight during their stay (Hicklin and Smith, 1984). All bird sampling was conducted under federal banding permits and was approved by the Mount Allison University Animal Care Committee under guidelines from the Canadian Council on Animal Care.
Tagged birds were tracked throughout their stay in Atlantic Canada using the Motus Wildlife Tracking Network (Taylor et al., 2017). Raw data were filtered by removing any detections with <3 consecutive hits, and by examining individual antenna signal plots for each tag (see Linhart et al., in press) following guidelines provided in the Motus R Book (Crewe et al., 2020). Birds were classified into three movement strategies depending on their tagging location. Sandpipers tagged within the Bay of Fundy remained within the Bay, while those tagged at Petit Cap either remained outside the Bay or moved into the Bay and remained there for the duration of their staging period in Atlantic Canada (see Linhart et al., in press for details). We calculated a minimum length of stay (as in Neima et al., 2022) for each bird by subtracting the deployment timestamp from the last known detection in Atlantic Canada. Detailed analyses of movement strategies, length of stay, and differences in site use between ages and tagging locations can be found in Linhart et al. (in press).
2.3 Prey availability
We collected sediment samples in 2018 and 2019 at our study sites to estimate prey availability. In 2018 we sampled in late July before bird migration. In 2019 we sampled twice, once in late July (pre-migration) and once in mid-September (late/post-migration). Samples were collected in a stratified random manner in habitat where shorebirds were known to forage, with transects running perpendicular to shore, differing in length depending on habitat available. In the Bay of Fundy in both years we completed two transects at each mudflat. Each transect was 500 m long and stratified into 100 m sections with two samples taken at random points in each stratum, for a total of 20 samples per mudflat per sampling period. Transects were ∼500 m apart at each mudflat. In 2018 at Petit Cap on the Northumberland Strait, we had five 125-m transects stratified into 25 m sections, with one sample taken at a random point in each stratum, for a total of 25 samples. To cover more habitat at Petit Cap in 2019, we again had five transects, but made them 300 m long and stratified into 100 m segments, with two samples per segment, for a total of 30 samples. In both years three of the transects at Petit Cap were on the ocean side of the barrier beach, and two were on the inner, marsh/mudflat side of the beach. At Cape Jourimain in 2019 we ran three transects, each 300 m long and stratified into 100 m segments, with two samples per segment, for a total of 18 samples. At each sampling point, we collected samples using a 5.5 cm core pushed into the sediment until there was resistance from the consolidated anaerobic layer. These were then passed through a mesh sieve (Bay of Fundy 250 μm; Northumberland Strait 500 μm to account for coarser sediment found during preliminary sampling) and retained invertebrates were stored in 95% ethanol until they were sorted by size and taxon. The prey base differs between the Bay and Strait, with many of the smaller taxa that would be lost in a 500 μm sieve in the Bay not present in the Strait, so availability of prey relevant to shorebirds remains comparable. We only counted invertebrates that were clearly alive at the time of sampling. Sorted invertebrates were measured and dried in a drying oven (40GC Gravity Convection Oven, Quincy Lab Inc., Chicago, IL, USA) at 90°C for at least 24 h. Dry weight was recorded in g (±0.00001 g). All shelled invertebrates were subsequently ashed in a muffle oven (Isotemp Programmable Muffle Furnace 650-750 Series, Fisher-Scientific, Waltham, MA, USA) at 550°C for 2 h to combust organic material. Remaining shells were re-weighed, and shell weight was subtracted from the original dry weight to estimate consumable biomass. Kober and Bairlein (2006) suggested sandpipers consumed shelled items <8 mm, but to ensure that possible prey were not improperly excluded, we included all bivalves with a shell length <10 mm.
To determine whether shorebirds were actively selecting areas within the habitat with high prey availability, we conducted behavioral observations (Linhart, 2021) and then immediately collected sediment cores from locations where birds were foraging. Samples were processed as described above and prey densities compared to samples obtained from transects which reflect overall prey abundance at each site.
2.4 Stable isotope and plasma metabolite sample processing
We measured levels of stable isotopes of 13C and 15N in blood plasma of adult and juvenile birds at both capture locations. To prepare for stable isotope analyses, samples were dried in a drying oven (40GC Gravity Convection Oven, Quincy Lab Inc., Chicago, IL, USA) at 70°C for 24 h. In 2018 samples were analyzed for δ13C and δ15N at the Stable Isotope in Nature Lab (SIN Lab, University of New Brunswick), using a Finnigan Delta Plus mass spectrometer (Thermo-Finnigan; Bremen, Germany) interfaced with a Costech 4010 elemental analyzer via the Conflo II. In 2019 we analyzed samples at the Environmental Analytics and Stable Isotope Lab (EASIL, Mount Allison University) using an Elementar PyroCube Elemental Analyzer (Elementar Analysensysteme GmbH, Hanau, Germany) and an Isoprime Precision Isotope Ratio Mass Spectrometer (Elementar UK Ltd., Cheadle, UK). Though samples were processed in different labs, the procedures were very similar, results were standardized to the same references, and the same operator worked in both labs, so results are comparable. Stable isotope values were given relative to standard references in δ(iX) format as follows, where X is either 13C or 15N, and R is the corresponding 13C/12C or 15N/14N isotope ratio.
All metabolites were quantified using commercially available assay kits. Plasma triglyceride (TRIG) concentration was quantified using Sigma Aldrich Triglyceride Determination Kit (Sigma-Aldrich, TR0100-1KT, Oakville, ON, Canada) using undiluted plasma. For β-OH-butyrate (BOHB) concentration, we used Cayman Chemical β-OH-butyrate (Ketone Body) Colorimetric Assay Kit (Cayman Chemical, 700190, Ann Arbor, MI, USA). Plasma samples were diluted for the BOHB assay; depending on the amount of plasma available we did between a 3 and 12× dilution using the buffer that came in the kit. BOHB was only measured in 2019 and the sample size was limited. To increase our sample size and improve generalizability, we also obtained samples collected in 2020 at Petit Cap (using the same protocol) for this assay. For both assays, samples were analyzed in duplicate on a 96-well plate in a spectrophotometer (Allsheng, AMR-100, Hangzhou, China). If duplicate samples had >20% difference in results they were re-run (if possible), or the data were excluded from analyses. Duplicates with acceptable variation (<20%) were averaged, with the average value used as the metabolite measure for each bird.
2.5 Statistical analyses
All analyses were completed using R statistical software, version 4.0.3 (R Core Team, 2020) with an R Studio interface. We set alpha at 0.05 for main effects, and 0.1 for interactions to ensure that main effects were not inappropriately interpreted in the presence of a potential interaction (as in Hamilton et al., 2006). Assumptions were tested using a Shapiro test for normality with visual inspection of Q-Q plots, and either Levene’s or Cochran’s test for homogeneity of variance with visual inspection of residuals. To account for unbalanced designs, we used type II Sums of Squares (Langsrud, 2003).
2.5.1 Invertebrate availability
To examine differences in prey availability between sites, years, and sample types (transect vs. foraging area), we completed a permutational multivariate analysis of variance (PERMANOVA, 999 permutations, Bray–Curtis distance matrix) using the vegan package in R (Oksanen et al., 2020). For each sample, invertebrate biomass was summed across size classes by taxon. In 2019, we had early and late season prey samples. To compare availability between years, we used only the early transects, as they were most comparable to 2018. Invertebrate biomasses were the response variables, and year, region (Bay vs. Strait), and type (foraging vs. transect) were predictors. When we detected significant multivariate effects, we used similarity percentages (simper) to determine relative contribution to the overall results of the different response variables. We also assessed multivariate dispersion (permdisp) with the betadisper function (vegan; Oksanen et al., 2020) to compare dispersion among levels of factors. We identified significant region × sample type and region × year interactions, which were investigated by splitting the analyses by region and developing models examining year, site and sample type for each region.
We also examined variability in invertebrate prey abundance between sites within each region to assess temporal and spatial variation. Using 2019 transect data, we completed separate linear mixed effect models for each region, with time (July vs. September) set as a fixed factor and transect nested within site, both as random factors. Invertebrate biomasses were pooled by sample and used as the response variable for each model. To meet assumptions data were log-transformed for the Bay of Fundy and square-root transformed for the Northumberland Strait. We extracted variance components for the random factors in the models and completed a variance component analysis to assess sources of variability within the system. To compare variability between regions we completed a Levene’s test using transect data from both regions, with log-transformed biomass as the response variable and region as the predictor.
2.5.2 Stable isotopes and dietary niche
We assessed differences in dietary niche breadth between adult and juvenile birds by comparing multivariate dispersion (permdisp analyses) of δ13C and δ15N signatures using the vegan package in R (betadisper function; Oksanen et al., 2020). We completed separate analyses for each region due to differences in the prey base. To visualize dietary niches, we used the SIBER package (Jackson et al., 2011) to generate plots.
2.5.3 Movement and metabolites
Linhart et al. (in press) identified three main staging strategies, with length of stay varying amongst them from approximately 2 to 3 weeks – birds captured in the Bay of Fundy remained there, whereas those captured on the Northumberland Strait either remained there or transferred into the Bay and remained there until they departed. We completed two sets of analyses to examine differences in plasma metabolites between birds using different staging strategies, one for tagged birds that had blood samples drawn, and one including a larger sample of birds bled from each site. For both analyses we calculated relative fuel loads to control for the fat content of each bird. Many studies have found weight affects metabolite levels in shorebirds (Guglielmo et al., 2002; Seaman et al., 2005; Lyons et al., 2008), and relative fuel loads allow us to control for fat content adjusted for body size, as juveniles can be smaller than adults. Gutiérrez et al. (2019) took a similar approach when comparing metabolic profiles of shorebirds of varying body sizes. Relative fuel loads at capture were calculated using the following equation from Anderson et al. (2019):
Estimated lean mass was calculated using separate regression equations for adults and juveniles, generated by Anderson et al. (2019) using wing cord to account for variability between individuals.
For the analysis of all bled birds (tagged and untagged) we ran an additive generalized linear model (glm) to investigate differences in TRIG values between Petit Cap and Johnson’s Mills. TRIG values were the response variable, with age, year, and site as predictors. We included time from capture to the bird being bled (bleed time) and relative fuel load as covariates. Bleed time is known to influence TRIG values, so although we constrained it to 20 min post capture to minimize this effect, we included it in the event the effect was still present. Further, there was a positive relationship between plasma triglyceride concentrations and relative fuel load for all age and site combinations (p = 0.003–0.04, R2 = 0.11–0.17), so inclusion of it in the model controlled for this factor. To assess BOHB values we ran another additive glm. Log transformed BOHB values were the response variable, age and site were categorical predictors, and relative fuel loads and bleed time were covariates. BOHB values were generally higher for juveniles than adults but we had a small sample size and therefore limited statistical power. To clarify the result, we obtained additional data from birds sampled at Petit Cap in 2020. Using only Petit Cap birds, we ran an additive glm with year (2019 and 2020) and age as factors, and bleed time and fuel loads as covariates to better assess differences in BOHB concentrations between ages with a larger sample size. For each of the above metabolites, sex of birds was also tested as a predictor by subsetting the data to include only sexed birds (as determined in Linhart et al., in press). We found no effect of sex for any model, so it was removed and sexes were combined for all analyses.
For tagged birds we modeled rank-transformed length of stay on strategy, age (HY and AHY), year and TRIG concentrations using a glm. BOHB was not included in models of length of stay because we had a small sample size and only had results for 1 year. We also ran a glm with TRIG as the response and strategy, age, year, relative fuel loads, and bleed time as predictors. We ran a similar model with BOHB as the response and strategy, age, relative fuel loads, and bleed time as predictors using data from 2019. BOHB had to be log transformed to meet assumptions. To examine differences in TRIG levels between birds using different movement strategies at Petit Cap we ran a binomial glm and used AIC model selection (R package MuMin; Bartoń, 2022) with movement strategy as the response variable (positive being “moved” into the Bay of Fundy and negative being “did not move”), age, day of year, year, and TRIG as the predictors.
3 Results
3.1 Prey availability
Differences in prey biomass between sample types (transects vs. foraging areas) varied among regions (Region × Type: F1,240 = 1.75, p = 0.09) and differences between regions varied by year (Region × Year: F1,240 = 4.53, p = 0.001), so we split by region to investigate these interactions (Table 2). Invertebrate availability varied by year in both regions, and in the Bay of Fundy in 2018 by site as well (Table 2 and Figure 2). In general, there was no evidence that Semipalmated Sandpipers foraged selectively in areas containing more prey biomass in the Bay of Fundy except for Pecks Cove in 2018 where samples collected in foraging areas had higher invertebrate abundance than those from transects. This appears to be driven mainly by amphipods (Figure 2). In contrast, sandpipers foraging in the Northumberland Strait did select foraging areas based on prey availability (Figure 2), driven mainly by selection of areas high in bivalves (simper: 50% dissimilarity, p = 0.02). Dispersion of prey community biomass measures in the Bay of Fundy varied between sites (permdisp: F1,120 = 4.15, p = 0.04), with Johnson’s Mills having higher dispersion than Pecks Cove. Dispersion was similar between sites on the Northumberland Strait (F1,122 = 0.96, p = 0.33).
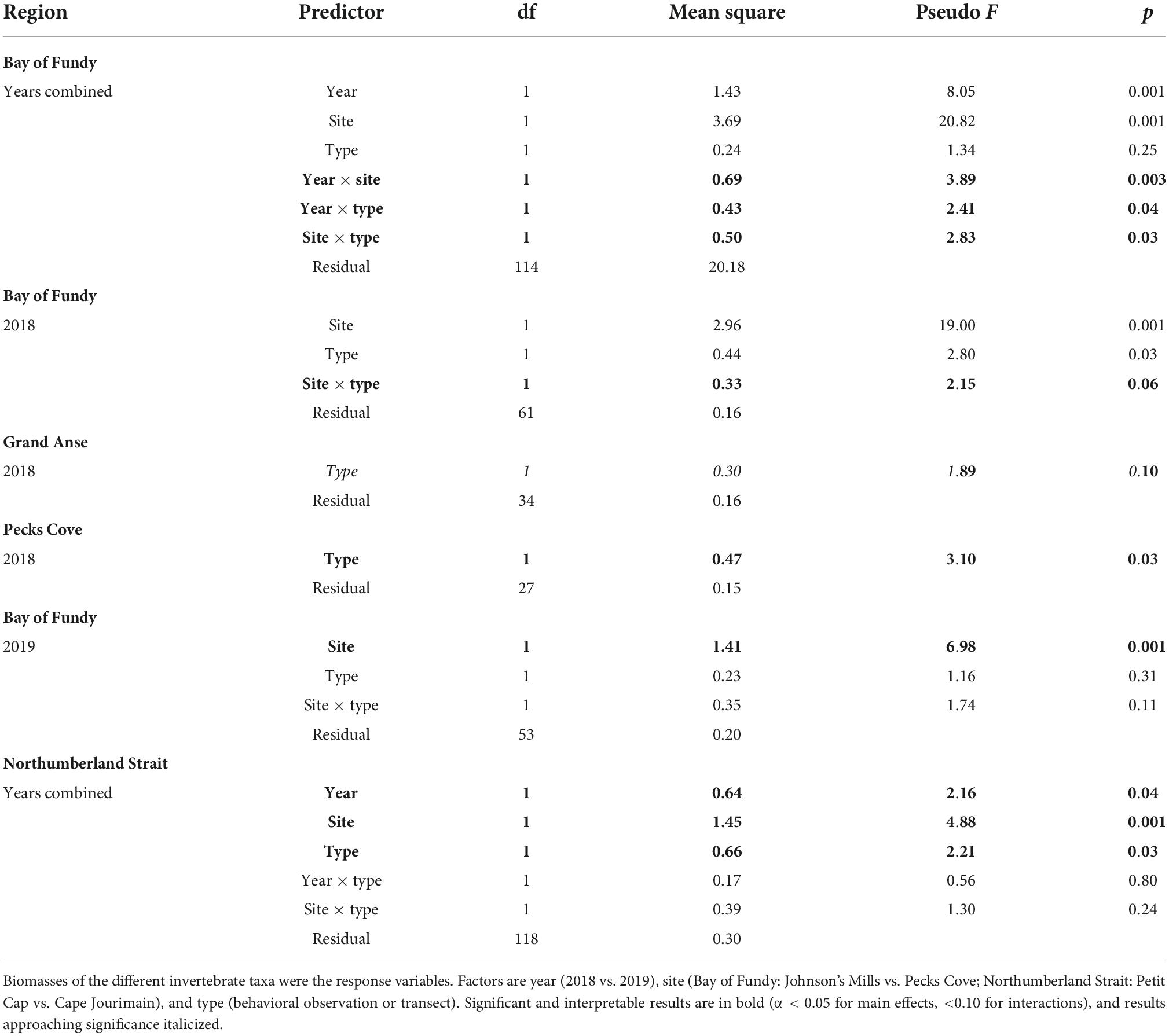
Table 2. Permutational multivariate analysis of variances (permutations = 999) of separate models analyzing prey biomass split by region.
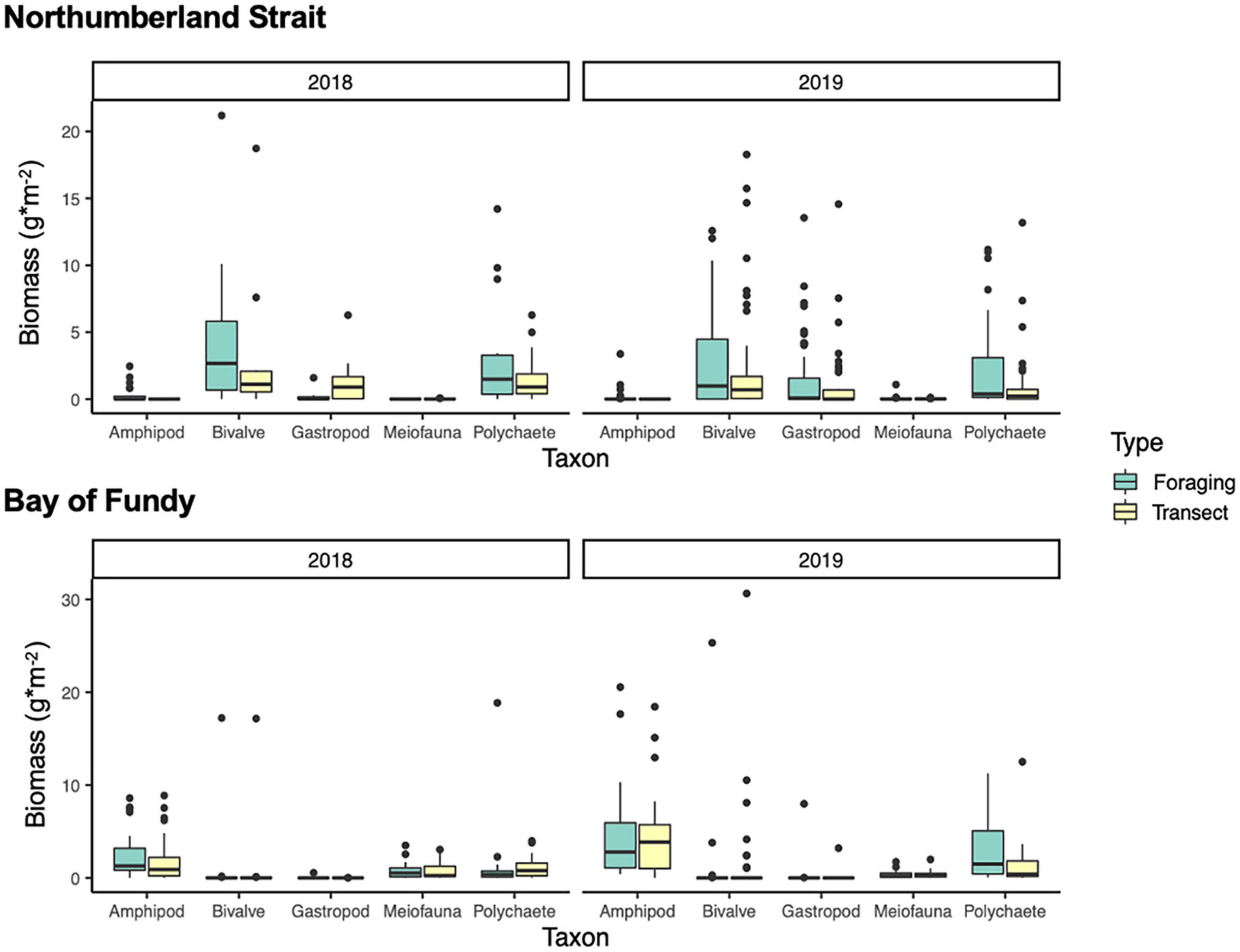
Figure 2. Dry biomass (g⋅m–2) of invertebrates in 2018 (left) and 2019 (right) in the Northumberland Strait (top) and Bay of Fundy (bottom) in locations where birds were foraging (represented selected habitat) and on transects (representing available habitat). Center line represents the median, with first and third quartiles shown in boxes. Whiskers show interquartile range. Points show data beyond the 5th and 95th percentile. Note that the y-axis scale differs between regions.
In the Northumberland Strait in 2019 more biomass was available at both study sites later in the season (X21 = 7.15, p = 0.007, see Supplementary Figure 1). Conversely, prey availability was consistent throughout migration in the Bay of Fundy (BoF Time: X21 = 0.86, p = 0.36). Using extracted variance components, we found that variation in both regions was largely at the level of sample (BoF: 79.8%, NuS: 77.3%), though sites in the Northumberland Strait appeared to have more variability than those in the Bay of Fundy (BoF: 7.2%, NuS: 11.1%). There was also a significant difference in variability between regions (Levene’s test: F1,167 = 18.89, p < 0.0001); the Northumberland Strait was more variable than the Bay of Fundy.
3.2 Estimated dietary niche
In 2018 the dietary niche based on blood plasma signatures for δ15N and δ13C of juvenile birds tended to be broader than adults, especially at Petit Cap (Figure 3), though a corresponding permdisp analysis found niches were similarly dispersed between adults and juveniles at both sites (Petit Cap: F1,27 = 1.72, p = 0.20; Johnson’s Mills: F1,43 = 1.06, p = 0.31). The sample size of juveniles at Petit Cap in 2018 was small (n = 8), probably leading to low statistical power. In 2019 the juvenile niche at Petit Cap was more widely dispersed than adults (Figure 3, permdisp: F1,26 = 3.90, p = 0.04). At Johnson’s Mills, juveniles appeared to have a narrower niche than adults, though the difference only approached significance (Figure 3, permdisp: F1,48 = 3.51, p = 0.07).
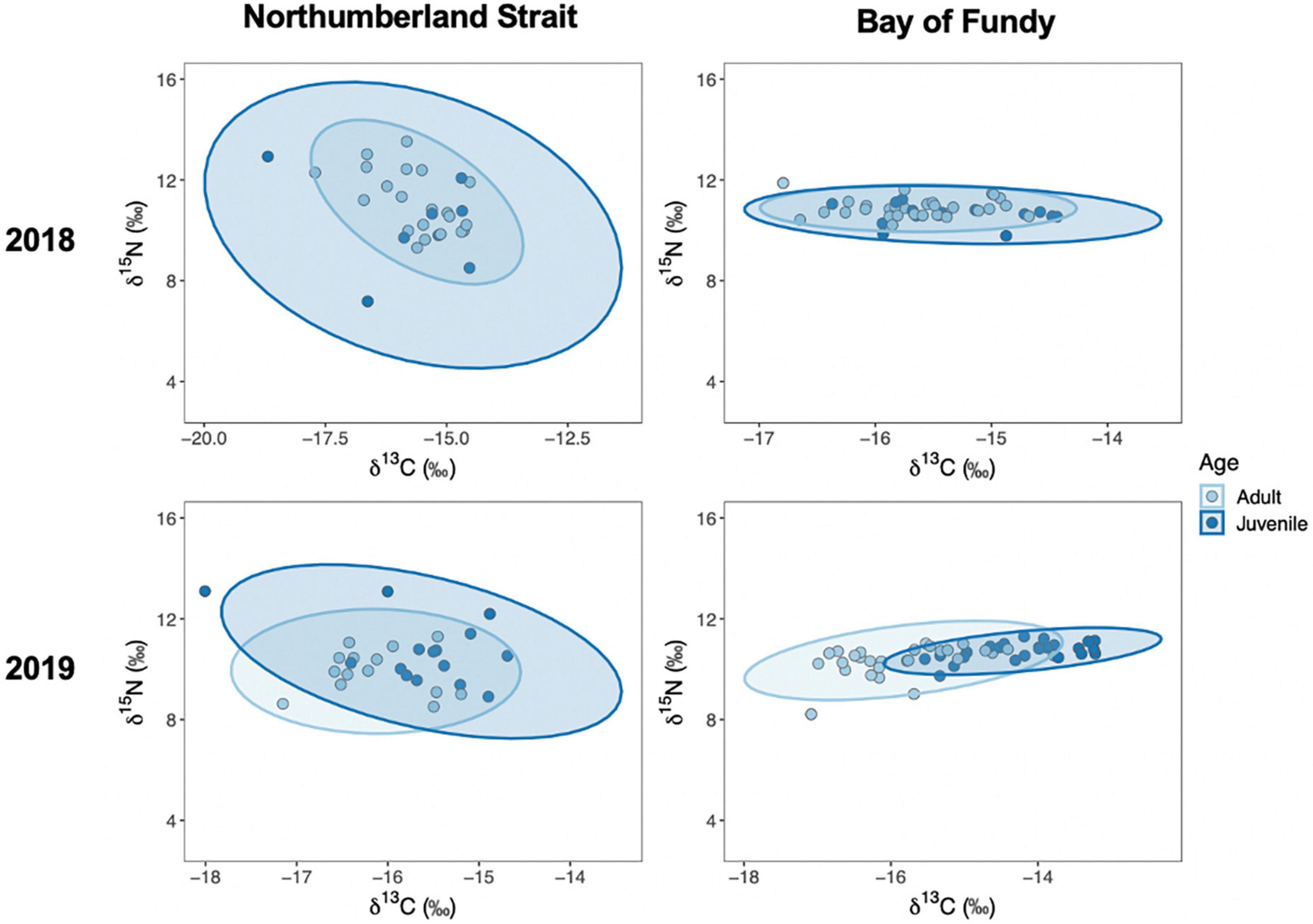
Figure 3. Isotopic dietary niches of Semipalmated Sandpiper adults (light blue) and juveniles (dark blue) captured at Petit Cap (left: 2018: Nadult = 21, Njuvenile = 8, 2019: Nadult = 14, Njuvenile = 14) and Johnson’s Mills (right: 2018: Nadult = 31, Njuvenile = 14, 2019: Nadult = 28, Njuvenile = 24) in 2018 (top), and in 2019 (bottom). Ellipses represent maximum likelihood estimates and were developed using δ15N and δ13C isotope signatures of blood plasma in parts per thousand.
3.3 Plasma metabolites
Birds sampled at Petit Cap had higher TRIG values than those captured at Johnson’s Mills, and there were no differences among ages or years (Table 3 and Figure 4). There was no relationship between TRIG values and bleed time, but there was a positive relationship between relative fuel loads and TRIG values (Table 3).
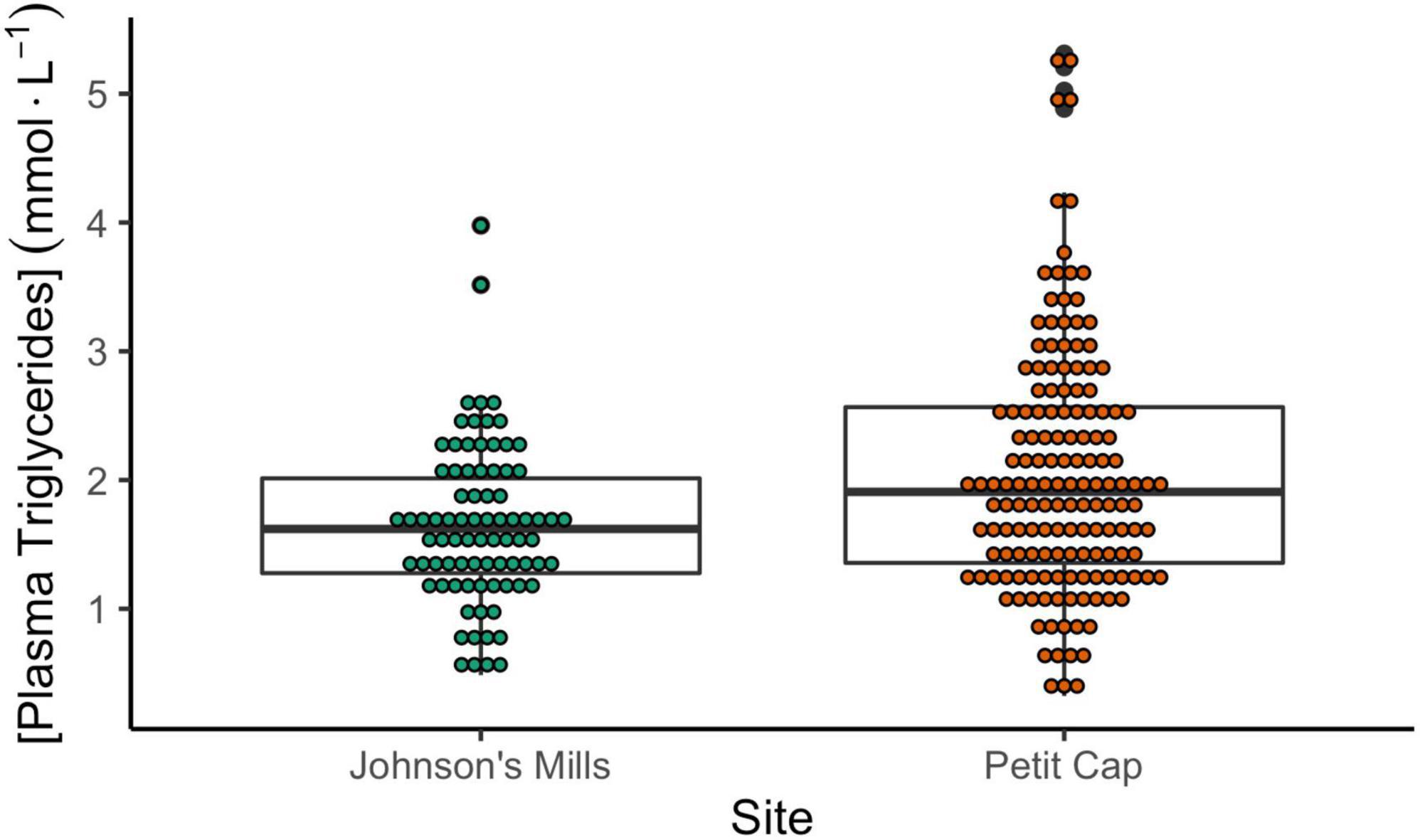
Figure 4. Concentration of plasma triglyceride values (mmol⋅L–1) of Semipalmated Sandpipers captured at Johnson’s Mills (Bay of Fundy) and Petit Cap (Northumberland Strait). Ages are pooled. Points show values from individual birds. Results of statistical comparisons are provided in Table 3. Note that values used for analyses were standardized by relative fuel loads.
β-OH-butyrate levels varied by site and the amount of time between capture and bleeding, but not by age or relative fuel loads (Table 3). There was a positive relationship between time to bleeding and BOHB values. Sandpipers at Petit Cap had higher BOHB values than those sampled at Johnson’s Mills, in 2019 (Table 3). Combining data from 2019 and 2020 (due to a small sample size for juveniles in 2019), we found that juveniles had significantly higher BOHB than adults, with no effect of year, bleed time, or fuel loads (Table 3 and Figure 5). Among birds for which sex was determined, we found that sex did not affect levels of either metabolite (TRIG model: X21 = 1.97, p = 0.16, BOHB model: X21 = 0.64, p = 0.42).
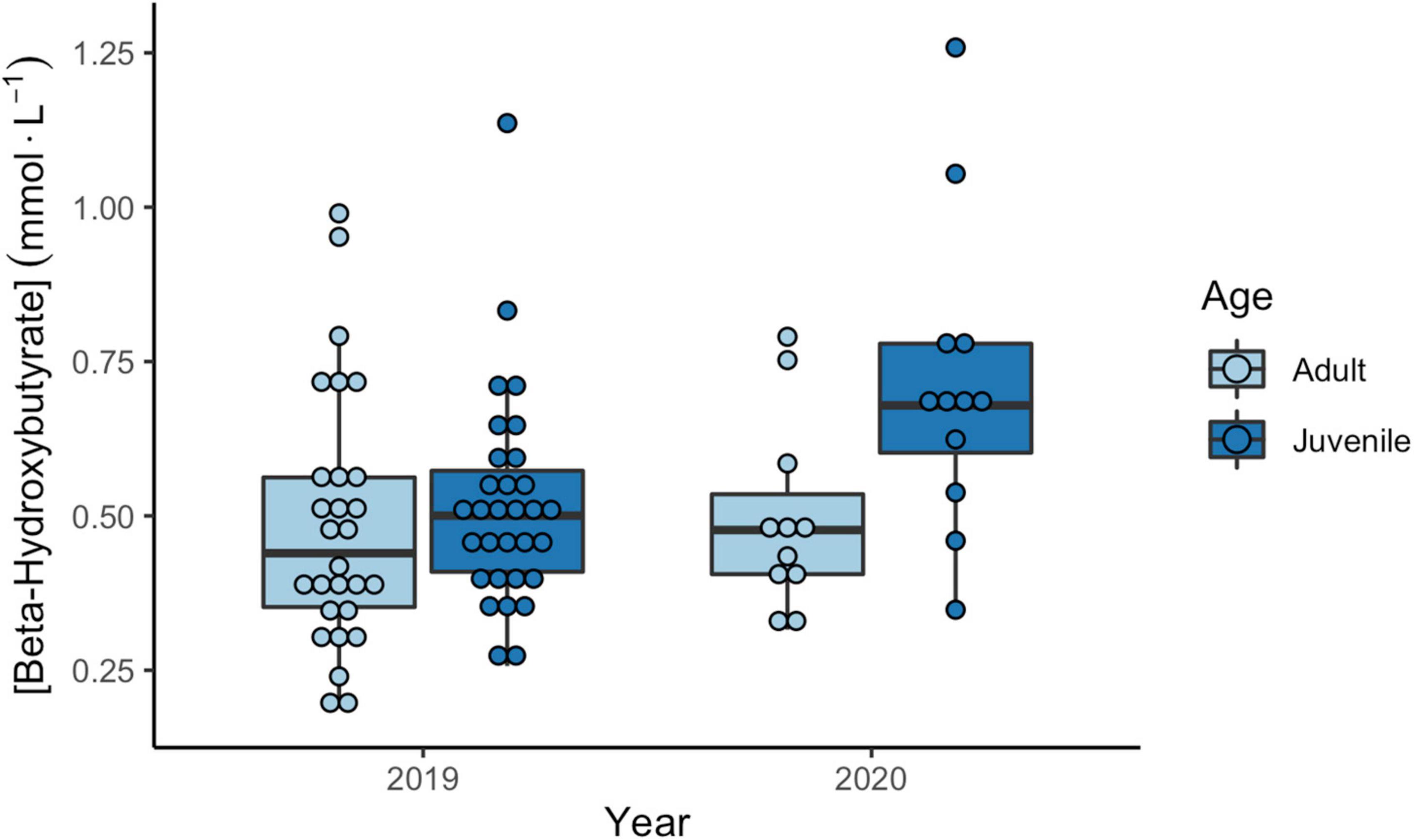
Figure 5. β-Hydroxybutyrate concentrations (mmol⋅L–1) of adult (light blue) and juvenile (dark blue) Semipalmated Sandpipers in 2019 (left) and 2020 (right) sampled at Petit Cap. Center line represents the median, with 1st and 3rd quartiles shown in boxes. Whiskers show interquartile range, and points show individual measures. Results of statistical comparisons are provided in Table 3.
Among tagged birds, TRIG values did not predict length of stay in Atlantic Canada (Table 4). We found that TRIG concentrations had a positive relationship with relative fuel loads and varied by both strategy and year but not by age (Table 4). TRIG values were higher in 2018 than 2019 (Figure 6) and were higher for birds using only sites outside the Bay than those tagged in the Bay of Fundy (Table 4). However, birds tagged at Petit Cap that subsequently moved into the Bay were not different from Bay of Fundy birds. Further, we found that birds at Petit Cap with lower TRIG were significantly more likely to move into the Bay of Fundy than those with higher TRIG values, particularly among adults, and that the best model to predict movement included TRIG and age, though age only approached significance (Table 5 and Figure 7). BOHB values appeared to vary by strategy, with higher levels for birds tagged at Petit Cap remaining outside the Bay than birds tagged in the Bay of Fundy (Table 4). BOHB did not vary by bleed time, relative fuel loads, or age (Table 4), though values trended higher for juveniles.
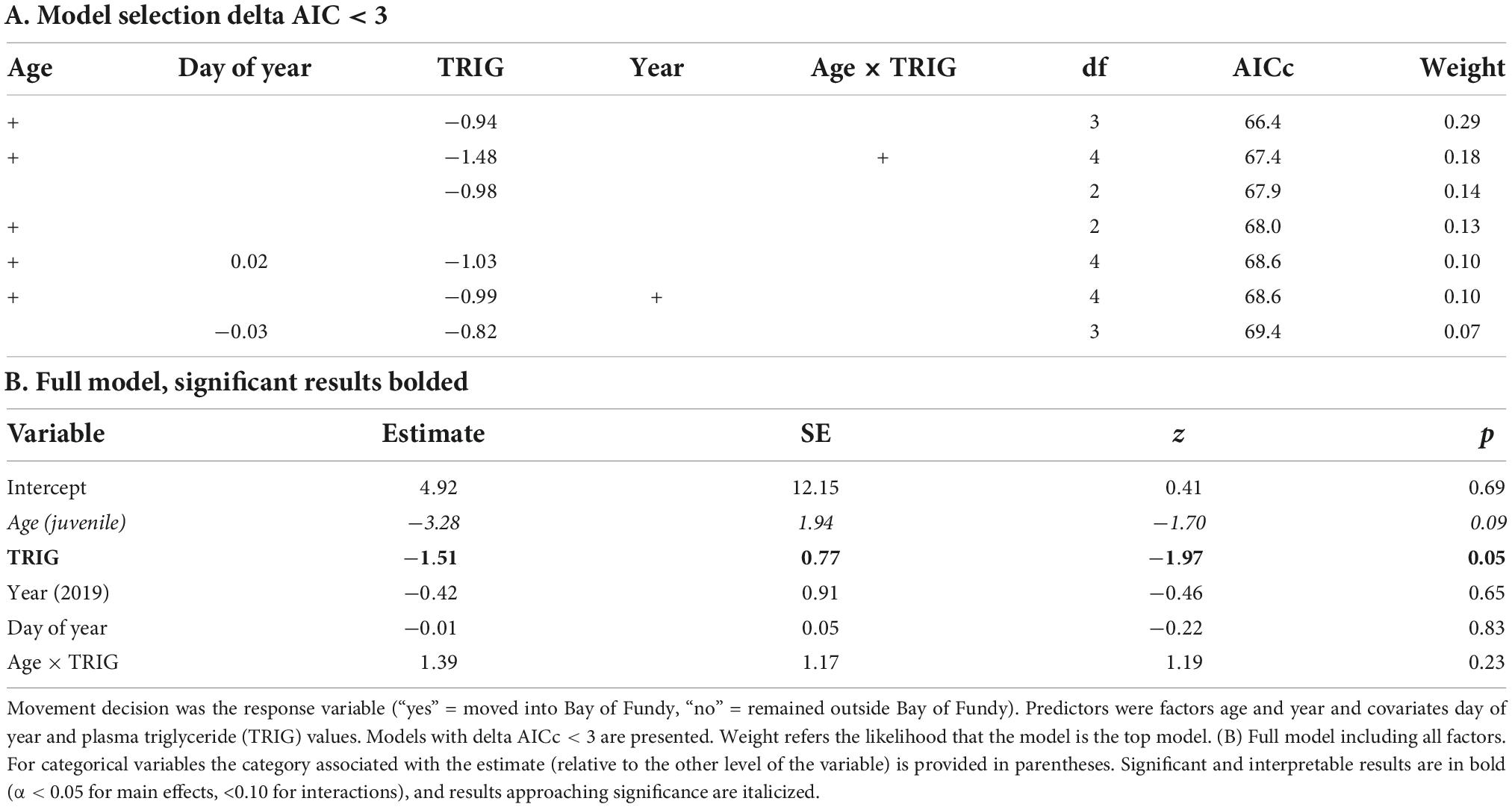
Table 5. AICc model selection (A) and full model output (B) of a binomial generalized linear model using data from birds tagged at Petit Cap.
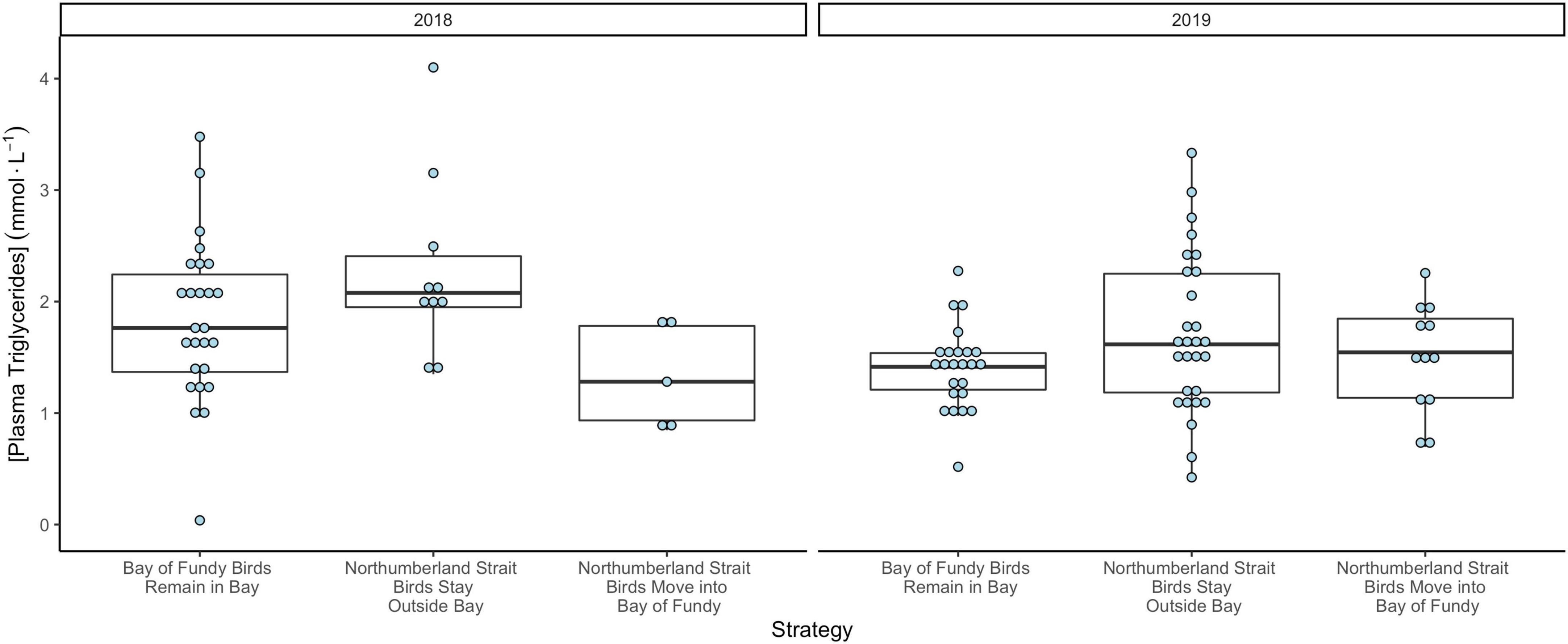
Figure 6. Plasma triglyceride concentrations (mmol⋅L–1) of Semipalmated Sandpipers in 2018 and 2019 using different movement strategies. Sandpipers sampled in the Bay of Fundy remained in the Bay, and those tagged on the Northumberland Strait either chose to stay on the coast or moved into the Bay. Results of statistical comparisons are provided in Table 4.
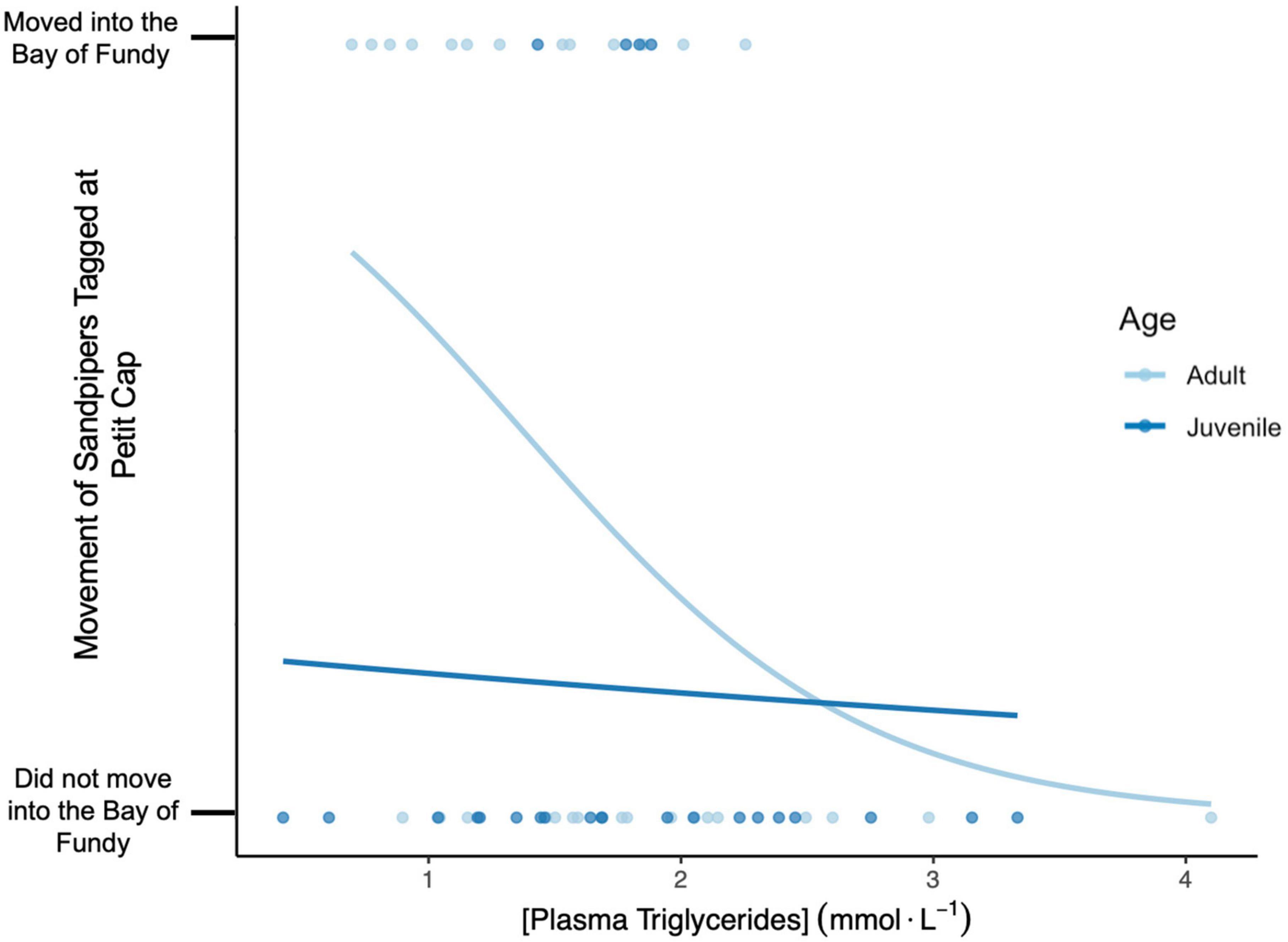
Figure 7. Movement decisions of tagged adult (light blue) and juvenile (dark blue) Semipalmated Sandpipers at Petit Cap (Northumberland Strait) relative to their corresponding plasma triglyceride concentration (mmol⋅L–1). The lines show predicted probability of movement for sandpipers tagged at Petit Cap into the Bay of Fundy dependent on TRIG concentration. Statistical results are provided in Table 5.
4 Discussion
4.1 Dietary niche and habitat variability
We found that the breadth of dietary niche and invertebrate availability varied between our study sites, and between years in each region. This is consistent with previous work conducted in the Bay of Fundy, which found variation in stable isotope signatures and invertebrate availability between years (Quinn and Hamilton, 2012). Our results suggest that annual differences also occur at sites outside the Bay of Fundy. Shorebird diets reflect the invertebrate availability at a site, and they are highly flexible as they encounter many different prey items throughout their life cycle (Hicklin and Smith, 1979; Skagen and Oman, 1996; Tsipoura and Burger, 1999; MacDonald et al., 2012; Gerwing et al., 2016). Sites on the Northumberland Strait had more variable prey abundance than those in the Bay of Fundy, and overall exhibit a wider range of habitat types. This is reflected in the observed dietary niches of sandpipers; birds sampled at Petit Cap appeared to have broader niches than birds at Johnson’s Mills (Figure 3). We also detected some differences in the breadth of dietary niches between adult and juvenile birds. Juveniles may have a broader niche than adults foraging on the Northumberland Strait. There are a number of possible explanations for this. First, juveniles use a broader range of sites along the Strait than adults (Doiron, 2021; Linhart et al., in press), so they may be exposed to a wider range of prey items, given the observed variability among sites in that region. Second, juveniles are inexperienced and unfamiliar with staging areas, which may cause them to sample the environment and forage more heavily on different food sources than would adults (Marchetti and Price, 1989), perhaps because they rely on prey that are readily available or easy to visualize (Wunderle, 1991). Juvenile sandpipers may also be forced to consume different prey items due to competition with adults, which has been seen in other bird species (Woodrey, 2000; Moore et al., 2003). However, passage of adults and juveniles is somewhat segregated in the region, with juveniles passing later (Gratto et al., 1984; Hicklin, 1987), so effects of such competition may be limited. Finally, they may be left with different choices due to changes in invertebrate availability through the season (Supplementary Figure 1).
4.2 Plasma metabolites and sandpiper movement
Sandpipers sampled at both of our study sites in Atlantic Canada exhibited high plasma triglyceride values relative to those seen in other parts of their range, including migratory (Lyons et al., 2008; Anderson et al., 2021) and non-migratory areas (Lyons et al., 2008; Linhart et al., 2022), consistent with the rapid weight gain they experience during staging on southbound migration. Sandpipers captured at Petit Cap (Northumberland Strait) had significantly higher TRIG and BOHB values than those captured at Johnson’s Mills (Bay of Fundy) when controlling for relative fuel loads and bleed time. This was a surprising finding, as higher fattening rates were expected in the Bay of Fundy, which hosts a large proportion of the Semipalmated Sandpiper population (Hicklin, 1987) and is recognized as critical shorebird habitat by the Western Hemisphere Shorebird Reserve Network (WHSRN Sites, 2021). The Bay of Fundy is food-dense, with large quantities of Corophium volutator, a high-quality prey item rich in essential fatty acids (Maillet and Weber, 2006). Quinn et al. (2017) found diet items (C. volutator, polychaetes, and biofilm) in the Bay of Fundy are rich in n-3 polyunsaturated fatty acids which are essential for migratory flight and contribute to TRIG levels. Less is known about prey quality at sites outside the Bay, though our results suggest sandpipers were selective of bivalves and polychaete worms which are known to be quality food items elsewhere (Stillman et al., 2005; Quinn et al., 2017; Mogle, 2021).
There are a number of potential explanations for the higher values of both TRIG and BOHB at Petit Cap. It is possible birds using sites outside the Bay are gaining weight faster, reflected through higher TRIG values. Alternatively, a diet high in fat could also generate high TRIG levels. Macromolecular composition of prey items has been shown to impact plasma triglyceride levels in birds (Smith et al., 2007; Smith and McWilliams, 2009). Based on prey available in locations where sandpipers chose to forage on the Northumberland Strait, they appeared to be consuming many bivalves, which are high in lipids (Mogle, 2021) and could explain the elevated TRIG levels we detected. A similar trend of high-fat diets being associated with high TRIG levels has been seen in Western Sandpipers (C. mauri; Seaman et al., 2005). The apparent paradox of high BOHB, which is typically elevated when fasting (Jenni-Eiermann and Jenni, 1991, 1996), present with elevated TRIG in foraging sandpipers at Petit Cap may also be diet driven. A diet high in fat and low in carbohydrates could force sandpipers into ketosis and cause them to burn fat as fuel, which has been seen in poultry (Ohtsu et al., 2013). This could result in elevated BOHB values, even when birds were actively foraging, which was the case for birds captured at Petit Cap.
It is also possible that different capture methods and timing contributed to observed differences between birds sampled at Johnson’s Mills and Petit Cap. Lower TRIG values at Johnson’s Mills could be consistent with birds that were roosting, not foraging as they were at Petit Cap, because they had time to deposit free-flowing triglycerides as fat, lowering the amount measurable by our method. However, we found no evidence in adults and only limited evidence in juveniles of a decline in TRIG levels with increasing roosting time (Supplementary Figure 2 and Supplementary Table 1). Therefore, it is unlikely that this would explain the difference between sites. BOHB was also unrelated to roosting time (Supplementary Figure 3 and Supplementary Table 2), so methodological differences are likely not relevant to this result either. Further, in an effort to assess possible effects of time of day on results, we compared birds captured during evening vs. night at Petit Cap and again found only limited evidence of effects (Supplementary Figure 4 and Supplementary Table 3). This is not surprising, as Semipalmated Sandpipers are known to feed actively during day and night in Atlantic Canada (Ouellette, 2021).
Regardless of the precise mechanism, the elevated TRIG values relative to other studies conducted outside the migration period (e.g., Lyons et al., 2008; Linhart et al., 2022) suggest that Semipalmated Sandpipers are able to gain weight efficiently at both Bay of Fundy and Northumberland Strait sites using a diverse range of prey items, reflective of the opportunistic foraging noted for the species elsewhere in Atlantic Canada (MacDonald et al., 2012). The potentially higher fattening rates observed on the Northumberland Strait may also contribute to shorter lengths of stay recorded for birds using sites outside the Bay (Linhart et al., in press).
Consistent with most other studies on Semipalmated Sandpipers (Lyons et al., 2005, 2008; Henkel and Taylor, 2015), we found no evidence of differences in TRIG levels among ages. However, in our study juveniles had BOHB concentrations 27–36% higher than adults. This result is also consistent with other studies investigating plasma metabolites in adult and juvenile sandpipers (Guglielmo et al., 2002; Lyons et al., 2008; Thomas and Swanson, 2013), and could suggest juveniles may be prone to more fasting or higher energy expenditures than adults. Juvenile sandpipers tended to explore more staging sites than adults (Linhart et al., in press), which exhibit strong regional fidelity (Neima et al., 2020). These additional movements may cause them to burn fat throughout their staging period, generating elevated BOHB levels. It is also possible the additional movements and diet choices of juveniles have compounding effects, leading to the higher BOHB levels we detected. Finally, due to their later arrival in the region juveniles may also be exposed to higher predation risk from migrating raptors, as seen in other species (Tringa glareola; Wichmann et al., 2004). Predator presence, largely attributable to the return of nesting Peregrine Falcons (Falco peregrinus) in the region, has been shown to affect sandpiper movement and habitat use (Sprague et al., 2008; Dekker et al., 2011), resulting in more energy expended in over ocean flocking during periods when they should be roosting (Dekker et al., 2011).
Among birds tagged at Petit Cap, those with lower TRIG levels were more likely to move into the Bay of Fundy, remaining there until they resumed their migration. Although there was no statistical interaction with age, this result was primarily driven by adults, with little evidence in juveniles, probably because juveniles in general were less likely to move into the Bay of Fundy (Linhart et al., in press). It is possible that sandpipers that were gaining weight less efficiently (as shown by lower TRIG levels) on the Northumberland Strait chose to move into the food-dense Bay of Fundy. Alternatively, this result could be an artifact of capture timing relative to migration strategy. Theoretically, some birds could have been captured during a brief stop on the Northumberland Strait while en route to the Bay of Fundy, and therefore may not have begun trying to refuel. However, if this was true we should have seen more tagged sandpipers moving into the Bay earlier in the season, when the bulk of arrivals would have happened. Linhart et al. (in press) found no evidence of this, and there was no effect of day of year in our analysis. Further, the Strait and Bay are only 40–50 km apart, making such intermediate stops unlikely. We therefore suggest that birds may be making decisions about whether to move based on success in refueling. Lyons et al. (2008) compared TRIG and BOHB values between sites at different migratory stages and found substantial differences in both metabolites, but they did not include movement between regions in their study. To our knowledge, our study is the first to detect a relationship between fattening rates, as measured by plasma triglycerides, and habitat selection decisions by individual shorebirds. Although this relationship needs additional study, it suggests that individual Semipalmated Sandpipers may vary in their ability to gain weight efficiently in particular habitats, and those that cannot refuel adequately may recognize this and adjust their habitat use.
5 Conclusion and conservation implications
The Bay of Fundy is one of the most important fall shorebird staging sites in the Atlantic Flyway and has been the focus of migratory shorebird conservation efforts for decades. Our work highlights the importance of conserving less recognized staging sites throughout Atlantic Canada. Not only are these sites outside the Bay used by a portion of the staging Semipalmated Sandpiper population (Linhart et al., in press), they offer a suitable prey base that may even allow birds using them to fatten more quickly than those staging in the Bay of Fundy. We also found that although juvenile sandpipers tended toward broader dietary niches, there were no differences in TRIG values, and by extension fattening rates, between the ages. This suggests juveniles that are using a more diverse prey base are still able to gain weight as effectively as adults. Additionally, the higher BOHB values we recorded in juvenile birds suggests they are possibly fasting more or expending more energy than adults, which aligns with the idea that juveniles are sampling more sites than adults while staging in Atlantic Canada (Linhart et al., in press) and as a result need to remain in the region slightly longer (Mann et al., 2017; Linhart et al., in press). Most notably, our plasma triglyceride and movement results suggest that individual sandpipers may make decisions about movement and habitat use based on their physiological state.
Over the past 40 years, shorebird habitat conservation in North America has focused on identifying and protecting important shorebird breeding, staging, and non-breeding sites where importance is typically defined by bird abundance. The Western Hemisphere Shorebird Reserve Network (WHSRN) has identified and nominated 112 sites in 18 countries throughout the Americas to date (WHSRN Sites, 2021) and has been extremely successful at raising awareness for shorebird conservation and protecting important habitat. However, to qualify at the lowest level, a site has to support a least 1% of the biogeographic population of a species or 20,000 shorebirds annually. Given resource limitations and the need to target sites with the biggest conservation payoff, thresholds like this are necessary. However, this means that the value of smaller sites may not be recognized, leaving them vulnerable to a variety of threats such as coastal development and human disturbance. Results from our study, coupled with those of Linhart et al. (in press), suggest that small sites in Atlantic Canada like those in the Northumberland Strait are indeed important to Semipalmated Sandpipers. This appears to be especially true for juvenile sandpipers, which appear to make use of a greater number of sites during staging (Linhart et al., in press) and potentially throughout migration (Van Brederode and Roersma, 2020). Our results point to the importance of conserving these sites. We suggest that in the future shorebird habitat conservation actions should consider the importance of smaller sites with multiple habitat types to support Semipalmated Sandpipers and other shorebird species during staging in Atlantic Canada and throughout the Western Hemisphere.
Data availability statement
The raw data supporting the conclusions of this article will be made available by the authors, without undue reservation. Movement data have been made available on Movebank (Movebank ID 2454115135).
Ethics statement
This animal study was reviewed and approved by the Mount Allison University Animal Care Committee under guidelines from the Canadian Council on Animal Care.
Author contributions
DH, JP, and RL conceived the study idea. RL wrote the manuscript and analyzed the data with substantial input from DH. DH and JP contributed to the resources and funding. All authors collected the data and assisted in editing the manuscript.
Funding
This work was funded by the Natural Sciences and Engineering Research Council of Canada (NSERC) Discovery Grant RGPIN-2017-05429 to DH, NSERC CRD grant CRDPJ 543678–19 to P. Taylor et al., Environment and Climate Change Canada (JP), the New Brunswick Wildlife Trust Fund (DH), Canada Summer Jobs, NBIF, Career Launcher Internships (Environment and Climate Change Canada), and Mount Allison University.
Acknowledgments
We thank V. Ouellette, A. MacDonald, M. Mogle, H. Mann, B. Nurse, J. Arseneault, and M. Fyfe and numerous volunteers for assistance in the field. We also thank to K. L. Morris-Cormier and the Johnson’s Mills Interpretive Centre Staff, to Birds Canada, especially L. Berrigan for tower maintenance, and to the Motus Wildlife Tracking System for data collection and processing. We appreciated the guidance from A. Morash and C. Morrissey in interpreting plasma metabolite results. M. A. Villard, A. Anderson, and K. Crosby provided comments on earlier versions of this manuscript.
Conflict of interest
The authors declare that the research was conducted in the absence of any commercial or financial relationships that could be construed as a potential conflict of interest.
Publisher’s note
All claims expressed in this article are solely those of the authors and do not necessarily represent those of their affiliated organizations, or those of the publisher, the editors and the reviewers. Any product that may be evaluated in this article, or claim that may be made by its manufacturer, is not guaranteed or endorsed by the publisher.
Supplementary material
The Supplementary Material for this article can be found online at: https://www.frontiersin.org/articles/10.3389/fevo.2022.1059005/full#supplementary-material
References
Anderson, A. M., Duijns, S., Smith, P. A., Friis, C., and Nol, E. (2019). Migration distance and body condition influence shorebird migration strategies and stopover decisions during southbound migration. Front. Ecol. Evol. 7:251. doi: 10.3389/fevo.2019.00251
Anderson, A. M., Friis, C., Gratto-Trevor, C. L., Harris, C. M., Love, O. P., Morrison, R. I. G., et al. (2021). Drought at a coastal wetland affects refuelling and migration strategies of shorebirds. Oecologia 197, 661–674. doi: 10.1007/s00442-021-05047-x
Andres, B. A., Gratto-Trevor, C., Hicklin, P., Mizrahi, D., Morrison, R. I. G., and Smith, P. A. (2012). Status of the semipalmated sandpiper. Waterbirds 35, 146–148. doi: 10.1675/063.035.0114
Bartoń, K. (2022). MuMIn: Multi-model inference. R package version 1.46.0. Available online at: https://CRAN.R-project.org/package=MuMIn
Crewe, T. L., Crysler, Z., and Taylor, P. D. (2020). A walk through the use of R for Motus automated radio-telemetry data. Available online at: https://motus.org/motusRBook/archives/MotusRBook2018-01.pdf (accessed September 11, 2020).
Dekker, D., Dekker, I., Christie, D., and Ydenberg, R. (2011). Do staging semipalmated sandpipers spend the high-tide period in flight over the ocean to avoid falcon attacks along shore? Waterbirds 34, 195–201. doi: 10.1675/063.034.0208
Doiron, P. (2021). Differences in use of Northumberland strait habitat and fattening rates among migratory shorebird species. Sackville, NB: Mount Allison University.
Dunn, P. O., May, T. A., McCollough, M. A., and Howe, M. A. (1988). Length of stay and fat content of migrant semipalmated sandpipers in eastern Maine. Condor 90, 824–835. doi: 10.2307/1368839
Galbraith, H., Jones, R., Park, R., Clough, J., Herrod-Julius, S., Harrington, B., et al. (2002). Global climate change and sea level rise: Potential losses of intertidal habitat for shorebirds. Waterbirds 25, 173–183. doi: 10.1675/1524-4695(2002)025[0173:GCCASL]2.0.CO;2
Gerwing, T. G., Gerwing, A. M. A., Drolet, D., Barbeau, M. A., and Hamilton, D. J. (2015). Spatiotemporal variation in biotic and abiotic features of eight intertidal mudflats in the upper Bay of Fundy, Canada. Northeast. Nat. 22:12. doi: 10.1656/045.022.m1201
Gerwing, T. G., Kim, J. H., Hamilton, D. J., Barbeau, M. A., and Addison, J. A. (2016). Diet reconstruction using next-generation sequencing increases the known ecosystem usage by a shorebird. Auk 133, 168–177. doi: 10.1642/AUK-15-176.1
Goss-Custard, J. D., Warwick, R. M., Kirby, R., McGrorty, S., Clarke, R. T., Pearson, B., et al. (1991). Towards predicting wading bird densities from predicted prey densities in a post-barrage Severn estuary. J. Appl. Ecol. 28, 1004–1026. doi: 10.2307/2404222
Gratto, G. W., Thomas, M. L. H., and Gratto, C. L. (1984). Some aspects of the foraging ecology of migrant juvenile sandpipers in the outer Bay of Fundy. Can. J. Zool. 62, 1889–1892. doi: 10.1139/z84-276
Gratto-Trevor, C. L. (2004). The North American bander’s manual for banding shorebirds (Charadriiformes, suborder Charadrii). Point Reyes Station, CA: North American Banding Council.
Gratto-Trevor, C. L., Smith, P. A., Morrison, R. I. G., Aubry, Y., and Cotter, R. (2012b). Population trends in semipalmated sandpipers from migration counts. Waterbirds 35, 96–105. doi: 10.1675/063.035.0110
Gratto-Trevor, C. L., Morrison, R. I. G., Mizrahi, D., Lank, D. B., Hicklin, P., and Spaans, A. L. (2012a). Migratory connectivity of semipalmated sandpipers: Winter distribution and migration routes of breeding populations. Waterbirds 35, 83–95. doi: 10.1675/063.035.0109
Guglielmo, C. G., O’Hara, P. D., and Williams, T. D. (2002). Extrinsic and intrinsic sources of variation in plasma lipid metabolites of free-living western sandpipers (Calidris mauri). Auk 119, 437–445. doi: 10.1093/auk/119.2.437
Gutiérrez, J. S., Sabat, P., Castañeda, L. E., Contreras, C., Navarrete, L., Peña-Villalobos, I., et al. (2019). Oxidative status and metabolic profile in a long-lived bird preparing for extreme endurance migration. Sci. Rep. 9:17616. doi: 10.1038/s41598-019-54057-6
Hamilton, D. J., Barbeau, M. A., and Diamond, A. W. (2003). Shorebirds, mud snails, and Corophium volutator in the upper Bay of Fundy, Canada: Predicting bird activity on intertidal mud flats. Can. J. Zool. 81, 1358–1366. doi: 10.1139/z03-130
Hamilton, D. J., Diamond, A. W., and Wells, P. G. (2006). Shorebirds, snails, and the amphipod (Corophium volutator) in the upper Bay of Fundy: Top–down vs. bottom–up factors, and the influence of compensatory interactions on mudflat ecology. Hydrobiologia 567, 285–306. doi: 10.1007/s10750-006-0062-y
Henkel, J. R., and Taylor, C. M. (2015). Migration strategy predicts stopover ecology in shorebirds on the northern Gulf of Mexico. Anim. Migr. 2, 63–75. doi: 10.1515/ami-2015-0003
Herbert, J. A., Mizrahi, D., and Taylor, C. M. (2022). Migration tactics and connectivity of a nearctic–neotropical migratory shorebird. J. Anim. Ecol. 91, 819–830. doi: 10.1111/1365-2656.13670
Hicklin, P. W., and Smith, P. C. (1979). The diets of five species of migrant shorebirds in the Bay of Fundy. Proc. N. S. Inst. Sci. 29, 483–488.
Hicklin, P. W., and Smith, P. C. (1984). Selection of foraging sites and invertebrate prey by migrant semipalmated sandpipers, Calidris pusilla (Pallas), in minas basin, Bay of Fundy. Can. J. Zool. 62, 2201–2210. doi: 10.1139/z84-321
Hicklin, P. W., Hounsell, R. G., and Finney, G. H. (1989). Fundy pull trap: A new method of capturing shorebirds (Nuevo método para la captura de Playeros (Charadriiformes)). J. Field Ornithol. 60, 94–101.
Hitchcock, C. L., and Gratto-Trevor, C. (1997). Diagnosing a shorebird local population decline with a stage-structured population model. Ecology 78, 522–534. doi: 10.1890/0012-9658(1997)078[0522:DASLPD]2.0.CO;2
Hobson, K. A., and Clark, R. G. (1993). Turnover of 13 C in cellular and plasma fractions of blood: Implications for nondestructive sampling in avian dietary studies. Auk 110, 638–641. doi: 10.2307/4088430
Jackson, A. L., Inger, R., Parnell, A. C., and Bearhop, S. (2011). Comparing isotopic niche widths among and within communities: SIBER – stable isotope bayesian ellipses in R. J. Anim. Ecol. 80, 595–602. doi: 10.1111/j.1365-2656.2011.01806.x
Jehl, J. R. (2007). Disappearance of breeding semipalmated sandpipers from Churchill, Manitoba: More than a local phenomenon. Condor 109, 351–360. doi: 10.1093/condor/109.2.351
Jenni-Eiermann, S., and Jenni, L. (1991). High plasma triglyceride values in small birds during migratory flight: A new pathway for fuel supply during endurance locomotion at very high mass-specific metabolic rates? Physiol. Zool. 65, 112–123. doi: 10.1086/physzool.65.1.30158242
Jenni-Eiermann, S., and Jenni, L. (1994). Plasma metabolite levels predict individual body-mass changes in a small long-distance migrant, the garden warbler. Auk 111, 888–899. doi: 10.2307/4088821
Jenni-Eiermann, S., and Jenni, L. (1996). Metabolic differences between the postbreeding, moulting and migratory periods in feeding and fasting passerine birds. Funct. Ecol. 10, 62–72. doi: 10.2307/2390263
Kober, K., and Bairlein, F. (2006). Shorebirds of the bragantinian peninsula II. Diet and foraging strategies of shorebirds at a tropical site in Northern Brazil. Ornitol. Neotrop. 17, 549–562.
Langsrud, Ø (2003). ANOVA for unbalanced data: Use type II instead of type III sums of squares. Stat. Comput. 13, 163–167. doi: 10.1023/A:1023260610025
Linhart, R. C. (2021). Movement, habitat use and metabolic condition of semipalmated sandpiper (Calidris pusilla) during migratory stopover and non-breeding periods. Sackville, NB: Mount Allison University.
Linhart, R. C., Hamilton, D. J., Paquet, J., and Gratto-Trevor, C. L. (in press). Evidence of differing staging strategies between adult and juvenile semipalmated sandpipers (Calidris pusilla) highlights the importance of small staging sites in Atlantic Canada. Ornithology. doi: 10.1093/ornithology/ukac056
Linhart, R. C., Hamilton, D. J., Paquet, J., Monteiro, J. O. N., Ramires, G. P., and Mobley, J. A. (2022). Movement and habitat use of non-breeding semipalmated sandpiper (Calidris pusilla) at the Banco dos Cajuais in Northeast Brazil. Conserv. Sci. Pract. 4:e12683. doi: 10.1111/csp2.12683
Lyons, J. E., Collazo, J. A., and Guglielmo, C. (2005). “Plasma lipid metabolites and refueling performance of semipalmated sandpipers at migratory stopovers,” in Proceedings of the one hundred and twenty-third stated meeting of the American ornithologists’ union: Abstract book, Santa Barbara, CA, 140.
Lyons, J. E., Collazo, J. A., and Guglielmo, C. G. (2008). Plasma metabolites and migration physiology of semipalmated sandpipers: Refueling performance at five latitudes. Oecologia 155, 417–427. doi: 10.1007/s00442-007-0921-x
Ma, Z., Hua, N., Peng, H., Choi, C., Battley, P. F., Zhou, Q., et al. (2013). Differentiating between stopover and staging sites: Functions of the southern and northern yellow sea for long-distance migratory shorebirds. J. Avian Biol. 44, 504–512. doi: 10.1111/j.1600-048X.2013.00213.x
MacDonald, E. C., Ginn, M. G., and Hamilton, D. J. (2012). Variability in foraging behavior implications for diet breadth among semipalmated sandpipers staging in the upper Bay of Fundy. Condor 114, 135–144. doi: 10.1525/cond.2012.100246
Maillet, D., and Weber, J.-M. (2006). Performance-enhancing role of dietary fatty acids in a long-distance migrant shorebird: The semipalmated sandpiper. J. Exp. Biol. 209, 2686–2695. doi: 10.1242/jeb.02299
Mann, H. A. R., Hamilton, D. J., Paquet, J. M., Gratto-Trevor, C. L., and Neima, S. G. (2017). Effects of extreme tidal events on Semipalmated sandpiper (Calidris pusilla) migratory stopover in the Bay of Fundy, Canada. Waterbirds 40, 41–49. doi: 10.1675/063.040.0106
Marchetti, K., and Price, T. (1989). Differences in the foraging of juvenile and adult birds: The importance of developmental constraints. Biol. Rev. 64, 51–70. doi: 10.1111/j.1469-185X.1989.tb00638.x
McGowan, C. P., Hines, J. E., Nichols, J. D., Lyons, J. E., Smith, D. R., Kalasz, K. S., et al. (2011). Demographic consequences of migratory stopover: Linking red knot survival to horseshoe crab spawning abundance. Ecosphere 2:art69. doi: 10.1890/ES11-00106.1
McWilliams, S. R., Guglielmo, C., Pierce, B., and Klaassen, M. (2004). Flying, fasting, and feeding in birds during migration: A nutritional and physiological ecology perspective. J. Avian Biol. 35, 377–393. doi: 10.1111/j.0908-8857.2004.03378.x
Mogle, M. J. (2021). Dynamics of intertidal biofilm in relation to semipalmated sandpiper (Calidris pusilla) migratory stopover and assessments of benthic invertebrate and biofilm nutritional content in the upper Bay of Fundy, Canada. Sackville, NB: Mount Allison University.
Moore, F., Mabey, S., and Woodrey, M. (2003). “Priority access to food in migratory birds: Age, sex and motivational asymmetries,” in Avian migration, eds P. Berthold, E. Gwinner, and E. Sonnenschein (Berlin: Springer Berlin Heidelberg), 281–292. doi: 10.1007/978-3-662-05957-9_19
Morrison, R. I. G., and Harrington, B. A. (1992). The migration system of red knot Calidris canutus rufa in the new world. Wader Study Group Bull. 64, 71–84.
Morrison, R. I. G., Mizrahi, D. S., Ross, R. K., Ottema, O. H., de Pracontal, N., and Narine, A. (2012). Dramatic declines of semipalmated sandpipers on their major wintering areas in the guianas, Northern South America. Waterbirds 35, 120–134. doi: 10.1675/063.035.0112
Neima, S. G., Hamilton, D. J., Gratto-Trevor, C. L., and Paquet, J. (2020). Intra- and interannual regional fidelity of semipalmated sandpipers (Calidris pusilla) during migratory stopover in the upper Bay of Fundy, Canada. Avian Conserv. Ecol. 15:art14. doi: 10.5751/ACE-01561-150114
Neima, S. G., Linhart, R. C., Hamilton, D. J., Gratto-Trevor, C. L., and Paquet, J. (2022). Length of stay and departure strategies of semipalmated sandpipers (Calidris pusilla) during post-breeding migration in the upper Bay of Fundy, Canada. Front. Ecol. Evol. 10:897197. doi: 10.3389/fevo.2022.897197
North American Bird Conservation Initiative Canada (2019). The state of Canada’s birds, 2019. Environment and climate change Canada, Ottawa, Canada, 12. Available online at: http://www.stateofcanadasbirds.org/
Ohtsu, H., Yakabe, Y., Yamazaki, M., Murakami, H., and Abe, H. (2013). Plasma lipid profiles and redox status are modulated in a ketogenic diet-induced chicken model of ketosis. J. Poult. Sci. 50, 212–218. doi: 10.2141/jpsa.0120161
Oksanen, J., Blanchet, G., Friendly, M., Kindt, R., Legendre, P., McGlinn, D., et al. (2020). vegan: Community ecology package. R package version 2.6-0. Available online at: https://github.com/vegandevs/vegan
Ouellette, V. (2021). Nocturnal and diurnal feeding behaviour of migratory shorebirds at a Northumberland strait stopover site. Sackville, NB: Mount Allison University.
Pyle, P. (2008). Identification guide to North American birds. Part II: Anatidae to alcidae. Point Reyes Station, CA: Slate Creek Press.
Quinn, J. T., and Hamilton, D. J. (2012). Variation in diet of semipalmated sandpipers (Calidris pusilla) during stopover in the upper Bay of Fundy, Canada. Can. J. Zool. 90, 1181–1190. doi: 10.1139/z2012-086
Quinn, J. T., Hamilton, D. J., and Hebert, C. E. (2017). Fatty acid composition and concentration of alternative food of semipalmated sandpipers (Calidris pusilla) in the upper Bay of Fundy, Canada. Can. J. Zool. 95, 565–573. doi: 10.1139/cjz-2016-0246
R Core Team (2020). R: A language and environment for statistical computing. Vienna: R Foundation for Statistical Computing.
Ramenofsky, M. (1990). “Fat storage and fat metabolism in relation to migration,” in Bird migration, ed. E. Gwinner (Berlin: Springer Berlin Heidelberg), 214–231. doi: 10.1007/978-3-642-74542-3_15
Seaman, D. A., Guglielmo, C. G., and Williams, T. D. (2005). Effects of physiological state, mass change and diet on plasma metabolite profiles in the western sandpiper Calidris mauri. J. Exp. Biol. 208, 761–769. doi: 10.1242/jeb.01451
Skagen, S. K., and Oman, H. D. (1996). Dietary flexibility of shorebirds in the western hemisphere. Can. Field Nat. 110, 419–444.
Smith, S. B., and McWilliams, S. R. (2009). Dietary macronutrients affect lipid metabolites and body composition of a migratory passerine, the white-throated sparrow (Zonotrichia albicollis). Physiol. Biochem. Zool. 82, 258–269. doi: 10.1086/597519
Smith, S. B., McWilliams, S. R., and Guglielmo, C. G. (2007). Effect of diet composition on plasma metabolite profiles in a migratory songbird. Condor 109, 48–58. doi: 10.1093/condor/109.1.48
Sprague, A. J., Hamilton, D. J., and Diamond, A. W. (2008). Site safety and food affect movements of semipalmated sandpipers (Calidris pusilla) migrating through the upper Bay of Fundy. Avian Conserv. Ecol. 3:art4. doi: 10.5751/ACE-00252-030204
Stevens, L. (1996). Avian biochemistry and molecular biology, 1st Edn. Cambridge: Cambridge University Press, 272. doi: 10.1017/CBO9780511525773
Stillman, R., West, A., Goss-Custard, J., McGrorty, S., Frost, N., Morrisey, D., et al. (2005). Predicting site quality for shorebird communities: A case study on the Humber estuary, UK. Mar. Ecol. Prog. Ser. 305, 203–217. doi: 10.3354/meps305203
Taylor, P. D., Crewe, T. L., Mackenzie, S. A., Lepage, D., Aubry, Y., Crysler, Z., et al. (2017). The Motus wildlife tracking system: A collaborative research network to enhance the understanding of wildlife movement. Avian Conserv. Ecol. 12:art8. doi: 10.5751/ACE-00953-120108
Thomas, N. E., and Swanson, D. L. (2013). Plasma metabolites and creatine kinase levels of shorebirds during fall migration in the prairie pothole region. Auk 130, 580–590. doi: 10.1525/auk.2013.12169
Tsipoura, N., and Burger, J. (1999). Shorebird diet during spring migration stopover on Delaware bay. Condor 101, 635–644. doi: 10.2307/1370193
Van Brederode, N. E., and Roersma, H. J. (2020). Turnover, passage population size and flight range of juvenile ruddy turnstones at a non-estuarine staging site in the Netherlands. Ardea 108, 213–222. doi: 10.5253/arde.v108i2.a9
Warnock, N. (2010). Stopping vs. Staging: The difference between a hop and a jump. J. Avian Biol. 41, 621–626. doi: 10.1111/j.1600-048X.2010.05155.x
WHSRN Sites (2021). WHSRN sites–WHSRN. Available online at: https://whsrn.org/whsrn-sites/ (accessed September 3, 2021).
Wichmann, G., Barker, J., Zuna-Kratky, T., Donnerbaum, K., and Rössler, M. (2004). Age-related stopover strategies in the wood sandpiper Tringa glareola. Ornis Fenn. 81, 169–179.
Williams, T. D., Guglielmo, C. G., and Martyniuk, C. J. (1999). Plasma lipid metabolites provide information on mass change over several days in captive western sandpipers. Auk 116, 994–1000. doi: 10.2307/4089679
Woodrey, M. (2000). Age-dependent aspects of stopover biology of passerine migrants. Stud. Avian Biol. 20, 43–52.
Keywords: Semipalmated Sandpiper, Calidris pusilla, migratory physiology, habitat selection, shorebird conservation, automated radio-telemetry, dietary niche, stable isotopes
Citation: Linhart RC, Hamilton DJ, Paquet J, Bellefontaine SC, Davis S, Doiron PB and Gratto-Trevor CL (2022) Variation in resource use between adult and juvenile Semipalmated Sandpipers (Calidris pusilla) and use of physiological indicators for movement decisions highlights the importance of small staging sites during southbound migration in Atlantic Canada. Front. Ecol. Evol. 10:1059005. doi: 10.3389/fevo.2022.1059005
Received: 30 September 2022; Accepted: 28 November 2022;
Published: 22 December 2022.
Edited by:
Arnaldo Marín, University of Murcia, SpainReviewed by:
David L. Swanson, University of South Dakota, United StatesJames Lyons, Patuxent Wildlife Research Center (USGS), United States
Copyright © 2022 Linhart, Hamilton, Paquet, Bellefontaine, Davis, Doiron and Gratto-Trevor. This is an open-access article distributed under the terms of the Creative Commons Attribution License (CC BY). The use, distribution or reproduction in other forums is permitted, provided the original author(s) and the copyright owner(s) are credited and that the original publication in this journal is cited, in accordance with accepted academic practice. No use, distribution or reproduction is permitted which does not comply with these terms.
*Correspondence: Rebeca C. Linhart, cmViZWNhbGluaGFydEB1cmkuZWR1
†Present Address: Rebeca C. Linhart, Department of Natural Resources Science, University of Rhode Island, Kingston, RI, United States
‡ORCID: Rebeca C. Linhart, orcid.org/0000-0003-3445-3385; Diana J. Hamilton, orcid.org/0000-0003-3917-2566; Julie Paquet, orcid.org/0000-0003-0317-6506; Sara C. Bellefontaine, orcid.org/0000-0002-5541-2972; Siena Davis, orcid.org/0000-0001-6719-0142; Parker B. Doiron, orcid.org/0000-0002-6266-3358