- 1School of Civil Engineering, Henan Polytechnic University, Jiaozuo, Henan, China
- 2GongQing Institute of Science and Technology, Gongqingchengshi, Jiangxi, China
- 3Hubei Key Laboratory of Disaster Prevention and Mitigation, China Three Gorges University, Yichang, Hubei, China
- 4Chinese Academy of Sciences and Ministry of Water Resources, Institute of Mountain Hazards and Environment, Chengdu, Sichuan, China
- 5Faculty of Public Safety and Emergency Management, Kunming University of Science and Technology, Kunming, Yunnan, China
- 6Key Laboratory of Geological Disaster Risk Prevention and Control and Emergency Disaster Reduction of Ministry of Emergency Management of the People's Republic of China, Kunming University of Science and Technology, Kunming, Yunnan, China
- 7Key Laboratory of Early Rapid Identification, Prevention and Control of Geological Disasters in Traffic Corridor of High Intensity Earthquake Mountainous Area of Yunnan Province, Kunming University of Science and Technology, Kunming, Yunnan, China
- 8Sichuan Communication Surveying & Design Institute Co. Ltd., Chengdu, Sichuan, China
- 9Key Laboratory of Lower Yellow River Channel and Estuary Regulation, MWR, Yellow River Institute of Hydraulic Research (YRCC), Zhengzhou, China
By means of the Graphic Method, Gray Correlation Method and Frequency Method, the total amount of loose solid materials, the amount of loose solid materials per unit area in the source area, and the risk degree and critical rainfall of the study area are determined, respectively. On the basis of the calculation results, the total amount of loose solid materials is 57.2 × 104 m3, the amount of loose solid materials per unit area is 0.13 m3/m2 (greater than 0.1 m3/m2), which better meets the initiation conditions for dilute debris flow. The Hazard Evaluation Model of Debris Flow in earthquake areas is established by the gray correlation method and the hazard index H = 0.725 is determined. According to the hazard classification standards for debris flows in earthquake areas, the debris flow in Lecugou was moderate after the earthquake. The critical rainfall value of 1H, calculated by the frequency method, ranged from 17.45 to 22.21 mm (Re = 25 mm) and 8.17 to 13.01 mm (Re = 50 mm). The critical rainfall value at 10 min ranged from 6.23 to 8.44 mm (Re = 25 mm) and 2.92 to 5.13 mm (Re = 50 mm), respectively. The maximum rainfall intensity of 45.5 mm/h was reached between 10:00 and 11:00 on July 29, 2019 and the cumulative rainfall reached 144 mm, far exceeding the critical rainfall of Legugou. Under the influence of earthquakes and human activities, the loose solid source amount and loose solid source amount per unit area increased greatly, and the critical rainfall for debris flow after earthquakes decreased sharply, which induced debris flows under the influence of heavy rainfall. This research will be helpful for the establishment of monitoring and early warning systems based on artificial intelligence methods, and can greatly improve the effectiveness of disaster prevention and mitigation.
Introduction
A debris flow is a typical solid–liquid two-phase flow, which commonly causes huge economic losses and casualties every year. Debris flows destroy the regional ecological environment, aggravate the process of soil erosion and create wasteland; their influence is far-reaching (Liu et al., 2020). China is a large mountainous country, including plateaus and hills, with a mountainous area of 6.66 million km2, accounting for 69.4% of the total land area. The mountainous population accounts for more than one third of the total population of China (Chen and Su, 2001). Statistics show that the area of debris flow activity in China is about 4.8 million km2, accounting for 72.1% of China’s mountainous area, and there are an average of approximately 80,000 debris flow activities in China every year. Sichuan province is one of the provinces which experiences the most geological disasters in China and they tend to have characteristics such as: a wide area, large scale, quick disasters, high outbreak frequency and long duration (Tang, 2019). The Wenchuan earthquake in 2008 and Lushan earthquake in 2013 induced a large number of secondary disasters, such as collapses and landslides. Landslides caused by the Wenchuan earthquake were distributed along the seismic fault and nearly 80% occurred in the near-fault region (Hu et al., 2014; Zhang et al., 2019). This suggests that seismic-induced slope failure should be strongly affected by certain features of near-fault waves (Zhu et al., 2022). Earthquake-induced landslides and debris flows have raised extensive concern as they have been not only responsible for repeated blockage of national highways and rivers but also responsible for loss of life and property, and also cause damage to the environment every year in the mountainous regions (Chen et al., 2011, 2021). Some theoretical and empirical models have been proposed to evaluate the stability of seismic slopes in a near-fault regions (Zhang et al., 2019), previous studies conducted experiments to investigate the effect of seismic activities on dynamic response of slope (Zhu et al., 2022). With the aim of reducing the impact of debris flow disasters, some early warning methods based on history statistical data and physical properties of the materials have been put into practice (Akcali and Arman, 2013; Papa et al., 2013; Liu et al., 2016).
The main earthquake areas were in the western mountainous areas of Sichuan, associated with high mountains, deep valleys, complex geological structures, and developed faults. These mountainous disaster-prone areas experience collapses, landslides and debris flows. An earthquake activates a large number of potential debris flow gullies, causing significant slope instability and rock mass destruction, so that these debris flow gullies will be in an active period for a long time in the future; the scale and frequency of debris flow outbreaks will increase significantly. The active period of debris flows will last for 20 ~ 30 years, particularly 5 ~ 10 years after the earthquake (Cui et al., 2010; Shen et al., 2019; Giordan et al., 2020; Liu et al., 2020).
Scholars, at home and abroad, have conducted a lot of research and exploration on the formation mechanism of debris flows. Du et al. (2022) analyzed the change of formation conditions of debris flows after the Wenchuan earthquake. Ni Huayong (Hu et al., 2019) studied the formation mechanism of gully bed erosion-dominated debris flows through investigations, mapping and remote sensing interpretation. Zhou Jian (Jianping et al., 2012) carried out centrifuge model testing, using a self-developed visual test device and referring to a debris flow field test, to study the formation mechanism of typical landslide debris flows. Kou (Li et al., 2022) studied the occurrence mechanism of mountain stream debris flows and Chen (Ningsheng et al., 2004, 2009) studied the formation mechanism of debris flows in arid seismically active areas.
On July 29, 2019, a medium-scale debris flow occurred in Legugou, Ergu Village, Qianjin Township, Ganluo County, Sichuan Province; it washed away the village cooperatives and the roads leading to the township, blocking the Tianba River at the outlet of the gully. Lianghong Power Station is located at the outlet of the watershed and suffered serious damage, with a direct economic loss of about 40 million RMB, seriously affecting the lives of local people. At present, there are abundant loose solid materials that may participate in debris flow activities. Once heavy rainfall occurs, it is more likely that large-scale debris flows will happen again, and this poses a direct and serious threat to the life, property and safety of the villagers in the Ergu Village, Qianjin Township, Goukou, and along the Tianba River.
The main objective of this work is to find the causes of the debris flow and analyze its development trend. This study uses gray correlation method to discuss the possibility of debris flow eruption in earthquake-affected areas and determine the risk level. On this basis, the frequency method is introduced to calculate the critical rainfall of debris flow initiation in the study area, and the critical rainfall range is determined. Combined with the field investigations and meteorological data, the possibility and possible damage degree of the debris flow in the study area can be revealed, which can provide useful information for future disaster prevention and mitigation for such type of debris flow.
Study site
Ganluo County is located in the southwest platform of Sichuan Province, to the north of Daliang Mountain and on the eastern foot of Xiaoxiangling Mountain. It is a high mountain valley area from the western edge of Sichuan basin to the Qinghai Tibet Plateau. The landform of the county is mainly medium sized mountains, accounting for 90.8% of the county area, while the river valley accounts for only about 2.2% of the total area. The land sloping at an angle greater than 25°is 1,357.41km2, accounting for 63.02% of the county area (Figure 1). Due to the strong crustal movement, the folds and faults in the county are very developed, forming large anticlines and synclines. Due to the broken terrain and small vegetation coverage, most of the faults are steep high-angle thrust faults, which are prone to landslips, landslides and debris flows. The geology comprises Paleozoic strata in the north and Mesozoic and ancient carbonate strata in the south. Legugou is located in the middle of Ganluo County and is an area which has suffered strong structural erosion of the central mountains. The overall terrain is low in the south and high in the north, with deep valleys and large topographic fluctuations. In the valley, the mountains are high and steep, with an average slope of more than 35 degrees (Figure 2), and the longitudinal gradient of the gully is larger, especially the branches in the upper stream of the main gully, whose longitudinal gradient is more than 350‰. The longitudinal slope increases from the downstream to the upstream and the width decreases. According to the geomorphological features of the basin, it is divided into two geomorphological units, the middle and upper reaches of the gully are developed, the bedrock is buried shallowly, the bank slope is steep, and the relative height difference between the gully and the ridge is large and mainly characterized by high mountain landform. The erosion in the middle and lower reaches of the gully is strong and the gully, with a depth of 50 ~ 100 m, is formed by undercutting. The relative height difference between the gully and the ridge is small and mainly characterized by low mountain landform.
Legugou is located in Ganluo County, Sichuan Province (east longitude: 102°45′29.11″, north latitude: 29°00′06.79″) (Figure 3). The length of the main gully is about 4.5 km, the drainage area is 4.5km2, the average gradient of the main gully is 284.4‰, the elevation is about 2,320 m, and the elevation of the gully mouth is about 1,040 m (with a relative height difference of 1,280 m). There is little development in the inner branch gully and only one branch gully has developed to a large scale. The study area has been affected by the folds, uplifts and faults of the Hengduan Mountain range and the sharp cutting action of rivers, undulating mountains and ravines. The elevation difference between the river section and the mountains on both sides is wide; the valley wall is steep and the riverbed is narrow, which shows the typical topography of high mountains and valleys (Figure 4). Due to the strong crustal movement, folds and faults are very frequent, forming large anticlines and synclines, and deep valleys with mountains and valleys. The exposed geology is mainly strongly to moderately weathered sandstone, dolomite and limestone, which is conducive to the generation of debris flow sources and the convergence of water sources. There are abundant material sources in the gully area, most of which are distributed near the gully, or directly accumulated in the gully. The loose structure of the deposits make them easy to initiate movement by water flow; they provide rich material source conditions for the formation of debris flows. According to the formation conditions, the movement mechanism of debris flows and the distribution of loose solid matter, the Legugou watershed is divided into three parts: clear water area, formation and circulation area (material source area) and accumulation area (Figure 5).
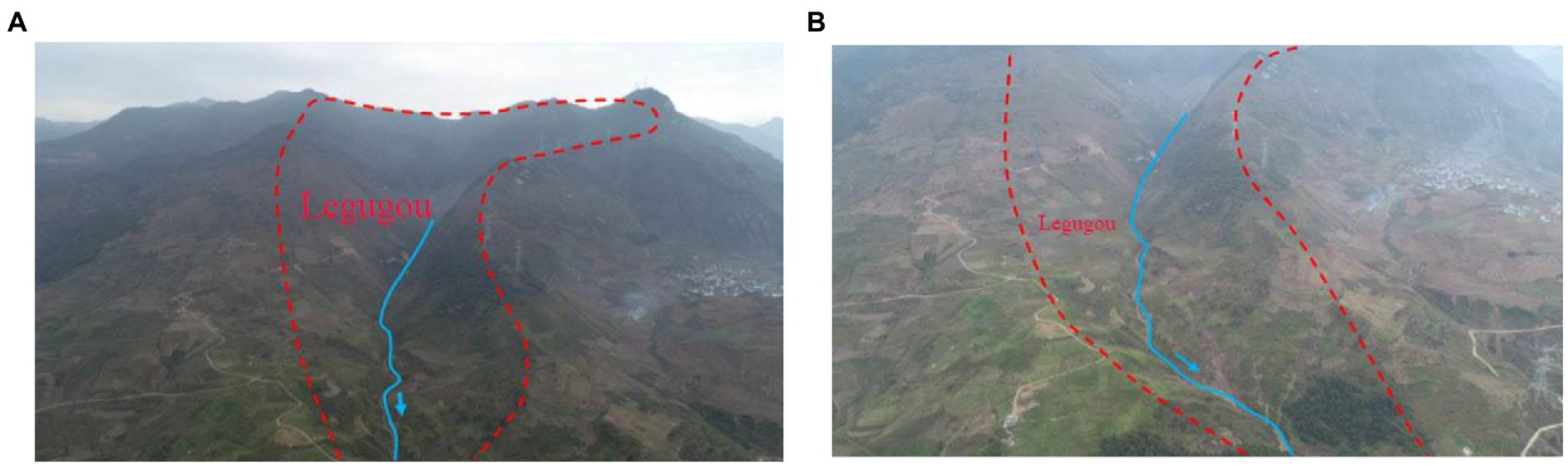
Figure 4. Geomorphological conditions of Legugou watershed. (A) Geomorphological conditions in the upper part of the watershed. (B) Geomorphological conditions in the middle part of the watershed.
The water source of the debris flow in Legugou mainly comes from atmospheric precipitation. The surface runoff from rainstorms is the main water source of debris flow as well as the main triggering factor. This area is located in the subtropical monsoon climate zone. It is humid and warm in summer, abundant in rainfall, dry and cold in winter and spring. However, because it is located at the eastern edge of the Hengduan Mountains (i.e., the transition zone between Western Sichuan Plateau and Sichuan Basin), the river valley is deep and the terrain is changeable, so that meteorological factors such as rainfall and temperature change can vary significantly, regionally and at different altitudes. The annual average precipitation in the high mountain area can reach 1,400 mm. The basin or gully lowland has less rainfall and the average annual rainfall is less than 1,000 mm. The highest 10-day precipitation recorded in the county occurred in late July, reaching 57.8 mm, and the lowest was recorded in early January, reaching 0.5 mm. The average annual precipitation is 980.78 mm, the maximum annual precipitation is 1179.9 mm (1994), and the minimum annual precipitation is 621.4 mm (2014). The precipitation is large and concentrated, and concentrated heavy rainfall often occurs in a short time. Rainfall is mainly concentrated from April to October, accounting for 93.14% of the annual rainfall. At the same time, this period is also a high incidence period of geological disasters, and the number of geological disasters in the months with less rainfall is small. It can be seen that the annual variation of rainfall in this area is large and the rainfall distribution is uneven within a year. The possibility of concentrated rainfall is high, which is an important factor that causes geological disasters, such as landslides, in this area.
Methodology
In order to identify the total amount of the loose solid materials in the watershed, determine the influence of various factors on debris flow initiation and the possibility of debris flow outbreak, and the critical rainfall for debris flow mobilization, historical data needs to be collected. However, field investigations are conducted after the debris flow event, and the dynamic characteristics of the debris flow are mainly based on the description of the local witnesses. Local residents do not have professional knowledge, and the described processes may be different from the real one, thus may lead to some inaccurate in the model calculations.
Graphic method of debris flow source materials in seismic area
Dynamic source materials of Erosional Debris Flow (Jianping et al., 2012): It is assumed that the V-shaped gully bed accumulation body will gradually form gullies from point C to point O, under the action of flood undercutting, and the right hand triangle shows the largest possible provenance of dynamic reserves for debris flow (Figure 6). The area of this region is:
where, θ is the natural angle of repose of the slope, and co = h is the original trench bed depth.
The dynamic reserve volume is:
where, V01 is the undercut erosion type dynamic reserve, and L1 is the length of gully bed accumulation.
Dynamic source materials of Lateral Erosional Debris Flow: It is assumed that U-shaped valley slope deposits will gradually collapse from point c2 to point d and form a debris flow source under the action of flood erosion and flood side erosion; any triangle is the largest possible source area of debris flow dynamic reserves. The area is:
where, θ is the natural angle of repose of the slope, α is the foot of the actually measured accumulation slope, ϕ is the angle between the integration and the natural angle of repose, and c2o = l is the measured slope length.
The volume of dynamic reserves is:
where, V02 is the lateral erosion type dynamic reserve and L2 is the length of the channel stack.
Gray correlation method (Binbin et al., 2013)
Eight factors are selected to evaluate risk:, comprising: debris flow scale X1/(103 m3), watershed area X2/(km)2, main gully length X3/(km), watershed relative height difference X4/(km), watershed cutting density X5/(km−1), channel gradient X6/(%), average annual rainfall X7/(mm) and earthquake intensity X8/(°). When the gray correlation method is adopted to establish the evaluation model, due to the incomparability of the influencing factors, the weights of each factor are quantitatively analyzed and normalized, and all of them are converted into values between 0 and 1 (Table 1), so as to prevent the leaping change of the converted values at the combination points of the evaluation factors.
Data averaging and dimensionless processing:
where, k = 1, 2,…, m, and m is the number of response factors; i = 0, 1,…, n, and n is the number of debris flow gullies.
Absolute difference sequence:
where, .
Correlation coefficient:
where, ρ is the resolution coefficient, with a value range of [0,1], generally 0.5.
Correlation degree:
Substitute the obtained correlation coefficients into Eq. (8) to find the correlation degree between the comparison sequence Xj and the reference sequence Xi.
Factor weight:
Evaluation function:
The weight of each factor is substituted into Eq. (10) to obtain the evaluation function of debris flow risk in an earthquake area:
Frequency method
The regional critical rainfall range of 1 h and 10 min of Legugou debris flow is calculated by using the critical rainfall (Lingkan, 1988), derived from the frequency of the debris flows and rainstorms. The frequency calculation formula is as follows:
where, is the critical rainfall index of debris flow, and X1 is the frequency of debris flow; the values are shown in Table 2. X2 is the rainstorm frequency with annual daily rainfall greater than (regional rainstorm frequency).
The formulas for calculating critical rainfall of 1 h and 10 min are shown in Table 3.
Among them: RE is the total effective rainfall, I10min is the rain intensity at 10 min, I1h is for 1 h rain intensity, is the mean value of the annual maximum 10 min rainstorm, is the annual maximum rainstorm average, and and are from the Handbook of Rainstorms and Floods in Small and Medium Watershed of Sichuan Province (Sichuan Provincial Department of Water Resources and Electric Power, 1984).
Results
The Legugou debris flow basin has a large area, rich sources of loose solids, and adverse geological phenomena such as more developed collapses and landslides. Through in-situ investigations, loose solid materials are widely distributed in the basin; the middle and lower reaches of the basin belong to debris flow source areas. There are many concentrated source areas in the gully area. In the survey, 18 relatively concentrated material sources were found, with landslide deposits and channel deposits as the main material sources, followed by slope erosion deposits, collapse deposits, and mineral waste residue (Figure 7).
The lithology of the exposed rock strata in Ganluo County is complex and some of them are weak and easily weathered Figure 8. Some of the rock is hard but tectonic activity easily produces fractures at stress concentration points, Figure 9. In a fault zone and its immediate vicinity, the rock mass is severely damaged. Interbedded soft and hard rock layers have also formed with strong differential weathering, and this produces rolling stones and collapses (Li et al., 2022; Figure 10). Therefore, the local formation lithology and geological structure are conducive to the production of a large number of loose solid materials. The calculation results of total source and dynamic reserves are shown in Table 4.
According to the emergency identification method and the index of secondary debris flow gullies of the Wenchuan 5.12 earthquake, put forward by Ningsheng et al. (2009), the number of loose solid materials in 0.1 m3/m2 is taken as the identification index of debris flow gully, and the number of loose solid materials in 2 m3/m2 is taken as the identification index of viscous debris flow, so as to quickly identify debris flow gullies. The quantity of loose solids in the Legugou watershed is:. Therefore, it can be judged that the Lagougou debris flow is belongs to diluted debris flow.
Influence of seismic activities on debris flow initiation
Previous studies have shown that almost all mountain disasters around the world (such as debris flows, landslides, and collapses) are distributed in the tectonic and seismically active belts formed by the rising mountains at the edges of the main plates. Therefore, to some extent, the seismic or tectonic active zone is also the active zone of debris flow (Muco et al., 2012). Wenchuan earthquake in 2008 caused a large number of various geological disasters (Huang and Li, 2009), among them the collapses and landslides produced a large number of loose solid materials. After the earthquake, debris flow became the main hazard (Fan et al., 2017). For example, the Chi-Chi earthquake in Taiwan in 1999 caused many landslides, which provided a large number of loose solid materials, resulting in frequent debris flow activities over the next decade (Chen and Hawkins, 2009). At least five disaster events occurred in Wenjiagou, Qingping Town, Mianzhu County, Sichuan Province from September 24, 2008 to September 18, 2010, which is a typical post-earthquake debris flow gully (Ni et al., 2011, 2012). On September 24, 2008, 72 mudslides were triggered by heavy rainfall in the middle of the Wenchuan earthquake-stricken area. Compared to the period before the earthquake, the critical cumulative precipitation and hourly rainfall intensity required to trigger mudslides after the earthquake significantly reduced (Tang et al., 2009). On March 25, 2007, the earthquake in Noto Peninsula (Ishikawa Prefecture) in Japan caused a long-distance debris flow in gullies (Okada et al., 2008). It can be seen that the occurrence of debris flow is closely related to earthquakes.
Ganluo County is located in the Anning River-Zemuhe seismic belt, with frequent earthquakes, and the epicenters are mainly distributed in the Moxi and Yalong River fault zones. In the 648 years from 1,327 to 1975, there were 147 earthquakes with a magnitude above 2.5, including 15 earthquakes with a magnitude above 5; the highest magnitude was 7.5. Generally speaking, Earthquakes of magnitude 6 and above are more likely to cause collapses and landslides. The higher the intensity, the more loose solid materials will be produced and the larger the scope of triggering debris flows will be (Cauzzi et al., 2018). In recent years, the Wenchuan earthquake (May 12, 2008), the Lushan earthquake (April 20, 2013), the Jiuzhaigou earthquake (July 7, 2017), and the Changning earthquake (June 6, 2019) all had a great impact on Ganluo County, especially the Lushan earthquake. The cohesive and effective cohesive force of soils gradually decrease with an increase in earthquake magnitude and the effective cohesive force of soil with higher saturation (saturation above 92%) can be reduced to 0 under strong vibrations (Mielenz, 1952). Frequent seismic activities destroy the internal structure of the soil, reduce its shear strength, cause collapse and landslides, and provide loose solid materials for the formation of debris flow. Moreover, seismic activity intensifies gully erosion, providing an effective surface for the accumulation of landslides on both sides of the river, which is conducive to the formation of debris flow. The Ganluo area has frequent geological tectonic movements, and strong crustal movements develop local folds and faults. Strong tectonic action has damaged the rock mass and the earthquake has further loosened and broken some rock masses, further damaging the stability of the rock and soil mass. This results in further deterioration of the geological conditions, which are prone to landslides and debris flows. An earthquake strengthens the intensity of heavy rainfall through a series of feedback actions (Ning-sheng et al., 2014). Through the statistics of rainfall data in Ganluo County, the frequency and scale of rainfall in the county after the earthquake are higher than those before the earthquake.
According to the basic data from the Legugou Basin (the seismic intensity is 6 degrees according to the 4.20 Lushan seismic intensity map), the factors are converted by using the conversion function in Table 5 to obtain the calculated data (Table 6).
According to Eq. (11), it is calculated that the risk degree of debris flow in Legugou watershed is H = 0.725. According to the classification standard of debris flow risk in earthquake areas, the debris flow in Legugou is moderately dangerous after the earthquake, and it may happen again.
Influence of human activities on debris flow initiation
Ganluo County is located in the mountainous area outside the Sichuan Basin, with high mountains and steep slopes, wide lands and sparsely populated areas. Normally, it should be well covered by deep forests. In fact, the county often suffers from serious natural events, such as hail and windstorms. According to the 25 years of recorded observation data for the county, there have been 42 strong hailstorms in that time. Strong winds above Grade 8 occur for an average of 65 days every year, reaching 128 days in most years, and the maximum wind force reaches Grade 12. In addition, forests, farmland and villages are intertwined, human activity is frequent, the illegal felling of trees and deforestation occur as well as land reclamation from time to time; the existing forest coverage rate is only 29.28%(Xiang, 1988). The area with slope greater than 30° accounted for 90.9% of Legugou watershed (Figure 2). The land use type map of the Legugou watershed was obtained through remote sensing interpretation (Figure 11). It can be seen from the map that the clear water area has good vegetation coverage and the probability of forming vegetation in the circulation area is less than 30%, while the vegetation coverage in the accumulation area is almost zero; the vegetation coverage rate is decreasing year by year. At present, human engineering activities in the Legugou basin include farming, urban construction, quarrying, road building and hydropower station construction. The steep slope reclamation and sloping farmland directly affect the mountain landform, which not only provides a solid material source for the occurrence of debris flow, but also intensifies water erosion. Excessive deforestation and water and soil loss, in some areas, accelerate the evolution of sloping land: the gullies are cut down and the slope stability is reduced. Under heavy rainfall conditions, it is easy to lose stability and directly enter the gully bed. In addition, Ganluo County is rich in minerals, according to the historical records of debris flow events, the wastes of road construction and mining are the components of solid sources. After on-site investigation, there is still a mineral waste residue source in the Legugou River Basin (Table 4). The unreasonable stacking of a large amount of earth and rock during mining, quarrying and road construction still provides a solid source for the formation of this debris flow.
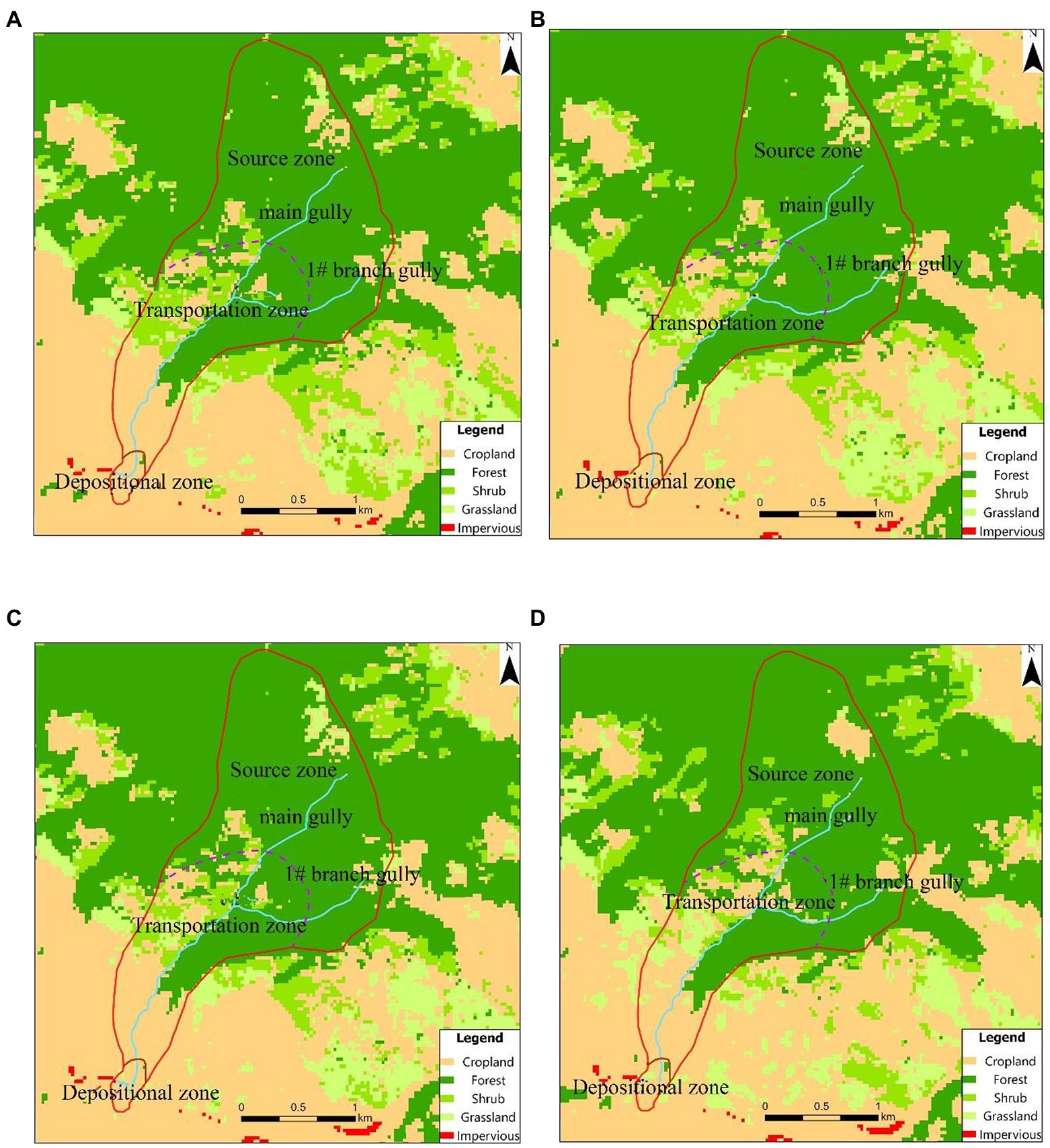
Figure 11. Land use type of Legugou Watershed in different years. (A) Image of year 2007. (B) Image of year 2009. (C) Image of year 2012. (D) Image of year 2019.
Influence of heavy rainfall
It can be seen from the correlation diagram between the average rainfall and the number of geological disasters in Ganluo County, Figure 12. The main hydrodynamic force of the debris flow is induced by rainfall in Legugou watershed. Rainfall processes are usually divided into two categories. The first concerns the fact that, on the basis of accumulated rainfall in the previous period, a certain rainfall process can reach the critical hydrodynamic condition to trigger loose solid materials moving in the basin, thus inducing debris flow. The second is that there is no effective accumulated rainfall before the occurrence of debris flow, or its contribution to the formation of debris flow is small, which mainly meets the critical hydrodynamic conditions of loose solid materials in the basin through short-term heavy rainfall processes, and induces debris flow in a short time (Chae et al., 2017). It is the latter rainfall process of debris flow which occurs in Legugou. Ganluo County has a subtropical climate with abundant rainfall, which is characterized by frequent local rainstorms and concentrated rainfall. According to the hourly and cumulative rainfall of Qianjin Township Station (from 21:00 to 15:00 on July 28, 2019 (Figure 13; Liu and He, 2020), the rainfall reached 37 mm/h at 21:00 on July 28 and the maximum rainfall intensity reached 45.5 mm/h between 10:00 and 11:00 on July 29, 2019; the cumulative rainfall reached 144 mm.
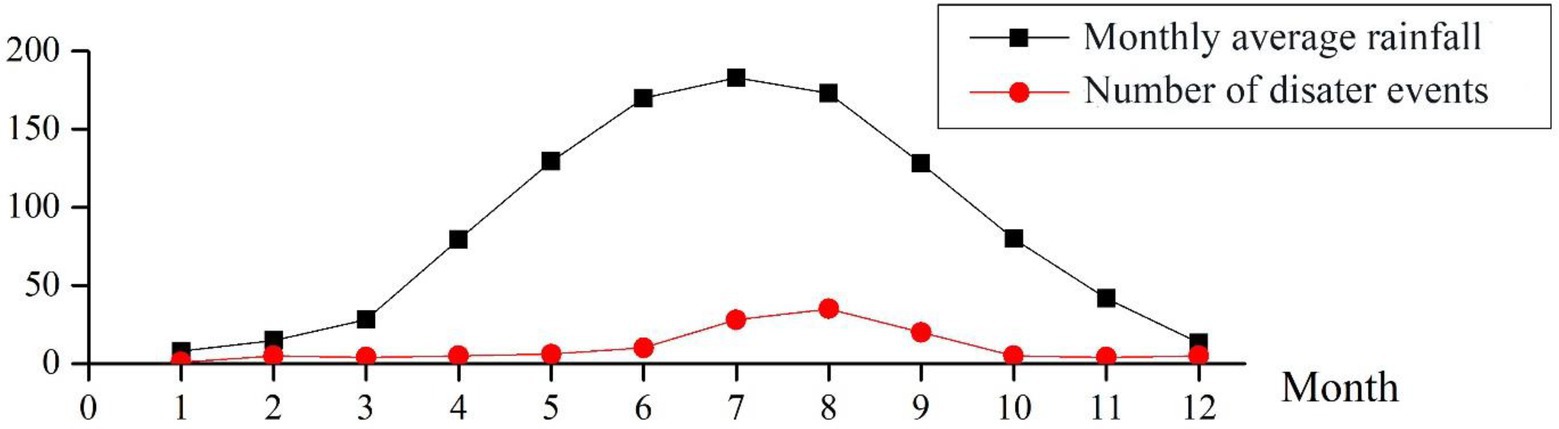
Figure 12. Correlation diagram between the average rainfall and the number of geological disasters in Ganluo County.
and is from the Handbook of Rainstorms and Floods in Small and Medium Watershed of Sichuan Province (Sichuan Provincial Department of Water Resources and Electric Power, 1984). By consulting relevant data, it is determined that the history of debris flow activity ranges from once every few years to once or twice a year, with a X1 value of 4. The probability of average annual rainfall of 50 mm or more in Ganluo County is 58% and the X2 value is 0.58; in addition, referring to the Handbook of Rainstorms and Floods in Small and Medium Watershed of Sichuan Province yields , .
The calculation results, according to the frequency method, are shown in Tables 7, 8.
Take points (25, 17.45) and (50, 8.17), as well as points (25, 22.21) and (50, 13.01), as the upper limit straight line and lower limit straight line intersecting the coordinate axis, and draw the 1H critical rainfall map (Figure 14A). Take points (25, 6.23) and (50, 2.92), as well as points (25, 8.44) and (50, 5.13) as the upper limit straight line and the lower limit straight line intersecting with the coordinate axis, and draw the 10 min critical rainfall map (Figure 14B). The four extreme points of critical rainfall in Figure 14A constitute the 1 h critical rainfall range and the four extreme points of critical rainfall in Figure 14B constitute the 10 min critical rainfall range. Figure 14 shows that the range of critical rainfall value for 1 h is 17.45 ~ 22.21 mm (Re = 25 mm) and 8.17 ~ 13.01 mm (R e= 50 mm); the 10 min critical rainfall values range from 6.23 to 8.44 mm (Re = 25 mm) and 2.92 to 5.13 mm (Re = 50 mm).
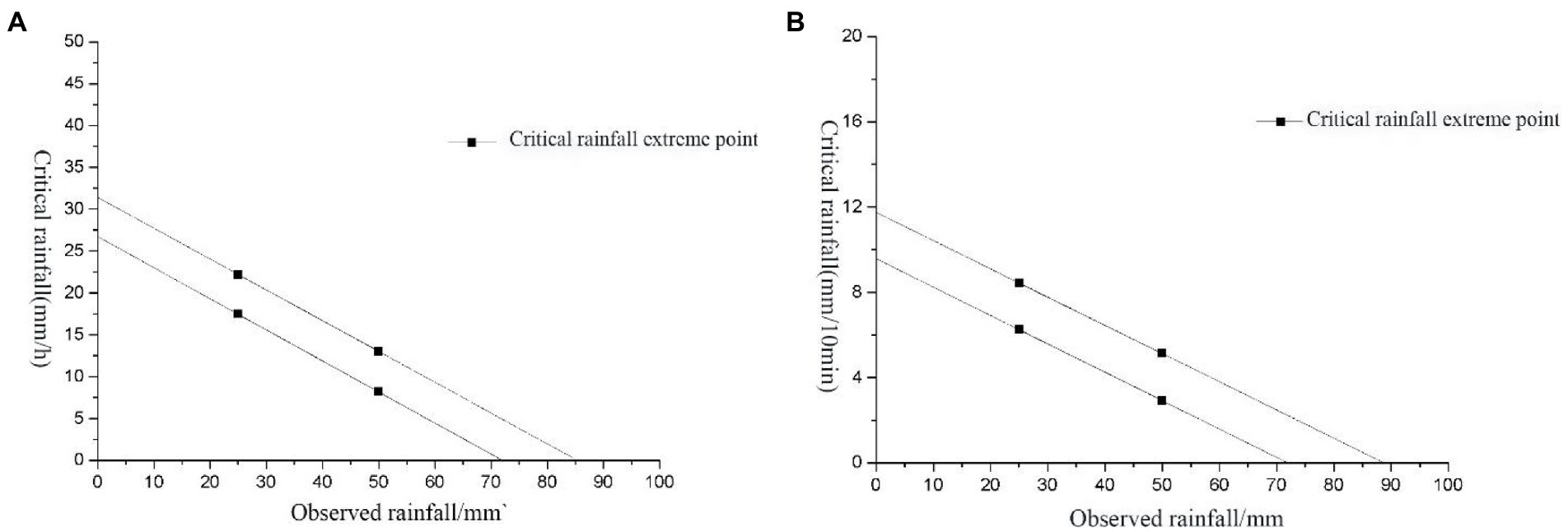
Figure 14. Critical rainfall of debris flow initiation in Legougou watershed. (A) 1 h critical rainfall. (B) 10 min critical rainfall.
According to the contour map of rainstorm volume attached to the Handbook of Rainstorm and Flood in Small and Medium Watershed of Sichuan Province, the average value of the maximum rainstorm in 10 min and 1 h in Qianjin Township, Ganluo County is 12.0 mm and 30.0 mm, respectively. On July 29, 2019, between 10:00 am and 11:00 am, the maximum rain intensity reached 45.5 mm/h and the accumulated rainfall reached 144 mm, far exceeding the critical rainfall of Leguogou, which caused the debris flow. Heavy rainfall was the main factor that induced this debris flow.
Comprehensive analysis
The Legugou watershed has a large relative height difference, steep terrain and a large longitudinal slope, which is conducive to the collection of debris flow materials. The gully area is large, with abundant rainfall and abundant water resources. Besides this, there are abundant loose solid materials for debris flow in the gully, which also provides conditions for the development of debris flow. Under the influence of long-term seismic activities, the soil in the basin is damaged to varying degrees, and its stability is reduced, which leads to collapses and landslides. A large number of loose material sources are piled up in the channel, which provides a large number of material sources for the formation of debris flow. Through the gray correlation method, it is calculated that Legugou is moderately dangerous due to the influence of previous seismic activities. Natural disasters, unscientific reclamation of mountain slopes and excessive felling of forest vegetation have caused soil erosion; the forest coverage rate is only 29.28%, which aggravates soil erosion. If continuous and concentrated heavy rainfall occurs, it is more likely to induce debris flow disasters. From June to July, 2019, the cumulative rainfall in Ganluo County reached 373 mm. On July 28th and 29th, the basin suffered from concentrated heavy rainfall. On the 29th, the cumulative rainfall reached 144 mm and the rainfall intensity reached the 20-year rainfall level. According to the calculation, the 1 h critical rainfall of Legugou watershed is 17.45 ~ 22.21 mm (Re = 25 mm) and 8.17 ~ 13.01 mm (Re = 50 mm), and the maximum rainfall intensity is 45.5 mm/h between 10:00 am and 11:00 am on the 29th, far exceeding the critical rainfall. Under the action of antecedent rainfall, rainwater infiltration leads to the increase of pore water pressure in the soil and the decrease of shear capacity. The runoff, forming after the confluence of surface water, has a strong undercutting erosion effect on the loose deposits in the gully bed, forming a deep gully. On the 29th, under the action of heavy rainfall, the water scour the gully, causing the stability of the gully bank landslide to decrease and then slide into the channel, resulting in the blockage of the channel. After the dam body burst, it played an enlarged role, and the original loose solid sources in the channel and the sliding loose landslide sources were entrained away by the water flow to form a debris flow.
In summary, the Legugou watershed itself has conditions for the development of debris flow. Under the influence of earthquakes and human activities, it is stimulated by concentrated heavy rainfall, which is the main factor inducing this debris flow.
Discussion
On July 29, 2019, the debris flow in Legugou was formed under the joint actions of an earthquake, human activities and heavy rainfall (the latter being the main factor inducing this debris flow). Through analysis, it can be determined that this debris flow belongs to a rainstorm gully type, in which the downstream slope has poor stability, and adverse geological phenomena, such as collapse and landslides often occur, which is the main material source of the debris flow. The total amount of loose solid materials in the basin is 57.2 × 104 m3 and the dynamic reserves that can participate in debris flow activities are 19.8 × 104 m3 which provides sufficient material source conditions for the recurrence of debris flow disasters. The terrain in the basin is steep, the longitudinal slope of the valley is large, the rainfall distribution is uneven, and the concentrated rainfall intensity is high. Under the influence of many factors, debris flow disasters will still occur.
From the perspective of topography, the relative height difference in the Legugou basin is large, the terrain is steep, and the longitudinal gradient of the valley is large, which is conducive to the collection and disaster of debris flow materials (Yu et al., 2020). The debris flow in the gully is rich in solid material sources. Under the influence of heavy rainfall, landslides, and collapses occur frequently in the gully area, and the amount of material sources in the watershed increases dramatically. Especially after the May 12 Earthquake and Lushan 4.20 Earthquake, the geological environment in the gully area is affected to varying degrees by the earthquake and geological structure, and the mountain is severely shaken, the stability of soil is reduced, and the phenomena of landslides and collapses increases. Recent years have been affected by extreme weather and frequent landslides and collapses have occurred in the gully area, a large number of loose solid sources have been added in the gully, and flash floods (at different scales) occur during every rainstorm.
There is abundant rainfall in the basin and concentrated heavy rainfall often occurs, which provides hydrodynamic force for the formation of debris flows. Earthquakes and unreasonable human activities destroy the soil stability in the basin, and the critical rainfall for debris flow initiation decreased significantly. The landslide sources in the middle and lower reaches of the gully are developed. Debris flows and mountain torrents scour the bank slope, forming a steep surface on both sides of the gully, which makes the landslide stability on both sides of the bank slope low and increases the possibility of landslides (Figure 15). Therefore, under heavy rainfall conditions, the scale and frequency of debris flow in Legugou will increase, from the original low-frequency debris flow to the intermediate-frequency debris flow.
Real-time monitoring and early warning have great significance in reducing the consequences caused by geological disasters (Chen et al., 2021). Most importantly, warning systems can avoid the large numbers of causalities and permits the timely evacuation of the community. Consequently, prediction and mitigation of landslide geo-hazards have attracted a lot of attention and remain hot issues within the fields of geotechnical engineering and engineering geology. A reliable early warning system, comprising monitoring indicators, data processing, communication, and dissemination of warnings and community evacuation, is a cost-effective means to alleviate or even avoid economic and fatal consequences by landslides (Intrieri et al., 2012). The early warning indicators (EWIs) aim at characterizing the evolution stages and are keys to determining the release of warnings to the public. Physical and statistical models have been developed to find the correlation between their occurrence and rainfall, and different triggering criteria, i.e., thresholds, for shallow landslides and debris flows have been proposed (Hu et al., 2010). However, even for a certain basin, the rainfall threshold is not constant. For instance, a rainfall far below the threshold can trigger debris flows when material supplies or slope failures are extensive, as in the case of valleys suffering earthquakes (Cui et al., 2011) Therefore, it is hard to define the rainfall threshold both literally and physically. The establishment of a monitoring and early warning system based on the underlying conditions and critical rainfall in the study area can effectively improve the forecast accuracy and better meet the needs of disaster prevention and mitigation.
Based on the field investigations and theoretical calculations, the total amount of loose solid materials in the study area was determined, and the hazard evaluation model of the debris flow in earthquake area was established by means of the gray correlation method. The hazard index of the debris flow in the earthquake area was identified and the level of danger can be determined. Subsequently, the critical rainfall of debris flow initiation is calculated with the help of frequency method, and the possibility of debris flow initiation is assessed synthetically combined with the rainfall conditions in this area. The results in this paper can provides data support for disaster prevention and mitigation, and also provide basic indicators for the establishment of monitoring and early warning systems.
Conclusion and advice
On 29 July 2019, a medium-scale debris flow occurred in Legu Village, Qianjin Township, Ganluo County. The debris flow destroyed 3 houses, washed away the villagers’ cooperative, roads and water supply lines. It blocked Tianba River located in the outlet of the watershed and caused a direct economic loss of 40 million RMB. At present, the loose solid materials that can participate in debris flow activities in the watershed are still large, and the possibility of debris flow initiation under the influence of heavy rainfall is very high.
Through analysis, it can be determined that the debris flow in Legugou belongs to rainstorm gully type, in which the downstream slope is poor in stability, and some unfavorable geological phenomena, such as collapse and landslides often occur. This was the main material source for the debris flow. The analysis revealed that there are favorable conditions for the development of debris flow in the study area. In terms of topography and geomorphology, the relative height difference and the longitudinal slope of gullies is large, and the terrain is steep, which is conducive to the confluence of loose solid materials. In terms of source materials, the loose solid materials in the watershed is abundant, and the total amount of solid materials is 57.2 × 104 m3, and the dynamic reserves that can participate in debris flow activity is 19.8 × 104 m3. From the point of view of water source conditions, the area of Legu watershed is larger, with abundant rainfall. In particular, after the 5.12 earthquake and the 4.20 Lushan earthquake, a large number of loose solid materials were sharply increased, while the critical rainfall for debris flow initiation was significantly decreased. Therefore, it is likely to trigger a large-scale debris flow by heavy rainfall in the future.
The debris flow in Legu gully watershed is mainly triggered by heavy rain. The susceptibility of debris flow in the study area is evaluated by gray correlation method, through calculation the susceptibility can be identified, and the corresponding conclusion is moderate.
Affected by earthquakes and geological structures, loose solid sources in the gully increased, rock mass was destroyed, soil stability decreased, and the critical rainfall for debris flow initiation decreased significantly. Under rainstorm conditions, the scale and frequency of debris flow will increase, and the original low-frequency debris flows will change into medium-frequency debris flows in the future. Therefore, it is necessary and urgent to implement the prevention and control project. On the basis of comprehensive analysis of the formation conditions, dynamic characteristics and development trend of the debris flow in Legu watershed, taking the complexity of construction conditions into consideration, the main engineering measures of “hold back ground runoff, and improve the stability of loose solid materials in the source zone” needed to be adopted to protect the safety of residential areas and facilities. This research can not only provide a reference for the analysis of the formation mechanism of debris flow after earthquake, but also provide an important basis for disaster prevention and mitigation of similar debris flow events.
Data availability statement
The original contributions presented in the study are included in the article/supplementary material, further inquiries can be directed to the corresponding authors.
Author contributions
NH: writing, methodology, reviewing, and editing. QF: data processing and writing. WZ: original draft and data processing. ZY: reviewing and editing. XC: conceptualization and methodology. All authors contributed to the article and approved the submitted version.
Funding
This work was financially supported by the National Key Research and Development Program of China (Grant No. 2020YFD1100701), and Hubei Key Laboratory of Disaster Prevention and Mitigation (China Three Gorges University) (2021KJZ04).
Acknowledgments
The authors would like to thank the financial supports from the National Key Research and Development Program of China (Grant No. 2020YFD1100701), and Hubei Key Laboratory of Disaster Prevention and Mitigation (China Three Gorges University) (2021KJZ04).
Conflict of interest
The XC was employed by Sichuan Communication Surveying & Design Institute Co. Ltd.
The remaining authors declare that the research was conducted in the absence of any commercial or financial relationships that could be construed as a potential conflict of interest.
Publisher’s note
All claims expressed in this article are solely those of the authors and do not necessarily represent those of their affiliated organizations, or those of the publisher, the editors and the reviewers. Any product that may be evaluated in this article, or claim that may be made by its manufacturer, is not guaranteed or endorsed by the publisher.
References
Akcali, E., and Arman, H. (2013). Landslide early warning system suggestion based on landslide – rainfall threshold: Trabzon Province. Teknik Dergi 24, 6307–6332.
Binbin, W. E. I., Qihua, Z. H. A. O., Gang, H. A. N., and Haiquan, Z. H. A. N. G. (2013). Grey correlation method based hazard assessment of debris flow in quake-hit area—taking debris flows in Beichuan as an example. J. Eng. Geol. 21, 525–533. doi: 10.1004.9665/2013/21(4)-0525-09
Cauzzi, C., Fah, D., Wald, D. J., Clinton, J., Losey, S., and Wiemer, S. (2018). ShakeMap-based prediction of earthquake-induced mass movements in Switzerland calibrated on historical observations. Nat. Hazards 92, 1211–1235. doi: 10.1007/s11069-018-3248-5
Chae, B. G., Park, H. J., Catani, F., Simoni, A., and Berti, M. (2017). Landslide prediction, monitoring and early warning: a concise review of state-of-the-art. Geosci. J. 21, 1033–1070. doi: 10.1007/s12303-017-0034-4
Chen, N.-S., Hu, G.-S., Deng, M.-F., Zhou, W., YANG, C.-L., Han, D., et al. (2011). Impact of earthquake on debris flows—a CASE study on the WENCHUAN earthquake. J. Earthq. Tsun. 05, 493–508. doi: 10.1142/S1793431111001212
Chen, H., and Hawkins, A. (2009). Relationship between earthquake disturbance, tropical rainstorms and debris movement: an overview from Taiwan. Bull. Eng. Geol. Environ. 68, 161–186. doi: 10.1007/s10064-009-0209-y
Chen, H., Li, G., Fang, R., and Zheng, M. (2021). Early warning indicators of landslides based on deep displacements: applications on Jinping landslide and Wendong landslide, China. Front. Earth Sci. 9:747379. doi: 10.3389/feart.2021.747379
Chen, H., and Su, D.-Y. (2001). Geological factors for hazardous debris flows in Hoser, Central Taiwan. Environ. Geol. 40, 1114–1124. doi: 10.1007/s002540100312
Cui, P., Chen, X. Q., Zhu, Y. Y., Su, F. H., Wei, F. Q., Han, Y. S., et al. (2011). The Wenchuan earthquake (May 12, 2008), Sichuan Province, China, and resulting geohazards. Nat. Haz. 56, 19–36. doi: 10.1007/s11069-009-9392-1
Cui, P., Jianqi, Z. H. U. A. N. G., Xingchang, C. H. E. N., Jianqiang, Z. H. A. N. G., and Xiaojun, Z. H. O. U. (2010). Characteristics and countermeasures of debris flow in Wenchuan area after the earthquake. J. Sichuan Univ. 5, 10–19. doi: 10.15961/j.jsuese.2010.05.004
Du, J. T., Choi, C. E., Yu, J. T., and Thakur, V. (2022). Mechanisms of submarine debris flow growth. J. Geophys. Res. Earth Surf. 127
Fan, X. M., Xu, Q., Scaringi, G., Dai, L. X., Li, W. L., Dong, X. J., et al. (2017). Failure mechanism and kinematics of the deadly June 24th, 2017 Xinmo landslide, Maoxian, Sichuan, China. Landslides 14, 2129–2146. doi: 10.1007/s10346-017-0907-7
Giordan, D., Adams, M. S., Aicardi, I., Alicandro, M., Allasia, P., Baldo, M., et al. (2020). The use of unmanned aerial vehicles (UAVs) for engineering geology applications. Bull. Eng. Geol. Environ. 79, 3437–3481. doi: 10.1007/s10064-020-01766-2
Hu, K., Cui, P., Wang, C., Li, Y., and Lu, X. (2010). Characteristic rainfall for warning of debris flows. J. Mt. Sci. 7, 207–214. doi: 10.1007/s11629-010-2022-2
Hu, W., Xu, Q., Wang, G. H., Asch, T. W. J., and Hicher, P. Y. (2014). Sensitivity of the initiation of debris flow to initial soil moisture. Landslides 12, 1139–1145. doi: 10.1007/s10346-014-0529-2
Hu, G. S., Zhao, C. Y., Chen, N. S., Chen, K. T., and Wang, T. (2019). Characteristics, mechanisms and prevention modes of debris flows in an arid seismically active region along the Sichuan-Tibet railway route, China: a case study of the Basu-Ranwu section, southeastern Tibet. Environ. Earth Sci. 78, 1–18. doi: 10.1007/s12665-019-8554-z
Huang, R., and Li, W. (2009). Analysis of the geo-hazards triggered by the 12 may 2008 Wenchuan earthquake, China. Bull. Eng. Geol. Environ. 68, 363–371. doi: 10.1007/s10064-009-0207-0
Intrieri, E., Gigli, G., Mugnai, F., Fanti, R., and Casagli, N. (2012). Design and implementation of a landslide early warning system. Eng. Geol. 147, 124–136. doi: 10.1016/j.enggeo.2012.07.017
Jianping, Q. I. A. O., Dong, H. U. A. N. G., Zongji, Y. A. N. G., and Huajun, M. E. N. G. (2012). Statistical method on dynamic reserve of debris flow's source materials in meizoseismal area of Wenchuan earthquake region. Chin. J. Geol. Haz. Con. 2, 1–6. doi: 10.16031/j.cnki.issn.1003-8035.2012.02.011
Li, N. D., Liu, W., and Zhao, J. H. (2022). Behavioural analysis and dynamic simulation of the debris flow that occurred in Ganluo County (Sichuan, China) on 30 august 2020. J. Mt. Sci. 19, 1495–1508. doi: 10.1007/s11629-021-7199-z
Lingkan, Y. A. O. (1988). A research on the calculation of critical rainfall with frequency of debris flow and torrential rain. J. Soil Water Conserv. 4, 72–78.
Liu, B., Han, S. Y., Gong, H., Zhou, Z. L., and Zhang, D. (2020). Disaster resilience assessment based on the spatial and temporal aggregation effects of earthquake-induced hazards. Environ. Sci. Pollut. Res. 27, 29055–29067. doi: 10.1007/s11356-020-09281-3
Liu, W., and He, S. M. (2020). Comprehensive modelling of runoff-generated debris flow from formation to propagation in a catchment. Landslides 17, 1529–1544. doi: 10.1007/s10346-020-01383-w
Liu, D., Zhang, S., Yang, H., Zhao, L., Jiang, Y., Tang, D., et al. (2016). Application and analysis of debris-flow early warning system in Wenchuan earthquake-affected area. Nat. Hazards Earth Syst. Sci. 16, 483–496. doi: 10.5194/nhess-16-483-2016
Mielenz, R. C. (1952). Physical-chemical properties and engineering performance of clays. Clays Clay Min. 1, 196–254. doi: 10.1346/CCMN.1952.0010122
Muco, B., Alexiev, G., Aliaj, S., Elezi, Z., Grecu, B., Mandrescu, N., et al. (2012). Geohazards assessment and mapping of some Balkan countries. Nat. Haz. 64, 943–981. doi: 10.1007/s11069-012-0185-6
Ni, H. Y., Zheng, W. M., Tie, Y. B., Su, P. C., Tang, Y. Q., Xu, R. G., et al. (2011). Formation and characteristics of post-earthquake debris flow: a case study from Wenjia gully in Mianzhu, Sichuan, SW China. Nat. Haz. 61, 317–335. doi: 10.1007/s11069-011-9914-5
Ni, H., Zheng, W., Tie, Y., Su, P., Tang, Y., Xu, R., et al. (2012). Formation and characteristics of post-earthquake debris flow: a case study from Wenjia gully in Mianzhu, Sichuan, SW China. Nat. Haz. 61, 317–335. doi: 10.1007/s11069-011-9914-5
Ningsheng, C., Peng, C., Xiaoying, W., and Baofeng, D. (2004). Testing study on strength reduction of gravelly soil in triggering area of debris flow under earthquake. Chin. J. Rock Mech. Eng. 23, 2743–2747. doi: 10.1008/2786.2009.01.011
Ningsheng, C. H. E. N., Rong, H. U. A. N. G., Huan, L. I., and Wanyin, X. I. E. (2009). Emergency judge method and index of debris-flow sites triggered by 512Wenchuan earthquake. J. Mt. Sci. 27, 108–114.
Ning-sheng, C., Yang, L., Hai-bo, Z., Ming-feng, D., and Da-wei, H. (2014). Combined impacts of antecedent earthquakes and droughts on disastrous debris flows. J. Mount. Sci. 11, 1507–1520. doi: 10.1007/s11629-014-3080-7
Okada, Y., Ochiai, H., Kurokawa, U., Ogawa, Y., and Asano, S. (2008). A channelised long run-out debris slide triggered by the Noto Hanto earthquake in 2007, Japan. Landslides 5, 235–239. doi: 10.1007/s10346-007-0090-3
Papa, M., Medina, V., Ciervo, F., and Bateman, A. (2013). Derivation of critical rainfall thresholds for shallow landslides as a tool for debris flow earlywarning systems. Hydrol. Earth Syst. Sci. 17, 4095–4107. doi: 10.5194/hess17-4095-2013
Shen, T., Wang, Y. S., Luo, Y. H., Xin, C. C., Liu, Y., and He, J. X. (2019). Seismic response of cracking features in Jubao Mountain during the aftershocks of Jiuzhaigou Ms7.0 earthquake. J. Mt. Sci. 16, 2532–2547. doi: 10.1007/s11629-019-5570-0
Sichuan Provincial Department of Water Resources and Electric Power. (1984). Handbook of Rainstorm and Flood Calculation for Small and Medium Watershed in Sichuan Province. Sichuan Provincial Department of Water Resources and Electric Power, Chengdu.
Tang, Y. (2019). Contested narratives at the Hanwang earthquake Memorial Park: where ghost industrial town and seismic memorial meet. Geoheritage 11, 561–575. doi: 10.1007/s12371-018-0309-9
Tang, C., Zhu, J., Li, W., and Liang, J. (2009). Rainfall-triggered debris flows following the Wenchuan earthquake. Bull. Eng. Geol. Environ. 68, 187–194. doi: 10.1007/s10064-009-0201-6
Xiang, G. A. O. (1988). Types of debris flows and the preventive measures in Ganluo County. Bull. Soil Water Conserv. 4, 35–43.
Yu, L. I., Binrui, G. A. N., Xiekang, W. A. N. G., Mingliang, C. H. E. N., and Jiawen, Z. H. O. U. (2020). Formation on mechanism of group flash flood debris flow disasters in Ganluo County, Sichuan Province in 2019. Bull. Soil Water Conserv. 6, 281–287. doi: 10.13961/j.cnki.stbctb.2020.06.040
Zhang, Y. B., Xiang, C. L., Che, Y. L., Cheng, Q. G., Xiao, L., Yu, P. C., et al. (2019). Permanent displacement models of earthquake-induced landslides considering near-fault pulse-like ground motions. J. Mt. Sci. 16, 1244–1257. doi: 10.1007/2Fs11629-018-5067-2
Keywords: debris flows, formation mechanism, earthquakes, grey correlation method, frequency method, human activities, artificial intelligence
Citation: He N, Fu Q, Zhong W, Yang Z, Cai X and Xu L (2022) Analysis of the formation mechanism of debris flows after earthquakes – A case study of the Legugou debris flow. Front. Ecol. Evol. 10:1053687. doi: 10.3389/fevo.2022.1053687
Edited by:
Hamzeh Ghorbani, Islamic Azad University, IranReviewed by:
Sahar Lajmorak, Institute for Advanced Studies in Basic Sciences (IASBS), IranMeysam Rajabi, Birjand University of Technology, Iran
Copyright © 2022 He, Fu, Zhong, Yang, Cai and Xu. This is an open-access article distributed under the terms of the Creative Commons Attribution License (CC BY). The use, distribution or reproduction in other forums is permitted, provided the original author(s) and the copyright owner(s) are credited and that the original publication in this journal is cited, in accordance with accepted academic practice. No use, distribution or reproduction is permitted which does not comply with these terms.
*Correspondence: Wei Zhong, emhvbmd3ZWlAaW1kZS5hYy5jbg==; Zhiquan Yang, eXpxMTk4MzgxNkBrdXN0LmVkdS5jbg==