- 1College of Life Sciences, China West Normal University, Nanchong, Sichuan, China
- 2Liziping Giant Panda’s Ecology and Conservation Observation and Research Station of Sichuan Province, Nanchong, Sichuan, China
Closely related birds that occur sympatrically will inevitably differ in the acquisition of food resources, nest site selection, and breeding times. The successful coexistence of birds that breed in the same area can be determined by investigating how they divide limited resources and reduce interspecific competition. Our study area is located at the eastern foot of the Qinghai-Tibet Plateau, with a high altitude and low annual average temperature. In this study, the life history characteristics and reproductive ecological parameters of two closely related species, the Chestnut Thrush (Turdus rubrocanus) and the Kessler’s Thrush (Turdus kessleri), were compared in detail in April–July of 2020, 2021, and 2022 in the scrub along the Liqiu River in Xinduqiao Town, Tibetan Autonomous Prefecture of Garzê, Sichuan. The results showed no differences in nesting material, nest cup size, clutch size, feeding strategy of nestlings, brood food type, and reproductive effectiveness between the Chestnut Thrush and the Kessler’s Thrush. However, the Kessler’s Thrush had a higher nesting height, thicker nest wall, earlier breeding time, smaller eggs, fewer brooding frequent and a longer brooding time compared to the Chestnut Thrush. In addition, they invested more time in parental care, resulting in a faster growth and development rate. The results indicate that birds with different ranges and breeding in the same range show convergent and divergent life history characteristics in terms of reproductive biology. Chestnut Thrush with low reproductive altitude shows the characteristics of low-altitude bird reproduction in part of reproductive biology, while Kessler’s Thrush with high reproductive altitude shows the characteristics of high-altitude bird reproduction in part of reproductive biology.
Introduction
The characteristics of avian life history are underpinned by ecological and behavioral patterns that formed during a long-term natural selection process and are adapted to their living environment (Martin, 2004). The evolution of avian life history is currently one of the hot topics in ornithological research. Among these, breeding is the most important part of the life history of birds, and research on avian breeding mainly focuses on the response of avian breeding characteristics to the environment (including biotic and abiotic factors; Partridge and Harvey, 1988; Southwood, 1988). Avian breeding traits have both a genetic aspect and an evolutionary aspect under the influence of the environment. In high-altitude alpine and subalpine mountains, avian breeding is subject to a series of environmental stressors, such as short breeding seasons, unpredictable climatic conditions, and limited food resources (Hille and Cooper, 2015). Under the influence of natural selection, these species of birds show large differences in many aspects, for example, breeding season, hatching period, clutch size, nestling stage, nestling growth pattern, reproductive success rate, mating system, and parental care strategy (Walters, 2003). We know very little about how the life history characteristics of birds change on the altitude gradient, especially the lack of in-depth and systematic research on how bird reproduction adapts to the high altitude environment.
According to the study of similar species at different breeding altitudes, birds at high altitudes have different life history characteristics. Compared with those at low altitudes, birds at high altitudes have fewer clutch sizes, fewer annual breeding nests, larger male investment, and longer nesting periods, hatching periods, nestling periods, and care periods for young birds leaving the nest (Badyaev, 1997; Badyaev and Ghalambor, 2001). High-altitude breeding Black Redstart (Phoenicurus ochruros) lay small clutches of large eggs, and increased parental input to the chicks did not accelerate chick development but rather slowed it down compared to chicks of lower altitude relatives (Mu et al., 2008). White-bellied Redstarts (Hodgsonius phaenicuroides) inhabiting high-elevation environments have a shorter breeding period, smaller clutch size, and larger egg size than their low-elevation counterparts (Lu et al., 2010). Compared to its low-altitude congeners, the Fire-tailed Sunbird elevated parental investments in its offspring by producing fewer eggs and providing more parental care to nestlings (Liang et al., 2020).
Most mountain birds breed at a limited altitude (Johnson et al., 2006). Compared with low-altitude areas, the climate in high-altitude areas is more unpredictable, with lower temperatures, stronger wind, greater frequency and depth of snow, and shorter seasons suitable for bird breeding (Johnston, 1954; Stewart et al., 1977). Under the condition of limited space and resources, birds cannot avoid the competition for breeding resources in order to survive. Previous studies have found that due to competition, two ecologically similar species cannot occupy the same ecological niche but replace each other to a certain extent with regard to food or other lifestyle characteristics (Sun, 2001). The habitats of sympatrically breeding birds can overlap greatly (Minot, 1981). The utilization of similar food sources or overlapping breeding domains will lead to competition, which can directly lead to a decrease in breeding performance (Minot, 1981; Minot and Perrins, 1986). Therefore, in order to maximize their fitness, in the long-term natural evolution process, the species formed a series of breeding and survival strategies (Atramentowicz, 1992; Sinervo et al., 1992).
The Chestnut Thrush (Turdus rubrocanus) and the Kessler’s Thrush (Turdus kessleri) belong to the genus Turdus in the Passerine family and are taxonomically closely related. During the breeding period, the Chestnut Thrush primarily inhabits forests (mountain and coniferous broad-leaved, coniferous, bamboo and mixed) at altitudes between 2,000 and 3,500 m, in particular the dense coniferous and coniferous broad-leaved mixed forest. Kessler’ Thrush, on the other hand, breeds in the bushes in rocky areas above the forest line at an altitude of 3,600–4,500 m (Mackinnon et al., 2000). Both the Chestnut Thrush and the Kessler’s Thrush occur in Xinduqiao Town, Tibetan Autonomous Prefecture of Garzê, Sichuan Province, and breed sympatrically there. The region is characterized by high altitude, a harsh climate, strong solar radiation, large differences in daily temperature, and a short season that is suitable for bird reproduction.
Because the altitude of the breeding distribution of Chestnut Thrush and Kessler’s Thrush is different, in this study area, it is the overlap area of the two species of blackbird breeding distribution altitude. It provides good natural study conditions to study what reproductive strategies and life history characteristics are adopted by two similar species breeding in the same domain in the alpine environment. Although Chestnut Thrush is a common species, few people have published about its reproductive ecology before, and there are some reports about it in Lianhua Mountain, Gansu Province. Nest site characteristics and nest success (Zhao and Sun, 2018), egg rejection and recognition mechanisms (Yi et al., 2020b), nestling discrimination and feeding habits during brooding (Yi et al., 2020a), behavioral plasticity in relation to head volume (Zhao and Sun, 2016), and Parental attendance reduce nest predation during the incubation period (Hu et al., 2017), and brief field observations describing the breeding habits of the Chestnut Thrush in Wanglang Nature Reserve, Sichuan (Zhang et al., 1986), while more detailed studies on the breeding biology of the Chestnut Thrush are less available. There is little research on the reproductive habits of Kessler’s Thrush, only Yang et al. (2012) and Gao et al. (2022) have reported on the reproductive habits of Kessler’s Thrush, lacking detailed reproductive biology information (Yang et al., 2012; Gao et al., 2022). In addition, there is no report on the comparison of reproductive parameters and behaviors of two species of thrush distributed in the same domain.
We aimed to study the breeding biology (nest, egg, brooding behavior, nestling development, and breeding success) of the Chestnut Thrush and the Kessler’s Thrush. By comparing the breeding biology of the two species of thrush, we will gain a better understanding of the convergence and divergence of the breeding characteristics of the thrushes inhabiting the same area in an alpine environment. It can provide a reference for future studies regarding the relationship between the breeding behavior and environment of birds, and the coexistence mechanisms of similar species, and further enrich the breeding biology data of the Chestnut Thrush and Kessler’s Thrush.
Materials and methods
Study area
The research site is located in the scrub along the Liqiu River in Xinduqiao town, Kangding city, Tibetan Autonomous Prefecture of Garzê, Sichuan Province (101º20′-101º50′E, 29º50′-30º10′N; Figure 1). The plant community is composed of Salix cupularis, Hippophae rhamnoides, Berberis sibirica, Cerasus serrula, Malus hupehensis, Lonicera rupicola, and Caragana sinica. The area has a mountainous cold temperate climate with an average altitude of 3,460 m, strong ultraviolet radiation all year round, a large daily temperature difference, low night temperatures, an annual average temperature of 5.2°C, and an annual average rainfall of 950 mm. The main natural disasters are hail, floods, low temperatures, lightning, etc. Hail and floods are common in June and July every year.
Methodology
From April to July during 2020, 2021, and 2022, we conducted a systematic search for Chestnut Thrush and Kessler’s Thrush nests in the study area. After finding the nest, we used the mobile phone software Two Step Road Outdoor Assistant to record and number the nest site. If there were no eggs in the nest, it was observed every 3 days, and the nests with eggs were observed every other day to determine their breeding status. Clutch sizes, egg weight, egg size, hatching period, brood period, number of nestlings, nestling weight and tarsus length were recorded and measured for each nest. Egg size and nestling tarsus length were measured with a digital vernier caliper (0.01 mm), and egg weight and nestling weight were measured with a portable electronic scale (0.01 g). The egg volume (cm3) was calculated as V = Kv × L × B2/1,000, where Kv is a constant equal to 0.51, L is the maximum egg length (mm) and B is the maximum egg width (mm) (Hoyt, 1979). To reduce disturbance to breeding activities, the nest site was measured after the nestling had left the nest. The measured parameters included the species of the tree where the nest was located, the height of the tree, the distance of the nest site from the ground, and the canopy density above the nest site.
During the nestling stage, the brooding behavior and nestlings were recorded. A miniature camera (DS-200S, Wireless Low-Power Battery Camera, Guangzhou, P.R. China) was used to monitor the breeding behavior of the Chestnut Thrush and the Kessler’s Thrush, and 3 nests of each species were selected for monitoring. Cameras are installed daily from 9 am to 11 am and retrieved from 5 pm to 7 pm. By analyzing the videos at a later stage, we summarized the daily brooding (i.e., behavior that keeps nestlings warm) frequency and time, feeding frequency per hour from the beginning to the end of the monitoring period, daily feeding frequency, and the frequency of cleaning feces. Daily measurement of nestling growth parameters (body weight and tarsus length) was done at the same time each day. Breeding success was evaluated by hatching rate, fledgling rate, breeding success rate, and nest success rate. The hatching rate is the percentage of the number of hatched eggs in the total number of successful nests, the breeding success rate is the proportion of the number of successful nests in the total number of nests, and breeding success means that at least one nestling survived until it left the nest. The fledgling rate is the ratio of a fledgling number to hatched nestling, and the success rate of nesting is the percentage of nests that hatched at least one nestling to the observed nest.
Data treatment and statistical analyses
Fitting nestling growth curve by Logistic regression (Ricklefs, 1967, 1968),
where W is the measure of body weight, a is the asymptotic value, k is the instantaneous growth rate, and b is the inflection point time value (d). t 10–90 is the time it takes for the nestling to reach 10–90% of the asymptotic value. Data analyses were performed using IBM SPSS Statistics for Windows version 24.0 (IBM Corp., Armonk, NY, United States). Kolmogorov–Smirnov was used to test the normal distribution of the data. The Mann–Whitney U-test is used to compare the continuous variables of non-normal distribution, and the Independent Samples t-test is used to compare the continuous variables of normal distribution. The Chi-square test is used to compare probability (such as hatching rate, fledgling rate, etc.). All of the tests were two-tailed and data were presented as mean ± SD, and p < 0.05 was considered statistically significant.
Results
Nests and nest site selection
We found a total of 100 Chestnut Thrush nests (56 nests in 2020, 33 nests in 2021, 11 nests in 2022) and a total of 19 Kessler’s Thrush nests (9 nests in 2020, 7 nests in 2021, 3 nests in 2022). The main nests were on shrubs such as Salix cupularis, Malus hupehensis, Hippophae rhamnoides, and Cerasus serrula, and the nesting time varied from 5 to 7 days. There were no differences in nest type and nesting material between the two species of thrushes. The nests were bowl-shaped with the opening pointing upward (Figure 2). It was mainly composed of bryophytes, mud, twigs, fine grass stems, fine root whiskers, and household garbage (soft plastic film, nylon, etc.).
Overall, the nests of the two species of thrushes were very similar. There was no difference in the size of the cup of the nest. There were also no differences in nest diameter (Chestnut Thrush: 9.645 ± 0.816 cm, Kessler’s Thrush: 9.778 ± 0.647 cm, Z = −0.553, p = 0.580, Mann–Whitney U test) and cup depth (Chestnut Thrush: 6.256 ± 0.765 cm, Kessler’s Thrush: 6.914 ± 1.417 cm, Z = −1.680, p = 0.093, Mann–Whitney U-test) between the Chestnut Thrush and Kessler’s Thrush. However, the outer diameter of the Kessler’s Thrush nest (15.821 ± 1.55 cm) was larger than that of the Chestnut Thrush nest (15.003 ± 1.161 cm), and the difference was significant (independent t-test: t = −2.221, p < 0.05). The nested wall of Kessler’s Thrush was thicker than that of Chestnut Thrush.
The nest site selection of the two thrushes showed both similarities and differences. There was no difference in the tree height for nesting between the two species of thrush (Chestnut Thrush: 4.095 ± 1.367 m, Kessler’s Thrush: 5.083 ± 2.17 m, Z = −1.789, p = 0.074, Mann–Whitney U-test). There was, however, a significant difference in height of the nest in the tree between the two types of thrush (Z = −2.264, p < 0.05, Mann–Whitney U-test), the nests of Kessler’s Thrushes (2.72 ± 1.37 m (n = 12)) were higher than that of Chestnut Thrushes (2.14 ± 0.80 (n = 59)). The results showed that there was a differentiation in nesting between the two species of thrushes in vertical distribution, and the nesting of Kessler’s Thrush was higher.
Breeding time and eggs
The earliest egg of Chestnut Thrush was laid on May 3 and the latest on July 2, while the earliest egg of Kessler’s Thrush was laid on April 15 and the latest on June 13. The laying period of both species was nearly 2 months, the earliest laying date of Kessler’s Thrush was 18 days earlier than that of Chestnut Thrush, and the latest laying date of Kessler’s Thrush was 19 days later than that of Chestnut Thrush. The two species of thrushes usually lay one egg a day, occasionally one egg every other day, and lay eggs in 3–5 days.
The average clutch size of Chestnut Thrush was 3.25 ± 0.63 (range: 2–4, n = 60), the eggs were oval, the average egg weight was 7.91 ± 0.79 g, the long diameter of the eggs was 31.83 ± 2.25 mm, and the short diameter of the eggs was 22.15 ± 1.13 mm, the eggshell was light blue, with irregular brown and purple patches and spots, denser at the blunt end of the egg (Figure 2A). The average clutch size of Kessler’s Thrush was 3.29 ± 0.62 (range 2–4, n = 14), the eggs were obtuse oval, the average egg weight was 6.90 ± 1.36 g, the long diameter of the eggs was 29.60 ± 2.23 mm, and the short diameter of the eggs was 21.70 ± 1.22 mm, the eggshell was light green, with rose-tan, light gray-brown, coffee-colored fine spots and stains, and the blunt end was dense (Figure 2B).
There was no significant difference in clutch size between Chestnut Thrush and Kessler’s Thrush (Z = −0.163, p = 0.870, Mann–Whitney U-test). However, the egg size of the two thrushes was significantly different. The mean egg weight of the Chestnut Thrush was significantly higher than that of Kessler’s Thrush (Z = −3.888, p < 0.01, Mann–Whitney U-test). The egg length diameter of the Chestnut Thrush was significantly larger than that of Kessler’s Thrush (Z = −5.616, p < 0.01, Mann–Whitney U-test). The egg short diameter of Chestnut Thrush was significantly larger than that of Kessler’s Thrush (Z = −2.082, p < 0.05, Mann–Whitney U-test). The egg volume of Chestnut Thrush (8.01 ± 1.06 cm3) was significantly larger than that of Kessler’s Thrush (7.18 ± 1.25 cm3) (Z = −3.789, p < 0.01, Mann–Whitney U-test). Three eggs are the most common clutch size for both species of thrush, accounting for 52.33 and 57.14%, respectively. The hatching of both the Chestnut Thrush and Kessler’s Thrush was done by the females, while the males were on guard. Both species of thrushes are asynchronous hatching birds. The hatchlings do not hatch on the same day but usually happen over 2–3 days, with most hatching within 2 days.
Parental care
The brooding period of both species of thrushes is 15–17 days. A total of 12,216 min of effective video were recorded during the brooding stage of the Chestnut Thrush and 15,221 min of effective video were recorded during the breeding stage of the Kessler’s Thrush. The brood was undertaken by the male and the female in both thrush species, and once the eggs hatched, the eggshells were immediately removed by the female bird. Nestlings will immediately accept insects. The brooding behavior included feeding and nestling brooding and removing the fecal sac. Both species of thrushes are fed in three ways: female-to-nestling, male-to-nestling, and male-to-female-to nestling (this pathway existed only in the early stage of the nestling feeding period).
The types and quantities of food fed by parents of two types of thrushes to their nestlings during their brooding period were statistically analyzed. Both species of thrushes fed their nestlings with animal food, which mainly included worms, earthworms, arthropods, and some insects that could not be recognized because of their small size or blurred video. According to the video recordings, Chestnut Thrushes fed 2,500 insects, of which 39.64% were worms, 40.96% were earthworms, 5.8% were arthropods, and 13.6% were unidentified worms. A total of 3,040 insects were fed to Kessler’s Thrush chicks, among which worms accounted for 56.95%, earthworms accounted for 15.49%, arthropods accounted for 17.37%, and unidentified worms accounted for 10.20%.
Statistical analysis of the feeding frequency of the two thrushes during the brooding period showed that the feeding frequency of Kessler’s Thrushes was significantly higher than that of the Chestnut Thrush (Kessler’s Thrush was 12.511 ± 4.148 times/h, Chestnut Thrush was 6.8093 ± 1.26 times/h, independent t-test: t = −4.555, p < 0.01). The feeding frequency of Chestnut Thrush increased first and remained stable after the chicks were 5 days old. The feeding frequency of Kessler’s Thrush increased at first, then increased slowly after the chicks were 5 days old, and decreased after they were 8 days old, showing a unimodal pattern (Figure 3A). The study on the brooding time and frequency of the two thrush species during the monitoring period showed that the brooding time of both decreased as the nestlings aged (Figure 3B). There is no significant difference between the two female thrush birds in their daily brooding time (Chestnut Thrush: 0.3519 ± 0.224; Kessler’s Thrush: 0.4158 ± 0.242; Independent t-test: t = −0.670, p = 0.510). However, the two thrush species adopted different ways of brooding their chicks. Chestnut Thrush females brooded nestlings more frequently but for a shorter time, while Kessler’s Thrush brooded the nestlings less frequently but for a longer time period (Figures 3C,D).
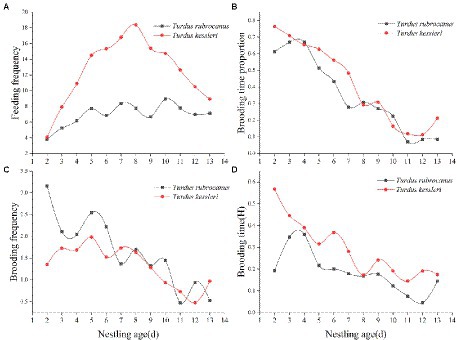
Figure 3. (A) Average feeding times per hour of two kinds of thrush in daily monitoring time. (B) The proportion of nesting time of female birds of the two thrush species were monitored daily. (C) The average number of times that two thrushes warmed their nestling per hour during daily monitoring. (D) The average warming time per hour of two thrush species was monitored daily.
Growth and development of nestlings
Nestlings from both Chestnut Thrushes and Kessler’s Thrushes are naked with red skin when they emerge from the eggs, light brown fetal feathers occur on both sides of the top of the head, middorsal line (upper back to tail base), and on both wings, and eyes are not open. At days 7 to 8, the eyes open in an oval shape, most of the body has feathers, the tail feathers and primary flight feathers are out of the feather sheath, the baby bird can crawl, and there is avoidance behavior. At days 12–13, the chicks are fully feathered, when frightened, they immediately leave the nest, however, their flying ability is poor. Once on the ground, they can fly jump, and take swift action. After diving into the dense forest, they do not return to the nest.
We fitted the measurements of body weight and tarsus length of 11 nestlings from 3 nests of Chestnut Thrushes and 9 nestlings from 3 nests of Kessler’s Thrushes using Logistic curve equations. The growth curves of body mass and tarsus length were both well-fitted by logistic regression (Figure 4), and the observed value is significantly correlated with the fitting value.
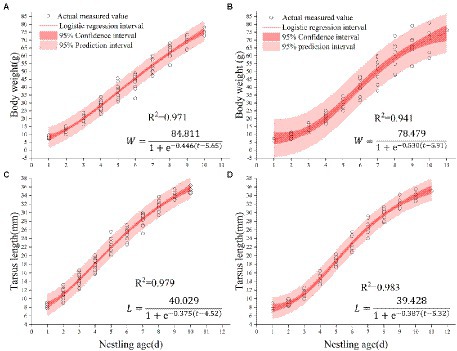
Figure 4. (A) Body weight fitting curve of Chestnut Thrush. (B) Body weight curve of Kessler’s Thrush. (C) Tarsus fitting curve of Chestnut Thrush. (D) Tarsus fitting curve of Kessler’s Thrush.
Nestlings grew rapidly, and Chestnut Thrush nestlings reached 90% of their asymptote weight at 9.96 days of age. The average weight of the hatchling is 8.22 ± 0.90 g, and the average weight on the 10th day is 74.74 ± 2.08 g, with an average increase of 7.39 ± 0.31 g/day. At the age of 8.29 days, the weight of Kessler’s Thrush nestlings reached 90% of the body weight of the asymptote. The average weight of the hatchling was 6.66 ± 0.59 g, and the average weight of the nestling on the 9th day was 67.00 ± 6.22 g, with an average daily increase of 8.24 ± 0.63 g. The hatchlings of Chestnut Thrushes were heavier than those of Kessler’s Thrushes, and the growth rate and relative growth rate of Kessler’s Thrush nestlings were higher than those of Chestnut Thrushes (Figure 5A). It took fewer days to complete the development of 10–90% of the body weight than that of Chestnut Thrush. The relative growth rate of the tarsus of Kessler’s Thrushes was higher than that of Chestnut Thrushes (Figure 5B). Kessler’s Thrush nestlings adopted a rapid development growth strategy.
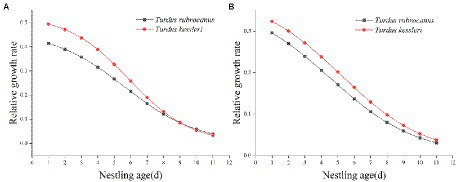
Figure 5. (A) The relative growth rate of body weight of two kinds of thrush. (B) The relative growth rate of tarsus of two kinds of thrush.
Breeding success
Of the 63 Chestnut Thrush nests with known breeding conditions, 36 nests successfully hatched birds (3 nests failed, 3 nests were predated, and 1 nest failed with an unknown cause). Of the remaining 27 nests, 5 nests were destroyed by humans, 15 nests were predated (28.57%), and 7 nests were abandoned (11.11%). The breeding success rate was 46%, the hatching rate was 95%, the fledging rate was 78% and the nesting success rate was 46%.
Of the 14 Kessler’s Thrush nests, 9 nests hatched successfully (2 nests were preyed on), 3 of the remaining 5 nests were preyed on (35.71%), and 2 nests were abandoned (14.29%). The breeding success rate was 50%, the hatching rate was 96.77%, the fledging rate was 80%, and the nesting success rate was 50%. There is no significant difference in the breeding success rate, hatching rate, fledgling rate, and nesting success rate between the two thrushes (Pearson Chi-square test p > 0.05).
Discussion
Nest and nest site selection
Nest site selection is a critical component of habitat selection, and its effectiveness directly affects breeding performance (Macdonald et al., 2016; Maisey et al., 2016). Nest site selection is influenced by different combinations of biotic and abiotic factors in the environment. Birds that coexist in the same environment will inevitably have different needs for food resources, nesting sites, and breeding phenology. The results of our study showed that the nest sites of the Chestnut Thrush and Kessler’s Thrush overlapped, but the nesting height was stratified. Kessler’s Thrushes nested significantly higher than Chestnut Thrushes. Sympatrically breeding birds may coexist successfully by dividing limited resources and reducing interspecific competition within the same area (Sommer and Worm, 2002; Atiénzar et al., 2013). For example, two sympatric Alpine Rose finches that breed in the Tibetan mountains divide their ecological niches by nesting on shrubs at different heights (Lu et al., 2011).
There is no difference in the size of the inner nest cup between Chestnut Thrush and Kessler’s Thrush, but the nest wall of Kessler’s Thrush is thicker than that of Chestnut Thrush. Kessler’s Thrush can breed at higher altitudes than Chestnut Thrush (Mackinnon et al., 2000), and with increasing altitude, temperatures decrease, and Kessler’s Thrush has thicker nest walls to adapt to colder conditions. It has been found that as the temperature decreases with the increase of latitude, the thickness of the wall of the open nest also increases, whereas the diameter of the inner nest cup does not change (Mainwaring et al., 2014a). It is also possible that Kessler’s Thrush breeds earlier than Chestnut Thrush start breeding earlier, starts breeding when the temperature is low and increases the thickness of the nest wall in order to maintain the temperature.
Breeding time and eggs
The specialized natural environment at high altitudes, such as the short season suitable for bird breeding and the colder climate, has certain restrictions on the breeding of birds (Kovshar, 1981; Badyaev, 1987). Breeding timing is considered a life history strategy, and different timing of breeding can also reduce the stress of reproductive competition (Minot and Perrins, 1986; Dhondt, 2010). Our research shows that the breeding time of Kessler’s Thrush is about half a month earlier than that of Chestnut Thrush. In previous studies of birds breeding in the same domain, similar trends have been confirmed. Birds breeding in the same domain can reduce the overlapping degree of the breeding period with other birds by adjusting their breeding time, to reduce the resource competition among different species during the breeding period (Macarthur, 1958; Prokesova and Kocian, 2004; Atiénzar et al., 2013).
The food restriction hypothesis suggests that the clutch size of birds is determined by the maximum food resources available (Lack, 1947, 1948; Lack, 1968; Martin, 1987). Under certain conditions, birds can ensure the maximum number of viable offspring by selecting the optimal clutch size and achieving the maximum reproductive effect (Charnov and Krebs, 1974). In addition to being influenced by food resources, avian clutch size is related to a variety of factors including latitude, altitude, nest structure, and nest predation rate (Skutch, 1985; Kulesza, 1990). Due to the nature of the environmental factors and food resources, the clutch sizes of sympatric species should tend to be similar. This is also supported by our results, which show no difference in clutch size between sympatrically breeding Chestnut Thrushes and Kessler’s Thrushes. In terms of egg size, the eggs of Chestnut Thrush are larger and heavier than those of Kessler’s Thrush. Compared to egg volumes of conspecifics at other elevations, egg volumes were larger with increasing elevation. The egg volume of Chestnut Thrush at different elevations is 2,100 m V = 7.05 ± 0.38 cm3 (Zhang et al., 1986), 2,700 m V = 7.5 ± 0.04 cm3 (Zhao and Sun, 2018), 3,400 m V = 8.01 ± 1.06 cm3 (this article), and the egg volume of Kessler’s Thrush at different elevations is 3,400 m V = 6.9 ± 1.36 cm3 (this article), 4,100 m V = 7.99 ± 0.84 cm3 (Yang et al., 2012). Toward higher elevations, organisms are increasingly exposed to cold, seasonal climates, strong solar radiation, low atmospheric pressures, and poor oxygen availability (Körner, 2007). Large eggs seem to have an advantage in harsh environments (Smith et al., 1995; Fox and Czesak, 2000). By lowering the surface area-to-volume ratios (Mcnab, 2002), larger offspring may buffer heat or water loss caused by the temperature and atmospheric conditions of high elevations. This will improve the adaptability of nestlings in harsh environments to make up for the cost of the environment (Smith et al., 1995; Fox and Czesak, 2000).
Parental care
Parental care is important for offspring survival and fitness (Buitron, 1988; Ghalambor et al., 2013), species living at higher elevations increase their parental care to compensate for low fecundity, thus allowing their nestling to survive in extreme environments (Badyaev and Ghalambor, 2001; Hille and Cooper, 2015; Boyle et al., 2016; Liang et al., 2020). The results showed that both species of thrushes were raised jointly by their parents, and the frequency of feeding nestling by Kessler’s Thrush was higher than that by Chestnut Thrush because Kessler’s Thrush nestling developed more rapidly and had more food requirements. The two thrush brood diets overlapped substantially, but the two thrush showed different preferences for the types of food. Chestnut Thrush preferred worms and earthworms, while Kessler’s Thrush primarily fed worms but incorporated a larger proportion of arthropods. The two species of thrush reduce competition among themselves through their preference for different foods. In terms of brooding nestlings, Chestnut Thrush adopted a strategy of brooding nestling more frequently and for a short time, while Kessler’s Thrush brooded nestlings less frequently but for a longer time. Species dwelling in the same environment may use different life history strategies to cope with the local environment (Auer et al., 2007).
Nestling growth
Nestlings are affected by a variety of internal and external factors during the process of growth and development. We found that the nestling growth and development of Kessler’s Thrush is faster than that of Chestnut Thrush. Because Kessler’s Thrush is distributed at higher altitudes, high-altitude birds increase the feeding of their parents and adapt to low temperatures, and develop faster than nestlings at low altitudes (Badyaev and Ghalambor, 2001). In addition, rapid growth is also an adaptation to the short breeding seasons in alpine environments.
Breeding success
The breeding success of birds is restricted by many factors, including the nutritional conditions during laying eggs, the weather conditions during the hatching or brooding period, predation, egg quality, and human disturbance (Angelstam et al., 1984; Moss and Watson, 1984; Brittas, 1988). As a result of low temperatures, strong seasonality, short breeding seasons and the strong fluctuation of food availability at high altitudes (Kovshar, 1981), the production of small clutches and large eggs is beneficial to increase the investment in each offspring to improve survival (Berven, 1982a, b; Blanckenhorn, 1997). Our results show that the breeding success rate, hatching rate, fledgling rate, nesting success rate, and predation rate of the Chestnut Thrush and Kessler’s Thrush are similar. Since the breeding distribution of the Chestnut Thrush and Kessler’s Thrush is sympatric in time and space, and they have the same environment and nest predation risk, they have the same breeding success. In the study area, the main cause of breeding failure was nest predation. The nest predation rate of Kessler’s Thrush was slightly higher than that of Chestnut Thrush. There are two possible reasons for this: (1) it is related to the feeding frequency of the parent birds, which is higher for the Kessler’s Thrush than for the Chestnut Thrush. High nest attendance and feeding frequencies of high-altitude species may in turn result in high exposures to potential predators (Conway and Martin, 2000) and therefore lower their breeding successes. (2) It is related to the nesting location because Kessler’s Thrush nests higher than Chestnut Thrush. Nest height may directly affect the breeding success of birds, as taller nests are more vulnerable to aerial predators, while lower nests are more vulnerable to ground predators (Piper and Catterall, 2004; Mainwaring et al., 2014b; Tabib et al., 2016).
In our study area, the primary threat comes from aerial predators, therefore higher nesting sites are more at risk. Although we have not been able to identify the predators of the two species of thrush in the study area, we have observed Eudynamys scolopaceus preying on Trochalopteron elliotii nestlings, Lanius tephronotus attacking T. elliotii nestlings, and studies have also found L. tephronotus predation on the eggs of Turdus merula maximus (Purbu et al., 2018). The second was nest abandonment and human destruction. The nest abandonment rate of Chestnut Thrush was higher than that of Kessler’s Thrush. A part of the study area is in a wetland Park which is a tourist attraction and is greatly disturbed by humans. A total of 5 Chestnut Thrush nests in this area were destroyed by human beings. The impact of extreme weather is also an important reason for forcing birds to abandon their nests. The study area usually experiences extreme weather such as snow, hail, strong winds, and heavy rain during the breeding season.
Conclusion
The results show that the breeding biology of the sympatrically breeding Chestnut Thrush and Kessler’s Thrush in the alpine environment shows convergent and divergent characteristics. There were no differences between the two thrushes in terms of nest material, clutch size, type of food fed to the nestling, and breeding performance. In terms of breeding time, Kessler’s Thrushes bred half a month earlier than Chestnut Thrushes and Chestnut Thrushes lay heavier and larger eggs. Kessler’s Thrushes invest more in their nestlings compared to Chestnut Thrushes and brooded their chicks for longer periods of time but less frequently than Chestnut Thrushes. The nestling growth rate of Kessler’s Thrushes is higher and they develop faster. These results suggest that the Chestnut Thrush with a lower breeding distribution, exhibits characteristics similar to that of low-altitude birds, and the Kessler’s Thrush with a higher breeding distribution resembles high-altitude birds with its breeding characteristics. This indicates that the breeding strategies of birds in the alpine environment are not only affected by environmental factors but also may be affected by genetics or phylogeny.
Data availability statement
The original contributions presented in the study are included in the article/Supplementary material, further inquiries can be directed to the corresponding author.
Ethics statement
The animal study was reviewed and approved by Research Ethics Review Committee of China west Normal University (CWNU2020D08).
Author contributions
HZ and YG: conceived and designed the study. HX, HZ, and YG: collected data and samples in the field. YG: analyzed the data. HZ and YG: wrote the manuscript. All authors contributed to the article and approved the submitted version.
Funding
This study was supported by the Science and Technology Department of Sichuan Province (2022JDR0033 and 2022JDJQ0060), the Second Tibetan Plateau Scientific Expedition (2019QZKK05010502), and the National Natural Science Foundation of China (32270552).
Conflict of interest
The authors declare that the research was conducted in the absence of any commercial or financial relationships that could be construed as a potential conflict of interest.
Publisher’s note
All claims expressed in this article are solely those of the authors and do not necessarily represent those of their affiliated organizations, or those of the publisher, the editors and the reviewers. Any product that may be evaluated in this article, or claim that may be made by its manufacturer, is not guaranteed or endorsed by the publisher.
References
Angelstam, P., Lindström, E., and Widén, P. (1984). Role of predation in short-term population fluctuations of some birds and mammals in Fennoscandia. Oecologia 62, 199–208. doi: 10.2307/4217304
Atiénzar, F., Belda, E. J., and Barba, E. (2013). Coexistence of Mediterranean tits: a multidimensional approach. Ecoscience 20, 40–47. doi: 10.2980/20-1-3516
Atramentowicz, M. (1992). Optimal litter size: does it cost more to raise a large litter in caluromys philander? Can. J. Zool. 70, 1511–1515. doi: 10.1139/z92-208
Auer, S. K., Bassar, R. D., Fontaine, J. J., and Martin, T. E. (2007). Breeding biology of passerines in a subtropical montane forest in northwestern Argentina. Condor 109, 321–333. doi: 10.1093/condor/109.2.321
Badyaev, A. (1987). Composition and distribution of the Pamir-Alay mountain avifauna (98 spp.) and peculiarities of avian biology in conditions of subalpine juniper bush belt. Moscow: Moscow University.
Badyaev, A. V. (1997). Altitudinal variation in sexual dimorphism: a new pattern and alternative hypotheses. Behav Eco 8, 675–690. doi: 10.1093/beheco/8.6.675
Badyaev, A. V., and Ghalambor, C. K. (2001). Evolution of life histories along elevational gradients: trade-off between parental care and fecundity. Ecology 82, 2948–2960. doi: 10.1890/0012-9658(2001)082[2948,EOLHAE]2.0.CO;2
Berven, K. A. (1982a). The genetic basis of altitudinal variation in the wood frog Rana sylvatica. I. an experimental analysis of life history traits. Evolution 36, 962–983. doi: 10.2307/2408075
Berven, K. A. (1982b). The genetic basis of altitudinal variation in the wood frog Rana sylvatica ii. An experimental analysis of larval development. Oecologia 52, 360–369. doi: 10.2307/4216627
Blanckenhorn, W. U. (1997). Altitudinal life history variation in the dung flies scathophaga stercoraria and sepsis cynipsea. Oecologia 109, 342–352. doi: 10.1007/s004420050092
Boyle, A. W., Sandercock, B. K., and Martin, K. (2016). Patterns and drivers of intraspecific variation in avian life history along elevational gradients: a meta-analysis. Biol. Rev. 91, 469–482. doi: 10.1111/brv.12180
Brittas, R. (1988). Nutrition and reproduction of the willow grouse Lagopus lagopus in Central Sweden. Ornis Scand. 19:49. doi: 10.2307/3676527
Buitron, D. (1988). Female and male specialization in parental care and its consequences in black-billed magpies. Condor 90, 29–39. doi: 10.2307/1368429
Charnov, E. L., and Krebs, J. R. (1974). On clutch size and fitness. Ibis 116, 217–219. doi: 10.1111/j.1474-919X.1974.tb00241.x
Conway, C. J., and Martin, T. E. (2000). Evolution of passerine incubation behavior: Influence of food, temperature, and nest predation. Evolution 54, 670–685. doi: 10.1111/j.0014-3820.2000.tb00068.x
Dhondt, A. A. (2010). Effects of competition on great and blue tit reproduction: intensity and importance in relation to habitat quality. J. Anim. Ecol. 79, 257–265. doi: 10.1111/j.1365-2656.2009.01624.x
Fox, C. W., and Czesak, M. E. (2000). Evolutionary ecology of progeny size in arthropods. Annu. Rev. Entomol. 45, 341–369. doi: 10.1146/annurev.ento.45.1.341
Gao, Y., Xiao, H., Zhou, H., and Zhang, Z. (2022). Breeding ecology of Kessler’s thrush (Turdus kessleri) in the western Sichuan plateau. Anim. Biol. 72, 275–287. doi: 10.1163/15707563-bja10083
Ghalambor, C. K., Peluc, S. I., and Martin, T. E. (2013). Plasticity of parental care under the risk of predation: how much should parents reduce care? Biol. Lett. 9:20130154. doi: 10.1098/rsbl.2013.0154
Hille, S. M., and Cooper, C. B. (2015). Elevational trends in life histories: revising the pace-of-life framework. Biol. Rev. 90, 204–213. doi: 10.1111/brv.12106
Hoyt, D. F. (1979). Practical methods of estimating volume and fresh weight of bird eggs. Auk 96, 73–77. doi: 10.1093/auk/96.1.73
Hu, Y. B., Zhao, Q. S., Lou, Y. Q., Chen, L. J., González, M. A., and Sun, Y. H. (2017). Parental attendance of chestnut thrush reduces nest predation during the incubation period: compensation for low nest concealment? J. Ornithol. 158, 1111–1117. doi: 10.1007/s10336-017-1476-1
Johnson, L. S., Ostlind, E., Brubaker, J. L., Balenger, S. L., Johnson, B. G., and Golden, H. (2006). Changes in egg size and clutch size with elevation in a Wyoming population of mountain bluebirds. Condor 108, 591–600. doi: 10.1093/condor/108.3.591
Johnston, R. F. (1954). Variation in breeding season and clutch size in song sparrows of the pacific coast. Condor 56, 268–273. doi: 10.2307/1364850
Körner, C. (2007). The use of ‘altitude’ in ecological research. Trends Ecol. Evol. 22, 569–574. doi: 10.1016/j.tree.2007.09.006
Kovshar, A. (1981). Peculiarities of the Bird Reproduction in Subalpine: On the Material of Passeriformes in the Tien Shan. Academy of Sciences of the Kazakh SSR, Alma-Ata.
Kulesza, G. (1990). An analysis of clutch-size in new world passerine birds. Ibis 132, 407–422. doi: 10.1111/j.1474-919X.1990.tb01059.x
Lack, D. (1947). The significance of clutch size. Ibis 89, 302–352. doi: 10.1111/j.1474-919X.1947.tb04155.x
Lack, D. (1948). The significance of clutch size. Part iii. Some interspecific comparisons. Ibis 90, 25–45. doi: 10.1111/j.1474-919X.1948.tb01399.x
Liang, D., Liu, Y., Gao, G., and Luo, X. (2020). Breeding biology of a high altitudinal aethopyga sunbird in southwestern China. J. Nat. Hist. 54, 2381–2390. doi: 10.1080/00222933.2020.1845408
Lu, X., Gong, G., and Ma, X. (2011). Niche segregation between two alpine rosefinches: to coexist in extreme environments. Evol. Biol. 38, 79–87. doi: 10.1007/s11692-010-9102-7
Lu, X., Yu, T., Liang, W., and Yang, C. (2010). Comparative breeding ecology of two white-bellied redstart populations at different altitudes. J. Field Ornithol. 81, 167–175. doi: 10.1111/j.1557-9263.2010.00274.x
Macarthur, R. H. (1958). Population ecology of some warblers of northeastern coniferous forests. Ecology 39, 599–619. doi: 10.2307/1931600
Macdonald, E. C., Camfield, A. F., Martin, M., Wilson, S., and Martin, K. (2016). Nest-site selection and consequences for nest survival among three sympatric songbirds in an alpine environment. J. Ornithol. 157, 393–405. doi: 10.1007/s10336-015-1286-2
Mackinnon, J. R., Mackinnon, J., Phillipps, K., and He, F. Q. (2000). A Field Guide to the Birds of China. Oxford: Oxford University Press.
Mainwaring, M. C., Deeming, D. C., Jones, C. I., and Hartley, I. R. (2014a). Adaptive latitudinal variation in common blackbird Turdus merula nest characteristics. Ecol. Evol. 4, 851–861. doi: 10.1002/ece3.952
Mainwaring, M. C., Hartley, I. R., Lambrechts, M. M., and Deeming, D. C. (2014b). The design and function of birds' nests. Ecol. Evol. 4, 3909–3928. doi: 10.1002/ece3.1054
Maisey, A. C., Carter, N. T., Incoll, J. M., and Bennett, A. F. (2016). Environmental influences on variation in nest characteristics in a long-term study population of the superb lyrebird, Menura novaehollandiae. Emu 116, 445–451. doi: 10.1071/MU16002
Martin, T. E. (1987). Food as a limit on breeding birds: a life-history perspective. Annu. Rev. Ecol. Syst. 18, 453–487. doi: 10.1146/annurev.es.18.110187.002321
Martin, T. E. (2004). Avian life-history evolution has an eminent past: does it have a bright future? Auk 121, 289–301. doi: 10.1093/auk/121.2.289
Mcnab, B. K. (2002). Minimizing energy expenditure facilitates vertebrate persistence on oceanic islands. Ecol. Lett. 5, 693–704. doi: 10.1046/j.1461-0248.2002.00365.x
Minot, E. O. (1981). Effects of interspecific competition for food in breeding blue and great tits. J. Anim. Ecol. 50:375. doi: 10.2307/4061
Minot, E. O., and Perrins, C. (1986). Interspecific interference competition--nest sites for blue and great tits. J. Anim. Ecol. 1986, 331–350. doi: 10.2307/4712
Moss, R., and Watson, A. (1984). Maternal nutrition, egg quality and breeding success of Scottish ptarmigan Lagopus mutus. Ibis 126, 212–220. doi: 10.1111/j.1474-919X.1984.tb08000.x
Mu, H., Liu, N., and Yang, M. (2008). Breeding of the black redstart Phoenicurus ochruros rufiventris in the southeastern qingzang (Qinghai-Tibetan) plateau. Acta Zool. Sin. 54, 201–208.
Partridge, L., and Harvey, P. H. (1988). The ecological context of life history evolution. Science 241, 1449–1455. doi: 10.1126/science.241.4872.1449
Piper, S. D., and Catterall, C. P. (2004). Effects of edge type and nest height on predation of artificial nests within subtropical Australian eucalypt forests. Forest Ecol. Mana. 203, 361–372. doi: 10.1016/j.foreco.2004.08.005
Prokesova, J., and Kocian, L. (2004). Habitat selection of two Acrocephalus warblers breeding in reed beds near malarky (Western Slovakia). Biologia 59, 637–644.
Purbu, T., Li, Z., Tsamchoe, C. Z., and Liu, J. (2018). Breeding ecology of the Turdus merula Maximus in Lhasa. Tibet. Plateau Sci. Res. 2, 31–37. doi: 10.16249/j.cnki.2096-4617.2018.01.004
Ricklefs, R. E. (1967). A graphical method of fitting equations to growth curves. Ecology 48, 978–983. doi: 10.2307/1934545
Ricklefs, R. E. (1968). Patterns of growth in birds. Ibis 110, 419–451. doi: 10.1111/j.1474-919X.1968.tb00058.x
Sinervo, B., Zamudio, K., Doughty, P., and Huey, R. B. (1992). Allometric engineering: a causal analysis of natural selection on offspring size. Science 258, 1927–1930. doi: 10.1126/science.258.5090.192
Skutch, A. F. (1985). Clutch size, nesting success, and predation on nests of neotropical birds reviewed. Ornithol. Monogr. 1985, 575–594. doi: 10.2307/40168306
Smith, H. G., Ohlsson, T., and Wettermark, K. J. (1995). Adaptive significance of egg size in the European starling: experimental tests. Ecology 76, 1–7. doi: 10.2307/1940626
Sommer, U., and Worm, B. (2002). Synthesis: Back to Santa Rosalia, or No Wonder There Are So Many Species[C]//Competition and Coexistence. Berlin, Germany: Springer, 207–218.
Stewart, R. M., Henderson, R. P., and Darling, K. (1977). Breeding ecology of the Wilson’s warbler in the high sierra Nevada, California. Living Bird 16, 83–102.
Sun, R. (2001). Principles of animal ecology. 3rd Edn. Beijing: Beijing Normal University Press, 70–71.
Tabib, R., Adamou, A. E., Ouakid, M. L., Glądalski, M., and Bańbura, J. (2016). Nesting height and nest-cup volume variation in rufous bush chats (Cercotrichas galactotes) inhabiting Algerian oases: consequences for breeding success. Avian Biol. Res. 9, 114–119. doi: 10.3184/175815516X14575520344
Yang, N., Zhang, K., Dou, L., Zhang, X., Li, B., Yue, B., et al. (2012). Observing on the breeding habits of Turdus kessleri. Sichuan. J. Zool. 31, 68–70.
Yi, T., Sun, Y. H., and Liang, W. (2020a). Egg rejection and egg recognition mechanism of chestnut thrushes (Turdus rubrocanus). Behav. Process. 178:104158. doi: 10.1016/j.beproc.2020.104158
Yi, T., Sun, Y. H., and Liang, W. (2020b). Nestling discrimination and feeding habits during brooding of chestnut thrushes. Avian Res 11, 1–7. doi: 10.1186/s40657-020-00202-z
Zhang, R., Liu, C., and Wang, C. (1986). Breeding habits of Turdus rubrocanus. Chinese J. Wildlife 2, 11–13. doi: 10.19711/j.cnki.issn2310-1490.1986.02.008
Zhao, Q., and Sun, Y. (2016). Behavioral plasticity is not significantly associated with head volume in a wild chestnut thrush (Turdus rubrocanus) population. Avian Res 7, 1–6. doi: 10.1186/s40657-016-0048-z
Keywords: sympatric breeding, alpine environment, nest site selection, brooding behavior, nestling growth
Citation: Gao Y, Xiao H, Han H, Wei W, Zhou H and Zhang Z (2022) Comparison of breeding strategies of two sympatric thrush species in an alpine environment. Front. Ecol. Evol. 10:1049983. doi: 10.3389/fevo.2022.1049983
Edited by:
Maria K. Oosthuizen, University of Pretoria, South AfricaReviewed by:
Li Ding, Southwest University of Science and Technology, ChinaSen Song, Lanzhou University, China
Copyright © 2022 Gao, Xiao, Han, Wei, Zhou and Zhang. This is an open-access article distributed under the terms of the Creative Commons Attribution License (CC BY). The use, distribution or reproduction in other forums is permitted, provided the original author(s) and the copyright owner(s) are credited and that the original publication in this journal is cited, in accordance with accepted academic practice. No use, distribution or reproduction is permitted which does not comply with these terms.
*Correspondence: Hong Zhou, zhouhong1026@163.com; Zejun Zhang, zhangzj@ioz.ac.cn