- 1College of Geography and Remote Sensing Sciences, Xinjiang University, Ürümqi, China
- 2Key Laboratory of Oasis Ecology of Education Ministry, Xinjiang University, Ürümqi, China
- 3Department of Natural Resources of Xinjiang Province, Land Consolidation and Rehabilitation Center, Ürümqi, China
- 4College of Ecology and Environment, Xinjiang University, Ürümqi, China
- 5Xinjiang Jinghe Observation and Research Station of Temperate Desert Ecosystem, Ministry of Education, Ürümqi, China
- 6Institute of Desert Meteorology, China Meteorological Administration, Ürümqi, China
To explore the differences in stoichiometric homeostasis and resorption efficiency of nitrogen (NRE) and phosphorus (PRE) of tree species in Kanas natural forest. We selected four primary tree species: Larix sibirica (LS), Picea obovata (PO), Pinus sibirica (PS), and Betula pendula (BP) and measured concentrations of carbon (C), nitrogen (N), and phosphorus (P) in fresh leaves, leaf litters, and soil. Our findings showed that compared to deciduous species (LS, BP), evergreen species (PO, PS) had higher fresh leaf C concentrations and C: N ratios. As opposed to evergreen plants, deciduous species have higher levels of fresh leaf N concentration, while the P concentration and C: P did not exhibit such a pattern. Mass-based NRE and PRE averaged 52.55 and 49.16%, respectively, with a significant difference among life forms in NRE but no varied in PRE. NRE increased with N concentration in fresh leaves of BP, PO, and LS, NRE decreased with N concentration in leaf litters of all species; PRE increased with P concentration in fresh leaves of all species, PRE decreased with P concentration in leaf litters of LS, PO, and PS. Only NRE of BP and PS and PRE of PS showed significant relationship with soil N and P concentrations, respectively. Neither NRE nor PRE was insignificantly related to mean annual temperature (MAT) for all species. The N concentration of all species showed strict homeostasis corresponding to their being limited by the N element. Only the P concentration of PS and PO and N: P ratio of PS varied with corresponding traits in soil. Overall, our results provide insight into the N and P nutrient use characteristics of tree species in the Kanas natural forest and can provide a scientific basis for regional ecological restoration.
Introduction
Plant nutrient resorption is one of the essential mechanisms for nutrient conservation and utilization by plants (Blanco et al., 2009). It refers to the process of transferring readily transferable nutrients from senescing tissues to other living parts for reuse in growth (Aerts, 1996; Brant and Chen, 2015), which can effectively reduce plants’ reliance on soil nutrients and have essential effects on critical processes such as nutrient uptake, productivity, inters-specific competition, and carbon cycling (Killingbeck, 1996; Seidel et al., 2019; Liu X. et al., 2020). Carbon (C), nitrogen (N), and phosphorus (P) are major macro elements necessary for life (Elser et al., 2000); their cycling in plant leaves–litter–soil systems has substantial effects on the function and stability of ecosystems. Due to the important function of N and P (Wang et al., 2022), previous studies generally pay more attention to the resorption efficiency of N (NRE) and P (PRE).
Previous studies have examined the differences in nutrient requirements and nutrient resorption between evergreen and deciduous tree species. Deciduous tree species are nutrient luxury consumption type due to the relatively frequent leaf drop and the occurrence of new leaves, as the high soil nutrient requirements. Evergreen species have long-lived leaves and low nutrient demand, so their evergreen habit is considered an adaptation to poor soil habitats (Aerts, 1995; Givnish, 2002). Aerts (1996) found that the NRE of evergreen species in temperate forests were significantly lower than deciduous species in temperate forests, while PRE were not significantly different between them. Tang et al. (2013) showed that deciduous species in subtropical broadleaf evergreen forests have higher PRE than evergreen species, but no significant difference in NRE. In addition, Lal et al. (2001) have shown that deciduous tree species in tropical arid habitats have higher NuRE than evergreen tree species. There is currently a lack of clarity regarding the variations in NuRE in different life forms.
NuRE can be affected by soil nutrients, climate factors, species-specific variations, and the nutrient concentrations of litters and fresh leaves (Yuan and ChenHan, 2009a; Wang et al., 2018, 2019). Yuan and ChenHan (2009a) have found that NRE decreased with mean annual temperature (MAT) and mean annual precipitation (MAP). In contrast, PRE increase with MAT and MAP. Bai et al. (2015) found that NRE decreased with altitude (ALT), while PRE were the opposite. See et al. (2015) reported that plant NuRE was positively correlated with soil nutrients, but it was also reported that plant NuRE was negatively correlated with soil nutrients (Tully et al., 2013) or not (Tang et al., 2013). Also, there is no consensus on the relationship between NuRE and leaf nutrient status. Some reports indicate that the higher the N content of mature leaves, the higher their NRE during senescence, while no such relationship exists for PRE (Zhou et al., 2016), and a few reports found that NuRE decreases with the nutrient content of mature leaves (Liu et al., 2014). The effects of leaf nutrients on NuRE are complex, and recent studies used NuRE to explain nutrient limitations. Moreover, studies on the relationship between NuRE and environmental factors (MAT and soil factors) and leaf nutrients have been limited in Taiga ecosystems.
Ecological stoichiometry, which is used to evaluate the balances of energy and chemical elements in ecosystems, is a powerful tool for understanding ecological processes and relationships among element cycles in plant leaves–litters–soil systems (Elser et al., 2010). Leaf carbon: nitrogen ratio (C: N ratio) and carbon: phosphorus ratio (C: P ratio) represent the capacity of plants to assimilate the C and reflect the efficiency of N and P utilization by plants (Yang et al., 2021). Furthermore, leaf nitrogen: phosphorus ratio (N: P ratio) can be used to determine nutrient limitations for growth. Stoichiometric homeostasis, a basis and core of ecological stoichiometry theory, comprehensively reflects species’ adaptability to environmental changes (Persson et al., 2010). Higher stoichiometric homeostasis in plants corresponds to the maintenance of ecosystem functions and stability (Yu et al., 2010). When soil nutrients limit plant growth, plants can adapt through a variety of physiological processes to improve the internal availability and use efficiency of the limiting nutrient, hence maintaining stability and the functions associated with it (Hessen et al., 2004). However, studies on stoichiometric homeostasis mainly focus on algae, zooplankton, and herb plants, with few studies on higher plants and even fewer on tree plants in the Taiga forest ecosystem (Deng et al., 2019). Therefore, the evaluation of stoichiometric homeostasis and C: N: P stoichiometry in this forest could enhance our knowledge of tree species’ adaptation processes, nutrient cycles, and ecosystem stability.
The Altai natural forest, as a typical representative of the western Siberian mountain taiga in China, preserves the original ecosystem represented by the taiga, which is the only distribution area of the Palearctic European-Siberian flora and fauna in China and has high scientific research value (Liu X. J. et al., 2020). It plays an important role in regional water vapor and nutrient cycling and diversity maintenance and provides an ideal research field for investigating the stoichiometric and NuRE of many different tree species under similar environmental conditions. In this study, we examined variations in C, N, and P concentrations and their ratios in the fresh leaves and leaf litters of four tree species in the Kanas National Nature Reserve, China. We analyzed the NuRE and stoichiometric homeostasis of four tree species. Specifically, we tested the following hypotheses: (1) NuRE would be different between evergreen species and deciduous species, (2) NuRE would be negatively correlated with the leaf litter N and P concentrations and positively correlated with the fresh leaf N and P concentrations, (3) NuRE would be influenced by environmental factors (MAT and soil factors), and (4) evergreen trees would exhibit greater stoichiometric homeostasis than deciduous trees.
Materials and methods
Study area
We conducted this research in Burqin County, Xinjiang Province, China, at the Kanas National Nature Reserve (87°01′45″E∼87°33′50″E, 48°36′18″N∼48°38′56″N, area 673 km2). The climate at the site is a typical temperate alpine mountainous climate, with a MAT of approximately 0.2°C, MAP of 1,065 mm, mainly concentrated in May–August, and snowfall primarily in January–April and September–December, with snowfall accounting for about 40% of the annual precipitation, and the frost-free period is about 90–108 days (Liu et al., 2016). The forests are mainly located on shady and semi-shady slopes from 1,300 to 2,300 m above sea level, and the soil type is mountain brown coniferous forest soil. The main tree species in the study area are Larix sibirica, Picea obovata, Pinus sibirica, and Betula pendula. The main species of the shrub layer are Lonicera caerulea, Rosa acicularis, Vaccinium Vitis-idaea, and Spiraea chamaedryfolia. The main species of the herb layer are Carex polyphylla, Poa nemoralis, and Elymus nutans (Liu X. J. et al., 2020).
Sample collection and laboratory analysis
We divided the natural forest into nine elevation gradients, two sample lines, and 18 study sites (each with a size of 20 m × 20 m) at 100 m intervals within the elevation distribution range of the natural forest (1,350∼2,150 m, Figure 1). Within each plot, five healthy individuals for each dominant tree species were randomly selected. Fresh leaves were collected in the upper central south-facing part of the crown with high-branch scissors and then mixed and packed into ventilated bags (July 2021). Leaf litters were shaken off from branches in the middle of the trees using a rod and put into ventilated bags (October 2021) (Du et al., 2017). At each site, we used a soil auger (diameter, 5 cm) at depths of 0–30 cm to collect five soil samples and put them into sealed bags. The basic characteristics of the sample plot are shown in Table 1.
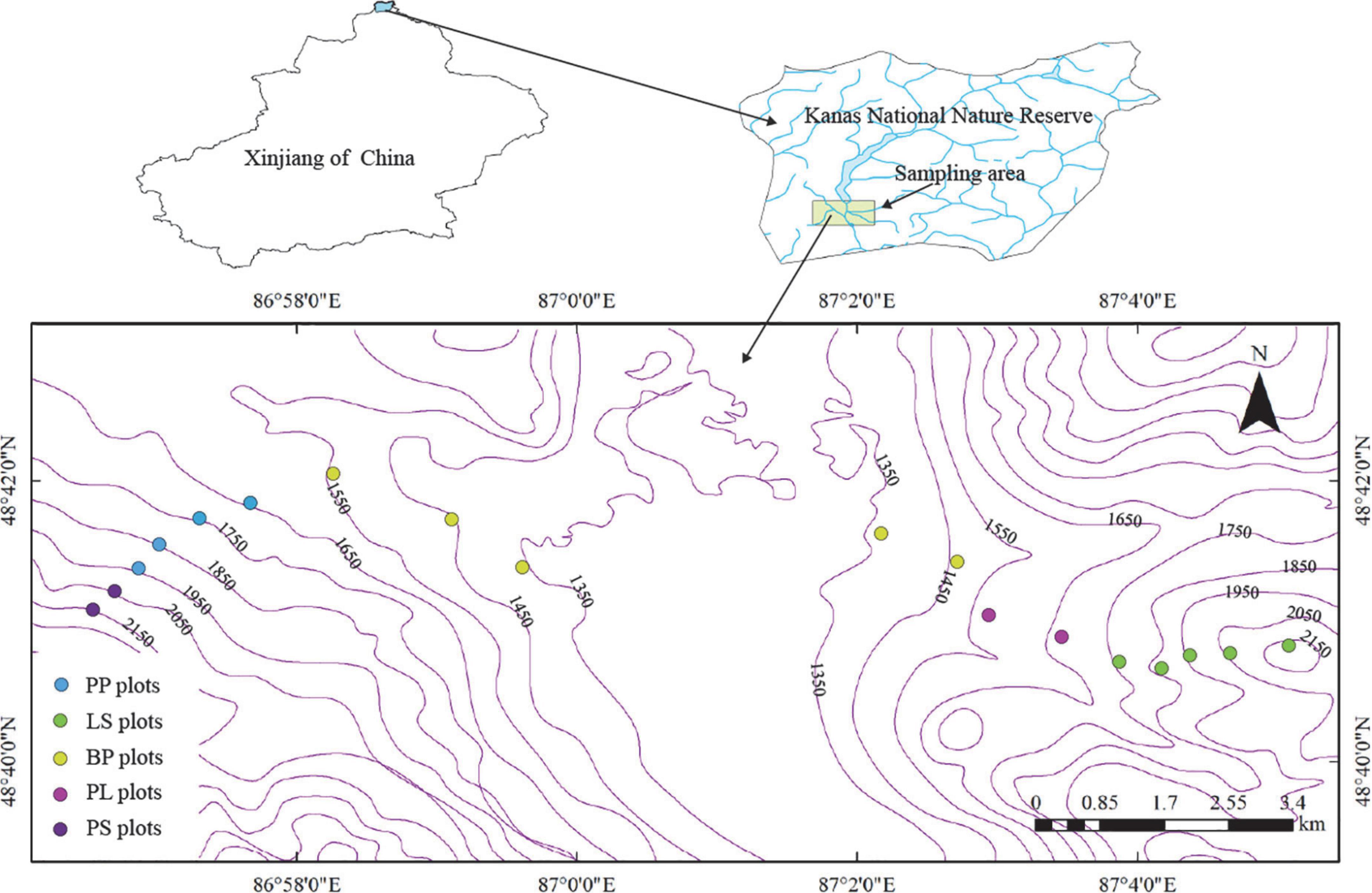
Figure 1. Distribution of sampling site. Betula pendula forest (BP), Picea obovata + Larix sibirica mixed forest (PL), Larix sibirica forest (LS), Picea obovata + Pinus sibirica mixed forest (PP), and Pinus sibirica forest (PS).
We brought all samples back to the lab. The soil samples were naturally air-dried, sieved, and stored for determination. Leaf samples were dried at 105°C for 20 min and then transferred to 70°C for 48 h. The dried samples were grinded and then selected by 80 mesh sieving. C concentrations were measured using the oil bath K2Cr2O7 titration. N concentrations were determined after semi-micro Kjeldahl digestion using a flow injection autoanalyzer. P concentrations were determined by the molybdenum–antimony colorimetric method.
Calculations
(1) We calculated nutrient resorption efficiency by using the nutrient concentrations in the fresh leaves and leaf litters and corrected the calculation by using the mass loss correction factor (MLCF) (Aerts, 1996; Vergutz et al., 2012). We used the following formula.
where CF and CL are nutrient concentrations in fresh leaves and leaf litters, respectively. The MLCF values of broad-leaved and coniferous species are 0.784 and 0.745, respectively (Vergutz et al., 2012).
(2) Plant homeostasis reflects the ability of organisms to maintain their nutrient composition relatively constant irrespective of changing environmental nutrient status (Persson et al., 2010; Yu et al., 2011), which is calculated by the following formula (Wang et al., 2021):
where y is the N and P concentrations (g.kg–1) or N: P ratio for fresh leaves, x is the corresponding value in the soil layer; c is a constant, and H is the homeostatic regulation coefficient. If the regression relationship is insignificant (p > 0.05), then the target organism is strictly homeostatic. If the regression relationship is significant (p < 0.05), then species are considered as not homeostatic, homeostatic, weakly homeostatic, weakly plastic, and plastic with | 1/H| ≥ 1, 0 < | 1/H| <0.25, 0.25 < | 1/H| < 0.5, 0.5 < | 1/H| < 0.75, and | 1/H | > 0.75, respectively (Persson et al., 2010).
Climate data collection
Climate data (MAT) were obtained from the station data deployed at Kanas Ecological Station (87°01′59″E, 48°41′21″N) by Forest Ecology, Xinjiang Academy of Forestry.
Statistical analysis
We used one-way analysis of variance (ANOVA) to analyze the leaves, litters, and soil C, N, and P concentrations and stoichiometric characteristics of different tree species. In testing the homogeneity of variance, the least significant difference (LSD) method was used for multiple comparisons if the square difference is homogeneous. Tamhane’s T2 method was utilized for multiple comparisons if the variance is uneven (α = 0.05). Redundancy analysis of NuRE with leaf and litter N and P concentrations was performed for different tree species. The simple linear regression was performed to uncover the relationship between N and P resorption rates and environmental factors. All data analyses were carried out with SPSS 19.0 and figures were generated via Origin 2021 and Canoco5.
Results
Carbon, nitrogen, and phosphorus stoichiometry of fresh leaves and leaf litters
Picea obovata had the highest and BP had the lowest concentration of C in fresh leaves, and the trend of leaf litter C concentrations remained consistent with that of fresh leaves (Figure 2). The fresh leaf N concentrations in BP and LS were significantly higher than in PO and PS (p < 0.05), and the leaf litter N concentrations in LS were significantly higher than in BP, PO, and PS (p < 0.05). PS had the lowest N concentration in both fresh and withered leaves. BP had the both highest P concentration in fresh leaves and leaf litters, while the lowest fresh leaves P concentrations in PO. PS had the lowest P concentrations in leaf litters. In general, the C, N, and P concentrations in fresh leaves are higher than those in leaf litters.
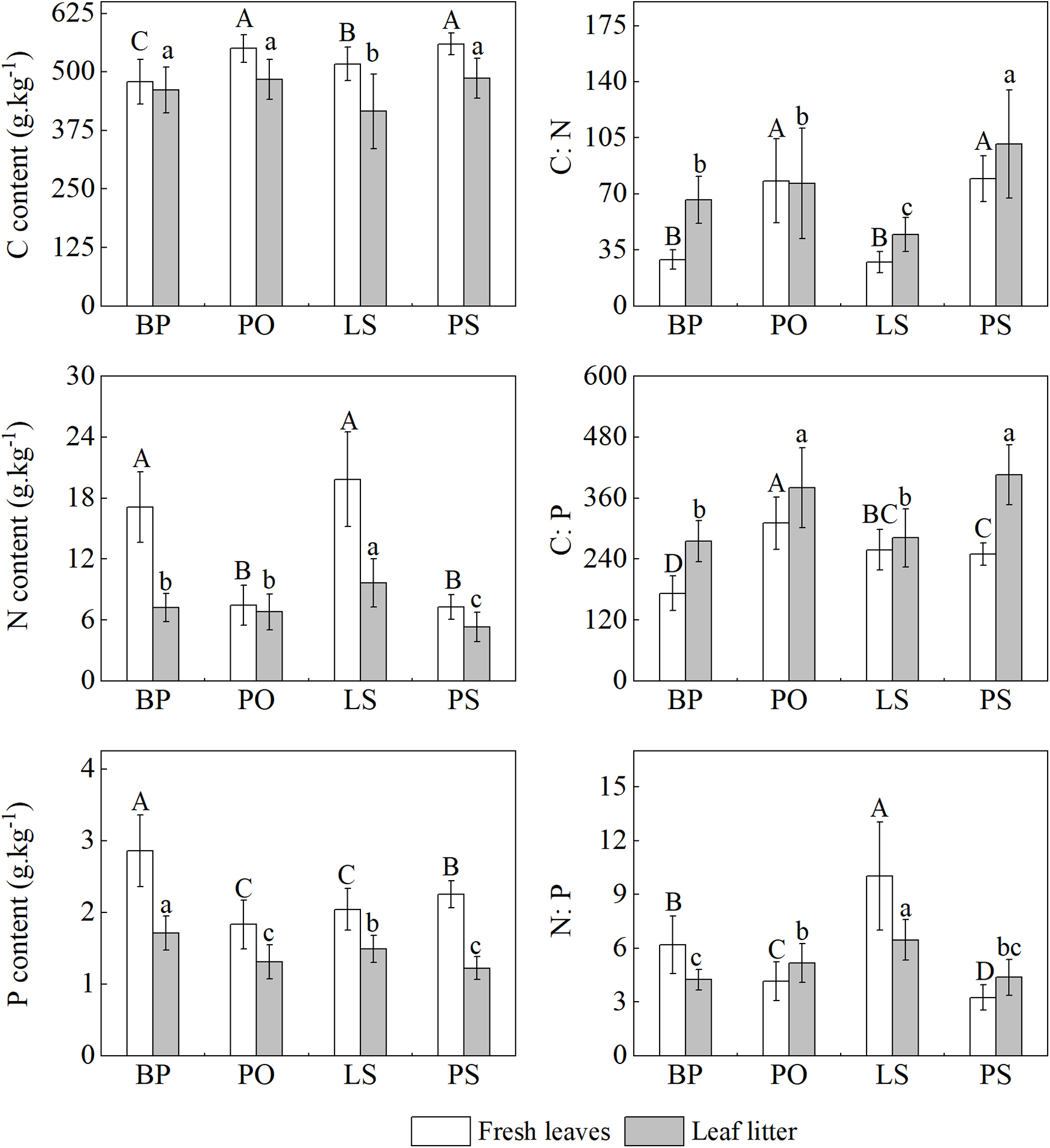
Figure 2. Leaf C, N, and P concentrations and stoichiometry of four tree species. Betula pendula (BP), Picea obovata (PO), Larix sibirica (LS), and Pinus sibirica (PS). Different lowercase letters represent significant differences in fresh leaves of different tree species (p < 0.05). Different capital letters represent significant differences in leaf litters of different tree species (p < 0.05).
We found inconsistent trends in C: N: P ratios of fresh and litter leaves in four tree species. The C: N ratio of fresh leaves showed an order of PS > PO > BP > LS, and C: N ratio in fresh leaves of PS and PO was significantly higher than that of LS and BP (p < 0.05). C: P ratio in fresh leaves showed an order of PO > LS > PS > BP. There was significant variability (p < 0.05) in N: P ratios of fresh leaves, with the trend of LS > BP > PO > PS. The trend of C: N: P of leaf litters was not consistent with that of fresh leaves, except for C: N. The order of C: P ratios in leaf litters was PS > PO > LS > BP, among which there was no significant difference in leaf litter C: P ratios between PS and PO, and between LS and BP (p > 0.05). In addition, the leaf litter N: P ratios were shown as LS > PO > BP > PS.
Nutrient resorption characteristics of different tree species
The mean NRE and PRE of four tree species were 52.55 and 49.16%, respectively (Figure 3). The mean NRE with a value of 62.51% for deciduous trees was significantly higher than evergreen trees with a value of 42.60% (p < 0.05, Figure 3A), while there was no significant difference among life forms in PRE (p > 0.05, Figure 3B). The mean NRE for BP, LS, PO, and PS were 65.93, 61.21, 30.41, and 46.84%, respectively (Figure 3A). The mean PRE for BP, LS, PO, and PS were 52.21, 44.89, 44.38, and 59.85%, respectively (Figure 3B). There was no significant difference in NRE between BP and LS (p > 0.05, Figure 3A). The NRE of PS were significantly higher than PO (p < 0.05, Figure 3A). Furthermore, while there were no significant difference in the PRE in BP and PS (p > 0.05), it was significantly higher in the PO and LS (p < 0.05, Figure 3B). The difference in PRE between PO and LS was insignificant (p > 0.05, Figure 3B).
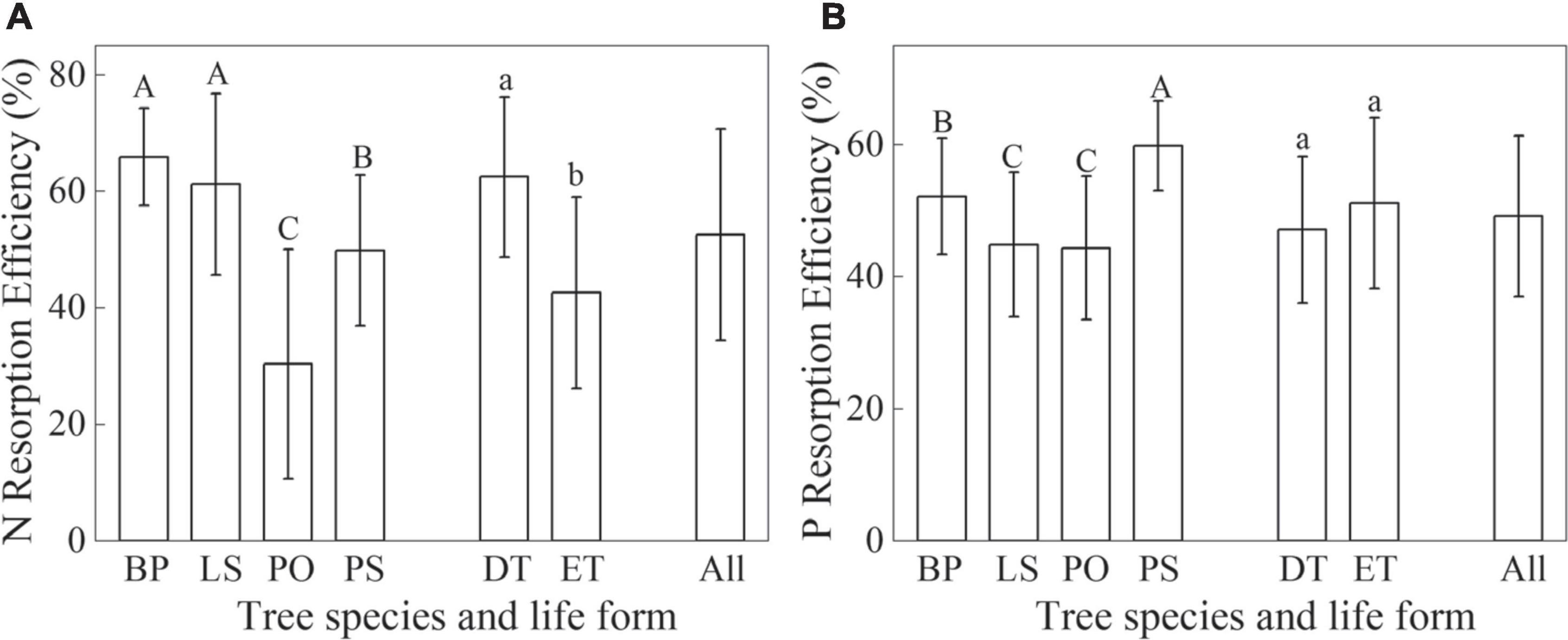
Figure 3. N (A) and P (B) resorption efficiencies of four tree species and different life forms in Kanas Natural Forest. Betula pendula (BP), Picea obovata (PO), Larix sibirica (LS), Pinus sibirica (PS), deciduous trees (DT), and evergreen trees (ET). Different capital and lowercase letters represent significant differences between different tree species and life forms, respectively (p < 0.05).
Correlations among nitrogen and phosphorus resorption efficiencies, nutrient concentrations of leaf, and environmental factors
Fresh leaf N concentrations of BP, PO, and LS were positively correlated with NRE (Figure 4), but this relationship disappeared for PS (Figure 4). PRE of all tree species were positively correlated with P concentrations of fresh leaves. NRE of all tree species were decreased with leaf litter N concentrations. PRE of LS, PO, and PS were decreased with leaf litter P concentrations, while PRE of BP were not related to leaf litter P concentrations. Only PRE of PS were influenced by N: P ratio of fresh leaves (Figure 4). N: P ratios of fresh leaves increased with NRE in LS, BP, and PO (Figure 4). Leaf litter N: P ratios decreased with NRE in PS, LS, and PO (Figure 4). Fresh leaves and leaf litter N: P ratios had no effect on the NRE of PS and BP, respectively (Figure 4).
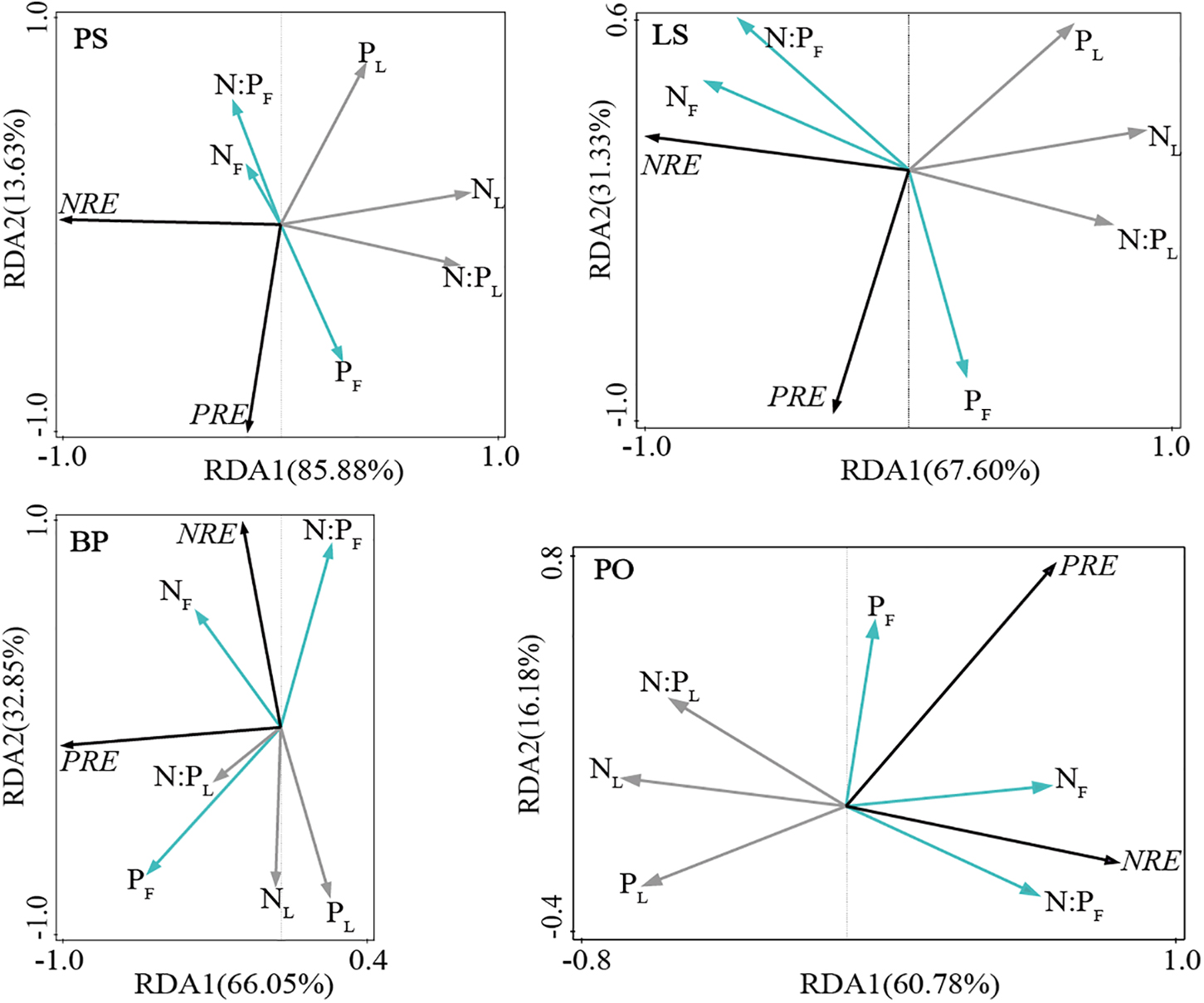
Figure 4. Redundancy analysis of leaf N, P resorption efficiencies and nutrients concentration and its stoichiometry ratios in different tree species. Fresh leaf N concentration (NF), fresh leaf P concentration (PF), fresh leaf N: P ratio (N: PF), leaf litter N concentration (NL), leaf litter P concentration (PL), and leaf litter N: P ratio (N: PL). Betula pendula (BP), Picea obovata (PO), Larix sibirica (LS), Pinus sibirica (PS), N resorption efficiency (NRE), and P resorption efficiency (PRE).
There was no significant relationship between MAT and NuRE (Figure 5I). PRE of BP and PS decreased with soil P concentrations (p < 0.05, Figures 5B,H). The NRE of PS had positive correlations with soil N concentrations (p < 0.05, Figure 5G).
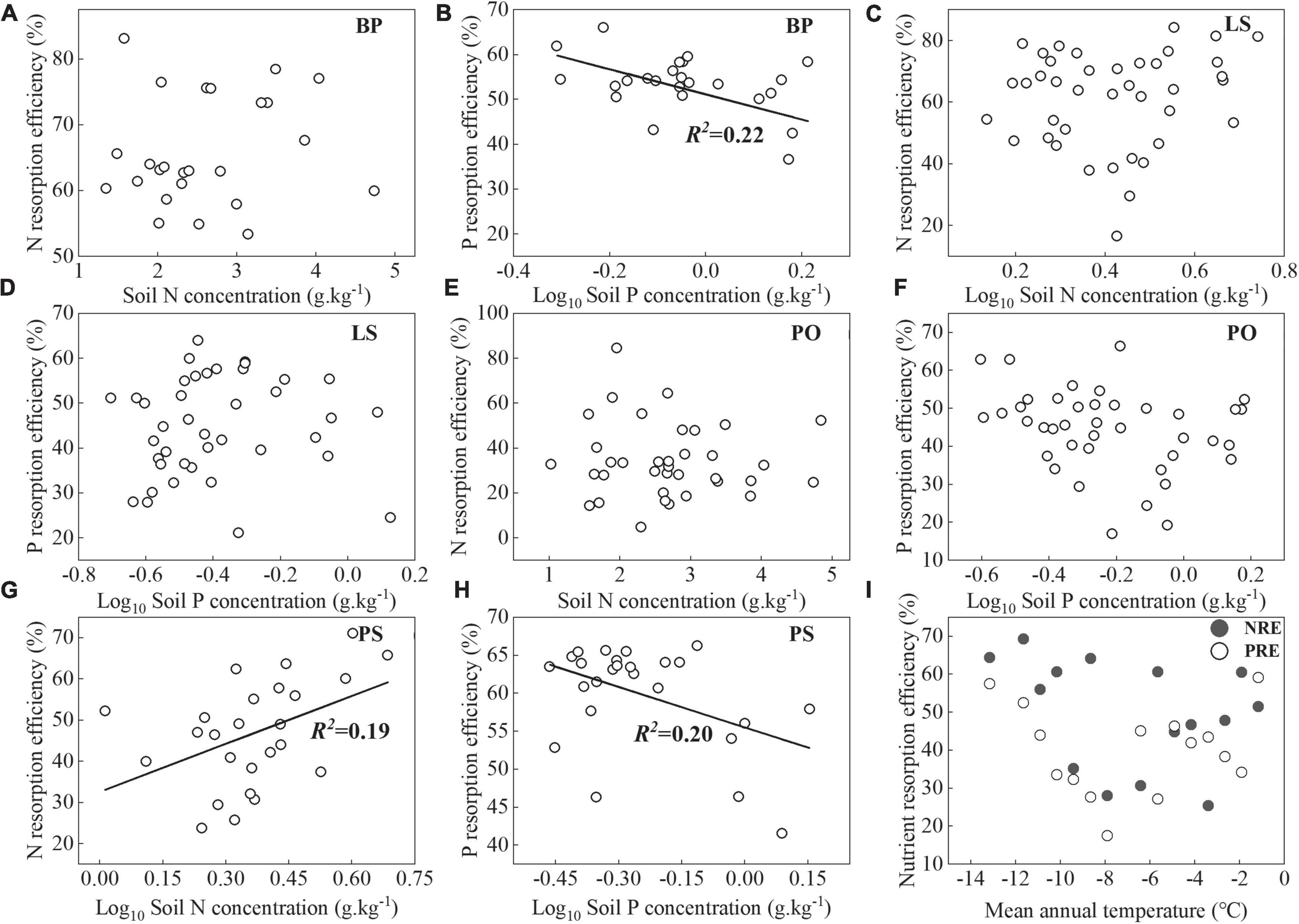
Figure 5. Relationships between N and P resorption efficiencies and MAT (I), and soil N and P concentrations (A–H). Lines were plotted for relationships with p < 0.05.
Stoichiometric homeostasis
Stoichiometric homeostasis of N, P, and N: P in the leaf could be detected as shown in Table 2, only the significant relationships are shown in Figure 6. We found strict homeostasis of N and P concentrations and N: P ratios in BP and LS (p > 0.05, Table 2). In addition, N concentrations and N: P ratios of PO and N concentrations of PS displayed strict homeostasis (p > 0.05, Table 2). However, P concentrations and N: P ratio of PS and P concentrations of PO were homeostatic (p < 0.05, Table 2 and Figure 6).
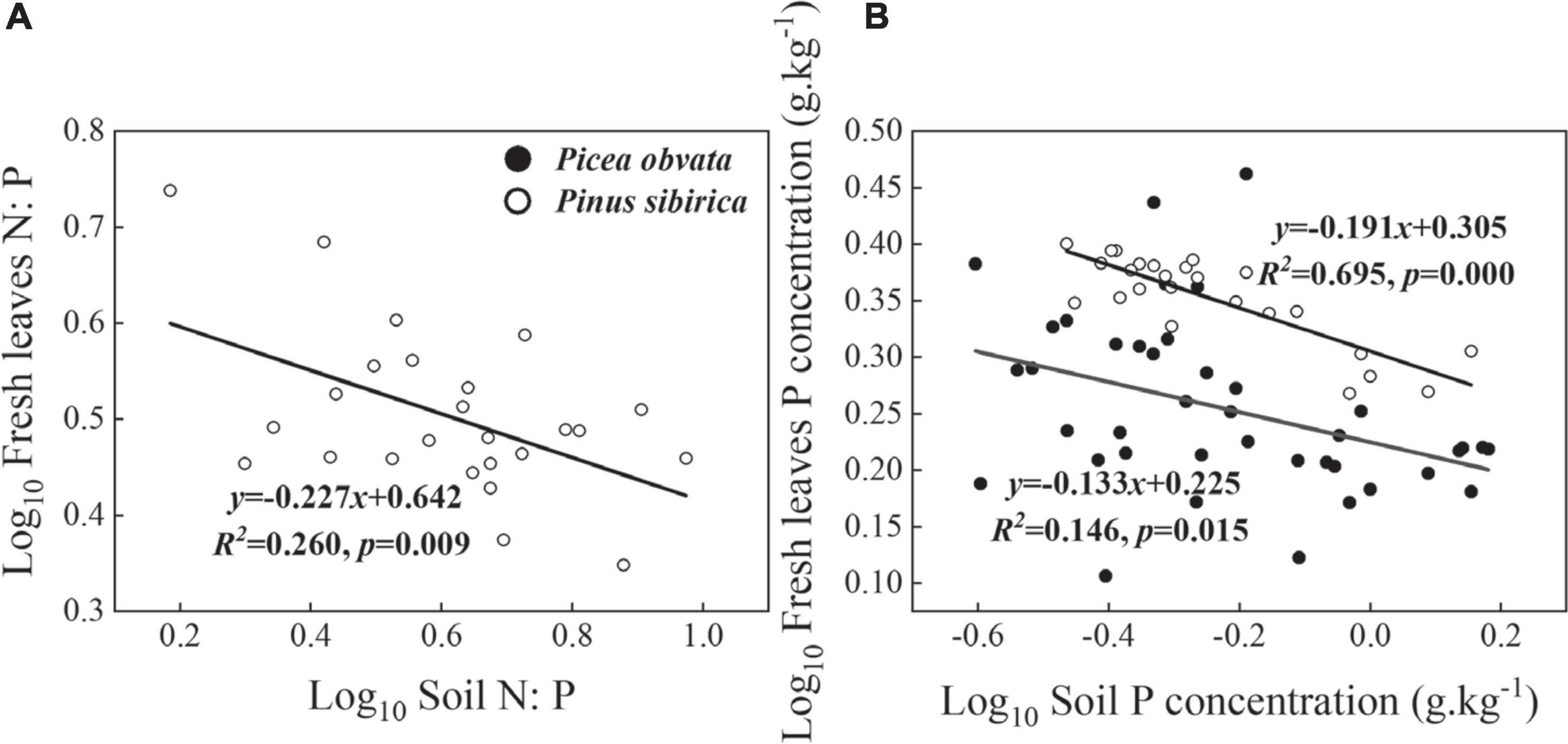
Figure 6. Relationships between fresh leaves and soil P concentrations in PS (A), and N: P in PS (B) and PO.
Discussion
Foliar nutrient concentrations and stoichiometry
C, N, and P are the basic elements that constitute the dry matter, proteins, amino acids, and nucleic acids of plants and play an essential role in various physiological and biochemical processes in plants (Ping et al., 2014). In the present study, we found that mean C, N, and P contents varied from 478.80 to 560.04 g.kg–1, 7.18 to 17.12 g.kg–1, and 1.82 to 2.85 g.kg–1, respectively (Figure 2). The C concentration was higher than the global average of 492 terrestrial plant species (464.20 g.kg–1) (Elser et al., 2000) and close to the mean value of alpine forest in Central Asia (518.65 mg/g) (Zhang et al., 2020), whereas the fresh leaf N concentration in this study was relatively lower compared with that of global and Chinese averages (20.09 g.kg–1 and 18.6 g.kg–1, respectively). The mean P concentration of fresh leaves in our study area was significantly higher than the Chinese average (1.21 g.kg–1) (Han et al., 2005) and karst forest plants in Southwest China (1.20 g.kg–1) (Zeng et al., 2015). This might be due to the fact that: First, the higher fire disturbance of Kanas natural forest may lead to an increase in the effective phosphorus concentration of the soil while reducing the soil N pool, resulting in a rapid decrease in soil inorganic N (Milla et al., 2005; Liu X. J. et al., 2020). Second, plants improve their cold tolerance via lower their cytosol freezing point to adapt to cold environment, while increasing the concentration of cellular P elements can effectively lower the cytosol freezing point and improve the success rate of plants to survive in a cold environment (Yang et al., 2021).
The C: N and C: P ratios of plant leaves are important indicators of carbon absorption ability, reflecting the nutrient usage efficiency of plants and having significant ecological significance (Wang et al., 2011a). In the present study, the C: N ratios of evergreen species were substantially greater than that of deciduous species, while the C: P ratios did not exhibit such a pattern, indicating the higher N utilization efficiency in evergreen trees. Previous studies found that plants were N-limited at N: P < 14, P-limited at N:P > 16, and joint limitation of N and P at 14 ≤ N: P ≤ 16 (Koerselman and Meuleman, 1996). In this study, the N: P ratios of all species were less than 14, indicating a N limitation of growth, which is consistent with the previous findings that plants are more susceptible to N-element limitation at high latitudes (Chapin and Moilanen, 1991).
As an essential part of the biogeochemical cycle in forest ecosystems, the decomposition process of leaf litters is an important way for plant nutrients to be returned to the soil (Zhang et al., 2015). The C, N, and P concentrations of leaf litters in this study were varied from 415.59 to 486.19, 5.19 to 9.67, and 1.22 to 1.70 g.kg–1, respectively. Compared with the global level of C (467.0 g.kg–1), N (10.0 g.kg–1), and P (0.7 g.kg–1) concentrations of leaf litters (Yuan and ChenHan, 2009b), C and P concentrations were higher in this study, and N concentration was lower, showing an overall pattern of low N and high C and P. Because leaf litter decomposition is primarily dependent on microorganisms, the abundance of leaf litter N and P facilitates the activity of microorganisms (particularly bacteria), which in turn promotes the rapid decomposition and nutrient release of leaf litters (Deng et al., 2020). Leaf litter C: N ratio < 40 and C: P ratio < 480 are usually considered the threshold values for favorable decomposition (Parton et al., 2007). In this study, C: N ratios were higher than 40, while C: P ratios were lower than 480 of leaf litters in four tree species (Figure 2), indicating that leaf litter decomposition was N-limited.
NuRE characteristics
Plant NuRE reflects the ability of plants to store and reuse nutrients and adapt to poor soil conditions (An et al., 2011). The average NRE of 52.55% and PRE of 49.16% (Figure 3) for four species in our study area were lower than the average values of woody plants in Mt. Dongling of Beijing, North China (NRE, 57.4% and PRE, 61.4%) (Zhang et al., 2015) and terrestrial plants of global (NRE, 62.10% and PRE, 64.90%) (Vergutz et al., 2012), but close to woody plants of Eastern China (NRE, 49.00% and PRE, 51.00%) (Tang et al., 2013) and global level (both∼ 51%) (Aerts, 1996). The difference in species composition from a specific habitat may result in a difference in NuRE deviation from the general patterns observed at a large scale (Vergutz et al., 2012). In addition, we found that the PRE in evergreen species (mean value of 47.13%) were not significantly (p > 0.05) different from deciduous species (mean value of 51.19 %), while the NRE of deciduous species (mean value of 52.57 %) were significantly (p < 0.05) higher than evergreen species (mean value of 42.60 %), which is in line with the results reported previously (Figure 4; Tang et al., 2013; Pi et al., 2016).
Previous studies varied with the relationship between plant leaf nutrient concentration and NuRE (An et al., 2005; Kobe et al., 2005; Vergutz et al., 2012; Zeng et al., 2017). In the present study, only PRE of BP and NRE of PS were not affected by leaf litter P concentration and fresh leaf N concentration, respectively (Figure 4). The rest of the NRE and PRE of four tree species were positively correlated with corresponding fresh leaves N and P concentrations and negatively correlated with leaf litter N and P concentrations (Figure 4). These results were in line with our second hypothesis and similar to the results reported elsewhere (Wang et al., 2019). The effect of leaf N and P concentrations on NuRE and N: P ratios necessarily makes some correlation between the latter two (Wang et al., 2019). In this study, N: P ratios of fresh leaves increased with NRE of LS, BP, and PO (Figure 4) and leaf litter N: P ratios decreased with NRE of PS, LS, and PO (Figure 4). In addition, PRE had no effect on N :P ratios of fresh leaves and leaf litters in BP, LS, and PO (Figure 4). The increase of NRE will make the residence time of N concentration in plant leaves longer (Aerts, 1996), thus increasing the N concentration of fresh leaves but causing a decrease in the N concentration of leaf litters, which in turn makes the N: P ratio of fresh leaves higher and finally leads to a positive correlation between NRE and N: P ratio of fresh leaves and a negative correlation with leaf litter N: P ratio.
NuRE may also be influenced by soil conditions (Killingbeck, 1996), climate, and climate change, either separately or in combination (Luyssaert et al., 2005; Vergutz et al., 2012). Inconsistent with the results of Vergutz et al. (2012) and Tang et al. (2013) and our third hypothesis, MAT had no effect on NuRE (p > 0.05, Figure 5) in the present study. This may be due to the small difference in climatic conditions across the study area and the limited variety of tree species in the Kanas natural forest. The MAP in our study area exceeds 1,065 mm (Liu et al., 2016), and soil water resources are not a limiting factor for the growth of tree species in this study. It is widely acknowledged that NuRE is determined by the relative cost the plants absorb nutrients from the leaf litters vs. soil, but not the absolute levels of soil nutrients (Wright and Westoby, 2003). In nutrient-rich soils, the energy cost for plants to absorb nutrients from soils was lower than that to obtain by resorption from leaf litters (Wright and Westoby, 2003). Contrary to the results of Zhang et al. (2015) but similar to the studies of Tang et al. (2013), we observed that both PRE of BP and PS decreased with soil P concentration (Figures 5B,H). Soil P concentration is relatively constant and mainly originates from the weathering of rocks (Wang et al., 2011b) and it had no correlation with leaf litter P concentration of BP and PS (Figures 7A,B). Along with the increase in soil P concentration, PS and BP were biased to take up P nutrients from the soil and reduced their PRE; thus, the soil P content was negatively correlated with PRE. The NRE increased with soil N concentration (Figure 5G), but decreased with leaf litter N concentration (Figure 4), and soil N concentration decreased with leaf litter N concentration (Figure 7C), suggesting that PS reduced its consumption of soil N concentration by elevating NRE and soil N concentration that were not primarily derived from the decomposition of leaf litters of PS. These results also suggest that plants tend to maintain a high NRE in this N-limiting soil (Han et al., 2013) and agreed with the fact that leaf litter decomposition was N-limited in this study.
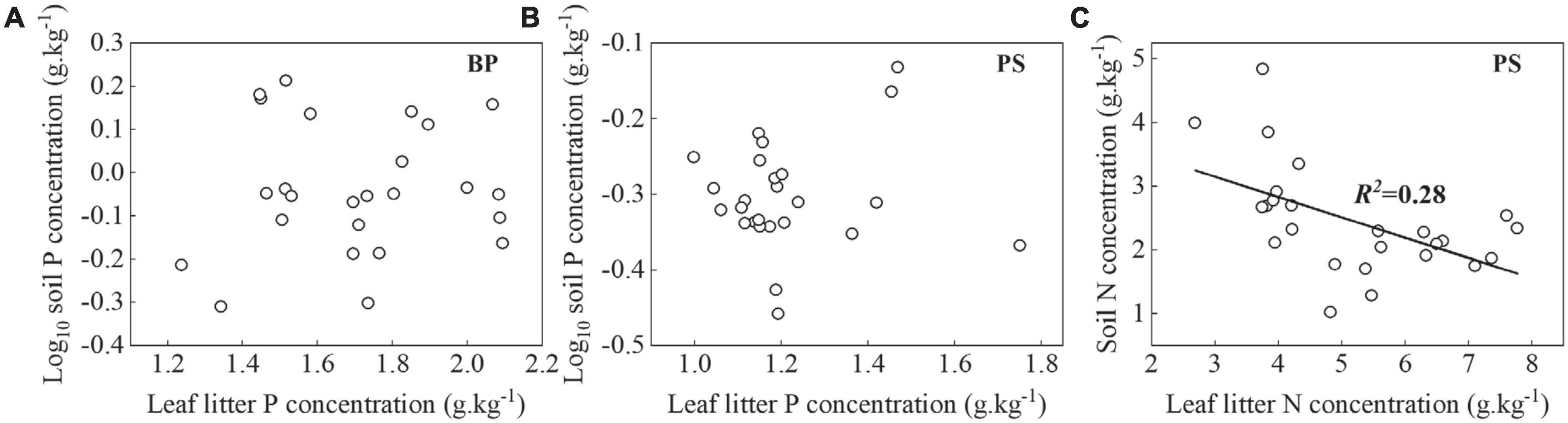
Figure 7. Relationships between leaf litter P concentration of BP and PS (A,B) and leaf litter N concentration of PS (C) and soil P and N concentrations, respectively. Lines were plotted for relationships with p < 0.05.
Homeostatic characteristics
Stoichiometric homeostasis reflects the balance between resource consumption and storage in plants during the growth period, and it is positively correlated with vegetation stability (Yu et al., 2010). In this study, fresh leaf N, P concentrations and N: P ratios of deciduous trees showed strict homeostasis, whereas the degree of P concentration in PS and PO and N: P ratio in PS was homeostatic (Table 2 and Figure 6). In PS, the N concentration was strictly homeostatic, while the degree of the N: P ratio was homeostatic. It might be due to the degree of P concentration in PS being homeostatic, which made the N: P ratios vary with the environment. The N concentrations of four tree species were strictly homeostatic, probably because N was a limiting element in this region, which was consistent with the Stability of Limiting Elements Hypothesis (Han et al., 2011). In terms of P concentration, the homeostasis of evergreen trees was lower than that of deciduous trees, which is contrary to our fourth hypothesis. Furthermore, it was reported that dominant species had higher homeostatic levels than other species, (Yu et al., 2010, 2015), since BP and LS were dominant species in Kanas our study area, they might be more conservative in their nutrient utilization patterns, thus more easily adapting to the barren environment and becoming dominant.
Conclusion
Our study suggested that stoichiometric characteristics, nutrient resorption patterns, and stoichiometric homeostasis varied among four tree species. First, evergreen species (PO, PS) had higher fresh leaf C concentrations and C: N ratios. As opposed to evergreen plants, deciduous species (BP, LS) have higher levels of fresh leaf N concentration and NRE, while the P concentration, C: P ratio, and PRE did not exhibit such a pattern. Overall, 52.55% of N and 49.16% of P were resorbed before senescence. Furthermore, the N: P ratio of four species was below 14, indicating a general N limitation. Second, NRE increased with N concentration but decreased with the N: P ratio in fresh leaves of BP, PO, and LS, respectively, NRE decreased with the N concentration of leaf litters in all species but increased with the N: P ratio of leaf litters in PS, LS, and PO; PRE increased with the P concentration of fresh leaves in all species but decreased with the P concentration of leaf litters in LS, PO, and PS. Only PRE decreased with the N: P ratio of fresh leaves in PS. In addition, the NRE of BP and PS decreased with soil N concentration and PRE of PS increased with soil P concentration. MAT had no effect on NRE and PRE in this study. Third, we found that only fresh leaf P concentration of PO and PS, and N: P ratio of PS varied with corresponding traits in soil. Our findings are essential for understanding nutrient cycling among forest components (plant leaves, litters, and soil) and plant adaptation strategies in Taiga forest ecosystems.
Data availability statement
The original contributions presented in the study are included in the article/supplementary material, further inquiries can be directed to the corresponding authors.
Author contributions
B and ZX conceived and designed the study. B and GX performed the experiments. B and TL wrote the manuscript. B and YW reviewed and edited the manuscript. All authors read and approved the manuscript.
Funding
This research was funded by the SCO Science and Technology Partnership Program and International Science and Technology Cooperation Program supported by the Xinjiang Province (No. 2022E01052).
Conflict of interest
The authors declare that the research was conducted in the absence of any commercial or financial relationships that could be construed as a potential conflict of interest.
Publisher’s note
All claims expressed in this article are solely those of the authors and do not necessarily represent those of their affiliated organizations, or those of the publisher, the editors and the reviewers. Any product that may be evaluated in this article, or claim that may be made by its manufacturer, is not guaranteed or endorsed by the publisher.
References
Aerts, R. (1995). The advantages of being evergreen. Trend Ecol. Evol. 10, 402–407. doi: 10.1016/S0169-5347(00)89156-9
Aerts, R. (1996). Nutrient resorption from senescing leaves of perennials: Are there general patterns? J. Ecol. 84, 597–608. doi: 10.2307/2261481
An, Y., Wan, S. Q., Zhou, X. H., Subedar, A. A., Wallace, L. L., and Luo, Y. Q. (2005). Plant nitrogen concentration, use efficiency, and contents in a tallgrass prairie ecosystem under experimental warming. Global Change Biol. 11, 1733–1744. doi: 10.1111/j.1365-2486.2005.01030.x
An, Z., Niu, D. C., Wen, H. Y., Yang, Y., Zhang, H. R., and Fu, H. (2011). Effects of N addition on nutrient resorption efficiency and C:N:P stoichiometric characteris-tics in Stipa bungeana of steppe grasslands in the Loess Plateau, China. Chinese J. Plant Ecol. 35, 801–807. doi: 10.3724/sp.J.1258.2011.00801
Bai, K. D., Mo, L., Liu, M., Zhang, D. N., He, C. X., Wan, X. C., et al. (2015). Nutrient resorption patterns of evergreen and deciduous tree species at different altitudes on Mao’re mountain, Guangxi. Acta Ecol. Sin. 35, 5776–5787. doi: 10.5846/stxb201312092914
Blanco, J. A., Imbert, J. B., and Castillo, F. J. (2009). Thinning affects nutrient resorption and nutrient-use efficiency in two pinus sylvestris stands in the Pyrenees. Ecol. Appl. 19, 682–698. doi: 10.1890/1051-0761-19.3.682
Brant, A. N., and Chen, H. Y. (2015). Patterns and mechanisms of nutrient resorption in plants. Crit. Rev. Plant Sci. 34, 471–486. doi: 10.1080/07352689.2015.1078611
Chapin, F. S. III, and Moilanen, L. (1991). Nutritional controls over nitrogen and phosphorus resorption from Alaskan birch leaves. Ecology 72, 709–715. doi: 10.2307/2937210
Deng, J., Chong, Y. J., Jia, X. M., Jiao, Z. Y., Ren, C. J., Han, X. H., et al. (2020). Leaf N and P resorption characteristics of typical tree species in the Ziwuling forest area on Loess Plateau. Acta Ecol. Sin. 40, 3698–3705. doi: 10.5846/stxb201906201294
Deng, J., Zhang, D., Zhang, W., Ren, C. J., Hao, W. H., Liu, C., et al. (2019). Carbon, nitrogen, and phosphorus stoichiometry and homeostasis characteristicsof leaves, soil, and microbial biomass of Robinia pseudoacacia forests in the LoessHilly Region of China. Acta Ecol. Sin. 39, 5527–5535. doi: 10.5846/stxb201808281845
Du, B. M., Ji, H. W., Peng, C., Liu, X. J., and Liu, C. J. (2017). Altitudinal patterns of leaf stoichiometry and nutrient resorption in Quercus variabilis in the Baotianman Mountains, China. Plant Soil 413, 193–202. doi: 10.1007/s11104-016-3093-9
Elser, J. J., Fagan, W. F., Kerkhoff, A. J., Swenson, N. G., and Enquist, B. J. (2010). Biological stoichiometry of plant production: Metabolism, scaling and ecological response to global change. New Phytol. 186, 593–608. doi: 10.1111/j.1469-8137.2010.03214.x
Elser, J. J., Sterner, R. W., Gorokhova, E., Fagan, W. F., Markow, T. A., Cotner, J. B., et al. (2000). Biological stoichiometry from genes to ecosystems. Ecol. Lett. 3, 540–550. doi: 10.1111/j.1461-0248.2000.00185.x
Givnish, T. J. (2002). Adaptive significance of evergreen vs. Deciduous leaves: Solving the triple paradox. Silva Fenn. 36, 703–743. doi: 10.14214/sf.535
Han, W. X., Fang, J. Y., Guo, D. L., and Zhang, Y. (2005). Leaf nitrogen and phosphorus stoichiometry across 753 terrestrial plant species in China. New Phytol. 168, 377–385. doi: 10.1111/j.1469-8137.2005.01530.x
Han, W. X., Fang, J. Y., Reich, P. B., Ian Woodward, F., and Wang, Z. H. (2011). Biogeography and variability of eleven mineral elements in plant leaves across gradients of climate, soil and plant functional type in China. Ecol. Lett. 14, 788–796. doi: 10.1111/j.1461-0248.2011.01641.x
Han, W. X., Tang, L. Y., Chen, Y. H., and Fang, J. Y. (2013). Relationship between the relative limitation and resorption efficiency of nitrogen vs phosphorus in woody plants. PloS One 8:e83366. doi: 10.1371/journal.pone.0083366
Hessen, D. O., Ågren, G. I., Anderson, T. R., Elser, J. J., and De Ruiter, P. C. (2004). Carbon sequestration in ecosystems: The role of stoichiometry. Ecology 85, 1179–1192. doi: 10.1890/02-0251
Killingbeck, K. T. (1996). Nutrients in senesced leaves: Keys to the search for potential resorption and resorption proficiency. Ecology 77, 1716–1727. doi: 10.2307/2265777
Kobe, R. K., Lepczyk, C. A., and Lyer, M. (2005). Resorption efficiency decreases with increasing green leaf nutrients in a global data set. Ecology 86, 2780–2792. doi: 10.1890/04-1830
Koerselman, W., and Meuleman, A. F. M. (1996). The vegetation N: P ratio: A new tool to detect the nature of nutrient limitation. J. Appl. Ecol. 33, 1441–1450. doi: 10.2307/2404783
Lal, C. B., Annapurna, C., Raghubanshi, A. S., and Singh, J. S. (2001). Effect of leaf habit and soil type on nutrient resorption and conservation in woody species of a dry tropical environment. Can. J. Bot. 79, 1066–1075. doi: 10.1139/b01-077
Liu, C. C., Liu, Y. G., Guo, K., Wang, S. J., and Yang, Y. (2014). Concentrations and resorption patterns of 13 nutrients in different plant functional types in the karst region of south-western China. Ann. Bot. 113, 873–885. doi: 10.1093/aob/mcu005
Liu, H., She, C. Y., Bai, Z. Q., Li, Q., Liu, D., and Han, Y. L. (2016). Dynamic changes of trunk sap flow of picea obovata in the Kanas National Reserve. J. Nanjing For. Univ. 40, 65–72. doi: 10.3969/j.issn.1000-2006.2016.01.011
Liu, X. J., Shan, Q., and Li, Y. Y. (2020). Leaf carbon, nitrogen and phosphorus stoichiometry in 72 understory plants in Kanas taiga. Ecol. Env. Sci. 29, 1302–1309. doi: 10.16258/j.cnki.1674-5906.2020.07.003
Liu, X., Wang, Z., Li, X. M., Rousk, K., and Bao, W. K. (2020). High nitrogen resorption efficiency of forest mosses. Annals of Botany 125, 557–563. doi: 10.1093/aob/mcz199
Luyssaert, S., Staelens, J., and De Schrijver, A. (2005). Does the commonly used estimator of nutrient resorption in tree foliage actually measure what it claims to? Oecologia 144, 177–186. doi: 10.1007/s00442-005-0085-5
Milla, R., Castro-Díez, P., Maestro-Martínez, M., and Montserrat-Martí, G. (2005). Does the gradualness of leaf shedding govern nutrient resorption from senescing leaves in mediterranean woody plants? Plant Soil 278, 303–313. doi: 10.1007/s11104-005-8770-z
Parton, W., Silver, W. L., Burke, I. C., Grassens, L., Harmon, M. E., Currie, W. S., et al. (2007). Global-scale similarities in nitrogen release patterns during long-term decomposition. Science 315, 361–364. doi: 10.1126/science.1134853
Persson, J., Fink, P., Goto, A., Hood, J. M., Jonas, J., and Kato, S. (2010). To be or not to be what you eat: Regulation of stoichiometric homeostasis among autotrophs and heterotrophs. Oikos 119, 741–751. doi: 10.1111/j.1600-0706.2009.18545.x
Pi, F. J., Yuan, C. J., Yu, L. F., Yan, L. B., Wu, L., and Yang, R. (2016). Ecological stoichiometry characteristics of plant leaves from the main dominant species of natural secondary forest in the central of Guizhou. Ecol. Env. Sci. 25, 801–807. doi: 10.16258/j.cnki.1674-5906.2016.05.011
Ping, C., Wang, C. K., and Quan, X. K. (2014). Influence of environmental changes on stoichiometric traits of nitrogen and phosphorus for Larix gmelinii trees. Acta Ecol. Sin. 34, 1965–1974. doi: 10.5846/stxb201306301805
See, C. R., Yanai, R. D., Fisk, M. C., Vadeboncoeur, M. A., Quintero, B. A., and Fahey, T. J. (2015). Soil nitrogen affects phosphorus recycling: Foliar resorption and plant–soil feedbacks in a northern hardwood forest. Ecology 96, 2488–2498. doi: 10.1890/15-0188.1
Seidel, F., Lopez, C. M. L., Celi, L., Bonifacio, E., Oikawa, A., and Yamanaka, T. (2019). N lsotope fractionation in tree tissues during N reabsorption and remobilization in Fagus crenata blume. Forests 10:330. doi: 10.3390/f10040330
Tang, L. Y., Han, W. X., Chen, Y. H., and Fang, J. Y. (2013). Resorption proficiency and efficiency of leaf nutrients in woody plants in eastern China. J. Plant Ecol. 6, 408–417. doi: 10.1093/jpe/rtt013
Tully, K. L., Wood, T. E., Schwantes, A. M., and Lawrence, D. (2013). Soil nutrient availability and reproductive effort drive patterns in nutrient resorption in Pentaclethra macroloba. Ecology 94, 930–940.
Vergutz, L., Manzoni, S., Porporato, A., Novais, R. F., and Jackson, R. B. (2012). Global resorption efficiencies and concentrations of carbon and nutrients in leaves of terrestrial plants. Ecol. Monogr. 82, 205–220. doi: 10.1890/11-0416.1
Wang, J. Y., Wang, S. Q., Li, R. L., Yan, J. H., Sha, L. Q., and Han, S. J. (2011a). C : N : P stoichiometric characteristics of four forest types’ dominant tree species in China. Chinese J. Plant Ecol. 35, 587–595. doi: 10.3724/SP.J.1258.2011.00587
Wang, K., Zhang, R. S., Song, L. N., Yan, T., and Na, E. H. (2021). Comparison of C: N: P stoichiometry in the plant–litter–soil system between poplar and elm plantations in the Horqin sandy land, China. Front. Plant Sci. 12:655517. doi: 10.3389/fpls.2021.655517
Wang, W. J., Qiu, L., Zu, Y. G., Su, D. X., An, J., Wang, H. Y., et al. (2011b). Changes in soil organic carbon, nitrogen, pH and bulk density with the development of larch (Larix gmelinii) plantations in China. Global Change Biol. 17, 2657–2676. doi: 10.1111/j.1365-2486.2011.02447.x
Wang, Z. N., Zhao, M., Yang, Y., Li, F. K., Wang, H., and Lv, S. J. (2019). Relationships between alfalfa leaf nutrient resorption and stoichiometric ratios of nitrogen, phosphorus, and potassium. Acta Pratacuiturae Sin. 28, 177–183. doi: 10.11686/cyxb2018750
Wang, Z. Q., Fan, Z. X., Zhao, Q., Wang, M. C., Ran, J. Z., Huang, H., et al. (2018). Global data analysis shows that soil nutrient levels dominate foliar nutrient resorption efficiency in herbaceous species. Front. Plant Sci 9:1431. doi: 10.3389/fpls.2018.01431
Wang, Z. Q., Gong, H. Y., Sardans, S., Zhou, Q. P., Deng, J. M., Niklas, K. J., et al. (2022). Divergent nitrogen and phosphorus allocation strategies in terrestrial plant leaves and fine roots: A global meta-analysis. J. Ecol. 1–17. doi: 10.1111/1365-2745.13985
Wright, I. J., and Westoby, M. (2003). Nutrient concentration, resorption and lifespan: Leaf traits of Australian sclerophyll species. Funct. Ecol. 17, 10–19. doi: 10.1046/j.1365-2435.2003.00694.x
Yang, H., Liu, W. J., Liu, H. M., and Cao, L. H. (2021). Nutrient contents and stoichiometric characteristics of plant leaf-litter-soil in alpine forest. J. Zhejiang Univ. 47, 607–618. doi: 10.3785/j.issn.1008-9209.2020.10.261
Yu, Q., Chen, Q. S., Elser, J. J., He, N. P., Wu, H. H., Zhang, G. M., et al. (2010). Linking stoichiometric homoeostasis with ecosystem structure, functioning and stability. Ecol. Lett. 13, 1390–1399. doi: 10.1111/j.1461-0248.2010.01532.x
Yu, Q., Elser, J. J., He, N. P., Wu, H. H., Chen, Q. S., Zhang, G. M., et al. (2011). Stoichiometric homeostasis of vascular plants in the inner Mongolia grassland. Oecologia 166, 1–10. doi: 10.1007/s00442-010-1902-z
Yu, Q., Wilcox, K., Pierre, K. L., Knapp, A. K., Han, X. G., and Smith, M. D. (2015). Stoichiometric homeostasis predicts plant species dominance, temporal stability, and responses to global change. Ecology 96, 2328–2335. doi: 10.1890/14-1897.1
Yuan, Z. Y., and ChenHan, Y. H. (2009a). Global-scale patterns of nutrient resorption associated with latitude, temperature and precipitation. Global Ecol. Biogeogr. 18, 11–18.
Yuan, Z. Y., and ChenHan, Y. H. (2009b). Global trends in senesced-leaf nitrogen and phosphorus. Global Ecol. Biogeogr. 18, 532–542. doi: 10.1111/j.1466-8238.2009.00474.x
Zeng, Y. L., Fang, X., Xiang, W. H., Deng, X. W., and Peng, C. H. (2017). Stoichiometric and nutrient resorption characteristics of dominant tree species in subtropical Chinese forests. Ecol. Evol. 7, 11033–11043. doi: 10.1002/ece3.3527
Zeng, Z. X., Wang, K. L., Liu, X. L., Zeng, F. P., Song, T. Q., Peng, W. X., et al. (2015). Stoichiometric characteristics of plants, litter and soils in karst plant communities of North-west Guangxi. Chinese J. Plant Ecol. 39, 682–693. doi: 10.17521/cjpe.2015.0065
Zhang, J. H., Tang, Z. Y., Luo, Y. K., Chi, X. L., Chen, Y. H., Fang, J. Y., et al. (2015). Resorption efficiency of leaf nutrients in woody plants on Mt. Dongling of Beijing, North China. J. Plant Ecol. 8, 530–538. doi: 10.1093/jpe/rtu042
Zhang, Y. J., Wang, K. Q., Song, Y. L., Su, M. B., Li, X. L., and Li, X. F. (2020). C, N, and P stoichiometric characteristics of leaf, litter and soil for subalpine forests in central Yunnan, China. Acta Ecol. Sin. 40, 7648–7658. doi: 10.5846/stxb201908301806
Zhou, L. L., Addo-Danso, S. D., Wu, P. F., Li, S. B., Zou, X. H., Zhang, Y., et al. (2016). Leaf resorption efficiency in relation to foliar and soil nutrient concentrations and stoichiometry of Cunninghamia lanceolata with stand development in southern China. J. Soils Sediments 16, 1448–1459. doi: 10.1007/s11368-016-1352-2
Keywords: ecological stoichiometry, Kanas natural forest, nutrient resorption rate, stoichiometric homeostasis, life form
Citation: Bagedeng, Xia G, Lin T, Xu Z and Wang Y (2022) Analysis of nutrient resorption efficiency and homeostasis of four tree species in Kanas natural forest, Xinjiang, China. Front. Ecol. Evol. 10:1046444. doi: 10.3389/fevo.2022.1046444
Received: 16 September 2022; Accepted: 04 October 2022;
Published: 14 November 2022.
Edited by:
Zhiqiang Wang, Southwest Minzu University, ChinaReviewed by:
Peng Shouzhang, Northwest A&F University, ChinaHuanhua Peng, Hunan University of Science and Technology, China
Copyright © 2022 Bagedeng, Xia, Lin, Xu and Wang. This is an open-access article distributed under the terms of the Creative Commons Attribution License (CC BY). The use, distribution or reproduction in other forums is permitted, provided the original author(s) and the copyright owner(s) are credited and that the original publication in this journal is cited, in accordance with accepted academic practice. No use, distribution or reproduction is permitted which does not comply with these terms.
*Correspondence: Zhonglin Xu, zlxu@xju.edu.cn; Yao Wang, wangyao@idm.cn
†These authors share first authorship