- 1School of Earth and Environmental Sciences, University of Manchester, Manchester, United Kingdom
- 2Department of Zoology/Ethology, Stockholm University, Stockholm, Sweden
- 3Department of Psychology, Neuroscience & Behaviour, McMaster University, Hamilton, ON, Canada
Although several hypotheses have been proposed to explain the emergence of social monogamy, its origin is still intensely debated. Monogamy has many potential drivers, but evolutionary causality among them remains unclear. Using phylogenetic comparative methods within a Bayesian framework we explored the evolution of monogamy in cichlids and in marine reef fishes because, while both groups are characterised by unusually high incidence of social monogamy, they face very different ecological challenges. For each group, we examined four classic hypotheses that explain the evolution of monogamy: female dispersal, male mate guarding, female–female intolerance, and the biparental care hypotheses. We also explored whether the ecological traits of diet and shelter use are evolutionarily coupled with these hypotheses or with monogamy. First, we found that the evolution of monogamy was predicted by male territoriality in cichlids and simultaneous male and female territoriality in marine reef fishes. We suggest that these results provide support for the male mate guarding hypothesis in cichlids and female–female intolerance hypothesis in marine reef fishes. Second, we demonstrate clear evidence against the biparental care hypothesis, as biparental care was a consequence, not a cause, of monogamy in our analyses. Third, as female dispersal drove the loss of monogamy in both cichlids and marine reef fishes, this suggests the female dispersal hypothesis is not driving the evolution of monogamy in either group. These findings in two highly-monogamous fish taxa largely support prior findings from primate and bird comparative studies and provide novel large-scale evidence for a link between mate guarding and the evolution of monogamy.
Introduction
By definition, males have smaller gametes than females. An important consequence of this fundamental difference in gametic investment between the sexes (i.e., anisogamy) is that viable eggs are generally the limiting factor dictating maximum reproductive rates across species (Bateman, 1948; Trivers, 1972; Hayward and Gillooly, 2011; Schärer et al., 2012; Collet et al., 2014). Thus, female reproductive success is typically limited by the number of viable eggs she can produce, whereas male reproductive success is limited by the number of receptive females he can access. While not universal (Schlupp, 2021), these alternative limitations on reproductive success between the sexes commonly manifest in greater intra-sexual competition and stronger sexual selection on males than females (Janicke et al., 2016). The evolution of monogamous mating systems therefore becomes a puzzle, as males that mate with only one female reduce their potential reproductive output (Bateman, 1948; Trivers, 1972; Kappeler, 2014). Yet social monogamy is found across a wide variety of animal taxa and is even the majority strategy among birds, some groups of fishes, and particular groups of primates (Griffith et al., 2002; Whiteman and Côté, 2004; Opie et al., 2013).
One reason for the continuous debate and lack of consensus about the origins of monogamy across vertebrates is that many different life history factors have been proposed as drivers of monogamy, with primarily parental care mode, adult dispersal patterns, and territoriality considered the main selective factors (Whiteman and Côté, 2004; Dixson, 2013; Kappeler, 2013, 2014; Lukas and Clutton-Brock, 2013; Mabry et al., 2013; Opie et al., 2013; Klug, 2018; Kvarnemo, 2018). One explanation, the biparental care hypothesis, is that monogamy evolved to promote paternal care (Kleiman, 1977; Wittenberger and Tilson, 1980) when offspring are so costly to rear that both parents must provide extensive care by protecting young from predation or provisioning them with food (Lack, 1968; Gubernick and Teferi, 2000; Reichard, 2003; Tumulty et al., 2014). The other three hypotheses implicate the spatial distribution of mates or resources as the selective factors promoting the evolution of monogamy. The dispersed female hypothesis (Kleiman, 1977) suggests that when females are spread out and at low density, males are physically unable to monopolise multiple females within a breeding cycle. Thus dispersed resources, such as food or the shelter used to avoid predators, may force females to live solitarily and in turn make it uneconomical for males to find and mate with multiple receptive females. Third, the male mate guarding hypothesis (Parker, 1970; Thornhill and Alcock, 1983) argues that the benefits of exclusive access to a fertile female can also lead to monogamy. Male mate guarding occurs when the cost of finding a new mate or sharing a mate is extraordinarily high, such as when there is a strongly male-biased sex ratio, the window of fertility is extremely short, the density of rival males in the population is high, or food scarcity limits the time males can spend searching for a female (Iribarne et al., 1995; Rondeau and Sainte-Maire, 2001; Brotherton and Komers, 2003; Bennett et al., 2012). Finally, the female–female intolerance hypothesis (Wittenberger and Tilson, 1980; Reichard, 2003) suggests that female–female intolerance may arise where there are limited resources, leading to distinct female-held territories even at high population densities (Mathews, 2002; Brotherton and Komers, 2003; Schubert et al., 2009; Lukas and Clutton-Brock, 2013). Female–female intolerance alone does not explain why both females and males would be monogamous, as males could still move between multiple females in this system. However, female mate guarding, a subset of female–female intolerance where the guarded resource is mates or paternal care, can prevent males from leaving female-held territories, resulting in female enforced monogamy (Petrie, 1992; Hunter et al., 1993; Grafe and Bitz, 2004; Palanza et al., 2005; Baniel et al., 2018).
These hypotheses for the evolution of monogamy have primarily been assessed comparatively in birds and mammals (Brotherton and Komers, 2003; Whiteman and Côté, 2004; Lukas and Clutton-Brock, 2013; Opie et al., 2013) and only rarely in other taxa. In mammals and birds, females are generally the limiting sex not only because they produce fewer and larger gametes than males, but also because they are typically the sex which more commonly provides parental care following fertilisation, minimally via internal embryonic development or calcifying fertilised eggs (Trivers, 1972; Clutton-Brock, 1991). Primates appear to provide an extreme case where extended lactational amenorrhea increases infanticide risk and mate guarding males may provide protection from infanticide (Opie et al., 2013). However, male mate guarding may also provide more general benefits in terms of protection against infanticidal males or against predators. In fish, the drivers of monogamy remain enigmatic (Whiteman and Côté, 2004). Teasing apart the relative importance of alternative hypotheses for the evolution of monogamy remains a key empirical challenge. Furthermore, while ecological pressures (either predation rates or food availability) underly each of these hypotheses, how ecological pressures influence the evolution of monogamy has seldom been directly addressed within a single study (Kappeler, 2013; Klug, 2018).
Fishes comprise more than half the total number of described vertebrate species (May, 1990; Nelson et al., 2016; Froese and Pauly, 2022), and demonstrate a great diversity of life history and ecology traits. Further, social monogamy is common in several well-studied groups of fish, in particular among the cichlids and marine reef fishes (Avise et al., 2002; Whiteman and Côté, 2004; Taylor and Knight, 2008). Cichlid fishes are speciose and provide a rich testing ground for evolutionary models of trait evolution between highly varied mating systems and parental care modes (Mank et al., 2005; Egger et al., 2006; Mank and Avise, 2006). Whereas all cichlids have some form of parental care and biparental care is common in this group (Fricke, 1986; Whiteman and Côté, 2004; Duponchelle et al., 2008), marine reef fishes provide an interesting contrast as they rarely provide parental care (Whiteman and Côté, 2004). Therefore, these groups should be considered separately to account for the possible role of parental care in the evolution of monogamy and to avoid masking evolutionary signals by combining two very different groups of animals. Here we set out to test the prediction that biparental care, female dispersion, mate guarding, and female–female intolerance are associated with the evolution of social monogamy in cichlids and marine reef fishes. We used territoriality as a proxy for mate guarding because explicit evidence of mate guarding among fishes is rare (Kokko and Morrell, 2005). In some species, an integral function of territoriality is mate guarding as it ensures extended exclusive access to limited mates (Fricke, 1986; Brotherton and Komers, 2003), however, territoriality can also function as a way to guard resources (Hinsch and Komdeur, 2017). Thus we tested the prediction that the life history traits of biparental care, female dispersal, male territoriality, and female territoriality are driven by resource (food and shelter) distribution in cichlids and marine reef fishes.
Materials and Methods
Data collection
Species were classified as monogamous or not according to their social mating system, rather than genetic brood structure. This is for two reasons: (1) there is a paucity of genetic data about mating system across fishes (Sefc et al., 2008; Lee et al., 2016; Fitzpatrick, 2020), and (2) genetic brood structure is related to postcopulatory sexual selection generally (Jennions and Petrie, 2000; Pitnick and Hosken, 2010; Lüpold et al., 2020), and in fishes in particular (Rowley et al., 2019). Hence, a species was categorised as monogamous when both sexes were observed to associate with only one mate during the mating period and a species was classified as non-monogamous if such exclusive pair bonding was not observed or was a minority strategy in an observed wild population. Also, we did not use mating system information from captive populations (e.g., animals maintained in aquaria), as husbandry conditions can lead to monogamy but might not reflect natural reproductive behaviours.
We classified female sociality as dispersed or non-dispersed (Supplementary Table S1.1). Species were categorised as female dispersed when females do not travel, feed, or guard territories in the presence of other female conspecifics, and do not form spawning aggregations during the breeding season. Territoriality was recorded as present if individuals of that species are spatially associated with a particular area and defend that area (Brown, 1975; Maher and Lott, 2000; Potts et al., 2014). We then further subset territoriality into female-only, male-only, joint (males and females defending territories together) or separate territoriality (each sex holding a separate territory simultaneously, Supplementary Table S1.1). For territoriality analyses in cichlids, species with male-only, joint, and separate sex territoriality were considered to have male territoriality and species with female-only, joint, and separate sex territoriality were considered to have female territoriality (Supplementary Table S1.2). In marine reef fishes, male territoriality was present in all species with available data except one species with female-only territoriality recorded (Supplementary Table S1.2). Therefore, in marine reef fishes a single territoriality analysis was performed which principally examined transitions between no territoriality and territoriality in females, i.e., species with no female territoriality versus species with joint and separate sex territoriality. We used male territoriality as a proxy for male mate guarding and female territoriality as a proxy for female–female intolerance. We assume that territoriality may function to guard physical resources and/or mates; although mate guarding can occur in the absence of territoriality, individuals that guard territories have the potential to also guard individuals within that territory (Reavis and Barlow, 1998; Yamamoto et al., 1999; Brotherton and Komers, 2003; Mathews, 2003; Yokoi et al., 2015).
Parental care was recorded as biparental or not (including maternal, paternal, and no care, Supplementary Table S1.1). Anti-predation strategy was defined as primarily sheltering (hiding from predators in or around a physical structure) or not (including shoaling, actively defending, and fleeing, Supplementary Table S1.1). Diet was defined as guardable food or non-guardable food. Guardable food sources are those which are site-specific, for example, sessile invertebrates and aufwuchs, whereas non-guardable food sources are transient sources, e.g., fishes, motile invertebrates, and planktonic algae (Supplementary Table S1.1).
Information on mating system, female dispersal, territoriality, parental care mode, anti-predator strategy, and diet were taken directly from the individual species pages on FishBase; an online freely available aggregation of fish data (Supplementary Table S1.1; Froese, 1997; Strona, 2014; Thorson et al., 2014). Additional information from 41 further sources was also included (Fricke, 1980, 1986; Lobel and Johannes, 1980; Thresher, 1980, 1984; Wallace and Selman, 1981; Hourigan and Kelley, 1985; Colin and Clavijo, 1988; Brichard, 1989; Colin, 1989; Tricas, 1989; Barlow, 1991; Colin and Bell, 1991; Fowler, 1991; Roberts and Ormond, 1992; Axelrod, 1993; Kuwamura, 1997; Nagoshi and Yanasigawa, 1997; DeMartini, 1998; Gibson, 1998; Righton et al., 1998; Coward and Bromage, 2000; Mylonas and Zohar, 2000; Avise et al., 2002; Zekeria et al., 2002; Murua and Saborido-Rey, 2003; Gibran et al., 2004; Heg et al., 2004; Whiteman and Côté, 2004; Ah-King et al., 2005; Genner and Turner, 2005; Gonzalez-Voyer et al., 2008; Fitzpatrick et al., 2009; Collette, 2010; Takemura et al., 2010; Sogabe and Ahnesjö, 2011; Bellwood and Pratchett, 2013; Pyron et al., 2013; Hughes et al., 2014; Mcbride et al., 2015; Dey et al., 2017).
Phylogenetic analysis
To carry out the phylogenetic analyses, a single consensus tree from Rabosky et al. (2013) was used. This molecular phylogeny is based on the sequence data of 13 genes from 7,822 extant species, representing 399 families (of approximately 550 total families). Sixty fossils were used to temporally calibrate the tree. We carried out our analyses separately in marine reef fishes and cichlids, where possible, to ensure any link between monogamy and the traits tested that are unique to either group was detected and not obscured by the larger scale analyses. The data were filtered to produce two subsets that matched species present in the tree: one cichlid and one marine reef fish subset. In these subsets, 600 cichlid species and 336 marine reef fish species remain, although not every species had data for mating system or every other category Figure 1 (a summary of the data is given in Supplementary Table S1.2). The data and trees used in all subsequent analyses are the smaller, pruned versions. As there are some traits with little variation among cichlids, members of a sister clade were included in each analysis to help resolve the ancestral state (126 species, mostly ricefishes, surfperches, clownfishes and damselfishes listed in Supplementary Table S1.3). The reef fishes subset is the monophyletic group Percoidei, but the data primarily comes from the butterflyfishes, triggerfishes, emperor breams, wrasses, and surgeons and tangs (Chaetodontidae, Balistidae, Lethrinidae, Labridae and Acanthuridae respectively, Supplementary Table S1.4).
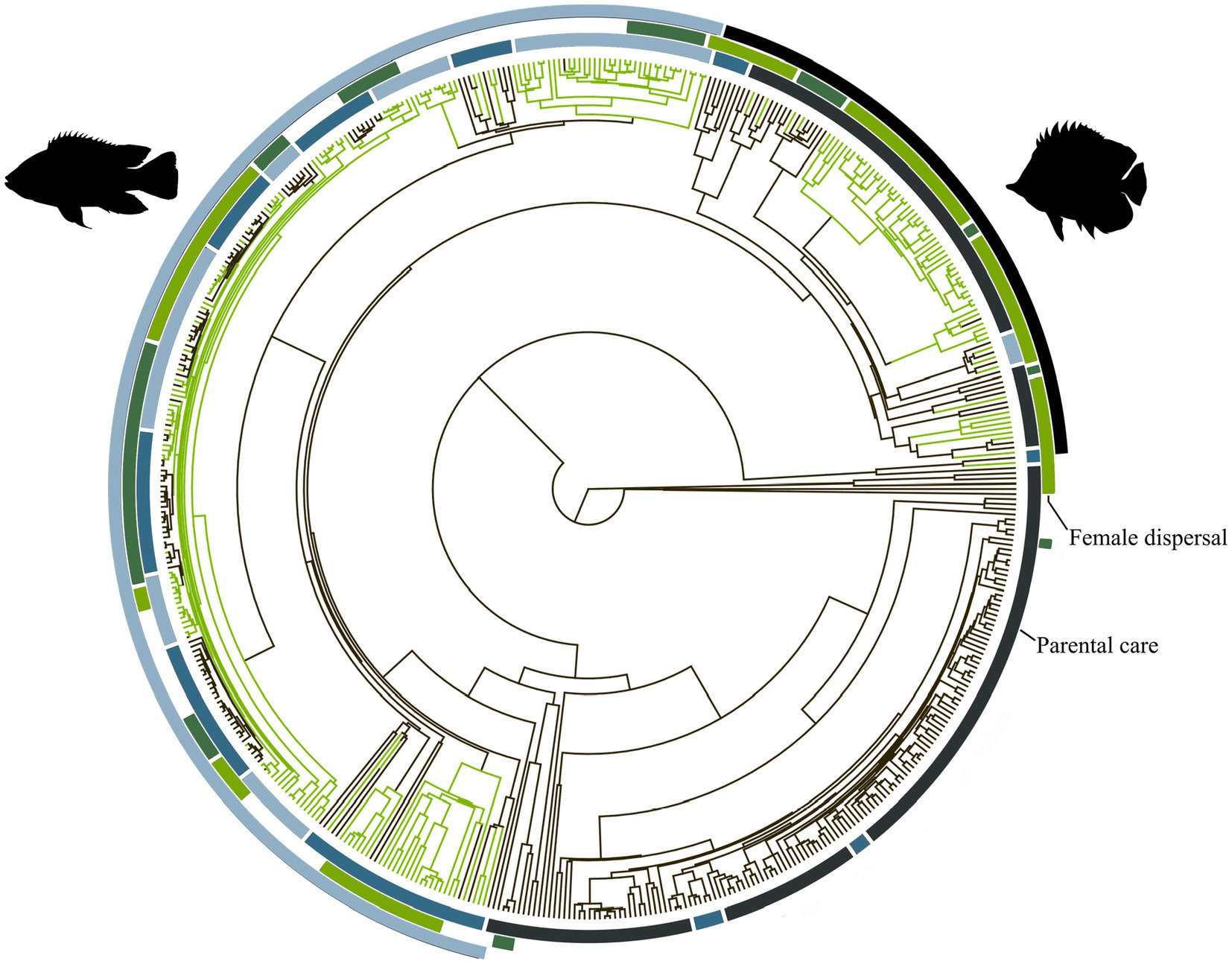
Figure 1. Phylogeny of the 546 fish species with known mating system analysed in this study. The branch colours correspond to mating system, where black = non-monogamous and green = monogamous. The inner band corresponds to parental care, where navy blue = no care, mid blue = uniparental care, and light blue = biparental care. The middle band corresponds to female dispersal, where dark green = multi-female groups and light green = dispersed females. The outer band shows the cichlids (left) and the marine reef fishes (right). Images are from PhyloPic.org. The cichlid image is courtesy of C. Julian under a creative commons attribution 3.0 unported licence (https://creativecommons.org/licenses/by/3.0/).
Each analysis considered the coevolution of two categorical traits which were binary coded (Table 1). To address the effect of each trait, we first analysed the links between each trait and mating system (Supplementary Table S1.2). To determine whether each of the life history traits was ultimately driven by ecology, where possible (Supplementary Table S1.2) we assessed each trait in a pairwise fashion with primary anti-predation strategy and diet via discrete analyses.
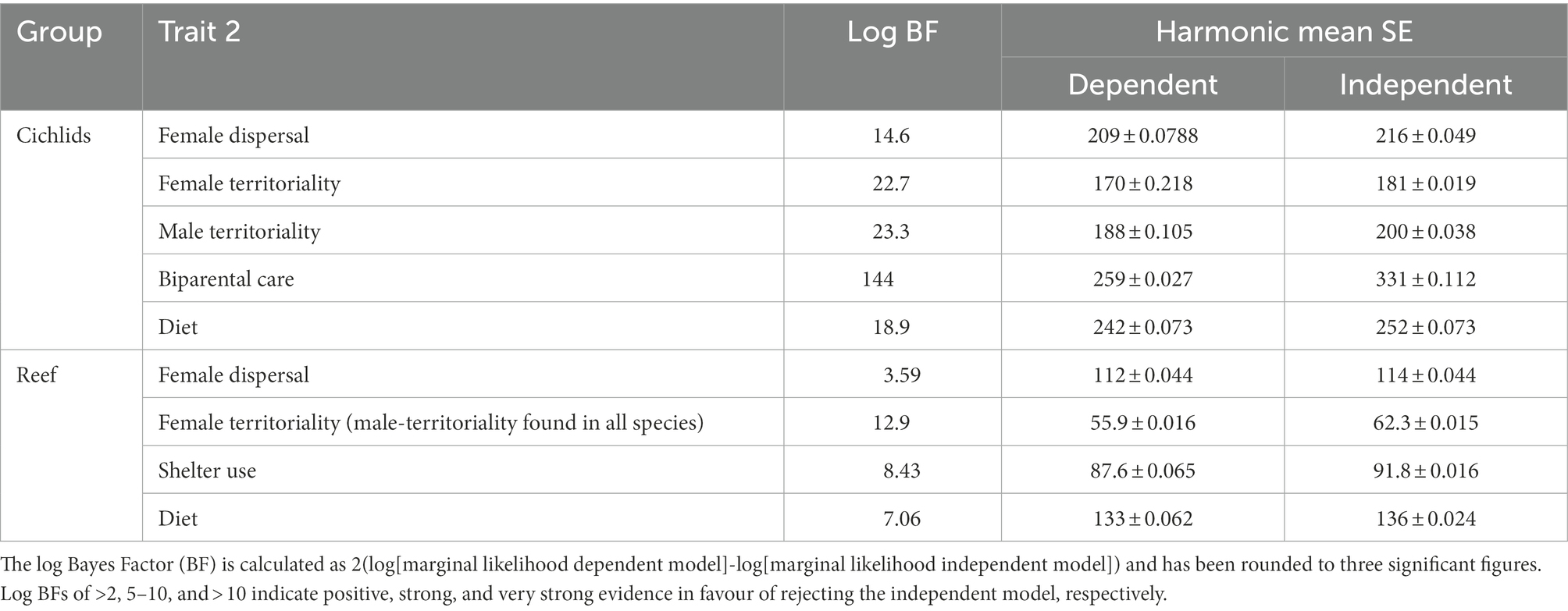
Table 1. Summary of the dependent (correlated evolution of the two traits) and independent (independent evolution of the two traits) models of mating system (monogamy) and the presence each of the given traits in cichlids and marine fishes.
BayesTraits
The discrete method within the BayesTraits program was used to test for correlated evolution between each of the traits considered (female dispersal, female territoriality, male territoriality, biparental care, sheltering from predators, and diet) and social monogamy following binary coding (Pagel and Meade, 1996; Lukas and Clutton-Brock, 2013; Opie et al., 2013, 2014). The discrete method can determine whether two traits are more likely to have evolved in conjunction or independently. To do this, two models were produced in each test: the first assumes the traits evolve independently of each other (independent model); the second allows for correlated evolution between the two traits (dependent model). A Markov-Chain Monte Carlo (MCMC) and reverse jump (RJ) approach were used to allow the derivation of a Bayesian posterior distribution for rate parameters, model log-likelihoods and ancestral states (Table 1). The relative fit of the independent and dependent models was assessed by calculating the Log Bayes Factor (Log BF = 2[Lh dependent model – Lh independent model], where Lh is the log likelihood of the model). If the Log BF was greater than two, we took this to mean there is evidence in favour of the dependent model (Pagel and Meade, 1996). The proportion of any one evolutionary transition being set as 0, i.e., did not occur, is the Z-score. When the Z-score for any transition in the dependent models was greater than 50% that transition is unlikely to have occurred across the group (Pagel and Meade, 1996), whereas transitions with Z-scores below 50% are likely to have occurred. The Z-scores from the eight transitions in each dependent model were interpreted to assess causality, i.e., if monogamy does not evolve when trait A is absent but does evolve when trait A is present and there is strong evidence of correlated evolution between the traits, we inferred that trait A appears to be driving the evolution of monogamy. Although rate coefficients for each transition indicate the magnitude with which each transition has likely occurred, we did not use these to infer causality because high transition rates can indicate both many occasions of correlated evolution and unstable trait combinations, and low transition rates can indicate a key basal transition or few correlated transitions near the tips of the tree.
Results
Cichlids
Female dispersal, male and female territoriality and biparental care are all associated with the evolution of social monogamy in cichlids (Table 1). All transitions are summarised in Figure 2. Male territoriality precedes the evolution of social monogamy (Supplementary Figure S1A), although not all territorial species are monogamous (Figure 3). Therefore, male territoriality appears to be necessary but perhaps not sufficient for the evolution of monogamy. The emergence of female territoriality generally coincides with the appearance of monogamy across tribes (Figure 3) and appears to maintain/ prevent the loss of social monogamy in cichlids, as monogamy is not lost in species with female territoriality (Supplementary Figure S2C). Conversely, biparental care follows the appearance of monogamy. Further, biparental care without monogamy is an evolutionarily unstable state, as either biparental care is readily lost or monogamy gained in such circumstances (Supplementary Figure S2B). In contrast, neither female dispersal nor a diet of guardable food enforce or maintain monogamy, as monogamy is lost when one of these traits is present (Supplementary Figures S2D,E). However, female dispersal and monogamy often appear together across tribes, whereas a diet of guardable food rarely coincides with monogamy (Figure 3).
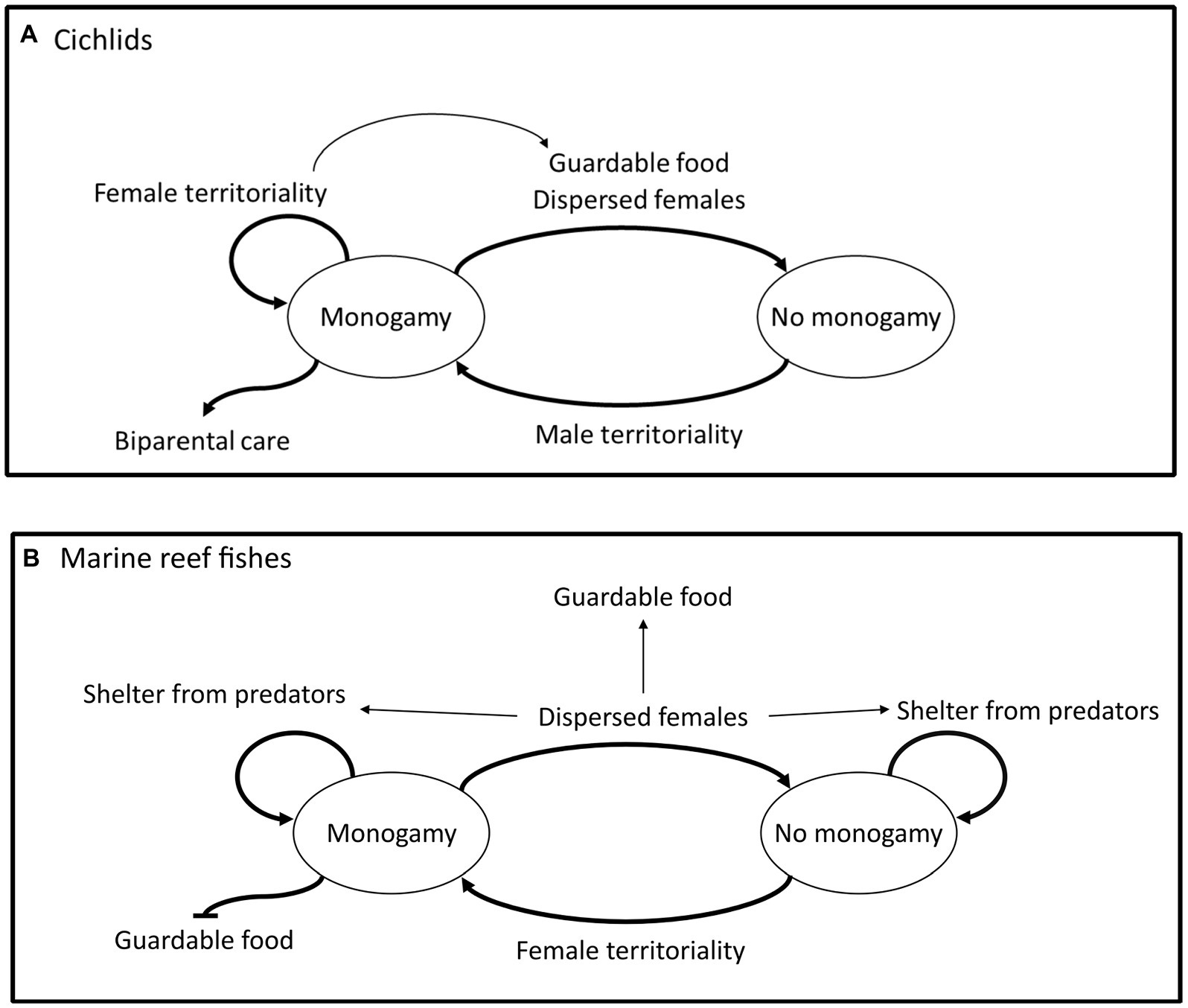
Figure 2. Summary of the discrete analyses which found strong evidence of directional correlated evolution between traits in cichlids (A), and in marine reef fishes (B). Thicker arrows indicate evolutionary transitions directly related to monogamy; traits placed by the thicker arrows are likely driving these transitions. For example, in cichlids male territoriality drives the evolution and maintenance of monogamy from non-monogamy and the evolution of biparental care is driven by monogamy. Thinner arrows represent evolutionary transitions between traits other than monogamy, for example, in cichlids female territoriality drives the evolution of a diet of guardable food.
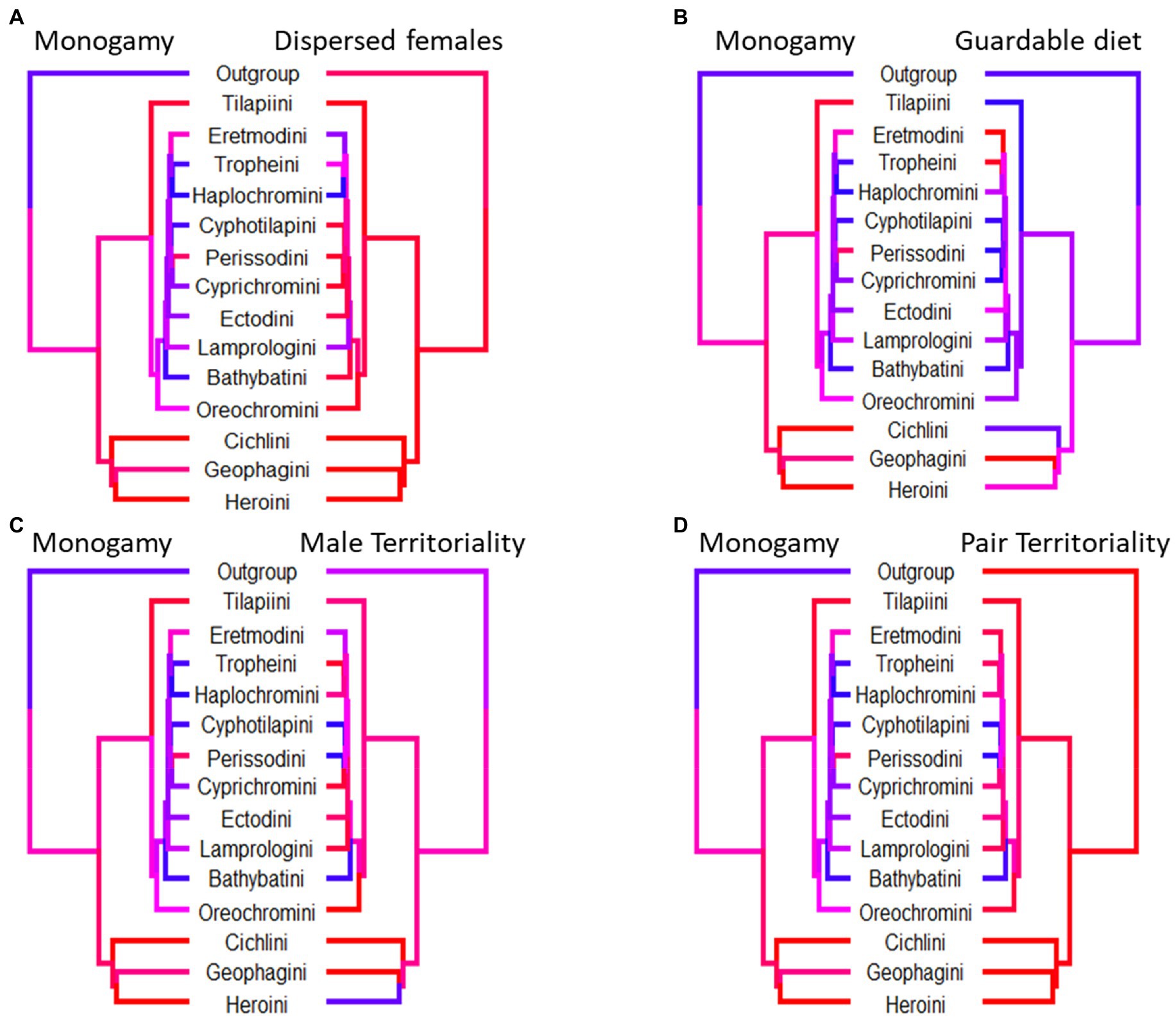
Figure 3. Phylogenetic trees where each tip corresponds to a tribe of cichlid fishes. Tip and branch colours correspond to the proportion of species with data present in the tribe which display the trait, with red corresponding to a value of 1, blue to a value of zero. For each tree we only included species which had data present for either trait and not all trees contain the same tribes. Although all paired traits evolve dependently with each other, the coincidence of monogamy and its paired trait differs. (A) Monogamy is slightly more prevalent in tribes with dispersed females. (B) Highly monogamous tribes are also slightly more likely to not have a diet of guardable food. (C) There is no clear pattern between the high incidence of monogamy and male territoriality across cichlid tribes. (D) Highly monogamous tribes are likely also tribes with a high incidence of female territoriality.
Female territoriality is strongly associated with and precedes the evolution of a diet of guardable food (Figure 2; Table 2; Supplementary Figure S3B). Despite evidence of dependent evolution between male territoriality and female dispersal and male territoriality and diet, the directionality of the relationships is unclear (Table 2; Supplementary Figure S3A). Analysis of correlated evolution between shelter use and mating system was not performed due to insufficient data for cichlids and there was no evidence of dependent evolution between diet and female dispersal or diet and female territoriality (Table 2; Supplementary Figure S2.3).
Marine reef fishes
Female dispersal, female territoriality, diet, and sheltering from predators are all associated with the evolution of social monogamy in reef fishes (Table 1). All transitions are summarised in Figure 2. Female territoriality and monogamy generally coincide across genera (Figure 4D). Furthermore, female territoriality appears to drive the evolution of monogamy, as species first gain female territoriality and subsequently monogamy (Supplementary Figure S2A). Additionally, sheltering from predators tends to coincide with monogamy across genera (Figure 4C) and prevents the loss and gain of monogamy (i.e., there are no transitions between mating systems in species that shelter from predators) in both directions and there is only an evolutionary switch between monogamy and non-monogamy when a species does not use shelter to avoid predation (Figure 4C; Supplementary Figure S2B). A diet of guardable food tends to precede the appearance of monogamy, but does not follow it (Figure 4C; Supplementary Figure S2C), with these traits generally coinciding across genera (Figure 4). Monogamy and female dispersal also tend to coincide across genera (Figure 4), however, monogamy can be lost in species with dispersed females in marine reef fishes (Figure 4D). Biparental care is very rare in marine reef fishes, with fewer than 2% of the species in our data set exhibiting biparental care (Supplementary Table S1.1). Therefore, an analysis to explore the coevolution between social monogamy and biparental care was not performed in this group.
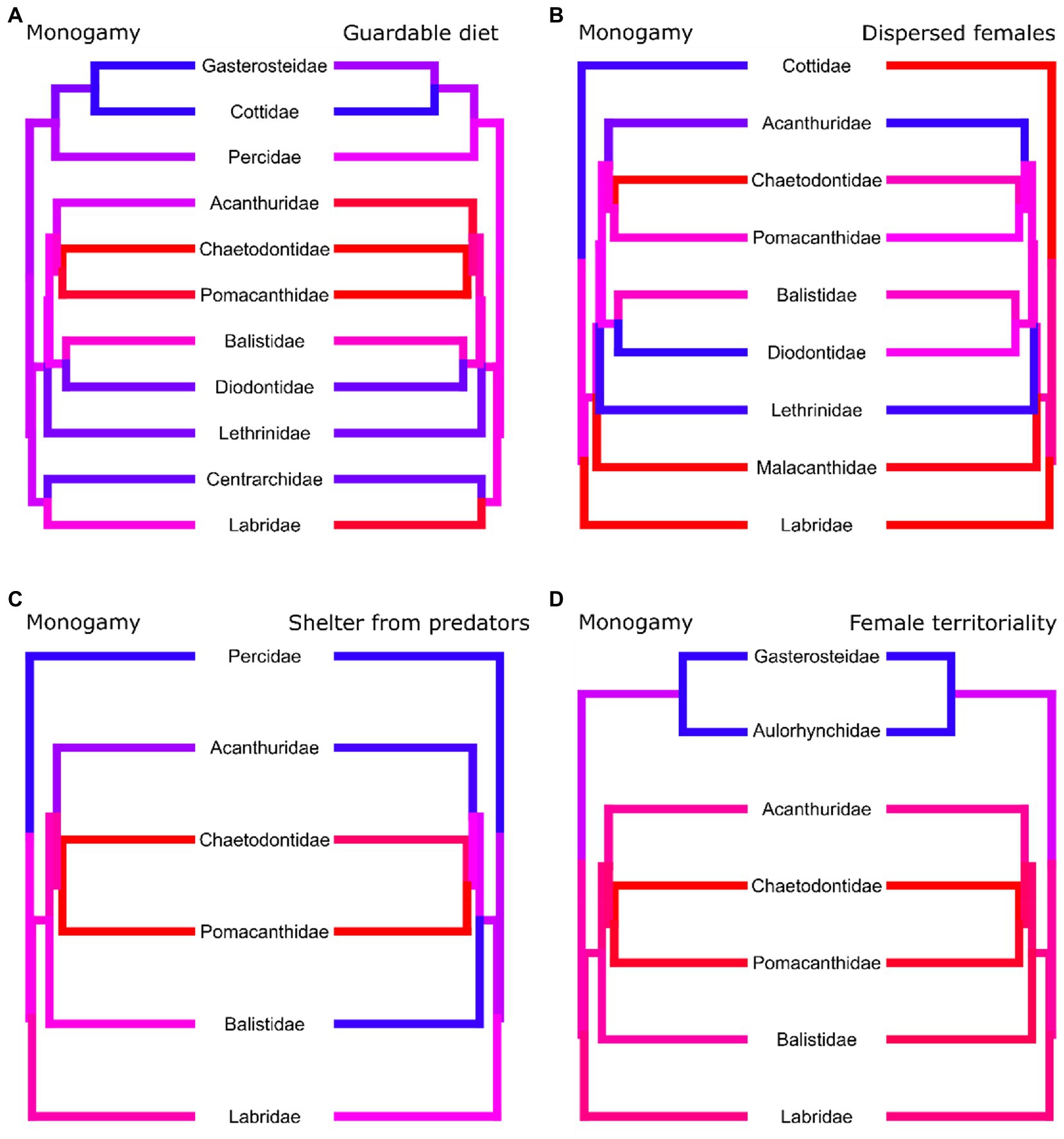
Figure 4. Phylogenetic trees where each tip corresponds to a genus of marine reef fishes. Tip and branch colours correspond to the proportion of species with data present in the genus which display the trait, with red corresponding to a value of 1 and blue to a value of zero. For each tree we only included species which had data present for both traits and not all trees contain the same genera. Although all paired traits evolve dependently with each other, the coincidence of monogamy and its paired trait differs. (A) Monogamy is more prevalent in genera with diets of guardable food. Highly monogamous genera are more likely to also have a high incidence of (B) dispersed females and (C) sheltering from predators and vice versa. (D) Genera with a high incidence of monogamy also have a high incidence of female territoriality.
Female dispersal is strongly associated with and drives the evolution of a diet of guardable food and use of shelter to avoid predators, as both a diet of guardable food and sheltering to avoid predators have evolved in species which first had dispersed females (Figure 2; Table 2; Supplementary Figures S4A,B). Similarly, diet is also strongly associated with and drives the evolution of using shelter to avoid predators (Figure 2; Table 2; Supplementary Figure S4C). Of the further pairwise analyses that were conducted in marine reef fishes, there was no evidence of correlated evolution between female territoriality and diet or female territoriality and female dispersal (Table 2).
Discussion
In cichlids, we found that male territoriality is necessary but not sufficient for the evolution of monogamy; although male territoriality is nearly ubiquitous in lineages where monogamy emerges, many species with male territoriality are not monogamous. However, the trigger for monogamy is not clear as it is not female dispersal, female territoriality or diet. In contrast, the emergence of female territoriality does appear key to the evolution of monogamy in marine reef fishes. We infer that territoriality is a proxy of mate guarding because mate guarding is often a fundamental aspect of territoriality (Brotherton and Komers, 2003) despite evidence of mate guarding being rare (Kokko and Morrell, 2005). Here, we clearly demonstrate the importance of territoriality in the evolution of monogamy and suggest our results provide some support for male mate guarding playing a non-exclusive role in cichlid monogamy. Further, the necessity of female territoriality for the evolution of monogamy in marine reef fishes provides evidence for the female–female intolerance hypothesis in this group, where female guarding of males is the causal factor, as has been found in primates (Lukas and Clutton-Brock, 2013; Opie et al., 2013). Monogamy has likely only been gained without female territoriality in a single occasion in marine reef fishes, which suggests that if this is an alternative evolutionary pathway it is rare. Further, our results do not rule out the idea that guarding limited resources other than available mates has driven the evolution of monogamy in both or either cichlids and marine reef fishes. We also found that female territoriality may help enforce/prevent the loss of monogamy in cichlids, suggesting that female–female intolerance maintains monogamy in this group. Female territoriality does drive the transition to using guardable food sources and, in turn, a diet of guardable food drives the loss of monogamy in cichlids. Biparental care has only evolved after the emergence of monogamy in cichlids, providing evidence against the biparental care hypothesis. Similar evidence against the biparental care hypothesis has also been shown in callitrichid monkeys (Dunbar, 1995; Opie et al., 2013). In marine reef fishes the biparental care hypothesis both could not be tested and is highly unlikely to drive the evolution of monogamy because almost no species in this group engage in any parental care. In both cichlids and marine reef fishes we found that dispersed females drive the loss of monogamy, possibly because the benefits to males of monogamy when females are highly dispersed are greatly reduced when breeding seasons are long or frequent (Tecot et al., 2016; Gomes et al., 2018), as is the case in most cichlids and marine reef fishes (Barlow, 1991; Yabuta and Berumen, 2014). In marine reef fishes, female dispersal drives the transition to using guardable food sources and shelters to avoid predation. In turn, shelter use prevents evolutionary transitions between monogamy and non-monogamy, e.g., a marine reef fish species that is both monogamous and shelters from predators will not lose monogamy, whereas a species that is monogamous but does not shelter from predators may lose monogamy. In marine reef fishes social monogamy prevents the evolution of a diet of guardable food.
Overall, we found that a suite of factors evolve dependently with monogamy. These factors either maintain, drive the loss of, or prevent the loss of monogamy in both cichlids and marine reef fishes. Further, several of these traits were found to coevolve. This highlights the complex interactions between life history and ecological traits which underly the evolution of monogamy in both groups. By considering these two groups separately we have shown here that there are likely multiple mechanisms, either converging or acting independently, resulting in the evolution, maintenance, and loss of social monogamy (Wittenberger and Tilson, 1980; Gowaty, 1996; Whiteman and Côté, 2004). The findings of our study demonstrate that groups with disparate ecologies and life histories, as in the case of cichlids and marine reef fishes, can have different evolutionary relationships between the same traits (McGaughran et al., 2010; Hawkins et al., 2012). This explains some of the conflicting results of prior studies into the origin of social monogamy (Lukas and Clutton-Brock, 2013; Mabry et al., 2013; Opie et al., 2013), because, even within vertebrate classes, which ecologically distinct groups are included and how many species’ data represent them can significantly impact the findings. When two groups have conflicting drivers of social monogamy, any of these drivers might be masked in an analysis performed on too high a taxonomic scale (Harvey and Pagel, 1991). This highlights important considerations for all phylogenetic reconstructive studies. Larger phylogenetic trees alone may not be able to reveal evolutionary patterns when there are multiple underlying relationships between traits. To better unmask potentially conflicting drivers of monogamy it is important to consider smaller, closely related, ecologically unique groups as well as running the analysis on higher taxa. Future work should consider multiple hypotheses of social monogamy evolution and assess the underlying ecological and life history factors. Further phylogenetic comparative studies should separately consider within-taxon groups with unique ecologies and life histories to avoid masking divergent evolutionary histories or overgeneralising evolutionary patterns across large taxa.
Our study was clearly limited by the overrepresentation in the dataset of more easily accessible, observable, and economically important fish species. Additionally, although we did reveal links between predation and monogamy and diet and monogamy, our proxies for predation pressure (sheltering) and diet (guardable food sources) were crude. It is thus possible that further analyses using alternative predation pressure and diet proxies may yield more conclusive results, however, further data on predation pressure collected in a manner comparable across fish habitats must be obtained for such an analysis to be viable. Similarly, because mate guarding is only one possible guarding strategy for a territorial individual, the relationship between mate guarding and monogamy should be empirically tested, or explicit instances of mate guarding as opposed to other forms of territoriality should be reported and included in comparative analyses.
Interestingly, we found that monogamy and biparental care are closely linked in cichlids, as they are in birds (Lack, 1968; Kleiman, 1977; Wittenberger and Tilson, 1980), while this is clearly not the case in marine reef fishes (Whiteman and Côté, 2004). Therefore, there are likely some fundamental life history differences between these two groups that enable biparental care in cichlids (and birds) and prevent its occurrence in marine reef fishes. We hypothesise that the method of egg release is the crucial factor. The near-universal nesting behaviour present in cichlids and birds enables a broad range of caring modes, such as guarding, egg rotation, and provisioning, to evolve. When care mode becomes more complex and costly, biparental care evolved as an effective breeding strategy. Conversely, egg release via broadcast spawning is extremely common in marine reef fishes, and precludes complex post-release care, hence biparental care will not evolve in such broadcast spawning species. Moreover, while we find there are multiple factors evolutionarily coupled with the evolution of monogamy in both groups, many of these are likely to be highly taxon specific and not generalisable to other groups, similarly to the case of lactational amenorrhoea driving monogamy in some primates (Opie et al., 2013). Overall, we demonstrate that male territoriality in cichlids and female territoriality in marine reef fishes drive the evolution of monogamy. Therefore, we find support for the mate guarding hypothesis in cichlids and the female–female intolerance hypothesis in marine reef fishes, but do not find support for a single hypothesis across both groups. We also clearly provide evidence against the biparental care and dispersed female hypotheses, but show that female dispersal instead may drive the loss of monogamy.
Data availability statement
The original contributions presented in the study are included in the article/Supplementary material, further inquiries can be directed to the corresponding author.
Ethics statement
Ethical review and approval were not required for the animal study because the research is based on published information (no animals were used).
Author contributions
ES, JF, SB, and SS contributed to the study design and approach. ES collated data, completed analyses, and wrote manuscript draft. JF, SB, and SS contributed to editing the manuscript, troubleshooting analyses, and verifying data. All authors contributed to the article and approved the submitted version.
Funding
This work was supported by the Natural Environment Research Council (NERC) EAO Doctoral Training Partnership (grant number NE/L002469/1 to ES), the Natural Sciences and Engineering Research Council (Discovery Grant number RGPIN-2016-05772 to SB), and the Royal Society (University Research Fellowship grant number UF110641 to SS).
Acknowledgments
We thank Tim Clutton-Brock for his helpful comments on an earlier version of this manuscript.
Conflict of interest
The authors declare that the research was conducted in the absence of any commercial or financial relationships that could be construed as a potential conflict of interest.
Publisher’s note
All claims expressed in this article are solely those of the authors and do not necessarily represent those of their affiliated organizations, or those of the publisher, the editors and the reviewers. Any product that may be evaluated in this article, or claim that may be made by its manufacturer, is not guaranteed or endorsed by the publisher.
Supplementary material
The Supplementary material for this article can be found online at: https://www.frontiersin.org/articles/10.3389/fevo.2022.1045383/full#supplementary-material
References
Ah-King, M., Kvarnemo, C., and Tullberg, B. S. (2005). The influence of territoriality and mating system on the evolution of male care: a phylogenetic study on fish. J. Evol. Biol. 18, 371–382. doi: 10.1111/j.1420-9101.2004.00823.x
Avise, J. C., Jones, A. G., Walker, D., and DeWoody, J. A. (2002). Genetic mating systems and reproductive natural histories of fishes: lessons for ecology and evolution. Annu. Rev. Genet. 36, 19–45. doi: 10.1146/annurev.genet.36.030602.090831
Axelrod, H. R. (1993). The most complete colored lexicon of cichlids. United States: T.F.H Publications.
Baniel, A., Cowlishaw, G., and Huchard, E. (2018). Jealous females? Female competition and reproductive suppression in a wild promiscuous primate. Proc. R. Soc. B Biol. Sci. 285, 1–9. doi: 10.1098/rspb.2018.1332
Barlow, G. W. (1991). “Mating systems among cichlid fishes,” in Cichlid fishes: Behaviour, ecology and evolution. ed. M. H. A. Keenleyside (London: Chapman and Hall).
Bateman, A. (1948). Intrasexual selection in drosophila. Heredity 2, 349–368. doi: 10.1038/hdy.1948.21
Bellwood, D. R., and Pratchett, M. S. (2013). “The origins and diversification of coral reef,” in Biology of butterflyfishes. eds. M. S. Pratchett, M. L. Berumen, and B. G. Kapoor (United States: CRC Press).
Bennett, V. J., Smith, W. P., and Betts, M. G. (2012). Evidence for mate guarding behavior in the Taylor’s Checkerspot butterfly. J. Insect Behav. 25, 183–196. doi: 10.1007/s10905-011-9289-1
Brichard, P. (1989). Pierre Brichard’s book of cichlids and all the other fishes of Lake Tanganyika. United States: T.F.H Publications.
Brotherton, P. N. M., and Komers, P. E. (2003). “Mate guarding and the evolution of social monogamy in mammals,” in Monogamy: Mating strategies and partnerships in birds, Humans and Other Mammals. eds. U. H. Reichard and C. Boesch (Cambridge: Cambridge University Press).
Clutton-Brock, T. H. (1991). The evolution of parental care. United States: Princeton University Press.
Colin, P. L. (1989). Aspects of the spawning of western Atlantic butterflyfishes (Pisces: Chaetodontidae). Environ. Biol. Fish 25, 131–141. doi: 10.1007/BF00002207
Colin, P. L., and Bell, L. J. (1991). Aspects of the spawning of labrid and scarid fishes (Pisces: Labroidei) at Enewetak atoll, Marshall Islands with notes on other families. Environ. Biol. Fish 31, 229–260. doi: 10.1007/BF00000690
Colin, P. L., and Clavijo, I. E. (1988). Spawning activity of fishes producing pelagic eggs on a shelf edge coral reef, southwestern Puerto Rico. Bull. Mar. Sci. 43, 249–279.
Collet, J. M., Dean, R. F., Worley, K., Richardson, D. S., and Pizzari, T. (2014). The measure and significance of Bateman’s principles. Proc. R. Soc. B Biol. Sci. 281:2973. doi: 10.1098/rspb.2013.2973
Collette, B. B. (2010). “Reproduction and development in epipelagic fishes,” in Reproduction and sexuality in marine fishes: Patterns and processes. ed. K. S. Cole (United States: University of California Press), 20–63.
Coward, K., and Bromage, N. R. (2000). Reproductive physiology of female tilapia broodstock. Rev. Fish Biol. Fish. 10, 1–25. doi: 10.1023/A:1008942318272
DeMartini, E. E. (1998). “Intertidal Spawning,” in Intertidal fishes: Life in two worlds. eds. M. Horn, K. Martin, and M. Chotkowski (United States: Academic Press).
Dey, C. J., O’Connor, C. M., Wilkinson, H., Shultz, S., Balshine, S., and Fitzpatrick, J. L. (2017). Direct benefits and evolutionary transitions to complex societies. Nature Ecol. Evol. 1, 1–8. doi: 10.1038/s41559-017-0137
Dixson, A. F. (2013). Male infanticide and primate monogamy. Proc. Natl. Acad. Sci. U. S. A. 110:E4937. doi: 10.1073/pnas.1318645110
Dunbar, R. I. (1995). The mating system of callitrichid primates: I. conditions for the coevolution of pair bonding and twinning. Anim. Behav. 50, 1057–1070. doi: 10.1016/0003-3472(95)80106-5
Duponchelle, F., Paradis, E., Ribbink, A. J., and Turner, G. F. (2008). Parallel life history evolution in mouthbrooding cichlids from the African Great Lakes. Proc. Natl. Acad. Sci. U. S. A. 105, 15475–15480. doi: 10.1073/pnas.0802343105
Egger, B., Obermüller, B., Phiri, H., Sturmbauer, C., and Sefc, K. M. (2006). Monogamy in the maternally mouthbrooding Lake Tanganyika cichlid fish Tropheus moorii. Proceedings. Biological Sciences/The Royal Society 273, 1797–1802. doi: 10.1098/rspb.2006.3504
Fitzpatrick, J. L. (2020). Sperm competition and fertilization mode in fishes: sperm competition in fishes. Philosop. Transact. Royal Society B: Biological Sci. 375:0074. doi: 10.1098/rstb.2020.0074rstb20200074
Fitzpatrick, J. L., Montgomerie, R., Desjardins, J. K., Stiver, K. A., Kolm, N., and Balshine, S. (2009). Female promiscuity promotes the evolution of faster sperm in cichlid fishes. Proc. Natl. Acad. Sci. U. S. A. 106, 1128–1132. doi: 10.1073/pnas.0809990106
Fowler, A. J. (1991). Reproductive biology of bisexual and all-female populations of chaetodontid fishes from the southern great barrier reef. Environ. Biol. Fish 31, 261–274. doi: 10.1007/BF00000691
Fricke, H. W. (1980). Mating systems, maternal and Biparental Care in Triggerfish (Balistidae). Z. Tierpsychol. 53, 105–122. doi: 10.1111/j.1439-0310.1980.tb01043.x
Fricke, H. W. (1986). Pair swimming and mutual partner guarding in monogamous butterflyfish (Pisces, Chaetodontidae): a joint advertisement for territory. Ethology 73, 307–333. doi: 10.1111/j.1439-0310.1986.tb00812.x
Froese, R. (1997). “The science in FishBase,” in Ecosystem approaches to fisheries: A global perspective. eds. V. Christensen and J. MacLean (Cambridge: Cambridge University Press).
Froese, R., and Pauly, D. (eds.). (2022). FishBase. World Wide Web electronic publication. Available at: http://www.fishbase.org/
Genner, M. J., and Turner, G. F. (2005). The mbuna cichlids of Lake Malawi: a model for rapid speciation and adaptive radiation. Fish Fish. 6, 1–34. doi: 10.1111/j.1467-2679.2005.00173.x
Gibran, F. Z., Santos, F. B., dos Santos, H. F., and Sabino, J. (2004). Scientific note courtship behavior and spawning of the hairy blenny Labrisomus nuchipinnis (Labrisomidae) in southeastern Brazil. Neotropical Ichthyology 2, 163–166. doi: 10.1590/S1679-62252004000300009
Gibson, R. N. (1998). “Movement and homing in intertidal fishes,” in Intertidal fishes: Life in two worlds. eds. M. Horn, K. Martin, and M. Chotkowski (United States: Academic Press).
Gomes, B. V., Guimarães, D. M., Szczupak, D., and Neves, K. (2018). Female dispersion and sex ratios interact in the evolution of mating behavior: a computational model. Sci. Rep. 8, 1–8. doi: 10.1038/s41598-018-20790-7
Gonzalez-Voyer, A., Fitzpatrick, J. L., and Kolm, N. (2008). Sexual selection determines parental care patterns in cichlid fishes. Evolution 62, 2015–2026. doi: 10.1111/j.1558-5646.2008.00426.x
Gowaty, P. A. (1996). “Battles of the sexes and origins of monogamy,” in Partnerships in birds: The study of monogamy. ed. J. M. Black (Oxford: Oxford University Press), 21–52.
Grafe, T. U., and Bitz, J. H. (2004). Functions of duetting in the tropical boubou, Laniarius aethiopicus: territorial defence and mutual mate guarding. Anim. Behav. 68, 193–201. doi: 10.1016/j.anbehav.2003.11.003
Griffith, S. C., Owens, I. P. F., and Thuman, K. A. (2002). Extra pair paternity in birds: a review of interspecific variation and adaptive function. Mol. Ecol. 11, 2195–2212. doi: 10.1046/j.1365-294x.2002.01613.x
Gubernick, D. J., and Teferi, T. (2000). Adaptive significance of male parental care in a monogamous mammal. Royal Society 267, 147–150. doi: 10.1098/rspb.2000.0979
Harvey, P. H., and Pagel, M. (1991). “The comparative method for studying adaptation,” in The comparative method in evolutionary biology. eds. P. H. Harvey and M. Pagel (Oxford: Oxford University Press), 21.
Hawkins, B. A., Mccain, C. M., Davies, T. J., Buckley, L. B., Anacker, B. L., Cornell, H. V., et al. (2012). Different evolutionary histories underlie congruent species richness gradients of birds and mammals. J. Biogeogr. 39, 825–841. doi: 10.1111/j.1365-2699.2011.02655.x
Hayward, A., and Gillooly, J. F. (2011). The cost of sex: quantifying energetic investment in gamete production by males and females. PLoS One 6:557. doi: 10.1371/journal.pone.0016557
Heg, D., Bachar, Z., Brouwer, L., and Taborsky, M. (2004). Predation risk is an ecological constraint for helper dispersal in a cooperatively breeding cichlid. Proc. R. Soc. B Biol. Sci. 271, 2367–2374. doi: 10.1098/rspb.2004.2855
Hinsch, M., and Komdeur, J. (2017). What do territory owners defend against? Proc. R. Soc. B Biol. Sci. 284:2356. doi: 10.1098/rspb.2016.2356
Hourigan, T. F., and Kelley, C. D. (1985). Histology of the gonads and observations on the social behavior of the Caribbean angelfish Holacanthus tricolor. Mar. Biol. 88, 311–322. doi: 10.1007/BF00392592
Hughes, R. N., Hughes, D. J., and Smith, I. P. (2014). Oceanography and marine biology: An annual review, 52. United States: CRC Press.
Hunter, F. M., Petrie, M., Otronen, M., Birkhead, T., and Møller, P. (1993). Why do females copulate repeatedly with one male? Trends Ecol. Evolution (Personal Edition) 8, 21–26. doi: 10.1016/0169-5347(93)90126-A
Iribarne, O., Fernandez, M., and Armstrong, D. (1995). Precopulatory guarding-time of the male amphipod Eogammarus oclairi: effect of population structure. Mar. Biol. 124, 219–223. doi: 10.1007/BF00347126
Janicke, T., Häderer, I. K., Lajeunesse, M. J., and Anthes, N. (2016). Darwinian sex roles confirmed across the animal kingdom. Sci. Adv. 2:e1500983. doi: 10.1126/sciadv.1500983
Jennions, M. D., and Petrie, M. (2000). Why do females mate multiply? A review of the genetic benefits. Biol. Rev. 75, 21–64. doi: 10.1017/S0006323199005423
Kappeler, P. M. (2013). Why male mammals are monogamous. Science 341, 469–470. doi: 10.1126/science.1242001
Kappeler, P. M. (2014). Lemur behaviour informs the evolution of social monogamy. Trends Ecol. Evol. 29, 591–593. doi: 10.1016/j.tree.2014.09.005
Klug, H. (2018). Why monogamy? A review of potential ultimate drivers. Front. Ecol. Evol. 6:30. doi: 10.3389/fevo.2018.00030
Kokko, H., and Morrell, L. J. (2005). Mate guarding, male attractiveness, and paternity under social monogamy. Behav. Ecol. 16, 724–731. doi: 10.1093/beheco/ari050
Kuwamura, T. (1997). “The evolution of parental care and mating systems,” in Fish communities in Lake Tanganyika. eds. H. Kawanabe, M. Hori, and M. Nagoshi (Japan: Kyoto University Press).
Kvarnemo, C. (2018). Why do some animals mate with one partner rather than many? A review of causes and consequences of monogamy. Biol. Rev. 1800, 1795–1812. doi: 10.1111/brv.12421
Lee, H. J., Heim, V., and Meyer, A. (2016). Genetic evidence for prevalence of alloparental care in a socially monogamous biparental cichlid fish, Perissodus microlepis, from Lake Tanganyika supports the “selfish shepherd effect” hypothesis. Ecol. Evol. 6, 2843–2853. doi: 10.1002/ece3.2089
Lobel, P. S., and Johannes, R. E. (1980). Nesting, eggs and larvae of triggerfishes (Balistidae). Environ. Biol. Fish 5, 251–252. doi: 10.1007/BF00005359
Lukas, D., and Clutton-Brock, T. H. (2013). The evolution of social monogamy in mammals. Science 341, 526–530. doi: 10.1126/science.1238677
Lüpold, S., De Boer, R. A., Evans, J. P., Tomkins, J. L., and Fitzpatrick, J. L. (2020). How sperm competition shapes the evolution of testes and sperm: a meta-analysis: sperm competition meta-analysis. Philosop. Transact. Royal Society B: Biological Sci. 375:0064. doi: 10.1098/rstb.2020.0064
Mabry, K. E., Shelley, E. L., Davis, K. E., Blumstein, D. T., and Van Vuren, D. H. (2013). Social mating system and sex-biased dispersal in mammals and birds: a phylogenetic analysis. PLoS One 8:980. doi: 10.1371/journal.pone.0057980
Maher, C. R., and Lott, D. R. (2000). A review of ecological determinants of territoriality within vertebrate species. American Midand Naturalist 143, 1–29. doi: 10.1674/0003-0031(2000)143[0001:AROEDO]2.0.CO;2
Mank, J. E., and Avise, J. C. (2006). The evolution of reproductive and genomic diversity in ray-finned fishes: insights from phylogeny and comparative analysis. J. Fish Biol. 69, 1–27. doi: 10.1111/j.1095-8649.2006.01132.x
Mank, J. E., Promislow, D. E. L., and Avise, J. C. (2005). Phylogenetic perspectives on the evolution of parental care in fishes. Evolution 59, 1570–1578. doi: 10.1111/j.0014-3820.2005.tb01806.x
Mathews, L. M. (2002). Tests of the mate-guarding hypothesis for social monogamy: does population density, sex ratio, or female synchrony affect behavior of male snapping shrimp (Alpheus angulatus)? Behav. Ecol. Sociobiol. 51, 426–432. doi: 10.1007/s00265-002-0465-3
Mathews, L. M. (2003). Tests of the mate-guarding hypothesis for social monogamy: male snapping shrimp prefer to associate with high-value females. Behav. Ecol. 14, 63–67. doi: 10.1093/beheco/14.1.63
May, R. M. (1990). How many species? Philosop. Transact. Royal Society B: Biological Sci. 330, 293–304. doi: 10.1098/rsta.1892.0001
Mcbride, R. S., Somarakis, S., Fitzhugh, G. R., Albert, A., Yaragina, N. A., Wuenschel, M. J., et al. (2015). Energy acquisition and allocation to egg production in relation to fish reproductive strategies. Fish Fish. 16, 23–57. doi: 10.1111/faf.12043
McGaughran, A., Torricelli, G., Carapelli, A., Frati, F., Stevens, M. I., Convey, P., et al. (2010). Contrasting phylogeographical patterns for springtails reflect different evolutionary histories between the Antarctic peninsula and continental Antarctica. J. Biogeogr. 37, 103–119. doi: 10.1111/j.1365-2699.2009.02178.x
Murua, H., and Saborido-Rey, F. (2003). Female reproductive strategies of marine fish species of the North Atlantic. J. Northwest Atl. Fish. Sci. 33, 23–31. doi: 10.2960/J.v33.a2
Mylonas, C. C., and Zohar, Y. (2000). Use of GnRHa-delivery systems for the control of reproduction in fish. Rev. Fish Biol. Fish. 10, 463–491. doi: 10.1023/A:1012279814708
Nagoshi, M., and Yanasigawa, Y. (1997). “Parental care patterns,” in Fish communities in Lake Tanganyika. eds. H. Kawanabe, M. Hori, and M. Nagoshi (Japan: Kyoto University Press).
Nelson, J. S., Grande, T. C., and Wilson, M. V. H. (2016). Fishes of the world (fifth). United States: John Wiley & Sons Ltd.
Opie, C., Atkinson, Q. D., Dunbar, R. I. M., and Shultz, S. (2013). Male infanticide leads to social monogamy in primates. Proc. Natl. Acad. Sci. 110, 13328–13332. doi: 10.1073/pnas.1307903110
Opie, C., Shultz, S., Atkinson, Q. D., Currie, T., and Mace, R. (2014). Phylogenetic reconstruction of bantu kinship challenges Main sequence theory of human social evolution. Proc. Natl. Acad. Sci. 111, 17414–17419. doi: 10.1073/pnas.1415744111
Pagel, M., and Meade, A. (1996). Bayesian analysis of correlated evolution of discrete characters by reversible-jump Markov chain Monte Carlo. Am. Nat. 167, 808–825.
Palanza, P., Della Seta, D., Ferrari, P. F., and Parmigiani, S. (2005). Female competition in wild house mice depends upon timing of female/male settlement and kinship between females. Anim. Behav. 69, 1259–1271. doi: 10.1016/j.anbehav.2004.09.014
Parker, G. A. (1970). Sperm competition and its evolutionary consequences in the insects. Biol. Rev. 45, 525–567. doi: 10.1111/j.1469-185X.1970.tb01176.x
Petrie, M. (1992). Copulation frequency in birds: why do females copulate more than once with the same male? Anim. Behav. 44, 790–792. doi: 10.1016/S0003-3472(05)80309-4
Pitnick, S., and Hosken, D. J. (2010). “Postcopulatory sexual selection in mammals,” in Evolutionary behavioral ecology. eds. D. F. Westneat and C. Fox (Oxford: Oxford University Press).
Potts, J. R., and Lewis, M. A. (2014). How do animal territories form and change? Lessons from 20 years of mechanistic modelling. Proc. R. Soc. B 281:231. doi: 10.1098/rspb.2014.0231
Pyron, M., Pitcher, T. E., and Jacquemin, S. J. (2013). Evolution of mating systems and sexual size dimorphism in north American cyprinids. Behav. Ecol. Sociobiol. 67, 747–756. doi: 10.1007/s00265-013-1498-5
Rabosky, D. L., Santini, F., Eastman, J., Smith, S. A., Sidlauskas, B., Chang, J., et al. (2013). Rates of speciation and morphological evolution are correlated across the largest vertebrate radiation. Nat. Commun. 4, 1–8. doi: 10.1038/ncomms2958
Reavis, R. H., and Barlow, G. W. (1998). Why is the coral-reef fish Valenciennea strigata (Gobiidae) monogamous? Behav. Ecol. Sociobiol. 43, 229–237. doi: 10.1007/s002650050487
Reichard, U. H. (2003). “Monogamy past and present,” in Monogamy: Mating strategies and partnerships in birds, humans and other mammals. eds. U. H. Reichard and C. Boesch (Cambridge: Cambridge University Press), 3–25.
Righton, D., Miller, M., and Ormond, R. (1998). Correlates of territory size in the butterflyfish Chaetodon austriacus (Ruppell). J. Exp. Mar. Biol. Ecol. 226, 183–193. doi: 10.1016/S0022-0981(97)00235-9
Roberts, C. M., and Ormond, R. F. G. (1992). Butterflyfish social behaviour, with special reference to the incidence of territoriality: a review. Environ. Biol. Fish 34, 79–93. doi: 10.1007/BF00004786
Rondeau, A., and Sainte-Maire, B. (2001). Variable mate-Gueding time and sperm allocation by male anow crabs (Chinonoecetes opilio) in responseto sexual competition, and their impact on the mating success of females. Biol.Bull 201, 204–217. doi: 10.2307/1543335
Rowley, A. G., Daly-Engel, T. S., and Fitzpatrick, J. L. (2019). Testes size increases with sperm competition risk and intensity in bony fish and sharks. Behav. Ecol. 30, 364–371. doi: 10.1093/beheco/ary174
Schärer, L., Rowe, L., and Arnqvist, G. (2012). Anisogamy, chance and the evolution of sex roles. Trends Ecol. Evol. 27, 260–264. doi: 10.1016/j.tree.2011.12.006
Schlupp, I. (2021). Male choice, female competition, and female ornaments in sexual selection. Oxford: Oxford University Press.
Schubert, M., Schradin, C., Rödel, H. G., Pillay, N., and Ribble, D. O. (2009). Male mate guarding in a socially monogamous mammal, the round-eared sengi: on costs and trade-offs. Behav. Ecol. Sociobiol. 64, 257–264. doi: 10.1007/s00265-009-0842-2
Sefc, K. M., Mattersdorfer, K., Sturmbauer, C., and Koblmüller, S. (2008). High frequency of multiple paternity in broods of a socially monogamous cichlid fish with biparental nest defence. Mol. Ecol. 17, 2531–2543. doi: 10.1111/j.1365-294X.2008.03763.x
Sogabe, A., and Ahnesjö, I. (2011). The ovarian structure and mode of egg production in two polygamous pipefishes: a link to mating pattern. J. Fish Biol. 78, 1833–1846. doi: 10.1111/j.1095-8649.2011.02973.x
Strona, G. (2014). Assessing fish vulnerability: IUCN vs FishBase. Aquat. Conserv. Mar. Freshwat. Ecosyst. 24, 153–154. doi: 10.1002/aqc.2439
Takemura, A., Rahman, M. S., and Park, Y. J. (2010). External and internal controls of lunar-related reproductive rhythms in fishes. J. Fish Biol. 76, 7–26. doi: 10.1111/j.1095-8649.2009.02481.x
Taylor, M. I., and Knight, M. E. (2008). “Mating Systems in Fishes,” in Fish reproduction. eds. M. J. Rocha, A. Arukwe, and B. G. Kapoor (United States: CRC Press), 277–310.
Tecot, S. R., Singletary, B., and Eadie, E. (2016). Why “monogamy” isn’t good enough. Am. J. Primatol. 78, 340–354. doi: 10.1002/ajp.22412
Thornhill, R., and Alcock, J. (1983). The evolution of insect mating systems. United States: Harvard University press.
Thorson, J. T., Cope, J. M., Patrick, W. S., Horson, J. A. T. T., and Ope, J. A. M. C. (2014). Assessing the quality of life history information in publicly available databases. Ecol. Appl. 24, 217–226. doi: 10.1890/12-1855.1
Thresher, R. E. (ed). (1980). Reef fish: Behaviour and ecology on the reef and in the aquarium. (Charleston, NC: Bartholomew & Son Ltd.).
Tricas, T. (1989). Determinants of feeding territory size in the coralivorous butterflyfish. Anim. Behav. 37, 830–841. doi: 10.1016/0003-3472(89)90067-5
Trivers, R. L. (1972). “Parental investment and sexual selection,” in Sexual selection and the descent of man. ed. B. Campbell (London: Heinemann Educational Books Ltd), 136–179.
Tumulty, J., Morales, V., and Summers, K. (2014). The biparental care hypothesis for the evolution of monogamy: experimental evidence in an amphibian. Behav. Ecol. 25, 262–270. doi: 10.1093/beheco/art116
Wallace, R. A., and Selman, K. (1981). Cellular and dynamic aspects of oocyte growth in Teleosts. Am. Zool. 21, 325–343. doi: 10.1093/icb/21.2.325
Whiteman, E. A., and Côté, I. M. (2004). Monogamy in marine fishes. Biol. Rev. 79, 351–375. doi: 10.1017/S1464793103006304
Wittenberger, J. F., and Tilson, R. L. (1980). The evolution of monogamy: hypotheses and evidence. Annu. Rev. Ecol. Syst. 11, 197–232. doi: 10.1146/annurev.es.11.110180.001213
Yabuta, S., and Berumen, M. L. (2014). “Social structures and spawning behavior of Chaetodon butterflyfishes,” in Biology of butterflyfishes. eds. M. S. Pratchett, M. L. Berumen, and B. G. Kapoor (United States: CRC Press), 200–225.
Yamamoto, M. E., Chellappa, S., Cacho, M. S. R. F., and Huntingford, F. A. (1999). Mate guarding in an Amazonian cichlid, Pterophyllum scalare. J. Fish Biol. 55, 888–891. doi: 10.1111/j.1095-8649.1999.tb00727.x
Yokoi, S., Okuyama, T., Kamei, Y., Naruse, K., Taniguchi, Y., Ansai, S., et al. (2015). An essential role of the arginine Vasotocin system in mate-guarding behaviors in triadic relationships of Medaka fish (Oryzias latipes). PLoS Genet. 11, 1–25. doi: 10.1371/journal.pgen.1005009
Keywords: cichlid, monogamy, mating system, biparental care hypothesis, mate guarding, female-female intolerance
Citation: Stanbrook E, Fitzpatrick JL, Balshine S and Shultz S (2022) The evolution of monogamy in cichlids and marine reef fishes. Front. Ecol. Evol. 10:1045383. doi: 10.3389/fevo.2022.1045383
Edited by:
Gergely Zachar, Semmelweis University, HungaryReviewed by:
Robin Dunbar, University of Oxford, United KingdomSheng-Feng Shen, Academia Sinica, Taiwan
Copyright © 2022 Stanbrook, Fitzpatrick, Balshine and Shultz. This is an open-access article distributed under the terms of the Creative Commons Attribution License (CC BY). The use, distribution or reproduction in other forums is permitted, provided the original author(s) and the copyright owner(s) are credited and that the original publication in this journal is cited, in accordance with accepted academic practice. No use, distribution or reproduction is permitted which does not comply with these terms.
*Correspondence: Emily Stanbrook, ✉ ZW1pbHkuYy5zdGFuYnJvb2tAZ21haWwuY29t