- 1Departamento de Biologia, Faculdade de Ciências, Universidade do Porto, Porto, Portugal
- 2CIBIO, Centro de Investigação em Biodiversidade e Recursos Genéticos, InBIO Laboratório Associado, Campus de Vairão, Universidade do Porto, Vairão, Portugal
- 3BIOPOLIS Program in Genomics, Biodiversity and Land Planning, CIBIO, Campus de Vairão, Vairão, Portugal
- 4Museum of Natural History of Funchal, RAM, Funchal, Portugal
- 5Department of Biology, University of Oxford, Oxford, United Kingdom
Understanding the trophic interactions of introduced predators is key for evidence-based management of biological invasions. This is particularly important in oceanic islands, where predator-prey networks often include numerous endemic and range-restricted species. Geckos are successful island colonizers and in recent years numerous species have established populations in a wide array of oceanic islands. One such species is the Moorish gecko (Tarentola mauritanica), which has colonized multiple islands across the Mediterranean basin, Caribbean and Macaronesia. The species was first reported in Madeira Island in 1993 and over the last 30 years has colonized most of the islands' southern coast and expanded to the nearby island of Porto Santo. Here, we used DNA metabarcoding to provide the first insights into the diet of this successful colonizer in its introduced range. The species' diet was mainly composed of ground-dwelling arthropods belonging to the families Porcellionidae (Isopoda), Julidae (Diplopoda) and Formicidae (Hymenoptera). The diet richness and composition were not affected by neither sex nor size of adult geckos, instead they both change across populations. However, trophic niche-width differed among size classes, with smaller geckos feeding on a wider range of prey. We identified over 160 different Operational Taxonomic Units in the diet of T. mauritanica, with 21.6% of them belonging to introduced invertebrates and 13.6% to native species. Native prey taxa included the endemic Madeira wall lizard (Teira dugesii), the sole native reptile to Madeira. We also detected several agricultural pests and disease vectors in the diet of this exotic predator, and 19 taxa identified as prey had not yet been recorded to Madeira. Of these, several are serious agricultural pests, highlighting how this introduced gecko can be used as a natural sampler, in particular for the early detection of invasive arthropod pests. This study emphasizes the importance of trophic studies for monitoring the impacts of introduced predators in fragile insular systems.
Introduction
Invasive species are a key threat to biodiversity at both the local, regional and global scales (Clavero and García-Berthou, 2005; McGeoch et al., 2010; Early et al., 2016). Their impact is particularly detrimental in oceanic islands, where they represent the main driver of population decline and extinction to numerous native taxa (Doherty et al., 2016). In fact, ca. 200 non-native reptile taxa were found to be established in at least one of 359 regions considered in a global analysis of reptile invasions, and the human-assisted expansion of reptiles to areas outside their native range was found to be accelerating, especially in islands (Capinha et al., 2017). Moreover, islands and coastal mainland regions are hotspots for the establishment of non-native species (Blackburn et al., 2016; Dawson et al., 2017), often hosting multiple non-native reptiles (Pitt et al., 2005), a group that is among the most successful and abundant vertebrates in small islands (Novosolov et al., 2016). Since insular ecosystems are generally unbalanced (often harboring a disproportionate number of empty niches compared to similar mainland areas), and various insular native species have evolved without predators, competitors or parasites, islands seem to be more susceptible to herpetofaunal invasion (Whittaker and Fernández-Palacios, 2007).
Advances in high-throughput sequencing and DNA metabarcoding allow for the simultaneous analysis of the diet of hundreds of animals from low quality/quantity eDNA in fecal or stomach contents (Taberlet et al., 2012; Alberdi et al., 2019; Ando et al., 2020). Molecular scatology studies offer important insights into the demographic effects of a predator over different types of prey and sometimes allow for the identification of potential suppressors of agricultural pests and disease vectors (e.g., Kemp et al., 2019; Tiede et al., 2020). Compared to morphology-based diet analysis, molecular techniques offer a higher resolution of an animal's diet (Gil et al., 2020) and, in the case of invasive predators, it offers a promising, non-invasive approach to assessing the prey base and consequently, impact of non-native species (Plimpton et al., 2021). These studies are particularly important in insular systems, where predator-prey networks might often include numerous endemic species, many of which with soft bodies that often go undetected in morphology-based studies.
Geckos are one of the most frequently introduced reptiles, with several species known to have successfully established new populations outside their native ranges, often with adverse ecological consequences (Hoskin, 2011; Buckland et al., 2014; Perella and Behm, 2020). Yet, although voracious predators, studies targeting the diet of introduced geckos are rare, especially those under a genomic approach (but see e.g., Cummings et al., 2021; Lamb et al., 2021; Romero-Cordero, 2021). Although some studies have used DNA metabarcoding to investigate the diet of insular geckos (Pinho et al., 2018; Lopes et al., 2019; e.g., Gil et al., 2020), these studies are predominantly from islands without continuous human occupation. Accordingly, while able to highlight the potential of geckos as sentinels of previously unrecorded species (see e.g., Santos et al., 2022), they did not focus on the assessment of their potential consumption of agricultural pests or human disease vectors.
The Moorish gecko, Tarentola mauritanica (Linnaeus, 1758), a complex of species encompassing six putative distinct taxa (Rato et al., 2016), is widespread throughout North Africa, most of the Iberian and Italian peninsulas and multiple other Mediterranean coastal regions (Vogrin et al., 2017). However, due to its frequent association to human settlements and capacity for long-distance dispersal, it has been introduced in multiple mainland and island regions across the Mediterranean basin, Macaronesia, Caribbean as well as North and South America (Carranza et al., 2000; Harris et al., 2004a,b). Although T. mauritanica is perceived as a voracious crepuscular predator (Martínez-Rica, 1974), the only studies devoted to the species' diet are based on morphological scatology of Spanish populations (Gil et al., 1994; Hódar et al., 2006). Hence, the prey base of T. mauritanica is unknown throughout much of the species' range, including the island ecosystems to which it has been introduced. However, some other dietary genomic studies have already assessed the diet of other species of insular Tarentola, such as T. gigas from Cabo Verde (Pinho et al., 2018; Lopes et al., 2019), and T. (boettgeri) bischoffi from Selvagens (Gil et al., 2020). Both species were found to have a generalist diet, feeding on vertebrates, invertebrates, and plants. The same generalist diet is also observed in T. mauritanica (Gil et al., 1994; Hódar et al., 2006).
In Madeira Island, T. mauritanica was first reported by Báez and Biscoito (1993) in a small locality, Garajau, 8 km east of Funchal but it now inhabits most of the island's south coast (Rato et al., 2021; Silva-Rocha et al., 2022). Furthermore, it has colonized the nearby island of Porto Santo (Jesus et al., 2008) and, according to its bioclimatic and environmental affinities, it is likely to further expand its range in Madeira, including some areas of the northern coast of the island, where it has not yet been recorded (Silva-Rocha et al., 2022).
Due to differences in size, sexes and ontogenic stages in ectothermic species, these groups often occupy singular ecological niches, with distinct food sources, and selective pressures (Werner and Gilliam, 1984; Bowler and Terblanche, 2008). This often translates into within-species differences in phenotypic traits and to shifts in the types and characteristics (e.g., size, hardness and locomotion) of food consumed. In T. mauritanica, variation in bite force is mostly determined by size variation and sexual dimorphism (Massetti et al., 2017, 2018). Additionally, differences in speed locomotion are also related to size and sex (Oliveira et al., 2022). Hence, it is expected that different size classes and sexes of T. mauritanica might have distinct diet compositions.
Here, we provide the first assessment of the diet of T. mauritanica under a DNA metabarcoding framework. Based on fecal samples collected in Madeira, we assess how niche width, diet composition, and prey richness are affected by sex, population and size. We also investigate if this introduced lizard is consuming (i) endemic species of particular conservation concern, (ii) economically relevant agricultural pests, or (iii) arthropods that may act as vectors of zoonotic human or domestic animal diseases. We anticipate that T. mauritanica is likely to have a diverse diet, consuming numerous native and non-native species to Madeira. We predict that due to the species' sexual dimorphism, males and females are likely to exhibit different diets. Furthermore, we hypothesize that smaller animals are likely to have a more diverse diet, since their forced exploration of suboptimal niches will most likely lead to a less selective diet. Lastly, considering the limited knowledge about the entomofauna of the island we predict that previously unrecorded species to Madeira might be detected on the species' diet.
Materials and methods
Study area
The Madeira archipelago is an autonomous region of Portugal located in the Atlantic Ocean, circa 650 km of north-western Africa (Figure 1). Madeira's climate is conditioned by its complex relief, configuration, and orientation (Santos, 2018), possessing a diverse spatial distribution of land use and landscape management, with several types of interleaved habitats in a short area. Banana plantations and vineyards occupy most of the island's agricultural area, and both crops are known to be affected by a large array of invertebrate pests (Ribeiro et al., 2009). Madeira was an important center for commerce connecting Europe to the New World and as such many animals and plant species were introduced to the archipelago, among which were new competitors, predators and parasites (Press, 1993; Wetterer et al., 2007; Seixas et al., 2019; Clemens and Allain, 2020; Rocha et al., 2020; Rato et al., 2021).
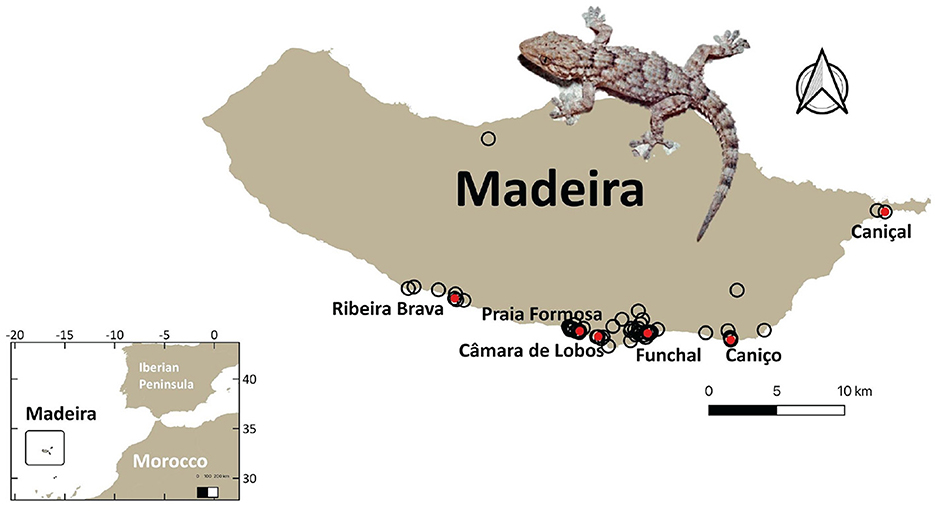
Figure 1. Known distribution records of the Moorish gecko Tarentola mauritanica in Madeira Island represented by black hollow circles (retrieved from the Global Biodiversity Information Facility (GBIF) database, www.gbif.org), and sampling sites depicted with red circles. The inset map denotes the geographic location of Madeira Island.
Sampling
Fieldwork took place in September 2020. Six localities throughout the southern coast of Madeira were sampled (Ribeira Brava, Praia Formosa, Câmara de Lobos, Funchal, Caniço and Caniçal; Figure 1), all corresponding to urban or peri-urban humanized habitats. Sampling was performed at night (from 8 p.m. to 1 a.m.) to match the species' typical crepuscular activity pattern (Luiselli and Capizzi, 1999; Hódar et al., 2006; Mori and Plebani, 2012; Fulgione et al., 2019), also observed in Madeira.
Geckos were captured by hand or noose and received a belly massage to release fresh pellets. All fecal samples were preserved in 96% ethanol until DNA extraction was performed. A total of 150 pellets of T. mauritanica (135 adults and 15 juveniles), were collected during fieldwork: 23 in Ribeira Brava, 26 in Praia Formosa, 31 in Câmara de Lobos, 23 in Funchal, 24 in Caniço, and 23 in Caniçal. Additionally, adult individuals were sexed by inspecting for the presence of hemipenes in males, either by eye or with the help of a laser light placed against the skin dorsally to the cloaca (following Atzori et al., 2007), resulting in 55 males and 80 females. The snout-vent length (SVL) of each individual was measured to the closest 0.01 mm using an electronic caliper, and the weight was obtained using a digital scale. All measurements were taken by the same person (C. Rato) to eliminate inter-observer error. Sampling permits and protocols were approved by the Madeiran delegation from the Instituto para a Conservação da Natureza e Florestas (ICNF), described in the acknowledgments section.
DNA extraction and amplification
From the 150 T. mauritanica pellets collected, only 127 (117 adults; 10 juveniles), corresponding to 45 males and 72 females, were used, as the remaining ones had only uric acid material with nearly no diet content. First, a major part of the ethanol was extracted from the tubes using appropriate micropipettes, followed by a dehydration in an incubator at 50°C for 24 h to remove the remaining ethanol (following Pinho et al., 2018). After that, DNA from ~200 mg of each T. mauritanica fecal sample was extracted using the Stool DNA Isolation Kit (Norgen Biotek Corp., Thorold, ON, Canada) following the manufacturer's guidelines. Two 50 μL elutions from each pellet, including eight extraction control samples, were obtained, and stored at −20°C in 96-well plates until amplification. A short fragment (205 bp) of the mitochondrial cytochrome c oxidase subunit I (COI) was amplified by Polymerase Chain Reaction (PCR) using the FwhF2-R2n primers from Vamos et al. (2017). These were modified to include Illumina adaptors and a 0–5 bp shift made of Ns was added between the adaptor and the primer to increase sequencing diversity and quality. The six primer variations were then combined before PCR reactions, resulting in mixed forward and reverse primer single solutions. The PCR reaction was comprised by 5 μL of QIAGEN Multiplex PCR Master Mix (Qiagen, Crawley, UK), 0.3 μL mix of forward primers, 0.3 μL mix of reverse primers, 3.4 μL of ultra-pure water, and 0.1 ng of DNA extract. To obtain as much information as possible from each scat, three PCR replicates were performed per fecal sample. Cycling conditions consisted in an initial denaturation step at 95°C for 15 min, followed by 45 cycles of 95°C denaturing for 30 s, annealing at 52°C for 45 s, extension at 72°C for 20 s, and a final extension at 60°C for 5 min. Success of all amplifications was checked by running the PCR products in 2% agarose gels.
Library preparation
The library preparation started with an initial PCR clean-up using Agencourt AMPure XP beads (Beckman Coulter, Brea, CA, USA) to remove primer dimer, followed by an indexing PCR to properly identify each amplified product. Indexing PCR was performed using 2.8 μL of ultra-pure water, 7 μL of 2 × Kapa HiFi, 0.7 μL of each Index (P7/P5), and 2.8 μL of cleaned PCR product. This was followed by the subsequent cycling conditions: initial denaturation of 95°C for 3 min, following 9 cycles of 95°C for 30 s, annealing at 55°C for 30 s, extension at 72°C for 30 s, and a final extension of 72°C for 5 min. PCR products underwent a second bead clean-up to remove remaining primer dimer, nucleotides, and enzymes that might interfere with the sequencing reaction.
Succeeding the mentioned steps, all cleaned diet samples were quantified using Epoch, and Nanodrop for confirmation, followed by a normalization to 20 nM and pool sampling. The final pool was diluted to 4 nM, tested for quality control in a TapeStation, and its concentration validated using qPCR. The library was run in a MiSeq sequencer (Illumina) using a MiSeq Reagent Kit v2 (Illumina, San Diego, CA, USA) for 2 × 250 bp target length and an expected average of 25.000 paired-end reads per sample.
Bioinformatics
Forward and reverse sequences were aligned using PEAR (Zhang et al., 2014), where base-pairs with q-scores lower than 26 were rejected. Afterwards, primer sequences were removed, and sample information was added to each assembled read using the “ngsfilter” command from OBITools (Boyer et al., 2016). Reads were then de-replicated into unique sequences (command “obiuniq”) and merged into a single fasta file. Finally, VSEARCH (Rognes et al., 2016) was used to denoise the dataset, remove chimeric sequences, cluster sequences into Operating Taxonomic Units (OTUs) of 99% similarity, and map raw reads to the resulting OTUs. The obtained OTU table and sequences were further cleaned using the R package LULU (Frøslev et al., 2017) to remove potential mtDNA nuclear copies and persisting PCR and sequencing errors.
Taxonomic assignment of OTUs was done using BOLDigger (Buchner and Leese, 2020) followed by manual inspection and curation, along with manual BLAST of some sequences on NCBI when species-level assignments were not possible with BOLD. The sequences with <90% of similarity were classified only to the class level, the ones with similarity between 90 and 95% were classified to the family level, and sequences presenting more than 95% of similarity were classified to the species or genus level (if sequences matched with several species of the same genus, the OTU was assigned to the genus level). In case of match with multiple genera or species, OTUs were assigned considering the species record in Madeira (Borges et al., 2008). After this step, all reads from OTUs identified in the eight extraction controls were subtracted from the corresponding sample batch (Evans et al., 2021), singletons (haplotypes with only one read) and replicates with fewer than 100 reads were discarded, and only OTUs present in at least two of the three replicates were maintained. Furthermore, OTUs identified as taxa unlikely to have been actively predated by T. mauritanica such as fungi, bacteria, and parasites, as well as known lab contaminations, such as humans (Homo sapiens) and pig (Sus scrofa), were not considered for further analysis.
Statistical analyses
Adult geckos were allocated to three size classes according to their SVL (small: 50.5–60.9 mm; medium: 60.9–71.3 mm; and large: 71.3–92.1 mm). Juveniles were not included in the analysis due to their small sample size. Statistical analyses were performed in R v.4.1.0 (R Core Team, 2021) and all figures were produced using the package ggplot2 v. 3.3.1 (Villanueva and Chen, 2019), unless stated otherwise.
Generalized Linear Models (GLMs) were used to estimate the effects of sex, population and size on individual prey richness (number of distinct prey taxa, i.e., OTUs, recorded per sample). Given that the data was not normally distributed (Shapiro-Wilk normality test: W = 0.942, P < 0.001), we fit a GLM with Poisson distribution using the function “lmer” from the package lme4 v.1.1-27.1 (Bates et al., 2014). Statistical significance of the GLM model was tested with a likelihood ratio test, using the function “anova” from the package car v.3.0-12 (Fox et al., 2012). Pairwise comparisons were performed with the function glht (Tukey test) from the package multcomp (Hothorn et al., 2008).
Permutational multivariate analysis of variance (PERMANOVA) was used to compare the diet composition between sexes, populations and size classes with the vegan R package (function “adonis”; Oksanen et al., 2013). Presence or absence of each prey OTU in each sample was used to build a Jaccard dissimilarity matrix using the “vegdist” function from the R package vegan (Oksanen et al., 2013). A homogeneity of dispersion test (PERMDISP) was also carried out to assess if the observed differences in PERMANOVA could be due to unequally dispersed values across the different groups (Anderson, 2006).
To calculate the diet niche-width of both sexes, populations and of the different size classes, prey rarefaction and extrapolation curves were built using the R package iNEXT v. 2.0.20 (Hsieh et al., 2016). Analyses were conducted with incidence frequencies for prey taxa.
Finally, a bipartite network was built using the package “bipartite” (Dormann et al., 2009) to represent the interactions between each gecko size class and their prey. Each interaction was further colored depending on whether the prey was known to be “native,” “introduced,” or of “unknown origin” (Borges et al., 2008; Aguiar et al., 2013; De Jong et al., 2014).
Results
Data filtering
The libraries generated ca. 14.7 million raw sequence reads, which were reduced to 10,867,717 reads during bioinformatic processing and 1,095 OTUs. Non-target amplification from different sources was observed both in samples, extractions, and PCR negative controls. Although representing 18.7% of the total reads, fungi, mainly ascomycota, represented most of the non-target OTU diversity (50.8%). An expected amount of T. mauritanica was observed as well, corresponding to 8.2% of read counts. Curiously, in 10 of the samples (4 adult females, and 6 adult males) we found the presence of two distinct haplotypes of T. mauritanica. After negative controls, singletons, replicates, and taxa filtering, the gecko's final diet dataset consisted in 6,851,212 reads from 127 samples and encompassed 162 OTUs (92 assigned to species-level).
Diet
From a total of 162 prey items detected, seven classes of Arthropoda (Arachnida, Chilopoda, Collembola, Diplopoda, Insecta and Malacostraca), one class of Mollusca (Gastropoda), and one class of Chordata (Reptilia), were identified (Supplementary Table S2). These encompassed at least 22 distinct orders, 74 families and 101 genera. In general, Porcellionidae, Julidae and Formicidae were the most frequent families in the diet of T. mauritanica, across almost all sampled populations (Figure 3).
The GLM analysis, indicated that neither sex (LR χ2 = 0.305; DF = 1; P-value = 0.581) nor SVL (LR χ2 = 0.028; DF = 1; P-value = 0.8866) showed an effect on the number of prey items consumed by T. mauritanica geckos, but richness was significantly different among populations (LR χ2 = 31.237; DF = 5; P-value < 0.001), with Caniçal and Caniço presenting the highest values of richness in contrast to Câmara de Lobos and Ribeira Brava with the lowest (Supplementary Figure S1). Nevertheless, none of the pairwise comparisons between population were statistically significant. Likewise, diet composition was not significantly different between males and females, nor among distinct size classes. However, diet composition was found to differ between populations (Table 1). Furthermore, the non-significance of the homogeneity of dispersion test (df = 5; SS = 0.027; Mean SS = 0.005; pseudo-F = 2.074; P-value = 0.074) supports this differentiation among populations.
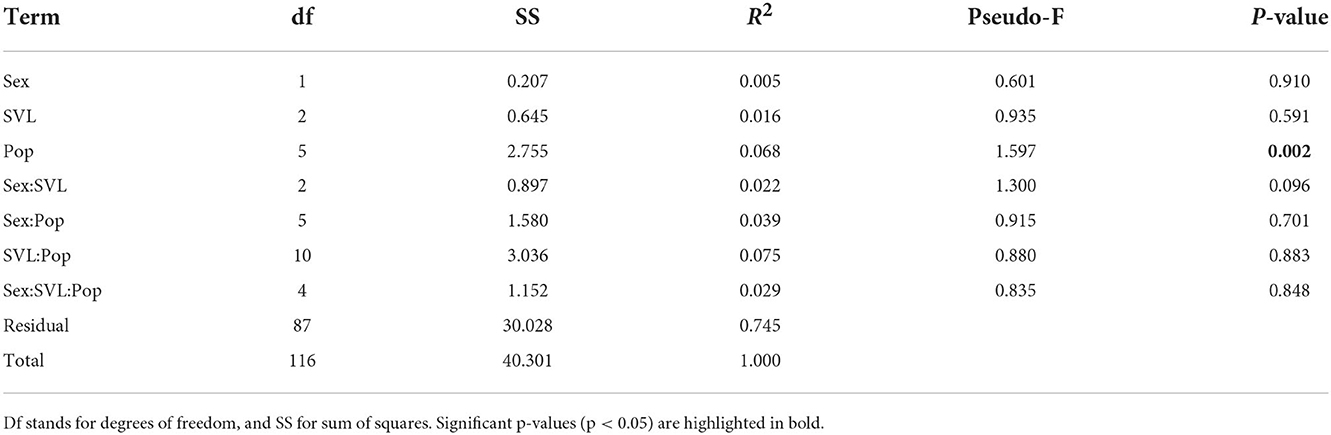
Table 1. PERMANOVA results of the effects of sex, population, and snout-vent length (SVL) on the diet composition of Tarentola mauritanica.
From the analysis of the rarefaction and extrapolation curves (Figure 2), populations, as well as females and males seem to feed on a similar range of prey items (females: 93–109; males: 91–112). Moreover, smaller geckos presented a wider trophic niche than larger ones, with a richness of 101 (90–113) prey items, in contrast with the 83 (77–90) and 60 (54–66) prey items in medium and large animals, respectively. For all rarefaction analysis, the minimum observed sample coverage was above 85%.
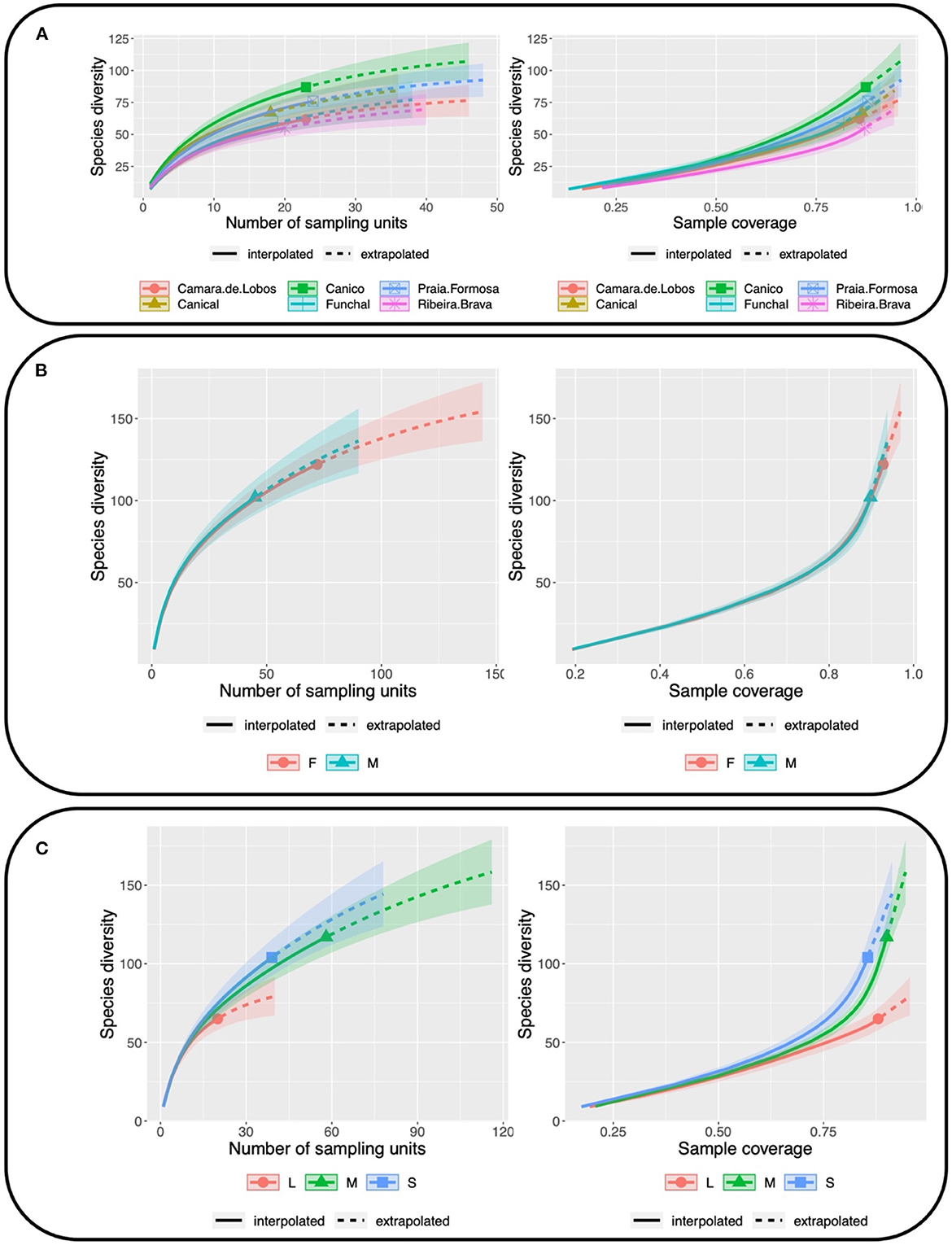
Figure 2. Diet rarefaction/extrapolation curves for (A) Populations, (B) sex (M, male; F, female) and (C) size (S, small; M, medium; L, large), obtained according to the number of sampling units (left) and sample coverage (right) using iNEXT package from R.
Out of the 92 species identified to be consumed by T. mauritanica (Supplementary Table S2), 21 are known to be native to Madeira representing ca. 3.1% of the gecko's diet (Figure 3). Most of these native species do not yet have their conservation status assessed. The exceptions to this, namely Aiolopus thalassinus thalassinus, Amegilla quadrifasciata maderae and the lizard Teira dugesii are listed as Least Concern (LC). The latter, T. dugesii, is the only native reptile species of the Madeira archipelago and was detected in 26.8% of the samples. Moreover, out of the 92 identified species, 19 of these taxa have not yet been recorded in Madeira. Although most of these new taxa occur in relatively low frequency in the samples (between 0.79 and 11.81%), the ant Nylanderia jaegerskioeldi, the pill-bug Armadillidium vulgare, and the woodlouse Porcellionides pruinosus appear in more than 20% of the scats. Species classified as introduced encompassed 31.7% of the diet of the Moorish gecko, with the species Ommatoiulus moreleti (63.8%), Periplaneta americana (30.7%) and Xystrologa grenadella (27.6%) being the most frequently detected across samples (see Figure 3). In fact, the millipede Ommatoiulus moreleti was the most consumed prey by T. mauritanica.
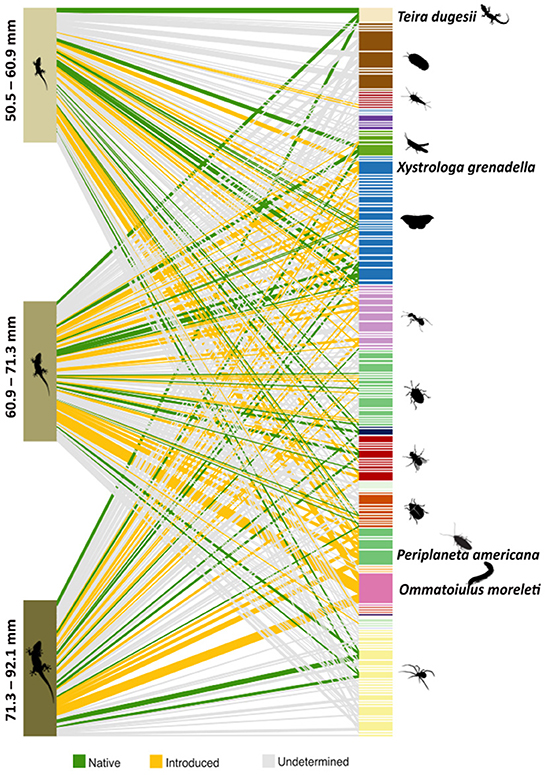
Figure 3. Frequency of occurrence network showing the Operating Taxonomic Units (OTUs) ingested by the different size classes of Tarentola mauritanica. Size interval (in mm) of each class is represented to the left of the figure. White lines separate the different OTUs, and the colouration is according to their taxonomic Order. The figure also depicts the most consumed introduced species (Ommatoiulus moreleti, Periplaneta Americana, and Xystrologa grenadella), and families (Porcellionidae, Formicidae, and Julidae).
We identified multiple species of agricultural insect pests as prey of T. mauritanica in Madeira—e.g., Paratrechina longicornis, Nezara viridula, Aphis gossypii, Viteus vitifoliae, Opogona sacchari, Sophonia orientalis, and Linepithema humile (Aguiar, 2005; Aguin-Pombo et al., 2007; Ribeiro et al., 2009). Furthermore, although we did not record the dengue vector Aedes aegypti—that in Madeira's south coast is currently sympatric to T. mauritanica—to be a prey of T. mauritanica, we detected Culex theileri, a known vector of several diseases such as the dog heartworm.
Discussion
This study provides the first metabarcoding assessment of the diet of T. mauritanica and is one of the first to use this technique to evaluate the diet of a non-native reptile in an oceanic island. We detected 162 prey items, including seven different classes of invertebrates, and the only native reptile to Madeira, the Madeira wall lizard Teira dugesii. The presence of two distinct haplotypes of T. mauritanica in 10 of the scat samples, may provide evidence that one of the haplotypes is associated with a T. mauritanica that might have felt prey to a conspecific gecko, to which the second haplotype belongs to. This suggests possible cannibalism and aligns with extensive previous observational evidence of cannibalism upon juveniles of T. mauritanica (e.g., Franco, 1980; González de la Vega, 1988; Salvador, 2016). Moreover, T. mauritanica is an extremely territorial species (Lozano-Del Campo and García-Roa, 2014; Martín et al., 2018), and antagonistic interspecific interactions are very common. Although it is hard to assess which kind of behavior led to these findings, this study is indeed the first DNA- based contribution to the topic.
We found that neither sex nor size influenced the diet richness or composition (Table 1). Likewise, Gil et al. (1994) found that the diet overlap between age and sex classes of T. mauritanica was generally high, for both prey size and type. Tarentola mauritanica has marked sexual dimorphism, with males being larger than females and able to bite harder (Massetti et al., 2017, 2018). Yet, although diet was expected to differ between sexes—as males have the capacity to feed on larger and harder prey—we found no sex-related differences in diet composition. This might reflect that the observed phenotypic sexual dimorphism in T. mauritanica might be mostly associated with male-male antagonism and mate acquisition, a known driver of sexual selection (e.g., Herrel et al., 1999a,b; Lappin and Husak, 2005; Husak and Fox, 2006; Husak et al., 2009). On the other hand, GLM results suggest that diet richness differs between populations (Table 1), likely reflecting spatial differences in prey availability (but see Borges et al., 2008).
We found that smaller animals have a wider niche than larger individuals (Figure 2). Tarentola mauritanica is extremely hierarchical, territorial and philopatric, with larger individuals selecting the best refugia and the not-so-far-away surrounding and top resources (wMartín et al., 2014; Martín et al., 2018). Hence, this spatial niche segregation might be inducing differences in trophic niche width among age classes, with smaller animals exploring suboptimal areas with poorer food resources, and where they cannot afford to be more selective.
Our findings are in line with other dietary studies based mainly on morphological examinations (Gil et al., 1994; Hódar et al., 2006), as well as with other recent metabarcoding studies targeting Tarentola geckos from the Cabo Verde and Selvagens archipelagos (Pinho et al., 2018; Gil et al., 2020). All studies highlight the generalist diet of the genus Tarentola, which is also corroborated in this study. Gil et al. (1994) and Hódar et al. (2006) identified coleopterans, arachnids, hymenopterans, lepidopterans and insect larvae as the most important groups in the diet of T. mauritanica. On the other hand, prey items such as oligochaetes, mites, springtails, aphids, termites, reptiles and fruits, were scarce. Morphology-based diet studies tend to underestimate soft-bodied prey incidence since only partially undigested items from soft body prey can be detected (Ingerson-Mahar, 2002), a limitation not affecting metabarcoding studies. Thus, although the results cannot be compared in a straightforward way, the diet composition in all studies was almost exclusively composed by arthropods, with Insecta representing the predominant class in all studies. Additionally, our metabarcoding study allowed for higher taxonomic resolution than morphology-based diet studies, with most prey items identified to the species-level (Supplementary Table S2).
The predominance of Porcellionidae, Julidae and Formicidae in the diet composition (Figure 3), can either indicate that these taxa are particularly abundant, or maybe reflect a genuine preference of T. mauritanica for these families compared to other prey in Madeira. Irrespective of the sampling location, Ommatoiulus moreleti (Family Julidae), a millipede native to the western Iberian Peninsula (Bailey and Mendonça, 1990), was the most consumed prey. In both their native range and in Madeira, T. mauritanica and O. moreleti overlap in distribution (Baker, 1984). Moreover, in Madeira, T. mauritanica is selecting more Mediterranean-like climates, which are more similar to the ones in its native range (Silva-Rocha et al., 2022). Hence, the detection of O. moreleti across most populations is an indication that this millipede is available in all of them, but its preference as prey could mean that apart from selecting Mediterranean environmental areas, the populations of T. mauritanica in Madeira are also choosing prey from their native geographic range.
Most identified prey groups comprised ground-dwelling arthropods (e.g., Julidae, Porcellionidae and Formicidae). These might be indicative that foraging can also take place on the ground, going in line with the previous research from Sánchez-Piñero (1994) and Hódar and Pleguezuelos (1999). Nonetheless, this strategy has only been observed in Mediterranean arid areas, where the availability of prey is lower, forcing the species to forage actively on the ground instead of the “sit-and-wait” behavior typical of this gecko (Perry and Werner, 1981; Ananjeva and Tsellarius, 1986). Another possible explanation, albeit unlikely, could be that flying invertebrates are feeding on ground-dwelling arthropods, and both prey levels are simultaneously amplified and identified as prey items of T. mauritanica. Nevertheless, the strong presence of some Lepidoptera families such as Cosmopterigidae, Tinidae and Blastobasidae also indicates that these geckos are not just ground feeders, foraging on the walls and cliffs, and possibly being attracted to artificial lights at night-time (Martín et al., 2018).
Our results revealed that the diet of T. mauritanica includes multiple species endemic to Madeira, such as the lizard Teira dugesii (26.8% of samples), and several arthropods. Yet, the relatively small proportion (ca. 3.1%) of endemic taxa detected as prey was unexpected, considering that over 979 arthropods species are endemic to Madeira (Borges et al., 2008). The predation on other lizards (dead or alive) was also observed in T. gigas from Cabo Verde (Pinho et al., 2018; Lopes et al., 2019), and in Tarentola (boettgeri) bischoffi from Selvagens (Gil et al., 2020), evidencing how common this might be on island systems where resources are more limited. Nevertheless, the metabarcoding approach allows only to have a qualitative perspective on the taxa T. mauritanica is feeding on, and not the quantity consumed (Pompanon et al., 2012). Therefore, even if only 3.1% of the diet is composed by endemic species, it is not possible to draw conclusions regarding the impact of T. mauritanica on these groups. Moreover, because this study reveals the dietary behavior of T. mauritanica during a single season, it is unclear if both diet richness and composition might change throughout the year (as observed in Gil et al., 1994), including the percentage of endemic prey taxa. Nevertheless, the ecological niche models of habitat suitability predict an expansion of T. mauritanica in Madeira island (Silva-Rocha et al., 2022), which could lead to a temporal shift of its diet composition and, consequently, on the impact on endemic fauna.
As global trade and international traveling increased over the last decades, so does the number of species establishing populations outside their native range (Holmes et al., 2009; Roques, 2010). Since invasive alien insects are often detrimental to agriculture, horticulture and forestry (Pimentel et al., 2005; Vilà et al., 2010), their timely detection is key for effective integrated pest management. Therefore, methods that provide rapid and accurate pest detection can aid deploying effective management strategies. In this context, metabarcoding techniques, combined with generalist, relatively abundant and easy to sample predators such as synanthropic geckos, can be used to maximize sampling resolution of non-native arthropods across both space and time (see Maslo et al., 2017; Montauban et al., 2021). As far as we know, no studies have yet explored the use of reptiles as early warning species for pest/disease vector species, a position for which T. mauritanica seems to be a good candidate. Thus, the discovery of 19 arthropod species not previously recorded in Madeira (Supplementary Table S2), many of them cataloged as agricultural pests, brings additional novelty to our study. Among these newly detected taxa, the ones already classified as serious agricultural pests elsewhere are Armadillidium vulgare (Douglas et al., 2017), and Frankliniella tritici (Funderburk et al., 2009, 2018; Cluever and Smith, 2016). Clearly, the lack of studies focused on the invertebrate fauna of the Madeira archipelago (but see e.g., Borges et al., 2008; Aguiar et al., 2013) might be the reason why these species are unknown to the region. In that sense, the results from this study should be used as reference for new monitoring of the entomofauna of the Madeira archipelago. Additionally, we found that T. mauritanica is feeding on Culex theileri, a mammophilic mosquito, widely distributed across Europe, North Africa, and southwest Asia and vector for several zoonotic diseases (Demirci et al., 2012). This species is a competent vector of human and domestic animal pathogens, caused by Wuchereria bancrofti (main cause of lymphatic filariasis) and Dirofilaria immitis (the dog heartworm), respectively (Demirci et al., 2012, 2014; Silva et al., 2014). In fact, C. theileri is likely the main vector of the dog heartworm disease in Madeira, which is estimated to affect 30% of the island's canines (Clemente, 1996; Santa-Ana et al., 2006).
Conclusion
Without proper estimates of the abundance of T. mauritanica and of its multiple prey species, it is hard to predict the possible impacts of this introduced gecko on the endemic fauna of Madeira. Moreover, the habitat suitability of T. mauritanica is predicted to increase in the future (Silva-Rocha et al., 2022), which will likely affect the species' trophic dynamics. Yet, this study indicates that, similarly to other species of the genus, T. mauritanica has a generalist diet, a characteristic that in combination with molecular diet analysis may allow for the early detection of invasive crop pests and other problematic arthropods. The identification of multiple agricultural pests in the diet of T. mauritanica indicates that it might act as a potential sampler and early warner of invasive pests. DNA metabarcoding offers a promising window into the predator-prey relationships of oceanic islands, which will certainly catalyse new avenues of ecological research in these increasingly threatened ecosystems.
Data availability statement
The datasets presented in this study can be found in online repositories. The names of the repository/repositories and accession number(s) can be found below: https://www.ebi.ac.uk/ena, PRJEB56502; https://figshare.com/s/e1f5965b36e74daf8e85; and https://figshare.com/s/0c2489cc82af48eec617.
Ethics statement
The animal study was reviewed and approved by Committee of Animal Experimentation of the University of Porto (Portugal) under the Directive 2010/63/EU of the European Parliament.
Author contributions
Conceptualization and supervision: CR and IS-R. Methodology: CR, BM, IS-R, RR, YG, and VM. Writing—original draft preparation: BM. Writing—review and editing: CR, IS-R, RR, YG, and VM. Project administration and funding acquisition: CR. All authors have approved the submitted version of this manuscript.
Funding
CR was supported by a postdoctoral contract from Foundation for Science and Technology (FCT), Portugal (DL57/2016/CP1440/CT0005). VM and RR by FCT through the program Stimulus of Scientific Employment, Individual Support—3rd Edition (contracts 2020.02547.CEECIND and 2020.01129.CEECIND, respectively). RR was further supported by a postdoctoral fellowship from ARDITI—Madeira's Regional Agency for the Development of Research, Technology and Innovation (M1420-09-5369-FSE-000002). Field and lab work was co-funded via national funds through FCT and EU funds European Regional Development Fund (ERDF) under the project PTDC/BIA-EVL/27958/2017—POCI-01-0145-FEDER-027958 (to CR). Work co-funded by the project NORTE-01-0246-FEDER-000063, supported by Norte Portugal Regional Operational Programme (NORTE2020), under the PORTUGAL 2020 Partnership Agreement, through the European Regional Development Fund (ERDF).
Acknowledgments
The authors would like to thank António Aguiar for providing crucial information, not yet published, regarding arthropods in Madeira Island. Specimens' collection and tissue sample transport were performed under the permits 05/IFCN/2020 and 03/IFCN/2020 provided by the Forests and Nature Conservation Institute from the Madeiran Autonomous Region.
Conflict of interest
YG was employed by Museum of Natural History of Funchal, RAM.
The remaining authors declare that the research was conducted in the absence of any commercial or financial relationships that could be construed as a potential conflict of interest.
Publisher's note
All claims expressed in this article are solely those of the authors and do not necessarily represent those of their affiliated organizations, or those of the publisher, the editors and the reviewers. Any product that may be evaluated in this article, or claim that may be made by its manufacturer, is not guaranteed or endorsed by the publisher.
Supplementary material
The Supplementary Material for this article can be found online at: https://www.frontiersin.org/articles/10.3389/fevo.2022.1044230/full#supplementary-material
Supplementary Table 1. Information about the T. mauritanica individuals from Madeira Island, used in this study.
Supplementary Figure 1. Graphical representation of the General Linear Model results denoting the statistically significant relationships between populations and richness. The y-axis represents the fitted values of the model, i.e., the sum of the estimated fixed effect coefficient and the predicted random intercept (see text for exact P-value).
References
Aguiar, A., Ilharco, F., Khadem, M., and Moreira, M. (2013). New records of aphids (Hemiptera: Aphidoidea) from Madeira and Azores archipelagos. Entomol. Monthly Magazine 149, 235–254.
Aguiar, A. M. F. (2005). “Exotic arthropod fruit pests and parasitoids found in the Archipelago of Madeira, Portugal since 1990,” in Actas do I Workshop de Fruticultura–Contributo para o seu desenvolvimento (Angra do Heroísmo),89–100.
Aguin-Pombo, D., Aguiar, A. F., and Kuznetsova, V. (2007). Bionomics and taxonomy of leafhopper Sophonia orientalis (Homoptera: Cicadellidae), a Pacific pest species in the Macaronesian Archipelagos. Ann. Entomol. Soc. Am. 100, 19–26. doi: 10.1603/0013-8746(2007)10019:BATOLS2.0.CO
Alberdi, A., Aizpurua, O., Bohmann, K., Gopalakrishnan, S., Lynggaard, C., Nielsen, M., et al. (2019). Promises and pitfalls of using high-throughput sequencing for diet analysis. Mol. Ecol. Resour. 19, 327–348. doi: 10.1111/1755-0998.12960
Ananjeva, N. B., and Tsellarius, A. Y. (1986). “On the factors determining desert lizards' diet,” in Studies in Herpetology. Proc. Eur. Herpetol. Meeting (Prague: Charles University, Prague), 445–448.
Anderson, M. J. (2006). Distance-based tests for homogeneity of multivariate dispersions. Biometrics 62, 245–253. doi: 10.1111/j.1541-0420.2005.00440.x
Ando, H., Mukai, H., Komura, T., Dewi, T., Ando, M., and Isagi, Y. (2020). Methodological trends and perspectives of animal dietary studies by noninvasive fecal DNA metabarcoding. Environ. DNA 2, 391–406. doi: 10.1002/edn3.117
Atzori, A., Berti, F., Cencetti, T., Fornasiero, S., Tamburini, M., and Zuffi, M. A. L. (2007). Advances in methodologies of sexing and marking less dimorphic gekkonid lizards: the study case of the Moorish gecko, Tarentola mauritanica. Amphibia Reptilia 28, 449–454. doi: 10.1163/156853807781374719
Báez, M., and Biscoito, M. (1993). “First record of Tarentola mauritanica (Linneus, 1758) from the island of Madeira (NE Atlantic),” in First Symposium of Fauna and Flora of the Atlantic Islands (Funchal).
Bailey, P., and Mendonça, T. R. D. (1990). The distribution of the millipede Ommatoiulus moreleti (Diplopoda, Julida: Julidae) in relation to other Ommatoiulus species on the south-western Iberian Peninsula. J. Zool. 221, 99–111. doi: 10.1111/j.1469-7998.1990.tb03779.x
Baker, G. (1984). Distribution, morphology and life history of the millipede Ommatoiulus moreletii (Diplopoda: Iulidae) in Portugal and comparisons with Australian populations. Aust. J. Zool. 32, 811–822. doi: 10.1071/ZO9840811
Bates, D., Mächler, M., Bolker, B., and Walker, S. (2014). Fitting linear mixed-effects models using lme4. arXiv preprint arXiv:1406.5823. doi: 10.18637/jss.v067.i01
Blackburn, T. M., Delean, S., Pyšek, P., and Cassey, P. (2016). On the island biogeography of aliens: a global analysis of the richness of plant and bird species on oceanic islands. Glob. Ecol. Biogeogr. 25, 859–868. doi: 10.1111/geb.12339
Borges, P. A. V., Abreu, C., Aguiar, A. M. F., Carvalho, P., Jardim, R., Melo, I., et al. (2008). Listagem dos fungos, flora e fauna terrestres dos arquipélagos da Madeira e Selvagens = A list of the terrestrial fungi, flora and fauna of Madeira and Selvagens archipelagos. Funchal, Madeira: Secretaria Regional do Ambiente e dos Recursos Naturais do Governo Regional da Madeira.
Bowler, K., and Terblanche, J. S. (2008). Insect thermal tolerance: what is the role of ontogeny, ageing and senescence? Biol. Rev. 83, 339–355. doi: 10.1111/j.1469-185X.2008.00046.x
Boyer, F., Mercier, C., Bonin, A., Le Bras, Y., Taberlet, P., and Coissac, E. (2016). obitools: a unix-inspired software package for DNA metabarcoding. Mol. Ecol. Resour. 16, 176–182. doi: 10.1111/1755-0998.12428
Buchner, D., and Leese, F. (2020). BOLDigger–a Python package to identify and organise sequences with the Barcode of Life Data systems. Metabarcod. Metagen. 4, e53535. doi: 10.3897/mbmg.4.53535
Buckland, S., Cole, N. C., Aguirre-Gutiérrez, J., Gallagher, L. E., Henshaw, S. M., Besnard, A., et al. (2014). Ecological effects of the invasive giant Madagascar day gecko on endemic Mauritian geckos: applications of binomial-mixture and species distribution models. PLoS ONE 9, e88798. doi: 10.1371/journal.pone.0088798
Capinha, C., Seebens, H., Cassey, P., García-Díaz, P., Lenzner, B., Mang, T., et al. (2017). Diversity, biogeography and the global flows of alien amphibians and reptiles. Diver. Distrib. 23, 1313–1322. doi: 10.1111/ddi.12617
Carranza, S., Arnold, E. N., Mateo, J. A., and López-Jurado, L. F. (2000). Long-distance colonization and radiation in gekkonid lizards, Tarentola (Reptilia: Gekkonidae), revealed by mitochondrial DNA sequences. Proc. R. Soc. London B 267, 637–649. doi: 10.1098/rspb.2000.1050
Clavero, M., and García-Berthou, E. (2005). Invasive species are a leading cause of animal extinctions. Trends Ecol. Evol. 20, 110. doi: 10.1016/j.tree.2005.01.003
Clemens, D. J., and Allain, S. J. (2020). First evidence of Fogo Island skink (Chioninia fogoensis) introduction to the island of Madeira. Herpetol. Bull. 152, 40–41. doi: 10.33256/152.4041
Clemente, M. (1996). Prevalence of canine dirofilariosis on Madeira Island. Detection and identification of microfilariae. Vet. Técn. 6, 34–37.
Cluever, J. D., and Smith, H. A. (2016). Pest information guide: eastern flower thrips Frankliniella tritici (Fitch): ENY-894/IN1137, 7/2016. EDIS 2016, 2.
Cummings, C., Gamble, T., and Wells, M. (2021). A comparison of the foraging biology of two tropical gecko species in disturbed areas. South Am. J. Herpetol. 19, 8–11. doi: 10.2994/SAJH-D-18-00026.1
Dawson, W., Moser, D., Van Kleunen, M., Kreft, H., Pergl, J., Pyšek, P., et al. (2017). Global hotspots and correlates of alien species richness across taxonomic groups. Nat. Ecol. Evol. 1, 1–7. doi: 10.1038/s41559-017-0186
De Jong, Y., Verbeek, M., Michelsen, V., de Place Bjørn, P., Los, W., Steeman, F., et al. (2014). Fauna Europaea-all European animal species on the web. Biodiver. Data J. 2, e4034. doi: 10.3897/BDJ.2.e4034
Demirci, B., Durmaz, E., and Alten, B. (2014). Influence of bloodmeal source on reproductive output of the potential West Nile vector, Culex theileri (Diptera: Culicidae). J. Med. Entomol. 51, 1312–1316. doi: 10.1603/ME13197
Demirci, B., Lee, Y., Lanzaro, G. C., and Alten, B. (2012). Identification and characterization of single nucleotide polymorphisms (SNPs) in Culex theileri (Diptera: Culicidae). J. Med. Entomol. 49, 581–588. doi: 10.1603/ME11139
Doherty, T. S., Glen, A. S., Nimmo, D. G., Ritchie, E. G., and Dickman, C. R. (2016). Invasive predators and global biodiversity loss. Proc. Nat. Acad. Sci. U. S. A. 113, 11261–11265. doi: 10.1073/pnas.1602480113
Dormann, C. F., Frueund, J., Bluethgen, N., and Gruber, B. (2009). Indices, graphs and null models: analyzing bipartite ecological networks. Open Ecol. J. 2, 7–24. doi: 10.2174/1874213000902010007
Douglas, J., Macfadyen, S., Hoffmann, A., and Umina, P. (2017). Crop Seedling Susceptibility to Armadillidium vulgare (Isopoda: Armadillidiidae) and Ommatoiulus moreletii (Diplopoda: Iulidae). J. Econ. Entomol. 110, 2679–2685. doi: 10.1093/jee/tox275
Early, R., Bradley, B. A., Dukes, J. S., Lawler, J. J., Olden, J. D., Blumenthal, D. M., et al. (2016). Global threats from invasive alien species in the twenty-first century and national response capacities. Nat. Commun. 7, 1–9. doi: 10.1038/ncomms12485
Evans, H. K., Bunch, A. J., Schmitt, J. D., Hoogakker, F. J., and Carlson, K. B. (2021). High-throughput sequencing outperforms traditional morphological methods in Blue Catfish diet analysis and reveals novel insights into diet ecology. Ecol. Evol. 11, 5584–5597. doi: 10.1002/ece3.7460
Fox, J., Weisberg, S., Adler, D., Bates, D., Baud-Bovy, G., Ellison, S., et al. (2012). Package ‘car'. Vienna: R Foundation for Statistical Computing, 16.
Franco, A. (1980). Nuevo dato sobre herpetofagia en Tarentola mauritanica. Doñana Acta Vertebrata 7, 262.
Frøslev, T. G., Kjøller, R., Bruun, H. H., Ejrnæs, R., Brunbjerg, A. K., Pietroni, C., et al. (2017). Algorithm for post-clustering curation of DNA amplicon data yields reliable biodiversity estimates. Nat. Commun. 8, 1188. doi: 10.1038/s41467-017-01312-x
Fulgione, D., Buglione, M., Rippa, D., Trapanese, M., Petrelli, S., Monti, D. M., et al. (2019). Selection for background matching drives sympatric speciation in Wall Gecko. Sci. Rep. 9, 1288. doi: 10.1038/s41598-018-37587-3
Funderburk, J., Freeman, J., Adkins, S., Hutton, S., Stansly, P., Smith, H., et al. (2018). Managing thrips and tospoviruses in tomato: ENY859/IN895, rev. 1/2018. EDIS 2018.
Funderburk, J., Reitz, S., Stansly, P., Schuster, D., Nuessly, G., and Leppla, N. (2009). Managing thrips in pepper and eggplant. EDIS 2009. doi: 10.32473/edis-in401-2009
Gil, M. J., Guerrero, F., and Pérez-Mellado, V. (1994). Seasonal variation in diet composition and prey selection in the Mediterranean gecko Tarentola mauritanica. Isr. J. Zool. 40, 61–74.
Gil, V., Pinho, C. J., Aguiar, C. A., Jardim, C., Rebelo, R., and Vasconcelos, R. (2020). Questioning the proverb ‘more haste, less speed': classic versus metabarcoding approaches for the diet study of a remote island endemic gecko. Peer J. 8, e8084. doi: 10.7717/peerj.8084
González de la Vega, J. P. (1988). Anfibios y Reptiles de la Provincia de Huelva. Huelva: Ediciones Ertisa.
Harris, D. J., Batista, V., Carretero, M. A., and Ferrand, N. (2004a). Genetic variation in Tarentola mauritanica (Reptilia: Gekkonidae) across the Strait of Gibraltar derived from mitochondrial and nuclear DNA sequences. Amphibia Reptilia 25, 451–459.
Harris, D. J., Batista, V., Lymberakis, P., and Carretero, M. A. (2004b). Complex estimates of evolutionary relationships in Tarentola mauritanica (Reptilia: Gekkonidae) derived from mitochondrial DNA sequences. Mol. Phylogenet. Evol. 30, 855–859. doi: 10.1016/S1055-7903(03)00260-4
Herrel, A., Aerts, P., Fret, J., and De Vree, F. (1999a). Morphology of the feeding system in agamid lizards: ecological correlates. Anat. Rec. 254, 496–507.
Herrel, A., Spithoven, L., Van Damme, R., and De Vree, F. (1999b). Sexual dimorphism of head size in Gallotia galloti: testing the niche divergence hypothesis by functional analyses. Funct. Ecol. 13, 289–297. doi: 10.1046/j.1365-2435.1999.00305.x
Hódar, J., and Pleguezuelos, J. (1999). Diet of the Moorish gecko Tarentola mauritanica in an arid zone of south-eastern Spain. Herpetol. J. 9, 29–32.
Hódar, J. A., Pleguezuelos, J. M., Villafranca, C., and Fernández-Cardenete, J. R. (2006). Foraging mode of the Moorish gecko Tarentola mauritanica in an arid environment: inferences from abiotic setting, prey availability and dietary composition. J. Arid Environ. 65, 83–93. doi: 10.1016/j.jaridenv.2005.08.006
Holmes, T. P., Aukema, J. E., Von Holle, B., Liebhold, A., and Sills, E. (2009). Economic impacts of invasive species in forests: past, present, and future. Ann. N. Y. Acad. Sci. 1162, 18–38. doi: 10.1111/j.1749-6632.2009.04446.x
Hoskin, C. J. (2011). The invasion and potential impact of the Asian House Gecko (Hemidactylus frenatus) in Australia. Austral. Ecol. 36, 240–251. doi: 10.1111/j.1442-9993.2010.02143.x
Hothorn, T., Bretz, F., and Westfall, P. (2008). Simultaneous inference in general parametric models. Biometrical J. 50, 346–363. doi: 10.1002/bimj.200810425
Hsieh, T., Ma, K., and Chao, A. (2016). iNEXT: an R package for rarefaction and extrapolation of species diversity (H ill numbers). Methods Ecol. Evol. 7, 1451–1456. doi: 10.1111/2041-210X.12613
Husak, J. F., and Fox, S. F. (2006). Field use of maximal sprint speed by collared lizards (Crotaphytus collaris): compensation and sexual selection. Evolution 60, 1888–1895. doi: 10.1111/j.0014-3820.2006.tb00532.x
Husak, J. F., Lappin, A. K., and Van Den Bussche, R. A. (2009). The fitness advantage of a high-performance weapon. Biol. J. Linn. Soc. 96, 840–845. doi: 10.1111/j.1095-8312.2008.01176.x
Ingerson-Mahar, J. (2002). “Relating diet and morphology in adult carabid beetles,” in The Agroecology of Carabid Beetles, ed J. M. Holland (Andover: Intercept Limited), 111–136.
Jesus, J., Lemos, A., Gonçalves, R., and Brehm, A. (2008). First record of Tarentola mauritanica (Linnaeus, 1758) on Porto Santo Island. Herpetozoa 20, 175–177.
Kemp, J., López-Baucells, A., Rocha, R., Wangensteen, O. S., Andriatafika, Z., Nair, A., et al. (2019). Bats as potential suppressors of multiple agricultural pests: a case study from Madagascar. Agric. Ecosyst. Environ. 269, 88–96. doi: 10.1016/j.agee.2018.09.027
Lamb, A. D., Lippi, C. A., Watkins-Colwell, G. J., Jones, A., Warren, D. L., Iglesias, T. L., et al. (2021). Comparing the dietary niche overlap and ecomorphological differences between invasive Hemidactylus mabouia geckos and a native gecko competitor. Ecol. Evol. 11, 18719–18732. doi: 10.1002/ece3.8401
Lappin, A. K., and Husak, J. F. (2005). Weapon performance, not size, determines mating success and potential reproductive output in the collared lizard (Crotaphytus collaris). Am. Nat. 166, 426–436. doi: 10.1086/432564
Lopes, R. J., Pinho, C. J., Santos, B., Seguro, M., Mata, V. A., Egeter, B., et al. (2019). Intricate trophic links between threatened vertebrates confined to a small island in the Atlantic Ocean. Ecol. Evol. 9, 4994–5002. doi: 10.1002/ece3.5105
Lozano-Del Campo, A., and García-Roa, R. (2014). Predation behavior with individuals aggregation on streetlights in Tarentola mauritanica (Squamata: Gekkonidae) from Southern Spain. Biharean Biol. 8, 120–121.
Luiselli, L., and Capizzi, D. (1999). Ecological distribution of the geckos Tarentola mauritanica and Hemidactylus turcicus in the urban area of Rome in relation to age of buildings and condition of the walls. J. Herpetol. 33, 316–319. doi: 10.2307/1565732
Martín, B., Pérez, H., and Ferrer, M. (2018). Effects of natural and artificial light on the nocturnal behaviour of the wall gecko. Anim. Biodivers. Conserv. 41, 209–215. doi: 10.32800/abc.2018.41.0209
Martínez-Rica, J. P. (1974). Contribución al estudio de la biología de los gecónidos ibéricos (Rept. Sauria). Public. Centro Pirenaico Biol. Exp. 5, 7–293.
Maslo, B., Valentin, R., Leu, K., Kerwin, K., Hamilton, G. C., Bevan, A., et al. (2017). Chiro surveillance: the use of native bats to detect invasive agricultural pests. PLoS ONE 12, e0173321. doi: 10.1371/journal.pone.0173321
Massetti, F., Kaliontzopoulou, A., Gomes, V., and Rato, C. (2018). Variation in morphology and functional performance across distinct evolutionary lineages of the Moorish gecko (Tarentola mauritanica) from the Iberian Peninsula. J. Zool. Syst. Evol. Res. 2018, 1–14. doi: 10.1111/jzs.12249
Massetti, F., Perera, A., Gomes, V., Rato, C., and Kaliontzopoulou, A. (2017). Morphological and functional implications of sexual size dimorphism in the Moorish gecko. Biol. J. Linn. Soc. 112, 197–209. doi: 10.1093/biolinnean/blx060
McGeoch, M. A., Butchart, S. H., Spear, D., Marais, E., Kleynhans, E. J., Symes, A., et al. (2010). Global indicators of biological invasion: species numbers, biodiversity impact and policy responses. Diver. Distrib. 16, 95–108. doi: 10.1111/j.1472-4642.2009.00633.x
Montauban, C., Mas, M., Wangensteen, O. S., i Monteys, V. S., Fornós, D. G., Mola, X. F., et al. (2021). Bats as natural samplers: first record of the invasive pest rice water weevil Lissorhoptrus oryzophilus in the Iberian Peninsula. Crop Prot. 141, 105427. doi: 10.1016/j.cropro.2020.105427
Mori, E., and Plebani, M. (2012). First records of Moorish gecko Tarentola mauritanica and Turkish gecko Hemidactylus turcicus (Squamata, Gekkonidae) in the Southern Metalliferous Hills, Tuscany, Italy. Atti Soc. Toscana Scienze Nat. 119, 51–54.
Novosolov, M., Rodda, G. H., Feldman, A., Kadison, A. E., Dor, R., and Meiri, S. (2016). Power in numbers. Drivers of high population density in insular lizards. Glob. Ecol. Biogeogr. 25, 87–95. doi: 10.1111/geb.12390
Oksanen, J., Blanchet, F. G., Kindt, R., Legendre, P., Minchin, P., O'hara, R., et al. (2013). Community ecology package. R Package Version 2, 321–326.
Oliveira, P., Gomes, V., Mochales, G., and Rato, C. (2022). Ontogenic differences and sexual dimorphism of the locomotor performance in a nocturnal gecko, Tarentola mauritanica. J. Exp. Zool. Part A. doi: 10.1002/jez.2645 [Epub ahead of print].
Perella, C. D., and Behm, J. E. (2020). Understanding the spread and impact of exotic geckos in the greater Caribbean region. Biodivers. Conserv. 29, 1109–1134. doi: 10.1007/s10531-020-01939-1
Perry, G., and Werner, Y. L. (1981). Food of Ptyodactylus hasselquistii guttatus (reptilia: gekkoninae) at two locations in Israel, in summer. Isr. J. Zool. 98–99.
Pimentel, D., Zuniga, R., and Morrison, D. (2005). Update on the environmental and economic costs associated with alien-invasive species in the United States. Ecol. Econ. 52, 273–288. doi: 10.1016/j.ecolecon.2004.10.002
Pinho, C. J., Santos, B., Mata, V. A., Seguro, M., Romeiras, M. M., Lopes, R. J., et al. (2018). What is the giant wall gecko having for dinner? conservation genetics for guiding reserve management in Cabo Verde. Genes 9, 599. doi: 10.3390/genes9120599
Pitt, W., Vice, D., and Pitzler, M. (2005). “Challenges of invasive reptiles and amphibians,” in Proceedings of the 11th Wildlife Damage Management Conference, eds D. L. Nolte and K. A. Fagerstone (Traverse City, MI: Wildlife Damage Management Working Group), 112–119.
Plimpton, L. D., Henger, C. S., Munshi-South, J., Tufts, D., Kross, S., and Diuk-Wasser, M. (2021). Use of molecular scatology to assess the diet of feral cats living in urban colonies. J. Urban Ecol. 7, juab002. doi: 10.1093/jue/juab022
Pompanon, F., Deagle, B. E., Symondson, W. O., Brown, D. S., Jarman, S. N., and Taberlet, P. (2012). Who is eating what: diet assessment using next generation sequencing. Mol. Ecol. 21, 1931–1950. doi: 10.1111/j.1365-294X.2011.05403.x
Press, J. R (1993). Introduced plants and their effects on the flora of Madeira. Boletin Museu Municipal Funchal 243–248.
R Core Team. (2021). R: A Language and Environment for Statistical Computing. R Foundation for Statistical Computing, Vienna, Austria. Available online at: http://www.R-project.org/ (accessed November 8, 2022).
Rato, C., Harris, D. J., Carranza, S., Machado, L., and Perera, A. (2016). The taxonomy of the Tarentola mauritanica species complex (Gekkota: Phyllodactylidae): bayesian species delimitation supports six candidate species. Mol. Phylogenet. Evol. 94, 271–278. doi: 10.1016/j.ympev.2015.09.008
Rato, C., Martins, B., Rocha, R., and Silva-Rocha, I. (2021). Uncovered genetic diversity in Hemidactylus mabouia (Reptilia: Gekkonidae) from Madeira Island reveals uncertain sources of introduction. Amphibia Reptilia 42, 369–375. doi: 10.1163/15685381-bja10052
Ribeiro, L., Silva, J., Aguiar, A., Pestana, M., and Rodrigues, M. (2009). “Main Pests and diseases of bananas on Madeira Island,” in I Workshop de Fruticultura–Contributo para o seu Desenvolvimento (Funchal), 123–132.
Rocha, R., Reino, L., Sepúlveda, P., and Ribeiro, J. (2020). Introduced population of ring-necked parakeets Psittacula krameri in Madeira Island, Portugal-call for an early action. Manag. Biol. Invas. 11, 576–587. doi: 10.3391/mbi.2020.11.3.15
Rognes, T., Flouri, T., Nichols, B., Quince, C., and Mahé, F. (2016). VSEARCH: a versatile open source tool for metagenomics. Peer J. 4, e2584. doi: 10.7717/peerj.2584
Romero-Cordero, B. (2021). Determination of invasion stage of the tokay gecko (Gekko gecko) in Puerto Rico: a comparison between two invasion sites (Ph.D.). Mayagüez: Universidad de Puerto Rico.
Roques, A. (2010). “Taxonomy, time and geographic patterns,” in Alien Terrestrial Arthropods of Europe BioRisk, eds A. Roques, M. Kenis, D. Lees, C. Lopez-Vaamonde, W. Rabitsch, J.-Y. Rasplus, et al. (Sofia: Pensoft Publishers), 11–26.
Salvador, A. (2016). “Salamanquesa común - Tarentola mauritanica (Linnaeus, 1758),” in Enciclopedia Virtual de los Vertebrados Españoles, eds A. Salvador and A. Marco. (Madrid: Museo Nacional de Ciencias Naturales). Available online at: http://www.vertebradosibericos.org/ (accessed November 8, 2022).
Sánchez-Piñero, F. (1994). Ecología de las comunidades de coleópteros en zonas áridas de la depresión de Guadix-Baza (Sureste de la Península Ibérica) (Ph.D.). Granada: Universidad de Granada.
Santa-Ana, M., Khadem, M., and Capela, R. (2006). Natural infection of Culex theileri (Diptera: Culicidae) with Dirofilaria immitis (nematoda: filarioidea) on Madeira Island, Portugal. J. Med. Entomol. 43, 104–106. doi: 10.1603/0022-2585(2006)0430104:NIOCTD2.0.CO
Santos, B., Pinho, C. J., Št'áhlavský, F., Mata, V. A., Lopes, R. J., and Vasconcelos, R. (2022). Diet study of geckos reveals the first records of pseudoscorpions on Desertas Islands (Cabo Verde). J. Arachnol. 50, 39–42. doi: 10.1636/JoA-S-20-085
Santos, J. M. F. (2018). Análise e modelação espácio-temporal do mosquito vetor do dengue na ilha da Madeira (Ph.D.). Lisbon: University of Lisbon.
Seixas, G., Salgueiro, P., Bronzato-Badial, A., Gonçalves, Y., Reyes-Lugo, M., Gordicho, V., et al. (2019). Origin and expansion of the mosquito Aedes aegypti in Madeira Island (Portugal). Sci. Rep. 9, 1–13. doi: 10.1038/s41598-018-38373-x
Silva, C. M. d., Sérvio, H. S., Ramos, R. A. N., Faustino, M. A. d. G., Alves, L. C., and Carvalho, G. A. d. (2014). Occurrence of immature forms of culicids (Insecta: Diptera) in the northeastern region of Brazil. Rev. Brasil. Parasitol. Vet. 23, 200–205. doi: 10.1590/S1984-29612014046
Silva-Rocha, I., Santos, J. M., Rocha, R., and Rato, C. (2022). Bioclimatic and local drivers modulating the expansion of an introduced temperate reptile in a subtropical island. Glob. Ecol. Conserv. 37, e02164. doi: 10.1016/j.gecco.2022.e02164
Taberlet, P., Coissac, E., Pompanon, F., Brochmann, C., and Willerslev, E. (2012). Towards next-generation biodiversity assessment using DNA metabarcoding. Mol. Ecol. 21, 2045–2050. doi: 10.1111/j.1365-294X.2012.05470.x
Tiede, J., Diepenbruck, M., Gadau, J., Wemheuer, B., Daniel, R., and Scherber, C. (2020). Seasonal variation in the diet of the serotine bat (Eptesicus serotinus): a high-resolution analysis using DNA metabarcoding. Basic Appl. Ecol. 49, 1–12. doi: 10.1016/j.baae.2020.09.004
Vamos, E. E., Elbrecht, V., and Leese, F. (2017). Short COI markers for freshwater macroinvertebrate metabarcoding. Metabarcod. Metagen. 1, e14625. doi: 10.3897/mbmg.1.14625
Vilà, M., Basnou, C., Pyšek, P., Josefsson, M., Genovesi, P., Gollasch, S., et al. (2010). How well do we understand the impacts of alien species on ecosystem services? A pan-European, cross-taxa assessment. Front. Ecol. Environ. 8, 135–144. doi: 10.1890/080083
Villanueva, R. A. M., and Chen, Z. J. (2019). ggplot2: Elegant Graphics for Data Analysis. Taylor and Francis. doi: 10.1080/15366367.2019.1565254
Vogrin, M., Corti, C., Mellado, V. P., Sá-Sousa, P., Cheylan, M., Pleguezuelos, J., et al. (2017). Tarentola mauritanica. The IUCN Red List of Threatened Species 2017: e.T61578A63716927. Downloaded on 23 February 2021. doi: 10.2305/IUCN.UK.2017-2.RLTS.T61578A63716927.en
Werner, E. E., and Gilliam, J. F. (1984). The ontogenetic niche and species interactions in size-structured populations. Annu. Rev. Ecol. Syst. 15, 393–425. doi: 10.1146/annurev.es.15.110184.002141
Wetterer, J. K., Espadaler, X., Wetterer, A. L., Aguin-Pombo, D., and Franquinho-Aguiar, A. M. (2007). Ants (Hymenoptera: Formicidae) of the Madeiran Archipelago. Sociobiology 49, 265–297.
Whittaker, R. J., and Fernández-Palacios, J. M. (2007). Island Biogeography: Ecology, Evolution, and Conservation. Oxford: Oxford University Press.
Keywords: agricultural pests, diet, disease vectors, early warner, invasive species, Tarentola mauritanica, DNA metabarcoding, Madeira Island
Citation: Martins B, Silva-Rocha I, Mata VA, Gonçalves Y, Rocha R and Rato C (2022) Trophic interactions of an invasive gecko in an endemic-rich oceanic island: Insights using DNA metabarcoding. Front. Ecol. Evol. 10:1044230. doi: 10.3389/fevo.2022.1044230
Received: 14 September 2022; Accepted: 21 November 2022;
Published: 02 December 2022.
Edited by:
Attila D. Sándor, University of Agricultural Sciences and Veterinary Medicine of Cluj-Napoca, RomaniaReviewed by:
Marcela Vidal, University of the Bío Bío, ChileJosé A. Jurado-Rivera, University of the Balearic Islands, Spain
Verónica Costa Neves, University of the Azores, Portugal
Copyright © 2022 Martins, Silva-Rocha, Mata, Gonçalves, Rocha and Rato. This is an open-access article distributed under the terms of the Creative Commons Attribution License (CC BY). The use, distribution or reproduction in other forums is permitted, provided the original author(s) and the copyright owner(s) are credited and that the original publication in this journal is cited, in accordance with accepted academic practice. No use, distribution or reproduction is permitted which does not comply with these terms.
*Correspondence: Catarina Rato, Y2F0aXJhdG9AZ21haWwuY29t