- 1Institute of Earth Sciences, The Hebrew University of Jerusalem, Jerusalem, Israel
- 2Department of Ecology, Evolution and Behavior, The Silberman Institute of Life Sciences, The Hebrew University of Jerusalem, Jerusalem, Israel
- 3The National Natural History Collections, The Hebrew University of Jerusalem, Jerusalem, Israel
- 4GeoQuEST Research Centre, School of Earth and Environmental, University of Wollongong, Wollongong, NSW, Australia
The stable isotopes composition of chemolithoautotrophic cave ecosystems is known to differ from epigenic caves. Here we show that in addition, dead carbon (devoid of 14C), is utilized and transferred throughout this ecosystem, rendering it unsuitable for radiocarbon dating. The connectivity of the Ayyalon Cave ecosystem with the surface is studied, along with its sources of energy and carbon, as well as the interconnections between its constituents. We use isotopic evidence to show that its ancient resilient ecosystem is based on an underground food web depending on rich biomass production by chemolithoautotrophic nutrient supplies, detached from surface photosynthesis. Carbon isotopic values indicate that: (1) the microbial biota use bicarbonate from the groundwater (23.34 pMC [% of modern carbon]) rather than the atmospheric CO2 above the water (71.36 pMC); (2) the depleted 14C signal is transferred through the entire ecosystem, indicating that the ecosystem is well-adapted and based on the cave biofilm which is in turn based on groundwater-dissolved inorganic carbon. Incubation of Ayyalon biofilm with 14C-labelled bicarbonate indicates uptake of the radio-labeled bicarbonate by sulfur-oxidizing proteobacteria Beggiatoa, suggesting that these sulfur-oxidizing microorganisms use the water-dissolved inorganic carbon for chemolithoautotrophic carbon fixation. Organic matter in the cave is much lighter in its stable nitrogen and carbon isotopes compared with respective surface values, as expected in chemolithoautotrophic systems. This evidence may be applicative to subsurface voids of ancient Earth environments and extraterrestrial systems.
1. Introduction
Chemoauotrophic micro-organisms use various metabolic strategies to produce biomass by fixing CO2 at the expense of energy extracted from chemical compounds (Engel, 2007), and support diverse food webs in caves (Culver, 2013). However, most human-accessible caves lack chemoautotrophic production. Chemoautotrophy-based karst ecosystems are often limited not only by energy, but also by the supply of elements such as iron, nitrogen, and sulfur (Sarbu et al., 1996). The dynamics of these elements have a strong influence on the magnitude and location of the energy base of food webs in the cave ecosystems, with zones such as the water-air interface acting as hotspots for productivity. Adaptations in diverse groups of cave-dwelling animals at the physiologic and molecular levels are affected by chemoautotrophy.
Cave chemolithoautotrophic processes were studied mainly since the mid-1990s, in association with studies of deep terrestrial groundwater and deep-sea hydrothermal vents (Stevens and McKinley, 1995). Chemolithoautotrophs have been commonly considered to be inefficient, relative to phototrophs. Therefore, chemolithoautotrophs have been assumed not to be important primary producers. However, under the absence of light and photosynthesis in the subsurface, chemolithoautotrophy can be important, sustaining many ecosystem-level processes (Culver and Pipan, 2019).
Chemolithoautotrophs use compounds present in rocks or groundwater. The energy is obtained by transferring electrons from one chemical compound to another. The electron donors include, for example, reduced sulfur compounds (e.g., hydrogen sulfide, elemental sulfur), molecular hydrogen (H2), ammonium (NH+), reduced iron (Fe2+) and manganese (Mn2+).
Along with photosynthesis, chemolithoautotrophy has been crucial in the transformation of chemical energy from one reservoir to another during Earth’s history and for the incorporation of inorganic carbon into the biospheric organic carbon. Only members of the domains Bacteria and Archaea are capable of chemolithoautotrophy.
In spite of the importance of chemolithoautotrophy, the knowledge of chemosynthetic systems of caves remains limited. Most chemolithoautotrophic caves with well-developed geomicrobiology ecosystems have sulfidic water (Northup et al., 2001; Hose, 2013; Palmer, 2013).
A good example of such well-developed ecosystem with 37 metazoan endemics is Movile Cave (Brad et al., 2021), which has a sulfidic groundwater pond that is close to the surface, but represents a regional aquifer. The aquifer is accessed not only through the cave, but also through boreholes and wells. The diverse hygropetric fauna of Movile is composed mainly of new species which are congeners of genera present in the epigeic surroundings that penetrated the “dry bells” of that cave system possibly in a relatively recent geological past (Sârbu, 2001). The first use of environmental isotopes to study a chemoautotrophic cave system was performed at Movile by Sarbu et al. (1996).
Frasassi Cave in Italy has 12 endemic species, and was studied using stable isotopes by Sarbu et al. (2000). In the Frasassi Cave, evidence for nitrogen fixation was found in the symbiotic bacterial community of Niphargus amphipods, Beggiatoa-dominated biofilms, and sulfidic sediment (Desai et al., 2013). Dattagupta et al. (2009) showed in the same cave that Niphargus ictus throughout the cave is colonized by a single phylotype of bacteria in the sulfur-oxidizing clade Thiothri.
Cueva de Villa Luz (Hose and Pisarowicz, 1999; Palmer, 2013) has several skylights and a large sulfidic water stream coming out from its subsurface voids. Its connection with the surface fauna is thus larger than the above-mentioned caves, sustaining a smaller number of endemism.
In various caves, including anchialine systems, in Europe, America and Australia, chemoautotrophy has been shown to be either aerobic (oxidation of hydrogen, sulfur, iron, manganese, ammonia or methane) or anaerobic (by methanogenesis, acetogenesis, denitrification or the reduction of sulfate, iron or ammonia) with multiple co-occurring chemolithotrophic metabolic processes in most subsurface systems. In some aquifer systems, 13C-depleted biomass indicated the presence of chemoautotrophic pathways through methane-linked carbon cycling, for example in Edwards Aquifer, Texas (Bishop et al., 2014; Hutchins et al., 2016), and Ox Bel Ha, Yucatán peninsula (Pohlman et al., 1997). Based on the prevalence and abundance of 13C-depleted lipids, it has been shown that biofilms in lava caves at Lava Beds National Monument, US, are also fueled by in situ chemolithoautotrophy (Selensky et al., 2021). Iron-based metabolism is practiced by reducing microorganisms in iron ore caves (Parker et al., 2018.). However, most of the last-mentioned caves lack Metazoa.
Previous studies have used stable environmental isotopes to study chemoautotrophic cave ecosystems. In Movile and Frasassi caves, organisms with a chemolithoautotrophic diet have δ13C values ranging from −38 to −46‰ and from −30 to −40‰ respectively (Sarbu et al., 1996, 2000). We hypothesize that such negative values are expected also in other similar chemoautotrophic caves, such as Ayyalon. We therefore test the stable environmental isotopes of Ayyalon, and compare them with their surface counterparts, for testing the dis/connection of the cave ecosystem from above-ground system.
Another hypothesis to be tested here, is that throughout a chemoautotrophic system, the carbon should be mostly ‘dead’, i.e., devoid of 14C, due to the deep sources of carbon used by the cave ecosystem. This contrasts with epigenic cave systems which rely on carbon produced by photosynthesis using atmospheric CO2 at the earth surface, i.e., carbon which is rich in 14C. To test our assumption, we measure the 14C content throughout the entire ecosystem, in order to differentiate chemoautotrophic components from associated surface phototrophic ones.
We also use the classic tools of carbon and nitrogen stable isotopes to further understand the ecosystem, from basic inorganic materials to highly-endemic arthropods (Por et al., 2013).
2. Ayyalon cave
2.1. Ayyalon hydrogeology
Ayyalon Cave (34.928157°E, 31.910891°N) is located ~20 km east of the Mediterranean coastline. The natural surface is ~100 m asl (above sea level).The Nesher Ramla quarry breached the natural surface during the second half of the 20th century, reaching Ayyalon Cave and associated watertable in 2006. The 2.7 km long Ayyalon Cave is within late Cretaceous (Turonian) massive limestone (Figure 1C). Ayyalon Cave was geologically covered by a ~ 100 m thick, almost impermeable chalk. Until its truncation by the quarry it had been effectively isolated from the surface by the impervious rock, which seems to have precluded percolation of water, organic matter and nutrients from photosynthetic surface environments.
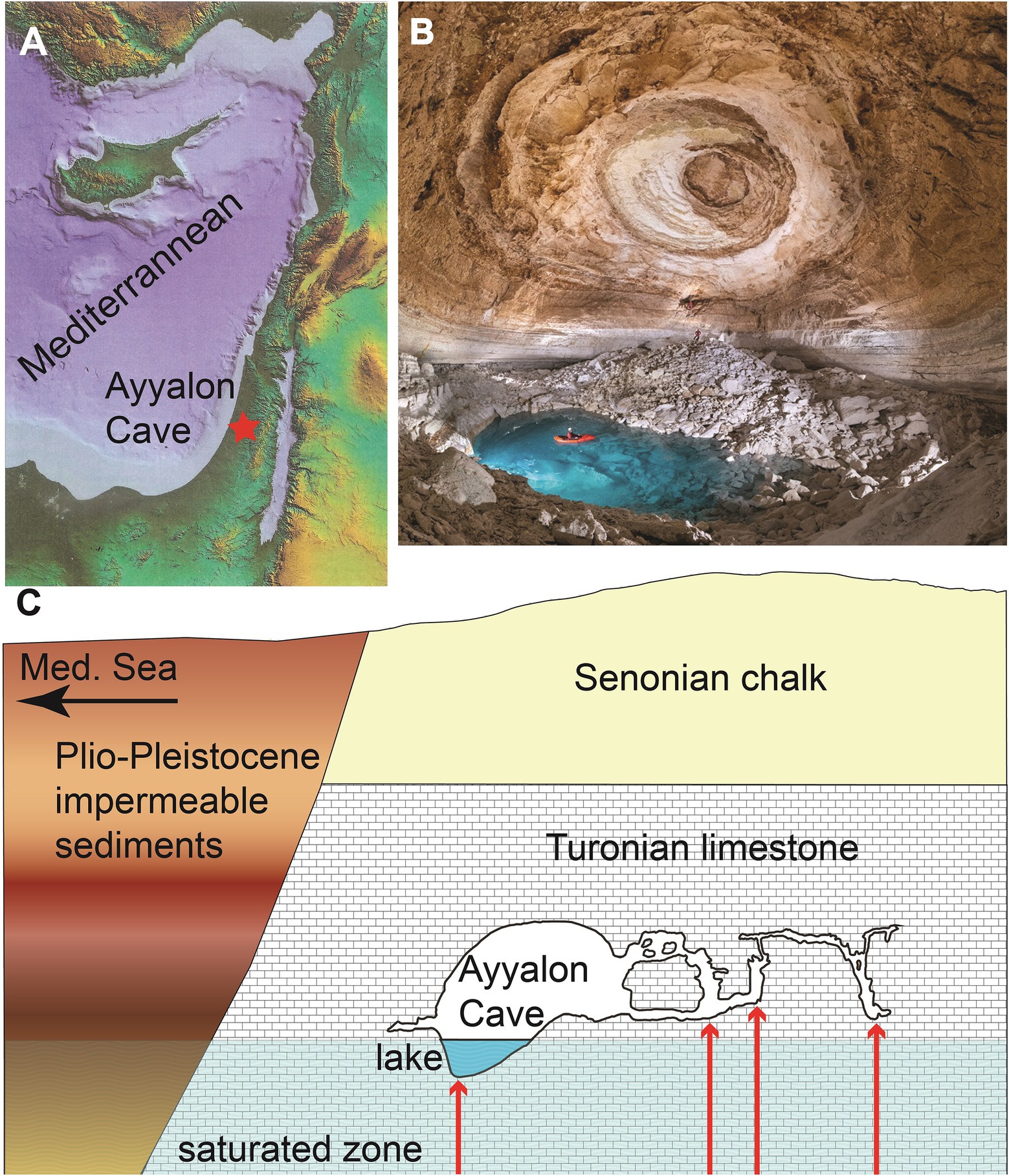
Figure 1. Ayyalon Cave configuration. (A) location; (B) the main chamber and groundwater pond of the cave, acting as the ecosystem focal point (photo: Boaz Langford); (C) schematic geological cross section of the cave and associated hydrogeology. For location of section see blue indicators in Figure 2A.
A phreatic aquifer is exposed within the cave as a pond, serving as a window into the warm-sulfidic groundwater (Frumkin and Gvirtzman, 2006). The watertable is fluctuating between ~12–24 m asl, thus the water depth of the pond has varied recently between 2 (in 2018) and 9 m (in 2022). The aquifer at Ayyalon vicinity is slightly hydrothermal (also termed ‘Ayyalon anomaly’)with relatively high salinity and H2S, compared with the regional groundwater (Frumkin and Gvirtzman, 2006). The ‘Ayyalon anomaly’ is a unique part of Israel’s largest aquifer, Yarkon-Taninim (Weinberger et al., 1994), whose regional freshwater is generally cooler, less saline, and devoid of H2S. Yarkon-Taninim recharge zone extends along the western slopes of Israel’s central highland. The western part of the aquifer is confined by Senonian chalks overlain by younger low-conductivity rocks, dominated by the Pliocene Yafo Formation marls, among other late Cenozoic sediments. Ayyalon Cave is located east of the confinement line.
West of the Nesher Ramla quarry, within the Mediterranean Coastal Plain, the facies of the late Cretaceous carbonate rocks changes laterally, becoming impervious to the west, and together with the overlying Yafo Formation clays, these units block westward groundwater flow (Frumkin et al., 2022). Under these confining and damming conditions within the regional aquifer, the artesian hydrothermal flow mixes with fresh water flowing laterally from the recharge zone along the upper aquifer. The water temperature is up to 30°C at the hydrothermal plumes rising from depth through karstic shafts (Frumkin et al., 2015), while the mean surface air temperature is 20°C. Ayyalon Cave groundwater pond temperature ranges from 28.5 to 29.6°C (Table 1). A thermal-infrared survey performed at the flat bottom of the quarry near the Ayyalon Cave showed groundwater temperatures varying spatially by > 3°C, in agreement with direct water temperature measurements in boreholes (Frumkin et al., 2015). The geothermal properties of the groundwater thus include ‘hot spots’ with temperatures around 2–3°C higher than in the surrounding aquifer. The sulfidic water contains no oxygen below the uppermost layer (Table 1). The spatial distribution of this anomalous water may indicate the possible limits of the aquatic ecosystem, and vice versa. Testing the isotopic features of the ecosystem can thus advance the understanding of water-ecosystem interconnection.
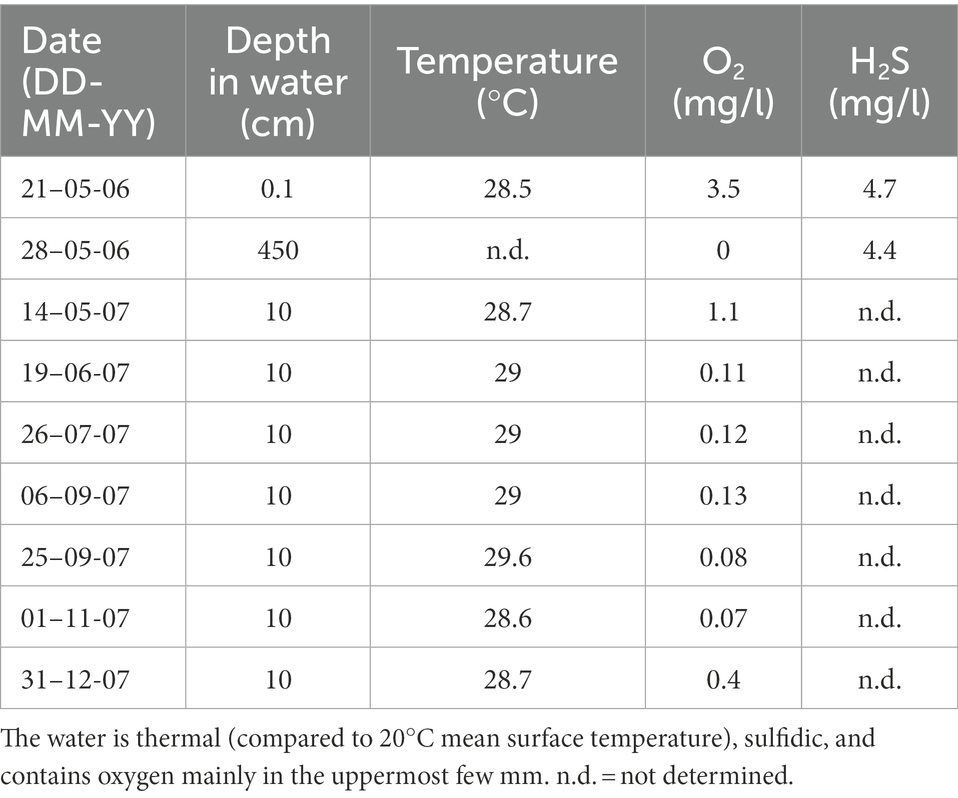
Table 1. Temperature, oxygen and hydrogen sulfide in Ayyalon Cave groundwater pond at various depths and seasons.
The plan and section of Ayyalon Cave (Figures 1C, 2B), show its affinity to the hypogene environment of rising hydrothermal plumes. The ascending groundwater caused karstic dissolution which formed a hypogenic maze, with intricate network of passages, chambers and rising-water shafts. Some of these have collapsed and advanced upwards, until reaching the surface and forming subaerial sinkholes (Frumkin et al., 2015) which serve as traps for surficial sediments, anthropogenic and zooarchaeological remains (Zaidner et al., 2014; Ullman et al., 2022).
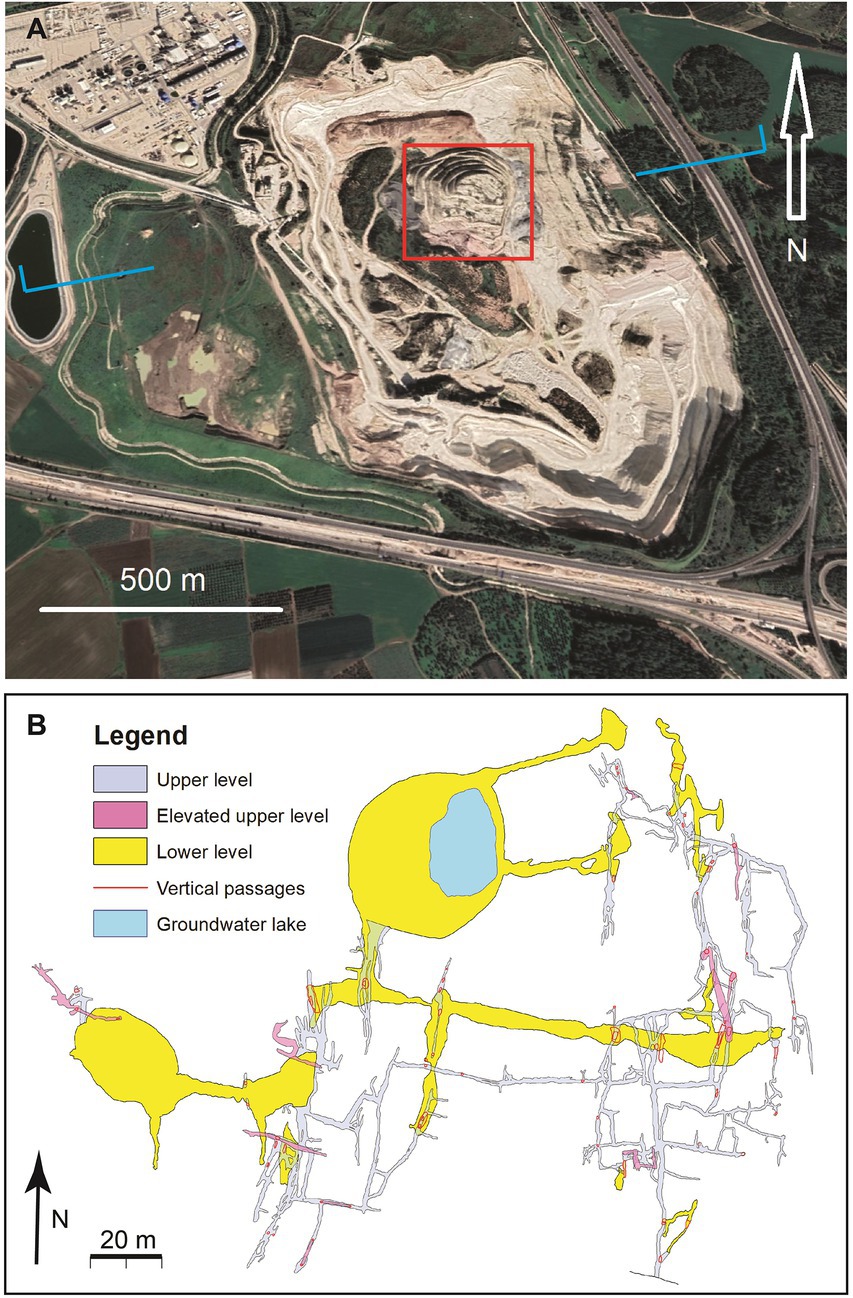
Figure 2. Plan view of Ayyalon Cave. (A) Google Earth view of Nesher-Ramla quarry with the central quarried ‘hill’ preserving the cave. Blue tags indicate general location of Figure 1C. (B) Ayyalon Cave plan, with 2.7 km long passages in three levels, vertical phreatic shafts indicating past rising water, and the groundwater pond where the ecosystem is centered.
2.2. Ayyalon microbial biota
Ayyalon Cave (and some neighboring smaller caves) is probably the only known deep (> 100 m below surface) sulfidic cave system with endemic metazoan fauna (Figure 1; Por et al., 2013). The cave ecosystem is closely associated with its groundwater pond whose surface is partly covered by microbial mats. Initial microscopic observations indicate that the microbial mass in the water includes filamentous sulfur bacteria with intracellular sulfur granules, spherical sulfur (?) bacteria, amoeboids and other protozoa. Thus the CO2 fixation may be performed by several microbial taxa. The microbial study of Ayyalon will be published elsewhere.
Metazoan taxa thrive in the water, on the microbial mats, and around the pond, where stranded relic biomats are observed. The Ayyalon Cave ecosystem demonstrates similar conditions and ecological networks to groundwater in smaller caves and drillings located a few hundred meters away (Frumkin et al., 2022).
2.3. Ayyalon fauna
In recent years, most Ayyalon Cave arthropods have been identified and described (Table 2). The species described up to 2022 include the following detritivores and predators: a copepod, a hot-spring shrimp, a crayfish, a pseudoscorpion, a scorpion, and a collembolan. They include endemic species, endemic genera, tribe and possibly endemic family (Levy, 2007; Ćurčić, 2008; Tsurnamal, 2008; Defaye and Por, 2010; Fet et al., 2011, 2017; Wagner, 2012).
Ayyalon Cave crustaceans’ stomach content has been studied microscopically in the laboratory in order to identify their food source (Tsurnamal, 2008; Wagner, 2012). Phylogenetic studies and molecular comparisons with congeneric relatives were performed on the prawn Typhlocaris ayyaloni (Guy-Haim et al., 2018).
Five of the seven arthropod species of the cave are already defined as new and endemic, restricted to the Ayyalon Cave system (Levy, 2007; Ćurčić, 2008; Tsurnamal, 2008; Defaye and Por, 2010; Fet et al., 2011, 2017; Wagner, 2012). The taxonomic position of two additional arthropod species (Metacyclops subdolus and Troglopedetes sp.) is still unclear, though probably they are also new endemics (Por et al., 2013).
2.3.1. Adaptive traits of Ayyalon fauna
The arthropod fauna of Ayyalon is partly (four species) stygobiont (obligate aquifer-dwelling), and partly (three species) troglobiont (obligate terrestrial cave-dwelling), documented around the pond in a hygropetric environment. The Ayyalon fauna show typical cave adaptations, such as the loss of eyes and pigmentation of the larger species, Typhlocaris ayyaloni (Tsurnamal, 2008) and Akrav israchanani (Levy, 2007).
In addition, some adaptations are more specific to Ayyalon fauna and similar cases. The unusual structure of pedipalp fingertips of Akrav israchanani may be an adaptive trait useful for preying on aquatic crustaceans abundant in the groundwater of the cave (Fet et al., 2011).
Laboratory observations on living Typhlocaris ayyaloni showed that these prawns do not respond to light, but are very sensitive to vibrations or to moving of objects in its vicinity (Tsurnamal, 2008). The prawns reacted by moving their sensory organs, or by getting into an alert position and retreating when an object was moving in the water as far as 15 cm away. Their stomach contents indicate that they feed directly on microbial mats and also on small crustaceans. The lack of egg-bearing females and very small prawns indicate that the groundwater pond of the cave is used by the prawns as a rich feeding ground, while other cavities of the surrounding aquifer may provide environmental conditions, such as cooler water, suitable for reproduction.
An interesting trait of the cave pond crustaceans is a predominantly upside-down swimming posture of Tethysbaena ophelicola, and the occasional back-down swimming at the pool surface of Typhlocaris ayyaloni. A similar trait was reported at the sulfurous cave stream in Frasassi, where the amphipod Niphargus ictus swims upside-down at the water surface (Flot et al., 2010). Our observation thus supports upside-down swimming to be a general adaptative trait of life for oxygen replenishing in a thin (mm thick) layer of oxygenated water at the top of the groundwater. The life of the sulfidophilic Tethysbaena under microaerobic and anaerobic conditions relates to the biochemical and physiological adaptations to anoxia of the whole crustacean order Thermosbaenacea (Por et al., 2013). Tethysbaena has developed survival mechanisms to anaerobism, resistance to hydrogen sulfide poisoning, to elevated water temperatures as well as to fluctuating dissolved mineral content. Sulfide is most probably detoxified by intestinal bacteria (Por, 2014).
3. Materials and methods
3.1. Cave measurements and sampling
A geologic-geomorphic field survey of Ayyalon Cave was conducted. The cave was surveyed using the following instruments: Leika™ ‘Disto’ laser range-meter, inclinometer and compass (Dasher, 2011); plans and cross-sections were drawn to the scale of 1:200 using LimeLight™ cave mapping software. The cave map is presented in Figure 2B. In order to avoid contamination of the cave, the equipment was cleaned and disinfected prior to field studies in the cave.
Aquatic arthropod samples were collected using 60 and 200 μm nets. Terrestrial arthropods were collected by traps and tweezers. Ultraviolet flashlights were used for locating scorpion remains. Inorganic and organic samples collected within the cave were transferred into a cooling reservoir (~5°C) until submission to the relevant laboratory (see below), which occurred within 18 h after sampling. Similar methods were used to collect aquatic and terrestrial arthropods from the surface outside the cave, for isotopic comparison. Three surface winter ponds were sampled at Innaba, (31.9080N 34.9590E). A pond, ~500 meters south-west of the quarry was not used, due to possible contamination from a nearby sewage treatment plant. Terrestrial arthropods were collected from the vicinity of the ponds.
Biological samples were separated at the Hebrew University and sent for morphological taxonomic identification by specialists of each arthropod group (Levy, 2007; Ćurčić, 2008; Tsurnamal, 2008; Defaye and Por, 2010; Fet et al., 2011, 2017; Wagner, 2012) and for isotopic analysis.
3.2. Carbon and nitrogen isotope analysis
We measured radiocarbon (coupled with δ13C) isotopic compositions which serve as direct indicators for the connectivity, carbon source of the ecosystem, and its food webs adaptations (Rundel et al., 2012). Each organic sample for 14C was pre-treated at the Weizmann Institute of Science. Any foreign carbon material which is not related to the sample and represents therefore a possible source of contamination was visually removed. Using Fourier Transform Infrared Spectroscopy (FTIR), the material was analyzed before and after pre-treatment to check the possible presence and removal of contaminants. Contaminants such as gypsum were removed by ultrasonication in distilled water. The material was then oxidized to CO2 and then graphitized for Accelerator Mass Spectrometry analysis and determination of radiocarbon content. A fraction of the CO2 was used for δ13C analysis. The AMS radiocarbon measurements were carried out on a NEC 1.5SDH-1 0.5MV Pelletron with a 40-sample MC-SNICS ion source. The accuracy of this system is higher than 0.4% and the machine background is lower than 0.03 pMC. Specific accuracy is noted per sample in Table 3. δ13C is reported by ‰ VPDB (relative to Peedee belemnite) with an accuracy of ±0.15‰. For additional data on preparation and 14C Measurement see Boaretto et al. (2009). Atmospheric isotopic values are given after Hua et al. (2021).
Organic samples were analyzed for δ13C and δ15N stable isotopes at the Institute of Earth Sciences, The Hebrew University of Jerusalem. Samples were dried in an oven at 50°C for 24 h. Calcite was removed from biofilms by adding 10% HCl. δ13C, δ15N and C/N ratios were measured by elemental analyzer / continuous flow isotope ratio mass spectrometry (EA/CF-IRMS). The system included: FlashEA 1,112 analyzer coupled with Thermo Finnigan Delta Plus XL isotope ratio mass spectrometer. The results are reported for δ13C ‰ VPDB (IAEA standard, value of zero 0.01123720) with an error up to 0.15‰, and for δ15N (‰ relative to air, IAEA-N-1 standard +0.4) with an error up to 0.15‰. Error in %C / %N ratio is up to 2%.
Inorganic samples were collected in clean glass bottles from cave pond water, mud at the pond bottom, limestone bedrock at the cave chamber wall, air above the water, and air in an upper cave passage. For dissolved inorganic δ13C in water, water was filtered in the field by 0.45 μm filter into a 100 ml glass bottle pre-treated with HgCl. An aliquot of sample was acidified with 5% H3PO4 in a gas stripping reactor and δ13C was measured for the liberated CO2 with a Thermo Scientific IRMS system with an accuracy of 0.1‰ VPDB. For solid-phase calcite samples, δ13C was measured by gas bench continuous-flow isotope ratio mass spectrometry (CF-IRMS) system including GasBench III connected to Delta Plux XL mass spectrometer with an error up to 0.1‰ (VPDB).
To test whether the difference between the cave and surface samples in Ayalon differ significantly in their isotope values we applied two complementary multivariate approaches, namely a parametric MANOVA (Clarke, 1993, using SPSS statistical package) and a non-parametric ANOSIM (Clarke, 1993) modification (using MATLAB software). For the MANOVA analysis we used Pillai’s trace parameter defined between zero and one where zero indicates no difference between the groups and a value closer to one indicates a more significant difference. In ANOSIM the R statistic is used, where R is expected to equal zero when groups do not differ and to be greater than zero when they do. The ANOSIM analysis was performed by comparing the observed Ro statistic to 10,000 random values of the statistic, Re, expected under the null hypothesis. The values of the statistic were calculated using distance matrix based on Euclidean distances. Random statistic values were obtained by randomly shuffling the observations groupings.
Table 4 shows the stable isotopes values, in which each number is averaged between two measurements.
3.3. Water measurement and analysis
Water temperature and oxygen concentration were measured in 10 cm depth of the cave pond using a Solinst Levellogger DIVER 3001. Dissolved oxygen was measured there with a WTW OXI 330 hand-held meter. Temperature, oxygen and hydrogen sulfide of the groundwater pond at various depths and seasons are given in Table 1.
Water from Ayyalon Cave pond was sampled directly from the water surface using a peristaltic pump from 10 to 450 cm depth. Each sample for H2S concentration was collected in three 1.5 liter bottles. Sulfide was precipitated in the field as ZnS by adding 1–2 ml 1N Zn-acetate to 1 liter sampling bottles prior to sampling. The samples were analyzed at the Geological Survey of Israel. Excess water was removed in the lab, and the remaining deposit and water were reacted in a beaker with KI, HCl, and I2, the latter in excess, which was then titrated using S2O3. For additional details see Gavrieli et al. (2001).
3.4. Primary productivity estimation
The 14C incubation method was used to estimate the uptake of dissolved inorganic carbon (DIC) by microbial mats from Ayyalon groundwater, as was done earlier for other chemoautotrophic caves (Porter et al., 2009). The method is based on the fact that the biological uptake of 14C-labeled DIC is proportional to the biological uptake of 12C-DIC. The incubation was performed in Aharon Oren’s laboratory at the Hebrew University according to Oren (2009).
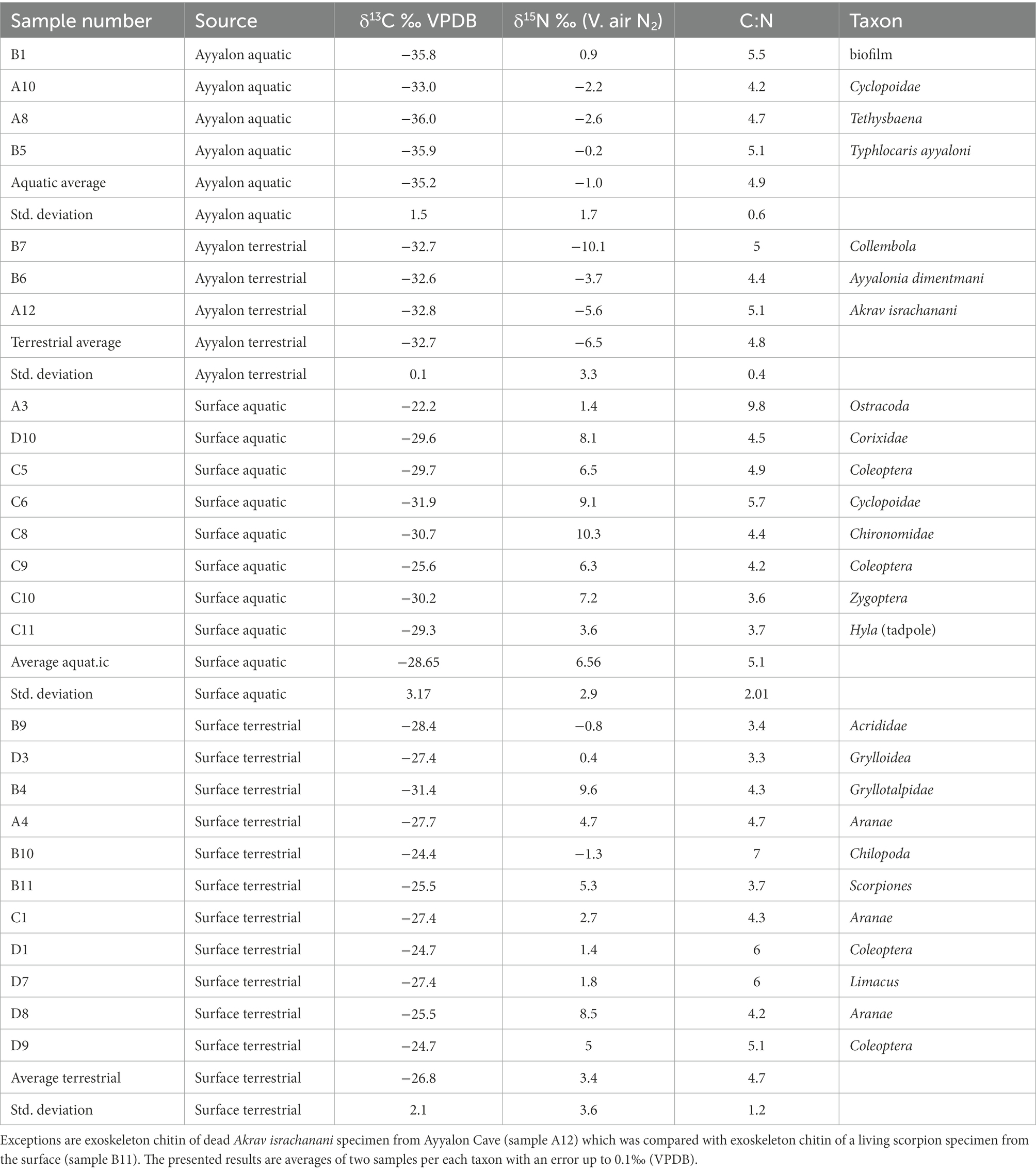
Table 4. Carbon and nitrogen stable isotope values of whole-body biogenic materials in Ayyalon Cave and associated surface near the cave.
4. Results and discussion
4.1. Radiocarbon
During the study, the atmospheric 14C content of CO2 outside the cave, in the upper cave levels, and above the groundwater pond were 104.0, 97.93, and 71.36 pMC, respectively, (Table 3; Figure 3A). In contrast, inorganic carbon compounds associated with the cave water and bedrock were highly depleted in 14C: The Cretaceous bedrock, mud (at the bottom of the pond), and inorganic dissolved carbon of the groundwater contained 0.0, 0.72, and 23.34 pMC, respectively. The mud value represents the dominance of old, ‘dead’ carbon in the inorganic mud, while the dissolved carbon includes a signature of some recent groundwater flowing laterally from the east.
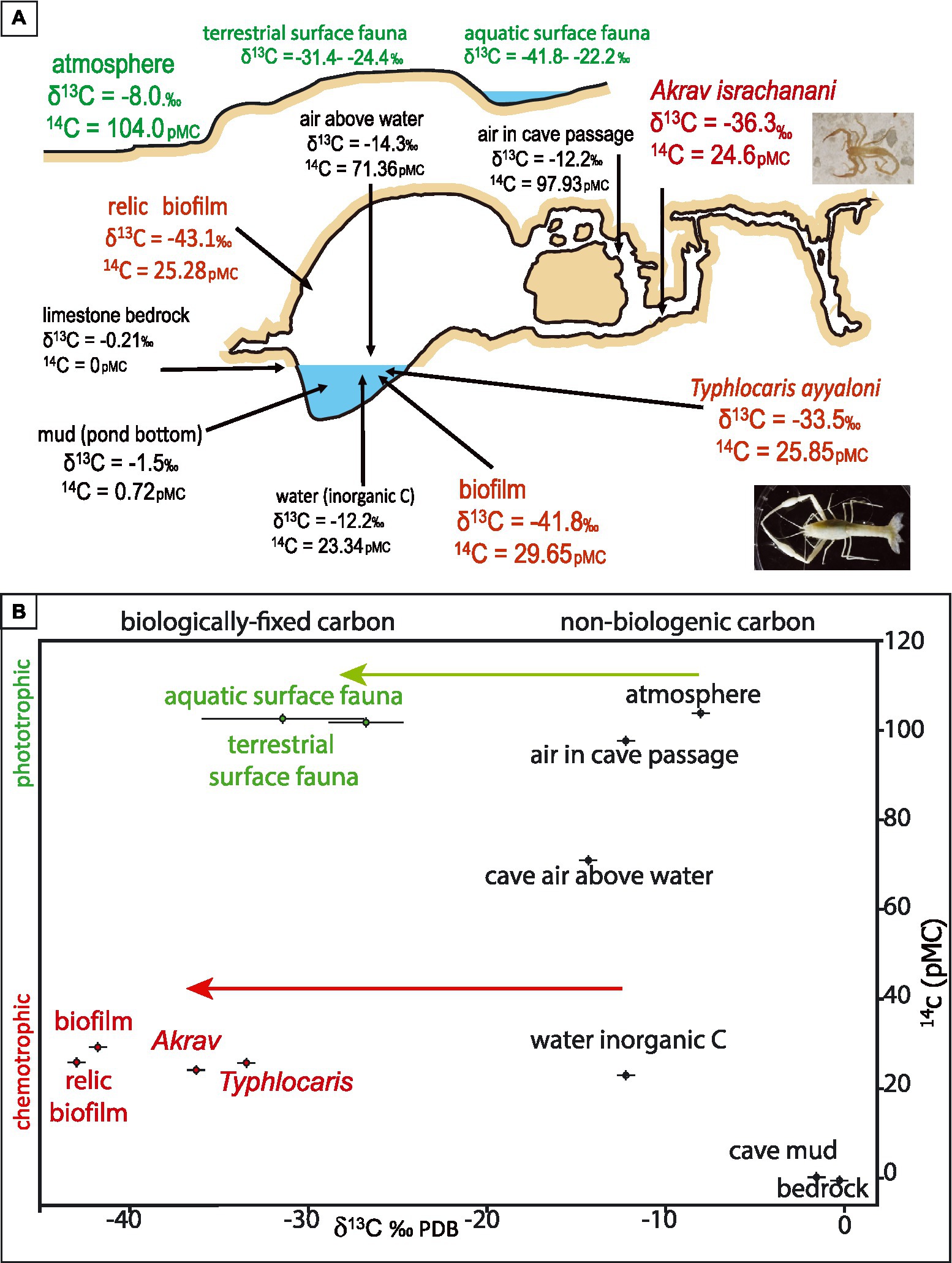
Figure 3. Carbon isotopes of the Ayyalon cave and surrounding surface, showing the isotopic difference between the surface phototrophic (green) and cave chemotrophic (red) systems. 14C of surface fauna was assumed to replicate the atmospheric value. (A) cartoon indicating the measured values of the samples and their general location. (B) the relation between 14C and δ13C of the system’s components. Green arrow represents ~ − 20‰ fractionation associated with photosynthesis by C3 plants, subsequently consumed by surface fauna (shown). Red arrow represents fractionation associated with chemoautotrophic sulfur-oxidizing bacteria (Ruby et al., 1987). Individual samples with 1σ laboratory error are shown, except for surface fauna, whose gross diversity is represented by the average values of 15 aquatic species and 11 terrestrial species, with 1σ standard deviation of each group (further details in Tables 3, 4).
The measured 14C content in the cave organisms was 29.65, 25.28, 25.85, and 24.6 pMC for the living biofilm, stranded relic biofilm (hanging above the pond, associated with previous high watertables), living aquatic prawn Typhlocaris ayyaloni and exoskeletons of the scorpion Akrav israchanani respectively, representing the highest trophic level of the aquatic and terrestrial Ayyalon ecosystem (Table 3; Figure 3A).
The intake of dissolved carbon from groundwater is further demonstrated by incubation of the biofilm with 14C-labeled bicarbonate (Figure 4). Ayyalon microbiota, sampled from the sulfidic cave pond, was incubated with 14C-labelled bicarbonate. Increase of radioactivity of the microbial lipids during incubation indicates uptake of the radiolabeled bicarbonate by Beggiatoa, suggesting that these sulfur-oxidizing microorganisms use water-dissolved inorganic carbon for chemolithoautotrophic carbon fixation. The experiment was performed with and without adding sulfide, showing similar results. Beggiatoa sp. of the microbial mat was sampled from the top of the water column (upper lines in Figure 4), and showed active CO2 fixation. Lower lines (Figure 4) indicate no carbon fixation by other bacteria sampled from the water body.
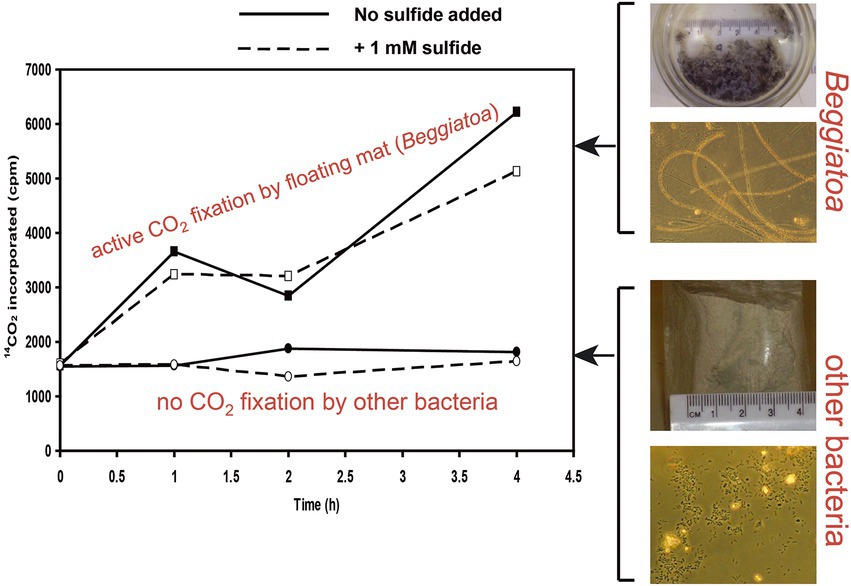
Figure 4. Incubation of Ayyalon microbiota, sampled from the sulfidic cave pond, with 14C-labelled bicarbonate. On the right, pictures show bulk samples as sampled within glass bottles, and microscopic view of Beggiatoa sp. and other bacteria. On the left, upper lines show active CO2 fixation by Beggiatoa sp. of the microbial mat sampled from the top of the water column. Lower lines indicate no carbon fixation by other bacteria sampled from the water body. Beggiatoa vs. other bacteria were differentiated by morphology and sulfur content. The increase of radioactivity of the microbial lipids during incubation indicates uptake of the radiolabeled bicarbonate by Beggiatoa, suggesting that these sulfur-oxidizing microorganisms use the water-dissolved inorganic carbon for chemolithoautotrophic carbon fixation. The experiment was performed with and without adding sulfide (dashed and solid lines respectively), showing similar results.
4.2. Stable isotopes
Stable isotopes (δ13C and δ15N) and C/N ratios of the biotic system were measured for terrestrial and aquatic organisms inside the cave, and for comparison, from similar taxonomic groups on the regional surface above the cave (Table 4; Figures 3, 5). The measurements inside the cave included the entire range of organisms, from biofilms through the living aquatic prawn Typhlocaris ayyaloni and exoskeletons of the scorpion Akrav israchanani.
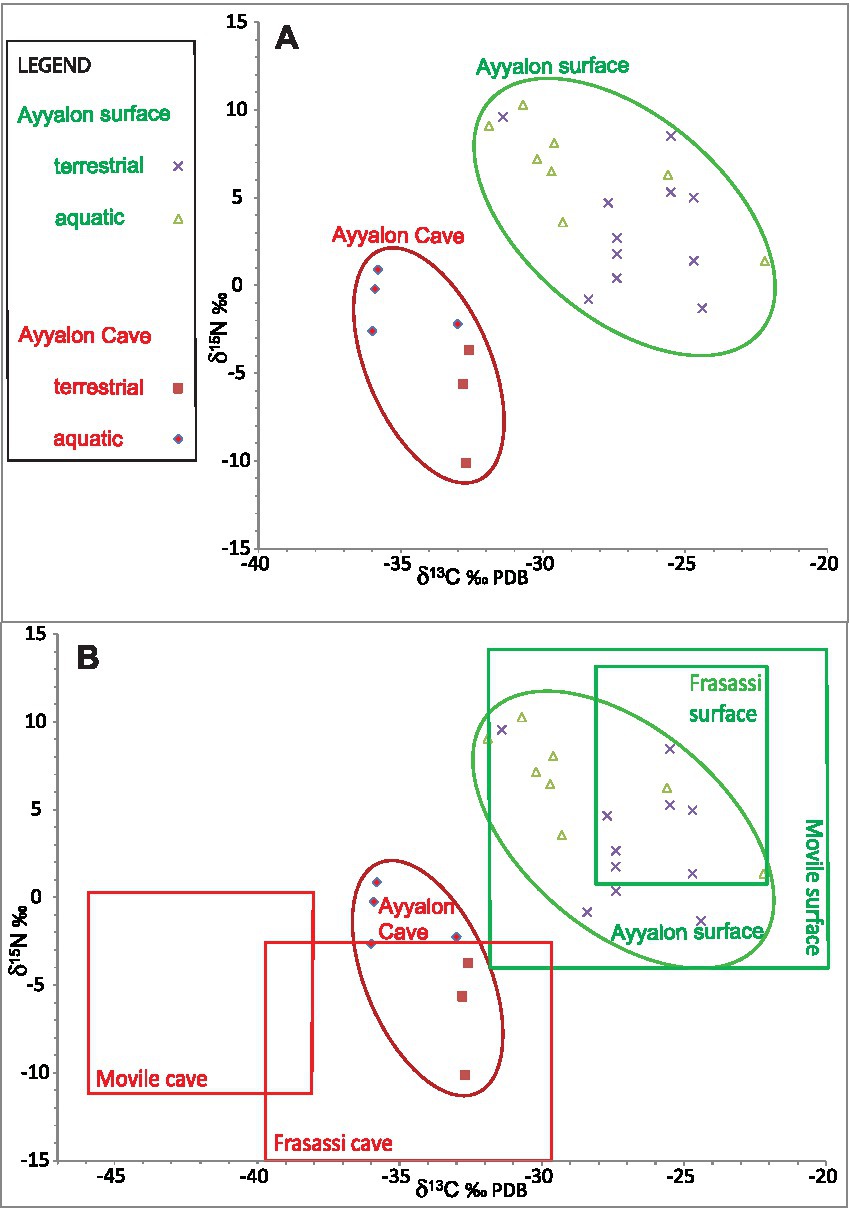
Figure 5. Bi-plots of nitrogen vs. carbon isotopes of organic matter at Ayyalon (present study), compared with previously studied caves. (A) from Ayyalon cave (red) and surrounding surface (green), divided to terrestrial and aquatic ecosystems. Cave and surface values fall in distinctively different fields: Organic matter in the cave is much lighter isotopically than respective surface values. Higher trophic levels generally fall to the right of their food source. Further details on individual samples are given in Table 4. (B) comparison between Ayyalon’s data (ellipses) and general fields of similar measurements in two other sulfidic cave ecosystems and their surficial counterparts (rectangles): Movile, Romania (Sarbu et al., 1996) and Frasassi, Italy (Sarbu et al., 2000). The distinctive isotopic depletion is shared by the three caves, suggesting similar controlling factors, with local variations in values.
Cave vs. surface isotopic values fall in distinctively different fields: Organic matter in the cave is lighter isotopically than surface values (for isotopic ranges, see Figure 5). Higher trophic levels generally fall to the right of their food source.
4.3. Inference from isotopic values
Radiocarbon is used here to decipher the ecosystem’s connectivity with the surface. Recently recharged groundwater, arriving from rainfall, carry an atmospheric signature. In the Judean hills, the radiocarbon content in the groundwater bicarbonate is ~80 pMC, representing mainly a combination of biogenic soil CO2 and smaller portion of old bedrock carbonate. At Ayyalon, our measured 14C values of air CO2 outside the cave, in the upper cave levels, and above the groundwater pond were 104.0, 97.93, and 71.36 pMC, respectively, (Table 3; Figure 3A).
The negligible 14C content of the cave mud (Table 3; Figure 3A) indicates the dominance of decomposed bedrock source. The 71.36 pMC of air above the water indicates the mixing of atmospheric CO2 from the upper cave levels with depleted carbon from the water. The 23.34 pMC of the cave groundwater inorganic carbon is highly depleted in 14C, in clear contrast with both the young atmosphere above the cave and the regional young groundwater. This indicates that the cave groundwater is mostly old, rising from the depth, after a long-term 14C decay and interaction with carbonate bedrock, in contrast with regional young groundwater, whose 14C content was ~80 pMC (Kroitoru et al., 1992). This verifies the hydrogeologic data, suggesting that Ayyalon groundwater comprises hydrothermal plumes rising from depth (Frumkin and Gvirtzman, 2006; Frumkin et al., 2015).
Cave water oxygen is limited to 0.02–3.5 mg/l, within the uppermost mm-thick groundwater layer, while deeper water demonstrates anaerobic conditions. This redox interface and associated environment, although poor in oxygen, allows for the rich metazoan life within the groundwater. Specific adaptations to these environmental conditions are discussed above (Section 2.3).
The Ayyalon 14C results (Table 3; Figure 3) indicate that: (1) the microbial biota (29.65 pMC) use mainly bicarbonate from the groundwater (23.34 pMC) with a smaller fraction of atmospheric CO2 from above the water (71.36 pMC); and (2) the depleted 14C signal passes through the entire ecosystem, indicating that the ecosystem is totally based on the biofilm which is in turn based on groundwater-dissolved inorganic carbon.
The increase of radioactivity of the microbial lipids during incubation of the biofilm with 14C-labeled bicarbonate (Figure 4) indicates uptake of the radio-labeled bicarbonate from groundwater, suggesting that the sulfur-oxidizing proteobacteria Beggiatoa use the water-dissolved inorganic carbon for chemolithoautotrophic carbon fixation. This is corroborated by the observation that the cave lacks any organic or inorganic material originating from the surface, due to the cave’s isolation.
Further insight into Ayyalon ecosystem and its adaptation is obtained from stable isotopes (δ13C and δ15N) of the biotic system (Table 4; Figure 5). Most organisms with a chemolithoautotrophic diet have quite negative δ13C values (e.g., –38 to −46‰ in Movile; −30 to −40‰ in Frasassi; Sarbu et al., 1996, 2000). This is mainly due to discrimination against 13C, associated with chemolithoautotrophic assimilation of inorganic carbon. Methane-driven chemoautotrophic carbon-fueled ecosystems can have δ13C values from −30 to −90‰ (Grey, 2016). While δ13C of dissolved inorganic carbon of Ayyalon Cave water is −12.2‰ VPDB, the δ13C of the biofilm is −41.8 and − 43.1‰ (for live and stranded relic samples respectively, Figure 3), slightly larger than expected for fractionation of stable carbon isotopes during chemolithoautotrophic growth of sulfur-oxidizing bacteria between the inorganic carbon of the water and the chemoautotrophic microbiome of the water (Degens, 1969; Ruby et al., 1987; Galimov, 2006). The reasons causing the difference should be further investigated. The depletion is larger than observed in most photosynthetically-derived organic material. The largest degree of fractionation of carbon isotopes occurs during the initial steps of reduction of inorganic carbon (i.e., carbon dioxide and bicarbonate) and results in a decreased 13C/12C isotope ratio in the organic product relative to that of the source carbon. Subsequent utilization of the organic carbon through trophic levels of the food chain leads to small but detectable increases in the 13C/12C isotopic ratio (Ruby et al., 1987; Galimov, 2006). Alternatively, isotopically light carbon may rise from the depths as 13C-depleted methane, followed by oxidation to CO2 and bicarbonate near the water-table, being then metabolized by the biofilm into organic matter (Grey, 2016).
Mean δ13C of aquatic and terrestrial species in the cave is −35.2 ± 1.5 and − 32.7 ± 0.1‰ respectively, both much lower than surface samples averaging −28.65 and − 26.8 ± 2.1‰ respectively, which are dominated by the lower carbon fractionation of C3 photosynthetic vegetation (Figure 3). Within the cave ecosystem, the higher trophic levels show variable isotopic shift relative to their diet (Figure 3B), with a positive shift in both aquatic (−33.0 to −36.3‰, Table 4) and terrestrial fauna (−32.6 to −33.5‰). This is in agreement with the expected isotopic shifts between diets and consumers (Galimov, 2006).
Similarly, the mean δ15N of Ayyalon aquatic and terrestrial organic samples is −1.0 ± 1.7‰ and − 6.5 ± 3.3‰ respectively, both lower than surface samples, whose average measured values are 6.56 ± 2.9 and 3.4 ± 3.6‰ respectively (Figure 5A). The negative trophic shift of N may be indicative of fluid-feeding consumers. McCutchan et al. (2003) showed that estimates of trophic shift for nitrogen differ systematically between fluid-feeding consumers and other consumers.
Both multivariate tests used here (MANOVA and ANOSIM; Clarke, 1993) indicate a highly significant effect of habitat (cave vs. surface) on the isotopic values of the samples. Pillai’s trace value was close to unity (0.995) with F(2,22) = 2,402 and sig. < 0.0001. The ANOSIM analysis produced similar results with the observed statistic, Ro = 0.0267, being significantly greater than zero – sig. < 0.0001 (Figure 6).
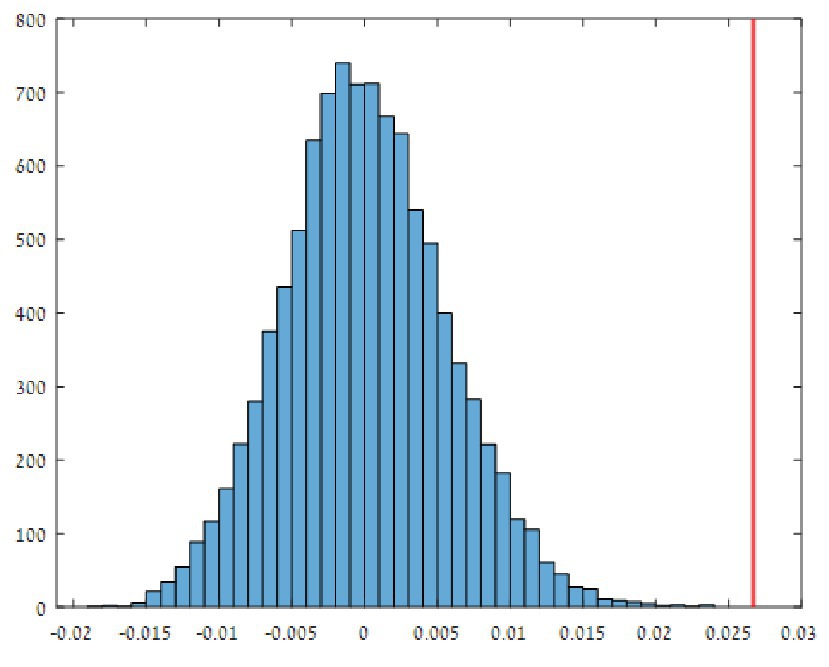
Figure 6. Results of analysis of similarity (ANOSIM) analysis with Euclidean distance as a dissimilarity metric between pairs of samples, generated by matrix permutation. A histogram of 10,000 random Re values is compared with the observed Ro value (Ro = 0.0267; red line), using MATLAB. This indicates a highly significant effect of habitat (cave vs. surface) on the isotopic values of the samples.
The combined evidence suggests that the sulfur-oxidizing microbiota obtain the necessary carbon for metabolic processes from bicarbonate in the groundwater, as well as nitrogen from nitrogen compounds in the water. The biofilm covering the cave water is thus the primary producer for the entire food chain in the cave, both inside and outside the water.
The higher trophic levels in Ayyalon are evident also by direct observations: The small crustacean Tethysbaena ophelicola has only biofilm cells in its stomach, and is itself found in the stomachs of the other crustaceans (Wagner, 2012), indicating that this species is a primary consumer in the Ayyalon ecosystem. The largest crustacean of Ayyalon, Typhlocaris ayyaloni, feeds also on biofilms in addition to predation of other crustaceans (Tsurnamal, 2008). This is in agreement with the isotopic evidence presented above.
The observed isotopic depletion relative to surface values at Ayyalon are in agreement with measurements in other sulfidic caves ecosystems, such as Movile (Sarbu et al., 1996), and Frassasi (Sarbu et al., 2000; Figure 5). All these caves show compatible isotopic differences between the surface ecosystem and the subsurface sulfidic chemolithoautotrophy counterpart (Figure 5B). These offsets of δ13C values of organisms inside the cave vs. outside are −18 to −14‰ at Movile, −8 to −12‰ at Frassasi, and − 4 to −11‰ at Ayyalon Cave. The offsets of δ15N are −7 to −14‰ at Movile, −16 to −17‰ at Frassasi, and − 9 to −10‰ at Ayyalon Cave. This indicates that the biofilms in all these sulfidic environments make similar use of carbon and nitrogen from deep sources rather than from the atmosphere.
However, there are basic differences between the various cave systems. Frassasi Cave has a better connection with the surface, including a natural entrance with open outflow of its subsurface sulfidic stream (Sarbu et al., 2000). The more secluded Movile Cave has a groundwater pond, is located under 20 m-thick caprock and soil, and is a rich, ecosystem with many endemic species (Sarbu et al., 1996; Brad et al., 2021). With several faunistic pieces of evidence indicating intermittent connection with the surface, Movile is believed to have been colonized by various species over a much shorter period than Ayyalon, including the late Quaternary. Other known chemolithoautotrophic caves, including anchialine ones, seem to be less isolated than Ayyalon, and commonly have less developed endemic ecosystems (Pohlman et al., 1997; Pohlman, 2011; Hutchins et al., 2016; Engel, 2019).
4.4. Endemism
The taxa of Ayyalon include endemic faunal tribes and/or families (the scorpion Akrav israchanani and the pseudoscorpion Ayyalonia dimentmani) evolving independently since the Miocene (Levy, 2007; Ćurčić, 2008; Fet et al., 2011). The high-rank endemism and the deep, well-covered setting, suggest that Ayyalon is one of the most secluded metazoan cave ecosystems known today, whose biota may have been virtually disconnected from other ecosystems for millions of years (Ćurčić, 2008; Fet, 2017; Guy-Haim et al., 2018; Frumkin et al., 2022). However, the stable isotopes data from Movile and Frasassi caves indicate that they are more depleted than their associated surface ecosystems compared with the Ayyalon system (Figure 5B). This might be associated with different emissions of deep-sourced gases, such as methane, in each cave.
The high endemism, long-term (millions of years) resilience (Frumkin et al., 2022), and in situ evolution of the Ayyalon fauna suggest that similar chemolithoautotrophic ecosystems could be self-sustained for long (geologic scale) periods, allowing significant behavioral and physiological evolution, associated with adaptations to subsurface secluded conditions (section 2.3.1).
5. Conclusion
This study shows that under the lack of epigenic sources of carbon and energy, the Ayyalon Cave ecosystem has adapted by using internal carbon and energy sources. This is demonstrated, for the first time, by the low 14C content of the ecosystem, indicating the uptake of inorganic carbon from old groundwater. The dead carbon used by microbial mats in the cave is recognized throughout all the trophic levels, demonstrating that in such ecosystems dead organisms cannot be dated by 14C.
The 14C evidence is corroborated by the stable isotopic values of the ecosystem, which show a major depletion of C and N isotopes throughout the cave ecosystem. The low values of δ13C and δ15N indicate underground chemolithoautotrophic production, rather than allochthonous import from epigean systems. The high endemism of the ecosystem shows that it has been detached from surface terrestrial and marine environments for a long period, amounting to millions of years. Our initial assumptions of the isotopic values of such an ecosystem thus prove to be true.
Our results also demonstrate the sustainability and adaptation of a deep isolated subsurface ecosystem. This may suggest the possibility of long-lived cave ecosystems early in the evolution of terrestrial life, and may shed light on potential extraterrestrial ecosystems. The subsurface of Mars and other planets is protected from the damaging effects of short-wave UV radiation and the oxidizing nature of the surface, thus providing a promising habitat for life (Chyba and Hand, 2005; Kaltenegger, 2017). In particular, caves and other subsurface environments may be habitable where sufficient geothermal heating permits the existence of liquid water. Such features make subsurface environments on exoplanets seem more plausible for life than their surface counterparts.
The secluded Ayyalon system is in great danger since it was breached by the quarry. The Nature Reserves Authority of Israel is urged to take active measures of conservation in order to avoid disturbance to the fragile system, and keep it as secluded as possible.
Data availability statement
The raw data supporting the conclusions of this article will be made available by the authors, without undue reservation.
Ethics statement
We declare that the research have been conducted in accordance with Frontiers guidelines on study ethics. The study is exempt from ethics approval, due to the following reasons: No vertebrates have been involved in the study. No research on human subjects have been conducted.
Author contributions
AF coordinated the study and wrote the paper. AC ensured biologic validity and edited the text. IN performed the field work as part of his thesis. All authors contributed to the article and approved the submitted version.
Funding
The Israel Hydrological Service and Israel Nature and Parks Authority financed the study (grant #0321869).
Acknowledgments
We are grateful for the following assistance: Elisabetta Boaretto of the Kimmel Center for Archaeological Science, Weizmann Institute of Science, helped with the laboratory analysis of Ayyalon carbon isotopes. Chanan Dimentman of the Hebrew University separated faunal samples and sent them for definition. Aharon Oren of the Hebrew University helped with the incubation of Ayyalon’s biofilm with radio-labeled 14C bicarbonate. Boaz Luz and Eugeni Barkan helped with C and N isotopic analysis. Ronen Ron of the Hebrew University performed the statistical analysis. Oren Kolodny and Efrat Gavish-Regev of the Hebrew University, Serban Sarbu of California State University and Amitai Cooper of Intel provided useful advice.
Conflict of interest
The authors declare that the research was conducted in the absence of any commercial or financial relationships that could be construed as a potential conflict of interest.
Publisher’s note
All claims expressed in this article are solely those of the authors and do not necessarily represent those of their affiliated organizations, or those of the publisher, the editors and the reviewers. Any product that may be evaluated in this article, or claim that may be made by its manufacturer, is not guaranteed or endorsed by the publisher.
References
Bishop, R., Humphreys, W. F., and Longley, G. (2014). Epigean and hypogean Palaemonetes sp. (Decapoda, Palaemonidae) from Edwards aquifer: an examination of trophic structure and metabolism. Subterr. Biol. 14, 79–102. doi: 10.3897/subtbiol.14.8202
Boaretto, E., Wu, X., Yuan, J., Bar-Yosef, O., Chu, V., Pan, Y., et al. (2009). Radiocarbon dating of charcoal and bone collagen associated with early pottery at Yuchanyan cave, Hunan Province, China. Proc. Natl. Acad. Sci. 106, 9595–9600. doi: 10.1073/pnas.0900539106
Brad, T., Iepure, S., and Sarbu, S. M. (2021). The chemoautotrophically based Movile Cave groundwater ecosystem, a hotspot of subterranean biodiversity. Diversity 13:128. doi: 10.3390/d13030128
Chyba, C. F., and Hand, K. P. (2005). Astrobiology: the study of the living universe. Annu. Rev. Astron. Astrophys. 43, 31–74. doi: 10.1146/annurev.astro.43.051804.102202
Clarke, K. R. (1993). Nonparametric multivariate analyses of changes in community structure. Aust. J. Ecol. 18, 117–143. doi: 10.1111/j.1442-9993.1993.tb00438.x
Culver, D. C., and Pipan, T., (2019). The Biology of Caves and Other Subterranean Habitats. Oxford: Oxford University Press.
Ćurčić, B. P. M. (2008). Ayyalonia dimentmani ng, n. sp. (Ayyaloniini n. trib., Chthoniidae, Pseudoscorpiones) from a cave in Israel. Archiv. Biol. Sci. 60, 331–339. doi: 10.2298/ABS0803331C
Dasher, G. R., (2011). On Station: A Complete Handbook for Surveying and Mapping Caves. Huntsville, AL: National Speleological Society.
Dattagupta, S., Schaperdoth, I., Montanari, A., Mariani, S., Kita, N., Valley, J. W., et al. (2009). A novel symbiosis between chemoautotrophic bacteria and a freshwater cave amphipod. ISME J. 3, 935–943. doi: 10.1038/ismej.2009.34
Defaye, D., and Por, F. D. (2010). Metacyclops (Copepoda, Cyclopidae) from Ayyalon cave, Israel. Crustaceana 83, 399–423. doi: 10.1163/001121610X12627655658320
Degens, E. T. (1969). “Biogeochemistry of Stable Carbon Isotopes” in Organic Geochemistry. eds. G. Eglinton and M. T. J. Murphy (Berlin, Heidelberg: Springer). doi: 10.1007/978-3-642-87734-6_14
Desai, M. S., Assig, K., and Dattagupta, S. (2013). Nitrogen fixation in distinct microbial niches within a chemoautotrophy-driven cave ecosystem. ISME J. 7, 2411–2423. doi: 10.1038/ismej.2013.126
Engel, A. S. (2007). Observations on the biodiversity of sulfidic karst habitats. J. Cave Karst Stud. 69, 187–206.
Engel, A. S. (2019). “Chemolithoautotrophy” in Encyclopedia of Caves. eds. W. B. White, D. C. Culver, and T. Pipan (Academic Press). 3rd edn. 267–276. doi: 10.1016/B978-0-12-814124-3.00030-3
Fet, V., Soleglad, M. E., and Zonstein, S. L. (2011). The genus Akrav Levy, 2007 (Scorpiones: Akravidae) revisited. Euscorpius 2011, 1–49. doi: 10.18590/euscorpius.2011.vol2011.iss134.1
Fet, V., Soleglad, M. E., Zonstein, S. L., Naaman, I., Lubaton, S., Langford, B., et al. (2017). The second record of a relict Akrav israchanani Levy, 2017 (Scorpiones: akravidae) from Levana cave, Israel. Euscorpius 2017, 1–12. doi: 10.18590/euscorpius.2017.vol2017.iss247.1
Flot, J. F., Wörheide, G., and Dattagupta, S. (2010). Unsuspected diversity of Niphargus amphipods in the chemoautotrophic cave ecosystem of Frasassi, Central Italy. BMC Evol. Biol. 10, 1–13. doi: 10.1186/1471-2148-10-171
Frumkin, A., Dimentman, C., and Naaman, I. (2022). Biogeography of living fossils as a key for geological reconstruction of the East Mediterranean: Ayyalon-Nesher Ramla system, Israel. Quat. Int. 624, 168–180. doi: 10.1016/j.quaint.2020.11.036
Frumkin, A., and Gvirtzman, H. (2006). Cross-formational rising groundwater at an artesian karstic basin: the Ayalon saline anomaly, Israel. J. Hydrol. 318, 316–333. doi: 10.1016/j.jhydrol.2005.06.026
Frumkin, A., Zaidner, Y., Na'aman, I., Tsatskin, A., Porat, N., and Vulfson, L. (2015). Sagging and collapse sinkholes over hypogenic hydrothermal karst in a carbonate terrain. Geomorphology 229, 45–57. doi: 10.1016/j.geomorph.2014.08.001
Galimov, E. M. (2006). Isotope organic geochemistry. Org. Geochem. 37, 1200–1262. doi: 10.1016/j.orggeochem.2006.04.009
Gavrieli, I., Yechieli, Y., Halicz, L., Spiro, B., Bein, A., and Efron, D. (2001). The sulfur system in anoxic subsurface brines and its implication in brine evolutionary pathways: the ca-chloride brines in the Dead Sea area. Earth Planet. Sci. Lett. 186, 199–213. doi: 10.1016/S0012-821X(01)00247-3
Grey, J. (2016). The incredible lightness of being methane-fuelled: stable isotopes reveal alternative energy pathways in aquatic ecosystems and beyond. Front. Ecol. Evol. 4:8. doi: 10.3389/fevo.2016.00008
Guy-Haim, T., Simon-Blecher, N., Frumkin, A., Naaman, I., and Achituv, Y. (2018). Multiple transgressions and slow evolution shape the phylogeographic pattern of the blind cave-dwelling shrimp Typhlocaris. Peer J 6:e5268. doi: 10.7717/peerj.5268
Hose, L. (2013). “Karst geomporphology: sulfur karst processes” in Treatise on geomorphology. eds. J. Shroder and A. Frumkin (San Diego, CA: Academic Press), 29–37.
Hose, L. D., and Pisarowicz, J. A., (1999). Cueva de villa luz, Tabasco, Mexico: Reconnaissance study of an active sulfur spring cave and ecosystem. Journal of Cave and Karst Studies 61,13–21.
Hua, Q., Turnbull, J. C., Santos, G. M., Rakowski, A. Z., Ancapichún, S., De Pol-Holz, R., et al. (2021). Atmospheric radiocarbon for the period 1950–2019. Radiocarbon 64, 1–23. doi: 10.1017/RDC.2021.95
Hutchins, B. T., Engel, A. S., Nowlin, W. H., and Schwartz, B. F. (2016). Chemolithoautotrophy supports macroinvertebrate food webs and affects diversity and stability in groundwater communities. Ecology 97, 1530–1542. doi: 10.1890/15-1129.1
Kaltenegger, L. (2017). How to characterize habitable worlds and signs of life. Annu. Rev. Astron. Astrophys. 55, 433–485. doi: 10.1146/annurev-astro-082214-122238
Kiefer, F. (1938). Cyclopiden (Crust. Cop.) aus süditalienischen Brunnen und Höhlen. Zoologischer Anzeiger 123, 1–12.
Kroitoru, L., Mazor, E., and Issar, A. (1992). Flow regimes in karstic systems: the Judean anticlinorium, Central Israel. Int. Continental Hydrogeol. 13, 339–354.
Levy, G. (2007). The first troglobite scorpion from Israel and a new chactoid family (Arachnida: Scorpiones). Zool. Middle East 40, 91–96. doi: 10.1080/09397140.2007.10638209
McCutchan, J. H. Jr., Lewis, W. M. Jr., Kendall, C., and McGrath, C. C. (2003). Variation in trophic shift for stable isotope ratios of carbon, nitrogen, and sulfur. Oikos 102, 378–390. doi: 10.1034/j.1600-0706.2003.12098.x
Northup, E., Kathleen, H., and Lavoie, D. (2001). Geomicrobiology of caves: a review. Geomicrobiol J. 18, 199–222. doi: 10.1080/01490450152467750
Oren, A. (2009). Saltern evaporation ponds as model systems for the study of primary production processes under hypersaline conditions. Aquat. Microb. Ecol. 56, 193–204. doi: 10.3354/ame01297
Palmer, A. N. (2013). “Sulfuric acid caves: morphology and evolution” in Treatise on Geomorphology. eds. J. Shroder and A. Frumkin (San Diego, CA: Academic Press), 241–257.
Parker, C. W., Auler, A. S., Barton, M. D., Sasowsky, I. D., Senko, J. M., and Barton, H. A. (2018). Fe (III) reducing microorganisms from iron ore caves demonstrate fermentative Fe (III) reduction and promote cave formation. Geomicrobiol J. 35, 311–322. doi: 10.1080/01490451.2017.1368741
Pohlman, J. W. (2011). The biogeochemistry of anchialine caves: progress and possibilities. Hydrobiologia 677, 33–51. doi: 10.1007/s10750-011-0624-5
Pohlman, J. W., Iliffe, T. M., and Cifuentes, L. A. (1997). A stable isotope study of organic cycling and the ecology of an anchialine cave ecosystem. Mar. Ecol. Prog. Ser. 155, 17–27. doi: 10.3354/meps155017
Por, F. (2014). Sulfide shrimp? Observations on the concealed life history of the Thermosbaenacea (Crustacea). Subterr. Biol. 14, 63–77. doi: 10.3897/subtbiol.14.7927
Por, F. D., Dimentman, C., Frumkin, A., and Naaman, I. (2013). Animal life in the chemoautotrophic ecosystem of the hypogenic groundwater cave of Ayyalon (Israel): a summing up. Nat. Sci. 05, 7–13. doi: 10.4236/ns.2013.54A002
Porter, M. L., Engel, A. S., Kane, T. C., and Kinkle, B. K. (2009). Productivity-diversity relationships from chemolithoautotrophically based sulfidic karst systems. International Journal of Speleology 38:4.
Ruby, E. G., Jannasch, H. W., and Deuser, W. G. (1987). Fractionation of stable carbon isotopes during chemoautotrophic growth of sulfur-oxidizing bacteria. Appl. Environ. Microbiol. 53, 1940–1943. doi: 10.1128/aem.53.8.1940-1943.1987
Rundel, P. W., Ehleringer, J. R., and Nagy, K. A. (eds.), (2012). Stable Isotopes in Ecological Research. New York, NY: Springer-Verlag.
Sârbu, S. M. (2001). “Movile, a chemoautotrophically based ecosystem” in Subterranean Ecosystems of the World 30. eds. H. Wilkens, D. D. Culver, and W. F. Humphreys (Amsterdam: Elsevier), 319–343.
Sarbu, S., Galdenzi, S., Menichetti, M., and Gentile, G. (2000). “Geology and biology of the Frasassi caves in Central Italy: an ecological multi-disciplinary study of a Hypogenic underground ecosystem” in Subterranean Ecosystems. ed. C. D. Wilkens H (New York, NY: Elsevier). 359–378.
Sarbu, S. M., Kane, T. C., and Kinkle, B. K. (1996). A chemoautotrophically based cave ecosystem. Science 272, 1953–1955. doi: 10.1126/science.272.5270.1953
Selensky, M. J., Masterson, A. L., Blank, J. G., Lee, S. C., and Osburn, M. R. (2021). Stable carbon isotope depletions in lipid biomarkers suggest subsurface carbon fixation in lava caves. Journal of geophysical research. Biogeosciences 126:e2021JG006430. doi: 10.1029/2021JG006430
Stevens, T. O., and McKinley, J. P. (1995). Lithotrophic microbial ecosystems in deep basalt aquifers. Science 270, 450–455. doi: 10.1126/science.270.5235.450
Tsurnamal, M. (2008). A new species of the stygobiotic blind prawn Typhlocaris Calman, 1909 (Decapoda, Palaemonidae, Typhlocaridinae) from Israel. Crustaceana 81, 487–501. doi: 10.1163/156854008783797534
Ullman, M., Brailovsky, L., Schechter, H. C., Weissbrod, L., Zuckerman-Cooper, R., Toffolo, M. B., et al. (2022). The early pre-pottery Neolithic B site at Nesher-Ramla quarry, Israel. Quat. Int. 624, 148–167. doi: 10.1016/j.quaint.2021.04.019
Wagner, H. P. (2012). Tethysbaena ophelicola n. sp.(Thermosbaenacea), a new prime consumer in the Ophel biome of the Ayyalon cave, Israel. Crustaceana 85, 1571–1587. doi: 10.1163/156854012X651646
Weinberger, G., Rosenthal, E., Ben Zvi, A., and Zeitoun, D. G. (1994). The Yarkon-Taninim groundwater basin, Israel. Hydrogeology, case study and critical review. J. Hydrol. 161, 227–255. doi: 10.1016/0022-1694(94)90130-9
Keywords: sulfidic cave, arthropod adaptation, radiocarbon, stable isotopes, environmental isotopes, chemolithoautotrophy, cave ecosystem, biofilm
Citation: Frumkin A, Chipman AD and Naaman I (2023) An isolated chemolithoautotrophic ecosystem deduced from environmental isotopes: Ayyalon cave (Israel). Front. Ecol. Evol. 10:1040385. doi: 10.3389/fevo.2022.1040385
Edited by:
Enrico Lunghi, University of L'Aquila, ItalyReviewed by:
Benjamin Hutchins, Texas State University, United StatesMaarten van Hardenbroek, Newcastle University, United Kingdom
Copyright © 2023 Frumkin, Chipman and Naaman. This is an open-access article distributed under the terms of the Creative Commons Attribution License (CC BY). The use, distribution or reproduction in other forums is permitted, provided the original author(s) and the copyright owner(s) are credited and that the original publication in this journal is cited, in accordance with accepted academic practice. No use, distribution or reproduction is permitted which does not comply with these terms.
*Correspondence: Amos Frumkin, ✉ YW1vcy5mcnVta2luQG1haWwuaHVqaS5hYy5pbA==
†These authors have contributed equally to this work and share first authorship