- 1U.S. Geological Survey, Western Ecological Research Center, Dixon Field Station, Dixon, CA, United States
- 2U.S. Geological Survey, Western Ecological Research Center, Point Reyes Field Station, Point Reyes Station, CA, United States
- 3U.S. Geological Survey, Western Ecological Research Center, Santa Cruz Field Station, Santa Cruz, CA, United States
Climate can have a strong influence on species distributions, and amphibians with different life histories might be affected by annual variability in precipitation in different ways. The Sierra Nevada of California, United States, experienced some of the driest and wettest years on record in the early 21st Century, with variability in annual precipitation predicted to increase with climate change. We examined the relationship between adult occupancy dynamics of three high elevation anurans and site and annual variation in measures of winter severity, summer wetness, and cumulative drought. We further evaluated how these weather conditions affected the probability that each species would reproduce, conditional on their occurrence at a site. We found that although different aspects of weather affected the occupancy dynamics of each species differently, adult occupancy probabilities were generally stable throughout our 15-year study period. The probability of reproduction, although slightly more variable than adult occupancy, was similarly stable throughout the study. Although occurrence of the three species was resilient to recent extremes in precipitation, more detailed demographic study would inform the extent to which amphibian populations will remain resilient to increasing severity, duration, and frequency of drought and flood cycles.
1. Introduction
Climate is one of the most important drivers of species distributions. The influence of climate can take many forms, ranging from obvious effects like climatic extremes that exceed the physiological tolerances of species (Evans et al., 2015) to subtle influences of climate on interspecific interactions (Dallalio et al., 2017). The current velocity of anthropogenic climate change, however, likely exceeds the pace at which species can adapt to new or more variable climates (Menéndez et al., 2006; Merkle et al., 2022). One aspect of global anthropogenic change that can be particularly important in limiting species distributions is climate variability.
Climate change is expected to influence the climate in different ways in different places. In California, United States, one of the major anticipated effects of climate change is to increase annual variability in precipitation, despite small changes to mean annual precipitation (Swain et al., 2018). Already in the early 21st Century, the Sierra Nevada has experienced some of the wettest and driest years on record, and the southwestern United States is currently in the midst of a multi-year “megadrought” (Williams et al., 2022). The predicted increase in the frequency and severity of droughts and floods (Huang and Swain, 2022) will likely have a large influence on many taxa, especially aquatic organisms or those that require surface waters for reproduction, like amphibians.
The anuran (frogs and toads) fauna of the high elevations of the Sierra Nevada, California, United States, is dominated by three species that differ substantially in life history strategies. The Sierra Nevada yellow-legged frog (Rana sierrae) historically inhabited naturally fishless, high elevation, permanent waterbodies. Long-lived adults of the species are semiaquatic, and larvae usually overwinter two or more times before undergoing metamorphosis (Fellers et al., 2013). Population declines following the historical introduction of trout (Knapp and Matthews, 2000; Knapp, 2005) and later lethal chytridiomycosis caused by the fungal pathogen Batrachochytrium dendrobatidis (Bd) caused extensive population declines and extirpations of the species (Briggs et al., 2005; Vredenburg et al., 2010), precipitating the listing of the frogs as endangered under the U.S. Endangered Species Act (ESA; U.S. Fish and Wildlife Service, 2014).
Population declines, potentially caused by chytridiomycosis (Lindauer and Voyles, 2019), also led to the listing of Yosemite toads (Anaxyrus canorus) as threatened under the ESA (U.S. Fish and Wildlife Service, 2014). Like Sierra Nevada yellow-legged frogs, Yosemite toads have a long-lived adult stage, but Yosemite toad adults are predominantly terrestrial (Morton and Pereyra, 2010; Liang, 2013). Adults breed at snowmelt in the shallow margins of wetlands, usually in meadows, and eggs and larvae develop quickly and metamorphose into juvenile toads in a few months (Mullaly, 1953; Kagarise Sherman and Morton, 1993). The reliance on shallow wetlands likely increases the chances of annual reproductive failure in this species (Kagarise Sherman and Morton, 1993; Sadinski et al., 2020). This toad, however, is less sensitive to introduced fish than Sierra Nevada yellow-legged frogs because Yosemite toad larvae are unpalatable to trout, the dominant introduced fish in the Sierra Nevada (Grasso et al., 2010).
In contrast to Sierra Nevada yellow-legged frogs and Yosemite toads, sierran treefrogs (Pseudacris sierra) are widespread in California and have a shorter adult lifespan (Ethier et al., 2021). Sierran treefrogs breed in a diversity of wetlands and, like Yosemite toads, typically undergo metamorphosis in a single season (Ethier et al., 2021). Like Sierra Nevada yellow-legged frogs, however, sierran treefrogs are palatable to fish (Grasso et al., 2010) and their occurrence is negatively related to the presence of fish (Matthews et al., 2001; Knapp, 2005).
These three anurans have different life history strategies, but all are adapted to the strongly seasonal environments of high elevations in the Sierra Nevada, and all are dependent on aquatic breeding sites. We sought to examine how recent extremes in annual precipitation affected adult occupancy dynamics and probability of reproduction of these anurans. Specifically, we estimated the influence of three climatic variables related to different aspects of water availability: winter severity (Snow Water Equivalent [SWE] in April), drought (minimum Palmer Drought Severity Index [PDSI] in September), and active season water balance (3-month Standardized Precipitation and Evapotranspiration Index [SPEI] in September) on probabilities of colonization, extirpation, and reproduction of each species. We relate our results to the life history strategy of each species and discuss how life history variation affects susceptibility and resilience to weather variability.
2. Materials and methods
2.1. Study area
We studied amphibian occupancy dynamics in Yosemite National Park (hereafter, Yosemite), California, from 2007 to 2021 (Figure 1). Yosemite is located in the central Sierra Nevada at elevations ranging from 648 to 3,997 m and is drained by two major rivers: the Merced River in the southern portion of the park and the Tuolumne River in the northern portion of the park. At high elevations, dominant trees are lodgepole pine (Pinus contorta), red fir (Abies magnifica), and white fir (Abies concolor). Lower elevations include additional Pinus spp., and meadows and riparian areas contain willows (Salix jepsoni), corn lily (Veratrum californicum), small camas (Camassia quamash), broad-leaf lupine (Lupinus latifolius), sedges (Cyperaceae), and grasses (Poaceae). Most precipitation at higher elevations falls as snow in the winter and early spring.
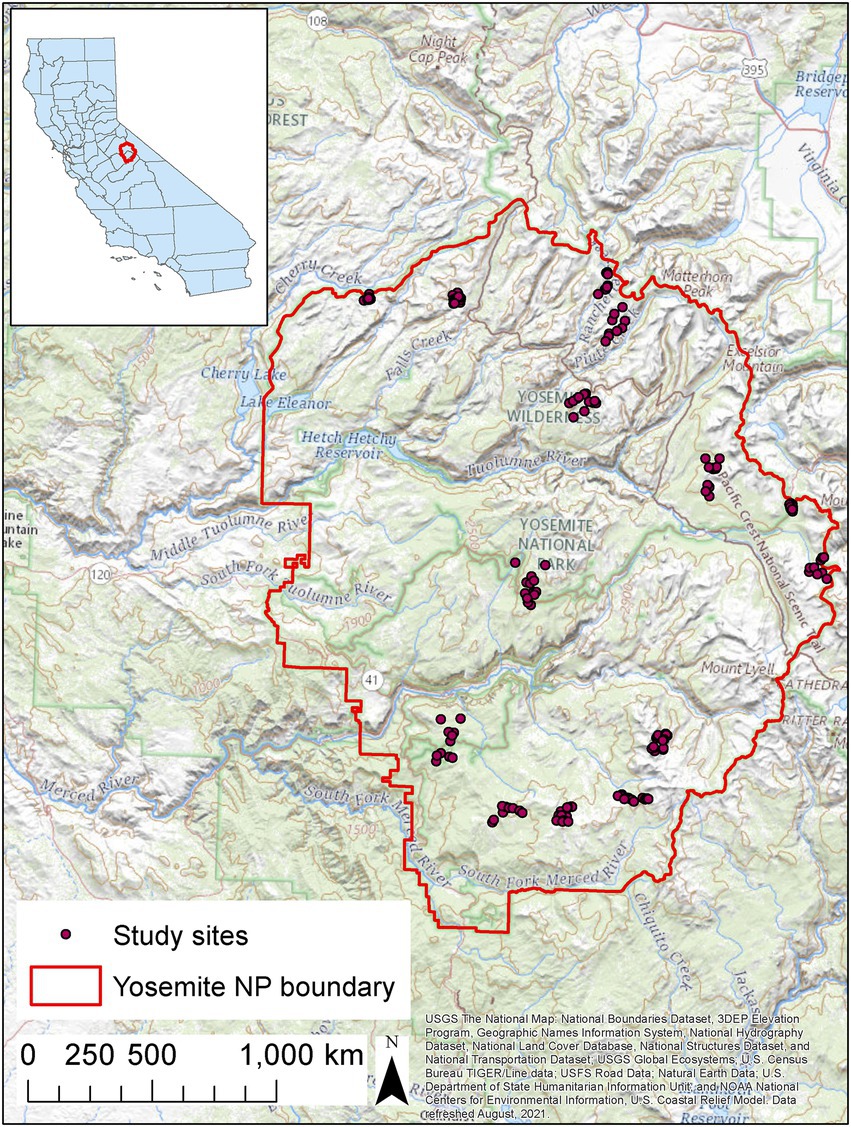
Figure 1. Locations of sites surveyed for amphibians in Yosemite National Park, California, United States, 2007–2021. All potential breeding sites in each of 14 watershed units were surveyed.
We divided Yosemite into 220 watershed units, each with 10–25 potential breeding sites, and randomly selected six watersheds from the Merced River basin and eight watersheds from the Tuolumne River basin (Figure 1; Fellers et al., 2015). The boundaries between our watershed units usually followed natural features and drainages, but they did not strictly conform to Hydrologic Unit Code (HUC) units mapped in the U.S. Geological Survey Watershed Boundary Dataset because of the great variation in the number of sites among mapped HUCs.
2.2. Field methods
We surveyed all potential breeding sites in each selected watershed unit using independent double observer Visual Encounter Surveys (VES; Crump and Scott, 1994; Fellers et al., 2015). Each site was independently surveyed by two biologists with surveys timed to start 15–60 min apart to help ensure independence between biologists while allowing time for disturbed amphibians to resume normal activity and be available for detection. To conduct surveys, each biologist walked slowly around the perimeter of ponds, lakes, and streams, or through meadows, while searching for eggs, larvae, subadults, and adults of each amphibian species. Biologists used a dip net to flush hiding amphibians and to capture larvae for positive identification when necessary. Each biologist maintained an independent count of the number of each life stage for each species at each site. In addition to counts of each life stage of each species, we recorded biologist identity, air and water temperature, maximum water depth, and site dimensions (length and width). The datasets generated for this study can be found on ScienceBase (Kleeman et al., 2022; doi: 10.5066/P9X6SKT8).
2.3. Analytical methods
We used multi-state dynamic occupancy models (Duarte et al., 2020; Rose et al., 2022) to estimate amphibian occurrence and breeding dynamics in Yosemite. These models allow for transitions between different occurrence states (i.e., unoccupied, occupied by adults only, and occupied by adults and pre-metamorphic life stages [eggs or larvae]) while accounting for imperfect state observations. Our model included three possible occurrence states for sites: (1) unoccupied by amphibians; (2) occupied by juvenile or adult amphibians only; and (3) occupied by adults and pre-metamorphic life stages indicative of breeding (whether or not adults were observed; i.e., breeding was conditional on adult occurrence). Our observation states matched the occurrence states, but our model allowed mis-classification without false positive detections. Specifically, we assumed that amphibians of any life stage could be missed during surveys. In other words, a site with only adults present (state 2) could be correctly classified with adults observed or misclassified as no amphibians observed, and a site with pre-metamorphic life stages (state 3) could be correctly classified with eggs or larvae observed or misclassified with only adults observed or with no amphibians observed.
In addition to these site-specific states, we also included additional hierarchical structure into the model to account for our clustering of sites within watershed units. We did this in two different ways, depending on the species. For widespread sierran treefrogs, which were observed in all sampled watersheds each year, we allowed mean probability of occurrence, extirpation probability, and colonization probability to vary among watersheds as a logit-normal random intercept for watershed. For Sierra Nevada yellow-legged frogs and Yosemite toads, which did not occur in all watersheds during all years, we added an additional level to the model to allow for occurrence dynamics at the watershed scale, and conditioned site occupancy on occurrence within the watershed using a multi-scale occupancy model. The multi-scale multi-state dynamic occupancy model was parameterized in terms of an initial occupancy state, extirpation probabilities, and colonization probabilities as follows.
For watershed-scale occupancy dynamics, we modeled the initial occurrence state in watershed i as
where ψ is the watershed-scale probability of occurrence and zi,1 is an indicator variable for presence (1) or absence (0) of the species in watershed i at the beginning of the study. For watershed-scale occupancy in subsequent years, we modeled occupancy dynamics as
where γWS is the probability an unoccupied watershed is colonized by the species, ϕ is the probability an occupied watershed remains occupied, μz_i,t is the probability watershed i is occupied in year t, given its occurrence status in year t – 1, and zi,t is an indicator for presence (1) or absence (0) of the species in watershed i in year t. We then modeled the initial occurrence state at site j, conditional on occurrence in the watershed in which site j was found, as
where initial_state defines a vector of multinomial probabilities for each site j, with states defined as 1 = unoccupied, 2 = occupied by adults only, and 3 = occupied by adults and pre-metamorphic life stages (i.e., used for breeding); θ is the probability the site is occupied by adult amphibians; zi,1 is the indicator variable for whether the watershed in which the site is found is occupied by the species; rj,1 is the conditional (on adult occurrence) probability that breeding occurred at the site in the initial year of the study; occ_mtxj,1 is a matrix of the true occupancy state of each site j in year 1; and categorical represents a categorical distribution. θ and r were further modeled as logit-linear functions of covariates using binomial regression (Table 1). Variables included in the model were based on knowledge of the ecology of the species and prior research (Knapp, 2005; Fellers et al., 2015; Halstead et al., 2021). Occurrence dynamics at the site scale were then modeled, conditional on watershed occurrence as for initial occupancy, with the transition matrix
where γ is the probability unoccupied site j is colonized by adults in year t; ε is the probability adults are extirpated from occupied site j in year t; r is the probability reproduction occurs at site j (conditional on adult occurrence at site j in year t); zi,t are indicator variables that do not allow sites in watersheds unoccupied in year t to be colonized that year and force occupied sites to become extirpated if the watershed in which they occur is not occupied the following year; and occ_mtxj,t is a matrix of the true occupancy state of each site j in year t. The transition matrix for sierran treefrogs, for which we did not use a multi-scale model but instead allowed mean probability of occurrence at sites to vary across watersheds, was identical except that all zi,t were fixed at 1. All probabilities (γ, ε, and r) were further modeled as logit-linear functions of covariates using binomial regression, with variables included in the model tailored to the ecology of each species (Table 1).
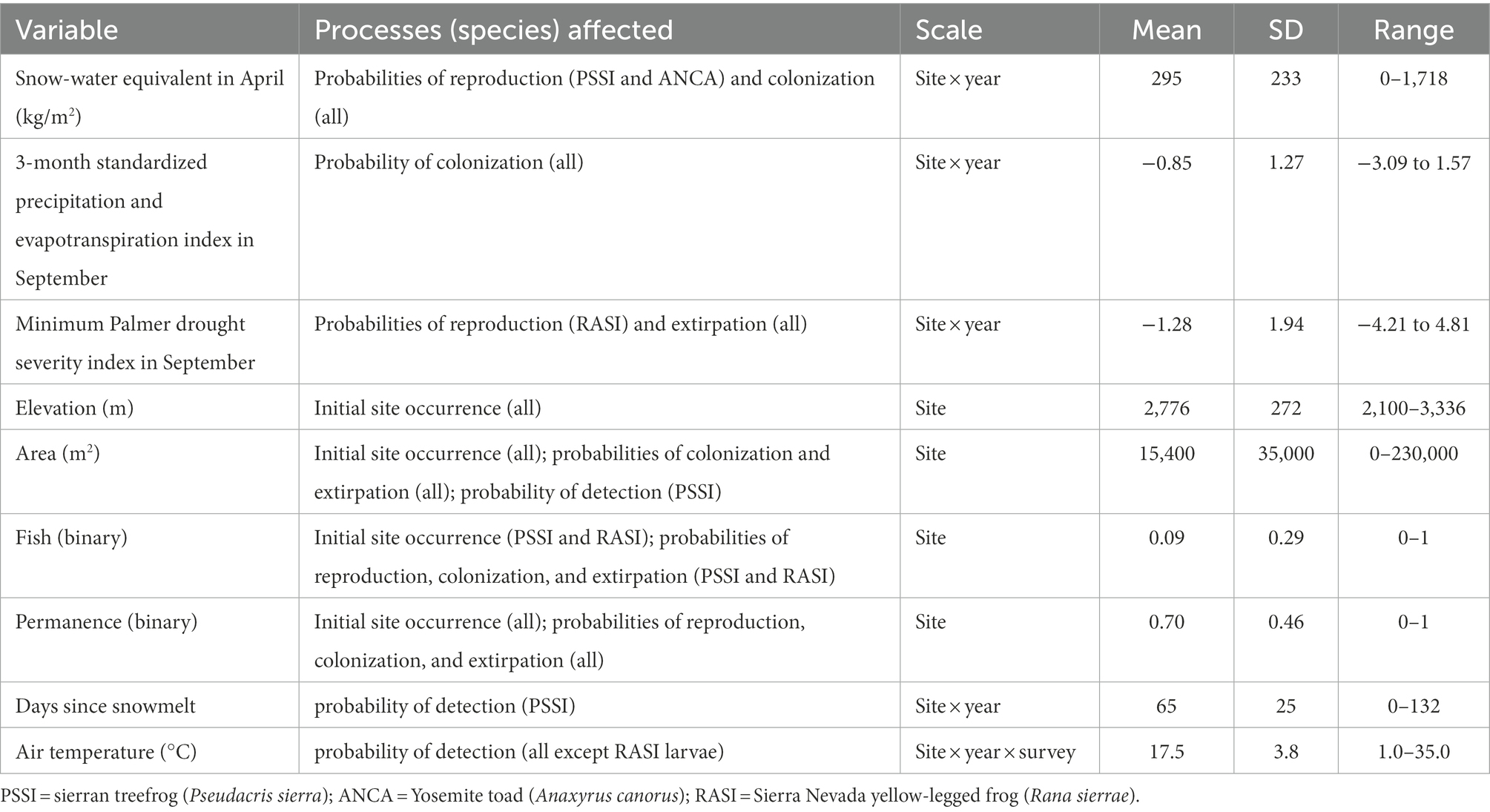
Table 1. Processes affected by and summaries of predictor variables included in the multi-scale multi-state models of amphibian occurrence and reproduction.
Conditional on the occurrence state of the site, the observation matrix was defined as
where p is the probability of detecting each life stage, adult (A) or pre-metamorphic (L), at site j in year t and survey k and obs_arrayj,t,k is the observed state at site j in year t and survey k. Note that we assumed that no false positives occurred: adults could not be detected if they were not present, nor could pre-metamorphic life stages be detected if breeding did not occur at the site. We allowed p to vary with site, year, and survey-specific variables based on prior research for each species (Fellers et al., 2015) using binomial regression with a logit link function (Table 1).
We selected variables to influence each parameter based on prior research in Yosemite (Table 1; Knapp, 2005; Fellers et al., 2015; Halstead et al., 2021) plus the three variables related to water availability above. In addition to variables recorded in the field, we used U.S. Geological Survey topographic maps to assign wetland hydroperiods, and we determined presence of fish based on gill net surveys (Fellers et al., 2015; Knapp et al., 2020). Date of snowmelt (earliest date at which all snow has melted) was estimated using a snow sensor at Gin Flat (2,070 m elevation near the western boundary of Yosemite between the Merced and Tuolumne rivers), and the number of days since snowmelt used as a potential variable affecting amphibian detection (Fellers et al., 2015). We used snow-water equivalent (SWE) in April, the month at which SWE typically reaches its maximum, from Daymet as a measure of the severity of the preceding winter and the amount of water available in snowpack (Thornton et al., 2020). To assess short-term effects of precipitation and evapotranspiration on occupancy dynamics and reproduction, we used the 3-month standardized precipitation and evapotranspiration index (SPEI03; Vose et al., 2014) in September, which integrated precipitation and evapotranspiration during the driest part of the year (June–August), when amphibians in this system are most active. We used the minimum Palmer Drought Severity Index (PDSI) in September to account for cumulative water deficits integrated across years as measured at the driest time of year (Vose et al., 2014). Each of these water availability variables was extracted for each site and year of the study.
We analyzed the model for each species using the package “NIMBLE” (de Valpine et al., 2022) in R 4.1.3 (R Core Team, 2022). Priors for each parameter in the model were selected to be uninformative but weakly regularizing: beta(α = 1, β = 1) for all probabilities, exponential(λ = 1) for all standard deviations, and Gaussian(mean = 0, SD = 1) for all logit-linear coefficients. We ran each model on 5 chains of 100,000 iterations each after a burn-in phase of 10,000 iterations and thinned each chain by a factor of 5, basing inference on 100,000 samples from the posterior distribution. The minimum effective sample size (the estimated number of statistically independent samples from the Markov chain Monte Carlo output after accounting for serial autocorrelation) across all parameters and species was 639 (Tables 2–4). We assessed convergence with the statistic (Gelman and Rubin, 1992) and examination of history plots. All plots appeared stationary and well-mixed, and the maximum across all monitored parameters was 1.02. Model code to reproduce analyses is available on GitLab (Halstead et al., 2022; doi: 10.5066/P9VR4SH3). Unless otherwise indicated, we summarize posterior distributions as median (95% equal-tailed interval).
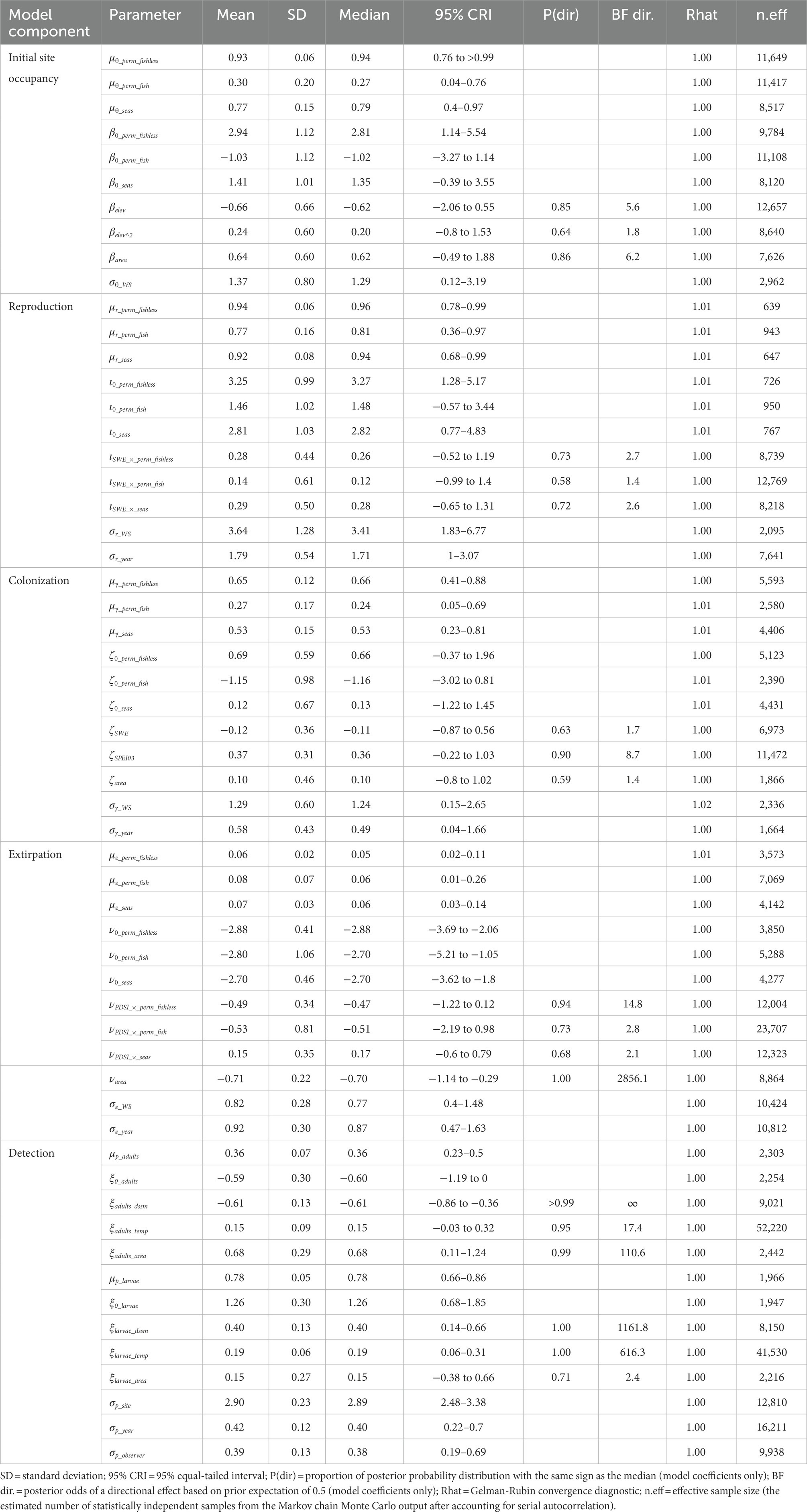
Table 2. Posterior summaries and Markov chain Monte Carlo (MCMC) diagnostics of parameters for the multi-state dynamic occupancy model for sierran treefrogs (Pseudacris sierra) in Yosemite National Park, California, United States, 2007–2021.
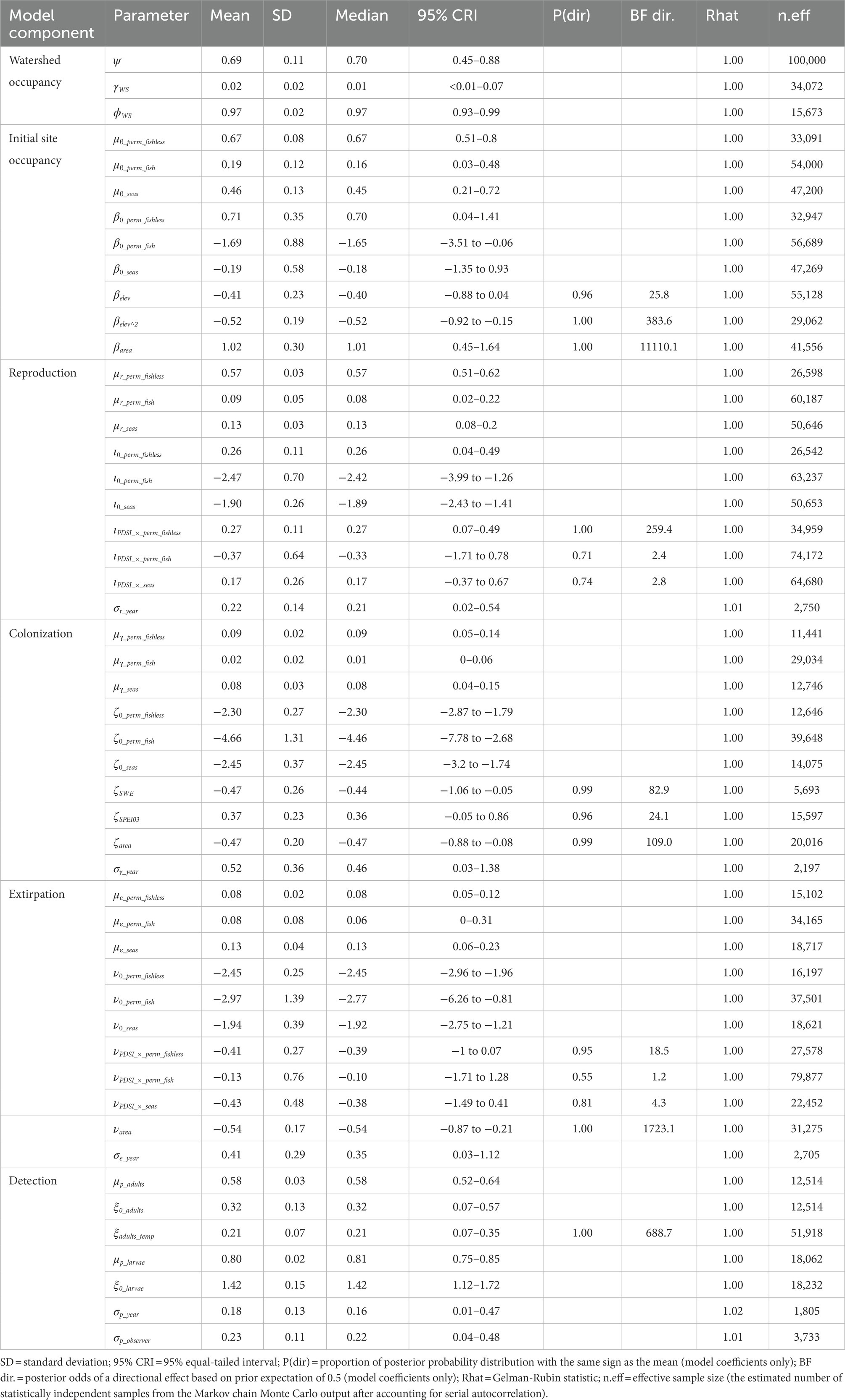
Table 3. Posterior summaries and Markov chain Monte Carlo (MCMC) diagnostics of parameters for the multi-state dynamic occupancy model for Sierra Nevada yellow-legged frogs (Rana sierrae) in Yosemite National Park, California, United States, 2007–2021.
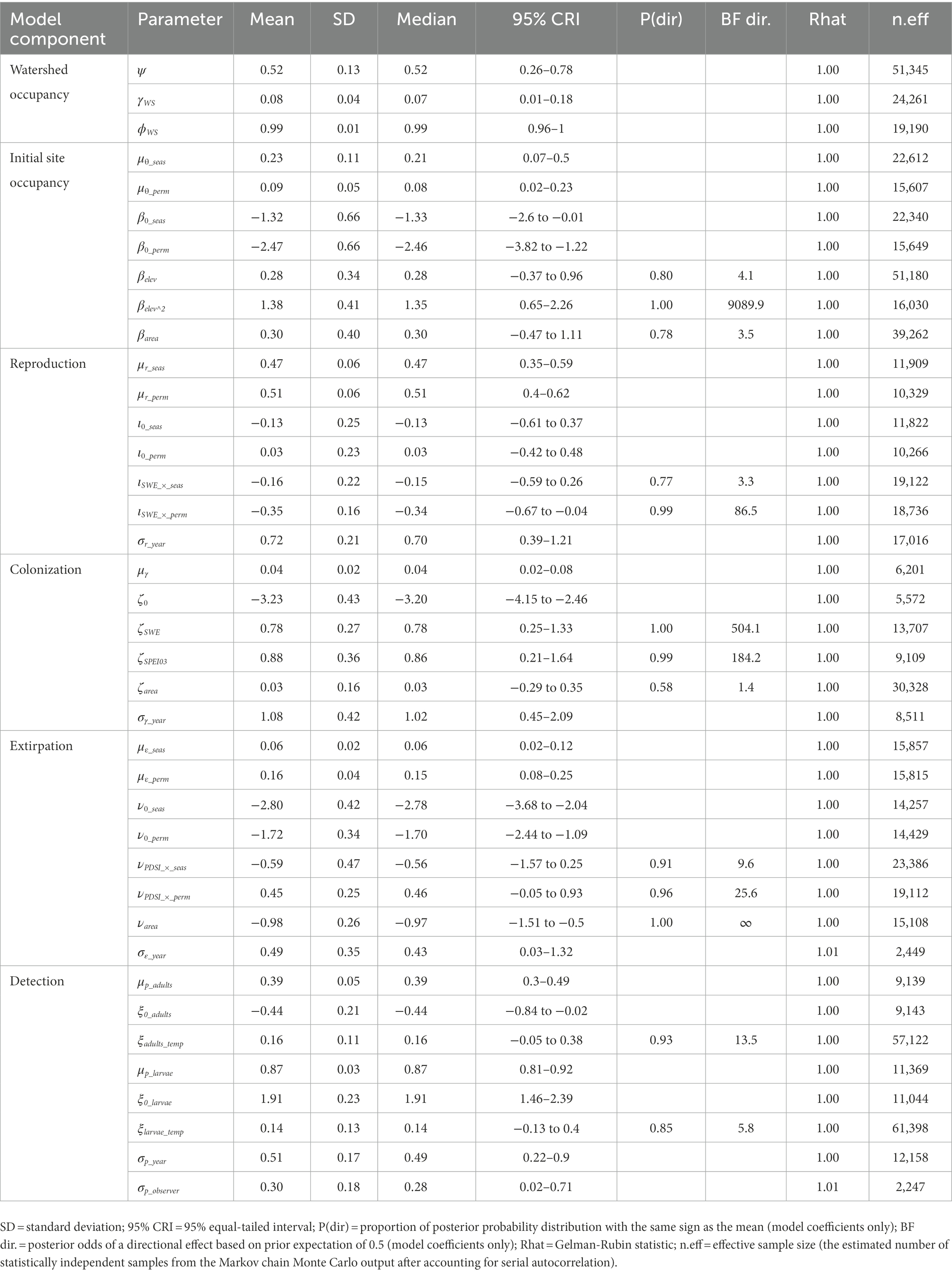
Table 4. Posterior summaries and Markov chain Monte Carlo (MCMC) diagnostics of parameters for the multi-state dynamic occupancy model for Yosemite toads (Anaxyrus canorus) in Yosemite National Park, California, United States, 2007–2021.
3. Results
Across 6,169 surveys of 174 sites in 14 watersheds and 15 years, we detected sierran treefrog adults 290 times and evidence of reproduction (eggs or larvae) 2,546 times. For Sierra Nevada yellow-legged frogs, we detected adults 637 times and evidence of reproduction 715 times. We had the fewest detections of Yosemite toads, with adults detected 274 times and evidence of reproduction 622 times.
The three climatic variables related to water availability that we evaluated varied substantially over the study period, but exhibited different patterns (Figure 2; Table 1). Across sites, annual April SWE averaged 295 kg/m2 (SD = 197; range = 29–695), reaching its maximum value in 2010 and its minimum value in 2013 (Figure 2; Table 1). Annual September minimum PDSI averaged −1.28 (SD = 1.86; range = −3.44 to 3.57) reaching maximum and minimum values in 2011 and 2008, respectively (Figure 2; Table 1). Annual SPEI03 in September averaged −0.85 (SD = 1.22, range = −2.90 to 1.05), with maximum and minimum values observed in 2017 and 2008, respectively (Figure 2; Table 1).
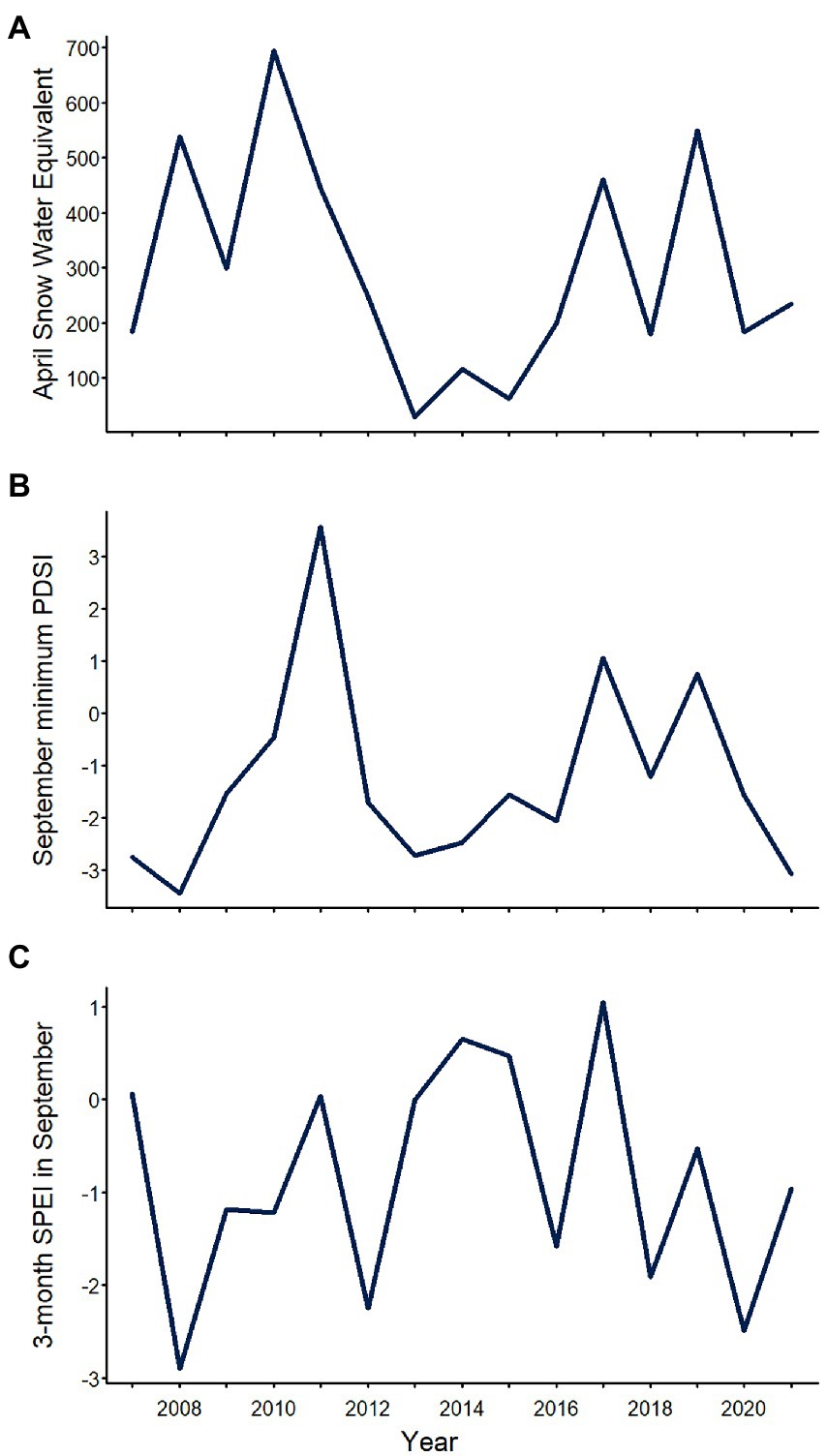
Figure 2. Mean annual (A) April snow water equivalent, (B) September minimum Palmer Drought Severity Index (PDSI; lower values = drier conditions), and (C) 3-month Standardized Precipitation Evapotranspiration Index (SPEI) in September in Yosemite National Park, California, United States, 2007–2021, averaged across surveyed sites.
Sierran treefrogs occurred in all watersheds in all years, but Sierra Nevada yellow-legged frogs and Yosemite toads occurred in only a subset of watersheds. In 2007, probability of occurrence of Sierra Nevada yellow-legged frogs in watersheds was median 0.70 (95% equal-tailed interval = 0.45–0.88). Colonization probability was low (0.02 [<0.01–0.07]) and watershed persistence probability was high (0.97 [0.93–0.99]); nonetheless, the probability of occurrence for Sierra Nevada yellow-legged frogs at the watershed scale decreased from 2007 to 2021 (Figure 3). Initial probability of occurrence for Yosemite toads (0.52 [0.26–0.78]) was lower than for Sierra Nevada yellow-legged frogs, but both colonization and watershed persistence probabilities were estimated to be higher (0.07 [0.01–0.18] and 0.99 [0.96–>0.99], respectively), resulting in an increase in the probability of occurrence of Yosemite toads at the watershed scale from 2007 to 2021 (Figure 3).
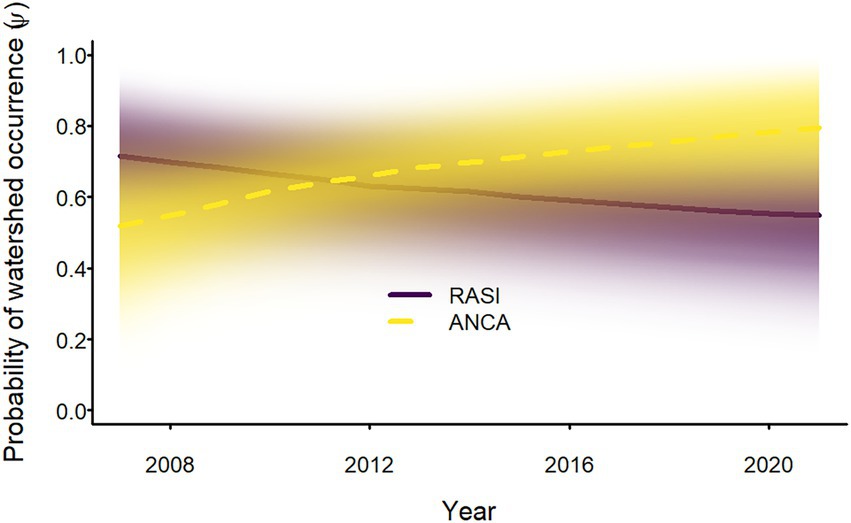
Figure 3. Annual probability of occurrence of adult Sierra Nevada yellow-legged frogs (Rana sierrae; RASI) and Yosemite toads (Anaxyrus canorus; ANCA) at the watershed scale in Yosemite National Park, California, United States, 2007–2021. Bold lines indicate posterior modes and the intensity of shading represents the posterior probability density.
Species varied in their probability of occurrence in 2007 and in their responses to elevation and area. The initial probability of occurrence of sierran treefrogs was generally much higher than for the other species (Tables 2–4; Figure 4). The initial probability of occurrence for both sierran treefrogs and Sierra Nevada yellow-legged frogs was highest in permanent fishless waterbodies, lower in seasonal wetlands, and lowest in permanent waterbodies with fish (Tables 2, 3; Figure 4). In contrast, the initial probability of occurrence for Yosemite toads was higher in seasonal wetlands than permanent waterbodies (Table 4; Figure 4). In sierran treefrogs, initial probability of occurrence was generally higher at lower elevations (Table 2; Figure 4). Relative to elevation, initial probability of occurrence for Sierra Nevada yellow-legged frogs peaked near 2,700 m elevation, whereas initial probability of occurrence for Yosemite toads was highest at the lowest and highest elevations sampled (Tables 3, 4; Figure 4). For all species and breeding site types, larger sites were more likely to be occupied initially than smaller sites (Tables 2–4; Figure 4).
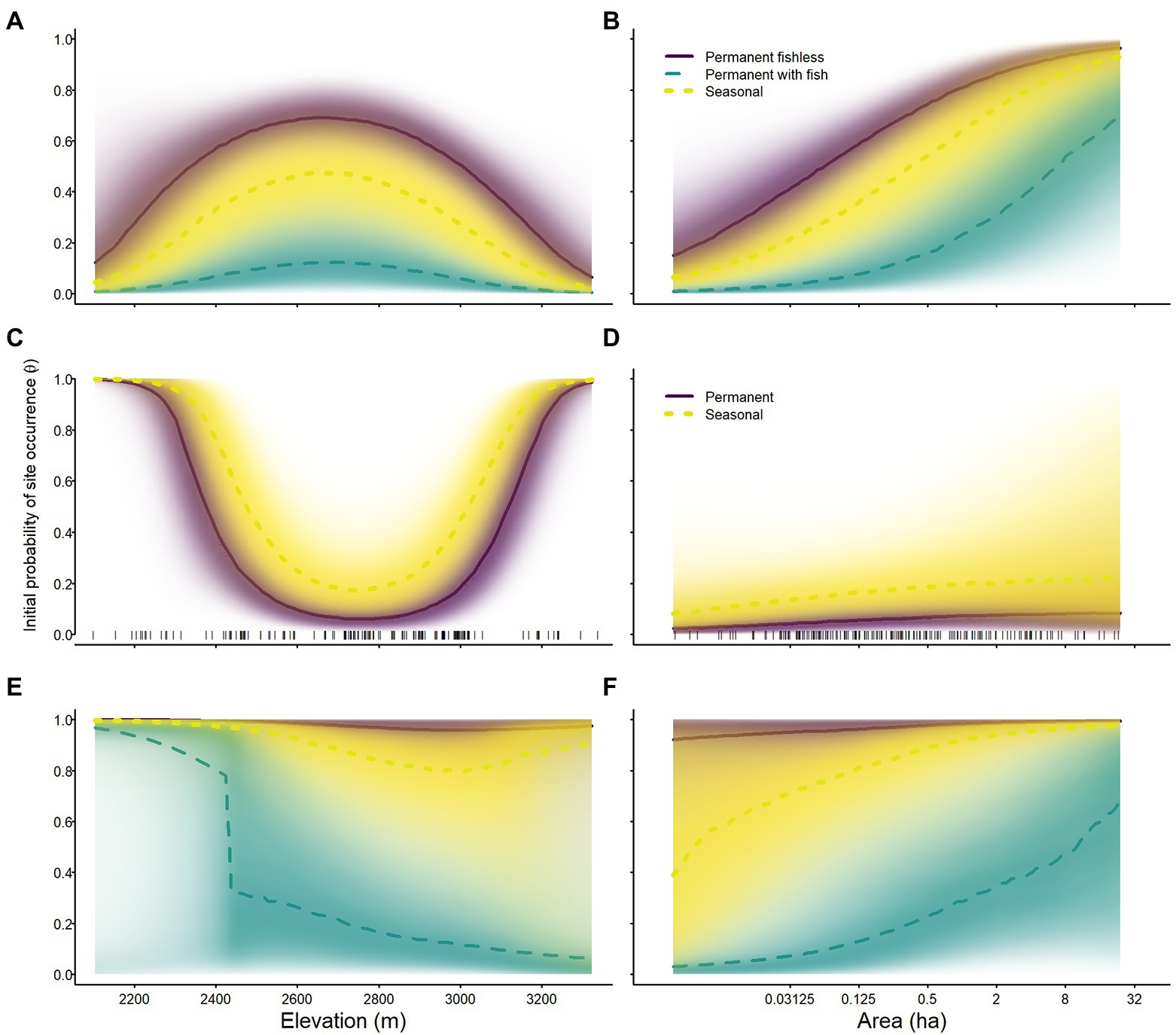
Figure 4. Initial probability of occurrence of Sierra Nevada yellow-legged frogs [Rana sierrae; (A,B)], Yosemite toads [Anaxyrus canorus; (C,D)], and sierran treefrogs [Pseudacris sierra; (E,F)] in relation to elevation (A,C,E), site area [(B,D,F); plotted on a log scale], and site type (indigo shading and solid line = permanent [and fishless for panels (A–D)]; aqua shading and dashed line = permanent with fish; gold shading and dotted line = seasonal) in Yosemite National Park, California, United States, 2007. Lines represent posterior modes and shading intensity represents the posterior probability distribution.
The probability of colonization varied across species, and was lowest for sierran treefrogs and Sierra Nevada yellow-legged frogs in permanent waterbodies with fish (Tables 2–4; Figure 5). Probability of colonization for all species was positively related to 3-month SPEI in September (Tables 2–4; Figure 5). April snow-water equivalent had a negative effect on colonization probability for Sierra Nevada yellow-legged frogs (Table 3; Figure 5), but a positive effect on colonization probability for Yosemite toads (Table 4; Figure 5). Colonization probabilities were higher in waterbodies without fish than waterbodies with fish, regardless of seasonality, for sierran treefrogs and Sierra Nevada yellow-legged frogs (Tables 2, 3; Figure 5). In addition to weather variables, site colonization probability for Sierra Nevada yellow-legged frogs was negatively related to site area (Table 3).
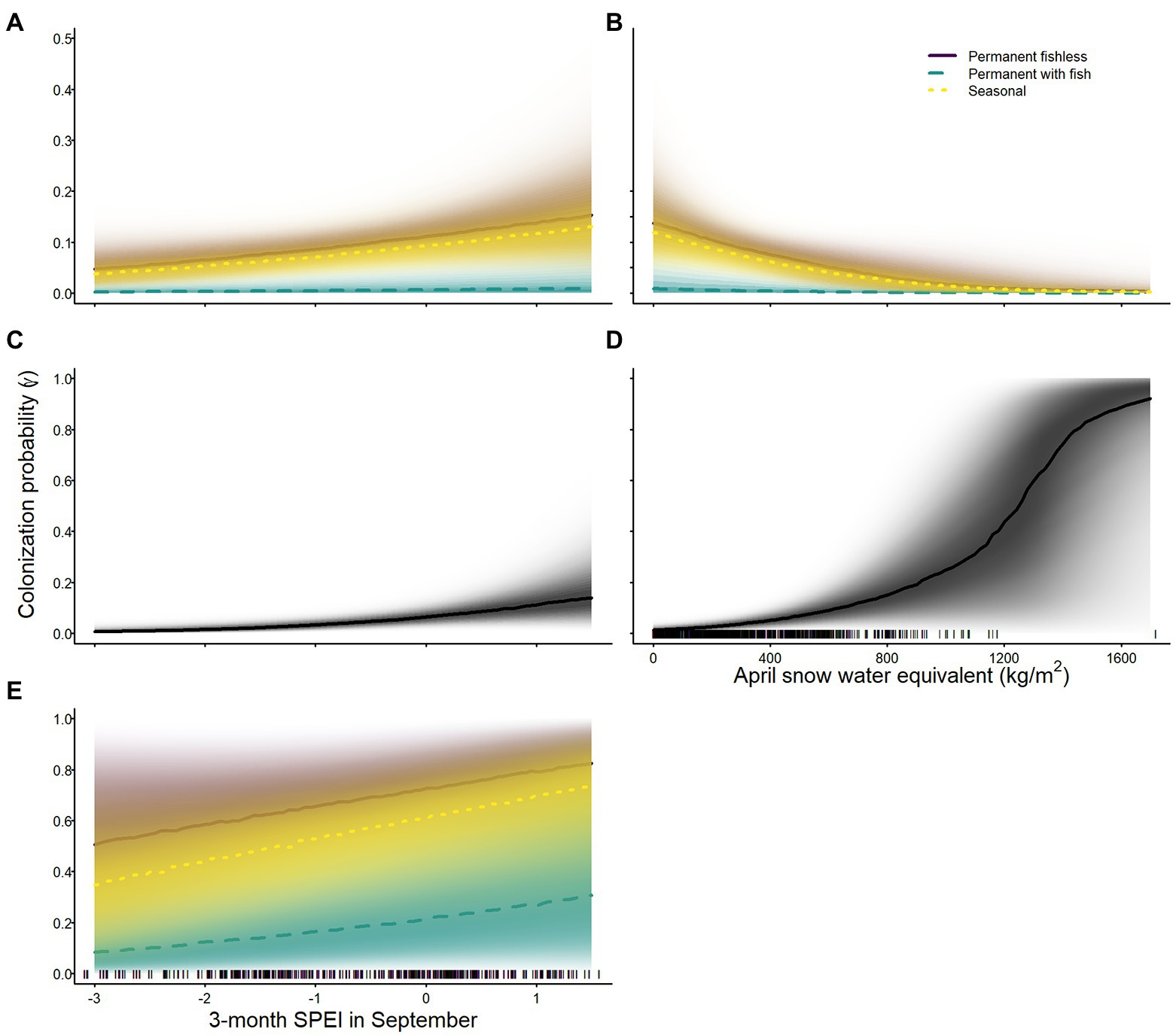
Figure 5. Probability of colonization for Sierra Nevada yellow-legged frogs [Rana sierrae; (A,B)], Yosemite toads [Anaxyrus canorus; (C,D)], and sierran treefrogs [Pseudacris sierra; (E)] in relation to 3-month Standardized Precipitation and Evapotranspiration Index (SPEI) in September (A,C,E), April snow water equivalent (B,D), and site type (indigo shading and solid line = permanent without fish; aqua shading and dashed line = permanent with fish; gold shading and dotted line = seasonal; black solid lines and shading = no distinction among site types) in Yosemite National Park, California, United States, 2007–2021. Lines represent posterior modes and shading intensity represents the posterior probability distribution.
Extirpation probabilities of all species were affected by minimum September PDSI, but the direction of effects and their magnitude varied across species and breeding site types (Tables 2–4; Figure 6). Extirpation probability for Sierra Nevada yellow-legged frogs was negatively related to minimum September PDSI (i.e., a lower extirpation probability when conditions were wetter in September) in all breeding site types, but evidence for an effect of PDSI on permanent sites with fish was weaker than for other breeding site types (Table 3; Figure 6). Minimum September PDSI had a negative effect on extirpation of Yosemite toads in seasonal wetlands, but a positive effect in permanent waterbodies (Table 4; Figure 6). Evidence of an effect of PDSI on the probability of extirpation for sierran treefrogs was substantial only for permanent fishless breeding sites, for which it had a negative effect (Table 2; Figure 6). Site area was negatively related to extirpation probability for all three species (Tables 2–4).
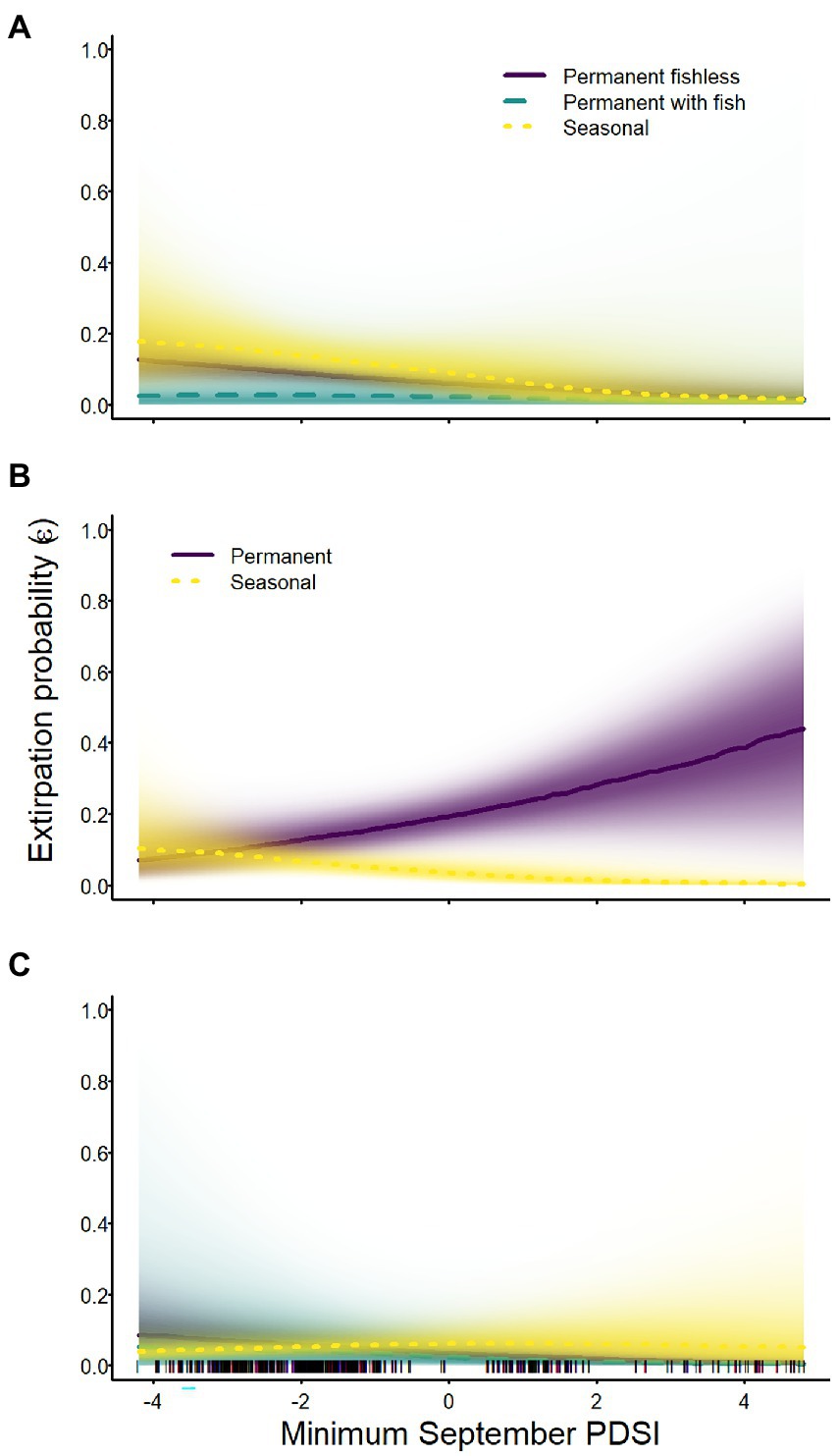
Figure 6. Probability of extirpation for Sierra Nevada yellow-legged frogs [Rana sierrae; (A)], Yosemite toads [Anaxyrus canorus; (B)], and sierran treefrogs [Pseudacris sierra; (C)] in relation to the minimum Palmer Drought Severity Index (PDSI) in September and site type (indigo shading and solid line = permanent [and fishless for panels (A,C)]; aqua shading and dashed line = permanent with fish; gold shading and dotted line = seasonal) in Yosemite National Park, California, United States, 2007–2021. Lines represent posterior modes and shading intensity represents the posterior probability distribution.
Although weather affected the probability of reproduction for Sierra Nevada yellow-legged frogs and Yosemite toads, evidence for an effect of weather variables on sierran treefrogs was weak (Tables 2–4; Figure 7). Probability of reproduction for Sierra Nevada yellow-legged frogs was positively related to minimum September PDSI in occupied permanent fishless waterbodies, but evidence for an effect of weather on reproduction in other breeding site types was weak (Table 3; Figure 7). Probability of reproduction for Yosemite toads was negatively related to April SWE regardless of whether wetlands were permanent or seasonal, but the effect was stronger in permanent waterbodies (Table 4; Figure 7). Probability of reproduction for sierran treefrogs was highest in permanent fishless waterbodies and lowest in permanent waterbodies with fish (Table 2; Figure 7). The effects of weather, breeding site type, and random annual variation resulted in differing patterns of annual probabilities of reproduction at occupied sites across species (Figure 8).

Figure 7. Probability of reproduction for Sierra Nevada yellow-legged frogs [Rana sierrae; (A)], Yosemite toads [Anaxyrus canorus; (B)], and sierran treefrogs [Pseudacris sierra; (C)] in relation to the minimum Palmer Drought Severity Index (PDSI) in September (A), April snow water equivalent [SWE; (B)], and site type (indigo shading and solid line = permanent [and fishless for panels (A,C)]; aqua shading and dashed line = permanent with fish; gold shading and dotted line = seasonal) in Yosemite National Park, California, United States, 2007–2021. Lines represent posterior modes and shading intensity represents the posterior probability distribution. In (C), white circles represent posterior modes, vertical lines represent 95% highest posterior density intervals, and the intensity of shading represents posterior probability densities.
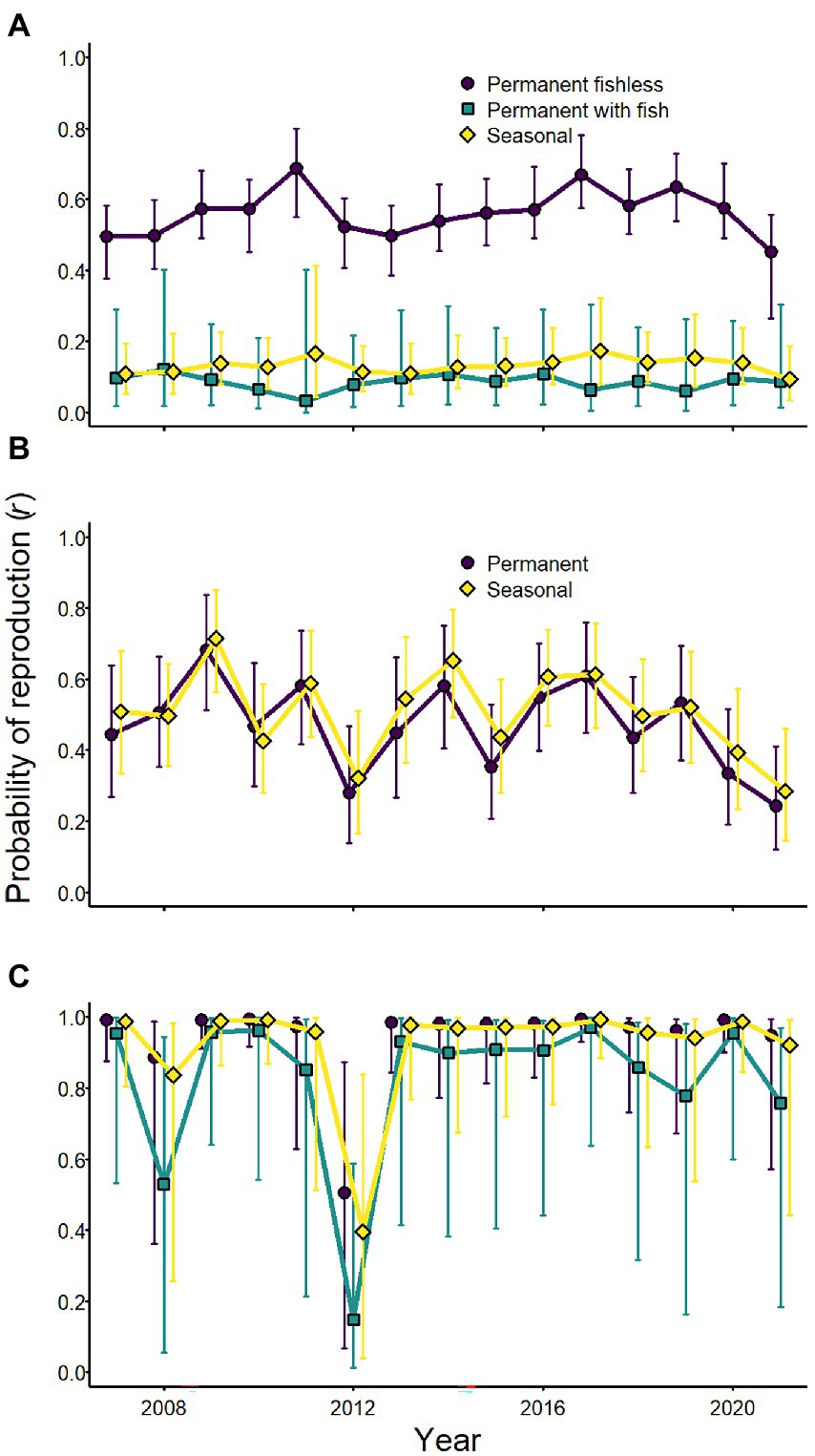
Figure 8. Annual probabilities of reproduction (conditional on adult occurrence) for Sierra Nevada yellow-legged frogs [Rana sierrae; (A)], Yosemite toads [Anaxyrus canorus; (B)], and sierran treefrogs [Pseudacris sierra; (C)] by site type (indigo circles and line = permanent [and fishless for panels (A,C)]; aqua squares and line = permanent with fish; gold diamonds and line = seasonal) based on modeled relationships with climate variables and random annual variation in Yosemite National Park, California, United States, 2007–2021. Points represent posterior medians; error bars represent 95% equal-tailed intervals.
Annual probability of site occurrence for adults of all species was relatively stable, but varied by breeding site type (Figure 9). For Sierra Nevada yellow-legged frogs and sierran treefrogs, probability of occurrence was consistently higher in permanent fishless and seasonal wetlands than in permanent waterbodies with fish (Figure 9). Adult Yosemite toads had moderately higher probability of occurrence in seasonal than permanent sites (Figure 9). Site occupancy growth rates were generally near 1 and turnover rates were generally low except for Yosemite toads between 2007 and 2008 and sierran treefrogs in permanent fishless sites in the same interval (Figure 9), when both species colonized a number of previously unoccupied sites.
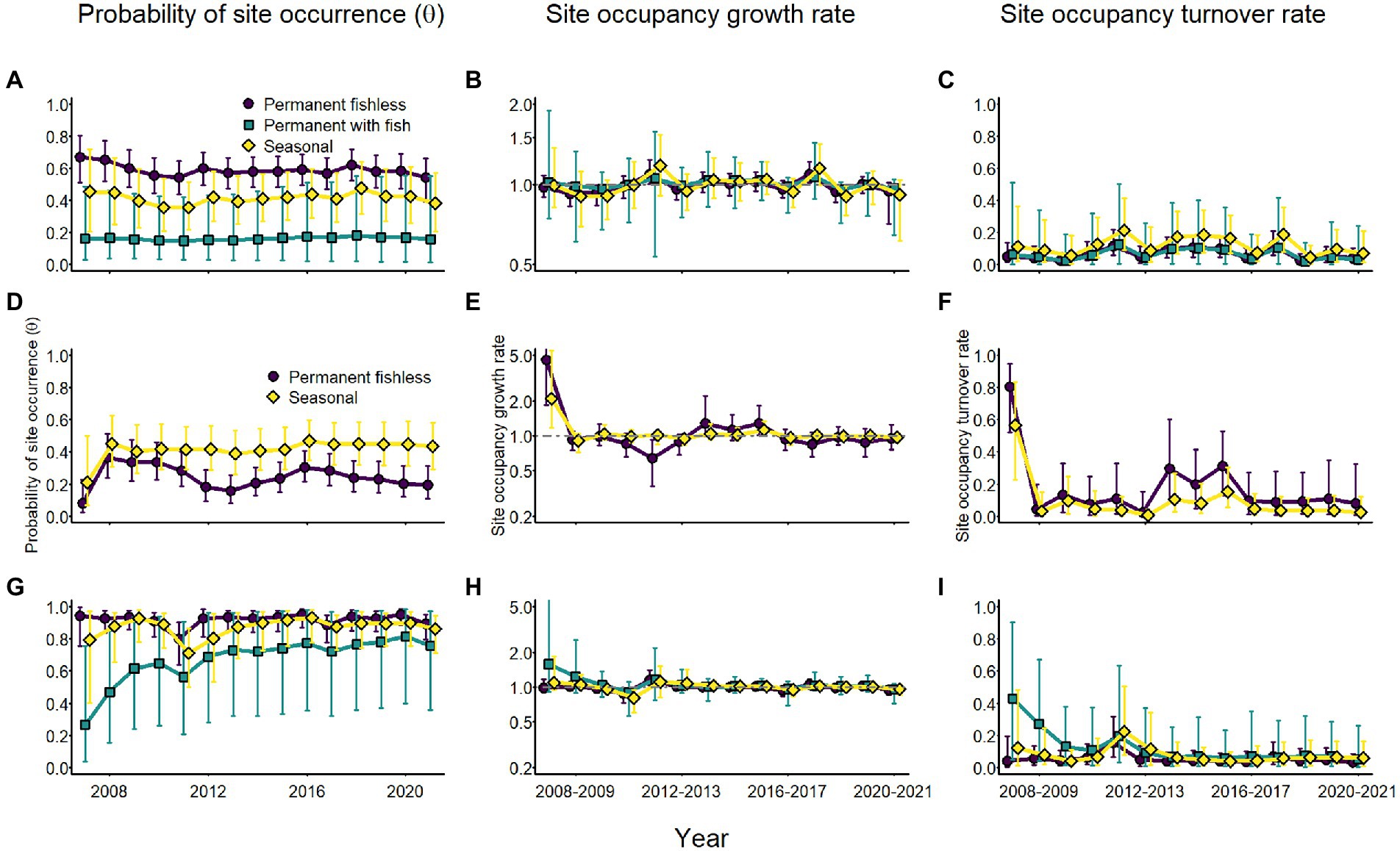
Figure 9. Annual estimates of site occupancy probability (A,D,G), site occupancy growth rate (B,E,H), and site occupancy turnover rate (C,F,I) for Sierra Nevada yellow-legged frogs [Rana sierrae; (A–C)], Yosemite toads [Anaxyrus canorus; (D–F)], and sierran treefrogs [Pseudacris sierra; (G–I)] by site type (indigo circles and line = permanent [and fishless for panels (A–C) and (G–I)]; aqua squares and line = permanent with fish; gold diamonds and line = seasonal) based on modeled relationships with climate variables and random annual variation in colonization and extirpation probabilities in Yosemite National Park, California, United States, 2007–2021. Points represent posterior medians; error bars represent 95% equal-tailed intervals. The gray horizontal dot-dash line at 1.0 in panels (B,E,H) indicates stable occupancy.
On average, detection probabilities for larvae were higher than detection probabilities for adults for all species (Tables 2–4; Figure 10). For all species, air temperature had a positive effect on detection probability for all life stages (Tables 2–4; Figure 10). In addition to air temperature, sierran treefrogs were more likely to be detected at larger occupied sites (Table 2; Figure 10). Days since snowmelt had opposite effects on adults and larvae of sierran treefrogs; adults had higher detection probability sooner after snowmelt, whereas larvae had higher detection probability longer after snowmelt (Table 2; Figure 10). For sierran treefrogs, detection probabilities varied substantially among sites (Table 2).
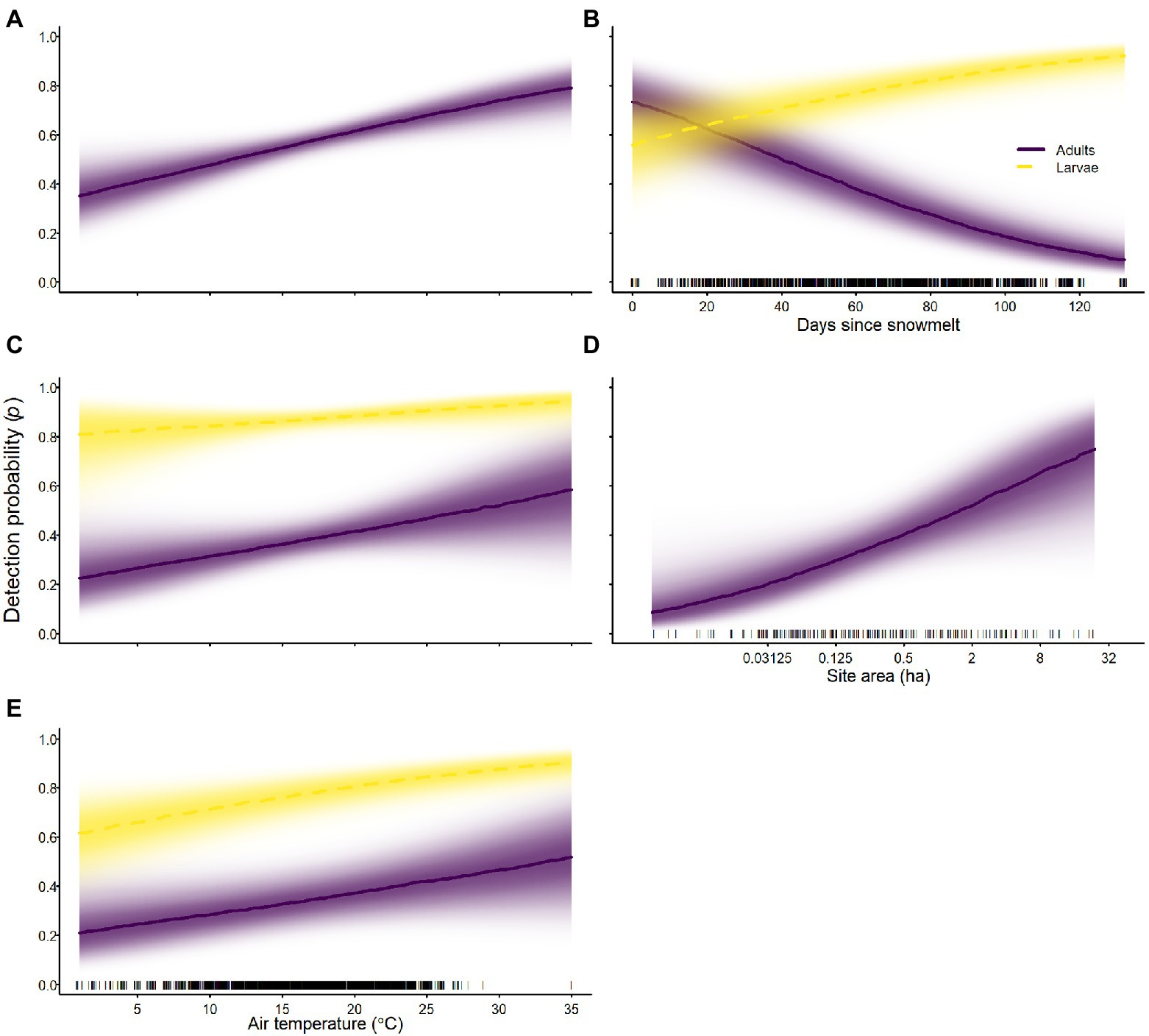
Figure 10. Probability of detection of Sierra Nevada yellow-legged frogs [Rana sierrae; (A)], Yosemite toads [Anaxyrus canorus; (C)], and sierran treefrogs [Pseudacris sierra; (B,D,E)] in relation to air temperature (A,C,E), days since snowmelt (B), site area (D), and life stage [adults (indigo solid lines and shading), larvae (gold dashed lines and shading)] in Yosemite National Park, California, United States, 2007–2021. Lines represent posterior modes; intensity of shading represents the posterior probability density.
4. Discussion
Despite substantial variation in climatic conditions related to water availability, occurrence of adult amphibians in Yosemite was stable. Although climatic variables affected transitions between adult occupancy states, these responses were not so strong as to result in large changes to the proportion of sites occupied in most years. Indeed, after the first 2 years of study, adult occupancy growth rates of all species were near 1.0. Thus, similar to native amphibians in the San Francisco Bay Area of California, United States (Moss et al., 2021), the occurrence of all three anurans was resilient to extremes in winter severity, summer precipitation, and drought over the last 15 years. Individual species, however, often responded differently to weather variables, and in some cases the response varied within species across wetland types.
Although the extirpation probability of all species was affected by the minimum PDSI in September, the response was quite variable across species and wetland types. For example, little evidence existed for an effect of PDSI on sierran treefrog extirpation for seasonal wetlands and permanent waterbodies containing fish, but moderately strong support existed for a negative effect of PDSI on extirpation in permanent waterbodies without fish (i.e., wetter site × year combinations were less likely to be extirpated). A similar pattern was observed for Sierra Nevada yellow-legged frogs: some support existed for a negative effect of PDSI on extirpation in seasonal wetlands, but support for a negative effect of PDSI on extirpation in permanent fishless wetlands was stronger. For both Sierra Nevada yellow-legged frogs and sierran treefrogs, lower probability of occurrence in waterbodies containing fish (Knapp and Matthews, 2000; Matthews et al., 2001; Knapp, 2005) likely superseded any effects of weather on extirpation of these species in these waterbodies. Thus, site characteristics, including co-occurring species, can influence the extent to which climate affects occupancy dynamics (Moss et al., 2021).
Yosemite toads, which were not affected by fish in our models (Knapp, 2005; Grasso et al., 2010; Fellers et al., 2015), exhibited even more context-dependence in the influence of weather on extirpation probabilities. Some support existed for a negative effect of PDSI on extirpation in seasonal sites for Yosemite toads, whereas support was strong for a positive effect of PDSI on Yosemite toad extirpation in permanent sites. The finding that wetter conditions were related to extirpation of Yosemite toads in permanent sites was unexpected. Related to our findings, in high elevation populations of the related boreal toad (Anaxyrus boreas boreas) larval survival was lower in permanent sites than in ephemeral sites (Crockett et al., 2020). We suspect, however, that the terrestrial adults of Yosemite toads might be more strongly tied to permanent waterbodies in dry years, but disperse to more favored meadow habitats in wet years (Berlow et al., 2013; Liang, 2013; Sadinski et al., 2020). Our models cannot distinguish whether extirpation is a result of mortality or emigration, and it is likely that in the case of Yosemite toad extirpation at permanent sites, migration might be the dominant process (Morton and Pereyra, 2010; Liang, 2013).
The colonization of unoccupied sites by all three species was positively related to SPEI03 in September. SPEI03 in September was chosen as a measure of active season (June–August) climatic wetness, and the positive relationship between colonization of unoccupied sites and wetness is likely because wetter conditions facilitate terrestrial activity of amphibians (Bartelt et al., 2004; Schmetterling and Young, 2008). For more aquatic species in particular, a wetter summer environment might allow them to disperse farther from wetlands and facilitate colonization of unoccupied sites, provided the active season is long enough for dispersal to occur.
Winter severity, in contrast to summer wetness, had contrasting effects on colonization probabilities of Sierra Nevada yellow-legged frogs and Yosemite toads. Yosemite toads were more likely to colonize unoccupied sites following more severe winters. Winter severity generally has two major effects on amphibians in the following active season. In high elevation systems snowpack dominates moisture availability, and soil moisture is generally high following severe winters (Bales et al., 2006). Higher soil moisture likely facilitates dispersal of terrestrial amphibians because they can more easily find moist refugia to maintain water balance as they move across the landscape (Baldwin et al., 2006). This is likely the dominant process increasing Yosemite toad colonization probability following severe winters. The other major effect of severe winters is to shorten the subsequent active season because snowmelt occurs later in the year (Bales et al., 2006). In the case of Sierra Nevada yellow-legged frogs, which had decreased colonization probabilities following severe winters, the short duration of the active season might have limited time for colonization of unoccupied sites. This might be especially true if colonization events are dominated by recently metamorphosed subadults, which would disperse through the landscape after undergoing metamorphosis in mid- to late summer. Colonization is not only affected by dispersal, however, but also by successful establishment at unoccupied sites.
In addition to weather, fish had a negative influence on colonization probabilities for Sierra Nevada yellow-legged frogs and sierran treefrogs. The negative effects of introduced fish on amphibian populations are well-known, and introduced trout are negatively related to the occurrence of Sierra Nevada and mountain yellow-legged frogs (Rana muscosa) and sierran treefrogs in the high elevations of the Sierra Nevada (Knapp and Matthews, 2000; Matthews et al., 2001; Knapp, 2005; Fellers et al., 2015). Sierra Nevada and mountain yellow-legged frog populations can rapidly increase in abundance following the removal of non-native fish (Knapp et al., 2007); our study provides additional evidence that introduced fishes inhibit colonization of sites by native amphibians, which further fragments the landscape and impairs the function of healthy amphibian metapopulations (Knapp et al., 2003).
Weather not only affected occupancy dynamics of adult anurans, it also affected the probability that both Sierra Nevada yellow-legged frogs and Yosemite toads would breed. Although support for an effect of April SWE on the probability Yosemite toads would breed was only weakly supported for seasonal wetlands, evidence for a negative effect of winter severity on the probability Yosemite toads would reproduce in permanent wetlands was strong. Yosemite toads typically breed at snowmelt in the shallow margins of wetlands, often in meadows (Liang et al., 2017; Sadinski et al., 2020). In more severe winters, when snowmelt occurs later, conditions might be less favorable for Yosemite toads to reproduce. The stronger relationship between probability of reproduction and winter severity in permanent sites might be caused by reduced snowpack limiting opportunities for Yosemite toads to breed in more favored meadow sites (Liang et al., 2017; Sadinski et al., 2020). For Sierra Nevada yellow-legged frogs, overwhelming support for a positive effect of wetness on the probability of larval occurrence existed for permanent fishless waterbodies, but little support existed for the effects of weather on the probability of larval occurrence in seasonal wetlands or those with fish, likely because these wetland types are unsuitable for Sierra Nevada yellow-legged frog reproduction (Knapp and Matthews, 2000; Knapp, 2005; Fellers et al., 2013). Thus, like extirpation, the influence of weather variables might be context-specific and secondary to the effects of habitat conditions and biotic relationships.
As expected, fish had a negative effect on the probability of larval occurrence for both Sierra Nevada yellow-legged frogs and sierran treefrogs (Knapp and Matthews, 2000; Matthews et al., 2001; Knapp, 2005). The negative effect of fish on reproduction was especially strong for Sierra Nevada yellow-legged frogs, which were 15 (5–71) times more likely to reproduce in permanent waterbodies without fish than those with fish. The negative effect of fish on these frogs is therefore evident for multiple processes and life stages, including the initial occupancy and colonization of adult life stages and the probability that adults will reproduce at the few sites where they co-occur with fish.
Annual probability of reproduction was more variable than adult occupancy, especially for Yosemite toads. Nonetheless, the probability of reproduction remained relatively stable when compared to the high variability in weather variables affecting Yosemite toad and Sierra Nevada yellow-legged frog reproduction. For Sierra Nevada yellow-legged frogs, annual probability of reproduction in permanent waterbodies without fish was remarkably stable. Two main mechanisms likely lead to this stability. First, the alpine lakes in which Sierra Nevada yellow-legged frogs typically breed are permanent and contain water in all years. Second, because Sierra Nevada yellow-legged frog tadpoles overwinter (Fellers et al., 2013), more than one cohort is typically available to be observed. As long as sites maintain water, therefore, observation of pre-metamorphic life stages integrates conditions for up to 3 years. Annual reproduction of Yosemite toads, on the other hand, was more variable. This annual variability was likely caused by the greater response of seasonal wetlands than permanent waterbodies to climatic variation (Moss et al., 2021). In particular, April SWE was highly variable among years and affected the probability of Yosemite toad reproduction in both permanent and seasonal sites. Additional variation might be caused by female toads skipping breeding in some years (Morton and Pereyra, 2010), as occurs with high elevation populations of the related boreal toad (Muths et al., 2010). Sierran treefrogs reproduced with high probability in all site types, regardless of weather conditions. The apparent exception in 2012 was likely caused by aberrant timing of surveys (i.e., after eggs had hatched and before tadpoles had matured enough to be easily sampled in a dip net). Although our model structure accounted for annual variation in larval detection probabilities, the nearly universally high detection probability of sierran treefrog tadpoles resulted in what we suspect is an artificially low probability of reproduction (and high probability of detection) when the timing of surveys relative to tadpole development was mismatched.
Initial occurrence probabilities largely agreed with past research on these species. For example, a positive relationship between site area and occupancy is generally expected across taxa (Gleason, 1922; Storch et al., 2005). A negative relationship between the occurrence of introduced fish and many anurans also is well-documented (Knapp and Matthews, 2000; Matthews et al., 2001; Knapp, 2005; Fellers et al., 2015). We did not evaluate the influence of introduced fish on Yosemite toads because past research does not suggest that toads are affected negatively by fish, and laboratory trials suggest that Yosemite toad larvae are unpalatable to trout, the dominant introduced fish in the Sierra Nevada (Knapp, 2005; Grasso et al., 2010; Fellers et al., 2015). The peak in initial occurrence for Sierra Nevada yellow-legged frogs near 2,700 m elevation and the corresponding trough for Yosemite toads at the same elevation might be an artifact of the composition of sites in our selected watersheds. A relatively high proportion of sites at the lowest and highest elevation watersheds we sampled were meadows, whereas lakes, ponds, and streams dominated mid-elevation sites. Therefore, the relationship between elevation and initial probability of occurrence for these species might be indicative of this artifact rather than representing larger patterns throughout the ranges of these species (Knapp, 2005).
Occurrence is a blunt instrument for evaluating effects of any variable on the status or trends of populations. Demographic changes leading to changes in abundance might be masked by using occupancy as the response variable of interest, and populations often decline substantially in abundance before becoming extirpated. Another limitation of our study was that we visited each site once when we expected that larval anurans would be present. Therefore, our measure of reproduction at each site did not indicate whether larvae successfully underwent metamorphosis and recruited into the subadult population. Additional follow-up visits to evaluate successful recruitment following reproduction would have limited the number of sites visited each year. Our measures of adult occupancy and probability of reproduction are nonetheless valuable for the long time series and large spatial extent.
Although our study found that amphibian occurrence and reproduction were robust to recent variability in water availability, as the climate becomes more variable in the Sierra Nevada (Swain et al., 2018), the life history strategies that amphibians have evolved over millennia might be inadequate to ensure the persistence of these species. For example, the reliance of Sierra Nevada yellow-legged frogs on permanent fishless waterbodies places them at risk of extirpation if sites shift from permanent lakes and streams to semi-permanent or seasonal wetlands. For a species that has already been extirpated from many historical sites, further extirpations could be costly. Limiting other stressors, such as removing invasive fish from permanent lakes and streams, would help to increase colonization probabilities and maintain the connectivity necessary for functioning Sierra Nevada yellow-legged frog metapopulations and foster resilience to climate change (Knapp et al., 2003, 2007). Yosemite toads are likely susceptible to further increases in the variability of precipitation in the Sierra Nevada as well. Although these toads are adapted to periodic, perhaps even frequent, recruitment failure (Morton and Pereyra, 2010; Liang et al., 2017; Sadinski et al., 2020), it is unclear whether the longevity of adult toads will enable persistence of the toads through megadroughts or severe winters associated with megafloods (Ault et al., 2016; Huang and Swain, 2022). A better understanding of the demography of Yosemite toads, especially the relationship between weather variables and adult survival and recruitment, would be necessary to evaluate the probability of this species persisting in a changing climate.
The occurrence of anurans in Yosemite has so far been resilient to recent extreme climatic events. Despite the influences of weather on adult occupancy dynamics and probability of reproduction, the occurrence of anurans in Yosemite has remained stable over the past 15 years. As the climate continues to change, changes to the hydrology of breeding sites and ecological communities might result in changes to the distribution and occurrence of anurans in the Sierra Nevada. Our study provides insight into the mechanisms exacerbating extirpations and facilitating colonization and reproduction of native anurans in the Sierra Nevada to inform conservation and management of these species.
Data availability statement
Data presented in the study are deposited in ScienceBase and are accessible at https://doi.org/10.5066/P9X6SKT8 (Kleeman et al., 2022). Code used to analyze the data are deposited on GitLab and is accessible at https://doi.org/10.5066/P9VR4SH3 (Halstead et al., 2022).
Ethics statement
The animal study was reviewed and approved by USGS Western Ecological Research Center Animal Care and Use Committee (protocol WERC-2014-01).
Author contributions
BH: conceptualization, methodology, formal analysis, investigation, writing–original draft, writing–review and editing, visualization, and supervision. PK: conceptualization, methodology, data curation, writing–review and editing, and supervision. JR: conceptualization, writing–review and editing, and visualization. GF: conceptualization, methodology, investigation, supervision, and project administration. All authors contributed to the article and approved the submitted version.
Funding
This project was funded by the U.S. Geological Survey (USGS) Ecosystems Mission Area through the USGS Amphibian Research and Monitoring Initiative (ARMI).
Acknowledgments
We thank the numerous technicians that contributed to this project, including Isaac Chellman, Kathryn Cooney, Jennifer Dhundale, David Dimitrie, Sara Dykman, Devon Edmonds, Emmy Johnson, Jane Lester, Patrick Lien, Mike McDonald, Britt Ousterhout, Anjana Parandhaman, John Romansic, Kelsey Ruehling, Heyo Tjarks, Andy Valencia, Liz Williams, and JP Zegarra. Surveys were conducted under U.S. Fish and Wildlife Service Permit TE-844852 and Yosemite National Park annual permits for Study ID 00544, and followed IACUC protocol WERC-2014-01. This work is publication 859 of the USGS ARMI. Any use of trade, product, or firm names is for descriptive purposes only and does not imply endorsement by the U.S. Government.
Conflict of interest
The authors declare that the research was conducted in the absence of any commercial or financial relationships that could be construed as a potential conflict of interest.
Publisher’s note
All claims expressed in this article are solely those of the authors and do not necessarily represent those of their affiliated organizations, or those of the publisher, the editors and the reviewers. Any product that may be evaluated in this article, or claim that may be made by its manufacturer, is not guaranteed or endorsed by the publisher.
References
Ault, T. R., Mankin, J. S., Cook, B. I., and Smerdon, J. E. (2016). Relative impacts of mitigation, temperature, and precipitation on 21st-century megadrought risk in the American southwest. Sci. Adv. 2:e1600873. doi: 10.1126/sciadv.1600873
Baldwin, R. F., Calhoun, A. J. K., and Demaynadier, P. G. (2006). Conservation planning for amphibian species with complex habitat requirements: a case study using movements and habitat selection of the wood frog Rana sylvatica. J. Herpetol. 40, 442–453. doi: 10.1670/0022-1511(2006)40[442:CPFASW]2.0.CO;2
Bales, R. C., Molotch, N. P., Painter, T. H., Dettinger, M. D., Rice, R., and Dozier, J. (2006). Mountain hydrology of the western United States. Water Resour. Res. 42, 1–13. doi: 10.1029/2005WR004387
Bartelt, P. E., Peterson, C. R., and Klaver, R. W. (2004). Sexual differences in the post-breeding movements and habitats selected by Western toads (Bufo boreas) in southeastern Idaho. Herpetologica 60, 455–467. doi: 10.1655/01-50
Berlow, E. L., Knapp, R. A., Ostoja, S. M., Williams, R. J., McKenny, H., Matchett, J. R., et al. (2013). A network extension of species occupancy models in a patchy environment applied to the Yosemite toad (Anaxyrus canorus). PLoS One 8:e72200. doi: 10.1371/journal.pone.0072200
Briggs, C. J., Vredenburg, V. T., Knapp, R. A., and Rachowicz, L. J. (2005). Investigating the population-level effects of chytridiomycosis: an emerging infectuous disease of amphibians. Ecology 86, 3149–3159. doi: 10.1890/04-1428
Crockett, J. G., Bailey, L. L., and Muths, E. (2020). Highly variable rates of survival to metamorphosis in wild boreal toads (Anaxyrus boreas boreas). Popul. Ecol. 62, 258–268. doi: 10.1002/1438-390X.12044
Crump, M. L., and Scott, N. J. J. (1994). “Visual encounter surveys” in Measuring and Monitoring Biological Diversity: Standard Methods for Amphibians. eds. W. R. Heyer, M. A. Donnelly, R. W. McDiarmid, L.-A. C. Hayek, and M. S. Foster (Washington and London: Smithsonian Institution Press), 84–92.
Dallalio, E. A., Brand, A. B., and Grant, E. H. C. (2017). Climate-mediated competition in a high-elevation salamander community. J. Herpetol. 51, 190–196. doi: 10.1670/15-157
de Valpine, P., Paciorek, C., Turek, D., Michaud, N., Anderson-Bergman, C., Obermeyer, F., et al. (2022). NIMBLE: MCMC, Particle Filtering, and Programmable Hierarchical Modeling. R package version 0.12.2. Available at: https://cran.r-project-org/package=nimble. doi: 10.5281/zenodo.1211190
Duarte, A., Peterson, J. T., Pearl, C. A., Rowe, J. C., McCreary, B., Galvan, S. K., et al. (2020). Estimation of metademographic rates and landscape connectivity for a conservation-reliant anuran. Landsc. Ecol. 35, 1459–1479. doi: 10.1007/s10980-020-01030-8
Ethier, J. P., Fayard, A., Soroye, P., Choi, D., Mazerolle, M. J., and Trudeau, V. L. (2021). Life history traits and reproductive ecology of north American chorus frogs of the genus Pseudacris (Hylidae). Front. Zool. 18:40. doi: 10.1186/s12983-021-00425-w
Evans, T. G., Diamond, S. E., and Kelly, M. W. (2015). Mechanistic species distribution modelling as a link between physiology and conservation. Conserv. Physiol. 3, 1–16. doi: 10.1093/conphys/cov056
Fellers, G. M., Kleeman, P. M., and Miller, D. A. W. (2015). Wetland occupancy of pond-breeding amphibians in Yosemite National Park, USA. J. North Am. Herpetol. 2015, 22–33. doi: 10.17161/jnah.vi1.11903
Fellers, G. M., Kleeman, P. M., Miller, D. A. W., Halstead, B. J., and Link, W. A. (2013). Population size, survival, growth, and movements of Rana sierrae. Herpetologica 69, 147–162. doi: 10.1655/herpetologica-d-12-00045
Gelman, A., and Rubin, D. B. (1992). Inference from iterative simulation using multiple sequences. Stat. Sci. 7, 457–472. doi: 10.1214/ss/1177011136
Gleason, H. A. (1922). On the relation between species and area. Ecology 3, 158–162. doi: 10.2307/1929150
Grasso, R. L., Coleman, R. M., and Davidson, C. (2010). Palatability and antipredator response of Yosemite toads (Anaxyrus canorus) to nonnative brook trout (Salvelinus fontinalis) in the Sierra Nevada Mountains of California. Copeia 2010, 457–462. doi: 10.1643/ch-09-033
Halstead, B. J., Kleeman, P. M., Rose, J. P., and Fellers, G. M. (2022). “Code to Analyze Multi-State, Multi-Scale Dynamic Occupancy Models for Amphibians in Yosemite National Park,” in U.S. Geological Survey Code Release. doi: 10.5066/P9VR4SH3
Halstead, B. J., Rose, J. P., and Kleeman, P. M. (2021). Time-to-detection occupancy methods: performance and utility for improving efficiency of surveys. Ecol. Appl. 31:e02267. doi: 10.1002/eap.2267
Huang, X., and Swain, D. L. (2022). Climate change is increasing the risk of a California megaflood. Sci. Adv. 8:eabq0995. doi: 10.1126/sciadv.abq0995
Kagarise Sherman, C., and Morton, M. L. (1993). Population declines of Yosemite toads in the eastern Sierra Nevada of California. J. Herpetol. 27, 186–198. doi: 10.2307/1564935
Kleeman, P. M., Halstead, B. J., Rose, J. P., and Fellers, G. M. (2022). “Multi-state occurrence data and predictors for amphibians in Yosemite National Park, 2007–2021,” in U.S. Geological Survey Data Release. doi: 10.5066/P9X6SKT8
Knapp, R. A. (2005). Effects of nonnative fish and habitat characteristics on lentic herpetofauna in Yosemite National Park, USA. Biol. Conserv. 121, 265–279. doi: 10.1016/j.biocon.2004.05.003
Knapp, R. A., Boiano, D. M., and Vredenburg, V. T. (2007). Removal of nonnative fish results in population expansion of a declining amphibian (mountain yellow-legged frog, Rana muscosa). Biol. Conserv. 135, 11–20. doi: 10.1016/j.biocon.2006.09.013
Knapp, R. A., and Matthews, K. R. (2000). Non-native fish introductions and the decline of the mountain yellow-legged frog from within protected areas. Conserv. Biol. 14, 428–438. doi: 10.1046/j.1523-1739.2000.99099.x
Knapp, R. A., Matthews, K. R., Preisler, H. K., and Jellison, R. (2003). Developing probabilistic models to predict amphibian site occupancy in a patchy landscape. Ecol. Appl. 13, 1069–1082. doi: 10.1890/1051-0761(2003)13[1069,DPMTPA]2.0.CO;2
Knapp, R. A., Pavelka, C., Hegeman, E. E., and Smith, T. C. (2020). The Sierra Lakes inventory project: non-native fish and community composition of lakes and ponds in the Sierra Nevada, California version 2. Environ. Data Initiative. doi: 10.6073/pasta/d835832d7fd00d9e4466e44eea87fab3
Liang, C. T. (2013). Movements and habitat use of Yosemite toads (Anaxyrus (formerly Bufo) canorus) in the sierra National Forest, California. J. Herpetol. 47, 555–564. doi: 10.1670/12-054
Liang, C. T., Grasso, R. L., Nelson-Paul, J. J., Vincent, K. E., and Lind, A. J. (2017). Fine-scale habitat characteristics related to occupancy of the Yosemite toad, Anaxyrus canorus. Copeia 105, 120–127. doi: 10.1643/ch-15-290
Lindauer, A. L., and Voyles, J. (2019). Out of the frying pan, into the fire? Yosemite toad (Anaxyrus canorus) susceptibility to Batrachochytrium dendobatidis after development under drying conditions. Herpetol. Conserv. Biol. 14, 185–198.
Matthews, K. R., Pope, K. L., Preisler, H. K., and Knapp, R. A. (2001). Effects of nonnative trout on Pacific treefrogs (Hyla regilla) in the Sierra Nevada. Copeia 2001, 1130–1137. doi: 10.1643/0045-8511(2001)001[1130:eontop]2.0.co;2
Menéndez, R., Megías, A. G., Hill, J. K., Braschler, B., Willis, S. G., Collingham, Y., et al. (2006). Species richness changes lag behind climate change. Proc. R. Soc. B Biol. Sci. 273, 1465–1470. doi: 10.1098/rspb.2006.3484
Merkle, J. A., Abrahms, B., Armstrong, J. B., Sawyer, H., Costa, D. P., and Chalfoun, A. D. (2022). Site fidelity as a maladaptive behavior in the Anthropocene. Front. Ecol. Environ. 20, 187–194. doi: 10.1002/fee.2456
Morton, M. L., and Pereyra, M. E. (2010). Habitat use by Yosemite toads: life history traits and implications for conservation. Herpetol. Conserv. Biol. 5, 388–394.
Moss, W. E., McDevitt-Galles, T., Muths, E., Bobzien, S., Purificato, J., and Johnson, P. T. J. (2021). Resilience of native amphibian communities following catastrophic drought: evidence from a decade of regional-scale monitoring. Biol. Conserv. 263:109352. doi: 10.1016/j.biocon.2021.109352
Mullaly, D. P. (1953). Observations on the ecology of the toad Bufo canorus. Copeia 1953, 182–183. doi: 10.2307/1439932
Muths, E., Scherer, R. D., and Lambert, B. A. (2010). Unbiased survival estimates and evidence for skipped breeding opportunities in females. Methods Ecol. Evol. 1, 123–130. doi: 10.1111/j.2041-210x.2010.00019.x
R Core Team (2022). R: A Language and Environment for Statistical Computing. Vienna, Austria: R Foundation for Statistical Computing.
Rose, J. P., Kleeman, P. M., and Halstead, B. J. (2022). Hot, wet and rare: modelling the occupancy dynamics of the narrowly distributed Dixie Valley toad. Wildl. Res. (in press). doi: 10.1071/WR22029
Sadinski, W., Gallant, A. L., and Cleaver, J. E. (2020). Climate’s cascading effects on disease, predation, and hatching success in Anaxyrus canorus, the threatened Yosemite toad. Glob. Ecol. Conserv. 23:e01173. doi: 10.1016/j.gecco.2020.e01173
Schmetterling, D. A., and Young, M. K. (2008). Summer movements of boreal toads (Bufo boreas boreas) in two western Montana basins. J. Herpetol. 42, 111–123. doi: 10.1670/07-125r1.1
Storch, D., Evans, K. L., and Gaston, K. J. (2005). The species-area-energy relationship. Ecol. Lett. 8, 487–492. doi: 10.1111/j.1461-0248.2005.00740.x
Swain, D. L., Langenbrunner, B., Neelin, J. D., and Hall, A. (2018). Increasing precipitation volatility in twenty-first-century California. Nat. Clim. Chang. 8, 427–433. doi: 10.1038/s41558-018-0140-y
Thornton, M. M., Shrestha, R., Wei, Y., Thornton, P. E., Kao, S., and Wilson, B. E. (2020). Daymet: Monthly Climate Summaries on a 1-km Grid for North America, Version 4. ORNL DAAC, Oak Ridge, TN. (Accessed August 16, 2022).
U.S. Fish and Wildlife Service (2014). Endangered and threatened wildlife and plants; endangered species status for Sierra Nevada yellow-legged frog and northern distinct population segment of the mountain yellow-legged frog, and threatened species status for Yosemite toad. Fed. Regist. 79, 24256–24310.
Vose, R. S., Applequist, S., Squires, M., Durre, I., Menne, M. J., Williams, C. N. Jr., et al. (2014). Improved historical temperature and precipitation time series for U.S. climate divisions. J. Appl. Meteorol. Climatol. 53, 1232–1251. doi: 10.1175/JAMC-D-13-0248.1
Vredenburg, V. T., Knapp, R. A., Tunstall, T. S., and Briggs, C. J. (2010). Dynamics of an emerging disease drive large-scale amphibian population extinctions. Proc. Natl. Acad. Sci. U. S. A. 107, 9689–9694. doi: 10.1073/pnas.0914111107
Keywords: anuran, climate change, drought, multi-state occupancy model, occurrence, reproduction, Yosemite National Park
Citation: Halstead BJ, Kleeman PM, Rose JP and Fellers GM (2023) Sierra Nevada amphibians demonstrate stable occupancy despite precipitation volatility in the early 21st Century. Front. Ecol. Evol. 10:1040114. doi: 10.3389/fevo.2022.1040114
Edited by:
Clare Aslan, Northern Arizona University, United StatesReviewed by:
Diogo Alagador, University of Évora, PortugalErin Muths, United States Department of the Interior, United States
Copyright © 2023 Halstead, Kleeman, Rose and Fellers. This is an open-access article distributed under the terms of the Creative Commons Attribution License (CC BY). The use, distribution or reproduction in other forums is permitted, provided the original author(s) and the copyright owner(s) are credited and that the original publication in this journal is cited, in accordance with accepted academic practice. No use, distribution or reproduction is permitted which does not comply with these terms.
*Correspondence: Brian J. Halstead, ✉ YmhhbHN0ZWFkQHVzZ3MuZ292
†Deceased