- INRAE, Sorbonne Université, CNRS, IRD, UPEC, Université Paris Cité, Institute of Ecology and Environmental Sciences of Paris, Versailles, France
In moths, mate finding relies on female-emitted sex pheromones that the males have to decipher within a complex environmental odorant background. Previous studies have shown that interactions of both sex pheromones and plant volatiles can occur in the peripheral olfactory system, and that some plant volatiles can activate the pheromone-specific detection pathway. In the noctuid moth Agrotis ipsilon, plant volatiles such as heptanal activate the receptor neurons tuned to the pheromone component (Z)7-12:OAc. However, the underlying mechanisms remain totally unknown. Following the general rule that states that one olfactory receptor neuron usually expresses only one type of receptor protein, a logic explanation would be that the receptor protein expressed in (Z)7-12:OAc-sensitive neurons recognizes both pheromone and plant volatiles. To test this hypothesis, we first annotated odorant receptor genes in the genome of A. ipsilon and we identified a candidate receptor putatively tuned to (Z)7-12:OAc, named AipsOR3. Then, we expressed it in Drosophila olfactory neurons and determined its response spectrum to a large panel of pheromone compounds and plant volatiles. Unexpectedly, the receptor protein AipsOR3 appeared to be very specific to (Z)7-12:OAc and was not activated by any of the plant volatiles tested, including heptanal. We also found that (Z)7-12:OAc responses of Drosophila neurons expressing AipsOR3 were not affected by a background of heptanal. As the Drosophila olfactory sensilla that house neurons in which AipsOR3 was expressed contain other olfactory proteins – such as odorant-binding proteins – that may influence its selectivity, we also expressed AipsOR3 in Xenopus oocytes and confirmed its specificity and the lack of activation by plant volatiles. Altogether, our results suggest that a still unknown second odorant receptor protein tuned to heptanal and other plant volatiles is expressed in the (Z)7-12:OAc-sensitive neurons of A. ipsilon.
Introduction
In most moth species, females release a unique mixture of chemicals, called a sex pheromone, in an attempt to attract males, which specifically recognize it from far away (Kaissling, 2014). All the pheromonal signals released into the air are immersed in an olfactory environment: plants also emit their own volatile molecules, which have diverse functions including communication with other organisms. In addition, the trajectory of pheromones can be quite erratic because the air can have an unpredictable and turbulent movement. The pheromonal signal is therefore quite fragmented and drowned in a very rich odor background, which has been shown to modulate pheromone detection (Deisig et al., 2014; Renou et al., 2015).
Moth pheromone compounds, like any odorant molecule, are recognized by transmembrane receptors located in the dendrite membrane of olfactory receptor neurons (ORNs), located in several thousand sensilla on the antenna (Fleischer and Krieger, 2018). In insects, the major family of such receptors are the odorant receptors (ORs). Each ORN usually expresses only one type of OR, detecting a limited number of chemicals, in addition to the obligatory co-receptor named Orco (Larsson et al., 2004), but various lines or evidence indicate that each ORN can express more than just one OR and Orco (Couto et al., 2005; Fishilevich and Vosshall, 2005; Koutroumpa et al., 2014; Schultze et al., 2014; McLaughlin et al., 2021; Herre et al., 2022; Task et al., 2022). In moths, pheromone compounds are detected by receptors belonging to particular OR lineages (Zhang and Löfstedt, 2015; Bastin-Héline et al., 2019; Montagné et al., 2021). Whereas the majority of ORs have a broad activation spectrum, i.e., they can recognize several odorant molecules, pheromone receptors (PRs) are often very specific to their ligand or at least narrowly tuned to similar chemicals (Zhang and Löfstedt, 2015). Once interaction occurs between an odorant and the corresponding OR-Orco complex, the signal is transformed by the ORN into an electrical signal that is transmitted along the antennal nerve to the brain primary olfactory centers, the antennal lobes (ALs). There, peripheral inputs are processed, enabling the insect to extract relevant information and adopt the correct behavior (Masse et al., 2009; Touhara and Vosshall, 2009).
It has been demonstrated that volatile plant compounds (VPCs) can modulate the male pheromone response at ORNs, ALs, and behavioral level. At the behavioral level, both field and laboratory studies have shown a synergistic effect of VPC/pheromone mixtures on pheromone trapping (Light et al., 1993; Landolt and Phillips, 1997; Deng et al., 2004) and on male orientation in wind tunnel experiments (Schmidt-Busser et al., 2009; Varela et al., 2011). Such synergism has also been evidenced within the male macroglomerular complex (a set of enlarged glomeruli dedicated to the process of pheromones in the ALs) in Cydia pomonella (Trona et al., 2013). At the pheromone-responsive ORN level, studies are more contrasted. Addition of a VPC to the pheromone usually results in a suppressive effect on the pheromone response (Den Otter et al., 1978; Pophof and van der Goes van Naters, 2002). For instance, a background of linalool (one of the most common VPCs in nature) appears to halve the response of ORNs tuned to the major component of the sex pheromone in Spodoptera littoralis (Party et al., 2009). Similarly, a background of some VPCs – namely linalool, (Z)3-hexenyl acetate, hexanal and heptanal – reduces the firing rate of pheromone-responsive ORNs in the black cutworm moth Agrotis ipsilon (Renou et al., 2015). However, the effects have been shown to be VPC-dependent in Heliothis virescens. In this species, VPCs like linalool, geraniol or (Z)3-hexenyl acetate diminish the response of (Z)11-16:Ald-specific ORNs and this suppressive effect has been shown to occur at the level of the PR protein (Hillier and Vickers, 2010; Pregitzer et al., 2012). By contrast, a mixture of (Z)11-16:Ald and β-caryophyllene appears to enhance the activity of the (Z)11-16:Ald ORNs (Hillier and Vickers, 2010). In Helicoverpa zea, mixtures of linalool or (Z)3-hexenol and the major sex pheromone component also synergize the response of pheromone-responsive ORNs (Ochieng et al., 2002). Moreover, it has unexpectedly been shown in A. ipsilon that ORNs tuned to (Z)7-12:OAc – one of the three components of the sex pheromone – can respond to stimulation with VPCs alone, notably linalool, (Z)3-hexenyl acetate and heptanal (Rouyar et al., 2015; Conchou et al., 2021). Consequently, backgrounds of these volatiles mask pheromone responses and impact male behavior, notably by altering the perception of the ratio of pheromone constituents (Dupuy et al., 2017; Hoffmann et al., 2020). However, the mechanisms underlying this response of pheromone-sensitive ORNs to VPCs remain unknown.
Several hypotheses can be put forward. Although it is generally accepted that ORNs express only one type of OR, many exceptions have been documented (Couto et al., 2005; Fishilevich and Vosshall, 2005; Koutroumpa et al., 2014; Schultze et al., 2014; McLaughlin et al., 2021; Herre et al., 2022). First, A. ipsilon (Z)7-12:OAc-responsive ORNs could express several ORs, one specific for the pheromone and other(s) recognizing VPCs. Second, some moth PRs could be less specific than expected and able to bind certain VPCs in addition to pheromone compounds. In order to test these hypotheses and determine whether activation by VPCs occurs at the level of the PR or not, the aim of the present work was to identify the A. ipsilon receptor tuned to (Z)7-12:OAc, taking advantage of its recently published genome (Wang et al., 2021), and to study its functional properties in heterologous systems, isolated from its natural neuronal environment.
Materials and methods
Annotation of OR genes in the Agrotis ipsilon genome and identification of candidate pheromone receptors
Agrotis ipsilon OR (AipsOR) gene models were created by aligning a set of 485 full length amino acid OR sequences previously identified in Noctuidae (including sequences from the A. ipsilon antennal transcriptome) on the genome assembly GCA_004193855.1 (BioProject PRJNA428387) using Exonerate v2.4.0 (Slater and Birney, 2005) with a score threshold set at 1,000, maximum intron length set at 10,000 and the three best results per query reported. Amino acid sequences translated from these gene models were extracted with the gffread utility from the Cufflinks package (Trapnell et al., 2010), then potential redundant sequences were clustered using CD-HIT protein (Fu et al., 2012).
To identify AipsORs belonging to the lineage of moth PRs, AipsOR sequences were aligned with PR sequences from Bombyx mori (Krieger et al., 2005), Heliothis virescens (Krieger et al., 2004) and Spodoptera littoralis (Meslin et al., 2022) using Clustal Omega v1.2.2 (Sievers and Higgins, 2018) and a phylogeny was built from this alignment using FastTree v2.1.11 (Price et al., 2009) as implemented in Geneious Prime® v2022.1 (Biomatters Ltd., Auckland, New Zealand). Then, a maximum-likelihood phylogeny of the PR lineage was built using PhyML 3.0 (Guindon et al., 2010) with default parameters.
Heterologous expression of AipsOR3 in Drosophila melanogaster and single-sensillum recordings
The AipsOr3 full-length open reading frame (ORF) was amplified by RT-PCR (forward primer 5′-ATGAAATTATTCTCTGATCTGTCTG-3′; reverse primer 5′-TTAATCTTCCTCCGCAACTG-3′) from cDNA prepared (Superscript II reverse transcriptase, Thermo Fisher Scientific, Carlsbad, CA, United States) from RNA extracted from male antennae (TRIzol® Reagent, Thermo Fisher Scientific), cloned into pCR™II-TOPO™ (Thermo Fisher Scientific) and then subcloned into the pUAST.attB vector. Plasmids were purified using the NucleoBond® Xtra Maxi Endotoxin Free kit (Macherey-Nagel, Düren, Germany). Plasmids were injected into fly embryos with the genotype y1 M{vas-int.Dm}ZH-2A w*; m{3xP3-RFP.attP}ZH-51C to generate flies carrying the UAS-AipsOr3 genetic construct on the region 51C of the second chromosome (BestGene Inc., Chino Hills, CA, United States). The UAS-AipsOr3 line was then crossed to the Or67dGAL4[2] mutant knock-in line (Kurtovic et al., 2007) to generate flies with the genotype w; UAS-AipsOr3; Or67dGAL4[2] that express the AipsOR3 receptor instead of the endogenous receptor Or67d.
Single-sensillum recordings on at1 sensilla were performed as described in de Fouchier et al., 2015. For the screening experiment, stimulus cartridges consisted of Pasteur pipettes containing a filter paper loaded with either 10 μg/μl of an hexane solution of the pheromone compound (1 μg μl−1) or 10 μl of a VPC diluted in mineral oil (1% v/v). Control cartridges contained 10 μl of hexane or mineral oil alone. Dose–response experiments with (Z)7-12:OAc were performed with decimal dilutions ranging from 1 μg μl−1 down to 100 pg μl−1, and those with heptanal were performed with 20, 10, 5 and 1% v/v dilutions (10 μl in the stimulus cartridge). Each of the recorded at1 ORNs was stimulated with all doses of the two compounds, as well as cartridges with solvent alone. For experiments with odorant backgrounds, stimuli were delivered using a custom-made device described in Rouyar et al., 2015. Backgrounds were generated using glass vials containing 1 ml of heptanal diluted in mineral oil (1% v/v) or mineral oil alone. Pheromone stimulations were made using a glass vial containing a filter paper loaded with 10 μl of (Z)7-12:OAc diluted in hexane (1 μg/μl). Source and purity of the chemicals used as stimuli can be found in Supplementary Table 1. Recordings were analyzed with the pCLAMP™ 11 software (Molecular Devices, San Jose, CA, United States) by subtracting the spontaneous firing rate before stimulation (in spikes·s−1, measured during 500 ms before the onset of stimulation) from the firing rate during the stimulation (measured during a 500 ms time window which started 200 ms after the onset of stimulation). Statistical analyses were done with Prism 9.3.1 (GraphPad Software, San Diego, CA, United States) and RStudio (2022.02.3; RStudio Team, 2022).
Heterologous expression of AipsOR3/AipsOrco in xenopus oocytes and two-electrode voltage-clamp
The AipsOrco full-length ORF was synthesized in vitro by Synbio Technologies (Monmouth Junction, NJ, United States). AipsOr3 and AipsOrco ORFs were then sub-cloned into the pCS2+ vector (Synbio Technologies). Plasmids were linearized with NotI, then capped cRNAs were synthesized using SP6 RNA polymerase with the mMESSAGE mMACHINE™ SP6 transcription kit (Thermo Fisher Scientific). Purified cRNAs were resuspended in nuclease-free water at a 2 μg/μl concentration and stored at −80°C. Mature defolliculated oocytes were purchased from EcoCyte Bioscience (Dortmund, Germany) and microinjected upon delivery with the mixture of 27.6 ng of AipsOR3 cRNA and 27.6 ng of AipsOrco cRNA using a Nanoject III injector (Drummond Scientific, Broomall, PA, United States).
After 2–3 days of incubation at 18°C in Ringer’s solution containing 5% dialyzed horse serum, 50 mg/L tetracycline, 100 mg/L streptomycin and 550 mg/L sodium pyruvate, two-electrode voltage-clamp recordings were performed at a holding potential of −80 mV. Intracellular glass electrodes were filled with 1:2 KCl (3 M): potassium acetate (3 M) and had resistances of 0.2–2.0 MΩ. Stock solutions of pheromone compounds and plant volatiles were prepared by diluting each compound to 1 M in dimethyl sulfoxide and were stored at −20°C until use. Before each experiment, the stock solution was diluted to the working concentration in Ringer’s buffer (10−5 M for pheromone compounds, 10−4 M for plant volatiles). Data acquisition and analysis were carried out with Digidata 1550A and pCLAMP™ 11 (Molecular Devices).
Results
Annotation of Agrotis ipsilon candidate pheromone receptors
Forty-one ORs were previously identified in the antennal transcriptome of A. ipsilon, including five ORs belonging to the PR clade: AipsOR1, 2, 3, 4, and 14 (Gu et al., 2014). We used the recently released genome of A. ipsilon (Wang et al., 2021) to annotate the complete repertoire of OR genes, and found a total of 76 genes (Supplementary Data Sheet 1). We notably identified five additional members of the PR clade, which were paralogues of AipsOR1 and AipsOR4 not found in the transcriptome (Figure 1). The phylogenetic analysis revealed that among those 10 candidate PRs, AipsOR3 was orthologous to the A. segetum receptor AsegOR4, which is narrowly tuned to (Z)7-12:OAc (Zhang and Löfstedt, 2013). Moreover, AipsOR3 was previously shown to be highly expressed in A. ipsilon male vs. female antennae (Gu et al., 2014). This receptor thus appeared as the best (Z)7-12:OAc candidate receptor.
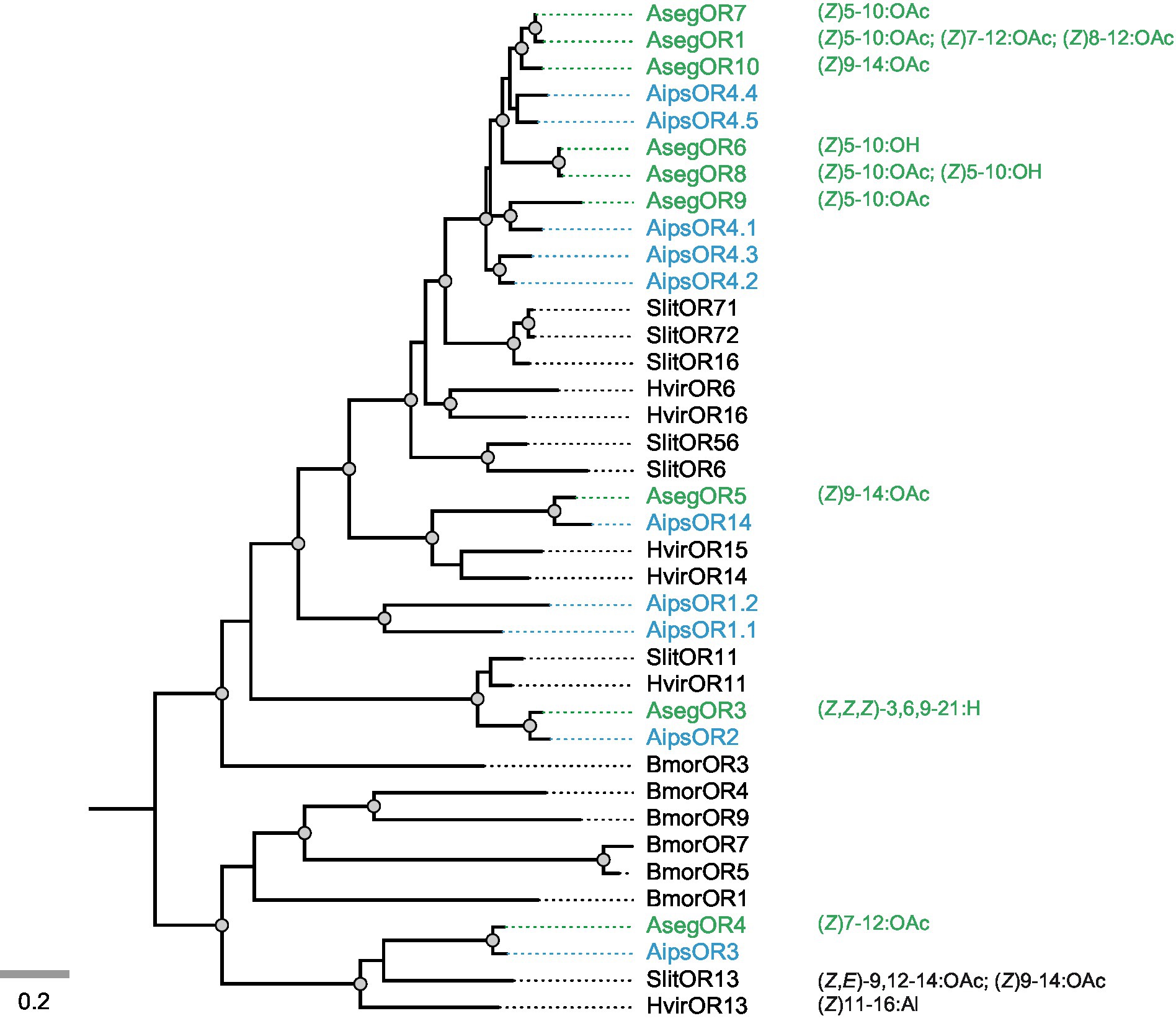
Figure 1. Phylogenetic position of Agrotis ipsilon ORs within the moth sex pheromone receptor clade. The maximum-likelihood tree was built from the alignment of amino acid sequences from A. ipsilon (Aips, in blue), A. segetum (Aseg, in green), Bombyx mori (Bmor), Heliothis virescens (Hvir) and Spodoptera littoralis (Slit). Nodes supported by the likelihood-ratio test (aLRT > 0.9) are shown with gray dots. The best ligands of AsegORs identified previously (Zhang and Löfstedt, 2013; Zhang et al., 2016) are indicated, as well as those of the AipsOR3 orthologues HvirOR13 and SlitOR13 (Große-Wilde et al., 2007; de Fouchier et al., 2015).
Functional characterization of AipsOR3 in drosophila at1 ORNs
To verify whether AipsOR3 was indeed the (Z)7-12:OAc receptor, we generated D. melanogaster lines expressing this receptor in at1 ORNs instead of the endogenous receptor DmelOR67d (Kurtovic et al., 2007). Antennae of transformed flies were stimulated with a range of pheromone compounds presented at high doses, including the three components of the A. ipsilon sex pheromone (Z)7-12:OAc, (Z)9-14:OAc and (Z)11-16:OAc (Gemeno and Haynes, 1998). Single-sensillum recordings showed that AipsOR3-expressing ORNs were significantly activated by (Z)7-12:OAc, with a mean response of ~200 spikes·s−1 (Figure 2A). A very modest response was recorded for the trans isomer (E)7-12:OAc, but it was not statistically different from the control (solvent alone). We tested whether AipsOR3 could be activated by VPCs known to activate (Z)7-12:OAc ORNs in A. ipsilon male antennae, but found no response to any of the four compounds tested (Figure 2A). This was confirmed by dose–response analyses: heptanal, the most active VPC on (Z)7-12:OAc ORNs, was unable to activate AipsOR3-expressing ORNs even when stimulated with a 20% solution, equivalent to more than 1,500 μg loaded in the stimulus cartridge (Figures 2B,C). (Z)7-12:OAc significantly activated the same ORNs starting at a dose of 1 μg.
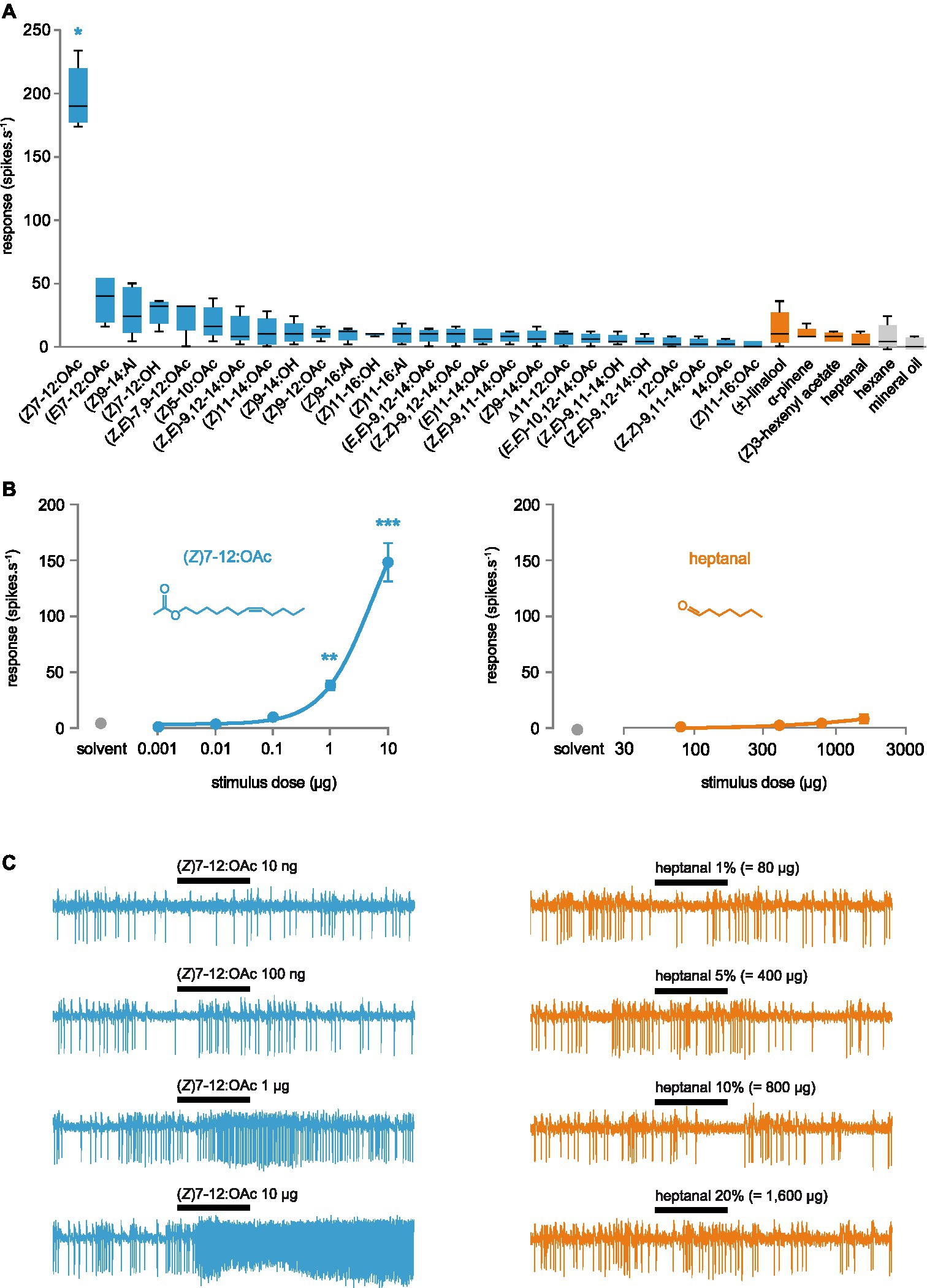
Figure 2. Functional analysis of AipsOR3 expressed in Drosophila at1 neurons. (A) Action potential frequency of at1 neurons expressing AipsOR3 in response to 26 moth pheromone compounds (10 μg in the stimulus cartridge – blue bars) and four volatile plant compounds known to be active on A. ipsilon (Z)7-12:OAc-responsive neurons (80 μg in the stimulus cartridge – orange bars). Boxes show the median and the first and third quartiles, and whiskers show the minimum and maximum values of the distribution (n = 5). *p < 0.05, significantly different from the response to solvent (Friedman ANOVA followed by a Dunn’s post-hoc test). (B) Dose–response curves (mean response ± SEM, n = 11) of at1 neurons expressing AipsOR3 when stimulated with the A. ipsilon pheromone compound (Z)7-12:OAc or the volatile plant compound heptanal. ***p < 0.001, **p < 0.01, significantly different from the response to solvent (Friedman ANOVA followed by a Dunn’s post-hoc test). (C) Example of a series of recordings obtained for an at1 neuron expressing AipsOR3. Black bars represent the stimulus (500 ms).
Functional characterization of AipsOR3 in xenopus oocytes
To test whether the lack of activation by VPCs may be due to the neuronal environment, we also expressed AipsOR3 (together with its co-receptor AipsOrco) in Xenopus oocytes and conducted functional studies. Again, AipsOR3 was strongly and quite specifically activated by (Z)7-12:OAc, and a very low current was measured in response to stimulation with (E)7-12:OAc (Figures 3A,B). As in Drosophila ORNs, no response was found for any of the four VPCs. Overall, these results show that we probably identified the receptor expressed in (Z)7-12:OAc ORNs in A. ipsilon male antennae and that this receptor was not activated by plant volatiles, even at a high dose.
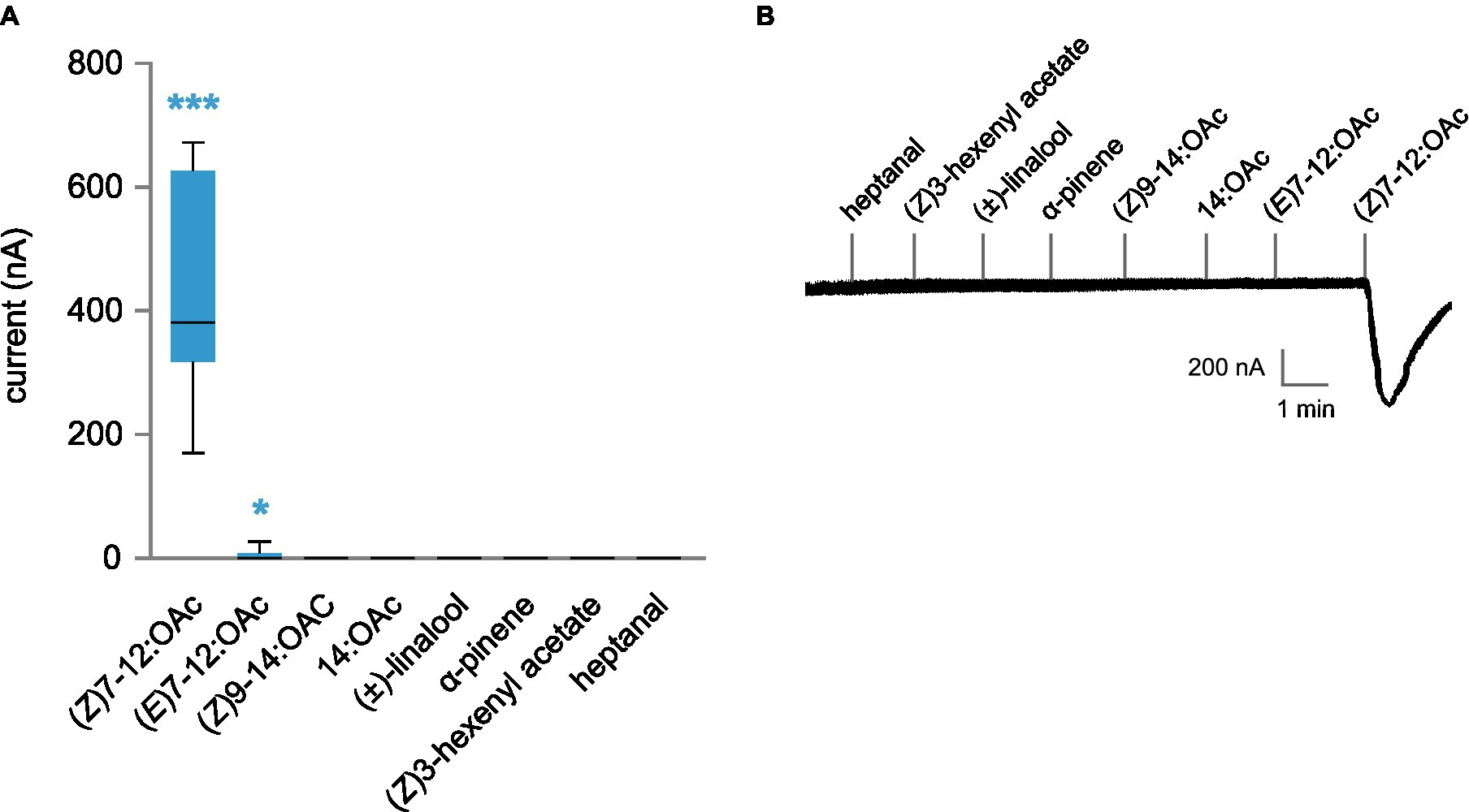
Figure 3. Functional analysis of AipsOR3 expressed in Xenopus oocytes. (A) Inward current measured by two-electrode voltage clamp in oocytes co-expressing AipsOR3 and AipsOrco in response to four moth pheromone compounds (10−5 M) and four volatile plant compounds (10−4 M). Boxes show the median and the first and third quartiles, and whiskers show the minimum and maximum values of the distribution (n = 12–17). ***p < 0.001, *p < 0.05, significantly different from 0 (Wilcoxon signed rank test). (B) Representative TEVC recording obtained for a Xenopus oocyte co-expressing AipsOR3 and AipsOrco.
Effect of a background of heptanal on AipsOR3 function
It has been shown previously in A. ipsilon that the response of (Z)7-12:OAc ORNs is diminished when pheromone stimulation is made in an odorant background of VPCs, notably heptanal (Rouyar et al., 2015; Conchou et al., 2021). We reproduced the same experiment with Drosophila at1 ORNs expressing AipsOR3 and found no difference between responses to (Z)7-12:OAc when presented in a heptanal background and a neutral background (Figure 4).
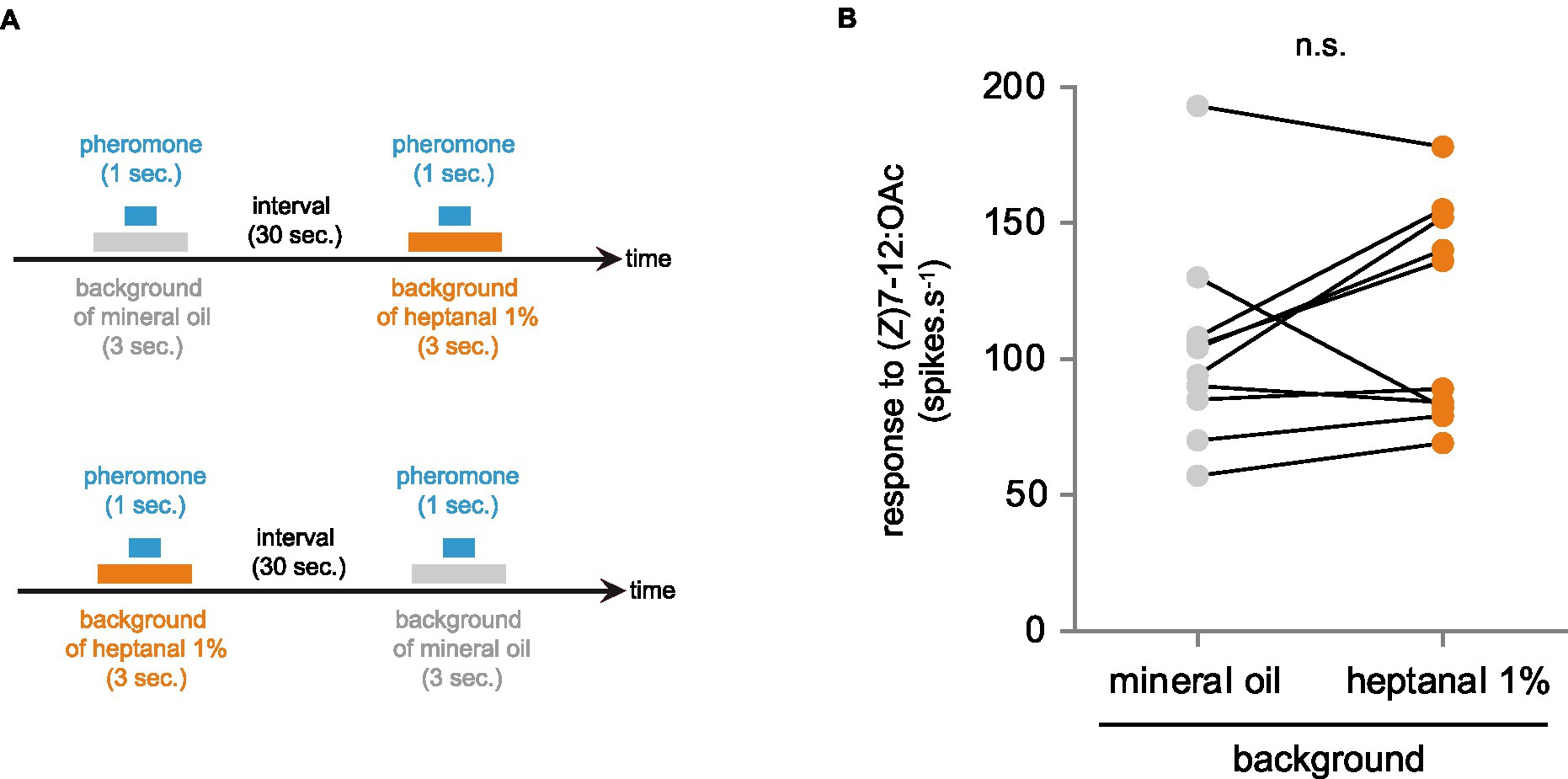
Figure 4. Effect of a background of heptanal on AipsOR3 function. (A) Protocol used to compare (Z)7-12:OAc responses of at1 neurons expressing AipsOR3 with or without a heptanal background. Neurons were alternatively stimulated with the neutral background (mineral oil) first or with the heptanal background first. (B) Action potential frequency of at1 neurons expressing AipsOR3 (n = 10) in response to (Z)7-12:OAc (10 μg in the glass vial) in a background of heptanal (1 mL of heptanal 1% in the glass vial). n.s., no significant difference between the two treatments (paired t-test, significance threshold set to 0.05).
Discussion
Although detection and central processing of sex pheromones and VPCs have been thought for long to be separated from each other, it is now clearly established that interactions between both classes of olfactory cues occur early in the peripheral olfactory system of male moths, usually resulting in a suppressive effect on the pheromone response (Den Otter et al., 1978; Pophof and van der Goes van Naters, 2002; Party et al., 2009). This is the case of pheromone-responsive ORNs of A. ipsilon males, for which a background of some VPCs – including heptanal – reduces the firing rate in response to a pulse of pheromone (Renou et al., 2015). The underlying mechanisms are not clearly understood, but another study conducted in A. ipsilon has demonstrated that some VPCs activate the pheromone ORNs by themselves (Rouyar et al., 2015), although it is not known if this phenomenon is direct or indirect. In this study, native A. ipsilon (Z)7-12:OAc-ORNs responded to heptanal starting at a 1% dilution with a spike frequency of around 60 spikes·s−1, reaching 125 spikes·s−1 at 10%. Interestingly, a previous report has already revealed that high doses of VPCs activate pheromone-responsive ORNs in a closely related species, A. segetum (Hansson et al., 1989). As it is generally accepted that ORNs express only one type of OR (but see exceptions to this, Couto et al., 2005; Fishilevich and Vosshall, 2005; Koutroumpa et al., 2014; Schultze et al., 2014; McLaughlin et al., 2021; Herre et al., 2022; Task et al., 2022), and considering that PRs are highly specific to pheromones, activation of pheromone ORNs by VPCs is puzzling. Would pheromone ORNs express more than one OR type? Would A. ipsilon PRs be less specific than previously thought?
To test these hypotheses, we worked at the level of the PR tuned to the pheromone compound (Z)7-12:OAc. First, we identified this receptor as AipsOR3. It belongs to the classical moth PR clade and is the orthologue of AsegOR4, also tuned to (Z)7-12:OAc in A. segetum (Zhang and Löfstedt, 2013). Interestingly, whereas some closely related PRs can respond to different pheromone compounds (Montagné et al., 2021), we have here a clear example of functional conservation. A large screening with 26 pheromone compounds revealed that AipsOR3, when expressed in Drosophila ORNs, was quite specific to (Z)7-12:OAc and did not respond to any of the four VPCs known to activate (Z)7-12:OAc ORNs in A. ipsilon. Moreover, a background of heptanal had no effect on the pheromone response of AipsOR3-expressing Drosophila ORNs, contrary to what has been observed in A. ipsilon (Z)7-12:OAc ORNs. Thus, heterologous expression of the receptor tuned to (Z)7-12:OAc does not recapitulate the functional properties of A. ipsilon (Z)7-12:OAc ORNs to VPCs.
The simpler interpretation of these results is that (Z)7-12:OAc ORNs express another OR responsible for the detection of VPCs. In fact, some cases of co-expression of several ORs within a single ORN have been revealed in different species. For instance, double in situ hybridization showed that at least two ORs are co-expressed in some Anopheles gambiae ORNs (Schultze et al., 2014). In D. melanogaster, some OR pairs are co-expressed in a single ORN and are both functional (Ebrahim et al., 2015; Lebreton et al., 2017). In the moth Ostrinia nubilalis, some pheromone-responsive ORNs co-express up to four different PR genes, and the broad tuning of these ORNs strongly suggest that at least several of these PRs are functional (Koutroumpa et al., 2014). Alternatively, non-OR membrane receptors could be responsible for VPC responses. In insects, olfactory detection is indeed performed not only via ORs but also via ionotropic receptors (IRs; Wicher and Miazzi, 2021). Whereas ORs and IRs are generally expressed in different ORN populations, a recent study in the mosquito Aedes aegypti has unexpectedly shown that IRs could be co-expressed with ORs in the same ORNs, and that both receptor types were functional (Herre et al., 2022). In Drosophila, Orco and the three IR co-receptors extensively overlap in expression (Task et al., 2022). It is plausible that A. ipsilon (Z)7-12:OAc ORNs express IRs in addition to AipsOR3, yet IRs are not the best candidates to explain VPC detection because several VPCs used in our study are known to be detected by ORs in moths (de Fouchier et al., 2017; Guo et al., 2021).
Although our results suggest that at least one OR is co-expressed with AipsOR3 in A. ipsilon ORNs, we cannot rule out other possibilities. First, our study may suffer from a protocol bias, as the stimulation system we used (Pasteur pipettes) differs from that used in Rouyar et al., 2015 (vials). Thus, the amount of heptanal reaching the antennae is difficult to compare. However, in our heptanal background experiment, we used exactly the same stimulation system as in Rouyar et al., 2015 and no response of AipsOR3-expressing Drosophila ORNs to 1% heptanal could be noticed at the onset on the background delivery. In addition, we found no effect of this background on the pheromone response of these ORNs, contrary to what has been observed for A. ipsilon (Z)7-12:OAc ORNs. Another explanation could be that AipsOR3 is in fact less specific in vivo than what we observed when expressed in Drosophila ORNs. Indeed, it is known that the sensillum environment can affect OR response profiles. For instance, odorant-binding proteins (OBPs) and/or chemosensory proteins (CSPs) are proposed to transport odorants within the sensilla lymph to the ORN membrane, with implications for ORN sensitivity and specificity (Große-Wilde et al., 2007; Forstner et al., 2009; Pelosi et al., 2014). Moreover, it has been shown that some OBPs can modulate olfactory physiology and the behavior that it drives (Xiao et al., 2019). Sensory Neuron Membrane Proteins (SNMPs) may also be part of the pheromone reception pathway (Jin et al., 2008). OBPs, CSPs and/or SNMPs, housed in Drosophila at1 sensilla, may strongly differ from those naturally occurring in A. ipsilon pheromone sensitive sensilla, and they could have affected the response of ORNs expressing AipsOR3. To test this hypothesis, we used another functional assay to isolate AipsOR3 from components of the sensillum environment (OBPs, CSPs, SNMPs), which consisted of in vitro expression in Xenopus oocytes coupled to two-electrode voltage clamp. In this system, odorants (pheromones and VPCs) were solubilized in water and carried to the OR via the use of dimethyl sulfoxide. Thus, VPCs should not encounter any solubilization nor transport issues from insect OBPs/CSPs/SNMPs to reach the OR, although the Xenopus oocyte solution used to solubilize odorants might probably not exactly recapitulate OBP function. By doing so, we confirmed that AipsOR3 responded strongly to (Z)7-12:OAc only, and no response was found for any of the four VPCs.
Taken all together, our results demonstrate that activation of (Z)7-12:OAc-sensitive ORNs by VPCs in A. ipsilon does not occur at the level of the AipsOR3 protein. A compelling explanation is that at least another receptor – which remains to be identified – is co-expressed in these neurons and participate in modulating pheromone responses in the presence of plant odors, but we cannot exclude other hypotheses. For instance, the exact function of OBPs/CSPs is still under study (Rihani et al., 2021) and these proteins may change the OR response threshold (Xiao et al., 2019), making the VPC responses not detectable in our expression systems. Apart OBPs/CSPs, other yet unknown elements of the A. ipsilon pheromone sensilla – not present in Drosophila at1 sensilla nor in the Xenopus oocyte aqueous environment – may also confer responses to heptanal in A. ipsilon pheromone ORNs. These could include sensillum shape, cuticle structure or lymph biochemistry, as recently reviewed by Schmidt and Benton, 2020. Alternatively, the response to heptanal in the native system may result from ORN interactions. Such functional interactions between ORNs grouped in the same sensillum have been evidenced earlier in D. melanogaster (Su et al., 2012; Zhang et al., 2019; Pannunzi and Nowotny, 2021). However, very careful examination of spike shape and size in hundreds single sensillum recordings on A. ipsilon pheromone ORNs revealed a complete recovering of spikes in response to either pheromone or heptanal and a homogenous spontaneous firing in the absence of stimulation. This makes highly improbable the contribution of another ORN type within the pheromone long trichoid sensilla, which are located on antennal branches. In addition, responses of non-pheromonal ORNs to heptanal and other plant volatiles were recorded from another category of olfactory sensilla, short hair sensilla, situated on the antennae stem. Conclusive experiments would come from a genome editing approach in A. ipsilon moths, in which AipsOR3 is knocked-out, and testing if the response to heptanal still occurs. Single cell transcriptomics from on A. ipsilon (Z)7-12:OAc-sensitive ORNs, although challenging, would definitively clarify the second OR identity.
Data availability statement
The original contributions presented in the study are included in the article/Supplementary material, further inquiries can be directed to the corresponding authors.
Author contributions
PV and RC conducted Drosophila single-sensillum recordings and analyzed data. ZL conducted Xenopus oocyte TEVC recordings and analyzed data. M-CF performed molecular cloning experiments. MR conceived experiments in odorized backgrounds and supervised single-sensillum recordings. NM and RC carried out bioinformatics and Drosophila genetics. EJ-J and NM conceived the study, supervised experiments, analyzed data, and wrote the manuscript. All authors contributed to the article and approved the submitted version.
Funding
This work has been funded by the French National Research Agency (ANR-11-BSV7-0026 and ANR-15-CE02-0010), the French Embassy in China (COMIX 2019-2020) and by a Chinese Scholarship Council (CSC) grant to ZL.
Acknowledgments
We thank Isabelle Touton and Pascal Roskam for A. ipsilon rearing, Philippe Touton and Fabien Tissier for Drosophila medium preparation, and Arthur Comte for ANOVA analyses.
Conflict of interest
The authors declare that the research was conducted in the absence of any commercial or financial relationships that could be construed as a potential conflict of interest.
Publisher’s note
All claims expressed in this article are solely those of the authors and do not necessarily represent those of their affiliated organizations, or those of the publisher, the editors and the reviewers. Any product that may be evaluated in this article, or claim that may be made by its manufacturer, is not guaranteed or endorsed by the publisher.
Supplementary material
The Supplementary material for this article can be found online at: https://www.frontiersin.org/articles/10.3389/fevo.2022.1035252/full#supplementary-material
References
Bastin-Héline, L., de Fouchier, A., Cao, S., Koutroumpa, F., Caballero-Vidal, G., Robakiewicz, S., et al. (2019). A novel lineage of candidate pheromone receptors for sex communication in moths. eLife 8:e49826. doi: 10.7554/eLife.49826
Conchou, L., Lucas, P., Deisig, N., Demondion, E., and Renou, M. (2021). Effects of multi-component backgrounds of volatile plant compounds on moth pheromone perception. Insects 12:409. doi: 10.3390/insects12050409
Couto, A., Alenius, M., and Dickson, B. J. (2005). Molecular, anatomical, and functional organization of the drosophila olfactory system. Curr. Biol. 15, 1535–1547. doi: 10.1016/j.cub.2005.07.034
de Fouchier, A., Sun, X., Monsempes, C., Mirabeau, O., Jacquin-Joly, E., and Montagné, N. (2015). Evolution of two receptors detecting the same pheromone compound in crop pest moths of the genus Spodoptera. Front. Ecol. Evol. 3:95. doi: 10.3389/fevo.2015.00095
de Fouchier, A., Walker, W. B. 3rd, Montagne, N., Steiner, C., Binyameen, M., Schlyter, F., et al. (2017). Functional evolution of lepidoptera olfactory receptors revealed by deorphanization of a moth repertoire. Nat. Commun. 8:15709. doi: 10.1038/ncomms15709
Deisig, N., Dupuy, F., Anton, S., and Renou, M. (2014). Responses to pheromones in a complex odor world: sensory processing and behavior. Insects 5, 399–422. doi: 10.3390/insects5020399
Den Otter, C., Schuil, H., and Oosten, A. S. V. (1978). Reception of host-plant odours and female sex pheromone in Adoxophyes orana (lepidoptera: Tortricidae): electrophysiology and morphology. Entomol. Exp. Appl. 24, 570–578. doi: 10.1111/j.1570-7458.1978.tb02818.x
Deng, J. Y., Wei, H. Y., Huang, Y. P., and Du, J. W. (2004). Enhancement of attraction to sex pheromones of Spodoptera exigua by volatile compounds produced by host plants. J. Chem. Ecol. 30, 2037–2045. doi: 10.1023/b:joec.0000045593.62422.73
Dupuy, F., Rouyar, A., Deisig, N., Bourgeois, T., Limousin, D., Wycke, M. A., et al. (2017). A background of a volatile plant compound alters neural and behavioral responses to the sex pheromone blend in a moth. Front. Physiol. 8:79. doi: 10.3389/fphys.2017.00079
Ebrahim, S. A., Dweck, H. K., Stokl, J., Hofferberth, J. E., Trona, F., Weniger, K., et al. (2015). Drosophila avoids Parasitoids by sensing their Semiochemicals via a dedicated olfactory circuit. PLoS Biol. 13:e1002318. doi: 10.1371/journal.pbio.1002318
Fishilevich, E., and Vosshall, L. B. (2005). Genetic and functional subdivision of the drosophila antennal lobe. Curr. Biol. 15, 1548–1553. doi: 10.1016/j.cub.2005.07.066
Fleischer, J., and Krieger, J. (2018). Insect pheromone receptors – key elements in sensing intraspecific chemical signals. Front. Cell. Neurosci. 12:425. doi: 10.3389/fncel.2018.00425
Forstner, M., Breer, H., and Krieger, J. (2009). A receptor and binding protein interplay in the detection of a distinct pheromone component in the silkmoth Antheraea polyphemus. Int. J. Biol. Sci. 5, 745–757. doi: 10.7150/ijbs.5.745
Fu, L., Niu, B., Zhu, Z., Wu, S., and Li, W. (2012). CD-HIT: accelerated for clustering the next-generation sequencing data. Bioinformatics 28, 3150–3152. doi: 10.1093/bioinformatics/bts565
Gemeno, C., and Haynes, K. F. (1998). Chemical and behavioral evidence for a third pheromone component in a North American population of the black cutworm moth, Agrotis ipsilon. J. Chem. Ecol. 24, 999–1011. doi: 10.1023/A:1022398318465
Große-Wilde, E., Gohl, T., Bouché, E., Breer, H., and Krieger, J. (2007). Candidate pheromone receptors provide the basis for the response of distinct antennal neurons to pheromonal compounds. Eur. J. Neurosci. 25, 2364–2373. doi: 10.1111/j.1460-9568.2007.05512.x
Gu, S. H., Sun, L., Yang, R. N., Wu, K. M., Guo, Y. Y., Li, X. C., et al. (2014). Molecular characterization and differential expression of olfactory genes in the antennae of the black cutworm moth Agrotis ipsilon. PLoS One 9:e103420. doi: 10.1371/journal.pone.0103420
Guindon, S., Dufayard, J. F., Lefort, V., Anisimova, M., Hordijk, W., and Gascuel, O. (2010). New algorithms and methods to estimate maximum-likelihood phylogenies: assessing the performance of PhyML 3.0. Syst. Biol. 59, 307–321. doi: 10.1093/sysbio/syq010
Guo, M., Du, L., Chen, Q., Feng, Y., Zhang, J., Zhang, X., et al. (2021). Odorant receptors for detecting flowering plant cues are functionally conserved across moths and butterflies. Mol. Biol. Evol. 38, 1413–1427. doi: 10.1093/molbev/msaa300
Hansson, B. S., van der Pers, J. N. C., and Löfqvist, J. (1989). Comparison of male and female olfactory cell response to pheromone compounds and plant volatiles in the turnip moth, Agrotis segetum. Physiol. Entomol. 14, 147–155. doi: 10.1111/j.1365-3032.1989.tb00946.x
Herre, M., Goldman, O. V., Lu, T. C., Caballero-Vidal, G., Qi, Y., Gilbert, Z. N., et al. (2022). Non-canonical odor coding in the mosquito. Cells 185, 3104–3123e3128. doi: 10.1016/j.cell.2022.07.024
Hillier, N. K., and Vickers, N. J. (2010). Mixture interactions in moth olfactory physiology: examining the effects of odorant mixture, concentration, distal stimulation, and antennal nerve transection on sensillar responses. Chem. Senses 36, 93–108. doi: 10.1093/chemse/bjq102
Hoffmann, A., Bourgeois, T., Munoz, A., Anton, S., Gevar, J., Dacher, M., et al. (2020). A plant volatile alters the perception of sex pheromone blend ratios in a moth. J. Comp. Physiol. A Neuroethol. Sens. Neural Behav. Physiol. 206, 553–570. doi: 10.1007/s00359-020-01420-y
Jin, X., Ha, T. S., and Smith, D. P. (2008). SNMP is a signaling component required for pheromone sensitivity in drosophila. Proc. Natl. Acad. Sci. U. S. A. 105, 10996–11001. doi: 10.1073/pnas.0803309105
Kaissling, K. E. (2014). “Pheromone reception in insects: the example of silk moths” in Neurobiology of Chemical Communication. ed. C. Mucignat-Caretta (Boca Raton, FL: CRC Press/Taylor & Francis)
Koutroumpa, F. A., Kárpáti, Z., Monsempes, C., Hill, S. R., Hansson, B. S., Jacquin-Joly, E., et al. (2014). Shifts in sensory neuron identity parallel differences in pheromone preference in the European corn borer. Front. Ecol. Evol. 2:65. doi: 10.3389/fevo.2014.00065
Krieger, J., Grosse-Wilde, E., Gohl, T., and Breer, H. (2005). Candidate pheromone receptors of the silkmoth Bombyx mori. Eur. J. Neurosci. 21, 2167–2176. doi: 10.1111/j.1460-9568.2005.04058.x
Krieger, J., Grosse-Wilde, E., Gohl, T., Dewer, Y. M., Raming, K., and Breer, H. (2004). Genes encoding candidate pheromone receptors in a moth (Heliothis virescens). Proc. Natl. Acad. Sci. U. S. A. 101, 11845–11850. doi: 10.1073/pnas.0403052101
Kurtovic, A., Widmer, A., and Dickson, B. J. (2007). A single class of olfactory neurons mediates behavioural responses to a Drosophila sex pheromone. Nature 446, 542–546. doi: 10.1038/nature05672
Landolt, P. J., and Phillips, T. W. (1997). Host plant influences on sex pheromone behavior of phytophagous insects. Annu. Rev. Entomol. 42, 371–391. doi: 10.1146/annurev.ento.42.1.371
Larsson, M. C., Domingos, A. I., Jones, W. D., Chiappe, M. E., Amrein, H., and Vosshall, L. B. (2004). Or83b encodes a broadly expressed odorant receptor essential for Drosophila olfaction. Neuron 43, 703–714. doi: 10.1016/j.neuron.2004.08.019
Lebreton, S., Borrero-Echeverry, F., Gonzalez, F., Solum, M., Wallin, E. A., Hedenstrom, E., et al. (2017). A drosophila female pheromone elicits species-specific long-range attraction via an olfactory channel with dual specificity for sex and food. BMC Biol. 15:88. doi: 10.1186/s12915-017-0427-x
Light, D. M., Flath, R. A., Buttery, R. G., Zalom, F. G., Rice, R. E., Dickens, J. C., et al. (1993). Host-plant green-leaf volatiles synergize the synthetic sex pheromones of the corn earworm and codling moth (lepidoptera). Chemoecology 4, 145–152.
Masse, N. Y., Turner, G. C., and Jefferis, G. S. (2009). Olfactory information processing in drosophila. Curr. Biol. 19, R700–R713. doi: 10.1016/j.cub.2009.06.026
McLaughlin, C. N., Brbic, M., Xie, Q., Li, T., Horns, F., Kolluru, S. S., et al. (2021). Single-cell transcriptomes of developing and adult olfactory receptor neurons in drosophila. eLife 10:e63856. doi: 10.7554/eLife.63856
Meslin, C., Mainet, P., Montagné, N., Robin, S., Legeai, F., Bretaudeau, A., et al. (2022). Spodoptera littoralis genome mining brings insights on the dynamic of expansion of gustatory receptors in polyphagous noctuidae. G3 Genes|Genomes|Genetics. 12:jkac131. doi: 10.1093/g3journal/jkac131
Montagné, N., Wanner, K., and Jacquin-Joly, E. (2021). “Olfactory genomics within the lepidoptera,” in Insect Pheromone Biochemistry and Molecular Biology (Second Edition). eds. Gary Blomquist and Richard Vogt (Cambridge: Academic Press), 469–505.
Ochieng, S. A., Park, K. C., and Baker, T. C. (2002). Host plant volatiles synergize responses of sex pheromone-specific olfactory receptor neurons in male Helicoverpa zea. J. Comp. Physiol. A Neuroethol. Sens. Neural Behav. Physiol. 188, 325–333. doi: 10.1007/s00359-002-0308-8
Pannunzi, M., and Nowotny, T. (2021). Non-synaptic interactions between olfactory receptor neurons, a possible key feature of odor processing in flies. PLoS Comput. Biol. 17:e1009583. doi: 10.1371/journal.pcbi.1009583
Party, V., Hanot, C., Said, I., Rochat, D., and Renou, M. (2009). Plant terpenes affect intensity and temporal parameters of pheromone detection in a moth. Chem. Senses 34, 763–774. doi: 10.1093/chemse/bjp060
Pelosi, P., Iovinella, I., Felicioli, A., and Dani, F. R. (2014). Soluble proteins of chemical communication: an overview across arthropods. Front. Physiol. 5:320. doi: 10.3389/fphys.2014.00320
Pophof, B., and van der Goes van Naters, W. (2002). Activation and inhibition of the transduction process in Silkmoth olfactory receptor neurons. Chem. Senses 27, 435–443. doi: 10.1093/chemse/27.5.435
Pregitzer, P., Schubert, M., Breer, H., Hansson, B. S., Sachse, S., and Krieger, J. (2012). Plant odorants interfere with detection of sex pheromone signals by male Heliothis virescens. Front. Cell. Neurosci. 6:42. doi: 10.3389/fncel.2012.00042
Price, M. N., Dehal, P. S., and Arkin, A. P. (2009). FastTree: computing large minimum evolution trees with profiles instead of a distance matrix. Mol. Biol. Evol. 26, 1641–1650. doi: 10.1093/molbev/msp077
Renou, M., Party, V., Rouyar, A., and Anton, S. (2015). Olfactory signal coding in an odor background. Biosystems 136, 35–45. doi: 10.1016/j.biosystems.2015.06.001
Rihani, K., Ferveur, J. F., and Briand, L. (2021). The 40-year mystery of insect odorant-binding proteins. Biomol. Ther. 11:509. doi: 10.3390/biom11040509
Rouyar, A., Deisig, N., Dupuy, F., Limousin, D., Wycke, M. A., Renou, M., et al. (2015). Unexpected plant odor responses in a moth pheromone system. Front. Physiol. 6:148. doi: 10.3389/fphys.2015.00148
RStudio Team (2022). RStudio: Integrated development for R. RStudio, PBC, Boston, MA. Available at: http://www.rstudio.com/
Schmidt, H. R., and Benton, R. (2020). Molecular mechanisms of olfactory detection in insects: beyond receptors. Open Biol. 10:200252. doi: 10.1098/rsob.200252
Schmidt-Busser, D., von Arx, M., and Guerin, P. M. (2009). Host plant volatiles serve to increase the response of male European grape berry moths, Eupoecilia ambiguella, to their sex pheromone. J. Comp. Physiol. A Neuroethol. Sens. Neural Behav. Physiol. 195, 853–864. doi: 10.1007/s00359-009-0464-1
Schultze, A., Breer, H., and Krieger, J. (2014). The blunt trichoid sensillum of female mosquitoes, Anopheles gambiae: odorant binding protein and receptor types. Int. J. Biol. Sci. 10, 426–437. doi: 10.7150/ijbs.8754
Sievers, F., and Higgins, D. G. (2018). Clustal omega for making accurate alignments of many protein sequences. Protein Sci. 27, 135–145. doi: 10.1002/pro.3290
Slater, G. S., and Birney, E. (2005). Automated generation of heuristics for biological sequence comparison. BMC Bioinf. 6:31. doi: 10.1186/1471-2105-6-31
Su, C. Y., Menuz, K., Reisert, J., and Carlson, J. R. (2012). Non-synaptic inhibition between grouped neurons in an olfactory circuit. Nature 492, 66–71. doi: 10.1038/nature11712
Task, D., Lin, C. C., Vulpe, A., Afify, A., Ballou, S., Brbic, M., et al. (2022). Chemoreceptor co-expression in Drosophila melanogaster olfactory neurons. eLife 11:e72599. doi: 10.7554/eLife.72599
Touhara, K., and Vosshall, L. B. (2009). Sensing odorants and pheromones with chemosensory receptors. Annu. Rev. Physiol. 71, 307–332. doi: 10.1146/annurev.physiol.010908.163209
Trapnell, C., Williams, B. A., Pertea, G., Mortazavi, A., Kwan, G., van Baren, M. J., et al. (2010). Transcript assembly and quantification by RNA-Seq reveals unannotated transcripts and isoform switching during cell differentiation. Nat. Biotechnol. 28, 511–515. doi: 10.1038/nbt.1621
Trona, F., Anfora, G., Balkenius, A., Bengtsson, M., Tasin, M., Knight, A., et al. (2013). Neural coding merges sex and habitat chemosensory signals in an insect herbivore. Proc. Biol. Sci. 280:20130267. doi: 10.1098/rspb.2013.0267
Varela, N., Avilla, J., Gemeno, C., and Anton, S. (2011). Ordinary glomeruli in the antennal lobe of male and female tortricid moth Grapholita molesta (Busck) (lepidoptera: Tortricidae) process sex pheromone and host-plant volatiles. J. Exp. Biol. 214, 637–645. doi: 10.1242/jeb.047316
Wang, Y., Fang, G., Chen, X., Cao, Y., Wu, N., Cui, Q., et al. (2021). The genome of the black cutworm Agrotis ipsilon. Insect Biochem. Mol. Biol. 139:103665. doi: 10.1016/j.ibmb.2021.103665
Wicher, D., and Miazzi, F. (2021). Functional properties of insect olfactory receptors: ionotropic receptors and odorant receptors. Cell Tissue Res. 383, 7–19. doi: 10.1007/s00441-020-03363-x
Xiao, S., Sun, J. S., and Carlson, J. R. (2019). Robust olfactory responses in the absence of odorant binding proteins. eLife 8:e51040. doi: 10.7554/eLife.51040
Zhang, D. D., and Löfstedt, C. (2013). Functional evolution of a multigene family: orthologous and paralogous pheromone receptor genes in the turnip moth, Agrotis segetum. PLoS One 8:e77345. doi: 10.1371/journal.pone.0077345
Zhang, D. D., and Löfstedt, C. (2015). Moth pheromone receptors: gene sequences, function, and evolution. Front. Ecol. Evol. 3:105. doi: 10.3389/fevo.2015.00105
Zhang, D. D., Wang, H. L., Schultze, A., Fross, H., Francke, W., Krieger, J., et al. (2016). Receptor for detection of a Type II sex pheromone in the winter moth Operophtera brumata. Sci Rep. 6:18576. doi: 10.1038/srep18576
Keywords: insect, olfaction, odorant receptor, sex pheromone, volatile organic compound
Citation: Vandroux P, Li Z, Capoduro R, François M-C, Renou M, Montagné N and Jacquin-Joly E (2022) Activation of pheromone-sensitive olfactory neurons by plant volatiles in the moth Agrotis ipsilon does not occur at the level of the pheromone receptor protein. Front. Ecol. Evol. 10:1035252. doi: 10.3389/fevo.2022.1035252
Edited by:
Erwan Poivet, Institut Pasteur, FranceReviewed by:
Jing-Jiang Zhou, Breeding Base of State Key laboratory for Green Pesticide and Agricultural Bioengineering, ChinaCarolina E. Reisenman, University of California, United States
Copyright © 2022 Vandroux, Li, Capoduro, François, Renou, Montagné and Jacquin-Joly. This is an open-access article distributed under the terms of the Creative Commons Attribution License (CC BY). The use, distribution or reproduction in other forums is permitted, provided the original author(s) and the copyright owner(s) are credited and that the original publication in this journal is cited, in accordance with accepted academic practice. No use, distribution or reproduction is permitted which does not comply with these terms.
*Correspondence: Nicolas Montagné, bmljb2xhcy5tb250YWduZUBzb3Jib25uZS11bml2ZXJzaXRlLmZy Emmanuelle Jacquin-JolyZW1tYW51ZWxsZS5qb2x5QGlucmFlLmZy
†These authors have contributed equally to this work and share first authorship
‡These authors have contributed equally to this work and share last authorship