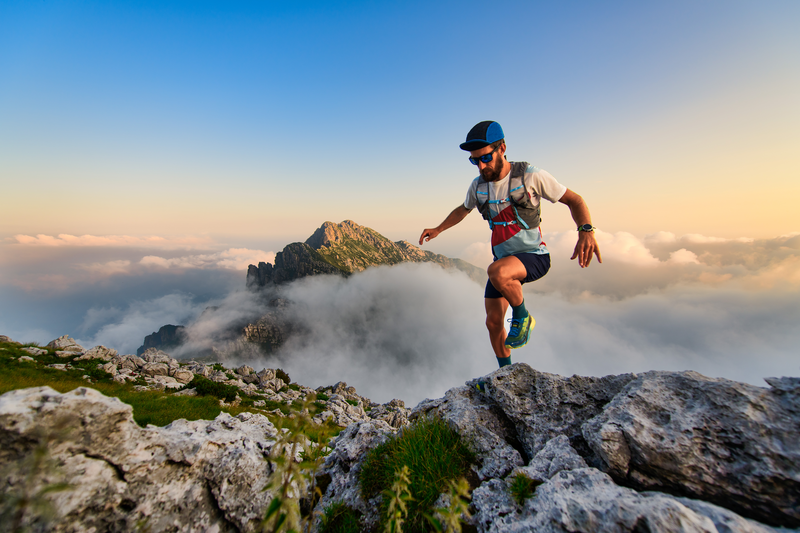
95% of researchers rate our articles as excellent or good
Learn more about the work of our research integrity team to safeguard the quality of each article we publish.
Find out more
EDITORIAL article
Front. Ecol. Evol. , 04 October 2022
Sec. Behavioral and Evolutionary Ecology
Volume 10 - 2022 | https://doi.org/10.3389/fevo.2022.1029958
This article is part of the Research Topic Optimal Bird Migration: Implications for Navigation, Physiology, and Stopover Ecology View all 8 articles
Editorial on the Research Topic
Optimal bird migration: Implications for navigation, physiology, and stopover ecology
During migration, birds cross considerable geographical barriers, experience changing weather conditions, and face unfamiliar environments with unpredictable resource availability and predation pressure. Consequently, most aspects of long-distance bird migration are expected to be under optimization pressure, given the high costs resulting from non-optimized behavior (Alerstam and Lindström, 1990). In addition to evolutionary adaptations and innate programs, migration requires behavioral flexibility (Åkesson and Helm, 2020), where individuals need to make decisions about landing, departing, flight directions, altitudes, general route, stopover choices, and predator and pathogen avoidance (Klinner et al., 2020; Sabal et al., 2021). Although some aspects of optimal migration have been criticized (e.g., Chernetsov, 2012), the theory serves as an essential framework for understanding the ecology and evolution of bird migration.
Naturally, a large set of tools and methods is required for addressing the many aspects of migration. Powerful tools such as radar tracking, radio tagging, studies of flight mechanics and energetic in wind tunnels, light-level geolocators, ringing data analysis, stable isotopes, and DNA sampling are widely used to discover patterns of migration (Kelly and Finch, 1998; Wink, 2006; Fiedler, 2009; Bauer et al., 2019). Using these tools, the articles in this topic cover various aspects of migration and stopover ecology, providing interesting insights about mass movements, spatial distribution, fuel deposition rate, the timing of migration, and social interactions during migration.
In recent years, Palaearctic long-distance migrants have dramatically shortened their wintering period in Africa (Lawrence et al., 2022). Climate change also affects the duration and timing of the breeding season (Hällfors et al., 2020). To quantify the overall advantages of migration, Snell and Thorup developed a computer simulation model based on temperature and NDVI maps (an indication for vegetation-rich areas). The resulted theoretical bird migration tracks indicate a distinct thermal benefit from crossing the Sahara, and that the routes and utilization of green spots are species-specific. Changes in vegetation growth and temperature profile owing to global change are also suggested as drivers of advanced spring arrival in central Israel, as shown by long-term ringing data (Aharon-Rotman et al.).
The importance of green vegetation at stopover sites, a proxy for food abundance, is directly shown by Schekler et al. who compared autumn vs. spring bird densities departing from stopover in Israel using radar data. In autumn, bird densities were low in the southern, extremely arid parts of the country. In spring, densities were the highest in the same but much greener region following winter rains. This pattern suggests that birds on the move can quickly respond to dramatic, short-term changes in productivity.
Time minimizing is a vital migration strategy in many species. Competition for territories may favor early arrival at breeding grounds in spring (Kokko, 1999). Therefore, compared with autumn, migrating birds usually travel faster in spring when rushing for their breeding sites (Nilsson et al., 2013; Lisovski et al., 2021). However, using ATLAS telemetry to track Red-backed Shrikes staging in Northern Israel, Zinßmeister et al. found support for the time-minimizing strategy during autumn. While the mechanism underlying adaptive timing remains unclear, prolonged staging at common stopover sites close to the breeding grounds may allow better timing of arrival in respect to climate and food conditions. Such behavior may confound any association between departure from wintering grounds and arrival at breeding grounds, as is the case of spring migrating Bar-tailed Godwits on their way from New Zealand to Alaska (Conklin et al., 2021). The American Golden Plover Spring migration strategies described by Lamarre et al. in this topic show an interesting similarity to the Bar-tailed Godwit's strategy. Departing from their wintering grounds in South America, American Golden Plover arrive at a common, primary stopover site in the mid-west U.S. grasslands between early April and mid-May. Interestingly, departure from the stopover site did not correlate with the distance to the breeding site, but rather with the local snow-free date at the breeding site. Furthermore, departure from the wintering site did not correlate with the snow-free date. Prolonged stopover “closer to home” may allow improved arrival timing at the breeding site to match optimal environmental conditions.
Despite their important role in stopover ecology, evidence of inter- and intra-specific interactions are scant in migration research. Competition over food resources negatively affects the body condition and fat deposition rate (FDR) at stopover sites (Shochat et al., 2002; de Zwaan et al., 2022). Using blood triglyceride concentrations as an indicator of body condition, Cohen et al. demonstrate density-dependent refueling in migratory passerines staging within urban forest patches along the northern coast of the Gulf of Mexico. These patterns were significant despite overall low bird densities, suggesting even lower FDR in peak migration season when densities are high. Strong competition for resources, which leads to low FDR, may be highly associated with urban landscapes, where birds are forced to crowd in small fragments of suitable habitat.
On the positive aspect of social dynamics, interactions among individuals may serve as important cues for departure. Deakin et al. provide a rare opportunity to learn how biased sex ratio affects Zugunruhe and several other traits (e.g., body mass and molt) in male Yellow-rumped Warblers in Canada. The authors experimentally manipulated photoperiod and sex ratio in the aviary before spring migration. They expected birds inhabiting a “male-biased environment” to accelerate migration restlessness and departure timing, owing to male-male competition over breeding territories. Contrary to the predictions however, males from the female-biased treatment displayed migratory restlessness a week before the males from the male-biased treatment. As males in this species display protandry (earlier migration of males compared with females), a female-rich environment may have served as a cue for the focal males for being late, as in their perception—most other males have “departed.”
Decision-making by migratory birds should be optimized by natural selection but is constrained by metabolic capacity, navigation mechanisms, and endogenous programs that underlie migratory behavior. Yet, the information on conditions, and recourses available for the birds, and the time to gather such information, may be limited when moving through unknown landscapes (Shochat et al., 2002). Though some of these crucial topics are not covered here, they are not any less important. In the age of ongoing and accelerating climate change and habitat degradation across the globe, it is crucial to understand the complex interplay among physiological, ecological, and behavioral mechanisms allowing birds to perform successful migrations. An improved understanding can guide mitigation programs and help conserving migratory birds that presently experience alarming population declines in almost all regions of the world (Bairlein, 2016; Rosenberg et al., 2019; Burns et al., 2021).
ES wrote the manuscript. CN, SL, and NC provided comments, additional references, and edited the document. All authors contributed to the article and approved the submitted version.
The authors declare that the research was conducted in the absence of any commercial or financial relationships that could be construed as a potential conflict of interest.
All claims expressed in this article are solely those of the authors and do not necessarily represent those of their affiliated organizations, or those of the publisher, the editors and the reviewers. Any product that may be evaluated in this article, or claim that may be made by its manufacturer, is not guaranteed or endorsed by the publisher.
Åkesson, S., and Helm, B. (2020). Endogenous programs and flexibility in bird migration. Front. Ecol. Evol. 8, 78. doi: 10.3389/fevo.2020.00078
Alerstam, T., and Lindström, Å. (1990). “Optimal bird migration: the relative importance of time, energy, and safety,” in Bird Migration (Berlin, Heidelberg: Springer), 331–351. doi: 10.1007/978-3-642-74542-3_22
Bairlein, F. (2016). Migratory birds under threat. Science 354, 547–548. doi: 10.1126/science.aah6647
Bauer, S., Shamoun-Baranes, J., Nilsson, C., Farnsworth, A., Kelly, J. F., Reynolds, D. R., et al. (2019). The grand challenges of migration ecology that radar aeroecology can help answer. Ecography 42, 861–875. doi: 10.1111/ecog.04083
Burns, F., Eaton, M. A., Burfield, I. J., Klvanová, A., Šilarová, E., Staneva, A., et al. (2021). Abundance decline in the avifauna of the European Union reveals cross-continental similarities in biodiversity change. Ecol. Evol. 11, 16647–16660. doi: 10.1002/ece3.8282
Chernetsov, N. (2012). “Optimal migration theory,” in Passerine Migration (Berlin: Springer), 59–73. doi: 10.1007/978-3-642-29020-6_4
Conklin, J. R., Lisovski, S., and Battley, P. F. (2021). Advancement in long-distance bird migration through individual plasticity in departure. Nat. Commun. 12, 1–9. doi: 10.1038/s41467-021-25022-7
de Zwaan, D. R., Huang, A., McCallum, Q., Owen, K., Lamont, M., and Easton, W. (2022). Mass gain and stopover dynamics among migrating songbirds are linked to seasonal, environmental, and life-history effects. Ornithology 139, ukac027. doi: 10.1093/ornithology/ukac027
Fiedler, W. (2009). New technologies for monitoring bird migration and behaviour. Ringing Migr. 24, 175–179. doi: 10.1080/03078698.2009.9674389
Hällfors, M. H., Antão, L. H., Itter, M., Lehikoinen, A., Lindholm, T., Roslin, T., et al. (2020). Shifts in timing and duration of breeding for 73 boreal bird species over four decades. Proc. Natl. Acad. Sci. USA 117, 18557–18565. doi: 10.1073/pnas.1913579117
Kelly, J. F., and Finch, D. M. (1998). Tracking migrant songbirds with stable isotopes. Trends Ecol. Evol. 13, 48–49. doi: 10.1016/S0169-5347(97)01299-8
Klinner, T., Buddemeier, J., Bairlein, F., and Schmaljohann, H. (2020). Decision-making in migratory birds at stopover: an interplay of energy stores and feeding conditions. Behav. Ecol. Sociobiol. 74, 10.
Kokko, H. (1999). Competition for early arrival in migratory birds. J. Anim. Ecol. 68, 940–950. doi: 10.1046/j.1365-2656.1999.00343.x
Lawrence, K. B., Barlow, C. R., Bensusan, K., Perez, C., and Willis, S. G. (2022). Phenological trends in the pre- and post-breeding migration of long-distance migratory birds. Global Change Biol. 28, 375–389. doi: 10.1111/gcb.15916
Lisovski, S., Neumann, R., Albrecht, T., Munclinger, P., Ahola, M. P., and Bauer, S. (2021), The Indo-European flyway: opportunities constraints reflected by Common Rosefinches breeding across Europe. J. Biogeogr. 48 1255–1266. doi: 10.1111/jbi.14085.
Nilsson, C., Klaassen, R. H., and Alerstam, T. (2013). Differences in speed and duration of bird migration between spring and autumn. Am. Nat. 181, 837–845. doi: 10.1086/670335
Rosenberg, K. V., Dokter, A. M., Blancher, P. J., Dauer, J. R., Smith, A. C., Smith, P. A., et al. (2019). Decline of the North American avifauna. Science 366, 120–124. doi: 10.1126/science.aaw1313
Sabal, M. C., Boyce, M. S., Charpentier, C. L., Furey, N. B., Luhring, T. M., Martin, H. W., et al. (2021). Predation landscapes influence migratory prey ecology and evolution. Trends Ecol. Evol. 36, 737–749. doi: 10.1016/j.tree.2021.04.010
Shochat, E., Abramsky, Z., Pinshow, B., and Whitehouse, M. E. A. (2002). Density-dependent habitat selection in migratory passerines during stopover: what causes the deviation from IFD? Evol. Ecol. 16, 469–488. doi: 10.1023/A:1020851801732
Keywords: ecophysiology, birds (Aves), migration, stopover, flight
Citation: Shochat E, Nilsson C, Lisovski S and Chernetsov N (2022) Editorial: Optimal bird migration: Implications for navigation, physiology, and stopover ecology. Front. Ecol. Evol. 10:1029958. doi: 10.3389/fevo.2022.1029958
Received: 28 August 2022; Accepted: 21 September 2022;
Published: 04 October 2022.
Edited and reviewed by: Jordi Figuerola, Doñana Biological Station, Spanish National Research Council (CSIC), Spain
Copyright © 2022 Shochat, Nilsson, Lisovski and Chernetsov. This is an open-access article distributed under the terms of the Creative Commons Attribution License (CC BY). The use, distribution or reproduction in other forums is permitted, provided the original author(s) and the copyright owner(s) are credited and that the original publication in this journal is cited, in accordance with accepted academic practice. No use, distribution or reproduction is permitted which does not comply with these terms.
*Correspondence: Eyal Shochat, ZXlhbC5zaG9jaGF0MUBnbWFpbC5jb20=
†ORCID: Eyal Shochat orcid.org/0000-0003-0991-9366
Cecilia Nilsson orcid.org/0000-0001-8957-4411
Simeon Lisovski orcid.org/0000-0002-6399-0035
Nikita Chernetsov orcid.org/0000-0001-7299-6829
Disclaimer: All claims expressed in this article are solely those of the authors and do not necessarily represent those of their affiliated organizations, or those of the publisher, the editors and the reviewers. Any product that may be evaluated in this article or claim that may be made by its manufacturer is not guaranteed or endorsed by the publisher.
Research integrity at Frontiers
Learn more about the work of our research integrity team to safeguard the quality of each article we publish.