- 1School of Marine and Atmospheric Sciences, Stony Brook University, Stony Brook, NY, United States
- 2Biological Sciences, San Jose State University, San Jose, CA, United States
- 3Department of Ecology and Evolution, Stony Brook University, Stony Brook, NY, United States
- 4Ocean Sciences Department, University of California, Santa Cruz, Santa Cruz, CA, United States
- 5Environmental Research Division, National Marine Fisheries Service, Southwest Fisheries Science Center, Monterey, CA, United States
- 6Marine Mammal Institute and Department of Fisheries, Wildlife, and Conservation Sciences, Oregon State University, Newport, OR, United States
- 7MARBEC Research Unit (IRD, IFREMER, CNRS, Univ. Montpellier), Sète, France
- 8College of Agricultural Sciences, Oregon State University, Corvallis, OR, United States
- 9Long Marine Laboratory Department of Ecology and Evolutionary Biology, University of California, Santa Cruz, Santa Cruz, CA, United States
Understanding the at-sea movements of wide-ranging seabird species throughout their annual cycle is essential for their conservation and management. Habitat use and resource partitioning of Laysan (Phoebastria immutabilis) and black-footed (Phoebastria nigripes) albatross are well-described during the breeding period but are less understood during the post-breeding period, which represents ~40% of their annual cycle. Resource partitioning may be reduced during post-breeding, when birds are not constrained to return to the nest site regularly and can disperse to reduce competitive pressure. We assessed the degree of spatial segregation in the post-breeding distributions of Laysan (n = 82) and black-footed albatrosses (n = 61) using geolocator tags between 2008 and 2012 from two large breeding colonies in the Northwestern Hawaiian Islands, Midway Atoll, and Tern Island. We characterized the species-and colony-specific foraging and focal distributions (represented by the 95 and 50th density contours, respectively) and quantified segregation in at-sea habitat use between species and colonies. Laysan and black-footed albatross showed consistent and significant at-sea segregation in focal areas across colonies, indicating that resource partitioning persists during post-breeding. Within breeding colonies, segregation of foraging areas between the two species was more evident for birds breeding at Tern Island. Spatial segregation decreased as the post-breeding season progressed, when spatial distributions of both species became more dispersed. In contrast to studies conducted on breeding Laysan and black-footed albatross, we found that sea surface temperature distinguished post-breeding habitats of black-footed albatrosses between colonies, with black-footed albatrosses from Midway Atoll occurring in cooler waters (3.6°C cooler on average). Our results reveal marked at-sea segregation between Laysan and black-footed albatross breeding at two colonies during a critical but understudied phase in their annual cycle. The observed variation in species-environment relationships underscores the importance of sampling multiple colonies and temporal periods to more thoroughly understand the spatial distributions of pelagic seabirds.
Introduction
Under the principle of competitive exclusion, spatial and temporal resource partitioning is a key mechanism to reduce competitive pressure for shared resources in sympatric species (Schoener, 1974). For colonial breeding seabirds that function as central place foragers when breeding, competition for resources near colonies may influence habitat use, prey selection, energetic expenditure, and ultimately population sizes (Furness and Birkhead, 1984; Lewis et al., 2001; Wakefield et al., 2013; Oppel et al., 2015; Jessopp et al., 2020; Weber et al., 2021). Habitat segregation in seabirds is well documented and occurs not just between sympatrically breeding species (Kappes et al., 2010; Young et al., 2010, 2015; Linnebjerg et al., 2013; Robertson et al., 2014; Barger et al., 2016), but also between age classes (Pettex et al., 2019), sexes (Lewis et al., 2002; Phillips R.A. et al., 2004; Camphuysen et al., 2015), and nearby populations of the same species (Rayner et al., 2011; Wiley et al., 2012; Ceia et al., 2015; Shaffer et al., 2017; Bolton et al., 2019). While resource partitioning is evident during breeding when competition for resources close to the colony is high, partitioning during the post-breeding period has received much less attention until recent years. During this period, when less constrained by the restrictions of central place foraging, spatial segregation may dissipate or be substantially reduced once breeding has ended and seabirds can range freely and disperse to reduce competitive pressures (Phillips et al., 2005; Shaffer et al., 2006; González-Solís et al., 2007; Egevang et al., 2010; Rayner et al., 2011; Wakefield et al., 2013). The degree of competitive interaction is also mediated by preferences for habitats where prey are associated with environmental characteristics that promote primary production and prey availability, elevating probabilities of co-occurrence (Pinaud and Cherel, 2005; Nur et al., 2011). This effect may be amplified when species are generalists (Dehnhard et al., 2020). As such, assessing environmental characteristics of habitats used by seabirds is critical to understanding mechanistic drivers of habitat use and the role that competitive interactions play in driving distributional segregation or overlap.
As highly mobile seabirds that forage in varied and dispersed habitat over the course of their breeding cycle (Tickell, 2000; Fernández et al., 2001; Croxall et al., 2005), albatross present suitable study species for assessing whether spatial segregation persists during post-breeding. When the spatial constraints of breeding are removed, differences in habitat use between these vagile species may be less evident. However, much less is known about albatross habitat use during post-breeding and thus it is unclear whether resource partitioning occurs outside the breeding period. As for other seabird species, the vast majority of tracking studies occur during breeding, when data loggers can be deployed and retrieved at the breeding colony with relative ease as tagged individuals are constrained to return to their nest for breeding (Jouventin and Weimerskirch, 1990; Weimerskirch et al., 1993; Grémillet et al., 2000; Burger and Shaffer, 2008; Wakefield et al., 2013; Gutowsky et al., 2014b). Understanding spatial habitat use and resource partitioning of albatrosses during post-breeding is important for several reasons. Since albatrosses periodically have “sabbatical” years when the skip breeding (Jouventin and Dobson, 2002; Arata et al., 2009), a significant portion of their at-sea habitat use occurs outside of the breeding period (potentially over 50%, as typical breeding durations last 6–7 months and sabbatical years can entail 7–9 months at-sea durations). Assessing habitat relative to threats outside of breeding and across colonies is a key to the effective conservation and management of albatross species. For example, a better understanding of post-breeding spatial distributions is needed to identify areas of increased by-catch risk (Wooller et al., 1992) due to overlap with fishing effort (Žydelis et al., 2011, 2013; Edwards et al., 2015). Further, intra-seasonal changes in post-breeding distributions are understudied, particularly with respect to spatial segregation between species. While investigations into post-breeding movements of seabirds are becoming more common, most spatial analyses of the post-breeding period examine the period in its entirety, with only a handful of studies analyzing temporal subsets of the post-breeding period (Frederiksen et al., 2016; Fromant et al., 2020; Lamb et al., 2020). Analyses conducted over longer timespans may mask important intra-seasonal movements and behaviors, such as selection of molting grounds and foraging behavior related to seasonal oceanographic changes (Conners, 2015). Analyses conducted at finer temporal scales are needed to identify dynamic drivers of habitat use and mechanisms like competitive pressures that drive spatial partitioning during subsets of the post-breeding period.
Studies of two closely related and sympatrically breeding species of albatross in the North Pacific, Laysan (Phoebastria immutabilis) and black-footed (P. nigripes) albatross, highlight the gaps in our understanding of their breeding and post-breeding distributions. While many studies have assessed and compared the spatial habitat use of Laysan and black-footed albatross, most of these studies have examined habitat use during breeding (Fernández et al., 2001; Hyrenbach et al., 2002; Finkelstein et al., 2006; Kappes et al., 2010, 2015; Conners et al., 2015; Gutowsky et al., 2015; Thorne et al., 2015, 2016; Gilmour et al., 2022). Drawing broad habitat conclusions from these studies is difficult due to the focus on a single species (Hyrenbach, 2001; Hyrenbach and Dotson, 2003; Young et al., 2009; Gutowsky et al., 2014b; Henry et al., 2021; Gilmour et al., 2022), deployments from sympatric species from a single colony (Hyrenbach et al., 2002; Finkelstein et al., 2006; Kappes et al., 2010, 2015; Block et al., 2011; Žydelis et al., 2011; Gutowsky et al., 2014a; Conners et al., 2015; Thorne et al., 2015, 2016; Antolos et al., 2017), or deployments of tagged individuals at-sea, which does not allow colony-level differences to be assessed (Hyrenbach, 2001; Hyrenbach and Dotson, 2003; Fischer et al., 2009; Suryan and Fischer, 2010). Further, previous studies have primarily focused on breeding Laysan and black-footed albatross at Tern Island (e.g., Kappes et al., 2010, 2015; Žydelis et al., 2011; Conners et al., 2015) despite the smaller population of both species breeding at Tern Island compared to Midway Atoll (approx. 3,200 and 4,300 breeding pairs of Laysan and black-footed albatross at Tern Island vs. approx. 408,000 and 22,000 breeding pairs of Laysan and black-footed albatross at Midway Atoll, respectively; Arata et al., 2009). Midway Atoll comprises approximately 60 and 30% of the world’s breeding populations of Laysan and black-footed albatross, respectively, and thus studying the at-sea movements of albatrosses from this colony is critical to understanding species-level patterns of habitat use.
When breeding, habitat differences in foraging Laysan and black-footed albatross are characterized by distinct oceanographic processes at both mesoscale (fronts and eddies) and basin scale oceanographic features (e.g., the location of the Transition Zone Chlorophyll Front) that differentiate habitat use between the species (Hyrenbach et al., 2002; Kappes et al., 2010; Thorne et al., 2015). Environmental conditions can influence habitat selection and foraging strategy on a seasonal scale (Lamb et al., 2020), and as such, interspecific patterns of segregation and spatial distributions change within an albatross’s annual cycle. For the post-breeding period, observational data on spatial distributions of the habitats of both of these species have been collected as far back as 1955 (Kuroda, 1955), which indicate that individual habitat use during the post-breeding period occurs in productive shelf waters and boundary currents (Wahl et al., 1989). Tagging studies of post-breeding black-footed albatross show that individuals can occur along the shelf and slope waters near Alaska (Fischer, 2008; Fischer et al., 2009; Suryan and Fischer, 2010) as well as the west coast of Canada (Žydelis et al., 2011), whereas post-breeding Laysan albatross are distributed in pelagic regions of the central and western Pacific (Gutowsky et al., 2014a; Henry et al., 2021). Analyses of environmental characteristics during the post-breeding period have been limited to a few years of observations, often from a single population (but see Henry et al., 2021), and it is unclear the extent to which previous observations can be generalized. The degree to which post-breeding habitat is shared or segregated between species and between colonies of the same species is similarly unclear.
Here, we assess whether resource partitioning between Laysan and black-footed albatrosses persists during post-breeding. We use data from global location sensing (GLS) loggers deployed on albatross at two breeding colonies across 5 consecutive years to address the following questions: First, do known differences in the spatial distributions of Laysan and black-footed albatrosses persist into and throughout the post-breeding period? Second, do post-breeding albatrosses of the same species but from different colonies share similar at-sea distributions? Third, if post-breeding segregation occurs, what environmental variables characterize each species’ habitats? Lastly, do Laysan and black-footed albatrosses show shifts in spatial distribution and changes in the environmental characteristics of their habitats over the post-breeding period? Addressing these questions will improve our understanding of habitat use and resource partitioning in North Pacific albatross, and may identify potential competitive interactions between species and colonies outside of breeding.
Materials and methods
Data collection
Study species
Laysan and black-footed albatross colonies are distributed throughout the North Pacific Ocean where they nest on low-lying, sandy tropical and sub-tropical islands from Mexico to Japan. Both species have consistent breeding cycles, initiating courtship and nesting in mid-November and subsequently incubating their eggs for approximately 50 days, brooding their young for approximately 1 month, and finally rearing chicks for several months until chicks fledge (total time for breeding ca. t ≈ 165 days for Laysan albatross; t ≈ 150 days for black-footed albatross; Warham, 1996; Tickell, 2000). Both species are colonially nesting central place foragers that are capable of traveling vast distances (>10,000 km) in a single foraging trip during the incubation and chick-guard phases of breeding (Fernández et al., 2001; Kappes et al., 2010, 2015). Following the breeding period, adults and fledglings leave the breeding colonies independently (Gutowsky et al., 2014b) and remain at sea until late fall or early winter when they return to their breeding colony (if breeding). During this time, wide-ranging foraging on productive waters and molting (partial or full) of feathers occurs before breeding adults return to island colony sites in late-October (Arata et al., 2009; Gutowsky et al., 2014a).
Study sites and light-level geolocator deployments
Geolocators (herein “loggers” or “GLS loggers”) were deployed on breeding Laysan and black-footed albatross at Sand Island, Midway Atoll National Wildlife Refuge (28.12°N, 177.23°W, hereafter “Midway”) and Tern Island, French Frigate Shoals (23.87°N, 166.28°W, hereafter “Tern”) from 2008 to 2012. We focus on this time period in order to allow for comparisons of habitat use between species and colonies as data collection was possible at both colonies during this time frame; tracking studies have not been possible at Tern Island since 2012, when a microburst hit the island and destroyed the field station. Both Midway and Tern are part of the Northwestern Hawaiian Islands archipelago, within the Papahānaumokuākea Marine National Monument. Birds were captured at their nest while incubating eggs or guarding chicks. Albatrosses were equipped with either a 6 g Lotek LTD 2400 (Midway) or 3.6 g Lotek LAT2500 (Tern) geolocation archival data logger (Lotek Wireless, St. John’s, Newfoundland). These devices measure changes in ambient light levels to estimate sunrise, sunset, and day length, and by evaluating these metrics against an internal clock, are able to estimate latitude and longitude (DeLong et al., 1992; Hill and Braun, 2001; Lisovski et al., 2012). Loggers were mounted on plastic identification bands placed around each bird’s tarsus using ultra-violet resistant cable ties and quick-setting epoxy. The total auxiliary package weighed <2% of bird body mass, the recommended maximum device weight of 3% (Phillips et al., 2003). Each logger recorded ambient light intensity every 60 s to determine the time of local sunrise and sunset.
For each day, single latitude was derived from day length, while a single longitude was calculated from the anticipated difference of local noon (estimated from light intensities) compared to Universal Time as informed by the calculated latitude (DeLong et al., 1992; Wilson et al., 1992). Loggers were additionally programmed to record immersion state (wet/dry) at various resolutions (ranging between every 32–432 s), as well as ambient temperature and sea-surface temperature (SST) when the tags were wet. All devices were removed from birds at their nests either 1–2 breeding seasons after the initial deployments; logger recovery rates were approximately 69% for Laysan albatross and 56% for black-footed albatross (Table 1). Success or failure of breeding was determined by examining mean departure dates for each species-colony class and identifying individuals that departed earlier than one standard deviation from the mean departure date, as individuals that fail breeding will typically leave the colony shortly after failure to begin foraging at sea. Laysan and black-footed albatross skip breeding in some years in order to molt an accumulation of worn-out primary feathers (Langston and Rohwer, 1996); individuals with deployments spanning more than one post-breeding season were considered “skip-breeders” for that year.
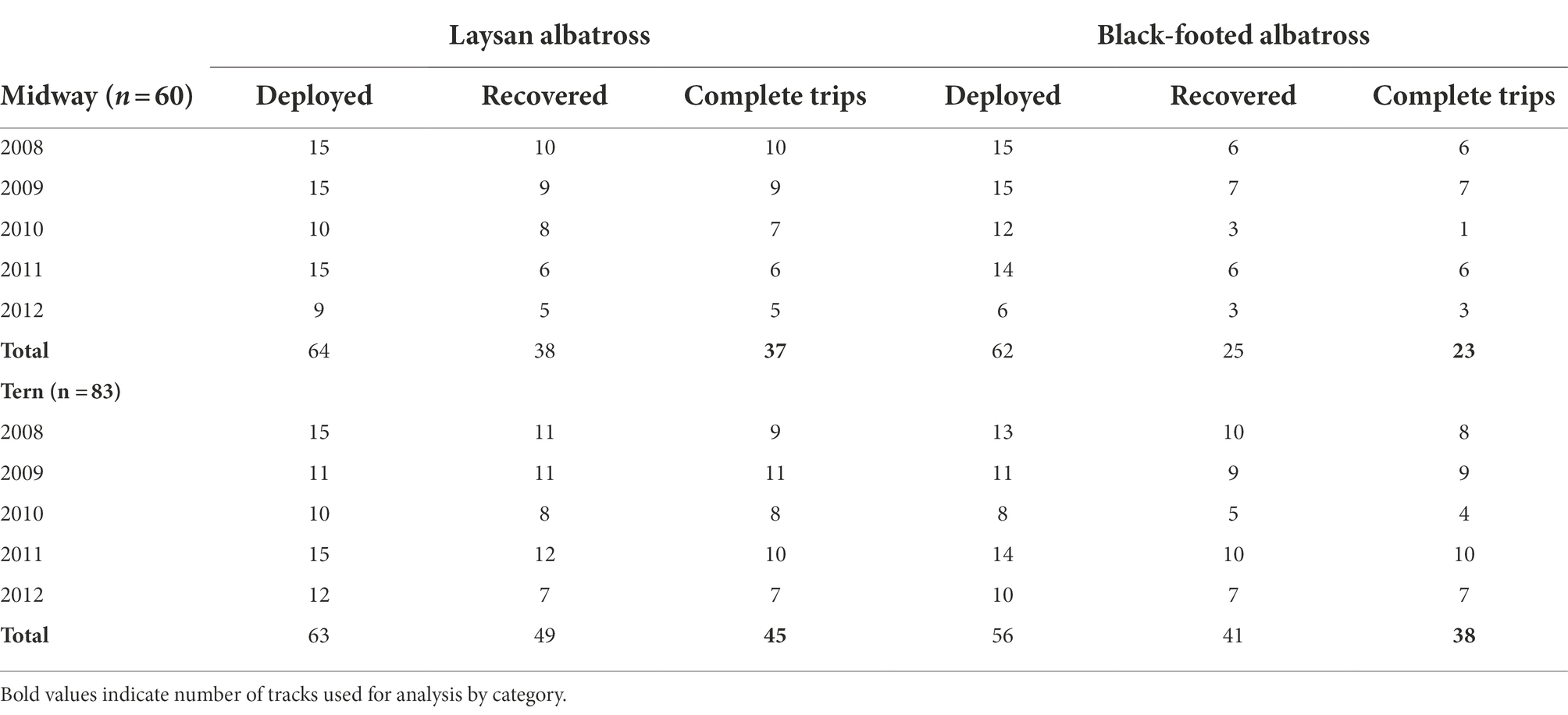
Table 1. Geolocator deployment sample size by species and colony, with number of loggers deployed, recovered, and had viable data and represented complete trips to and from the colony.
Estimating bird locations from light-level geolocation data
Global location sensing logger data were processed and analyzed in R v. 3.6.2 (R Core Development Team, 2019). Given the high variation in location estimates from light-based geolocation, especially around seasonal equinox periods (Wilson et al., 1992; Welch and Eveson, 1999; Phillips R. et al., 2004; Shaffer et al., 2005; Hinke et al., 2015), locations were re-estimated using the logged sunrise and sunset times using the R package “probGLS” (Merkel et al., 2016) which shows low spatial error (approx. 33% less error) relative to other GLS movement analysis packages (Halpin et al., 2021). This iterative forward step selection framework estimates positions from sunrise and sunset times using threshold techniques and generates a cloud of possible estimates for each location (n = 800, iterated 60 times forming weighted particles). These estimated positions were weighted by daily median SST recorded by the geolocators and compared to temporally matched remotely sensed high resolution SST data from NOAA.1 Estimated locations were further evaluated using a speed filter for periods when an individual was in flight or resting on the sea surface from the wet/dry sensor data. Wet/dry immersion data were available for all years except for 2009 at Tern. For these data, the logger was assumed to be dry for the entire period, potentially allowing for more dispersed distributions of animals tracked in this year. For both species, travel speeds lower than 10 km h−1 were assumed to be stationary (Antolos et al., 2017) compared to mean travel speeds in flight of 35–40 km h−1, though maximum travel speeds can be much higher than these mean values (Hyrenbach et al., 2002; Kappes et al., 2015). Using these weighted location particles, 100 possible/potential tracks were calculated, and the geographic median of the cloud of each position estimate was chosen as the final most likely track. Parameters used in this framework are reported in Table 2. Tracks spanning the entire annual deployment periods were generated and from these, segments of the track representing the post-breeding period were isolated by using SST records to identify departure from and return to the breeding colony (Conners, 2015). Location estimates and spatial analyses were limited to complete trips where birds were tracked continuously between their departure from and return to the breeding colony. Only tracks that included complete tracking logs and SST readings from deployment to retrieval were included in our analyses; deployments with logging failures or incomplete records due to insufficient device storage were excluded (n = 10). Original light-level data for three Laysan albatross tracks at Tern Island (two individuals in 2010 and one individual in 2012) were no longer accessible. In these instances, tracks originally processed by Melinda Conners were substituted for analysis (see Conners, 2015 for processing details).
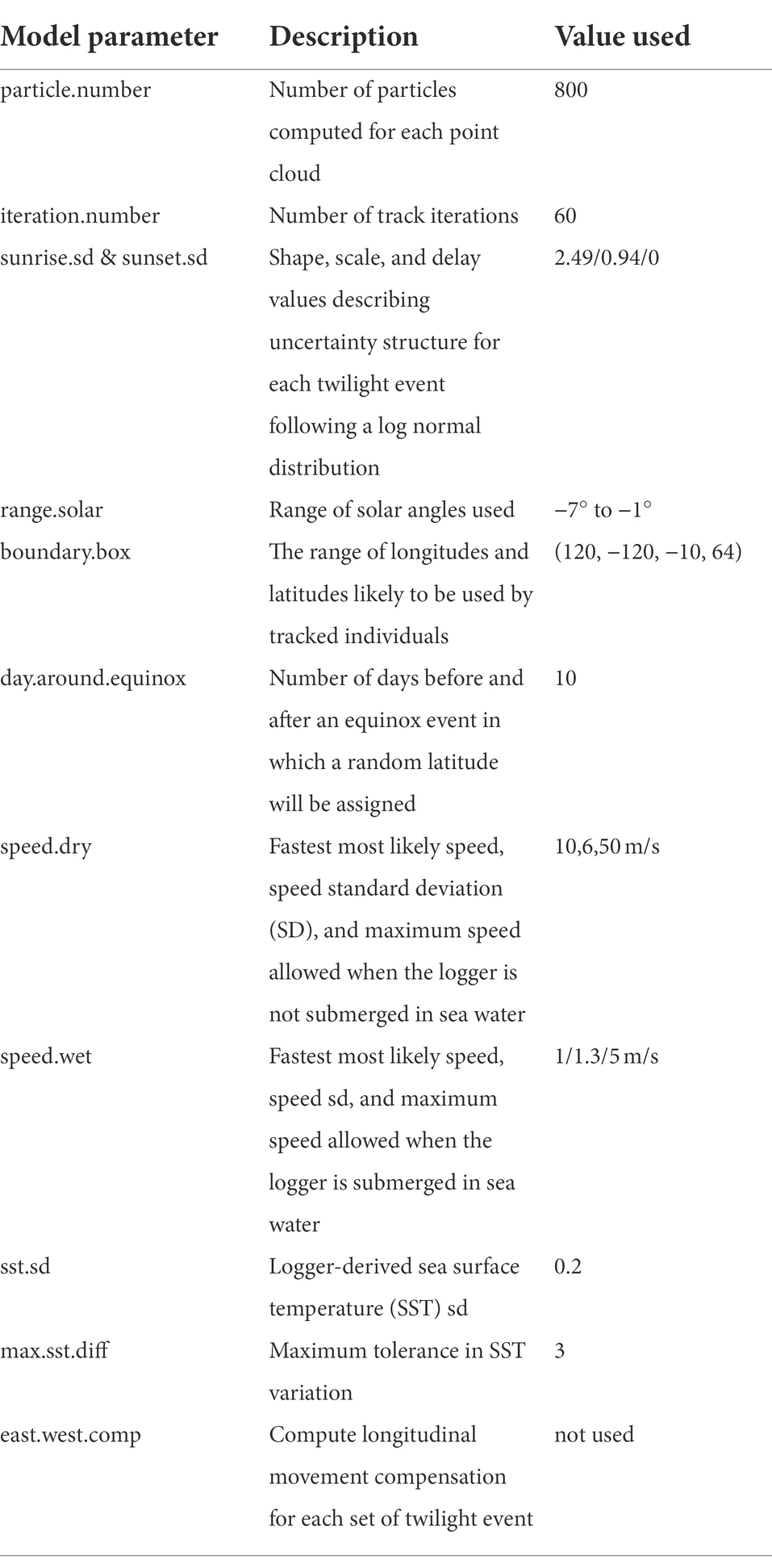
Table 2. “probGLS” algorithm parameters for estimation and analysis of positions using GLS data of black-footed albatross and Laysan albatross at Midway and Tern.
Data analysis
Quantifying overlap between foraging (95th percentile of utilization distribution) and focal (50th percentile of utilization distribution) areas
Prior work assessing overlap of highly mobile marine predators implemented a method of randomization whereby tracks were randomly reassigned to one of the groups being assessed (herein the “track ID permutation” method; Breed et al., 2006; Clay et al., 2016). This method permutes the label or ID assigned to a specific track while preserving the initial sample size and compares the overlap statistic for these randomized groups to the overlap statistic of the observed data. The null hypothesis is that the two groups are not more overlapped than would be expected by random chance given the observed trajectories. When implemented for this study, preliminary results did not align with visual inspections of overlap in post-breeding distributions; the track ID permutation method indicated significant segregation in cases when distributions showed considerable overlap. To address these inconsistencies, we compared the track ID permutation method with another method of creating overlap patterns under the null hypothesis in which tracks were generated using a correlated random walk (“CRW”; Kareiva and Shigesada, 1983) to determine whether CRWs might better represent the potential habitat available to highly mobile species (herein the “CRW method”). The details of this comparison are described in Supplementary material. The results of this comparison of randomization methods suggested that the CRWs provided a more reliable means of assessing significance of segregation in the observed GLS data by representing broader spatial habitat available to albatrosses (Supplementary Figure 1). We therefore used the CRW approach for analyses of segregation of albatross distributions.
Correlated random walks are simulated tracks that originate from the same starting locations as the true data and then allow for random walk trajectories from that origin, with the trajectories being determined by selected distributions for distance and turning angle. Here, we simulated subsequent points by randomly drawing a distance and angle independently from distributions of travel distances and turning angles fit using the initial data (Hazen et al., 2017, 2021). The package adehabitatLT was used to determine turning angles and distances between track points present in the data. Distributions were fit by examining Cullen and Frey graphs to select candidate distributions and subsequently testing goodness of fit (Cullen et al., 1999). A log-normal distribution was the best fit for each category of species and colony, aligning with previous findings in animal movement studies (Limpert et al., 2001; Diefenbach et al., 2008). As location and shape parameters were similar, the parameters for the aggregated comparison μ = 11.9 and σ = 0.9 were chosen to represent the lognormal distance distribution to draw from our CRWs. Similarly, all turning angle distributions were tested by species-colony category, with a uniform distribution (a = 0 and b = 359) showing the strongest fit for each category, and hence was used for the turning angle distribution of our CRWs.
Comparisons of overlap in post-breeding distributions
We produced Kernel Density Estimates (KDEs) of post-breeding distributions to calculate overlap metrics between species and colonies. Post-breeding position estimates were projected using a Lambert Cylindrical Equal Area projection (Wood et al., 2000), and KDEs were generated using the adehabitatHR package (Calenge, 2006) to determine utilization distributions following methods described by Kappes et al. (2015). We analyzed KDEs at a 300-km resolution following the findings of a double-tagging study by Halpin et al. (2021) which showed that the error associated with GLS loggers was approximately 300 km. A fixed kernel approach was used, which incorporated a bivariate normal kernel (Worton, 1989) and several estimates for the bandwidth h were explored, including the (1) reference bandwidth for standard bivariate normal distributions, (2) least-squares cross-validation (LSCV) derived bandwidth, (3) exact mean integrated square error bandwidth, and (4) bandwidth derived using a direct plug-in selector. Though fixed kernel estimates using the LSCV-derived smoothing parameter have been shown to produce the most accurate estimates (Seaman and Powell, 1996), the temporal resolution of GLS data, large sample size, and highly varied space use across individuals (Hemson et al., 2005) prevented convergence of the LSCV algorithm in numerous cases. The reference bandwidth href resulted in oversmoothing; given the large spatial extent and multimodal distribution of relocations, href was not appropriate for our study (Worton, 1995; Seaman et al., 1999). Therefore, we used a fixed search radius of 150 km to account for inherent location error and uncertainty in light-level geolocation (Phillips R. et al., 2004; Shaffer et al., 2005) and kept this search radius consistent across all analyses in order to facilitate comparisons between species and colonies (Powell, 2000; Kappes et al., 2015; Silverman, 2018). Kernel estimates based on Brownian bridge movement models were also considered, but a fixed kernel method was chosen as the 12-h time interval between relocations violated the assumption of conditional random movement between location pairs.
We assessed overlap for the following species and colony comparisons: between species (using data from both colonies; “species+colony-level comparison”), between albatross of the same species breeding at Tern and Midway, respectively (“colony-level comparison”), and between species breeding at the same colony (“species-level comparison”), and assessed how overlap varied through time. Gutowsky et al. (2015) found that tracks from a minimum of 17–21 individual albatross were needed to minimize variability of contour overlap assessments during the post-breeding period. Sample sizes in the present study were too small to consider interannual comparisons of segregation within and between populations (data were obtained from a maximum of 11 tags in a year; Table 1), so we pooled individual-level KDEs across all 5 years to reach a sufficient sample size. To assess how species+colony spatial relationships change throughout different stages of the post-breeding period, we assessed spatial distributions in bimonthly periods (June–July, August–September, and October–November), which included sufficient data for assessing overlap (a minimum of 17 individuals per species and at least five track points per track; Supplementary Table 1). Though some post-breeding tracks included points during March, April, and May, these months were excluded from our temporal comparisons, as they contained far fewer points than subsequent months, were not represented by all four species-colony groups, or did not include sufficient temporal overlap (e.g., only a few days in a given month) to allow overlap to be assessed. Tracks from individuals that skipped breeding in a given year were also trimmed to June–November for consistency in analysis of habitat use. Thus, for each species or colony comparison (species+colony-level, colony-level, and species-level), we assessed overlap in both foraging and focal areas at different temporal period within the post-breeding period (June–July, August–September, and October–November).
The degree of spatial overlap in foraging and focal areas was assessed using three metrics: the Utilization Distribution Overlap Index (“UDOI”; Fieberg and Kochanny, 2005), Bhattacharyya’s affinity (“BA”), and the probability of home range overlap statistic (“PHR”) for each overlap assessment using the adehabitatHR package. These three metrics were calculated in order to determine whether results were consistent across overlap indices. We compared the results of the metrics relative to spatial patterns observed in the KDEs. UDOI outputs range from 0 (no overlap) to 1 (complete overlap), but values >1 are possible if the spatial distributions are not uniform and show a high degree overlap (Fieberg and Kochanny, 2005). UDOI is calculated as
Equation 1 indicates calculation of UDOI with A1,2 representing the total area encompassed by both utilization distributions, and ÛD(x,y) representing the value of each utilization distribution in a given cell of the gridded distribution (KDEs computed from a bivariate normal distribution). Bhattacharyya’s affinity index ranges from 0, representing no overlap, to 1, representing complete overlap between spatial distributions. BA is calculated as
Equation 2 indicates calculation of BA. Similar to UDOI and BA, PHR is an index representing the probability of overlap, ranging from 0 to 1. PHR is a directional statistic, and is thus represented by two values (i.e., the probability of species A being found in the home range of species B, and vice versa), and is calculated as
Equation 3 indicates calculation of PHR with PHRi,j and PHRj,i representing the two directions of overlap. All metrics were calculated as conditional indices (i.e., reflecting the probability species A is in the focal area of species B given that species A is in its own focal area) to allow for direct comparisons at various probability contours. Following the results of the simulation study, which suggested that randomizations produced with the CRW method resulted in more appropriate assessments of overlap (see Results); we assessed the significance of segregation using this method.
General trip length characteristics were calculated as additional summary characteristics, including mean departure date, mean return date, trip length, the area of the foraging and focal areas, the distance from the breeding colony to the centroids of the foraging and focal areas (calculated as the centroid of the polygon of the 95th and 50th UDs, respectively), and the bearing from the breeding colony to the centroids of the foraging and focal areas.
Environmental data
Kappes et al. (2010) investigated the impact of a number of environmental variables on foraging locations during the breeding period and found that SST was the most important variable correlated with Laysan and black-footed albatross habitat use. Chlorophyll-a concentration (as a proxy for primary productivity) has also been found to be an important predictor of albatross habitat (Žydelis et al., 2011; Henry et al., 2021). Albatross rely on wind to move between breeding and foraging areas, and accordingly wind has been shown to be an important environmental variable that influences albatross foraging behavior and life history (Weimerskirch et al., 2000, 2012; Barnes, 2004; Suryan et al., 2008; Thorne et al., 2016; Richardson et al., 2018). Henry et al. (2021) found that SST, wind, and chlorophyll-a concentrations were the most important habitat predictors for Laysan albatross, and therefore we used these three variables to compare environmental characteristics of Laysan and black-footed albatross post-breeding habitat. We used monthly composites of Aqua MODIS Chlorophyll-a (‘Chl-a’; mg m−3), with a 4-km spatial resolution, wind speed (m s−1, hourly) from the MERRA-2 reanalysis product with a 0.125° spatial resolution, and SST recorded in situ on GLS loggers (°C) from the post-breeding period. We used the monthly composite for Chl-a as higher temporal resolutions did not provide sufficient spatial coverage due to cloud cover. Wind speed was calculated from u and v components of the 10-m height surface model, and the hourly data from the MERRA-2 reanalysis was averaged into daily composites to match the temporal resolution of processed GLS data. For both Chl-a and wind, the mean value from within a 300-km buffer of each albatross track point was used to match the accuracy of processed GLS data. Chl-a was downloaded using Marine Geospatial Ecology Tools (Roberts et al., 2010) in ArcGIS 10 and wind components were downloaded from the MERRA-2 reanalysis (Gelaro et al., 2017, https://disc.gsfc.nasa.gov). Loggers recorded SST in situ when submersion was detected and were averaged into daily values to match the temporal resolution of processed GLS data.
Results
Of 245 deployments across 5 years, 60 produced complete (suitable for analysis) tracks for albatrosses breeding at Midway (Laysan albatross = 37, black-footed albatross = 23) and 83 produced complete tracks for the colony at Tern (Laysan albatross = 45, black-footed albatross = 38), for a total of 143 tracks included in the analysis (Table 1). Trip durations were broadly similar across all species-colony groups, with the exception of black-footed albatross from Midway, which showed shorter average trip durations than the other classes by approximately 20–30% (Table 3). Laysan albatross from both colonies showed longer average post-breeding trips than black-footed albatross. Laysan albatross from Tern initiated post-breeding departures earliest (26 May ±56.85 days), and Laysan albatross from Midway returned from post-breeding foraging latest (26 November ±11.53 days). Laysan albatross from Tern showed the highest variability in post-breeding trip length, principally due to high variability in departure dates. Across 5 years of observations, 13 Laysan albatross had failed breeding attempts (Midway = 5, Tern = 8) and five black-footed albatross had failed breeding attempts (Midway = 2, Tern = 3). Notably, half of failed breeding attempts for Laysan at Tern occurred in 2011. We identified one skip-breeder across our sample size of 143 individuals (a Laysan albatross at Midway which skipped the 2010–2011 breeding season).
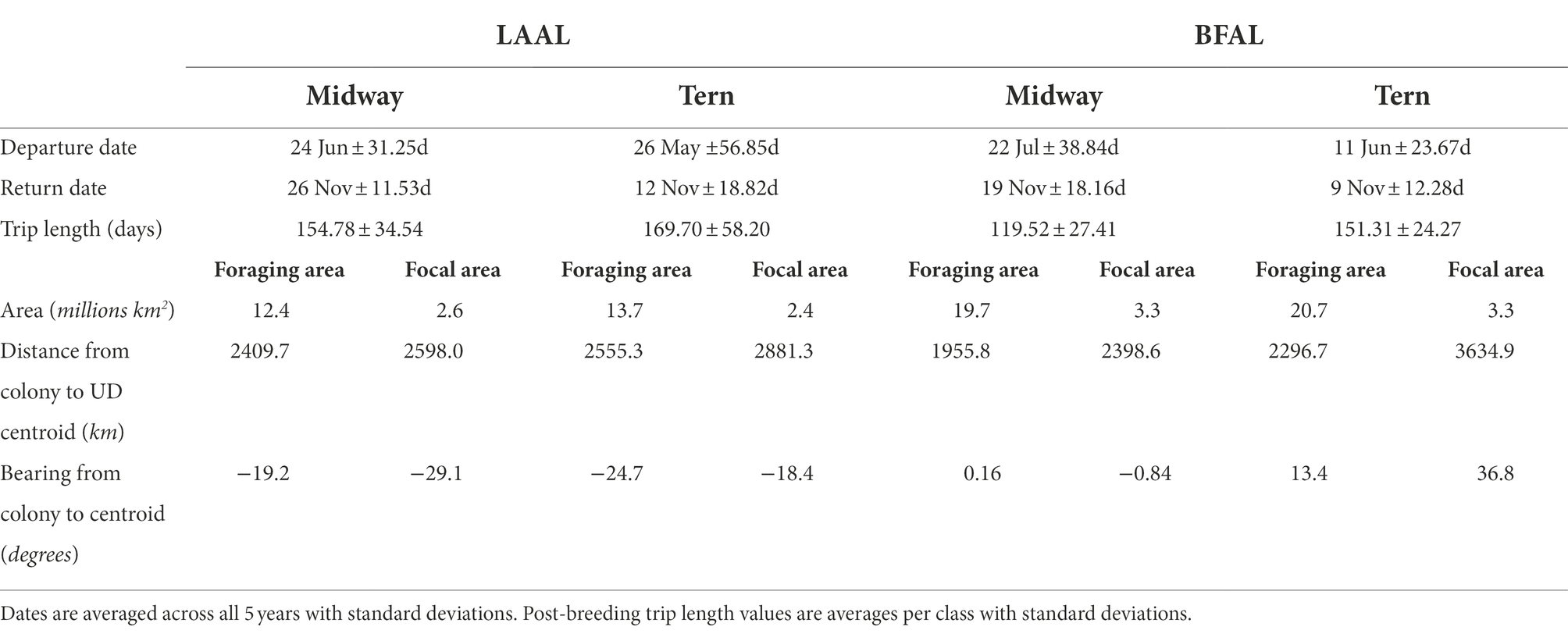
Table 3. Summary trip characteristics of departure date, return date, trip length, area of focal and foraging areas, distance from colony to the centroid of the focal and foraging areas, and bearing from the colony to the centroid of the focal and foraging areas by species (LAAL = Laysan albatross; BFAL = black-footed albatross) and breeding colony for both foraging and focal areas (represented by the 95 and 50th utilization distribution, respectively).
Both Laysan and black-footed albatross generally showed broad post-breeding distributions (Figure 1) and significant at-sea segregation of both foraging and focal areas (Table 4). The focal area for Laysan albatross, when data from both the Tern and Midway colonies were aggregated, was distributed within the Subarctic Gyre in the Oyashio Current and bordered the northern front of the North Pacific Transition Zone (NPTZ). Focal areas of black-footed albatross across both colonies were predominantly in the northeastern Pacific along the California and Alaska Current regions, as well as in the central Aleutian Islands and the Bering Sea. However, north central regions of the Pacific near the central Aleutian Island chain were important for both species throughout the post-breeding period. Broadly, Laysan albatross showed more pelagic distributions, including the Alaska Stream and Oyashio Current whereas black-footed albatross generally associated with coastal shelf habitat throughout the observed range. Pelagic distributions for black-footed albatross were much more limited, with the exception of the western portion of the NPTZ and the central Bering Sea. As a result of the use of different regions within the North Pacific, Laysan and black-footed albatross aggregated across both breeding sites over 5 years showed significant at-sea segregation of both the foraging and focal areas (Figure 1; Table 4; only p values for UDOI are described here to facilitate interpretation).
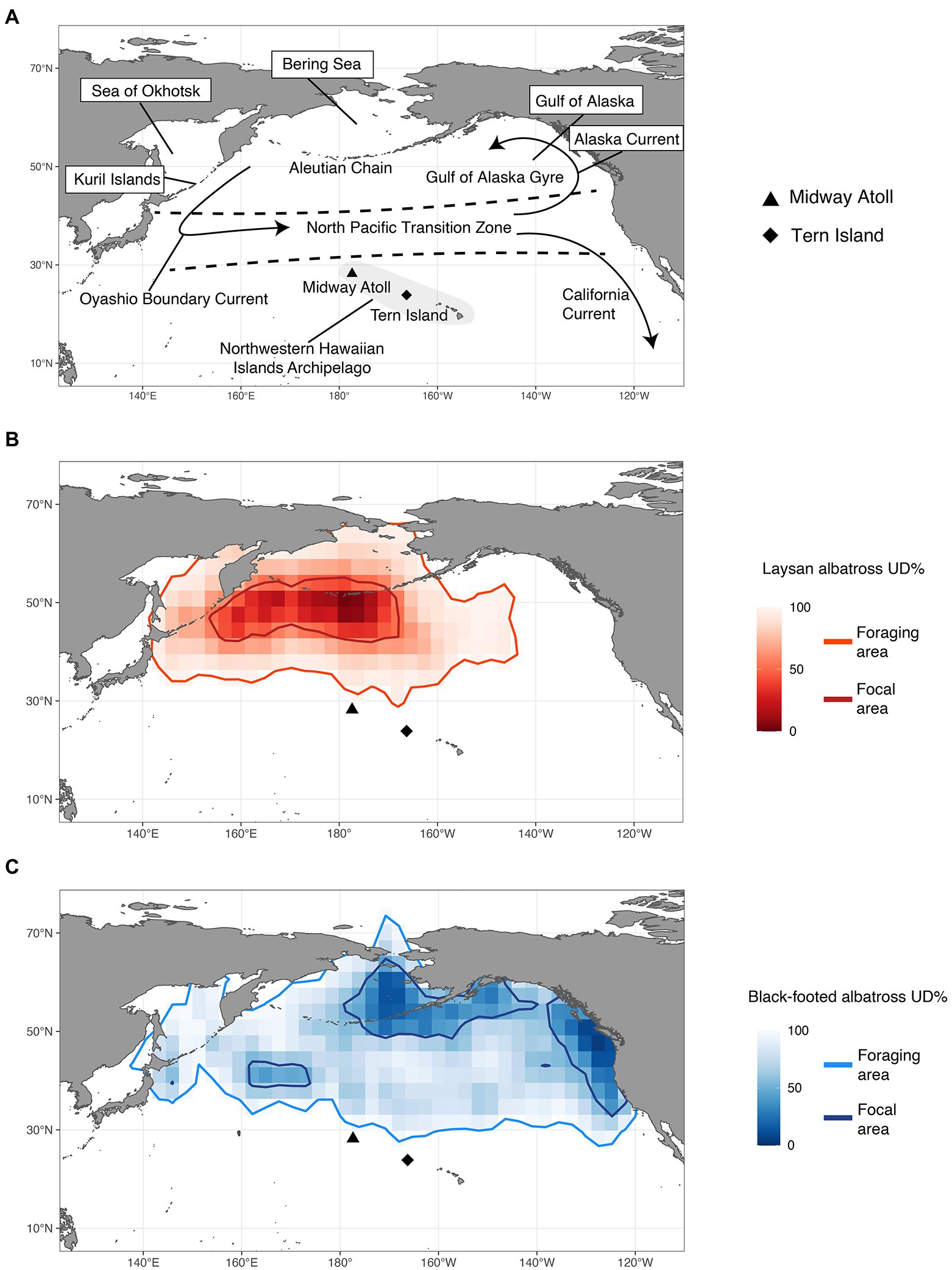
Figure 1. (A) Important oceanographic locations and features; overall utilization distribution (UD) densities of (B) Laysan albatross and (C) black-footed albatross from both colonies with contours of the foraging area (95th UD) and focal area (50th UD) for both species.
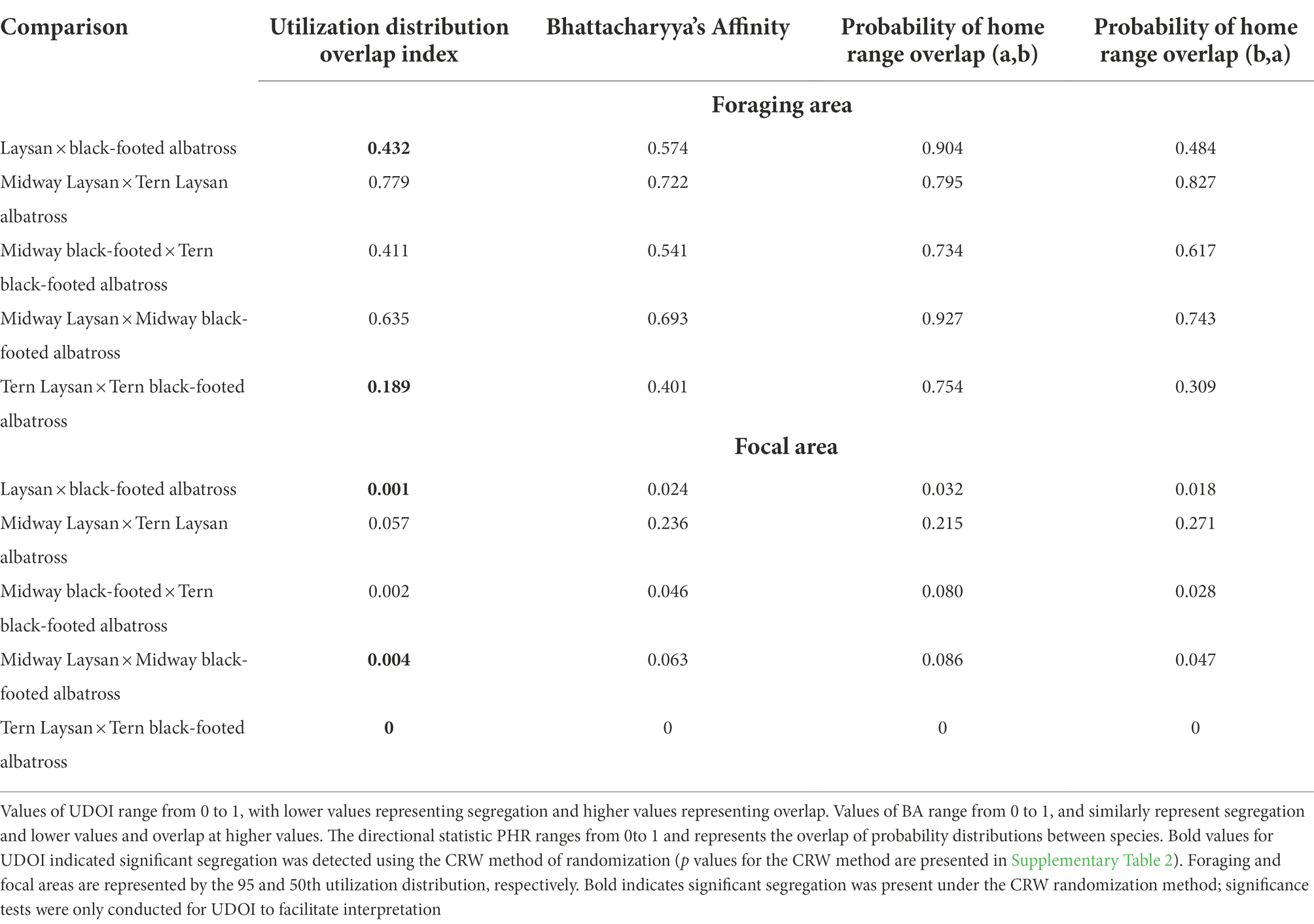
Table 4. Values of overlap indices for Laysan and Black-footed albatross breeding at Midway and Tern Island for all post-breeding points.
When examining habitat use by colony, foraging and focal areas were smaller for Laysan albatross than black-footed albatross (Table 3). Distance of centroids of foraging and focal areas showed more variability among black-footed albatross, with Midway black-footed albatross’ foraging distribution centroid occurring closest to the breeding colony among all species-colony groups and Tern black-footed albatross focal distribution furthest among all classes. Distances from the colony to the centroids of foraging and focal areas were similar (foraging and focal centroids ca. 2,410 and 2,598 km away from Midway; ca. 2,555 and 2,881 km away from Tern) for both colonies of Laysan albatross. For both foraging and focal areas, Laysan albatross generally flew to the northwest from colonies while black-footed albatross generally flew northeastern (Table 3).
Segregation between foraging areas of Laysan albatross breeding at Midway and Tern was low (Figures 2A,C; UDOI = 0.779), while segregation of focal areas was high (UDOI = 0.057), and significant segregation was not detected in either case (Table 4). Foraging areas of Laysan albatross breeding at Tern and Midway both included broad regions of the central and western North Pacific. However, subtle differences in Laysan focal habitat use occurred between the two colonies, despite the presence of considerable overlap in both foraging and focal areas when data from throughout the post-breeding period were examined. High densities of Laysan albatross breeding at Midway were observed on the northwestern Pacific eastward of the Kuril Islands, whereas densities of Laysan albatross breeding at Tern were highest toward the central Aleutian Islands and central areas of the Bering Sea. Laysan albatross from Tern did not appear to utilize the Oyashio Current as frequently or as densely as Laysan albatross from Midway. In addition, the habitat used by Laysan albatross breeding at Tern did not include the Sea of Okhotsk while this region was within the focal area of Laysan albatross breeding at Midway. The habitat of Laysan albatross breeding at Tern occupied a similar longitudinal range as those breeding at Midway (85 and 88° longitude respectively), but the distribution was further east, extending to seamount areas of the northwestern Pacific, which were not accessed by individuals from Midway. Laysan albatross breeding at Midway used habitats that extended farther north than those breeding at Tern Island. The overall latitudinal range of post-breeding habitat for birds from Midway Atoll was approximately 25% greater than conspecifics breeding at Tern (range of approximately 37 and 47° latitude for Laysan albatross breeding at Tern and Midway, respectively), though the area of the foraging and focal areas was similar between the two colonies (Table 3).
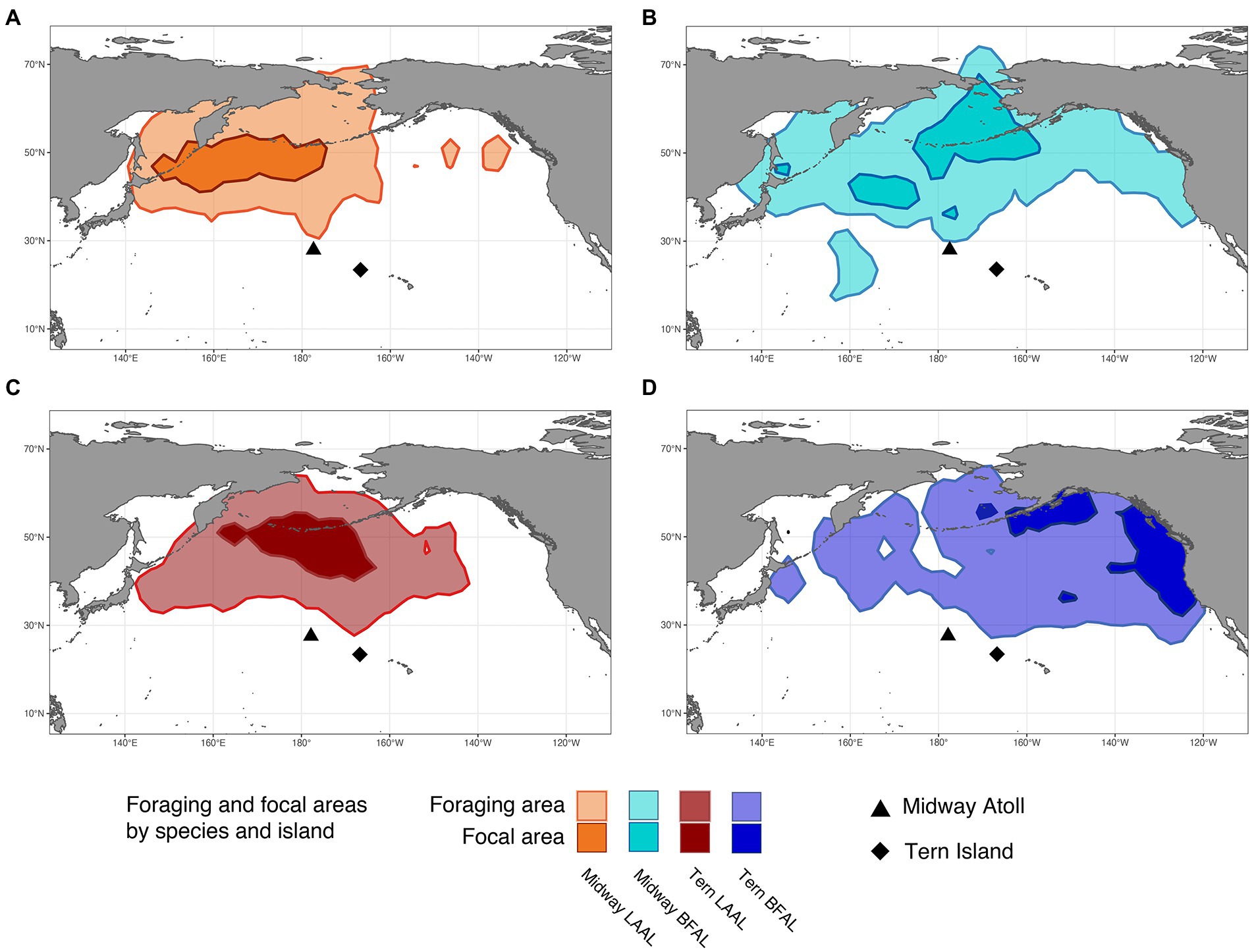
Figure 2. Foraging and focal areas (95 and 50th utilization distributions, respectively) for: (A) Midway Laysan albatross, (B) Midway black-footed albatross, (C) Tern Laysan albatross, and (D) Tern black-footed albatross.
Habitats used by black-footed albatross showed moderate segregation in foraging areas (UDOI = 0.411) between the Midway and Tern breeding colonies, which was not found to be significant, while focal areas between the two colonies showed higher, though insignificant segregation (UDOI = 0.002; Figures 2B,D; Table 4). Most notably, the foraging area of Midway black-footed albatross encompassed the entire northern extent of the North Pacific basin, and extended to higher latitudes than conspecifics breeding at Tern, which primarily used habitats in the eastern North Pacific. The focal distribution of black-footed albatross from Midway extended from the northern side of the central Aleutian Islands and the entire Pribilof Islands through the Northern Bering Sea. The black-footed albatross focal habitat breeding at Tern was much more coastal in comparison, focused on coastal regions of the Gulf of Alaska and along the western coast of the United States and Canada. However, black-footed albatross focal habitat from both Tern and Midway included regions south of the eastern Aleutian Islands. Longitudinal ranges were similar for black-footed albatross breeding at Midway and Tern (range of 109°), and encompassed the entire longitudinal range of the northern North Pacific (range of 103–97° respectively). Latitudinal ranges of black-footed albatross were highly disparate between the breeding colonies (range of 53° latitude for Midway black-footed albatross, 37° latitude for Tern black-footed albatross), with albatross breeding at Midway extending further north into the Chukchi Sea than those breeding at Tern.
The degree of at-sea segregation of albatross breeding at the same colony varied between Tern and Midway. When all time periods were analyzed together, the foraging area of Laysan albatross breeding at Midway showed strong overlap with that of black-footed albatross breeding at Midway and was not found to be significantly segregated (UDOI = 0.635, p = 0.64, Figures 2A,B; Tables 4, 5). In contrast, focal areas of Laysan and black-footed albatross breeding at Midway were significantly segregated (UDOI = 0.004, p < 0.01), with limited overlap in the focal areas of both species occurring in regions south of the central and western Aleutian Islands. Laysan and black-footed albatross breeding at Tern showed significant segregation of both foraging and focal areas (UDOI = 0.189, p < 0.01 for foraging areas; UDOI = 0, p < 0.01 for focal areas; Figures 2C,D; Table 4).
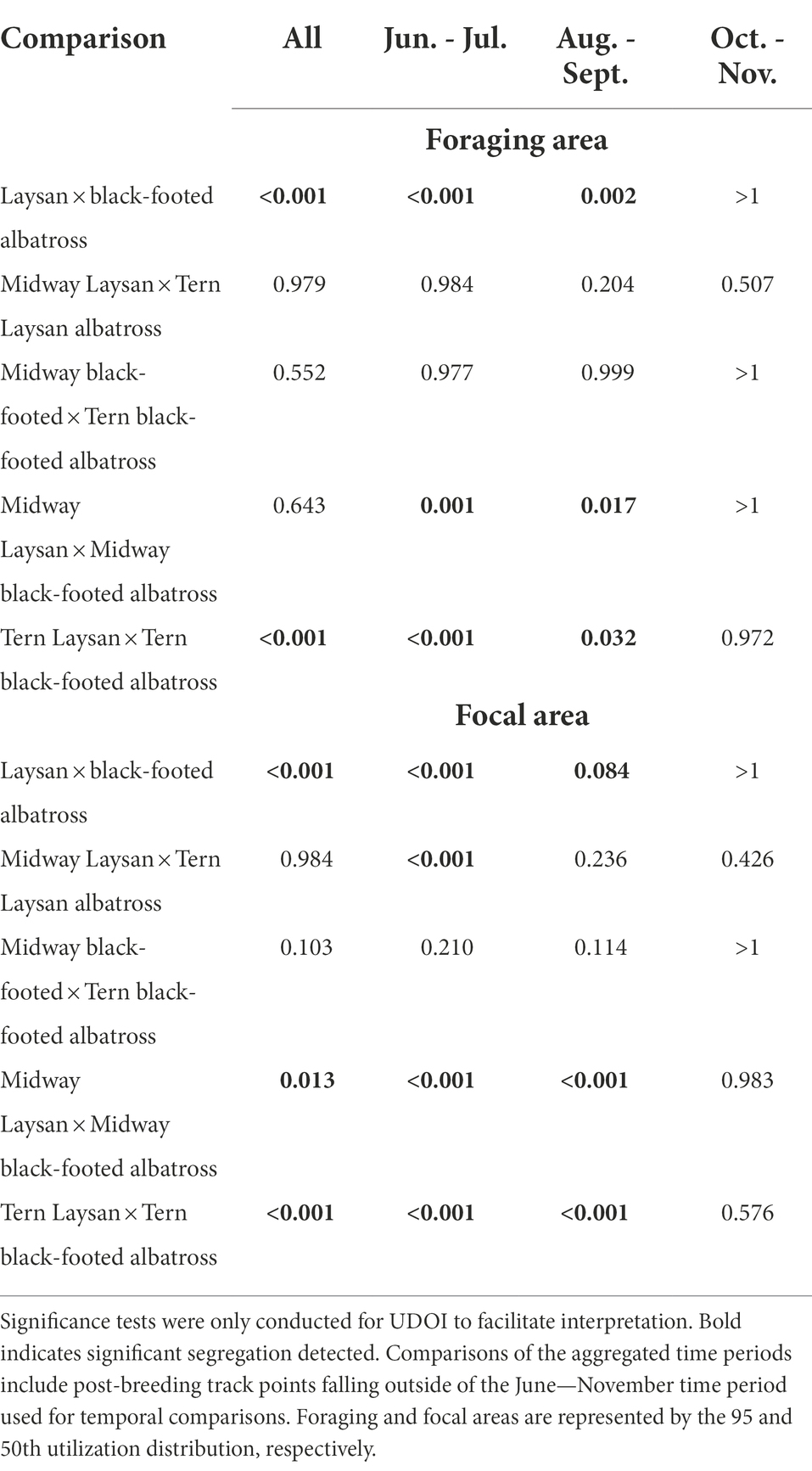
Table 5. p values for comparisons conducted using the CRW randomization method by bi-monthly periods and in aggregate (aggregate category includes all post-breeding points, including those outside the June–November temporal comparisons).
The spatial distributions of both species showed temporal changes throughout the post-breeding period (Figure 3). For both species, conspecifics breeding at different colonies showed more segregation of focal areas from June through September, and showed more overlap in October and November. Black-footed albatross primarily used habitats in the eastern North Pacific in June and July, and then additionally used waters of the central and western North Pacific from August through November, exhibiting particularly dispersed distributions in October and November. Laysan albatross primarily used habitat in the central Pacific from June through September, and then dispersed to include habitats in more western and northern regions of the North Pacific in October and November. Both species from both breeding colonies showed increased use of the Bering Sea in October and November. During the first 4 months of the post-breeding period, Laysan and black-footed albatrosses showed significant at-sea segregation in both foraging and focal areas (Table 5), with Laysan albatross primarily occurring in the central western North Pacific and black-footed albatross primarily occurring further east. However, during October and November, the two species showed more overlap in their habitats, particularly around the central Aleutian Islands and in the Bering Sea.
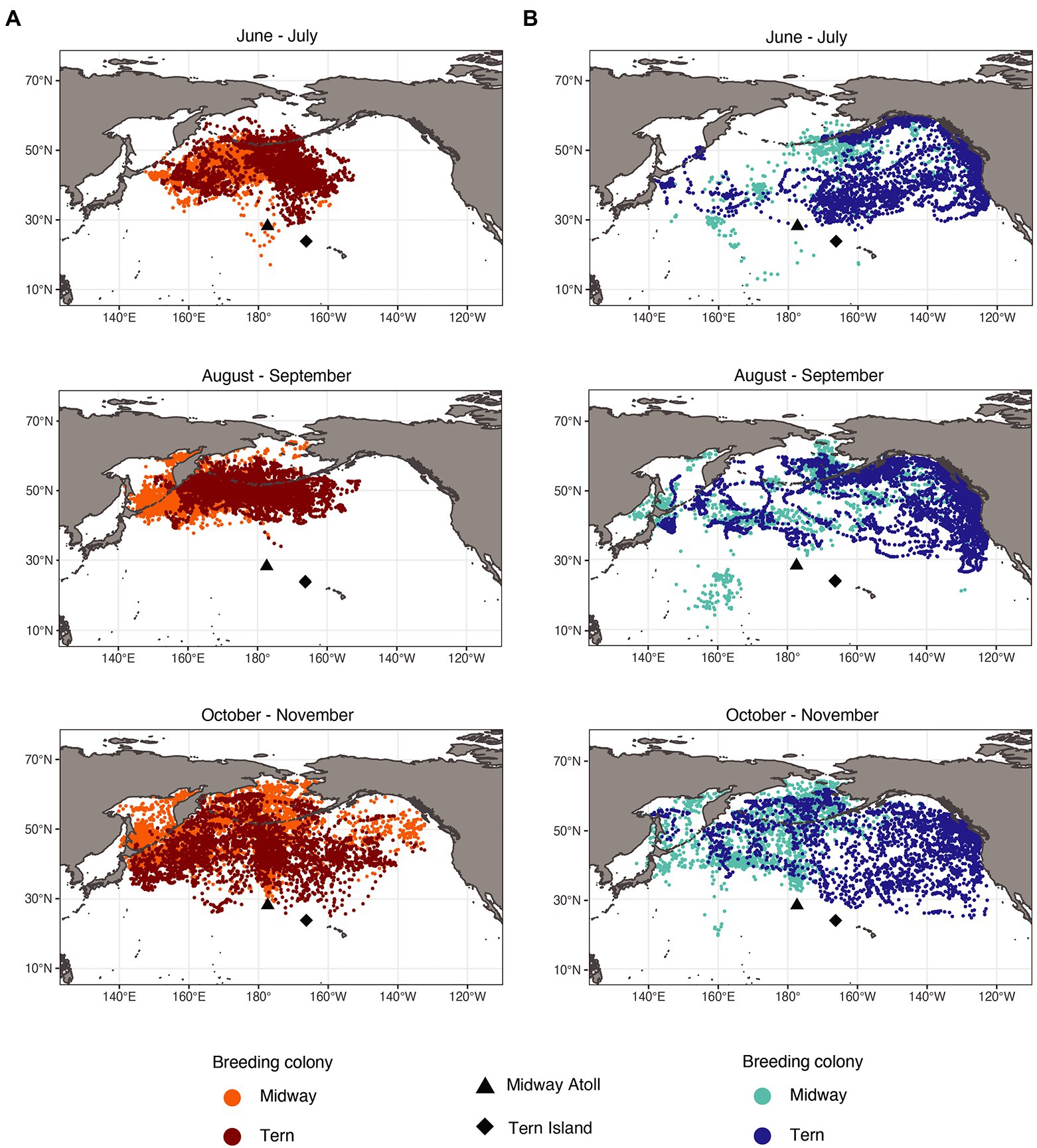
Figure 3. Laysan albatross (A) and black-footed albatross (B) post-breeding locations by month and breeding colony. Laysan and black-footed albatross breeding at Midway both left and returned to the breeding colony later than those breeding at Tern. As a result of departure and return dates, fewer Laysan albatross from Midway were tracked in June, and fewer Laysan albatross from Tern were tracked in November. Similarly, fewer black-footed albatross from Midway were tracked in June and July, and fewer black-footed albatross from Tern were tracked in November.
The foraging areas of Laysan and black-footed albatross breeding at Midway showed significant segregation in June/July and August/September but not in October/November and not when data were assessed over the full post-breeding period (Table 5). Laysan and black-footed albatross showed significant segregation when assessed over the full post-breeding period (aggregated Laysan and aggregated black-footed foraging and focal areas; Tern Laysan and Tern black-footed foraging and focal areas; and Midway Laysan and Midway black-footed focal areas), showing significant segregation from June/July until the final bimonthly period (October–November), at which point distributions showed considerable overlap. For both species, there was considerable overlap in habitat use of albatross from the two colonies through time in both foraging and focal areas, though we found significant segregation of habitats used by Laysan albatross breeding at Tern and Midway in June/July.
Chlorophyll-a of habitats used by black-footed albatross breeding at Tern were highly variable from June through October and both species at both colonies used regions with higher Chl-a during August and September (Figure 4A). Laysan albatross breeding at Tern used habitats with slightly lower Chl-a than those breeding at Midway throughout the post-breeding period (Figure 4A). Habitat use in relation to wind speed was highly similar across both species and breeding colonies and showed little variation throughout the post-breeding period, with the exception of marginally faster wind speeds in albatross habitat during October and November (Figure 4B). Black-footed albatross breeding at Tern generally used habitats with warmer SSTs than those breeding at Midway, particularly in June through August (Figure 4C). Within colonies, SST in habitats used by Laysan albatross was broadly similar from June to September, though SST was somewhat lower and more variable in October and November for Laysan albatross breeding at Tern (Figure 4C).
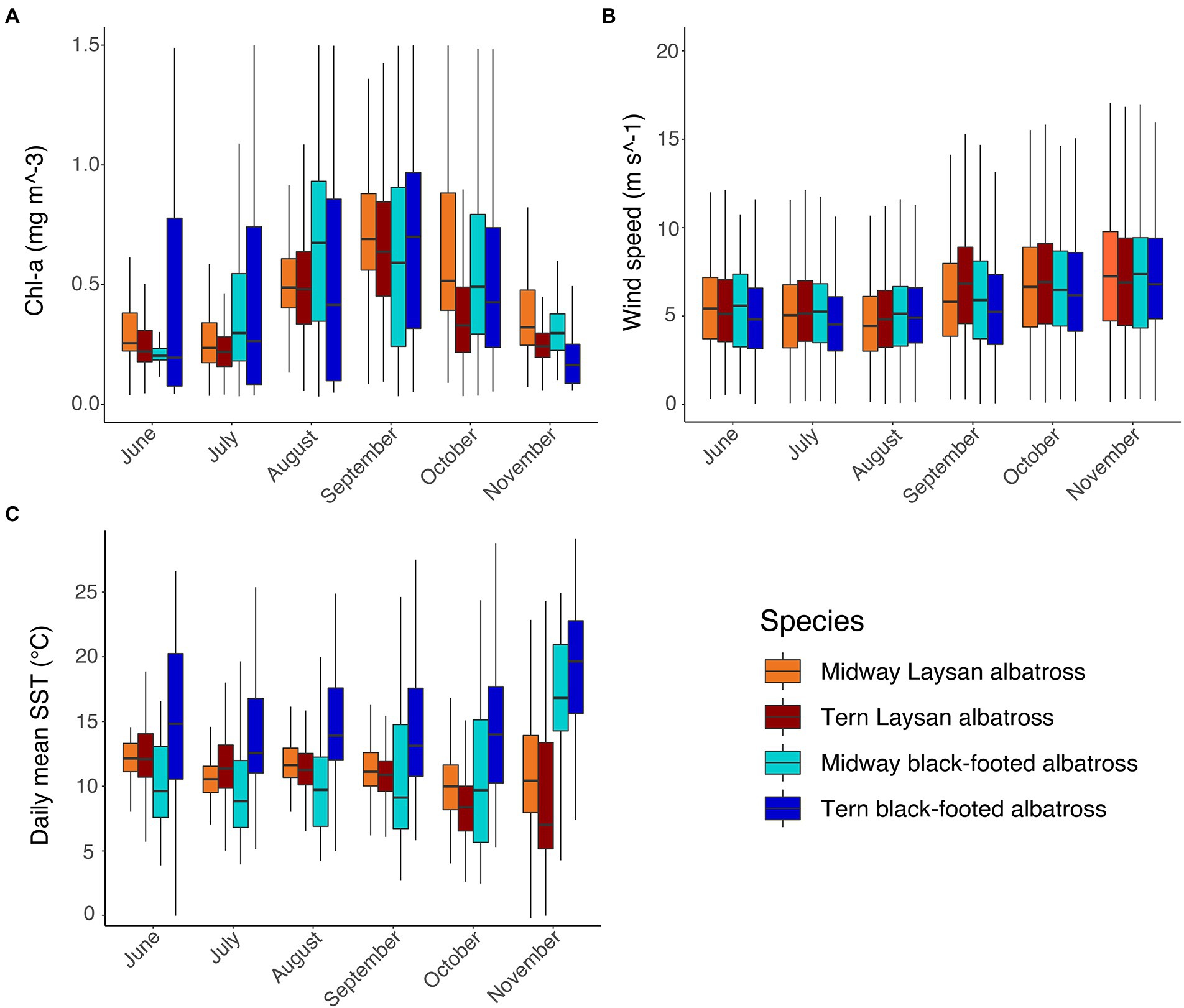
Figure 4. Environmental characteristics in post-breeding habitat of Laysan and black-footed albatross by month, species, and breeding colony: (A) Chlorophyll-a concentrations (mg m−3), (B) wind speed (m s−1), and (C) SST (°C).
Discussion
Spatial segregation in post-breeding distributions of Laysan and black-footed albatross
Our results provide a comprehensive assessment of the post-breeding distributions of Laysan and black-footed albatross in the central North Pacific and contribute to the growing literature on habitat use and dispersal of post-breeding seabirds. While previous work has examined post-breeding dispersal within a more limited scope (shorter 1–2 year study periods or species comparisons from a single breeding colony; Young et al., 2009; Gutowsky et al., 2014b; Henry et al., 2021), here we assess GLS data from 143 individual tracks, encompassing the entire post-breeding period over 5 years at two breeding colonies, representing the most comprehensive analysis to date that integrates tracking data from multiple north Pacific albatross colonies. Our findings revealed that from June to September, Laysan and black-footed albatross exhibited divergent patterns of habitat use during post-breeding both within and across breeding colonies, in both foraging and focal areas. These spatial differences resulted in distinct geographic separation of oceanographic habitat.
Resource partitioning during the breeding period has long been recognized in sympatrically breeding colonial seabirds (Ashmole, 1971; Schoener, 1974), and this work demonstrates that resource partitioning between Laysan and black-footed albatrosses is maintained during the post-breeding period. The mechanism driving this partitioning between species remains unclear, as environmental variables investigated here did not suggest consistent differences at the species-level. Laysan and black-footed albatross are both generalist predators that consume a wide array of prey types, such as mesopelagic fish, squid, crustaceans, coelenterates, and scavenged fisheries offal, but have shown preferences for different prey types while foraging near their breeding grounds (Harrison et al., 1983; Arata et al., 2009; Conners et al., 2018). Though generalists, these species have been shown to forage on different prey types during the highly constrained chick-brood phase of the breeding season (with black-footed albatrosses consuming more flying fish eggs and crustaceans than Laysan albatrosses that primarily target mesopelagic squid and fish), though this differentiation in diet is not present while birds are incubating eggs (Conners et al., 2018). Habitat partitioning may occur as a result of different prey types being targeted, a causal relationship which is not necessarily detectable through the analysis of the environmental variables selected for this study. Inter-colony density-dependent competitive interactions are one mechanism that could account for this partitioning (Wakefield et al., 2013).
Spatial segregation between breeding colonies of Laysan and black-footed albatross
Tracking studies of marine megafauna are often conducted at singular locations or study colonies, which could bias our understanding of spatial distributions if habitat use is not consistent across regions, populations or colonies (Hart and Hyrenbach, 2009; Bolton et al., 2019; Hays et al., 2020). We found that the degree of habitat segregation varies between different breeding colonies and species. For example, we observed differences, though no significant segregation, in the focal areas of black-footed albatross breeding at Tern and Midway (Figures 2B,D; Table 5). Previous studies of breeding black-footed albatross from Tern highlighted the importance of coastal areas of the northeastern Pacific to their foraging habitat (Fernández et al., 2001; Hyrenbach et al., 2002; Naughton et al., 2007; Arata et al., 2009; Hyrenbach et al., 2010) but we found that focal areas of black-footed albatross from Midway occurred in the Bering Sea and along the Aleutian Islands. Fischer et al. (2009) found this region to be important to black-footed albatross in the post-breeding period. The colony of origin of those birds was unknown, though results here suggest they likely were part of the Midway breeding colony. Laysan albatross from Tern and Midway showed similar patterns of habitat use in foraging areas, though focal areas between colonies showed significant segregation in June/July. Henry et al. (2021) compared at-sea distributions of Laysan albatross from Tern Island and Guadalupe Island (which was not in the foraging range of albatross in this study) and also found moderate overlap of foraging areas and minimal overlap of focal areas during the post-breeding period. Young et al. (2009) observed similar patterns across colonies at two breeding sites in the main Hawaiian Islands, finding greater overlap of broad foraging ranges during the non-breeding period compared to <50% overlap at the focal utilization distribution level. Together, these findings highlight the importance of including multiple breeding colonies of a species when assessing the at-sea habitat use of seabirds (e.g., Young et al., 2009, 2015; Mendez et al., 2017, 2020; Henry et al., 2021).
Environmental variables characterizing post-breeding habitats of Laysan and black-footed albatross
We found divergent patterns of habitat use in relation to SST and Chl-a across species-colony groups. Colony-level differences were observed in the SST of habitats used by black-footed albatross, with black-footed albatross from Tern consistently foraging in warmer water than their conspecifics breeding at Midway, divergent trends that were not observed in Laysan albatross breeding at different colonies. These differences are reflected in the metrics of spatial overlap, as black-footed albatross between colonies showed more segregation at the focal level (though observed segregation was not found to be significant). Habitat modeling can be used to predict locations where albatross are likely to occur based on environmental drivers (e.g., Žydelis et al., 2011), but differences in species-environment relationships between colonies may require data from multiple colonies to comprehensively understand habitat requirements, as our results show. Additionally, our results suggest that species-environmental relationships changed seasonally, particularly for Chl-a, which showed considerable seasonal variability in albatross habitat. Differences in environmental variables in albatross habitat over the course of the post-breeding period and differences in SST occurring in habitats used by black-footed albatross at Tern and Midway violate the assumption that species-environmental relationships hold constant across space and time. Caution needs to be taken when generalizing these species’ preferred foraging habitat.
Our findings also suggest that some environmental characteristics of Laysan and black-footed albatross habitat differ from patterns observed during the breeding period. Using data from albatross tagged at Tern, Hyrenbach et al. (2002) and Kappes et al. (2015) found that black-footed albatross used warmer habitats than Laysan albatrosses during breeding. While this held true for black-footed albatross from Tern during post-breeding, black-footed albatross from Midway used habitats with lower median SST than that of both Laysan colonies for 4 of the 6 post-breeding months. We found that wind did not vary considerably between habitats used by either species at either breeding colony, and align with Kappes et al. (2015) who found that wind had a relatively small impact on Laysan and black-footed albatross foraging behavior during the breeding period. While wind likely influences Laysan and black-footed albatross distribution (Suryan et al., 2008; Thorne et al., 2016), the low variability we observed within albatross habitat may reflect the fact that albatrosses are consistently selecting regions of moderate to high wind speed.
Temporal trends in spatial distributions of post-breeding Laysan and black-footed albatrosses
Both Laysan and black-footed albatross had more dispersed distributions late in the post-breeding period (October/November), and as a result any at-sea segregation observed within or between species earlier in the post-breeding season did not occur during this time frame. While changes in distributions during this time period may represent some transitory portions of tracks representing gradual travel to and from the breeding sites, we removed directional travel by eliminating track points in periods during which recorded SST changed rapidly. As such, the broad distributions observed are unlikely to be fully explained by directional travel from foraging areas to the colony (Figure 3). Further, these dispersed distributions were not observed early in post-breeding when birds were transiting to and arriving in foraging areas. Regardless of the drivers of these distributions, our results suggest that both Laysan and black-footed albatross are widely distributed and share habitat across the North Pacific in October/ November, and thus threats to these species such as by-catch risk due to overlap with fisheries are less localized during this time.
Conclusion
Assessing species distributions across the span of their annual cycle is critical to building an effective conservation strategy. This study provides a comprehensive analysis of the post-breeding distribution of Laysan and black-footed albatross breeding at Midway Atoll and Tern Island. We found significant at-sea segregation between these two species, both across and within breeding colonies, indicating that resource partitioning persists during post-breeding. Patterns of habitat use in relation environmental characteristics differed between black-footed albatrosses breeding at different colonies, highlighting that species-environmental relationships may vary across space and time. Temporal patterns of species’ distributions revealed that any observed at-sea segregation diminished in the latter months of the post-breeding period, when albatross from both species and colonies showed dispersed distributions. Our results provide a clearer picture of the post-breeding distribution of Laysan and black-footed albatross than previously available, information which can assist managers in understanding albatross habitat use and assessing spatial components of threats such as bycatch risk. This work highlights the importance of sampling across time periods and across colonies, populations, or social groups when conducting analyses of distribution and habitat use, particularly for protected species for which management and conservation may be impacted by our understanding of their distribution.
Data availability statement
The datasets presented in this study can be found in online repositories. The names of the repository/repositories and accession number(s) can be found at: BirdLife International (http://www.seabirdtracking.org), under dataset ID numbers 1927, 1928, 1929, and 1930.
Ethics statement
The animal study was reviewed and approved by UC Santa Cruz–IACUC and San Jose State University–IACUC.
Author contributions
FJ modeled GLS data and conducted overlap analyses. FJ, JS, and LT conducted correlated random walk analyses. MC, CC, MG, and SS conducted fieldwork. EH and SB helped to draft the manuscript. DC assisted with data collection and coordination. SS led and organized fieldwork and coordinated the study. LT oversaw analyses, manuscript preparation, and synthesis. All authors contributed to the article and approved the submitted version.
Funding
This research was part of the Tagging of Pacific Pelagics (TOPP) program, funded in part by the Moore Foundation, Packard Endowment Grant to UCSC, the National Ocean Partnership Program (N00014–02–1–1012), and the Office of Naval Research (N00014–00–1–0880 and N00014–03–1–0651). All protocols employed in this study were approved by the Institutional Animal Care and Use Committees at UCSC.
Acknowledgments
We thank Ellie Heywood for her input and help with spatial analyses in R, and for assistance with coding. We would like to acknowledge and thank Scott Seganti, Sarah Youngren, Ruth Brown, Dan Rapp, Caitlin Kroeger, and Abram Fleishman for their field work contributions at Tern Island and Sarah Gutowsky for her field work at Midway Atoll.
Conflict of interest
The authors declare that the research was conducted in the absence of any commercial or financial relationships that could be construed as a potential conflict of interest.
Publisher’s note
All claims expressed in this article are solely those of the authors and do not necessarily represent those of their affiliated organizations, or those of the publisher, the editors and the reviewers. Any product that may be evaluated in this article, or claim that may be made by its manufacturer, is not guaranteed or endorsed by the publisher.
Supplementary material
The Supplementary material for this article can be found online at: https://www.frontiersin.org/articles/10.3389/fevo.2022.1028317/full#supplementary-material
Footnotes
References
Antolos, M., Shaffer, S. A., Weimerskirch, H., Tremblay, Y., and Costa, D. P. (2017). Foraging behavior and energetics of albatrosses in contrasting breeding environments. Front. Mar. Sci. 4:414. doi: 10.3389/fmars.2017.00414
Arata, J. A., Sievert, P. R., and Naughton, M. B. (2009). Status Assessment of Laysan and Black-Footed Albatrosses, North Pacific Ocean, 1923–2005. Virginia: US Geological Survey Reston.
Barger, C. P., Young, R. C., Will, A., Ito, M., and Kitaysky, A. S. (2016). Resource partitioning between sympatric seabird species increases during chick-rearing. Ecosphere 7:e01447. doi: 10.1002/ecs2.1447
Barnes, J. P. (2004). How flies the albatross - the flight mechanics of dynamic soaring. SAE Technical Papers.
Block, B. A., Jonsen, I. D., Jorgensen, S. J., Winship, A. J., Shaffer, S. A., Bograd, S. J., et al. (2011). Tracking apex marine predator movements in a dynamic ocean. Nature 475, 86–90. doi: 10.1038/nature10082
Bolton, M., Conolly, G., Carroll, M., Wakefield, E. D., and Caldow, R. (2019). A review of the occurrence of inter-colony segregation of seabird foraging areas and the implications for marine environmental impact assessment. IBIS 161, 241–259. doi: 10.1111/ibi.12677
Breed, G. A., Bowen, W. D., McMillan, J. I., and Leonard, M. L. (2006). Sexual segregation of seasonal foraging habitats in a non-migratory marine mammal. Proc. R. Soc. B Biol. Sci. 273, 2319–2326. doi: 10.1098/rspb.2006.3581
Burger, A. E., and Shaffer, S. A. (2008). Application of tracking and data-logging Technology in Research and Conservation of seabirds. Auk 125, 253–264. doi: 10.1525/auk.2008.1408
Calenge, C. (2006). The package “adehabitat” for the R software: a tool for the analysis of space and habitat use by animals. Ecol. Model. 197, 516–519. doi: 10.1016/j.ecolmodel.2006.03.017
Camphuysen, K. C. J., Shamoun-Baranes, J., van Loon, E. E., and Bouten, W. (2015). Sexually distinct foraging strategies in an omnivorous seabird. Mar. Biol. 162, 1417–1428. doi: 10.1007/s00227-015-2678-9
Ceia, F. R., Paiva, V. H., Ceia, R. S., Hervías, S., Garthe, S., Marques, J. C., et al. (2015). Spatial foraging segregation by close neighbours in a wide-ranging seabird. Oecologia 177, 431–440. doi: 10.1007/s00442-014-3109-1
Clay, T. A., Manica, A., Ryan, P. G., Silk, J. R. D., Croxall, J. P., Ireland, L., et al. (2016). Proximate drivers of spatial segregation in non-breeding albatrosses. Sci. Rep. 6, 1–13. doi: 10.1038/srep29932
Conners, M. G. (2015). Comparative behavior, diet, and post-breeding strategies of two sympatric North Pacific albatross species (Phoebastria sp.) [UC Santa Cruz]. Conners_ucsc_0036E_10949
Conners, M. G., Goetsch, C., Budge, S. M., Walker, W. A., Mitani, Y., Costa, D. P., et al. (2018). Fisheries exploitation by albatross quantified with lipid analysis. Frontiers in marine. Front. Mar. Sci. 5:113. doi: 10.3389/fmars.2018.00113
Conners, M. G., Hazen, E. L., Costa, D. P., and Shaffer, S. A. (2015). Shadowed by scale: subtle behavioral niche partitioning in two sympatric, tropical breeding albatross species. Move. Ecol. 3, 1–20. doi: 10.1186/s40462-015-0060-7
Croxall, J. P., Silk, J. R. D., Phillips, R. A., Afanasyev, V., and Briggs, D. R. (2005). Global circumnavigations: tracking year-round ranges of nonbreeding albatrosses. Science 307, 249–250. doi: 10.1126/science.1106042
Cullen, A. C., Frey, H. C., and Frey, C. H. (1999). Probabilistic Techniques in Exposure Assessment: A Handbook for Dealing With Variability and Uncertainty in Models and Inputs. New York: Springer Science & Business Media.
Dehnhard, N., Achurch, H., Clarke, J., Michel, L. N., Southwell, C., Sumner, M. D., et al. (2020). High inter-and intraspecific niche overlap among three sympatrically breeding, closely related seabird species: generalist foraging as an adaptation to a highly variable environment? J. Anim. Ecol. 89, 104–119. doi: 10.1111/1365-2656.13078
DeLong, R. L., Stewart, B. S., and Hill, R. D. (1992). Documenting migrations of northern elephant seals using day length. Mar. Mamm. Sci. 8, 155–159. doi: 10.1111/j.1748-7692.1992.tb00375.x
Diefenbach, D. R., Long, E. S., Rosenberry, C. S., Wallingford, B. D., and Smith, D. R. (2008). Modeling distribution of dispersal distances in male white-tailed deer. J. Wildl. Manag. 72, 1296–1303. doi: 10.2193/2007-436
Edwards, A. E., Fitzgerald, S. M., Parrish, J. K., Klavitter, J. L., and Romano, M. D. (2015). Foraging strategies of laysan albatross inferred from stable isotopes: implications for association with fisheries. PLoS One 10:e0133471. doi: 10.1371/journal.pone.0133471
Egevang, C., Stenhouse, I. J., Phillips, R. A., Petersen, A., Fox, J. W., and Silk, J. R. D. (2010). Tracking of Arctic terns Sterna paradisaea reveals longest animal migration. Proc. Natl. Acad. Sci. 107, 2078–2081. doi: 10.1073/pnas.0909493107
Fernández, P., Anderson, D. J., Sievert, P. R., and Huyvaert, K. P. (2001). Foraging destinations of three low-latitude albatross (Phoebastria) species. J. Zool. 254, 391–404. doi: 10.1017/S0952836901000899
Fieberg, J., and Kochanny, C. (2005). Quantifying home-range overlap: the importance of the utilization distribution. J. Wildl. Manag. 69, 1346–1359. doi: 10.2193/0022-541x(2005)69[1346:qhotio]2.0.co;2
Finkelstein, M., Keitt, B. S., Croll, D. A., Tershy, B., Jarman, W. M., Rodriguez-Pastor, S., et al. (2006). Albatross species demonstrate regional differences in North Pacific marine contamination. Ecol. Appl. 16, 678–686. doi: 10.1890/1051-0761(2006)016[0678:ASDRDI]2.0.CO;2
Fischer, K. N. (2008). Marine habitat use of black-footed and Laysan albatrosses during the post-breeding season and their spatial and temporal overlap with commercial fisheries [Oregon State University]. Available at: https://ir.library.oregonstate.edu/concern/graduate_thesis_or_dissertations/zg64tp53d
Fischer, K. N., Suryan, R. M., Roby, D. D., and Balogh, G. R. (2009). Post-breeding season distribution of black-footed and Laysan albatrosses satellite-tagged in Alaska: inter-specific differences in spatial overlap with North Pacific fisheries. Biol. Conserv. 142, 751–760. doi: 10.1016/j.biocon.2008.12.007
Frederiksen, M., Descamps, S., Erikstad, K. E., Gaston, A. J., Gilchrist, H. G., Grémillet, D., et al. (2016). Migration and wintering of a declining seabird, the thick-billed murre Uria lomvia, on an ocean basin scale: conservation implications. Biol. Conserv. 200, 26–35. doi: 10.1016/j.biocon.2016.05.011
Fromant, A., Bost, C.-A., Bustamante, P., Carravieri, A., Cherel, Y., Delord, K., et al. (2020). Temporal and spatial differences in the post-breeding behaviour of a ubiquitous southern hemisphere seabird, the common diving petrel. R. Soc. Open Sci. 7:200670. doi: 10.1098/RSOS.200670
Furness, R. W., and Birkhead, T. R. (1984). Seabird colony distributions suggest competition for food supplies during the breeding season. Nature 311, 655–656. doi: 10.1038/311655a0
Gelaro, R., McCarty, W., Suárez, M. J., Todling, R., Molod, A., Takacs, L., et al. (2017). The modern-era retrospective analysis for research and applications, version 2 (MERRA-2). J. Clim. 30, 5419–5454. doi: 10.1175/JCLI-D-16-0758.1
Gilmour, M. E., Felis, J., Hester, M., Young, L., and Adams, J. (2022). Laysan albatross exhibit complex behavioral plasticity in the subtropical and subarctic North Pacific Ocean. Mar. Ecol. Prog. Ser. 697, 125–147. doi: 10.3354/MEPS14148
González-Solís, J., Croxall, J. P., Oro, D., and Ruiz, X. (2007). Trans-equatorial migration and mixing in the wintering areas of a pelagic seabird. Front. Ecol. Environ. 5, 297–301. doi: 10.1890/1540-9295(2007)5[297:TMAMIT]2.0.CO;2
Grémillet, D., Wilson, R. P., Wanless, S., and Chater, T. (2000). Black-browed albatrosses, international fisheries and the Patagonian shelf. Mar. Ecol. Prog. Ser. 195, 269–280. doi: 10.3354/meps195269
Gutowsky, S. E., Gutowsky, L. F. G., Jonsen, I. D., Leonard, M. L., Naughton, M. B., Romano, M. D., et al. (2014a). Daily activity budgets reveal a quasi-flightless stage during non-breeding in Hawaiian albatrosses. Move. Ecol. 2, 23–14. doi: 10.1186/s40462-014-0023-4
Gutowsky, S. E., Leonard, M. L., Conners, M. G., Shaffer, S. A., and Jonsen, I. D. (2015). Individual-level variation and higher-level interpretations of space use in wide-ranging species: an albatross case study of sampling effects. Frontiers in marine. Science 2:93. doi: 10.3389/fmars.2015.00093
Gutowsky, S. E., Tremblay, Y., Kappes, M. A., Flint, E. N., Klavitter, J., Laniawe, L., et al. (2014b). Divergent post-breeding distribution and habitat associations of fledgling and adult black-footed albatrosses Phoebastria nigripes in the North Pacific. IBIS 156, 60–72. doi: 10.1111/ibi.12119
Halpin, L. R., Ross, J. D., Ramos, R., Mott, R., Carlile, N., Golding, N., et al. (2021). Double-tagging scores of seabirds reveals that light-level geolocator accuracy is limited by species idiosyncrasies and equatorial solar profiles. Methods Ecol. Evol. 12, 2243–2255. doi: 10.1111/2041-210X.13698
Harrison, C. S., Hida, T. S., and Seki, M. P. (1983). Hawaiian seabird feeding ecology. Wildl. Monogr. 85, 3–71.
Hart, K. M., and Hyrenbach, K. D. (2009). Satellite telemetry of marine megavertebrates: the coming of age of an experimental science. Endanger. Species Res. 10, 9–20. doi: 10.3354/esr00238
Hays, G. C., Rattray, A., and Esteban, N. (2020). Addressing tagging location bias to assess space use by marine animals. J. Appl. Ecol. 57, 1981–1987. doi: 10.1111/1365-2664.13720
Hazen, E. L., Abrahms, B., Brodie, S., Carroll, G., Welch, H., and Bograd, S. J. (2021). Where did they not go? Considerations for generating pseudo-absences for telemetry-based habitat models. Move. Ecol. 9, 1–13. doi: 10.1186/S40462-021-00240-2/TABLES/2
Hazen, E. L., Palacios, D. M., Forney, K. A., Howell, E. A., Becker, E., Hoover, A. L., et al. (2017). WhaleWatch: a dynamic management tool for predicting blue whale density in the California current. J. Appl. Ecol. 54, 1415–1428. doi: 10.1111/1365-2664.12820
Hemson, G., Johnson, P., South, A., Kenward, R., Ripley, R., and Macdonald, D. (2005). Are kernels the mustard? Data from global positioning system (GPS) collars suggests problems for kernel home-range analyses with least-squares cross-validation. J. Anim. Ecol. 74, 455–463. doi: 10.1111/j.1365-2656.2005.00944.x
Henry, R. W., Shaffer, S. A., Antolos, M., Félix-Lizárraga, M., Foley, D. G., Hazen, E. L., et al. (2021). Successful Long-distance breeding range expansion of a top marine predator. Front. Ecol. Evol. 9:274. doi: 10.3389/fevo.2021.620103
Hinke, J. T., Polito, M. J., Goebel, M. E., Jarvis, S., Reiss, C. S., Thorrold, S. R., et al. (2015). Spatial and isotopic niche partitioning during winter in chinstrap and Adélie penguins from the South Shetland Islands. Ecosphere 6:art125. doi: 10.1890/es14-00287.1
Hyrenbach, K. D. (2001). Albatross response to survey vessels: implications for studies of the distribution, abundance, and prey consumption of seabird populations. Mar. Ecol. Prog. Ser. 212, 283–295. doi: 10.3354/meps212283
Hyrenbach, K. D., and Dotson, R. C. (2003). Assessing the susceptibility of female black-footed albatross (Phoebastria nigripes) to longline fisheries during their post-breeding dispersal: an integrated approach. Biol. Conserv. 112, 391–404. doi: 10.1016/S0006-3207(02)00337-3
Hyrenbach, K. D., Fernandez, P., and Anderson, D. J. (2002). Oceanographic habitats of two sympatric North Pacific albatrosses during the breeding season. Mar. Ecol. Prog. Ser. 233, 283–301. doi: 10.3354/meps233283
Hyrenbach, D., Hester, M., Adams, J., Michael, P., Vanderlip, C., Keiper, C., et al. (2010). Synthesis of habitat use by black-footed albatross tracked from Cordell Bank National Marine Sanctuary (2004–2008) and Kure atoll seabird sanctuary 2008: A special report to NOAA. NOAA.
Jessopp, M., Arneill, G. E., Nykänen, M., Bennison, A., and Rogan, E. (2020). Central place foraging drives niche partitioning in seabirds. Oikos 129, 1704–1713. doi: 10.1111/oik.07509
Jouventin, P., and Dobson, F. S. (2002). Why breed every other year? The case of albatrosses. Proc. R. Soc. Lond. Ser. B Biol. Sci. 269, 1955–1961. doi: 10.1098/rspb.2002.2080
Jouventin, P., and Weimerskirch, H. (1990). Satellite tracking of wandering albatrosses. Nature 343, 746–748. doi: 10.1038/343746a0
Kappes, M. A., Shaffer, S. A., Tremblay, Y., Foley, D. G., Palacios, D. M., Bograd, S. J., et al. (2015). Reproductive constraints influence habitat accessibility, segregation, and preference of sympatric albatross species. Move. Ecol. 3, 34–24. doi: 10.1186/s40462-015-0063-4
Kappes, M. A., Shaffer, S. A., Tremblay, Y., Foley, D. G., Palacios, D. M., Robinson, P. W., et al. (2010). Hawaiian albatrosses track interannual variability of marine habitats in the North Pacific. Prog. Oceanogr. 86, 246–260. doi: 10.1016/j.pocean.2010.04.012
Kareiva, P. M., and Shigesada, N. (1983). Analyzing insect movement as a correlated random walk. Oecologia 56, 234–238. doi: 10.1007/BF00379695
Kuroda, N. (1955). Observations on pelagic birds of the Northwest Pacific. Condor 57, 290–300. doi: 10.2307/1364734
Lamb, J. S., Satgé, Y. G., and Jodice, P. G. R. (2020). Seasonal variation in environmental and behavioural drivers of annual-cycle habitat selection in a nearshore seabird. Divers. Distrib. 26, 254–266. doi: 10.1111/DDI.13015
Langston, N. E., and Rohwer, S. (1996). Molt-breeding tradeoffs in albatrosses: life history implications for big birds. Oikos 76, 498–510. doi: 10.2307/3546343
Lewis, S., and Benvenuti, S., Dall–Antonia, L., Griffiths, R., Money, L., Sherratt, T. N., Wanless, S., and Hamer, K. C. (2002). Sex-specific foraging behaviour in a monomorphic seabird. Proc. R. Soc. Lond. Ser. B Biol. Sci. 269, 1687–1693. doi: 10.1098/rspb.2002.2083
Lewis, S., Sherratt, T. N., Hamer, K. C., and Wanless, S. (2001). Evidence of intra-specific competition for food in a pelagic seabird. Nature 412, 816–819. doi: 10.1038/35090566
Limpert, E., Stahel, W. A., and Abbt, M. (2001). Log-normal distributions across the sciences: keys and clues: on the charms of statistics, and how mechanical models resembling gambling machines offer a link to a handy way to characterize log-normal distributions, which can provide deeper insight into v. Bioscience 51, 341–352. doi: 10.1641/0006-3568(2001)051[0341:LNDATS]2.0.CO;2
Linnebjerg, J. F., Fort, J., Guilford, T., Reuleaux, A., Mosbech, A., and Frederiksen, M. (2013). Sympatric breeding auks shift between dietary and spatial resource partitioning across the annual cycle. PLoS One 8:e72987. doi: 10.1371/journal.pone.0072987
Lisovski, S., Hewson, C. M., Klaassen, R. H. G., Korner-Nievergelt, F., Kristensen, M. W., and Hahn, S. (2012). Geolocation by light: accuracy and precision affected by environmental factors. Methods Ecol. Evol. 3, 603–612. doi: 10.1111/j.2041-210X.2012.00185.x
Mendez, L., Borsa, P., Cruz, S., de Grissac, S., Hennicke, J., Lallemand, J., et al. (2017). Geographical variation in the foraging behaviour of the pantropical red-footed booby. Mar. Ecol. Prog. Ser. 568, 217–230. doi: 10.3354/meps12052
Mendez, L., Prudor, A., and Weimerskirch, H. (2020). Inter-population variation in the behaviour of adult and juvenile red-footed boobies Sula sula. IBIS 162, 460–476. doi: 10.1111/ibi.12779
Merkel, B., Phillips, R. A., Descamps, S., Yoccoz, N. G., Moe, B., and Strøm, H. (2016). A probabilistic algorithm to process geolocation data. Move. Ecol. 4:26. doi: 10.1186/s40462-016-0091-8
Naughton, M. B., Romano, M. D., and Zimmerman, T. S. (2007). A conservation action plan for the black-footed albatross (Phoebastria nigripes) and Laysan albatross (P. immutabilis), Ver 1.0. USFWS report, 40.
Nur, N., Jahncke, J., Herzog, M. P., Howar, J., Hyrenbach, K. D., Zamon, J. E., et al. (2011). Where the wild things are: predicting hotspots of seabird aggregations in the California current system. Ecol. Appl. 21, 2241–2257. doi: 10.1890/10-1460.1
Oppel, S., Beard, A., Fox, D., Mackley, E., Leat, E., Henry, L., et al. (2015). Foraging distribution of a tropical seabird supports Ashmole’s hypothesis of population regulation. Behav. Ecol. Sociobiol. 69, 915–926. doi: 10.1007/s00265-015-1903-3
Pettex, E., Lambert, C., Fort, J., Dorémus, G., and Ridoux, V. (2019). Spatial segregation between immatures and adults in a pelagic seabird suggests age-related competition. J. Avian Biol. 50:jav.01935. doi: 10.1111/jav.01935
Phillips, R. A., Silk, J. R. D., Croxall, J. P., Afanasyev, V., and Bennett, V. J. (2005). Summer distribution and migration of nonbreeding albatrosses: individual consistencies and implications for conservation. Ecology 86, 2386–2396. doi: 10.1890/04-1885
Phillips, R., Silk, J., Croxall, J., Afanasyev, V., and Briggs, D. (2004). Accuracy of geolocation estimates for flying seabirds. Mar. Ecol. Prog. Ser. 266, 265–272. doi: 10.3354/meps266265
Phillips, R. A., Silk, J. R. D., Phalan, B., Catry, P., and Croxall, J. P. (2004). Seasonal sexual segregation in two Thalassarche albatross species: competitive exclusion, reproductive role specialization or foraging niche divergence? Proceedings of the Royal Society of London. Proc. Roy. Soc. London Ser. B. Biol. Sci. 271, 1283–1291. doi: 10.1098/rspb.2004.2718
Phillips, R. A., Xavier, J. C., and Croxall, J. P. (2003). Effects of satellite transmitters on albatrosses and petrels. Auk 120, 1082–1090. doi: 10.2307/4090279
Pinaud, D., and Cherel, Y. (2005). Effect of environmental variability on habitat selection, diet, provisioning behaviour and chick growth in yellow-nosed albatrosses. Mar. Ecol. Prog. Ser. 298, 295–304. doi: 10.3354/meps298295
Powell, R. A. (2000). “Animal home ranges and territories and home range estimators” in Research Techniques in Animal Ecology: Controversies and Consequences, vol. 442. eds. B. Luigi and K. F. Todd (New York: Columbia University Press), 65–110.
Rayner, M. J., Taylor, G. A., Thompson, D. R., Torres, L. G., Sagar, P. M., and Shaffer, S. A. (2011). Migration and diving activity in three non-breeding flesh-footed shearwaters Puffinus carneipes. J. Avian Biol. 42, 266–270. doi: 10.1111/j.1600-048X.2010.05238.x
Richardson, P. L., Wakefield, E. D., and Phillips, R. A. (2018). Flight speed and performance of the wandering albatross with respect to wind. Movement. Ecology 6, 1–15. doi: 10.1186/s40462-018-0121-9
Roberts, J. J., Best, B. D., Dunn, D. C., Treml, E. A., and Halpin, P. N. (2010). Marine geospatial ecology tools: an integrated framework for ecological geoprocessing with ArcGIS, python, R, MATLAB, and C++. Environ. Model. Softw. 25, 1197–1207. doi: 10.1016/j.envsoft.2010.03.029
Robertson, G. S., Bolton, M., Grecian, W. J., Wilson, L. J., Davies, W., and Monaghan, P. (2014). Resource partitioning in three congeneric sympatrically breeding seabirds: foraging areas and prey utilization. Auk 131, 434–446. doi: 10.1642/AUK-13-243.1
Schoener, T. W. (1974). Resource partitioning in ecological communities. Science 185, 27–39. doi: 10.1126/science.185.4145.27
Seaman, D. E., Millspaugh, J. J., Kernohan, B. J., Brundige, G. C., Raedeke, K. J., and Gitzen, R. A. (1999). Effects of sample size on kernel home range estimates. J. Wildl. Manag. 63:739. doi: 10.2307/3802664
Seaman, D. E., and Powell, R. A. (1996). An evaluation of the accuracy of kernel density estimators for home range analysis. Ecology 77, 2075–2085. doi: 10.2307/2265701
Shaffer, S. A., Cockerham, S., Warzybok, P., Bradley, R. W., Jahncke, J., Clatterbuck, C. A., et al. (2017). Population-level plasticity in foraging behavior of western gulls (Larus occidentalis). Move. Ecol. 5:27. doi: 10.1186/s40462-017-0118-9
Shaffer, S. A., Tremblay, Y., Awkerman, J. A., Henry, R. W., Teo, S. L. H., Anderson, D. J., et al. (2005). Comparison of light-and SST-based geolocation with satellite telemetry in free-ranging albatrosses. Mar. Biol. 147, 833–843. doi: 10.1007/s00227-005-1631-8
Shaffer, S. A., Tremblay, Y., Weimerskirch, H., Scott, D., Thompson, D. R., Sagar, P. M., et al. (2006). Migratory shearwaters integrate oceanic resources across the Pacific Ocean in an endless summer. Proc. Natl. Acad. Sci. U. S. A. 103, 12799–12802. doi: 10.1073/pnas.0603715103
Suryan, R. M., Anderson, D. J., Shaffer, S. A., Roby, D. D., Tremblay, Y., Costa, D. P., et al. (2008). Wind, waves, and wing loading: morphological specialization may limit range expansion of endangered albatrosses. PLoS One 3:e4016. doi: 10.1371/journal.pone.0004016
Suryan, R. M., and Fischer, K. N. (2010). Stable isotope analysis and satellite tracking reveal interspecific resource partitioning of nonbreeding albatrosses off Alaska. Can. J. Zool. 88, 299–305. doi: 10.1139/Z10-002
Thorne, L. H., Conners, M. G., Hazen, E. L., Bograd, S. J., Antolos, M., Costa, D. P., et al. (2016). Effects of El Niño-driven changes in wind patterns on North Pacific albatrosses. J. R. Soc. Interface 13:20160196. doi: 10.1098/rsif.2016.0196
Thorne, L. H., Hazen, E. L., Bograd, S. J., Foley, D. G., Conners, M. G., Kappes, M. A., et al. (2015). Foraging behavior links climate variability and reproduction in North Pacific albatrosses. Move. Ecol. 3, 1–15. doi: 10.1186/s40462-015-0050-9
Wahl, T. R., Ainley, D. G., Benedict, A. H., and DeGange, A. R. (1989). Associations between seabirds and water-masses in the northern Pacific Ocean in summer. Mar. Biol. 103, 1–11. doi: 10.1007/BF00391059
Wakefield, E. D., Bodey, T. W., Bearhop, S., Blackburn, J., Colhoun, K., Davies, R., et al. (2013). Space partitioning without territoriality in gannets. Science 341, 68–70. doi: 10.1126/science.1236077
Warham, J. (1996). The Behaviour, Population Biology and Physiology of the Petrels. San Diego, California: Academic Press.
Weber, S. B., Richardson, A. J., Brown, J., Bolton, M., Clark, B. L., Godley, B. J., et al. (2021). Direct evidence of a prey depletion “halo” surrounding a pelagic predator colony. Proc. Natl. Acad. Sci. 118:e2101325118. doi: 10.1073/PNAS.2101325118
Weimerskirch, H., Guionnet, T., Martin, J., Shaffer, S. A., and Costa, D. P. (2000). Fast and fuel efficient? Optimal use of wind by flying albatrosses. Proc. R. Soc. B Biol. Sci. 267, 1869–1874. doi: 10.1098/rspb.2000.1223
Weimerskirch, H., Louzao, M., de Grissac, S., and Delord, K. (2012). Changes in wind pattern alter albatross distribution and life-history traits. Science 335, 211–214. doi: 10.1126/science.1210270
Weimerskirch, H., Salamolard, M., Sarrazin, F., and Jouventin, P. (1993). Foraging strategy of wandering albatrosses through the breeding season: a study using satellite telemetry. Auk 110, 325–342. doi: 10.1093/auk/110.2.325
Welch, D. W., and Eveson, J. P. (1999). An assessment of light-based geoposition estimates from archival tags. Can. J. Fish. Aquat. Sci. 56, 1317–1327. doi: 10.1139/f99-049
Wiley, A. E., Welch, A. J., Ostrom, P. H., James, H. F., Stricker, C. A., Fleischer, R. C., et al. (2012). Foraging segregation and genetic divergence between geographically proximate colonies of a highly mobile seabird. Oecologia 168, 119–130. doi: 10.1007/s00442-011-2085-y
Wilson, R. P., Ducamp, J. J., Rees, W. G., Culik, B. M., and Niekamp, K. (1992). Estimation of location: Global coverage using light intensity. Wildlife Telemetry: Remote Monitoring and Tracking of Animals, 131–134.
Wood, A. G., Naef-Daenzer, B., Prince, P. A., and Croxall, J. P. (2000). Quantifying habitat use in satellite-tracked pelagic seabirds: application of kernel estimation to albatross locations. J. Avian Biol. 31, 278–286. doi: 10.1034/j.1600-048X.2000.310302.x
Wooller, R. D., Bradley, J. S., and Croxall, J. P. (1992). Long-term population studies of seabirds. Trends Ecol. Evol. 7, 111–114. doi: 10.1016/0169-5347(92)90143-Y
Worton, B. J. (1989). Kernel methods for estimating the utilization distribution in home-range studies. Ecology 70, 164–168. doi: 10.2307/1938423
Worton, B. J. (1995). Using Monte Carlo simulation to evaluate kernel-based home range estimators. J. Wildl. Manag. 59:794. doi: 10.2307/3801959
Young, H. S., Maxwell, S. M., Conners, M. G., and Shaffer, S. A. (2015). Pelagic marine protected areas protect foraging habitat for multiple breeding seabirds in the Central Pacific. Biol. Conserv. 181, 226–235. doi: 10.1016/j.biocon.2014.10.027
Young, H. S., McCauley, D. J., Dirzo, R., Dunbar, D., and Shaffer, S. A. (2010). Niche partitioning among and within sympatric tropical seabirds revealed by stable isotope analysis. Mar. Ecol. Prog. Ser. 416, 285–294. doi: 10.3354/meps08756
Young, L. C., Vanderlip, C., Duffy, D. C., Afanasyev, V., and Shaffer, S. A. (2009). Bringing home the trash: do colony-based differences in foraging distribution lead to increased plastic ingestion in Laysan albatrosses? PLoS One 4, 11–13. doi: 10.1371/journal.pone.0007623
Žydelis, R., Lewison, R. L., Shaffer, S. A., Moore, J. E., Boustany, A. M., Roberts, J. J., et al. (2011). Dynamic habitat models: using telemetry data to project fisheries bycatch. Proc. R. Soc. B Biol. Sci. 278, 3191–3200. doi: 10.1098/rspb.2011.0330
Keywords: habitat, overlap, distribution, loggers, movement ecology, spatial ecology, post-breeding, segregation
Citation: Jordan FD, Shaffer SA, Conners MG, Stepanuk JEF, Gilmour ME, Clatterbuck CA, Hazen EL, Palacios DM, Tremblay Y, Antolos M, Foley DG, Bograd SJ, Costa DP and Thorne LH (2022) Divergent post-breeding spatial habitat use of Laysan and black-footed albatross. Front. Ecol. Evol. 10:1028317. doi: 10.3389/fevo.2022.1028317
Edited by:
José F. Fontanari, University of São Paulo, BrazilReviewed by:
Edison Barbieri, Agência de Agronegócio e Tecnologia de São Paulo (APTA), BrazilSandro Martinelli Reia, Purdue University, United States
Copyright © 2022 Jordan, Shaffer, Conners, Stepanuk, Gilmour, Clatterbuck, Hazen, Palacios, Tremblay, Antolos, Foley, Bograd, Costa and Thorne. This is an open-access article distributed under the terms of the Creative Commons Attribution License (CC BY). The use, distribution or reproduction in other forums is permitted, provided the original author(s) and the copyright owner(s) are credited and that the original publication in this journal is cited, in accordance with accepted academic practice. No use, distribution or reproduction is permitted which does not comply with these terms.
*Correspondence: Fredrick Dallas Jordan, fredrickdallasjordan@gmail.com
† Deceased
‡Present addresses: Morgan E. Gilmour, Santa Cruz Field Station, U.S. Geological Survey, Western Ecological Research Center (WERC), Santa Cruz, CA, United States
Corey A. Clatterbuck, Office of Information Management and Analysis, California State Water Resources Control Board, Sacramento, CA, United States