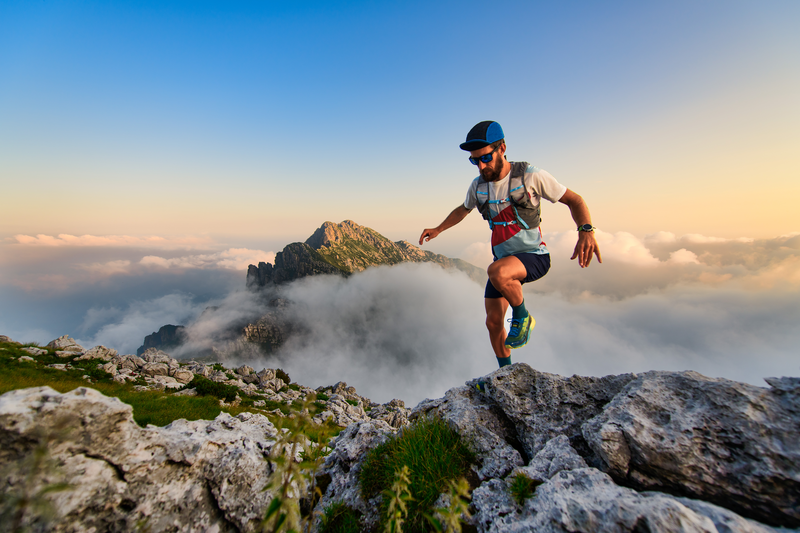
95% of researchers rate our articles as excellent or good
Learn more about the work of our research integrity team to safeguard the quality of each article we publish.
Find out more
ORIGINAL RESEARCH article
Front. Ecol. Evol. , 23 November 2022
Sec. Chemical Ecology
Volume 10 - 2022 | https://doi.org/10.3389/fevo.2022.1027201
This article is part of the Research Topic Chemical Ecology of Arthropods, Plants, and Fungi: Bioactivity and Importance of Natural Products View all 5 articles
Plants are not only used as energy and nutrient resources for herbivores. Plants can be ingested because of their activity against host parasites and other pathogens. This so-called medicinal role of plants is well reported in ethnopharmacology and under-reported in wild animals. More studies on wild animals are needed because any plant in the world contains bioactive compounds, and probably all plants, no matter how toxic they are, experience herbivory. For example, we tested the activity of extracts and essential oils from Papaver rhoeas and Echium plantagineum against a selection of laboratory pathogens because Great bustards Otis tarda preferred these plants during the mating season, with male fecal droppings showing a higher frequency of P. rhoeas particles than the fecal droppings of females. We hypothesized that P. rhoeas could be helpful for males in the mating season if any part of this plant harbors bioactivity against parasites and other pathogens. Males’ immune system is weakened during the mating season because of their investment in secondary sexual characters and sexual display. As a first exploration of the bioactivity of these plants, we evaluated extracts of both plants against a sample of laboratory models, including a flagellated protozoon (Trichomonas gallinae), a nematode (Meloidogyne javanica) and a fungus (Aspergillus niger). Non-polar and polar extracts of the aerial parts of P. rhoeas, especially the extracts of flowers and capsules, and the extracts of leaves and flowers of E. plantagineum showed activity against nematodes and trichomonads. The bioactivity of plants against parasites could explain the foraging behavior of stressed animals. The chemical communication underpinning the capacity of fauna to recognize those plants is far less known.
Several studies have reported anti-parasitic effects of plants both in livestock (Russo et al., 2009 and references therein; Villalba et al., 2014) and wild animals (Lefèvre et al., 2012; Huffman et al., 2013). These effects are usually due to secondary metabolites such as terpenes, alkaloids, glycosides and tannins (Githiori et al., 2006), which are typically non-nutritional and frequently have toxic or even poisonous properties (de Roode et al., 2013). To distinguish whether these substances are ingested inadvertently or as a means to confront infectious diseases would require very detailed behavioral observations that are extremely difficult to record in wild species under field conditions (Abbott, 2014). Experimental studies are routinely performed with livestock. Positive influence of phytogenic compounds against pathogens and improving the health has been described in poultry (Latek et al., 2022 and references therein) and cattle (e.g., Villalba et al., 2010; Villalba and Landau, 2012). In wild birds the phytogenics’ positive effects are under-explored. Wild birds use taste and smell as chemosensory criteria (Mrazova et al., 2019; Grieves et al., 2022), but the chemical signals and chemoreceptors of avian olfaction are poorly understood. Field examples must first be identified to enable the subsequent study of chemical interactions between wild birds and plants with active compounds against avian pathogens.
One of the wild species in which self-medication has been suggested is the Great Bustard (Otis tarda). Bustards are affected by a wide range of pathogens and parasites, including numerous bacteria, protozoa, helminths and arthropods. García-Montijano et al. (2002), reported that parasitic and fungal infections could cause up to 30% mortality of free-living Great Bustards in Spain (14.3% of death in juveniles was due to small intestine obstruction with cestodes, and fungal pneumonia and air saculitis by Aspergillus fumigatus were responsible for 16.7% of death in adults). The protozoans Eimeria spp., Cryptosporidium spp., Giardia spp., Trichomonas spp., Histomonas spp. and Hexamita spp. are commonly present in their digestive tracts (Bailey, 2008; Whitman et al., 2019). Trichomonas gallinae and Entamoeba anatis cause oropharyngeal diseases in bustards (Silvanose et al., 1998). Cestodes (Hispaniolepis sp., Raillietina cesticillus, Schistometra (Otiditaenia) conoides, and Idiogenes otidis), nematodes (Capillaria sp., Syngamus trachea, Cyathostoma sp., Heterakis gallinae, H. isolonche, Aprocta orbitalis, Oxyspirura hispanica, and Trichostrongylus sp.), insects (including mallophagan such as Otilipeurus turmalis, and fly maggots such as Lucilia sericata) and ticks (Rhipicephalus sanguineus, and Hyalomma sp.) also infest bustards (Cordero del Campillo et al., 1994; Bailey, 2008; Alonso and Palacín, 2015; Whitman et al., 2019).
In previous studies (Bravo et al., 2014; Whitman et al., 2019) we investigated the antiparasitic and antimicrobial effects of blister beetles (Meloidae), which are avoided by most animals but consumed by great bustards. Blister beetles contain cantharidin, a highly toxic monoterpene that could have positive effects in controlling the parasite load of the host (Whitman et al., 2019). We found that great bustards males consumed more blister beetles than females, which suggested that males could use cantharidin to reduce their parasite load (Bravo et al., 2014), increase their sexual activity and attractiveness (Heneberg, 2016) or both. Standard pharmacological experiments in controlled trials are required to shed light on this possibility. Even so, these studies will represent a difficult, if not impossible, task with great bustards due to legal restrictions on their capture and handling, and the stress they undergo in enclosures.
Although great bustards eat beetles and other invertebrates in spring and summer, their diet is mostly vegetarian over the whole year, including mainly leaves of green plants (Lane et al., 1999; Rocha et al., 2005; Bravo et al., 2016; see review of Faragó, 2019). Some of these plants could also have properties that might help great bustards control their parasite load. For example, Lane et al. (1999) found that most weeds were consumed in proportions expected by their abundance, but noted two exceptions, the common poppy Papaver rhoeas and the purple viper’s bugloss Echium plantagineum. The diet of great bustards showed much higher foraging ratios of these two plants in April compared to other months (Figure 1). Since April is the month when males reach the peak of display activity and most mating events occur (Magaña, 2007; Alonso et al., 2010a,b), we hypothesize that consumption of these two plants could help them reduce the negative effects of parasites during this important phase of their reproductive cycle, as is the case with blister beetles. Males could benefit from the medicinal properties of these plants during a period when they are subjected to high stress and reduced immune resistance to infections due to their strenuous investment in sexual display (Folstad and Karter, 1992; Roberts et al., 2004; Alonso et al., 2010a,b). Parasite over-loads may indeed affect the fitness or reproductive status of the host (Boyce, 1990; Coop and Holmes, 1996), and chemical compounds helping to control parasites when the threat of parasitism is greater may have benefits on health and reproductive success (Castella et al., 2008a,b).
Figure 1. Monthly foraging ratios of corn poppy (P. rhoeas, open circles) and purple viper’s bugloss (E. plantagineum, filled circles) peaked in April, coinciding with maximum mating activity of great bustards (vertical bars, right axis). Figure composed with plant consumption and availability (Lane et al., 1999), and weekly mating attempts (Magaña, 2007). Vertical bars represent the number of mating attempts per hour, defined as males full-displaying in very close proximity to one or more females (<3 m) with at least one of them showing obvious precopulatory behavior, independently of whether these attempts were followed by effective copulations or not. The number of mating attempts is a reliable indicator of mating success, as indicated by the significant correlation between rates of effective copulations and copulation attempts (Alonso et al., 2010b). The mating attempt rate before and after the period depicted in this figure is negligible.
To test this hypothesis, our present study had the following objectives. First, we examined whether the consumption of P. rhoeas and E. plantagineum is male-biased. A higher proportion of these plants in the diet of males would support the hypothesis that males use them to reduce their parasite load during their strenuous display season, in a similar way as that suggested for cantharidin (Bravo et al., 2014). Alternatively, or complementary to a self-medication function affecting only males, both plants might also have a prophylactic function for females during the peak copulation period in April when exposure to sexually transmitted diseases is highest. Second, we investigated whether extracts and essential oils (EOs) from P. rhoeas and E. plantagineum have antiparasitic activity against the flagellated protozoon Trichomonas gallinae, the endoparasitic nematode Meloidogyne javanica, and the fungus Aspergillus niger. Third, we analyzed the chemical composition of extracts to identify what components could be active against pathogens. The fulfilment of these three objectives is not a demonstration of self-medication in Great bustards, which requires a standard pharmacological experimental setup, but a necessary step towards it. The bioactivity of plants against laboratory parasites and other pathogens not specific to bustards could point to additional utilities of their plant preferences other than nutrition.
Flowering Papaver rhoeas and Echium plantagineum plants were collected in May 2019 during the mating season, near Valdetorres del Jarama (Spain) and at one of the largest great bustard leks of central Spain (Figure 2, UTM: 40.708987, −3.495657; Alonso and Alonso, 1996; Lane et al., 2001; Martín et al., 2002; Bautista et al., 2017). These plant species were chosen based on the great bustard’s preferences (Figure 1) and their potential toxicity as reported in the literature (Grauso et al., 2020, see Discussion).
Figure 2. Great Bustard distribution in the Iberian Peninsula (green areas). E. plantagineum (right) and P. rhoeas (middle) were collected in central Spain (red star: Valdetorres del Jarama, Madrid) within the Great Bustard area distribution. Map distribution from SEO/BirdLife (2015) updated with own data.
The plant parts were separated (flowers and leaves, plus capsules in the case of P. rhoeas), and dried at 40°C for 48 h. Ground (40 g) flowers were extracted with methanol; and 50 and 30 g of ground leaves and capsules were sequentially extracted with solvents of increasing polarity: hexane (Hex), ethyl acetate (EtOAc) and ethanol (EtOH) in a Soxhlet apparatus, to obtain extracts with different compositions from apolar to polar. The solvents were evaporated under vacuum to give dry extracts.
Essential oils (volatile extracts, EOs) were obtained by hydrodistillation for 2 h in a Clevenger-type apparatus according to the European Pharmacopoeia. Ground flowers (100 g) were distilled with 2 l of water. The residual water (infusion, IF) was freeze-dried (100 ml) or subjected to a liquid–liquid extraction (150 ml) with dichloromethane (DCM; l50 ml × 3 times).
T. gallinae trophozoites isolated from a common wood pigeon (Columba palumbus) from Central Spain were employed to determine the antiprotozoal activity. The strain was maintained by serial passes in 10 ml tubes with trypticase yeast maltose (TYM) medium. The tests were carried out 48 h after a serial pass when trophozoites were at exponential growth phase. One hundred and fifty μl of a culture containing 500,000 trophozoites/ml were placed in each well of microtiter flat-bottom plates. Extracts and essential oils (EO) were tested at several concentrations (400, 200, and 100 μg/ml) dissolved in 2 μl dimethyl sulphoxide (DMSO). After 24 h incubation at 37°C the antiprotozoal activity was obtained by the colourimetric tetrazolium (MTT) method. Briefly, plates were centrifuged and the TYM was carefully removed. Ten μL of MTT/phenazine methosulfate PMS (1.25 mg/ml; 0.1 mg PMS) were added to each well. After incubation for 15 min to allow the reduction of MTT, 50 μl of a sodium dodecyl sulphate (SDS) solution (10 g SDS, 1,5 μl HCl; 100 ml distilled water) were added to dissolve formazan crystals obtained as a result of the reduction of MTT. Once the crystals had dissolved (15–30 min), the plate was read on a spectrophotometer at 620 nm. The activity was calculated as percentage growth inhibition as follows:
where At is the absorbance of treated wells, Ac the absorbance of control wells (not treated) and Ab the absorbance of blank wells (culture medium and vehicle only). All assays were carried out in triplicate and were repeated at least three times independently to confirm the results. Because At, Ab and Ac were means, the resulting Growth inhibition should collect and combine the errors of the means. Uncertainties of means were propagated (Harvard, 2007) using a first-order series approximation with the command AroundReplace[expr, {s1 → Around[x1,δ1], s2→ Around[x2,δ2],…}] in software Mathematica online (Wolfram Research, 2022) as follows:
The nematicidal activity has been evaluated against the root-knot nematode Meloidogyne javanica as a target model of endoparasitic nematode because it is relatively easy to maintain under laboratory conditions in the absence of animal models. Additionally, plant and animal parasitic nematodes share many of the traits that contribute to their successful parasitism despite host differences, including their ability to arrest development at key stages of their life cycle; ability to locate and infect their host and ability to manipulate their host interface for nutrient uptake and long-term survival (Maule and Curtis, 2011). The expected effect does not preclude investigating activity against specific animal nematodes, as those cited in the main studies (e.g., Huffman et al., 2013; Villalba et al., 2014).
The nematodes used to determine anthelminthic activity came from a population of Meloidogyne javanica maintained on Solanum lycopersicum plants (var. Marmande) in pot cultures at 25°C and 70% relative humidity (RH). The bioassays were carried out as described by Andrés et al. (2018). Freeze-dried infusions were dissolved in distilled water at concentrations adjusted to water-infusion yield (27 and 8 mg/ml for P. rhoeas and E. plantagineum). Nematode inoculum (500 J2 in water) was filtered (25 μm), and the nematodes were suspended in 500 μl of lyophilized infusion treatments. Four aliquots (100 μl) of the nematode suspension (approximately 100 J2) and controls (water) were placed in 96-well plates. The organic extracts were prepared by dilution in a DMSO-Tween solution (0.5% Tween 20 in DMSO) at 20 mg/ml and 5 μl of this solution were added to 95 μl of water containing 90–150 nematodes (final concentration of 1 mg/ml and 5% DMSO). Treatments were replicated four times. As a control, four wells were treated with the water/DMSO/Tween 20 in the same volume as the tests. The plates were covered to prevent evaporation and were maintained in the dark at 25°C. After 24, 48, and 72 h, the dead J2 were counted under a binocular microscope. The nematicidal activity data are presented as percent dead J2s corrected according to Schneider-Orelli’s formula (Schneider-Orelli, 1947). Dose–response curves were obtained by testing serial dilutions covering an activity range between 100% and below 50%.
Aspergillus niger (donated by Dr. K. Leiss, Wageningen University) was used to determine de antifungal activity (Sainz et al., 2019). The fungal parasite model Aspergillus niger belongs to one of the most widely distributed genera of endophytic fungi pathogen responsible for aspergillosis in animals, including birds (García-Montijano et al., 2002; Bennett, 2010); avian aspergilloses are caused mainly by A. fumigatus and less commonly by A. flavus or A. niger (Tokarzewski et al., 2012). A variety of captive and free-roaming wild birds show aspergillosis from A. niger (Arne et al., 2021).
A. niger was maintained in Petri dishes with potato dextrose agar (PDA) medium. The tests were carried out 24 h after pass when the spores were not yet observed. Two ml of PDA mixed with 40 μl of each extract / EO suspended in ethanol and 10 μl of MTT at 5 mg/ml were filled in 12 well plates. The final concentration of extract/EO in the well was 1 mg/ml. Each test was performed in quadruplicate. Once the agar had solidified, the mycelium was inoculated by pitting. The plates were maintained in the dark at 28°C for 48 h. Finally, the plates were scanned for reading and the diameter of the colonies was measured with the Image-J program (Schneider et al., 2012). The percentage of mycelium inhibition was calculated according to Schneider-Orelli’s formula:
where b is the average of diameters of the treatment replicates and k the average of diameters of the control replicates.
To obtain the spores, 5 ml of saline solution were added in three-day PDA Petri dish of A. niger culture. The spores were resuspended in saline solution with a sterile swab and filtered to remove the mycelium. Extracts and EOs were dissolved in 1% DMSO at a final concentration of 800 μg/ml in each well of a 96-well microplate. Each well contained 20 μl of spores (at a concentration of 7.5 × 105 spores/ml), 100 μl of RPMI medium and 160 μl of extract. The assays were made in quadruplicate. After 48 h incubation at 30°C, the plates were revealed using the MTT colorimetric method. Briefly, 25 μl of RPMI with 5 mg/ml of MTT and 1 mg of menadione were added to each well and incubated 3 h in the dark. The medium was removed and 200 μl of 5% isopropanol in HCl (1 M) were added. After 30 min of incubation at room temperature and gentle agitation, the optical density was measured at 490 nm. For the calculation of the percentage of germination inhibition, the Schneider-Orelli’s formula adapted was used:
where b is the average absorbance of the treatment and k the average absorbance of the control. Uncertainties of mean antifungal activities were propagated as explained before.
The essential oils and hexane extracts where analyzed by gas chromatography mass spectrometry (GC–MS) using a Shimadzu GC-2010 gas chromatograph coupled to a Shimadzu GCMS-QP2010 Ultra mass detector (electron ionization, 70 eV). Sample injections (1 μl) were carried out by an AOC-20i equipped with a 30 m × 0.25 mm i.d. capillary column (0.25 μm film thickness) Teknokroma TRB-5 (95%) dimethyl-(5%) diphenylpolisiloxane. Working conditions were as follows: split ratio (20:1), injector temperature 300°C, temperature of the transfer line connected to the mass spectrometer 250°C, initial column temperature 100°C, then heated to 290°C at 7°C/min. Full Scan was used (m/z 35–900). Electron ionization mass spectra and retention data were used to assess the identity of compounds by comparing them with those found in the Wiley 229 and NIST 17 Mass Spectral Database. All extracts (4 μg/μl) were dissolved in DCM for injection.
The alcoholic extracts (EtOH and MeOH) and aqueous extracts (infusions) where analyzed by liquid chromatography coupled to mass spectrometry (HPLC–MS) in a Shimadzu apparatus equipped with LC-20 AD pump and a CTO-10AS VP column oven coupled to a mass spectrometer with simple quadrupole as analyzer (LCMS-2020 QP), with an electrospray ionization source (ESI). An ACE 3 C18 column (150 mm × 4.6 mm, 3 μm particle size) with an ACE3 C18 analytical pre-column was used for the separation. The compounds were eluted with Methanol (LC–MS grade; MeOH): MiliQ water with 1% acetic acid starting in 10% MeOH during 5 min to continue with a gradient 10:67% MeOH during 20 min, 67:100% MeOH during 10 min, 100% MeOH during 10 min and 100:10% MeOH during 8 min, with a flow rate of 0.5 ml/min. The nitrogen flow (drying gas for solvent evaporation) was 15 l/min. The potential for the electrospray capillary was +4.50 kV and a Full Scan was used in positive mode (m/z 100–700) with a potential of 1.40 kV and a capillary temperature of 250°C. The stock solutions of the extracts were injected at 0.25 mg/ml with a 5 μl injection through an automatic injector (SIL-20A XR). All extracts were dissolved in 100% MeOH for injection.
We relied on previously collected data (Bravo et al., 2016) with which we carried out a different statistical analysis. The effect of sex was calculated in that study on the proportion of green vegetables (legumes, weeds, and cereal plants), arthropods, seeds, and fruits, whereas here, two weed species (P. rhoeas and E. plantagineum) were analyzed independently of one another. We look for a sex effect on the proportional consumption of each plant because males could eat them in higher proportions due to a bigger physiological stress.
Fecal samples were collected at nine sites within the study area (Bravo et al., 2016), but in the present study they were grouped for analysis in just two seasons: mating (April) and non-mating (November–January and July, respectively pre-mating and post-mating in Bravo et al., 2016). Here we used generalized linear mixed models (GLMMs, binomial error, logit link function) for P. rhoeas and E. plantagineum consumption by great bustards. Since the nine sites differed in the availability of P. rhoeas and E. plantagineum, collecting site was included as a random factor in the GLMMs. Likelihood-ratio tests were used to assess the significant effect of sex. Sample sizes were 81 female and 97 male fecal droppings during the mating season, and 222 female and 223 male fecal droppings during the non-mating season.
P. rhoeas and E. plantagineum particles were identified and quantified by micro-histological techniques in fecal samples (see Bravo et al., 2016 and references therein), including the number of microscope fields in which they occurred. The proportion of each plant in the total dry weight of the droppings of males and females was calculated. We tested the effect of sex on the proportional consumption of each plant in fecal samples. The GLMM was repeated in the non-mating season as a complementary control of the main hypothesis: during mating, males could eat both plants in higher proportions than females to counteract their higher parasite load due to stress, whereas in the non-mating season this sex difference could be smaller or even statistically non-significant.
Using different solvents, a total of 17 extracts were obtained (Table 1) containing low polarity (hexane, Hex), medium polarity (ethyl acetate, EtOAc), polar (ethanol, EtOH and methanol, MeOH, dichloromethane infusion IDCM), water soluble (infusion, I, infusion-freeze dried, IFD) and volatile (essential oil, EO) compounds from the plants. The highest yields corresponded to the methanolic/ethanolic extracts. P. rhoeas flowers (MeOH and IFD) gave higher yields than E. plantagineum flowers.
Table 1. Extracts and yields (% plant dry weight) obtained in each part of P. rhoeas and E. plantagineum.
The extracts were tested in vitro against a range of target organisms. Table 2 shows their effects on T. gallinae and A. niger (mycelial growth). T. gallinae was the most sensitive target organism, with P. rhoeas being more active than E. plantagineum. Among P. rhoeas extracts, flowers-EO (F-EO), flowers-IFD (F-IFD) and capsules-Hex (C-Hex) showed strong trichomonacidal effects, followed by flowers-MeOH (F-MeOH) and leaf-EtOH (L-EtOH) extracts (Table 2). The most active extracts from E. plantagineum were leaf-EtOH (L-EtOH) and F-IFD, followed by F-EO and leaf-EtOAc (L-EtOAc). Therefore, the trichomonacidal compounds could be (1) volatiles and apolar compounds present in the flowers and capsules of P. rhoeas (EO/Hex), polar compounds present in the flower infusion-freeze dried (IFD) of P. rhoeas and E. plantagineum and non-volatile polar compounds present in the L-EtOH leaf extract of E. plantagineum.
A. niger mycelial growth was moderately inhibited by the flower freeze dried infusion of E. plantagineum (IFD, 52%; Table 2). However, these extracts did not affect A. niger spore germination (data not shown).
Table 2. Activity of P. rhoeas (Pr) and E. plantagineum (Ep) extracts and essential oil on Trichomonas gallinae (% mortality) and Aspergillus niger (% mycelial growth inhibition).
The extracts were also tested on an endoparasite nematode model (M. javanica). The results are shown in Table 3. Freeze dried flower infusions from both plant species demonstrated strong nematicidal effects (up to 5% dilutions). Among organic extracts, the MeOH flower extract of E. plantagineum was also active against M. javanica (81.3%).
Table 3. Activity of extracts and essential oil from P. rhoeas (Pr) and E. plantagineum (Ep) on M. javanica. Results are expressed as percent mortality ± SE.
In short, P. rhoeas contains antiprotozoal and anthelmintic compounds, while E. plantagineum contains components with antiprotozoal, anthelmintic and, to a lesser extent, antifungal activity.
The GC–MS analysis of the essential oil (EO) from P. rhoeas flowers showed the presence of alkanes (36.3%), fatty acids (32.68%, with palmitic acid being the major one: 31%), alcohols (12.5%) fatty acid esters (8.0%) and triacylglycerol (3%). The flower capsules of P. rhoeas contained fatty acid esters (42.5%), alcohols (17.4%), alkanes (9.3%), palmitic acid (7.0%) and stigmastane (4.5%) in the Hex extract. Similarly, their EtOAc extract contained fatty acid esters (42%), stigmastane (11.4%), fatty acids (8.2%) and an alkane diterpene (6%; Supplementary Table S1).
The EO from E. plantagineum flowers showed a high content in alkanes (61.25%), fatty acids (3%), alcohols (1.2%), fatty acid esters (2.3%) and ketone (2.1%; Supplementary Table S2).
The HPLC-MS analysis of the polar extracts (leaves ethanolic, L-EtOH; flowers methanolic F-EtOH; flower infusion lyophilized, F-IFD; dichloromethane extract of flower infusion, F-IDCM) are shown in Supplementary Tables S3 (P. rhoeas) and S4 (E. plantagineum). To categorize the extracts, the peaks have been arbitrarily grouped as polar and medium polarity compounds according to their retention times (1–10 min and 10–50 min). The possible alkaloidal components were tentatively identified in these extracts eluted between 10 and 50 min as part of the medium polarity class.
Among P. rhoeas extracts, the freeze-dried infusion of flowers contained a high percentage of polar components (92%). The alcoholic extracts had similar amounts of polar (L-EtOH and F-MeOH, 69–68%) and medium polarity peaks (21–18%), with more possible alkaloids identified in the flowers (14%). However, most of the possible alkaloids were concentrated in the F-IFD partitioned with dichloromethane (F-IDCM, 67%, see Supplementary Table S3).
These extracts showed the presence of molecular ions compatible with previously reported alkaloids (Oh et al., 2018). The leaf EtOH extract showed (S)-3′-hydroxy-N-methylcoclaurine-like ions (4%), followed by rhoeadine /isorhoeadine (2.5%), dihydroberberine (2%), and allocryptopine (1.54%). The flower MeOH extract showed rhoeadine /isorhoeadine (8%), allocryptopine and cowlteropine (3%) compatible ions. The IDCM of the flower infusion concentrated most of the possible alkaloidal compounds, showing molecular ions compatible with rheagenine / cryptopine (49%), glaucamine /allocryptopine (7%), dihydroberberine (2%), rhoeadine /isorhoeadine (0.9%) and dihydroberberine (0.7%). The freeze-dried flower infusion IFD showed molecular ions compatible with rheagenine/cryptopine (1.64%) and allocryptopine (0.54%, see Supplementary Table S3).
E. plantagineum alcoholic extracts (L-EtOH /F-MeOH) contained different amounts of polar components (73/54%) and similar amounts with medium polarity (19/18%). Most of the tentatively identified alkaloids (except echimidine and lycopsamine, which were identified by direct comparison with an authentic sample) were concentrated in the flower organic extracts (F-MeOH/IDCM, 31/40%, see Supplementary Table S4).
Several molecular ions compatible with pyrrolicidine alkaloids (PAs) reported in E. plantagineum (Colegate et al., 2005; Skoneczny et al., 2015; Sixto et al., 2019; Mädge et al., 2020) were found in these extracts. Overall, echimidine was the most abundant. The leaf EtOH extract contained echimidine (5.8%) and molecular ions compatible with echiumine-NOx (1.87%) molecular ions. The flower MeOH showed molecular ions for echimidine (13.7%), lycopsamine (3%), and molecular ions compatible with intermedine (5.2%), heliotrine (4.7%), heliotrine-Nox (2.6%), and echiumine-NOx (1.5%). The flower IDCM extract showed molecular ions compatible with echimidine (25.6%) and molecular ions compatible with echiuplatine (5.07%), echimidine-NOx (4.15%), acetyllycopsamine (3.1%), echiumine-NOx (1.24%) and intermedine (1.12%). The flower IFD extract showed echimidine (4.93%) and molecular ions compatible with intermedine (2.62%), heliotrine-NOx (1.72%), and echiumine-NOx (1.18%, see Supplementary Table S4).
During the mating season, the dry weight proportion of Papaver rhoeas was higher in male droppings (24.9 ± 22.8%) than in female droppings (21.5 ± 23.6%, see GLMM results in Table 4). The sex difference was not significant in the non-mating season (males: 7.3 ± 13.7%, females: 6.7 ± 15.8%, Table 4). As for Echium plantagineum, the dry-weight proportion in male droppings (0.9 ± 0.2%) was marginally (p < 0.10) higher than in female droppings (0.1 ± 0.5%) in the mating season, and higher in the non-mating season (males: 1.5 ± 4.4%, females: 0.6 ± 3.8, Table 4).
Table 4. Results of generalized linear mixed models (GLMMs) of dry weight proportion of Papaver rhoeas and Echium plantagineum in great bustard fecal droppings in central Spain in each season.
We found antiparasitic effects in Papaver rhoeas and Echium plantagineum, two plants strongly preferred by great bustards during the mating season. From these plants we obtained two categories of active extracts: apolar-medium polarity (EO, Hex and EtOAc) and polar (alcoholic: EtOH/MeOH, and aqueous infusion: IFD). We investigated their chemical composition and tested their activity on a sample of common laboratory pathogens. Based on these results and a review of the antipathogenic properties of both plants, we infer that great bustards feed on them to reduce their load of pathogens.
Non-polar and polar extracts of aerial parts of P. rhoeas, especially flowers and capsules, showed activity against nematodes and trichomonads. Among non-polar extracts, the flower essential oil (EO) and the capsule hexane extract showed strong trichomonacidal effects, the effect of EO being particularly powerful. A study of P. rhoeas EO from aerial parts collected in Turkey showed phytol (52.8%), tricosane (7.8%), 2-pentadecanone (6%) and heneicosane (5.3%) as the major compounds (Dogan and Bagci, 2014), while the EO studied here was characterized by fatty acids, mostly palmitic acid, and alkanes. The non-polar extract (Hex) of the capsules mostly contained fatty acid esters. Palmitic acid has been reported as being the main component of non-polar (Hex) extracts of P. rhoeas leaves (Grauso et al., 2019).
Previous results have shown trichomonacidal effects on Trichomonas vaginalis of Nigella sativa seed oil containing fatty acids (Mahmoud et al., 2016). However, this is the first report on trichomonacidal effects of P. rhoeas lipid extracts. Trichomonads are unable to biosynthesize fatty acids and cholesterol but can incorporate these compounds from the medium without further modification (Beach et al., 1990). Therefore, externally supplied fatty acids and sterols could interfere with Trichomonads lipid metabolism.
The bioactivity of P. rhoeas polar extracts correlated with their content in polar compounds (peaks with retention times of <10 min). The alcoholic ones (L-EtOH, F-MeOH) showed trichomonacidal effects, while the F-IFD showed potent trichomonacidal and nematicidal effects. Nematicidal activity has been previously reported for aqueous extracts (4%) of P. rhoeas leaves against M. javanica (Alikarami et al., 2018). These extracts contained molecular ions compatible with reported Papaver alkaloids (Oh et al., 2018), mostly concentrated in the dichloromethane partition of the flower infusion (F-IDCM). Since the F-IDCM partition, rich in these ions, was not active, the trichomonacidal/nematicidal effects of P. rhoeas polar extracts cannot be attributed to these alkaloids-compatible ions, indicating that more polar components of the extracts are responsible for the observed effects. Among P. rhoeas components, alkaloids are the most representative, especially (+)-rhoeadine, along with N-methylasimilobine, rhoeagenine, epiberberine and canadine, depending on the plant origin (Grauso et al., 2020). Minor alkaloids included roemerine (Grauso et al., 2020), with reported antibacterial, antifungal and anthelmintic activities (Lin et al., 2014; Coban et al., 2017). Furthermore, alkaloids such as allocryptopine, potopine and berberine were nematicidal against Strongyloides stercolaris larvae (Satou et al., 2002). P. rhoeas also contains flavonoids, phenols, organic acids and vitamin C (Sanchez-Mata et al., 2012; Morales et al., 2014; Sanchez-Mata and Tardío, 2016). Flavonoids may reduce the oxidative stress and enhance immunity (Hmamou et al., 2022), so birds eat them presumably as a prophylactic drug (Catoni et al., 2008) against pathogens. Polyphenols regulate immune and inflammatory responses during enteric bacterial and parasitic infections in livestock (Williams et al., 2020), and organic acids can significantly reduce microbial contamination in turkeys (Evans et al., 2017).
Corn poppy (P. rhoeas) has been used since ancient times as a food ingredient and traditional remedy (Grauso et al., 2020), but cases of poisoning with P. rhoeas in adults, children and animals have been described (McNaughton and Harper, 1964; Couplan, 1990). Poppy poisoning in humans can cause nausea, vomiting, altered mental state, headache, convulsions, miotic pupils, lethargy and disorientation (Gunaydin et al., 2015; Koçak et al., 2016). Papaver species are actively toxic or narcotic and unpalatable to grazing animals. Animals are safe since the odour and taste of the plants render them obnoxious but there are reports of cattle and horses being poisoned by P. rhoeas (McNaughton and Harper, 1964). Nonetheless, great bustards include P. rhoeas in their diet throughout most of the year and, to our knowledge, they are not poisoned by corn poppies. The diet composition of great bustards and the activity of P. rhoeas extracts shown here support the hypothesis of a self-medication function for this plant species during the bird’s mating season (Bravo et al., 2014).
Extracts of the aerial parts of E. plantagineum (non-polar and polar) also showed trichomonacidal and nematicidal effects. The flower essential oil (EO) of E. plantagineum, with moderate trichomonacidal effects, was characterized by alkanes. Similarly to P. rhoeas extracts, the bioactivity of E. plantagineum polar extracts also correlated with their content in high polarity compounds. The alcoholic F-MeOH showed trichomonacidal effects, while the freeze-dried infusion (IFD) showed potent trichomonacidal and nematicidal effects. Two pyrrolizidine alkaloids (echimidine and lycopsamine) and PA-compatible molecular ions reported in E. plantagineum (Colegate et al., 2005; Skoneczny et al., 2015; Sixto et al., 2019; Mädge et al., 2020) were found in the polar extracts, mostly concentrated in the DCM extracted organic fraction of the flowers’ infusion (IDCM). Echimidine was the most abundant in all extracts. Since the PA-rich IDCM partition was not active, the trichomonacidal and nematicidal effects of E. plantagineum polar extracts cannot be attributed to these alkaloids. However, nematicidal effects of PAs on Meloidogyne incognita have been reported for heliotrine, lasiocarpine and monocrotaline, but these effects were dependent on the PA structure and the exposure period (168 h; Thoden et al., 2009).
E. plantagineum produces secondary metabolites, including pyrrolizidine alkaloids (PA) in the aerial parts and seeds (Colegate et al., 2005; Weston et al., 2013; Zhu et al., 2016), echimidine and echiumine N-oxide being particularly abundant (Skoneczny et al., 2015). PAs are easily reduced to free bases and are metabolized by the herbivorous cytochrome P-450 oxidases, which give rise to pyrrol alkylating intermediates. Reactive pyrroles damage cellular DNA and are dangerous to cattle, horses, sheep, pigs, and rats, affecting also humans (Cheeke and Piersongoeger, 1983; Peterson and Jago, 1984; Cheeke, 1988; Schramm et al., 2019). Harmful effects on bird health have also been described as a result of PA consumption (Pass et al., 1979; Savaris et al., 2019).
Great bustards could eat P. rhoeas and E. plantagineum based on the antipathogenic effects of these plants. The use of plants with active secondary metabolites for preventing or reducing parasite and pathogen loads (self-medication) has been described in invertebrates (Christe et al., 2003; Singer et al., 2009; Milan et al., 2012), mammals (Huffman, 1997, 2001; Villalba et al., 2017) and birds (Lafuma et al., 2001; Masello et al., 2018; Fonturbel et al., 2020). As for great bustards, Bravo et al. (2014) described for the first time a probable case of self-medication by ingestion of toxic insects. They found that bustards included two blister beetles of the family Meloidae in their diet. These beetles contain cantharidin, a highly toxic compound that can be even lethal for bustards if ingested in high doses (Sanchez-Barbudo et al., 2012). Bravo et al. (2014) found a male-biased consumption of blister beetles, and interpreted it as a way to enhance their attractiveness to females by reducing their parasite load. Before selecting a mate for copulation, a female bustard may examine the cloaca of the displaying male and usually pecks it, probably looking for macroparasites such as Otiditaenia conoides. Bravo et al. (2014) suggested that a higher consumption of blister beetles by males could be a sexually-selected mechanism to enhance their mating success. The hypothesis of self-medication in bustards was supported by Whitman et al. (2019), who demonstrated antiparasitic effects of extracts from blister beetles (Berberomeloe majalis) against different models (protozoan, nematodes, ticks and insects). Heneberg (2016) proposed that bustard males self-medicated seeking sexual arousal rather than antipathogenic effects. Both effects are not mutually exclusive. Regardless of the primary function of blister beetle consumption, we show here that plants in the diet of bustards are also active against laboratory models of pathogens. We present the antiparasitic results for two plant species as an example. Although the toxicity of P. rhoeas and E. plantagineum differs, great bustards show a marked preference for both plants during the mating season (Figure 1), and during that season we found higher amounts of P. rhoeas in male than in female fecal samples (Table 4). Why could males be more interested in this plant than females and why during the mating season? Courtship is strenuous for males in most polygynous species and particularly in great bustard males, who show the most strongly skewed mating success reported among lekking birds, suggesting an extreme intensity of sexual selection in this species (Alonso et al., 2010a). Males develop costly ornaments every spring and perform exhausting displays to attract females (Alonso et al., 2010a,b). It is known that physiological investment in sexually selected characters competes with investment in immune response (Pap et al., 2009), a trade-off which is not as demanding for females, with the consequence of smaller loads of parasites and pathogens in this sex compared to males (Pap et al., 2010). Great bustard males would hold higher load of parasites and other pathogens than females and still sire a number of descendants in the next generation if females chose to mate them, according to the Handicap Principle (Zahavi, 1975, 1977). Attracting females while keeping pathogens may be quite demanding, so polar components in P. rhoeas’ capsules and flowers would help males control pathogens, reduce fatigue (sensu Heneberg, 2016), or both. Measuring these effects in vivo is beyond any feasible experimental setup, at least with current designs and legal restrictions on experimenting with this vulnerable species. But inferring the causal links is reasonable, so we put forward the challenging hypothesis that great bustard males disproportionately foraged on P. rhoeas during the mating season because some non-nutritional compounds were present in this plant. Differentiating between the antipathogenic effect of species rich in secondary metabolites and the consumption for their nutritional value is one of the difficulties in the interpretation of self-medication in animals (Huffman, 2003). For example, alkaloids and other phytochemicals, in low doses and in appropriate mixtures, can be helpful for the health of some animals (Huffman, 1997; Lozano, 1998, and references therein). Often, the limits between nutrients, drugs and toxins are determined by the dose ingested (Raubenheimer and Simpson, 2009). A recent hypothesis on self-medication behaviour in animals considers the increase in the consumption of species already present in the usual diet (Lisonbee et al., 2009), in contrast to the traditional hypothesis that considered the ingestion of specific compounds by animals only in response to infection (Huffman, 2003).
Activity of E. plantagineum against pathogens tested in the present study was noticeably higher than that of P. rhoeas, but the proportion of Echium plantagineum dry weight in fecal droppings of great bustards was about 50 times smaller than that of P. rhoeas during the mating season, and seven times smaller in the non-mating season. The harmful effects of pyrrolizidine alkaloids on bird health described in previous studies (Pass et al., 1979; Savaris et al., 2019) could explain the small amount of E. plantagineum in great bustard fecal droppings, but not its higher proportional dry weight in males compared to females during the non-mating season, unless we admit that males would have a greater need of these compounds than females also outside the mating season. We cannot discard that males would also benefit more than females from the properties of E. plantagineum during the months we define as non-mating season [November–January, and July, see Bravo et al., 2016]. Males indeed start displaying and fighting to establish their dominance hierarchy in the male group in December–January (Magaña et al., 2011), so these winter months could also be highly energy demanding. As for July, this is the hottest month of the year, when males suffer the debilitating effects of a much lower heat resistance compared to females (Alonso et al., 2016), coinciding with the moult of the flight feathers.
Taken together, our results support the view that male bustards consumed two plants because the exertion of courtship put them under immunological stress. Though both plants showed activity against pathogen models in laboratory conditions, these results do not prove the self-medication hypothesis (Lozano, 1998). The ability to learn improved health and a previous bitter taste (for example) has received low attention (Huffman, 1997); even less attention has been given to the functional characterization of chemical communication between plants with active compounds against pathogens and wild birds. We acknowledge detailed investigation of both plants’ pharmacological and phytochemical value against wild-bird pathogens is needed. But until a safe methodology is developed to study experimentally wild birds, field studies of plant compounds against pathogens in big-size steppe birds are warranted.
The raw data supporting the conclusions of this article will be made available by the authors, without undue reservation.
Ethical review and approval was not required for the animal study because It was an observational study. We did not capture the birds. Only droppings were collected.
LB-S, JA and AG-C conceived the study. LB-S, MG-M, RM-D, MA, JA, and AG-C provided research resources. MG-M, RM-D, MA, and AG-C designed the methods. LB-S and PB collected plant samples. JA and CB contributed field data of Great Bustards. PB and MG-M conducted the experiments. LB-S, PB, MG-M, RM-D, MA, CB, and AG-C analyzed the data. PB wrote the manuscript’s first draft. All authors contributed to subsequent editions. LB-S, JA, and AG-C sought funding acquisition. LB-S, PB, and AG-C dealt with administrative tasks. All authors contributed to the article and approved the submitted version.
This research was funded by FEDER/Ministerio de Ciencia, Innovación y Universidades – Agencia Estatal Consejo Superior de Investigaciones Científicas of Spain, grants no. CGL2017-87206-P and PID2019-106222RB-C31. PB was funded with a training contract of European Social Fund and Comunidad de Madrid no. PEJ-2018-AI/AMB-10263.
We thank the research team led by AGC for technical assistance to PB. We acknowledge partial support of the publication fee by the CSIC Open Access Publication Support Initiative through its Unit of Information Resources for Research (URICI).
The authors declare that the research was conducted in the absence of any commercial or financial relationships that could be construed as a potential conflict of interest.
All claims expressed in this article are solely those of the authors and do not necessarily represent those of their affiliated organizations, or those of the publisher, the editors and the reviewers. Any product that may be evaluated in this article, or claim that may be made by its manufacturer, is not guaranteed or endorsed by the publisher.
The Supplementary material for this article can be found online at: https://www.frontiersin.org/articles/10.3389/fevo.2022.1027201/full#supplementary-material
C-Hex, Capsules-hexane; C-EtOAc, Capsules-ethyl acetate; C-EtOH, Capsules-ethanol; DCM, Dichloromethane; DMSO, Dimethyl sulphoxide; Ep, Echium plantagineum.; EtOAc, Ethyl acetate; EtOH, Ethanol; EO, Essential oil; F-MeOH, Flowers-Methanol; F-IFD, Flowers-Infusion-freeze dried; F-IDCM, Flowers-Infusion-dichloromethane; GC–MS, Gas chromatography mass spectrometry; GLMM, Generalized linear mixed model; Hex, Hexane; HPLC-MS, Chromatography coupled to mass spectrometry; IDCM, Infusion-dichloromethane; IFD, Infusion-freeze dried; J2, Second stage juvenile nematode; L-Hex, Leaves-hexane; L-EtOAc, Leaves-Ethyl acetate; L-EtOH, Leaves-Ethanol; MeOH, Methanol; MMT, Colorimetric tetrazolium assay; PA, Pyrrolicidine alkaloids; PDA, Potato dextrose agar; PMS, Phenazine methosulfate; Pr, Papaver rhoeas; SDS, sodium dodecyl sulphate; TYM, Trypticase yeast maltose medium.
Abbott, J. (2014). Self-medication in insects: current evidence and future perspectives. Eco. Ento. 39, 273–280. doi: 10.1111/een.12110
Alikarami, M., Charehgani, H., and Abdollahi, M. (2018). Nematicidal activity of some plant extracts on root-knot nematode on tomato (Solanum lycopersicum) in vitro and in vivo conditions. Iran. J. Plant Prot. Sci. 48, 317–326. doi: 10.22059/ijpps.2017.227694.1006766
Alonso, J. C., and Alonso, J. A. (1996). The great bustard Otis tarda in Spain: present status, recent trends and an evaluation of earlier censuses. Biol. Conserv. 77, 79–86. doi: 10.1016/0006-3207(95)00137-9
Alonso, J. C., Magaña, M., Martín, C. A., and Palacín, C. (2010a). Sexual traits as quality indicators in lekking male great bustards. Ethology 116, 1084–1098. doi: 10.1111/j.1439-0310.2010.01827.x
Alonso, J. C., Magaña, M., Palacín, C., and Martin, C. A. (2010b). Correlates of male mating success in great bustard leks: the effects of age, weight, and display effort. Behav. Ecol. Sociobiol. 64, 1589–1600. doi: 10.1007/s00265-010-0972-6
Alonso, J. C., and Palacín, C. (2015). “Avutarda–Otis tarda” in Enciclopedia Virtual de los Vertebrados Españoles. eds. A. Salvador and M. B. Morales 2015th ed (Madrid: Museo Nacional de Ciencias Naturales).
Alonso, J. C., Salgado, I., and Palacín, C. (2016). Thermal tolerance may cause sexual segregation in sexually dimorphic species living in hot environments. Behav. Ecol. 27, 717–724. doi: 10.1093/beheco/arv211
Andrés, M. F., González-Coloma, A., Muñoz, R., De la Peña, F., Julio, L. F., and Burillo, J. (2018). Nematicidal potential of hydrolates from the semi industrial vapor-pressure extraction of Spanish aromatic plants. Environ. Sci. Pollut. Res. 25, 29834–29840. doi: 10.1007/s11356-017-9429-z
Arne, P., Risco-Castillo, V., Jouvion, G., Le Barzic, C., and Guillot, J. (2021). Aspergillosis in wild birds. J. Fungi 7:241. doi: 10.3390/jof7030241
Bailey, T. A. (2008). Diseases and medical management of Houbara bustards and other Otididae. Abu Dhabi, UAE: Emirates Printing Press L.L.C.
Bautista, L. M., Bravo, C., Ponce, C., Unzué-Belmonte, D., and Alonso, J. C. (2017). Food availability but not sex determines morning foraging area size in the great bustard Otis tarda, the most sexually size-dimorphic bird species. Ardeola 64, 289–303. doi: 10.13157/arla.64.2.2017.ra1
Beach, D. H., Holz, G. G., Singh, B. N., and Lindmark, D. G. (1990). Fatty-acid and sterol-metabolism of cultured Trichomonas vaginalis and Tritrichomonas foetus. Mol. Biochem. Parasitol. 38, 175–190. doi: 10.1016/0166-6851(90)90021-d
Bennett, J. W. (2010). “An overview of the genus Aspergillus” in Aspergillus: Molecular biology and genomics. eds. M. Machida and K. Gomi (Wymondham: Caister Academic Press), 1–17.
Boyce, M. S. (1990). The red queen visits sage grouse leks. Am. Zool. 30, 263–270. doi: 10.1093/icb/30.2.263
Bravo, C., Bautista, L. M., Garcia-Paris, M., Blanco, G., and Alonso, J. C. (2014). Males of a strongly polygynous species consume more poisonous food than females. PLoS One 9:e111057. doi: 10.1371/journal.pone.0111057
Bravo, C., Ponce, C., Bautista, L. M., and Alonso, J. C. (2016). Dietary divergence in the most sexually size-dimorphic bird. Auk 133, 178–197. doi: 10.1642/auk-15-206.1
Castella, G., Chapuisat, M., and Christe, P. (2008a). Prophylaxis with resin in wood ants. Anim. Behav. 75, 1591–1596. doi: 10.1016/j.anbehav.2007.10.014
Castella, G., Chapuisat, M., Moret, Y., and Christe, P. (2008b). The presence of conifer resin decreases the use of the immune system in wood ants. Eco. Ento. 33, 408–412. doi: 10.1111/j.1365-2311.2007.00983.x
Catoni, C., Peters, A., and Schaefer, H. M. (2008). Life history trade-offs are influenced by the diversity, availability and interactions of dietary antioxidants. Anim. Behav. 76, 1107–1119. doi: 10.1016/j.anbehav.2008.05.027
Cheeke, P. R. (1988). Toxicity and metabolism of pyrrolizidine alkaloids. J. Anim. Sci. 66, 2343–2350. doi: 10.1002/ajpa.20477
Cheeke, P. R., and Piersongoeger, M. L. (1983). Toxicity of Senecio jacobaea and pyrrolizidine alkaloids in various laboratory-animals and avian species. Toxicol. Lett. 18, 343–349. doi: 10.1016/0378-4274(83)90116-9
Christe, P., Oppliger, A., Bancala, F., Castella, G., and Chapuisat, M. (2003). Evidence for collective medication in ants. Ecol. Lett. 6, 19–22. doi: 10.1046/j.1461-0248.2003.00395.x
Coban, I., Toplan, G. G., Ozbek, B., Gurer, C. U., and Sariyar, G. (2017). Variation of alkaloid contents and antimicrobial activities of Papaver rhoeas L. growing in Turkey and northern Cyprus. Pharm. Biol. 55, 1894–1898. doi: 10.1080/13880209.2017.1340964
Colegate, S. M., Edgar, J. A., Knill, A. M., and Lee, S. T. (2005). Solid-phase extraction and HPLC-MS profiling of pyrrolizidine alkaloids and their N-oxides: a case study of Echium plantagineum. Phytochem. Anal. 16, 108–119. doi: 10.1002/pca.828
Coop, R. L., and Holmes, P. H. (1996). Nutrition and parasite interaction. Int. J. Parasitol. 26, 951–962. doi: 10.1016/s0020-7519(96)80070-1
Cordero del Campillo, M., Castañón-Ordóñez, L., and Reguera-Feo, A. (1994). Índice Catálogo de Zooparásitos Ibéricos (in Spanish). León, Spain: Universidad de León, Secretariado de Publicaciones.
Couplan, F. (1990). “Les belles vénéneuses: plantes sauvages toxiques” in Encyclopédie des plantes comestibles de l'Europe, Vol. 3. Flers: Equilibres Aujourd’houi, 1–379.
de Roode, J. C., Lefevre, T., and Hunter, M. D. (2013). Self-medication in animals. Science 340, 150–151. doi: 10.1126/science.1235824
Dogan, G., and Bagci, E. (2014). Essential oil composition of Papaver rhoeas L. (Corn poppy, Papaveraceae) from Turkey. Hacet. J. Biol. Chemis. 42, 545–549.
Evans, N. P., Collins, D. A., Pierson, F. W., Mahsoub, H. M., Sriranganathan, N., Persia, M. E., et al. (2017). Investigation of medium chain fatty acid feed supplementation for reducing Salmonella typhimurium colonization in Turkey poults. Foodborne Pathog. Dis. 14, 531–536. doi: 10.1089/fpd.2016.2273
Faragó, S. (2019). Spectrum of plant and animal diet of European great bustard (Otis tarda tarda) – an overview. Ornis Hung. 27, 62–84. doi: 10.2478/orhu-2019-0004
Folstad, I., and Karter, A. J. (1992). Parasites, bright males, and the immunocompetence handicap. Am. Nat. 139, 603–622. doi: 10.1086/285346
Fonturbel, F. E., Osorio, F., Riffo, V., Nuñez, M., Bastias, R., and Carvallo, G. O. (2020). Mamma knows best: why a generalist hummingbird selects the less abundant moss for nest building. Ecology 101:e03045. doi: 10.1002/ecy.3045
García-Montijano, M., Tébar, A. M., Barreiro, B., Rodriguez, P., Alonso, J. C., Martín, C. A., et al. (2002). "Postmortem findings in wild great bustards (Otis tarda) from Spain: a clinical approach", in: 4th scientific meeting EAZWV joint with the annual meeting of the European Wildlife Disease Association (EWDA). Heidelberg, Germany: European Association of Zoo-and Wildlife Veterinarians (EAZWV).
Githiori, J. B., Athanasiadou, S., and Thamsborg, S. M. (2006). Use of plants in novel approaches for control of gastrointestinal helminths in livestock with emphasis on small ruminants. Vet. Parasitol. 139, 308–320. doi: 10.1016/j.vetpar.2006.04.021
Grauso, L., de Falco, B., Motti, R., and Lanzotti, V. (2020). Corn poppy, Papaver rhoeas L.: A critical review of its botany, phytochemistry and pharmacology. Phytochem. Rev. 20, 227–248. doi: 10.1007/s11101-020-09676-7
Grauso, L., Emrick, S., Bonanomi, G., and Lanzotti, V. (2019). Metabolomics of the alimurgic plants Taraxacum officinale, Papaver rhoeas and Urtica dioica by combined NMR and GC-MS analysis. Phytochem. Anal. 30, 535–546. doi: 10.1002/pca.2845
Grieves, L. A., Gilles, M., Cuthill, I. C., Szekely, T., MacDougall-Shackleton, E. A., and Caspers, B. A. (2022). Olfactory camouflage and communication in birds. Biol. Rev. Camb. Philos. Soc. 97, 1193–1209. doi: 10.1111/brv.12837
Gunaydin, Y. K., Dundar, Z. D., Cekmen, B., Akillj, N. B., Koylu, R., and Cander, B. (2015). Intoxication due to Papaver rhoeas (Corn Poppy): five case reports. Case Rep. Med. 2015, 1–3. doi: 10.1155/2015/321360
Harvard (2007). A summary of error propagation. Cambridge, MA: Harvard University Press. Available at: https://www.academia.edu/14672973 [Accessed September 2022].
Heneberg, P. (2016). On Otis tarda and Marquis de Sade: what motivates male great bustards to consume blister beetles (Meloidae)? J. Ornithol. 157, 1123–1125. doi: 10.1007/s10336-016-1369-8
Hmamou, A., Eloutassi, N., Alshawwa, S. Z., Al Kamaly, O., Kara, M., Bendaoud, A., et al. (2022). Total phenolic content and antioxidant and antimicrobial activities of Papaver rhoeas L. organ extracts growing in Taounate region, Morocco. Molecules 27:854. doi: 10.3390/molecules27030854
Huffman, M. A. (1997). Current evidence for self-medication in primates: A multidisciplinary perspective. Am. J. Physical Anthropol. 104, 171–200. doi: 10.1002/(SICI)1096-8644(1997)25+<171::AID-AJPA7>3.0.CO;2-7
Huffman, M. A. (2001). Self-medicative behavior in the African great apes: an evolutionary perspective into the origins of human traditional medicine. Bioscience 51, 651–661. doi: 10.1641/0006-3568(2001)051[0651:Smbita]2.0.Co;2
Huffman, M. A. (2003). Animal self-medication and ethno-medicine: exploration and exploitation of the medicinal properties of plants. Proc. Nutr. Soc. 62, 371–381. doi: 10.1079/pns2003257
Huffman, M. A., Nakagawa, N., Go, Y., Imai, H., and Tomonaga, M. (2013). “Primate self-medication and the treatment of parasite infection” in Monkeys, apes, and humans: Primatology in Japan. eds. M. A. Huffman, N. Nakagawa, Y. Go, H. Imai, and M. Tomonaga (Tokyo: Springer Japan), 13–23.
Koçak, S., Karabulut, K., Ertekin, B., Nak, H., and Cander, B. (2016). Red poppy (Papaver Rhoeas) poisoning: a report of three cases. Cyp. J. Med. Sci. 1, 11–13. doi: 10.5152/cjms.2016.25
Lafuma, L., Lambrechts, M. M., and Raymond, M. (2001). Aromatic plants in bird nests as a protection against blood-sucking flying insects? Behav. Process. 56, 113–120. doi: 10.1016/s0376-6357(01)00191-7
Lane, S. J., Alonso, J. C., Alonso, J. A., and Naveso, M. A. (1999). Seasonal changes in diet and diet selection of great bustards (Otist. tarda) in north-West Spain. J. Zool. 247, 201–214. doi: 10.1111/j.1469-7998.1999.tb00984.x
Lane, S. J., Alonso, J. C., and Martín, C. A. (2001). Habitat preferences of great bustard Otis tarda flocks in the arable steppes of Central Spain: are potentially suitable areas unoccupied? J. Appl. Ecol. 38, 193–203. doi: 10.1046/j.1365-2664.2001.00577.x
Latek, U., Chlopecka, M., Karlik, W., and Mendel, M. (2022). Phytogenic compounds for enhancing intestinal barrier function in poultry-a review. Planta Med. 88, 218–236. doi: 10.1055/a-1524-0358
Lefèvre, T., Chiang, A., Kelavkar, M., Li, H., Li, J., Lopez, C., et al. (2012). Behavioural resistance against a protozoan parasite in the monarch butterfly. J. Anim. Ecol. 81, 70–79. doi: 10.1111/j.1365-2656.2011.01901.x
Lin, R.-J., Wu, M.-H., Ma, Y.-H., Chung, L.-Y., Chen, C.-Y., and Yen, C.-M. (2014). Anthelmintic activities of Aporphine from Nelumbo nucifera Gaertn. cv. Rosa-plena against Hymenolepis nana. Int. J. Mol. Sci. 15, 3624–3639. doi: 10.3390/ijms15033624
Lisonbee, L. D., Villalba, J. J., Provenza, F. D., and Hall, J. O. (2009). Tannins and self-medication: implications for sustainable parasite control in herbivores. Behav. Process. 82, 184–189. doi: 10.1016/j.beproc.2009.06.009
Lozano, G. A. (1998). “Parasitic stress and self-medication in wild animals” in Stress and behavior. eds. A. P. Møller, M. Milinski, and P. J. B. Slater, Advances in the Study of Behavior, vol. 27 (San Diego, USA: Academic Press), 291–317.
Mädge, I., Gehling, M., Schoene, C., Winterhalter, P., and These, A. (2020). Pyrrolizidine alkaloid profiling of four Boraginaceae species from northern Germany and implications for the analytical scope proposed for monitoring of maximum levels. Food Addit. Contam. - Chem. Anal. Control Expo. Risk Assess. 37, 1339–1358. doi: 10.1080/19440049.2020.1757166
Magaña, M. (2007). Comportamiento reproductivo de la avutarda común (Order No. 10172022). PhD thesis. Universidad Complutense de Madrid.
Magaña, M., Alonso, J. C., and Palacin, C. (2011). Age-related dominance helps reduce male aggressiveness in great bustard leks. Anim. Behav. 82, 203–211. doi: 10.1016/j.anbehav.2011.04.014
Mahmoud, M., Aminou, H. A., and Hashem, H. A. (2016). ¿Son los ácidos grasos responsables del mayor efecto del aceite y el extracto alcohólico de Nigella sativa sobre su extracto acuoso sobre los trofozoítos de Trichomonas vaginalis? Rev. Enferm. Parasit. 40, 22–31.
Martín, C. A., Alonso, J. C., Alonso, J., Pitra, C., and Lieckfeldt, D. (2002). Great bustard population structure in Central Spain: concordant results from genetic analysis and dispersal study. Proc. R. Soc. Lond. B Biol. Sci. 269, 119–125. doi: 10.1098/rspb.2001.1858
Masello, J. F., Martinez, J., Calderon, L., Wink, M., Quillfeldt, P., Sanz, V., et al. (2018). Can the intake of antiparasitic secondary metabolites explain the low prevalence of hemoparasites among wild Psittaciformes? Parasit. Vectors 11, 357–372. doi: 10.1186/s13071-018-2940-3
Maule, A. G., and Curtis, R. (2011). “Parallels between plant and animal parasitic nematodes” in Genomics and molecular genetics of plant-nematode interactions. eds. J. Jones, G. Gheysen, and C. Fenoll, 221–251. doi: 10.1007/978-94-007-0434-3_11
Milan, N. F., Kacsoh, B. Z., and Schlenke, T. A. (2012). Alcohol consumption as self-medication against blood-borne parasites in the fruit Fly. Curr. Biol. 22, 488–493. doi: 10.1016/j.cub.2012.01.045
Morales, P., Ferreira, I. C. F. R., Carvalho, A. M., Sánchez-Mata, M. C., Cámara, M., Fernández-Ruiz, V., et al. (2014). Mediterranean non-cultivated vegetables as dietary sources of compounds with antioxidant and biological activity. LWT - Food Sci. Technol. 55, 389–396. doi: 10.1016/j.lwt.2013.08.017
Mrazova, A., Sam, K., and Amo, L. (2019). What do we know about birds' use of plant volatile cues in tritrophic interactions? Curr. Opin. Insect Sci. 32, 131–136. doi: 10.1016/j.cois.2019.02.004
Oh, J.-H., Ha, I. J., Lee, M. Y., Kim, E.-O., Park, D., Lee, J.-H., et al. (2018). Identification and metabolite profiling of alkaloids in aerial parts of Papaver rhoeas by liquid chromatography coupled with quadrupole time-of-flight tandem mass spectrometry. J. Sep. Sci. 41, 2517–2527. doi: 10.1002/jssc.201701402
Pap, P. L., Czirjak, G. A., Vagasi, C. I., Barta, Z., and Hasselquist, D. (2010). Sexual dimorphism in immune function changes during the annual cycle in house sparrows. Naturwissenschaften 97, 891–901. doi: 10.1007/s00114-010-0706-7
Pap, P. L., Vagasi, C. I., Czirjak, G. A., Titilincu, A., Pintea, A., and Barta, Z. (2009). Carotenoids modulate the effect of coccidian infection on the condition and immune response in moulting house sparrows. J. Exp. Biol. 212, 3228–3235. doi: 10.1242/jeb.031948
Pass, D. A., Hogg, G. G., Russell, R. G., Edgar, J. A., Tence, I. M., and Rikard-Bell, L. (1979). Poisoning of chickens and ducks by pyrrolizidine alkaloids of Heliotropium europaeum. Aust. Vet. J. 55, 284–288. doi: 10.1111/j.1751-0813.1979.tb14711.x
Peterson, J., and Jago, M. (1984). Toxicity of Echium plantagineum (Paterson's curse). 2. Pyrrolizidine alkaloid poisoning in rats. Aust. J. Agric. Res. 35, 305–315. doi: 10.1071/AR9840305
Raubenheimer, D., and Simpson, S. J. (2009). Nutritional PharmEcology: doses, nutrients, toxins, and medicines. Integr. Comp. Biol. 49, 329–337. doi: 10.1093/icb/icp050
Roberts, M. L., Buchanan, K. L., and Evans, M. R. (2004). Testing the immunocompetence handicap hypothesis: a review of the evidence. Anim. Behav. 68, 227–239. doi: 10.1016/j.anbehav.2004.05.001
Rocha, P., Marques, A. T., and Moreira, F. (2005). Seasonal variation in great bustard Otis tarda diet in South Portugal with a focus on the animal component. Ardeola 52, 371–376.
Russo, R., Autore, G., and Severino, L. (2009). Pharmaco-toxicological aspects of herbal drugs used in domestic animals. Nat. Prod. Commun. 4, 1777–1784. doi: 10.1177/1934578X0900401230
Sainz, P., Andrés, M. F., Martínez-Díaz, R. A., Bailén, M., Navarro-Rocha, J., Díaz, C. E., et al. (2019). Chemical composition and biological activities of Artemisia pedemontana subsp. assoana essential oils and hydrolate. Biomol. Ther. 9:558. doi: 10.3390/biom9100558
Sanchez-Barbudo, I. S., Camarero, P. R., Garcia-Montijano, M., and Mateo, R. (2012). Possible cantharidin poisoning of a great bustard (Otis tarda). Toxicon 59, 100–103. doi: 10.1016/j.toxicon.2011.10.002
Sanchez-Mata, M. C., Loera, R. D. C., Morales, P., Fernandez-Ruiz, V., Camara, M., Marques, C. D., et al. (2012). Wild vegetables of the Mediterranean area as valuable sources of bioactive compounds. Genet. Resour. Crop. Evol. 59, 431–443. doi: 10.1007/s10722-011-9693-6
Sanchez-Mata, M. D. C., and Tardío, J. (2016). Mediterranean wild edible plants: Ethnobotany and food composition tables. Springer-Verlag New York.
Satou, T., Koga, M., Matsuhashi, R., Koike, K., Tada, I., and Nikaido, T. (2002). Assay of nematocidal activity of isoquinoline alkaloids using third-stage larvae of Strongyloides ratti and S. venezuelensis. Vet. Parasitol. 104, 131–138. doi: 10.1016/s0304-4017(01)00619-7
Savaris, T., Biffi, C. P., Ogliari, D., Wicpolt, N., Molossi, F. A., Melchioretto, E., et al. (2019). Experimental poisoning by Crotalaria lanceolata and Crotalaria pallida seeds in broilers. Pesqui. Vet. Bras. 39, 863–869. doi: 10.1590/1678-5150-pvb-6271
Schneider, C. A., Rasband, W. S., and Eliceiri, K. W. (2012). NIH image to ImageJ: 25 years of image analysis. Nat. Methods 9, 671–675. doi: 10.1038/nmeth.2089
Schneider-Orelli, O. (1947). Entomologisches Praktikum: Einführung in die land-und forstwirtschaftliche Insektenkunde. Aarau, Sauerländer & Co.
Schramm, S., Kohler, N., and Rozhon, W. (2019). Pyrrolizidine alkaloids: biosynthesis, biological activities and occurrence in crop plants. Molecules 24:44. doi: 10.3390/molecules24030498
SEO/BirdLife (2015). Avutarda común (Otis tarda). Available at: https://www.seo.org/ave/avutarda-comun [Accessed July 26, 2020].
Silvanose, C. D., Samour, J. H., Naldo, J. L., and Bailey, T. A. (1998). Oro-pharyngeal protozoa in captive bustards: clinical and pathological considerations. Avian Pathol. 27, 526–530. doi: 10.1080/03079459808419378
Singer, M., Mace, K., and Bernays, E. (2009). Self-medication as adaptive plasticity: increased ingestion of plant toxins by parasitized caterpillars. PLoS One 4:4796. doi: 10.1371/journal.pone.0004796
Sixto, A., Perez-Parada, A., Niell, S., and Heinzen, H. (2019). GC-MS and LC-MS/MS workflows for the identification and quantitation of pyrrolizidine alkaloids in plant extracts, a case study: Echium plantagineum. Braz. J. Pharm. 29, 500–503. doi: 10.1016/j.bjp.2019.04.010
Skoneczny, D., Weston, P. A., Zhu, X., Gurr, G. M., Callaway, R. M., and Weston, L. A. (2015). Metabolic profiling of pyrrolizidine alkaloids in foliage of two Echium spp. invaders in Australia: a case of novel weapons? Int. J. Mol. Sci. 16, 26721–26737. doi: 10.3390/ijms161125979
Thoden, T. C., Boppre, M., and Hallmann, J. (2009). Effects of pyrrolizidine alkaloids on the performance of plant-parasitic and free-living nematodes. Pest Manag. Sci. 65, 823–830. doi: 10.1002/ps.1764
Tokarzewski, S., Ziółkowska, G., and Nowakiewicz, A. (2012). Susceptibility testing of Aspergillus niger strains isolated from poultry to antifungal drugs - a comparative study of the disk diffusion, broth microdilution (M 38-a) and Etest® methods. Pol. J. Vet. Sci. 15, 125–133. doi: 10.2478/v10181-011-0123-7
Villalba, J. J., Costes-Thire, M., and Ginane, C. (2017). Phytochemicals in animal health: diet selection and trade-offs between costs and benefits. Proc. Nutr. Soc. 76, 113–121. doi: 10.1017/s0029665116000719
Villalba, J. J., and Landau, S. Y. (2012). Host behavior, environment and ability to self-medicate. Small Rumin. Res. 103, 50–59. doi: 10.1016/j.smallrumres.2011.10.018
Villalba, J. J., Miller, J., Ungar, E. D., Landau, S. Y., and Glendinning, J. (2014). Ruminant self-medication against gastrointestinal nematodes: evidence, mechanism, and origins. Parasite 21:31. doi: 10.1051/parasite/2014032
Villalba, J. J., Provenza, F. D., Hall, J. O., and Lisonbee, L. D. (2010). Selection of tannins by sheep in response to gastrointestinal nematode infection. J. Anim. Sci. 88, 2189–2198. doi: 10.2527/jas.2009-2272
Weston, P. A., Weston, L. A., and Hildebrand, S. (2013). Metabolic profiling in Echium plantagineum: presence of bioactive pyrrolizidine alkaloids and napthoquinones from accessions across southeastern Australia. Phytochem. Rev. 12, 831–837. doi: 10.1007/s11101-013-9306-4
Whitman, D. W., Andres, M. F., Martinez-Diaz, R. A., Ibanez-Escribano, A., Olmeda, A. S., and Gonzalez-Coloma, A. (2019). Antiparasitic properties of cantharidin and the blister beetle Berberomeloe majalis (Coleoptera: Meloidae). Toxins 11:234. doi: 10.3390/toxins11040234
Williams, A. R., Andersen-Civil, A. I. S., Zhu, L., and Blanchard, A. (2020). Dietary phytonutrients and animal health: regulation of immune function during gastrointestinal infections. J. Anim. Sci. 98:11. doi: 10.1093/jas/skaa030
Wolfram Research, Inc. (2022). Mathematica online. Champaign, Illinois. Available at: https://www.wolfram.com/mathematica/online/ [Accessed September 2022].
Zahavi, A. (1975). Mate selection - selection for a handicap. J. Theor. Biol. 53, 205–214. doi: 10.1016/0022-5193(75)90111-3
Zahavi, A. (1977). Cost of honesty - (further remarks on handicap principle). J. Theor. Biol. 67, 603–605. doi: 10.1016/0022-5193(77)90061-3
Zhu, X., Skoneczny, D., Weidenhamer, J. D., Mwendwa, J. M., Weston, P. A., Gurr, G. M., et al. (2016). Identification and localization of bioactive naphthoquinones in the roots and rhizosphere of Paterson's curse (Echium plantagineum), a noxious invader. J. Exp. Bot. 67, 3777–3788. doi: 10.1093/jxb/erw182
Keywords: Otis tarda, Papaver rhoeas, Echium plantagineum, antiparasitic activity, self-medication
Citation: Bautista-Sopelana LM, Bolívar P, Gómez-Muñoz MT, Martínez-Díaz RA, Andrés MF, Alonso JC, Bravo C and González-Coloma A (2022) Bioactivity of plants eaten by wild birds against laboratory models of parasites and pathogens. Front. Ecol. Evol. 10:1027201. doi: 10.3389/fevo.2022.1027201
Received: 24 August 2022; Accepted: 31 October 2022;
Published: 23 November 2022.
Edited by:
Milica Ljaljevic Grbic, University of Belgrade, SerbiaReviewed by:
Georg Petschenka, University of Hohenheim, GermanyCopyright © 2022 Bautista-Sopelana, Bolívar, Gómez-Muñoz, Martínez-Díaz, Andrés, Alonso, Bravo and González-Coloma. This is an open-access article distributed under the terms of the Creative Commons Attribution License (CC BY). The use, distribution or reproduction in other forums is permitted, provided the original author(s) and the copyright owner(s) are credited and that the original publication in this journal is cited, in accordance with accepted academic practice. No use, distribution or reproduction is permitted which does not comply with these terms.
*Correspondence: Luis M. Bautista-Sopelana, bG0uYmF1dGlzdGFAY3NpYy5lcw==; Azucena González-Coloma, YXp1QGljYS5jc2ljLmVz
†Deceased
Disclaimer: All claims expressed in this article are solely those of the authors and do not necessarily represent those of their affiliated organizations, or those of the publisher, the editors and the reviewers. Any product that may be evaluated in this article or claim that may be made by its manufacturer is not guaranteed or endorsed by the publisher.
Research integrity at Frontiers
Learn more about the work of our research integrity team to safeguard the quality of each article we publish.