- 1Department of Interdisciplinary Life Sciences, Konrad-Lorenz Institute of Ethology, University of Veterinary Medicine Vienna, Vienna, Austria
- 2Department of Vertebrate Zoology and Ecology, Nicolaus Copernicus University in Toruṅ, Toruṅ, Poland
- 3School of Animal, Plant, and Environmental Sciences, University of the Witwatersrand, Johannesburg, South Africa
- 4Hatherly Laboratories, University of Exeter, Exeter, United Kingdom
- 5Department of Biology, Lund University, Lund, Sweden
Editorial on the Research Topic
Ecophysiological adaptations associated with animal migration
Seasonal migration to track favorable environmental conditions and resources is a widespread strategy across all animal taxa, including insects, fish, birds, and mammals. Migration distances can range from meters to tens of thousands of kilometers (Hansson and Åkesson, 2014). This period is often resource-demanding (Sapir et al., 2011) and migrants regularly encounter challenging conditions en route, including extreme and/or fluctuating temperature, humidity, solar radiation, osmotic stress, pathogen pressure, pollution, and hypoxia (Figure 1). Migrants cope with these challenges via a multitude of physiological adaptations (e.g., McCormick and Saunders, 1987; Piersma and Van Gils, 2011; Gwinner, 2012; Cooper-Mullin and McWilliams, 2016; Hegemann et al., 2019), which can conflict with other important annual-cycle and life-history events such as growth, reproduction and molt. Migration therefore represents one of the most significant physiological challenges in the life of an animal, yet our understanding of the interplay between different physiological adaptations and the currencies involved (energy, nutrients, time, etc.) is still limited (Hegemann et al., 2019). To better understand the ecology and evolution of migration and to highlight important directions for future work, this Research Topic presents studies demonstrating recent advancements in our knowledge of the ecophysiological underpinnings of migration at different stages and scales.
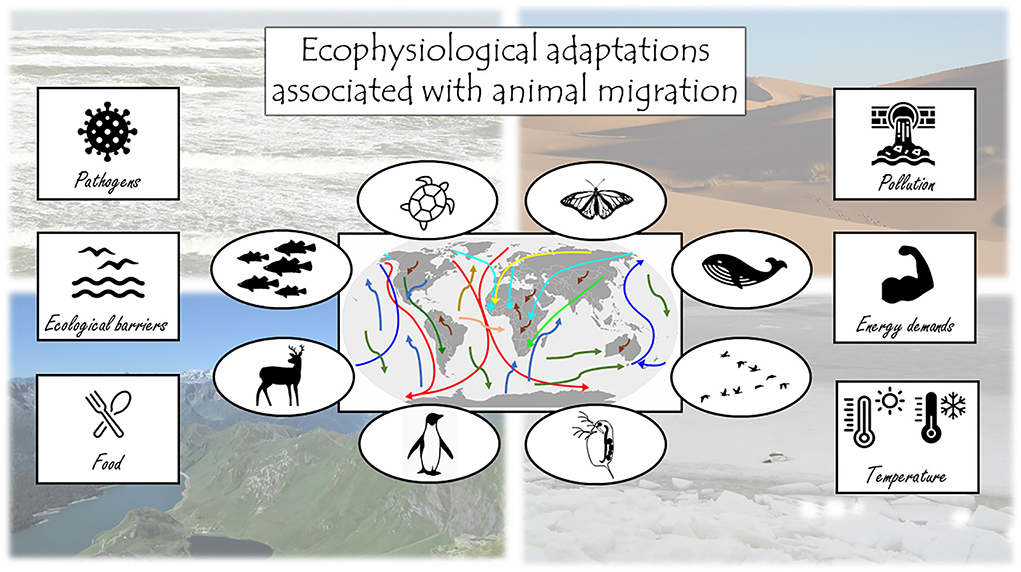
Figure 1. Migrating animals of all taxa experience a multitude of challenges during their seasonal migrations. In this Research Topic, we collected 14 papers that investigate several of those challenges in different taxa including insects, fish, birds and mammals.
Preparing for migration
Animals typically undergo dramatic physiological and behavioral changes in preparation for migration (McCormick and Saunders, 1987; Piersma and Van Gils, 2011), with the accumulation of energy reserves being particularly important for endurance migrants (Guglielmo, 2018). Many species accumulate significant amounts of fuel before their seasonal migration starts and this is usually controlled by endogenous programs (Maggini and Bairlein, 2010; Maggini et al., 2017). Cornelius et al. show that even facultative migrants, i.e., animals that migrate irregularly in response to changing conditions, can quickly increase their fat stores when necessary.
Other changes prior to migration often include reorganization of body composition to reduce maintenance costs for tissues not necessary during endurance exercise (Piersma et al., 1999; Piersma and Van Gils, 2011). Piersma et al. show that Bar-tailed Godwits (Limosa lapponica) shrink their digestive tract but maintain a higher relative size of their heart and flight muscles while accumulating fuel stores. This poses a particular challenge to animals that stop to replenish depleted fuel stores during migration. Refueling at stopover sites starts at a low rate (i.e., little food is converted to body mass) to accumulate lean mass, followed by a hyperphagic phase to accumulate fat stores, and a final phase to reallocate protein from the digestive system to flight muscle synthesis to facilitate further migration (Handby et al.; Guglielmo et al.). These processes have been studied most intensively in birds, and further work is needed to confirm that similar dramatic changes in body composition occur in other migratory animals.
Dealing with variable or changing abiotic conditions along the route
Migration poses countless challenges and mortality is highest during the migratory period in many animals (e.g., Sillett and Holmes, 2002; Klaassen et al., 2014; Kantola et al., 2019). Individuals must be prepared to meet high energetic demands and cope with additional environmental challenges, which can vary substantially along migratory routes (Figure 1). One of the most predictable abiotic changes migrants can experience as they move across geographic areas is a change in photoperiod, somewhat akin to ‘jet lag' in humans, and requiring re-organization of circadian rhythms. Two studies in this Research Topic show that migratory animals are particularly proficient in responding to changing photoperiod, both in the circadian rhythms of body temperature in migrating geese (Eichhorn et al.) and daily migratory activity in two passerine species (Åkesson et al.).
Migrating animals often cross ecological barriers, where little to no opportunities for resting or refueling may be available. Successful crossing of these barriers can be highly dependent on current environmental conditions, thus requiring individuals to carefully choose when to embark on the journey (Fransson et al., 2008; Deppe et al., 2015; Dossman et al., 2016). An impressive example for long barrier crossings among insects is the globe skimmer dragonfly (Pantala flavescens), which is hypothesized to migrate from India to East Africa by crossing the Indian Ocean. Hedlund et al. used an energetic flight model and wind trajectory analysis to show that this transoceanic migration is possible, but likely requires the capacity to select favorable winds.
Environmental conditions can also be unpredictable, including extreme weather events or challenging temperatures. Snow buntings (Plectrophenax nivalis), for example, maintain cold endurance during spring migration, presumably to cope with cold temperatures en route and at their Arctic breeding grounds (Le Pogam et al.). On the other end of the spectrum, desert-crossing passerines facing high temperatures during stopover adjust their activity patterns according to their species-specific dehydration risk (Paces et al.). These results show that flexible behavioral and physiological responses to challenging abiotic conditions are crucial for migrating animals to successfully complete migration.
Physiological trade-offs to facilitate successful migration
Coping with the high resource costs of migration often requires physiological trade-offs with other traits, such as growth, breeding and predator evasion. To deal with these trade-offs, two main strategies can be used: (i) minimizing the time spent migrating, i.e., traveling fast even if expensive, and (ii) minimize the total energy cost of migration, i.e., traveling at speeds that conserve energy (Alerstam and Lindström, 1990; Hedenström and Alerstam, 1997; Alerstam et al., 2003). Clerc et al. use two migrating bat species in which both time-minimizers and energy-minimizers co-occur within the same population, providing an excellent study system to test predictions. Using several cutting-edge techniques to estimate energetic state, they demonstrate that the two strategies can extend to the intraspecific level, as migratory strategies seem to vary between sexes, and that thermoregulation (specifically torpor in bats) might be an important regulator of stopover decisions.
During migration, animals often have to reallocate resources and this may affect several physiological systems including the immune system (Buehler et al., 2010). The immune system is important for survival (e.g., Wilcoxen et al., 2010; Hegemann et al., 2015; Roast et al., 2020), particularly in migrants which cope with novel diseases and high risk of disease transmission (Altizer et al., 2011; Westerdahl et al., 2014). Yet, trade-offs with other demands of migration can lead to modulation of immune function while migrating (e.g., Owen and Moore, 2006, 2008; Buehler et al., 2008, 2010; Eikenaar and Hegemann, 2016; Hegemann et al., 2018; Eikenaar et al., 2020). Hegemann et al. extend this knowledge by showing that immune function differs among species and among individuals depending on migratory strategy and timing. Nevertheless, migrants are exposed to varying pathogen landscapes as they move (Altizer et al., 2011; Westerdahl et al., 2014; Hall et al., 2016), which may compound the physiological challenges already experienced. For example, gut parasites have been thought to disrupt osmoregulation in salmon moving from fresh- to saltwater, but Finlay et al. did not find such effects, suggesting that fish either cope with the presence of parasites or that negative effects become apparent at a later stage of infection. Further studies investigating varying immune function and its interactions with infection rates are of paramount importance to understand how parasite and disease exposure influence migratory animals (Binning et al., 2022), particularly in the context of predicting future disease outbreaks and pandemics (Carlson et al., 2022).
Endurance exercise such as migration can also lead to relatively high oxidative stress (Costantini et al., 2008, 2019; Jenni-Eiermann et al., 2014), necessitating appropriate defense mechanisms (Eikenaar et al., 2022). McWilliams et al. provide a comprehensive review showing that birds can adjust their diet to augment their defenses by, for example, consuming foods rich in antioxidants. Furthermore, Cooper-Mullin and McWilliams show in a field experiment that recovering from oxidative damage may be an important function of migratory stopovers in addition to refueling. These studies further illustrate the complexity of the physiological adaptations required for animals to successfully complete migrations.
Conclusions and outlook
Migration allows many species to survive and thrive in dynamic environmental conditions, by exploiting diverse parts of the planet on a seasonal basis. However, migration also poses considerable risks and challenges for animals, often requiring trade-offs among physiological and behavioral processes. The collection of papers in this Research Topic shows how technological and analytical advancements permit the study of migratory strategies and physiological mechanisms in increasing detail. Importantly, many studies are now possible directly in the field or during short-term captivity, which eliminates many biases related to long-term captive experiments. Current rapid advances in tracking technologies should further accelerate our possibilities (Jetz et al., 2022). We anticipate a vibrant future for research on the ecophysiological adaptations associated with migration, and hope this Research Topic inspires future studies to elucidate the mechanisms of physiological adaptations, particularly in non-avian species and across multiple physiological systems. Such studies are also crucial to understand the potential role of physiological flexibility in responses to anthropogenic disturbances and rapidly changing environmental conditions under global change.
Author contributions
All authors contributed equally to drafting and editing of the present article. All authors contributed to the article and approved the submitted version.
Funding
AH was supported by a grant from the Swedish Research Council (2018–04278).
Acknowledgments
We thank all authors and reviewers who were involved in this Research Topic and greatly contributed to the quality and success of the work presented here.
Conflict of interest
The authors declare that the research was conducted in the absence of any commercial or financial relationships that could be construed as a potential conflict of interest.
Publisher's note
All claims expressed in this article are solely those of the authors and do not necessarily represent those of their affiliated organizations, or those of the publisher, the editors and the reviewers. Any product that may be evaluated in this article, or claim that may be made by its manufacturer, is not guaranteed or endorsed by the publisher.
References
Alerstam, T., Hedenström, A., and Åkesson, S. (2003). Long-distance migration: evolution and determinants. Oikos 103, 247–260. doi: 10.1034/j.1600-0706.2003.12559.x
Alerstam, T., and Lindström, Å. (1990). “Optimal bird migration: the relative importance of time, energy, and safety,” in Bird Migration, ed E. Gwinner (Berlin; Heidelberg: Springer), 331–351.
Altizer, S., Bartel, R., and Han, B. A. (2011). Animal migration and infectious disease risk. Science 331, 296–302. doi: 10.1126/science.1194694
Binning, S. A., Craft, M. E., Zuk, M., and Shaw, A. K. (2022). How to study parasites and host migration: a roadmap for empiricists. Biol. Rev. 97, 1161–1178. doi: 10.1111/brv.12835
Buehler, D. M., Piersma, T., Matson, K., and Tieleman, B. I. (2008). Seasonal redistribution of immune function in a migrant shorebird: annual-cycle effects override adjustments to thermal regime. Am. Nat. 172, 783–796. doi: 10.1086/592865
Buehler, D. M., Tieleman, B. I., and Piersma, T. (2010). How do migratory species stay healthy over the annual cycle? A conceptual model for immune function and for resistance to disease. Integr. Comp. Biol. 50, 346–357. doi: 10.1093/icb/icq055
Carlson, C. J., Albery, G. F., Merow, C., Trisos, C. H., Zipfel, C. M., Eskew, E. A., et al. (2022). Climate change increases cross-species viral transmission risk. Nature 607, 555–562. doi: 10.1038/s41586-022-04788-w
Cooper-Mullin, C., and McWilliams, S. R. (2016). The role of the antioxidant system during intense endurance exercise: lessons from migrating birds. J. Exp. Biol. 219, 3684–3695. doi: 10.1242/jeb.123992
Costantini, D., Dell'Ariccia, G., and Lipp, H.-P. (2008). Long flights and age affect oxidative status of homing pigeons (Columba livia). J. Exp. Biol. 211, 377–381. doi: 10.1242/jeb.012856
Costantini, D., Lindecke, O., Pētersons, G., and Voigt, C. C. (2019). Migratory flight imposes oxidative stress in bats. Curr. Zool. 65, 147–153. doi: 10.1093/cz/zoy039
Deppe, J. L., Ward, M. P., Bolus, R. T., Diehl, R. H., Celis-Murillo, A., Zenzal, T. J., et al. (2015). Fat, weather, and date affect migratory songbirds' departure decisions, routes, and time it takes to cross the Gulf of Mexico. Proc. Natl. Acad. Sci. U.S.A. 112, E6331–E6338. doi: 10.1073/pnas.1503381112
Dossman, B. C., Mitchell, G. W., Norris, D. R., Taylor, P. D., Guglielmo, C. G., Matthews, S. N., et al. (2016). The effects of wind and fuel stores on stopover departure behavior across a migratory barrier. Behav. Ecol. 27, 567–574. doi: 10.1093/beheco/arv189
Eikenaar, C., and Hegemann, A. (2016). Migratory common blackbirds have lower innate immune function during autumn migration than resident conspecifics. Biol. Lett. 12, 20160078. doi: 10.1098/rsbl.2016.0078
Eikenaar, C., Winslott, E., Hessler, S., and Isaksson, C. (2020). Oxidative damage to lipids is rapidly reduced during migratory stopovers. Func. Ecol. 34, 1215–1222. doi: 10.1111/1365-2435.13540
Eikenaar, C., Winslott, E., Schmaljohann, H., Wang, H.-L., and Isaksson, C. (2022). Can differential fatty acid composition help migrating birds to limit oxidative lipid damage? Physiol. Behav. 249, 113768. doi: 10.1016/j.physbeh.2022.113768
Fransson, T., Barboutis, C., Mellroth, R., and Akriotis, T. (2008). When and where to fuel before crossing the Sahara desert – extended stopover and migratory fuelling in first-year garden warblers Sylvia borin. J. Avian Biol. 39, 133–138. doi: 10.1111/j.0908-8857.2008.04361.x
Guglielmo, C. G. (2018). Obese super athletes: fat-fueled migration in birds and bats. J. Exp. Biol. 221, jeb165753. doi: 10.1242/jeb.165753
Gwinner, E. (2012). Bird Migration: Physiology and Ecophysiology. Springer Science and Business Media.
Hall, R. J., Brown, L. M., and Altizer, S. (2016). Modeling vector-borne disease risk in migratory animals under climate change. Integr. Comp. Biol. 56, 353–364. doi: 10.1093/icb/icw049
Hansson, L.-A., and Åkesson, S., (eds.). (2014). Animal Movement Across Scales. Oxford, UK: Oxford University Press. doi: 10.1093/acprof:oso/9780199677184.001.0001
Hedenström, A., and Alerstam, T. (1997). Optimum fuel loads in migratory birds: distinguishing between time and energy minimization. J. Theoret. Biol. 189, 227–234. doi: 10.1006/jtbi.1997.0505
Hegemann, A., Alcalde Abril, P., Muheim, R., Sjöberg, S., Alerstam, T., Nilsson, J.-Å., et al. (2018). Immune function and blood parasite infections impact stopover ecology in passerine birds. Oecologia 188, 1011–1024. doi: 10.1007/s00442-018-4291-3
Hegemann, A., Fudickar, A. M., and Nilsson, J.-Å. (2019). A physiological perspective on the ecology and evolution of partial migration. J. Ornithol. 160, 893–905. doi: 10.1007/s10336-019-01648-9
Hegemann, A., Marra, P. P., and Tieleman, B. I. (2015). Causes and consequences of partial migration in a passerine bird. Am. Nat. 186, 531–546. doi: 10.1086/682667
Jenni-Eiermann, S., Jenni, L., Smith, S., and Costantini, D. (2014). Oxidative stress in endurance flight: an unconsidered factor in bird migration. PLoS ONE 9, e97650. doi: 10.1371/journal.pone.0097650
Jetz, W., Tertitski, G., Kays, R., Mueller, U., Wikelski, M., Åkesson, S., et al. (2022). Biological Earth observation with animal sensors. Trends Ecol. Evol. 37, 293–298. doi: 10.1016/j.tree.2021.11.011
Kantola, T., Tracy, J. L., Baum, K. A., Quinn, M. A., and Coulson, R. N. (2019). Spatial risk assessment of eastern monarch butterfly road mortality during autumn migration within the southern corridor. Biol. Conserv. 231, 150–160. doi: 10.1016/j.biocon.2019.01.008
Klaassen, R. H. G., Hake, M., Strandberg, R., Koks, B. J., Trierweiler, C., Exo, K.-M., et al. (2014). When and where does mortality occur in migratory birds? Direct evidence from long-term satellite tracking of raptors. J. Anim. Ecol. 83, 176–184. doi: 10.1111/1365-2656.12135
Maggini, I., and Bairlein, F. (2010). Endogenous rhythms of seasonal migratory body mass changes and nocturnal restlessness in different populations of northern wheatear Oenanthe oenanthe. J. Biol. Rhythms 25, 268–276. doi: 10.1177/0748730410373442
Maggini, I., Bulte, M., and Bairlein, F. (2017). Endogenous control of fuelling in a migratory songbird. Sci. Nat. 104, 93. doi: 10.1007/s00114-017-1514-0
McCormick, S. D., and Saunders, R. L. (1987). “Preparatory physiological adaptations for marine life of salmonids: osmoregulation, growth, and metabolism,” in American Fisheries Society Symposium, 1–229.
Owen, J. C., and Moore, F. R. (2006). Seasonal differences in immunological condition of three species of thrushes. Condor 108, 389–398. doi: 10.1093/condor/108.2.389
Owen, J. C., and Moore, F. R. (2008). Swainson's thrushes in migratory disposition exhibit reduced immune function. J. Ethol. 26, 383–388. doi: 10.1007/s10164-008-0092-1
Piersma, T., Gudmundsson, G. A., and Lilliendahl, K. (1999). Rapid changes in the size of different functional organ and muscle groups during refueling in a long-distance migrating shorebird. Physiol. Biochem. Zool. 72, 405–415. doi: 10.1086/316680
Piersma, T., and Van Gils, J. A. (2011). The Flexible Phenotype: A Body-Centred Integration of Ecology, Physiology, and Behaviour. Oxford, UK: Oxford University Press.
Roast, M. J., Aranzamendi, N. H., Fan, M., Teunissen, N., Hall, M. D., and Peters, A. (2020). Fitness outcomes in relation to individual variation in constitutive innate immune function. Proc. R. Soc. B Biol. Sci. 287, 20201997. doi: 10.1098/rspb.2020.1997
Sapir, N., Butler, P. J., Hedenström, A., and Wikelski, M. (2011). “Energy gain and use during animal migration,” in Animal Migration A Synthesis', eds E. J. Milner-Gulland, J. M. Fryxell, and A. R. E. Sinclair (Oxford, UK: Oxford University Press), 52–67.
Sillett, T. S., and Holmes, R. T. (2002). Variation in survivorship of a migratory songbird throughout its annual cycle. J. Anim. Ecol. 71, 296–308. doi: 10.1046/j.1365-2656.2002.00599.x
Westerdahl, H., Bensch, S., Nilsson, J.-Å., Sehgal, R., Tesson, S., and Hasselquist, D. (2014). “Pathogens and hosts on the move,” in Animal Movement Across Scales, eds L.-A. Hansson, and S. Åkesson (New York, NY: Oxford University Press), 126–148.
Keywords: animal migration, physiology, bird, mammal, insect, fish, trade-off
Citation: Maggini I, Noakes MJ, Hawkes LA and Hegemann A (2022) Editorial: Ecophysiological adaptations associated with animal migration. Front. Ecol. Evol. 10:1022173. doi: 10.3389/fevo.2022.1022173
Received: 18 August 2022; Accepted: 23 August 2022;
Published: 07 September 2022.
Edited and reviewed by: Jordi Figuerola, Doñana Biological Station (CSIC), Spain
Copyright © 2022 Maggini, Noakes, Hawkes and Hegemann. This is an open-access article distributed under the terms of the Creative Commons Attribution License (CC BY). The use, distribution or reproduction in other forums is permitted, provided the original author(s) and the copyright owner(s) are credited and that the original publication in this journal is cited, in accordance with accepted academic practice. No use, distribution or reproduction is permitted which does not comply with these terms.
*Correspondence: Ivan Maggini, aXZhbi5tYWdnaW5pQHZldG1lZHVuaS5hYy5hdA==