- 1Grassland Research Institute, Chinese Academy of Agricultural Sciences, Hohhot, China
- 2Grassland Station of Xilinhot Forestry and Grassland Bureau, Xilinhot, China
- 3College of Plant Protection, Hebei Agricultural University, Baoding, China
For the past several decades, both species biodiversity and productivity of desert steppe have been reduced due to excessive use and climate factors. To counteract this, Chinese government has supported large-scale grassland ecological restoration programs since the year 2000. The policy needs a standard for the evaluation of the effects of such restorative measures on the grasslands after decades. Grassland insect diversity plays an important role in the maintenance of plant species and functional diversity. To understand the relation of grazing management and insect diversity, we use a complete two factor design, two fencing periods (3 or 7 years) and three grazing intensities (0, 6, or 12 sheep per ha), to examine the response of the insect diversity to fencing and grazing in desert steppe. We found almost no significant differences in either plant or insect species diversity between the sites fenced for 3 and 7 years, as the pressure of grazing increased, insect diversity decreased to a greater extent at 7-year enclosure sites than at 3-year sites. We recommend the most suitable grazing intensity for the sustainability of biodiversity of the desert steppe in Inner Mongolia is light grazing (8 sheep/ha 0.5 yr−1), and the most suitable fencing period is three years, which suggest that policies that remove livestock from the desert grassland for long periods (7 + years) are not beneficial for maintaining insect diversity, and heavy grazing lead ecological environment weaker and insect diversity decreasing. Thus, periodic livestock grazing is important in the design of management actions to preserve biodiversity.
Introduction
The “desert steppe” is a transitional region between the desert and traditional grassland steppes on the Inner Mongolia plateau and is particularly vulnerable to desertification (Yong et al., 2021). The region is dominated by Stipa klemenzii Roshev. (Poaceae) and is important for the livelihood of pastoral communities (Ren et al., 2011). However, for the past several decades, both species’ biodiversity and grassland productivity have been reduced, causing serious damage to the ecology of the region (Zhang et al., 2010, 2020). Overgrazing is the main factor that accelerates steppe degradation (He et al., 2019). The Chinese government has supported an integrated portfolio of large-scale grassland ecological restoration programs since 2000 to counteract grassland degradation, mainly involving fencing for grazing control, increased numbers of protected areas, and other forms of support to local communities (Cheng et al., 2016). However, the policy lacks a general standard for the evaluation of the effects of such restorative measures on grasslands. Insects are the most biologically diverse group of animals and are important primary consumers (pests), secondary consumers (natural enemies), and decomposers (carrion and fecal insects) in grassland ecosystems, playing an important role in maintaining healthy grassland ecosystems (Yang and Yuan, 2019). Insects have been used in a wide range of ecological and environmental applications as they occupy almost every type of terrestrial and aquatic habitat and are extremely sensitive to environmental change (Gerlach et al., 2013). Therefore, an increasing number of studies are using insects as indicator species for the assessment of the success of grassland restoration (Alison et al., 2013; Alignan et al., 2018; Dröse et al., 2021). Studies assessing the impact of grazing, as the main utilization of grassland, have used insect diversity as an indicator with inconsistent results. Compared with grazing exclusion, light or moderate grazing results in high insect diversity, whereas heavy grazing results in low insect diversity (Ma et al., 2017), some studies reported that grazing increased insect diversity (Jerrentrup et al., 2014; Johansson et al., 2017; Zhu et al., 2017, 2020a), while others showed reduced or no significant effects on insect diversity in response to grazing (Wardle et al., 2001). Factors that may have influenced these inconsistent results may include differences in the type of grassland and grazing pattern, including both grazing intensity and the size of the herbivores and insect groups present (O’Neill et al., 2010; Zhu et al., 2015; Ma et al., 2017). In this study, we sampled fields in the desert steppe region of Inner Mongolia with different fencing periods and grazing intensities. We measured insect diversity to provide a reference evaluation of the recovery of the steppes and a scientific basis for the relationship between insect communities and the steppe environment. We hypothesized that insect diversity in pastures that had been fenced longer would be reduced compared with those fenced for shorter times as a result of the negative influence of fencing and, in addition, that the insect diversity would be higher in lightly grazed pastures compared with those subjected to no grazing or heavy grazing. We also expected changes in the species abundance distribution, with reductions in species evenness in longer-fenced pastures compared with those fenced for shorter times as the most competitive group will tend to increase its dominance in the process of community succession. We also hypothesized that there would be variations in the group proportions as some groups would be more affected by grazing than others.
Materials and methods
Study area
The study was conducted at Sonid Right Banner (42°47′19″N, 112°40′20″E, 972 m a.s.l.) and Sonid Left Banner (43°42′44″N, 113°36′12″E, 1070 m a.s.l.) temperate desert steppe in Inner Mongolia, China. This region conforms to a typical arid and semiarid temperate continental climate with rich heat and is characterized by a mean annual temperature of 3.1°C and a mean annual precipitation of 180 mm, with the growing season mainly from May to September (Ren et al., 2011). Evaporation (between 0.15 and 0.30) is several dozen times more than the “precipitation and moisture index.” According to the Chinese Soil Database, the main soil types are chestnut soil, brown soil, and sierozem (China Soil Database, Institute of Soil Science, Chinese Academy of Sciences, 20191). The soil has a thickness of 15–25 cm, with a thin layer of humus. The vegetation is dominated by Stipa klemenzii Roshev., Cleistogenes songorica Roshev., and Artemisia frigida Willd. The grass is sparse and short with less than 30% of coverage, a height of 20–30 cm, and low primary production (Tana et al., 2011).
Design of experiment
We used two periods of fencing enclosures and three grazing intensities in a complete randomized block design to create a total of six treatments (Supplementary Table 1). Two fencing periods, i.e., 3 and 7 years, were selected. For each fencing period, three 45-ha plots (six in total) were established under the same conditions. In each plot, three 15-ha sections (18 in total) were divided and treated with three grazing intensities, i.e., no grazing (CK), light grazing (8 sheep/ha; LG), and high grazing (15 sheep/ha; HG). All the sample plots were enclosed all year round, and the CK plots were not used for grazing, while the LG and HG treatment areas were grazed from May to October every year.
Vegetation and identification
The vegetation of each paddock was surveyed. Three 1 × 1-m2 quadrats were then randomly placed within each sample plot replication, and the vegetation composition and average height and coverage were recorded. The average height of the vegetation was measured. The coverage of the sward was assessed as the percentage of aboveground vegetation within the boundaries of the quadrat. Plants were identified to the species or morphospecies level using specialized literature (Cao, 2017), and the numbers were recorded.
Insect sampling and identification
We surveyed insects using three randomly located 20 × 20-m2 quadrats within each sample plot replication. There were 54 quadrats in total. Insects were sampled between July and August 2012 using a checkerboard sweep net method (38 cm in diameter), whereby samples were collected by making a total of 250 sweeps, with five vertical sweeps (every 5 m) and five horizontal sweeps (every 80 cm) (Andreas and Teja, 2002). The sampled insects were stored in plastic bags with ethyl acetate, followed by preservation in 95% ethanol before sorting into taxonomic groups. We identified insects to the species or morphospecies level according to appropriate identification keys (Nonnaizab et al., 1988, 1999).
Phototropic insects, such as Lepidoptera, were attracted using a white tent (1 × 1 × 2 m) and a high-pressure mercury lamp (250 W). For insect collection, killing jars supplied with a small amount of ether were used. All samples were sorted to the species or morphospecies level in the field and kept in glassine envelopes. Sampling was carried out from 9.00 to 12.00 p.m. between July and August 2012. All specimens were identified in consultation with taxonomic experts.
Data analysis
All statistical analyses were conducted in R version 4.1.3 (R Core Team, 2022). Differences in plant cover, plant height, plant Shannon–Wiener index, and plant Simpson index among the two fencing periods and three grazing intensities were analyzed using linear mixed-effects models with the “lme” function of the nlme package. Linear mixed-effects models were followed by the analysis of variance (ANOVA, with the “anova” function) and Tukey tests using the multcomp package.
The insect diversity index was calculated by Past 4.08 software (Hammer et al., 2001). To assess insect community diversity patterns in different grazing intensities and fencing years, principal coordinate analysis (PCoA) with Bray–Curtis distances was used in the vegan package (Oksanen et al., 2018). Permutational multivariate analysis of variance (PERMANOVA) (Anderson, 2017) was used to evaluate differences in communities using the “adonis” function in the vegan package (Oksanen et al., 2018).
To analyze the relationships between grazing intensities and fencing years (fixed effects) and insect abundance, richness, Shannon diversity, and Simpson diversity (response variables), we created generalized linear mixed models (GLMMs) using the glmmTMB function in the glmmTMB package with the model: diversity measure ∼ grazing × fencing + (1| grazing/plot). This was followed by the R2 explained by fixed and random factors using the “r.squaredGLMM” function of the MuMIn R package. A Poisson link was used for species richness, whereas a Gaussian link was used for the other measures of diversity. Log transformation of data was used in cases where improvement was required for model fit. Redundancy analysis (RDA) was used to visualize the associations between the abundances of insect orders and the plant attributes, using the rda package. Ordination plots were compiled for insect order abundance in relation to plant variables, including plant species richness, cover, height, and plant Shannon–Wiener index, using the “envfit” function of the vegan package with 999 permutations.
Results
The total number of plant species found in the plots was as follows: 47 species in no grazed pastures, 48 species in lightly grazed pastures, 41 species in heavily grazed pastures, 47 in 3-year fencing grasslands, and 48 species in the 7-year fencing grasslands (Supplementary Table 2). In a comparison of the two types of fencing grasslands, 33 plant species were found in both treatments, 14 plant species were found only in the 3-year fencing treatment, and 15 plant species were exclusive to the 7-year fencing treatment (Supplementary Figure 1). No differences in plant species richness, plant Shannon diversity, plant cover and plant height were found (P > 0.05, Table 1 and Supplementary Figure 2). The ungrazed (CK) sites had a higher Shannon diversity than the heavily grazed (HG) sites (P < 0.05), which did not differ from the lightly grazed (LG) sites (P > 0.05, Supplementary Figure 3). Plant species richness showed no differences between the three grazing intensity sites. Significant differences were found for plant cover and plant height, which were highest in the CK sites, lowest in the HG sites, and intermediate in the LG sites (Supplementary Figure 3).
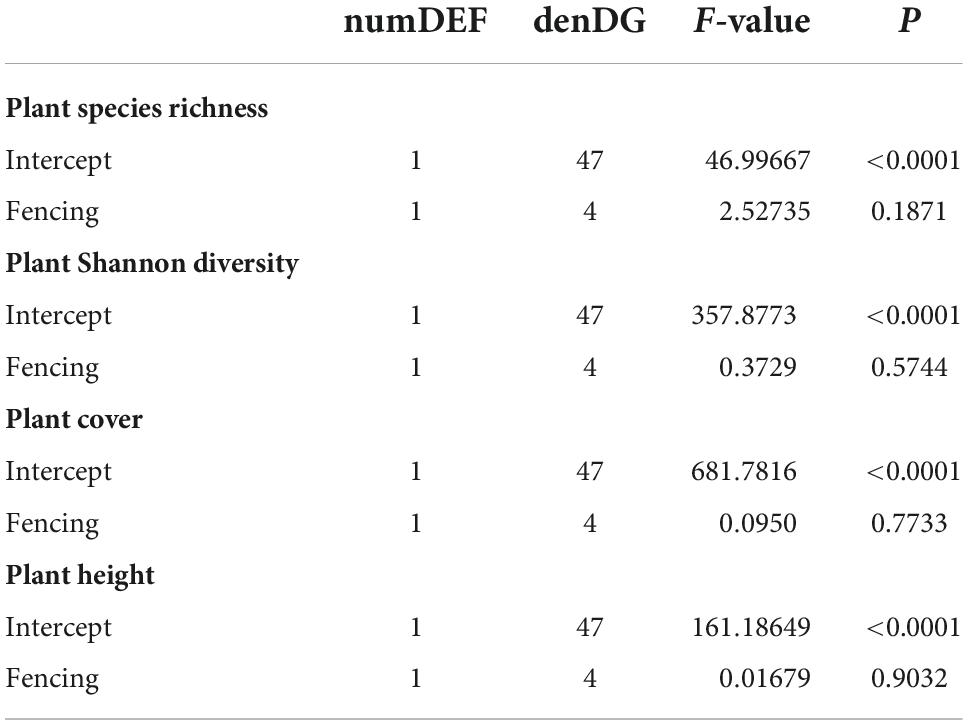
Table 1. ANOVA tables of mixed-effects models with plant species richness, plant Shannon diversity, plant height, and plant cover as dependent variables in two fencing periods in a desert steppe: 3-year enclosure and 7-year enclosure. The plant cover was log-transformed.
In total, 2601 individuals were collected from all sites, which belonged to 165 species of 53 families of eight orders (Supplementary Table 3). Cicadellidae, Gomphoceridae, Coreidae, and Meloidae represented most of the collected insects (Figure 1), accounting for 35, 15, 7.7, and 5% of the total abundance, respectively (Figure 1). Cicadellidae was the most abundant family in 3-year enclosure sites, representing 45% of the total abundance, followed by Gomphoceridae with 7.5% of the total abundance. In the 7-year enclosure sites, the proportion of Cicadellidae decreased to 26%, while Gomphoceridae increased to 22% of the total abundance (Figure 1). Overall, the species composition differed significantly between the different enclosure sites and different grazing sites (grazing: R2 = 0.03, P = 0.048; fencing: R2 = 0.07, P = 0.001). Our PERMANOVA pairwise comparisons indicated that all pairs of grazing intensity sites differed from each other, and the PCoA ordination plot showed clear distinctions, especially between insect communities from 3-year and 7-year enclosure sites (Figure 2). Among the 7-year enclosure sites, CK sites were markedly more distant from the LG and HG sites, which were closer to each other. This was also the case for the 3-year enclosure sites.
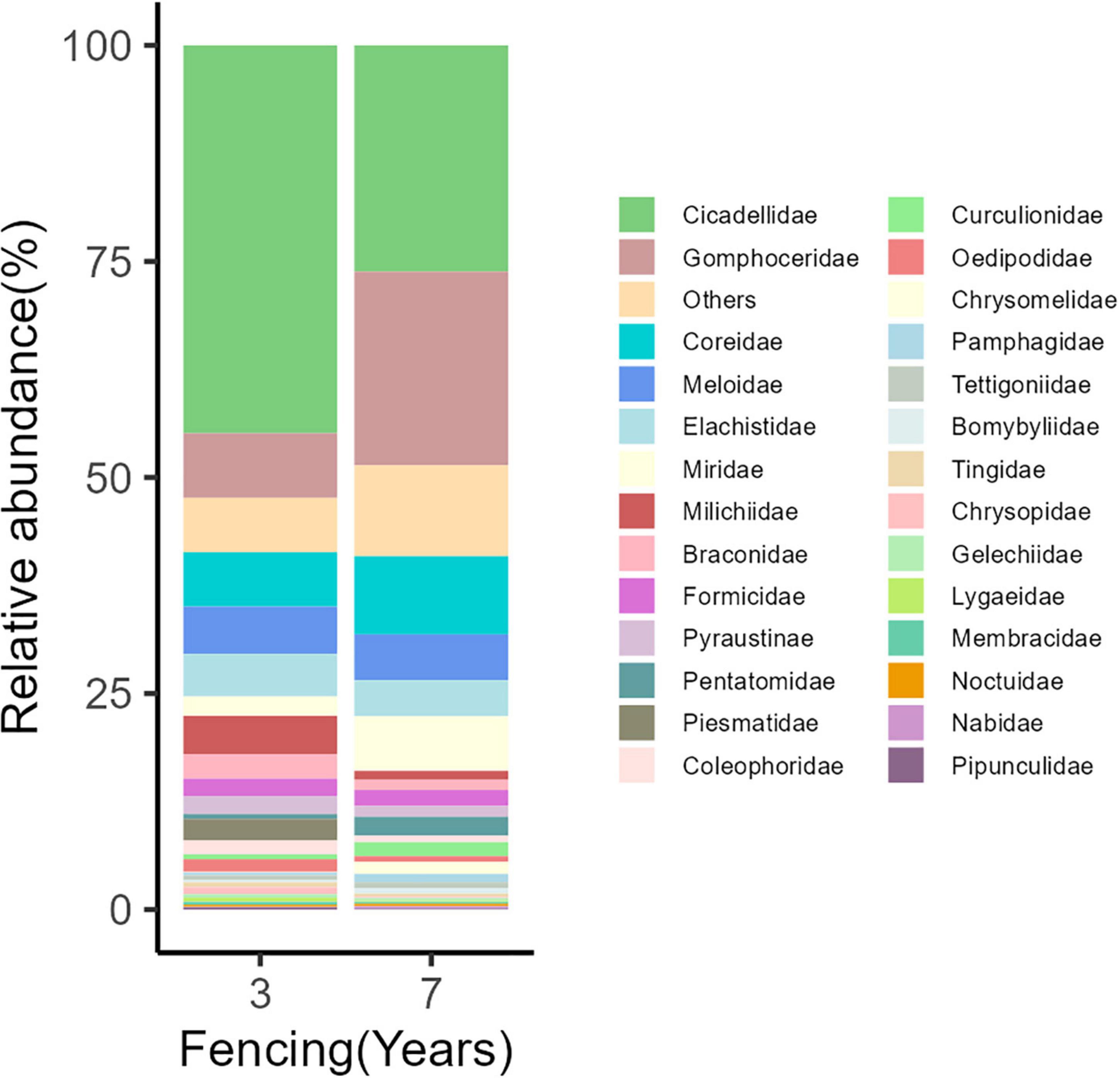
Figure 1. Proportional abundance of insect species collected from two fencing periods in a desert steppe.
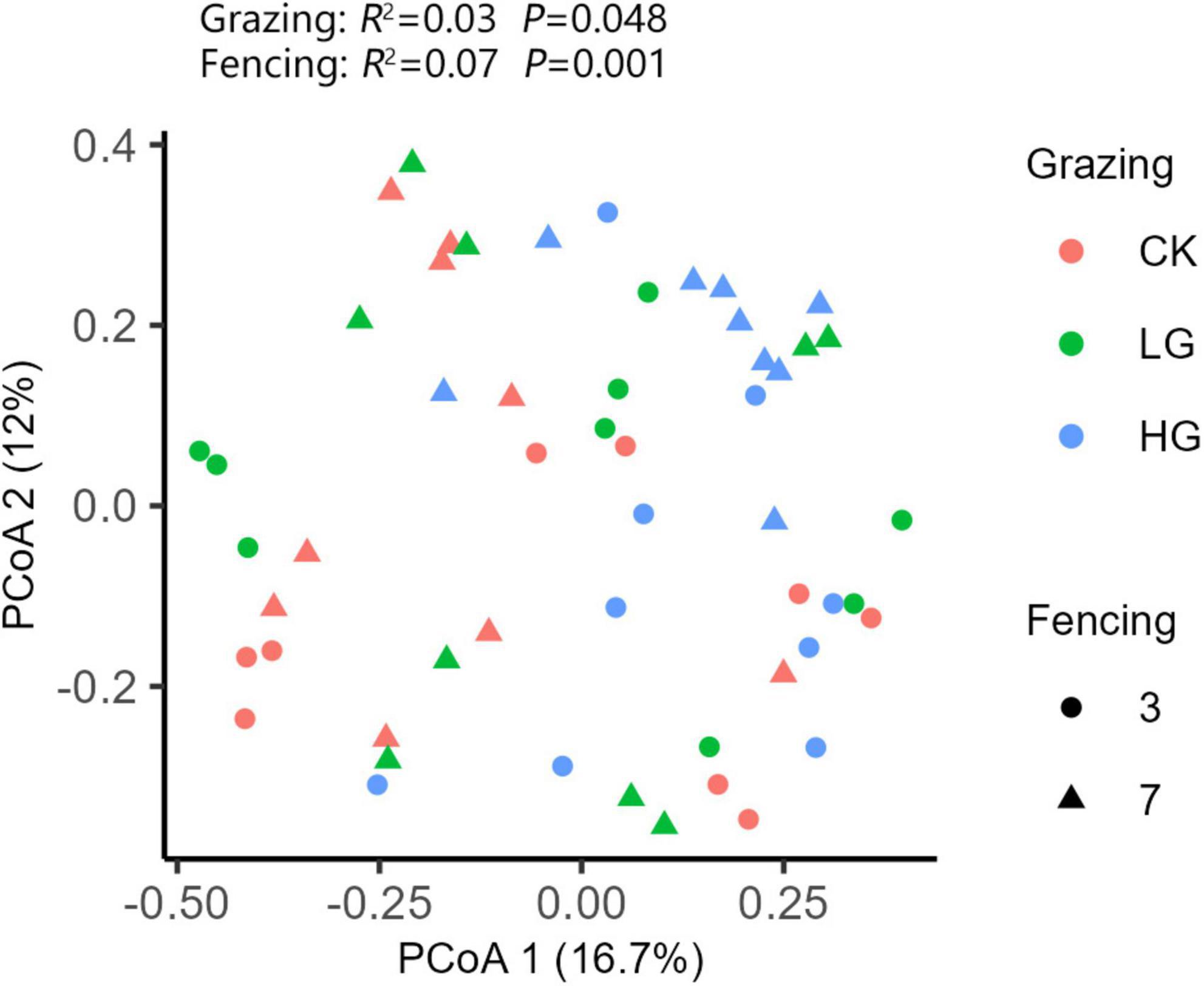
Figure 2. Principal coordinate analysis (PCoA) of insect communities across two fencing periods and three grazing intensities. PCoA was generated by the Bray–Curtis dissimilarity method.
Insect abundance was higher in the CK sites than in the HG sites (P < 0.05), whereas there was no difference between the CK and LG sites (P > 0.05, Table 2 and Figure 3A). This was consistent with the trend of insect species richness (Table 3 and Figure 3B). However, there were no differences in insect Shannon and Simpson diversity indices between the CK and LG sites and between the CK and HG sites (Tables 4, 5 and Figures 3C,D).
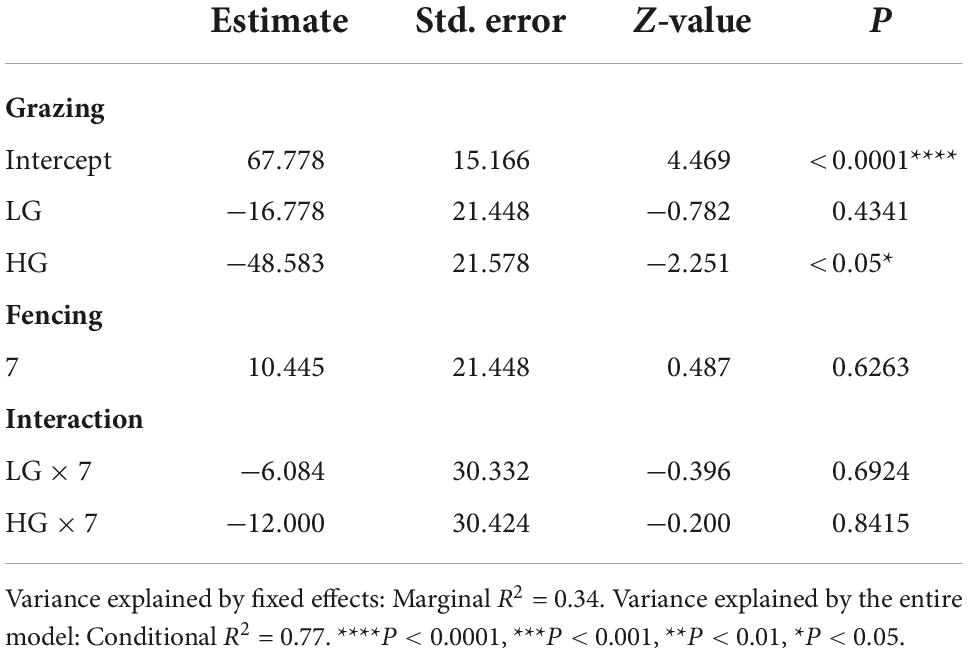
Table 2. Results of generalized linear mixed models (GLMMs) for species abundance of insects sampled after 3 or 7 fencing years in three grazing treatments in a desert steppe: Ungrazed plot (CK, used as reference), light grazed (LG), and heavily grazed (HG).
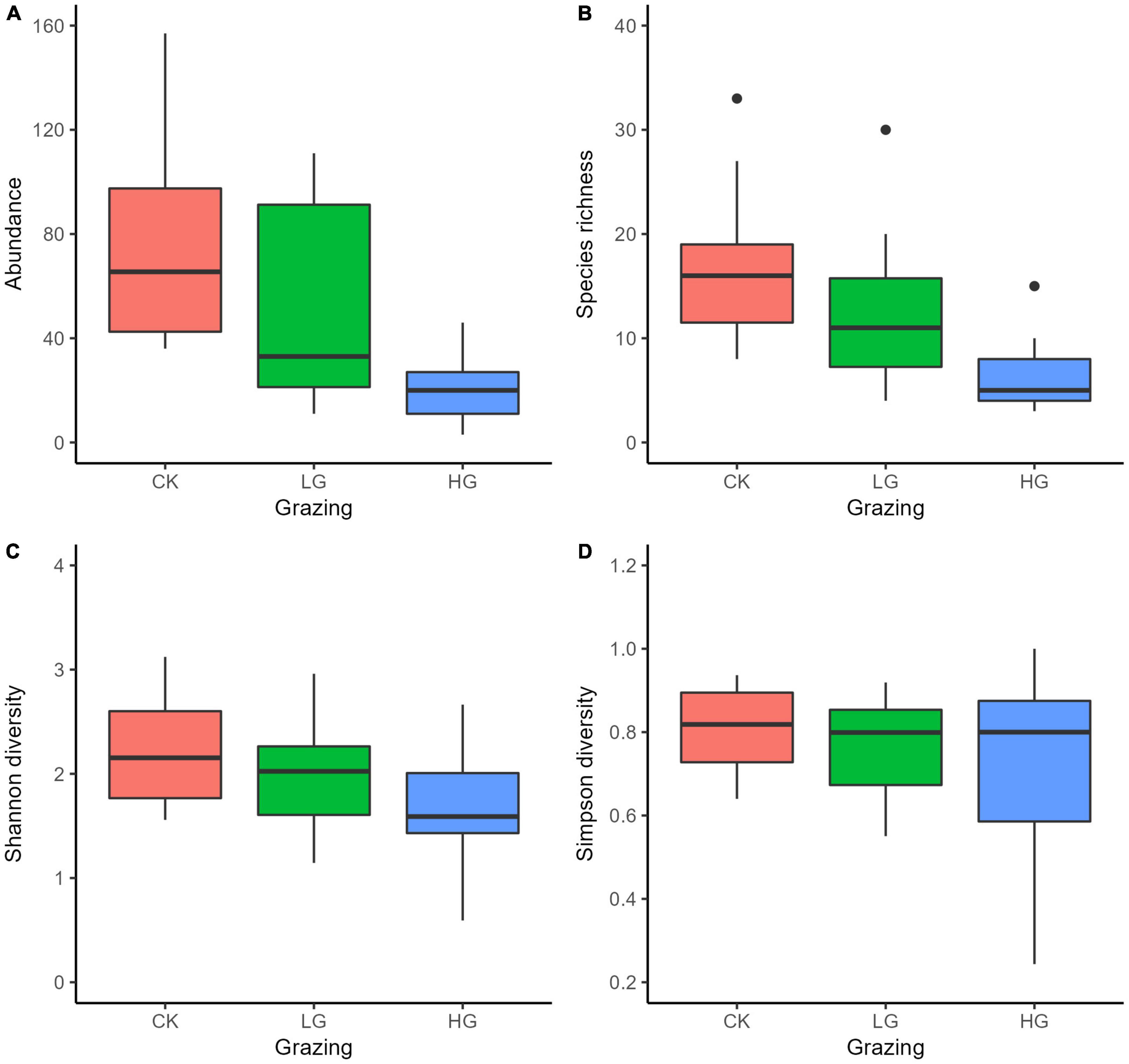
Figure 3. Boxplots of insect species abundance (A), richness (B), Shannon diversity (C), and Simpson diversity (D) in three grazing intensities in a desert steppe: Ungrazed plot (CK), light grazed (LG), and heavily grazed (HG).
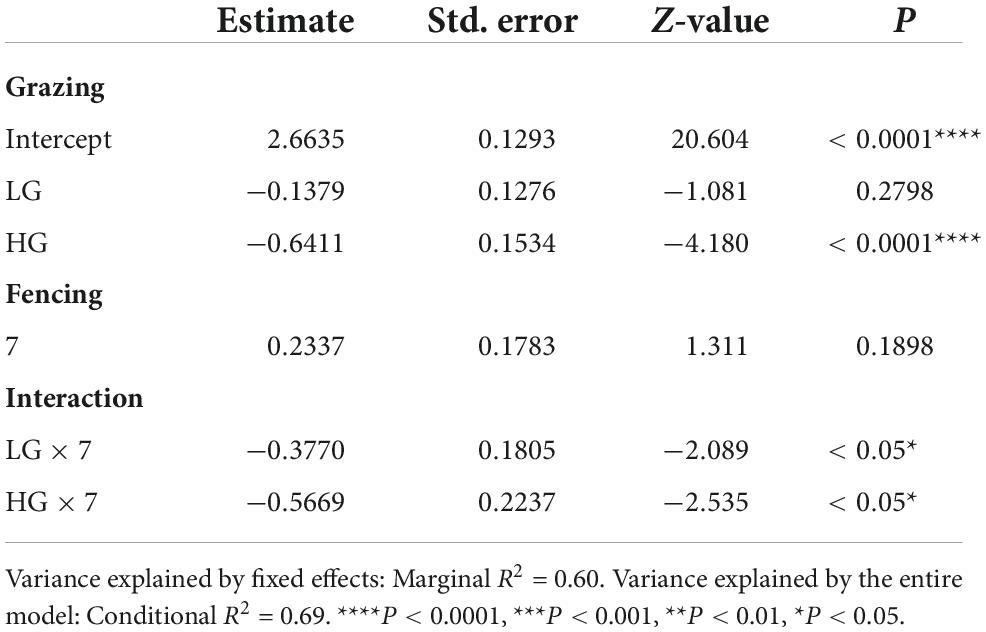
Table 3. Results of generalized linear mixed models (GLMMs) for species richness of insects sampled after 3 or 7 fencing years in three grazing treatments in a desert steppe: Ungrazed plot (CK, used as reference), light grazed (LG), and heavily grazed (HG).
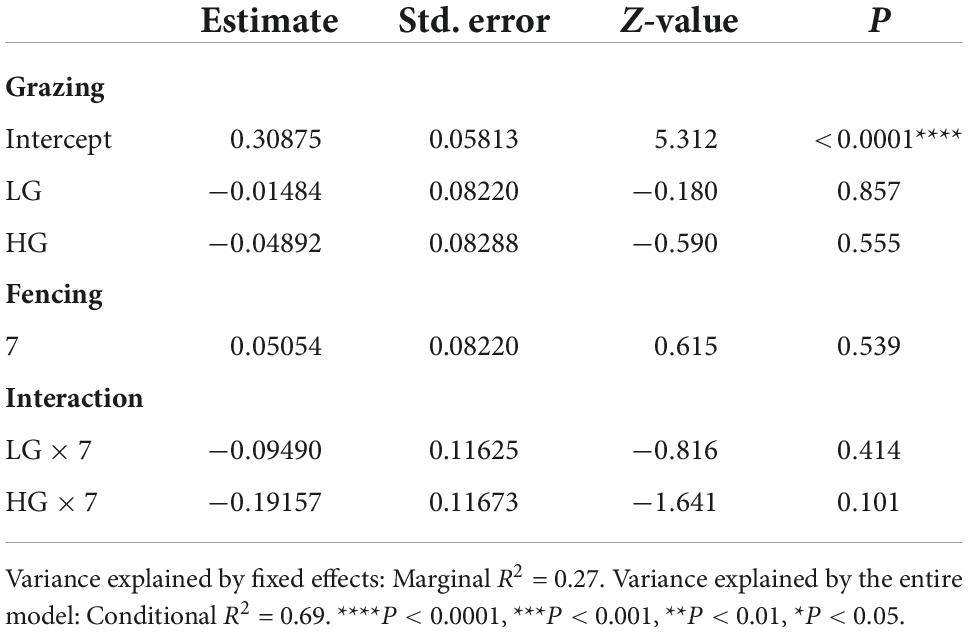
Table 4. Results of generalized linear mixed models (GLMMs) for species Shannon diversity of insects sampled after 3 or 7 fencing years in three grazing treatments in a desert steppe: Ungrazed plot (CK, used as reference), light grazed (LG), and heavily grazed (HG).
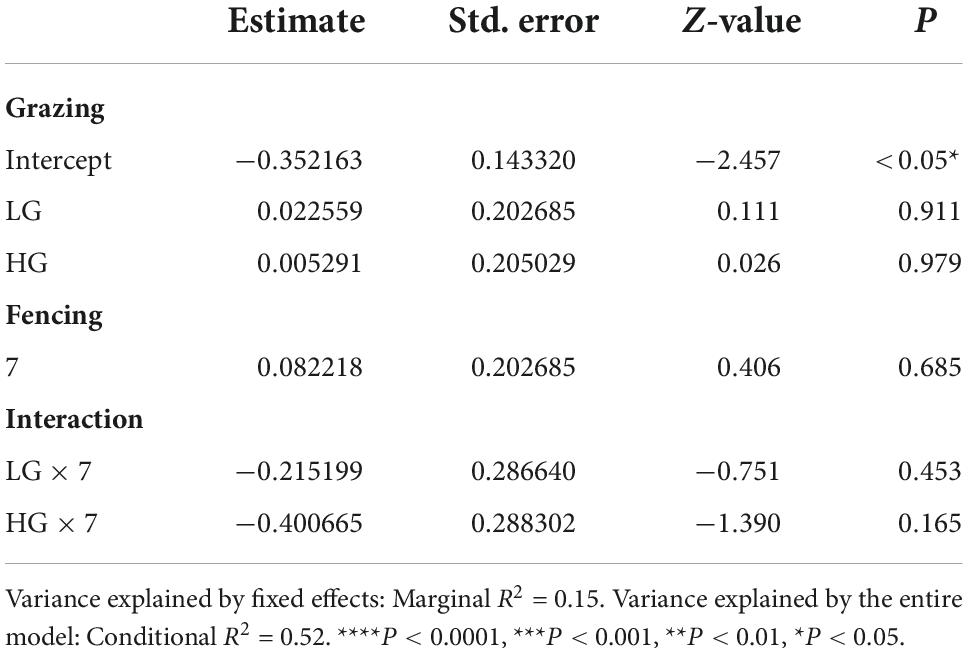
Table 5. Results of generalized linear mixed models (GLMMs) for species Simpson diversity of insects sampled after 3 or 7 fencing years in three grazing treatments in a desert steppe: Ungrazed plot (CK, used as reference), light grazed (LG), and heavily grazed (HG).
There was no difference in insect abundance between the 3- and 7-year enclosure sites (P = 0.6236, Table 2 and Figure 4A). We also found no significant interactions between grazing and fencing in relation to insect abundance (Table 2 and Supplementary Figure 4A). Higher insect abundance was seen in the LG sites, compared with the CK and HG sites in the 3-year enclosure sites, while the CK sites showed the highest abundance in the 7-year enclosure sites (Supplementary Figure 4A). Insect species richness did not differ between the two enclosure times (Table 3 and Figure 4B). However, we found a significant relationship between species richness and both grazing and fencing (Table 3 and Supplementary Figure 4B). In 7-year enclosure sites, species richness was significantly higher in the CK sites than in the LG and HG sites (Table 3 and Supplementary Figure 4B). Therefore, grazing strongly reduced species richness in the 7-year enclosure sites. The Shannon and Simpson diversity indices varied with the different lengths of fencing without showing any obvious trend (Tables 4, 5 and Supplementary Figures 4C,D). Diversity was higher in the 3-year enclosure sites than in the 7-year sites (Supplementary Figures 4C,D), implying that the longer fencing time did not significantly improve the insect diversity. Grazing reduced both Shannon and Simpson diversities, although not significantly (Tables 4, 5).
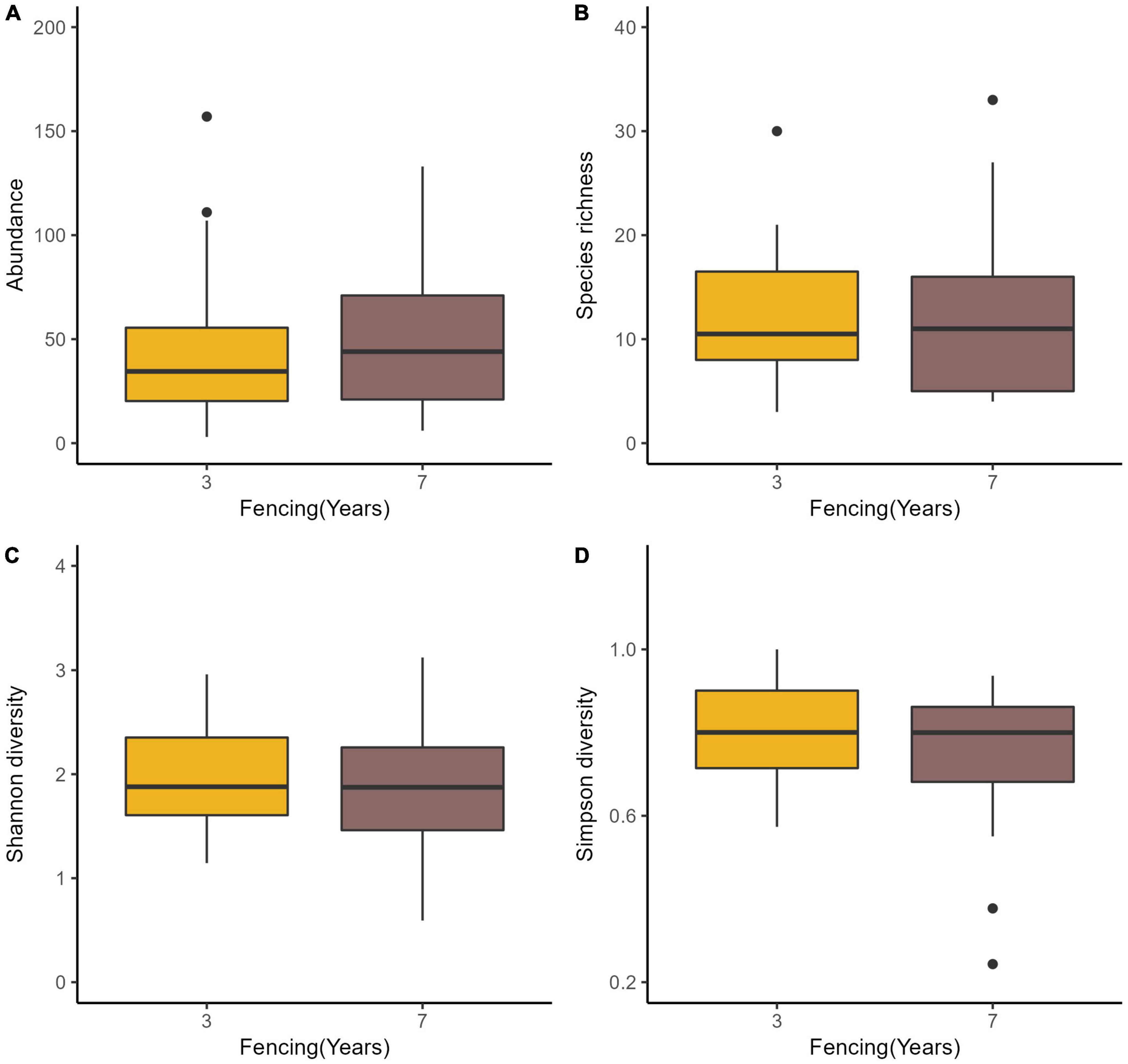
Figure 4. Boxplots of insect species abundance (A), richness (B), Shannon diversity (C), and Simpson diversity (D) in two fencing periods in a desert steppe: 3 fencing years and 7 fencing years.
About 2.87% of the insect composition variation was explained by plant attributes (F = 2.56; P = 0.001) in the RDA model (Figure 5). Most of the variation in abundance was explained by axis 1, which was negatively associated with all the plant attributes, the same as the second axis of the RDA. Axis 1 separated the CK sites from the LG and HG sites while axis 2 separated the LG sites from the CK sites and some of the HG sites for all plant attributes. Hemiptera were found to be more abundant in the localities with higher plants while Orthoptera were more abundant in areas with lower plants. Coleoptera, Hymenoptera, and Lepidoptera were negatively associated with all plant attributes. In the CK sites fenced for 3 years, Neuroptera was strongly related to plant cover.
Discussion
In this study, we investigated the effects of fencing and grazing and their interactions on the structure of the insect communities of the desert grassland. Fencing represents one of the restoration measures applied in grassland areas to mitigate the negative effects of grazing on productivity and the ecosystem. In contrast to our expectations, we found almost no significant differences in either plant or insect species diversity between the sites fenced for 3 and 7 years. This showed that the fenced pastures established similar biological communities, irrespective of the fencing period, suggesting that fencing was successful in terms of community recovery (Steiner et al., 2016). Furthermore, fencing was found to have a significant effect on vegetation. For a certain period of time, enclosure eliminates disturbances to plant community structures produced by livestock trampling and foraging and enhances seedling germination, conducive to the recovery of plant productivity and the succession of community structures (Wang et al., 2020). Grazing can also alter the structures of plant communities by reducing species dominance and promoting the restoration of rare species (Pulungan et al., 2019). Our results showed that grazing significantly affected both plant height and cover, although it had no significant effect on the plant species diversity.
Our study showed varying degrees of change in the dominant insect group in relation to the different fencing periods. The proportion of the dominant insect family—Gomphoceridae—was found to be quite different in each plot, becoming more dominant as the time of enclosure increased, while that of Cicadellidae declined (Figure 1). This illustrates that extended fencing periods, together with pasture enclosure, away from grazing cattle and other large animals, allow previously less abundant insect groups to assume positions of dominance in the community in contrast to others (Zhao et al., 2010). Biotic organisms have been found to vary in their sensitivities to grassland restoration and degradation (Helbing et al., 2021), and our findings support this opinion (Figure 4). Besides direct effects, livestock grazing can also affect insect diversity indirectly in many cases. These indirect effects mainly include changes in vegetation, the spatial heterogeneity of the community, and microclimatic conditions resulting from grazing (Jerrentrup et al., 2014; Zhu et al., 2020b). We observed that the abundance of Orthoptera was negatively associated with vegetation height in the pastures. This may be due to the supply of oviposition sites, with more new growth providing greater numbers of sites and changes in microclimate favoring locust hatching following grazing (Zhu et al., 2020b).
According to previous studies, different grazing management strategies significantly influences the composition of grassland insect communities (Poyry et al., 2005; KÅrösi et al., 2012). Our study showed that different fencing periods and grazing intensities had different effects on the insect community. We observed that light grazing maintained insect variety in areas fenced for shorter times while the variety decreased with increased grazing intensity in pastures fenced for longer time periods (Supplementary Figure 4). We could see that species diversity and insect richness did not increase as the fencing period increased. It was, thus, possible to reach a maximum insect diversity during the restoration. As the pressure of grazing increased, insect diversity decreased to a greater extent at 7-year enclosure sites than at 3-year sites, suggesting that fencing for 7 years had no significant advantage, even in the presence of interference by large animals, probably because the long-term enclosure promoted the status of dominant species and the vegetation structure became progressively homogenized (Karg et al., 2015). These changes in the vegetation community led to a decline in the variety of insects. In contrast, in the plots fenced for 3 years with light grazing, species richness did not differ significantly from sites without grazing, demonstrating that light disturbances benefited both the number and diversity of insects due to the maintenance of high plant diversity (Koch et al., 2016; Ferreira et al., 2020). We found no significant effects of the fencing period and grazing intensity on insect Shannon and Simpson diversity. Similar to insect species richness, after the inclusion of grazing, the insect Shannon diversity decreased to a greater extent for 7-year of enclosure than for 3-year of the enclosure. This further demonstrated that extending the fencing period does not contribute significantly to the maintenance of biodiversity. Hence, grasslands, where grazing has been prohibited, should gradually be restored according to the policy of “rotation grazing.”
It was found that under the same fencing period, the ungrazed plots showed the highest diversity, with light grazing showing the second highest diversity, and heavy grazing the lowest diversity. This indicated that an increase in grazing stocking rates altered the abiotic and biotic properties so that the dominance of more competitive or disturbance-tolerant species was favored, thus reducing insect diversity (Gossner et al., 2016). It has been found that when the grazing system was changed, by allowing disturbance by sheep in the desert steppe, the plant species, insect species, soil, and environment changed as a result (Deng et al., 2014; Zhou et al., 2017). Previous research has shown that factors such as biomass, coverage, and species composition affect indicators such as the diversity and richness of insects (Zhu et al., 2012; Jerrentrup et al., 2014). These findings were supported by our RDA results (Figure 5). Orthoptera was favored by a low cover and low height of plants in the HG sites, indicating that Orthoptera species were influenced by grazing through modifications in the plant community structures, which is consistent with the results of recent findings (Schwarz and Fartmann, 2022). In contrast, Diptera and Hymenoptera were more strongly influenced by plant height as they tend to have specific habitat requirements, such as for refugees or oviposition (Morris, 2000).
In the present study, the results may have been influenced by the climate at the time of sampling as during the months from April to August 2010, the rainfall recorded was less than that at other times over the Sonid Right Banner and the Sonid Left Banner in Inner Mongolia, and the critical period for herbage growth was during June, July, and August. Continuous drought and less rainfall, in addition to the higher-than-average temperatures, may have affected both the growth of herbage and the accumulation of grass productivity. This is likely to have decreased the insect diversity of the grassland, especially in the plots with slight disturbance. Thus, further research on fencing and grazing, together with continuous monitoring of insect diversity, and the study of the influence of environmental factors such as climate are of great importance to gain a deeper and more comprehensive understanding of the regulatory mechanisms underlying environmental influences on the recovery of insect diversity.
Data availability statement
The original contributions presented in this study are included in the article/Supplementary material, further inquiries can be directed to the corresponding author.
Ethics statement
The animal study was reviewed and approved by the Ethics Committee of the Chinese Academy of Agricultural Sciences.
Author contributions
NW and XS conceived and designed the methods and framework, analyzed and interpreted the data, wrote the manuscript, and discussed the results. JW contributed to the collection and interpretation of the data and discussion of results. LW conducted the analyses and wrote the manuscript. All authors contributed to the article and approved the submitted version.
Funding
This project was funded by the National Natural Science Foundation of Inner Mongolia (2020JQ04), the Critical Technology Project of Inner Mongolia (No. 2021ZD0011-2-4), and the National Science and Technology Fundamental Resources Investigation Program of China (Grant No. 2019FY100400).
Acknowledgments
We thank Xiaoshuan Bai and Hongwei Li for their help in collecting specimens.
Conflict of interest
The authors declare that the research was conducted in the absence of any commercial or financial relationships that could be construed as a potential conflict of interest.
Publisher’s note
All claims expressed in this article are solely those of the authors and do not necessarily represent those of their affiliated organizations, or those of the publisher, the editors and the reviewers. Any product that may be evaluated in this article, or claim that may be made by its manufacturer, is not guaranteed or endorsed by the publisher.
Supplementary material
The Supplementary Material for this article can be found online at: https://www.frontiersin.org/articles/10.3389/fevo.2022.1021677/full#supplementary-material
Supplementary Figure 1 | Venn diagrams of differences in the plant species in two fencing periods (A) and differences in the plant species in three grazing intensities (B).
Supplementary Figure 2 | Boxplots of plant species richness (number of species, A), plant Shannon diversity (B), plant cover (C), and plant height (D) in two fencing periods in a desert steppe: 3 fencing years and 7 fencing years.
Supplementary Figure 3 | Boxplots of plant species richness (number of species, A), plant Shannon diversity (B), plant height (C), and plant cover (D) in three grazing intensities in a desert steppe: Ungrazed plot (CK), light grazed (LG), and heavily grazed (HG).
Supplementary Figure 4 | Boxplots showing monthly variations of insect species abundance (A), richness (B), Shannon diversity (C), and Simpson diversity (D) in two fencing periods in a desert steppe: Ungrazed plot (CK), light grazed (LG), and heavily grazed (HG).
Supplementary Table 1 | Experimental design of grazing trial.
Supplementary Table 2 | Plant species in each plot in a desert grassland in Inner Mongolia.
Supplementary Table 3 | Insect species and abundances in each plot in a desert grassland in Inner Mongolia.
Footnotes
References
Alignan, J. F., Debras, J. F., and Dutoit, T. (2018). Orthoptera prove good indicators of grassland rehabilitation success in the first French natural asset reserve. J. Nat. Conserv. 44, 1–11. doi: 10.1016/j.jnc.2018.04.002
Alison, J., Duffield, S. J., Morecroft, M. D., Marrs, R. H., and Hodgson, J. A. (2013). Successful restoration of moth abundance and species-richness in grassland created under agri-environment schemes. Biol. Conserv. 213, 51–58. doi: 10.1016/j.biocon.2017.07.003
Anderson, M. J. (2017). “Permutational multivariate analysis of variance (PERMANOVA),” in Wiley statsref: Statistics reference online, eds N. Balakrishnan, T. Colton, B. Everitt, W. Piegorsch, F. Ruggeri, and J. L. Teugels (New York, NY: John Wiley & Sons), 1–15. doi: 10.1002/9781118445112.stat07841
Andreas, K., and Teja, T. (2002). Contrasting responses of plant and insect diversity to variation in grazing intensity. Biol. Conserv. 106, 293–302. doi: 10.1016/S0006-3207(01)00255-5
Cheng, J. M., Jing, G. H., Wei, L., and Jing, Z. B. (2016). Long-term grazing exclusion effects on vegetation characteristics, soil properties and bacterial communities in the semi-arid grasslands of China. Ecol. Eng. 97, 170–178. doi: 10.1016/j.ecoleng.2016.09.003
Deng, L., Sweeney, S., and Shangguan, Z. P. (2014). Grassland responses to grazing disturbance: Plant diversity changes with grazing intensity in a desert steppe. Grass Forage Sci. 69, 524–533. doi: 10.1111/gfs.12065
Dröse, W., Podgaiski, L. R., Gossner, M. M., Meyer, S. T., Hermann, J. M., Leidinger, J., et al. (2021). Passive restoration of subtropical grasslands leads to incomplete recovery of ant communities in early successional stages. Biol. Conserv. 264:109387. doi: 10.1016/j.biocon.2021.109387
Ferreira, P. M. A., Andrade, B. O., Podgaiski, L. R., Dias, A. C., Pillar, V. D., Overbeck, G. E., et al. (2020). Long-term ecological research in southern Brazil grasslands: Effects of grazing exclusion and deferred grazing on plant and arthropod communities. PLoS One 15:e0227706. doi: 10.1371/journal.pone.0227706
Gerlach, J., Samways, M., and Pryke, J. (2013). Terrestrial invertebrates as bioindicators: An overview of available taxonomic groups. J. Insect Conserv. 17, 831–850. doi: 10.1007/s10841-013-9565-9
Gossner, M. M., Lewinsohn, T. M., Kahl, T., Grassein, F., Boch, S., Prati, D., et al. (2016). Land-use intensification causes multitrophic homogenization of grassland communities. Nature 540, 266–269. doi: 10.1038/nature20575
Hammer, Ø, Harper, D. A., and Ryan, P. D. (2001). PAST: Paleontological statistics software package for education and data analysis. Palaeontol. Electron. 4, 1–9.
He, M., Zhou, G., Yuan, T., van Groenigen, K. J., Shao, J., and Zhou, X. (2019). Grazing intensity significantly changes the C: N: P stoichiometry in grassland ecosystems. Glob. Ecol. Biogeogr. 29, 355–369. doi: 10.1111/geb.13028
Helbing, F., Fartmann, T., and Poniatowski, D. (2021). Restoration measures foster biodiversity of important primary consumers within calcareous grasslands. Biol. Conserv. 256:109058. doi: 10.1016/j.biocon.2021.109058
Jerrentrup, J. S., Wrage-Monnig, N., Röver, K. U., and Isselstein, J. (2014). Grazing intensity affects insect diversity via sward structure and heterogeneity in a long-term experiment. J. Appl. Ecol. 51, 968–977. doi: 10.1111/1365-2664.12244
Johansson, V., Knape, J., and Franzén, M. (2017). Population dynamics and future persistence of the clouded apollo butterfly in southern Scandinavia: The importance of low intensity grazing and creation of habitat patches. Biol. Conserv. 206, 120–131. doi: 10.1016/j.biocon.2016.12.029
Karg, J., Kujawa, K., Manhart, C., Marschalek, H., Neugebauer, K. R., and Sachteleben, J. (2015). Restoration of subalpine species-rich grasslands: Short-term vs long-term changes in the fensity and fiversity of above-ground insects. Pol. J. Ecol. 63, 142–158.
KÅrösi, Á, Batáry, P., Orosz, Á, Rédei, D., and Báldi, Á (2012). Effects of grazing, vegetation structure and landscape complexity on grassland leafhoppers (hemiptera: Auchenorrhyncha) and true bugs (hemiptera: Heteroptera) in Hungary. Insect Conserv. Divers. 5, 57–66. doi: 10.1111/j.1752-4598.2011.00153.x
Koch, C., Conradi, T., Gossner, M. M., Hermann, J. M., Leidinger, J., Meyer, S. T., et al. (2016). Management intensity and temporary conversion to other land use types affect plant diversity and species composition of subtropical grasslands in southern Brazil. Appl. Veg. Sci. 19, 589–599. doi: 10.1111/avsc.12262
Ma, J., Huang, X., Qin, X., Ding, Y., Hong, J., Du, G. L., et al. (2017). Large manipulative experiments revealed variations of insect abundance and trophic levels in response to the cumulative effects of sheep grazing. Sci. Rep. 7:11297. doi: 10.1038/s41598-017-11891-w
Morris, M. G. (2000). The effects of structure and its dynamics on the ecology and conservation of arthropods in British grasslands. Biol. Conserv. 95, 129–142. doi: 10.1016/S0006-3207(00)00028-8
Nonnaizab, Liu, Q., Yan, D. P., and Fan, X. F. (1988). Fauna of inner Mongolia (hemiptera: Heteroptera). Hohhot: People press of Inner Mongolia.
Nonnaizab, Qi, B. Y., and Li, Y. B. (1999). Insects of inner Mongolia China. Hohhot: People Press of Inner Mongolia.
Oksanen, J., Blanchet, F. G., Friendly, M., Kindt, R., Legendre, P., McGlinn, D., et al. (2018). Vegan: Community Ecology Package. Available online at: https://CRAN.R-project.org/package=vegan (accessed October 23, 2022).
O’Neill, K. M., Olson, B. E., Wallander, R., Rolston, M. G., and Seibert, C. E. (2010). Effects of livestock grazing on grasshopper abundance on a native rangeland in Montana. Environ. Entomol. 39, 775–786. doi: 10.1603/EN09173
Poyry, J., Lindgeren, S., Salminen, J., and Kuussaarim, M. (2005). Responses of butterfly and moth species to restored cattle grazing in semi-natural grasslands. Biol. Conserv. 122, 465–478. doi: 10.1016/j.biocon.2004.09.007
Pulungan, M. A., Suzuki, S., Gavina, M. K. A., Tubay, J. M., Ito, H., Nii, M., et al. (2019). Grazing enhances species diversity in grassland communities. Sci. Rep. 9:11201. doi: 10.1038/s41598-019-47635-1
R Core Team (2022). R: A language and environment for statistical computing. Vienna: R Foundation for Statistical Computing.
Ren, H. R., Zhou, G. S., and Zhang, X. S. (2011). Estimation of green aboveground biomass of desert steppe in inner Mongolia based on red-edge reflectance curve area method. Biosyst. Eng. 109, 385–395. doi: 10.1016/j.biosystemseng.2011.05.004
Schwarz, C., and Fartmann, T. (2022). Traditional grazing management creates heterogeneous swards and fosters grasshopper densities. Insect Sci. 1–14. doi: 10.1111/1744-7917.13041
Steiner, M., Öckinger, E., Karrer, G., Winsa, M., and Jonsell, M. (2016). Restoration of semi-natural grasslands, a success for phytophagous beetles (curculionidae). Biodivers. Conserv. 25, 3005–3022. doi: 10.1007/s10531-016-1217-4
Tana, Wang, H., and Zhao, S. Z. (2011). Effects of grazing sheep on forage nutrition contents in recovering degraded grassland. Chin. J. Grassl. 33, 44–50.
Wang, F., He, Y. T., Fu, G., Niu, B., Zhang, H. R., Li, M., et al. (2020). Effects of enclosure on plant and soil nutrients in different types of alpine grassland. J. Resour. Ecol. 11, 290–297. doi: 10.5814/j.issn.1674-764X.2020.03.006
Wardle, D. A., Barker, G. M., Yeates, G. W., Bonner, K. I., and Ghani, A. (2001). Introduced browsing mammals in New Zealand natural forests: Aboveground and belowground consequences. Ecol. Monogr. 71, 587–614.
Yang, X. Z., and Yuan, M. L. (2019). Effect of grazing on insect communities in grassland ecosystems: Research status and progress. Pratacult. Sci. 36, 2937–2951. doi: 10.11829/j.issn.1001-0629.2018-0040
Yong, M., Masato, S., Banzragch, N., Bi, L. G., Gao, H. L., and Wang, Y. S. (2021). Impacts of land surface conditions and land use on dust events in the inner Mongolian grasslands, China. Front. Ecol. Evol. 9:664900. doi: 10.3389/fevo.2021.664900
Zhang, G., Kang, Y., Han, G., and Sakurai, K. (2010). Effect of climate change over the past half century on the distribution, extent and NPP of ecosystems of inner Mongolia. Glob. Change Biol. 17, 377–389. doi: 10.1111/j.1365-2486.2010.02237.x
Zhang, G., Sui, X., Li, Y., Jia, M., Wang, Z., Han, G., et al. (2020). The response of soil nematode fauna to climate drying and warming in stipa breviflora desert steppe in inner Mongolia. China. J. Soils Sediments 20, 2166–2180. doi: 10.1007/s11368-019-02555-5
Zhao, H. R., Meng, Q. F., and Gao, W. T. (2010). Effect of enclosure period on structure of insect communities on grassland in western Jilin province. J. Northeast For. Univ. 38, 108–111. doi: 10.13759/j.cnki.dlxb.2010.11.037
Zhou, G., Zhou, X., He, Y., Shao, J., Hu, Z., Liu, R., et al. (2017). Grazing intensity significantly affects belowground carbon and nitrogen cycling in grassland ecosystems: A meta-analysis. Glob. Change Biol. 23, 1167–1179. doi: 10.1111/gcb.13431
Zhu, H., Qu, Y., Zhang, D., Li, J., Wen, M., Wang, D. L., et al. (2017). Impacts of grazing intensity and precipitation on a grasshopper assemblage (orthoptera: Acrididae) in a meadow steppe. Ecol. Entomol. 42, 458–468. doi: 10.1111/een.12403
Zhu, H., Li, H., Yang, Z., Ahungu, A. B., Fei, S., Luo, W., et al. (2020a). Intensive grazing enhances grasshopper fitness and abundance in a meadow steppe. Agric. Ecosyst. Environ. 300:107012. doi: 10.1016/j.agee.2020.107012
Zhu, H., Nkurunziza, V., Wang, J., Guo, Q., Ruan, H., and Wang, D. L. (2020b). Effects of large herbivore grazing on grasshopper behaviour and abundance in a meadow steppe. Ecol. Entomol. 619, 1357–66. doi: 10.1111/een.12919
Zhu, H., Wang, D. L., Guo, Q. F., Liu, J., and Wang, L. (2015). Interactive effects of large herbivores and plant diversity on insect abundance in a meadow steppe in China. Agric. Eco. Env. 212, 245–52. doi: 10.1016/j.agee.2015.07.008
Keywords: enclosure, livestock grazing, arid steppe, China, insect community biodiversity
Citation: Wang N, Song X, Wang J and Wang L (2022) Impacts of different fencing periods and grazing intensities on insect diversity in the desert steppe in Inner Mongolia. Front. Ecol. Evol. 10:1021677. doi: 10.3389/fevo.2022.1021677
Received: 17 August 2022; Accepted: 02 November 2022;
Published: 30 November 2022.
Edited by:
Isabel Marques, University of Lisbon, PortugalReviewed by:
Josip Skejo, University of Zagreb, CroatiaSamuel Adu-Acheampong, University of Ghana, Ghana
Copyright © 2022 Wang, Song, Wang and Wang. This is an open-access article distributed under the terms of the Creative Commons Attribution License (CC BY). The use, distribution or reproduction in other forums is permitted, provided the original author(s) and the copyright owner(s) are credited and that the original publication in this journal is cited, in accordance with accepted academic practice. No use, distribution or reproduction is permitted which does not comply with these terms.
*Correspondence: Liming Wang, wanglm1990@126.com
†These authors have contributed equally to this work and share first authorship