- College of Forestry, Gansu Agricultural University, Lanzhou, China
Soil carbon pools are important for maintaining the stability of the carbon cycle in terrestrial ecosystems and regulating climate change. However, it is not clear how soil carbon pools change under different vegetation restoration types at high altitudes during frequent seasonal freeze-thaws (FTs). Therefore, we studied the seasonal FT variability (before freezing, early stages of freezing, stable freeze stage, thawing stage) of soil organic carbon (SOC), microbial biomass carbon (MBC), dissolved organic carbon (DOC), and easily oxidized organic carbon (EOC) under three vegetation restoration types (Grassland, GL; Caragana korshinskii, CK; Xanthoceras sorbifolia, XS) on the Longzhong Loess Plateau region. We found that during the seasonal FT, the 0–40 cm SOC, MBC, DOC, and EOC contents were higher in XS vegetation than in GL and CK vegetation, but the sensitivity index of SOC was lower in XS vegetation (sensitivity index = 2.79 to 9.91). In the 0–40 cm soil layer, the seasonal FT process reduced the MBC content and increased the DOC content in the three vegetation soils. Meanwhile, DOC and EOC contents accumulated obviously in the stable freezing period and decreased significantly in the thawing period. We also found that the SOC, MBC, DOC, and EOC contents were higher in the surface soils (0–10 cm) than in the underlying soils (10–20 and 20–40 cm), while the sensitivity of the soil carbon pool fractions to seasonal FT processes differed considerably between soil depths. Redundancy analysis (RDA) showed that soil total nitrogen, temperature, total phosphorus, and soil water content were important environmental factors influencing soil carbon pool fractions during seasonal FT. This study suggested that in the Longzhong Loess Plateau region, soil MBC and DOC were more susceptible to seasonal FT phenomena and that the soil system of the Xanthoceras sorbifolia vegetation had a stronger soil C sequestration function during the seasonal FT process.
Introduction
The relationship between carbon cycling in terrestrial ecosystems and global climate change has become one of the topics of widespread interest in disciplines such as soil science and environmental ecology (Man et al., 2018; Luo et al., 2020). Soil carbon pools are often considered to be one of the most important carbon pools on Earth, as the carbon stored in soils far exceeds the total carbon in vegetation and the atmosphere (Carvalhais et al., 2014). Soil carbon pools are therefore important for maintaining the stability and ecological balance of terrestrial ecosystem carbon pools and regulating climate change (Malcolm et al., 2009; Man et al., 2018). Soil organic carbon (SOC) is an important source and sink of atmospheric carbon dioxide (CO2), and its content maintains the soil-atmosphere carbon balance and can evaluate soil health, carbon pool changes, and carbon cycle processes (Liu et al., 2020; Ma et al., 2020a). However, SOC is composed of a variety of reservoirs with varying degrees of sensitivity to the external environment, including labile and recalcitrant carbon pools (Guo et al., 2018; Wang et al., 2020a). Among these, soil labile carbon pools such as microbial biomass carbon (MBC), dissolved organic carbon (DOC), and easily oxidized organic carbon (EOC), which can directly participate in soil biochemical transformation processes (Wu et al., 2020). It can be used to reflect the characteristics of changes in soil quality and carbon pools caused by small changes in the external environment (Guo et al., 2018). Therefore, the study of soil labile carbon pools is of great significance to clarify the dynamics of soil carbon pools and to further understand the carbon cycling processes in terrestrial ecosystems.
Seasonal freeze-thaw (FT) is a physical and geological natural phenomenon in which soil water undergoes more frequent phase changes during seasonal transitions due to cyclical changes in ambient temperature (Chou and Wang, 2021). It is widespread at mid to high latitudes and altitudes around the world and is particularly pronounced at high altitudes in northeast and northwest China (Bo et al., 2021; Huang et al., 2021). As an abiotic stress in soils (Cheng and Wu, 2007), FT first occurs in the topsoil and gradually extends to the subsoil (Liang et al., 2021). This process has a potentially important impact on the carbon cycle of the soil (Guo et al., 2018; Huang et al., 2021). Vegetation plays an important role in the terrestrial carbon cycle (Malcolm et al., 2009; Wilkinson and Sherratt, 2016), but seasonal FT processes affect soil moisture distribution and directly influence vegetation growth activity (Bo et al., 2021). Studies have shown differences in the buffering effect of vegetation types on soil FT phenomena (Xiao et al., 2019; Bo et al., 2021). Among them, forest systems had a higher buffering adaptation capacity to soil temperature under FT than grassland (Bo et al., 2021), and forest SOC was more sensitive than grassland in response to FT (Xiao et al., 2019). Also, FT processes in the soil significantly increased anaerobic respiration in soils (Koponen et al., 2006), inhibited the mineralization of OC in soils (Bo and Brandt, 2003), and further increased soil DOC content and reduced soil MBC content (Li et al., 2019). Therefore, understanding the seasonal FT variation of soil carbon pools under different vegetation types is of great significance to improve the study of the regional carbon cycle.
The Longzhong Loess Plateau is located in the arid and semi-arid region of northern China and is an important part of the Loess Plateau, which is one of the most ecologically fragile areas in the world due to its high altitude and severe soil erosion phenomenon (Deng et al., 2018). However, in the context of global climate change, influenced by geographical location, soil texture, and climate, the region exhibits significant seasonal FT from late autumn to early spring each year (Bo et al., 2021). Numerous studies have revealed that the effects of FT cycling processes on soil properties in different areas of the Loess Plateau vary depending on the type of vegetation (Ye and Li, 2018; Wang et al., 2019; Bo et al., 2021; Liu et al., 2021a). Studies have shown that arboreal land soils freeze later than bare land (Bo et al., 2021) and that dam farmland was better able to retain soil moisture during FT, resulting in higher water migration and increment in the soil than slope farmland (Wang et al., 2019). Other studies have shown that FT cycling processes weaken soil shear strength (Liu et al., 2021a) and disrupt the internal soil microstructure (Ye and Li, 2018; Liu et al., 2021a), which in turn affects soil carbon stocks and carbon cycling processes in the region (Bo and Brandt, 2003; Li et al., 2019; Xiao et al., 2019). Over the past few decades, a project known as “the Conversion of Farmland to Forests and Grasses Program” has been put into place in this region to efficiently manage soil erosion and realize ecological restoration and its virtuous cycle (Yang et al., 2022a). During the revegetation process in this area, a large amount of sloping land was abandoned for natural recovery or converted to grassland and tree shrub forest (Xiao et al., 2019). It has been pointed out that vegetation restoration is an important measure to obtain ecological benefits, effectively increasing biodiversity (Deng et al., 2019; Yan et al., 2019), changing soil properties (Williams-Linera et al., 2021; Zhao et al., 2022), and significantly affecting the composition and function of regional ecosystems (Fu et al., 2020). However, the combination of external factors has produced a change in surface vegetation cover during the restoration process for different vegetation types (Feng et al., 2022). This change also represents, to some extent, the growth trend and condition of the vegetation (Feng et al., 2022). At the same time, vegetation can provide organic carbon to soil systems through exudation from surface plant litter and underground root secretions, thereby enhancing SOC stocks (Wang et al., 2020a). However, differences in the selection of restored tree species have led to differences in the recovery of its components (biodiversity, richness) during the long-term restoration of vegetation, resulting in large differences in surface vegetation composition, plant diversity, and community structure characteristics (Engel and Abella, 2011; Yang et al., 2017; Fu et al., 2020). This difference further results in differences in the amount and type of litter and roots produced by different vegetation restoration types (Feng et al., 2022; Sui et al., 2022), thus altering the SOC content and the transformation trends of the associated pools of organic carbon (Wang et al., 2020a). Studies have shown that forest systems with a complex composition of surface species have a higher capacity for soil water retention and insulation than grasslands (Bo et al., 2021), while their well-developed root systems relax soil compaction, alter soil physical properties (Buyer et al., 2016; Yang et al., 2022b) and provide rich plant and animal residues to the soil. In addition, the density and structure of the underground plant root system also affect the sensitivity of the soil to external hydrothermal conditions (Poulos et al., 2007; Singh et al., 2011), resulting in differences in soil ecosystem carbon cycling processes between vegetation types. However, little is known about how carbon pools in the soil of different vegetation restoration types change during seasonal FT in the context of global climate change in one of the most ecologically fragile regions in the world (the Longzhong Loess Plateau).
To fill the knowledge gap, this study investigated the characteristics of SOC, MBC, DOC, and EOC in the soil profiles of three typical vegetation restoration types (Grassland, GL; Caragana korshinskii, CK; Xanthoceras sorbifolium, XS) on the Longzhong Loess Plateau during seasonal freezing and thawing. Our specific research objectives were (a) to study the dynamic changes in SOC, MBC, DOC, and EOC in soil profiles of different vegetation restoration types during seasonal FT; (b) to identify the main environmental factors affecting the changes in soil carbon pools during seasonal FT. We hypothesized that: (1) there are differences in the patterns of change in SOC, MBC, DOC, and EOC during seasonal freezing and thawing between different vegetation restoration types due to differences in surface vegetation types and external environmental influences, and (2) there is a significant delay in the influence of the external environment on the carbon pools of the soil profile, making the response of the surface SOC, MBC, DOC, and EOC to seasonal freezing and thawing significantly different from that of the underlying soil.
Materials and methods
Study area
The study area (104°12′48″–105°01′06″ E, 35°17′54″–36°02′40″ N) is located in the experimental monitoring area of the Institute of Soil and Water Conservation Science, Dingxi City, Gansu Province, China. The area is part of the Anjiagou watershed, with a watershed area of 8.56 km2 and a watershed elevation between 2,100 and 2,250 m, which is typical of the high-altitude area of the Longzhong Loess Plateau (Wang et al., 2020a). The climatic characteristics of the basin are typical of a mid-temperate semi-arid climate (Yang et al., 2022a), with an average annual precipitation of 386.70 mm, mainly concentrated between July and September, accounting for about 52.7% of the total annual precipitation (Figure 1). The area has a large annual temperature difference (mean annual temperature of 6.3°C), with frequent natural phenomena of drought, frost, and seasonal soil freezing and thawing (Bo et al., 2021). Soil types in the basin are dominated by typical loess. The area was deforested and cleared before the 1990s, and in 1999, the Conversion of Farmland to Forests and Grasses Program was introduced, planting mainly trees, shrubs, and herbs in a 1:2:7 ratio (Wang et al., 2020a; Yang et al., 2022a). At present, the types of artificial vegetation restored in the area include herbaceous plants such as Gramineae, and Leguminosae, as well as silvicultural species such as Caragana korshinskii and Xanthoceras sorbifolium. Among them, Caragana korshinskii vegetation is a common afforestation species in the restoration of the Loess Plateau (Bo et al., 2021). Xanthoceras sorbifolia vegetation is a native species with high economic and ecological value in the watershed, with a planting area in China of more than 170,000 ha (Ma et al., 2020b; Wang et al., 2020a).
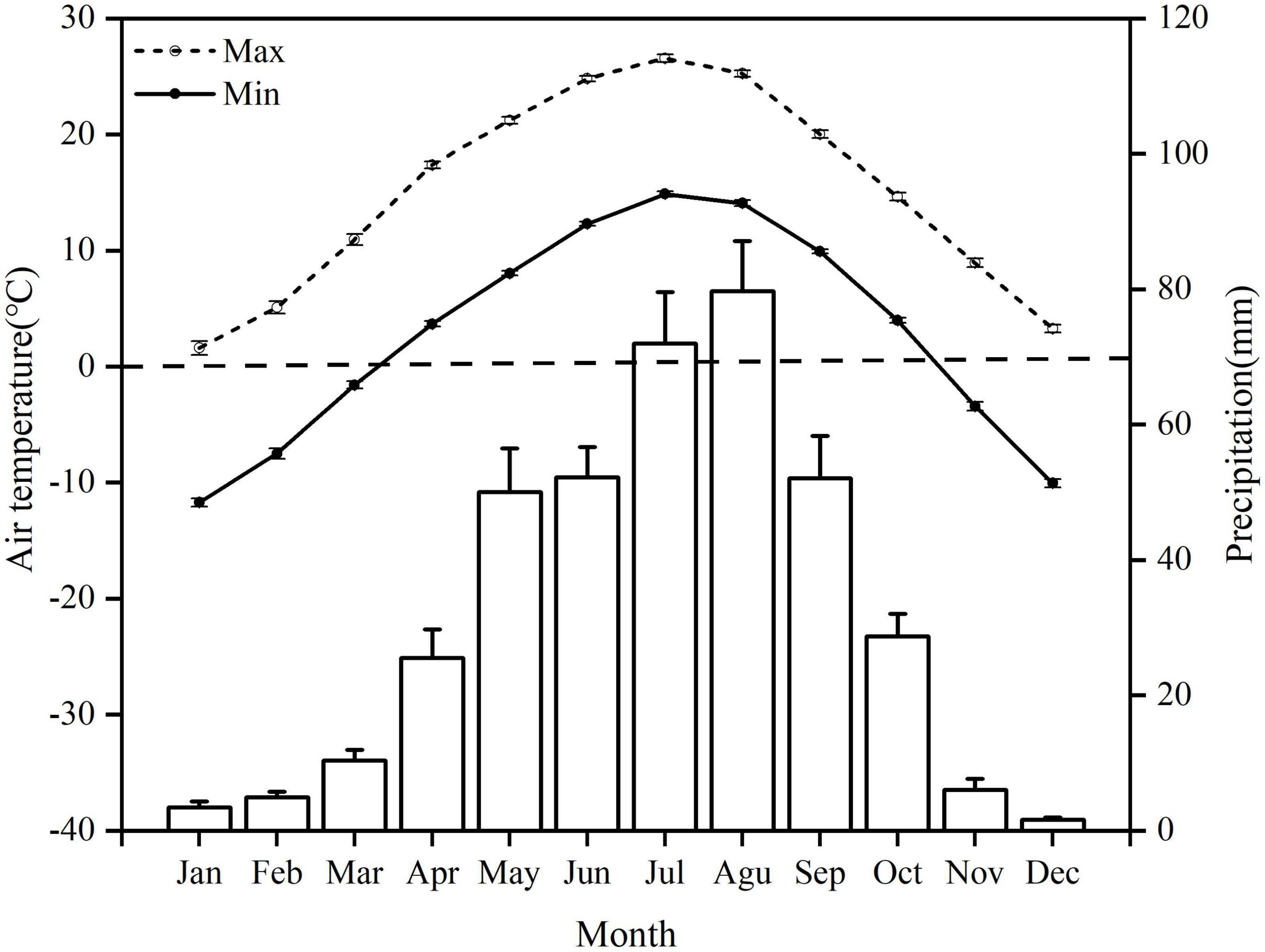
Figure 1. Mean monthly maximum and minimum air temperatures (lines) and monthly precipitation (bars) for 1998–2018 at the study site. The error bars indicate standard errors.
Experimental design
Through automatic measurements of meteorological data (1998–2018) for the experimental area by the meteorological observatory of the Institute of Soil and Water Conservation in Dingxi, Gansu Province, China (Figure 1), the air minimum temperature in the area was below 0°C from November to March each year. Therefore, at the end of October 2019, we selected three typical vegetation restoration experimental areas (Grassland, GL; Caragana korshinskii, CK; Xanthoceras sorbifolia, XS) with similar soil types and soil disturbance histories in the region (Table 1; Wang et al., 2020a; Yang et al., 2022a). A GS3 temperature and humidity sensor (METER Group, Pullman, WA, USA) was set up in each experimental area to automatically monitor and collect soil temperatures from 0 to 40 cm (0–10, 10–20, and 20–40 cm) every 30 min (Figure 2). A completely randomized design was used, with three randomly selected plots (8 × 8 m) within each experimental area, with a buffer zone of greater than 5 m between plots. We measured the physical properties of the soil at 0–40 cm in the sample plots in July 2019 (before the experiment) (Table 2).
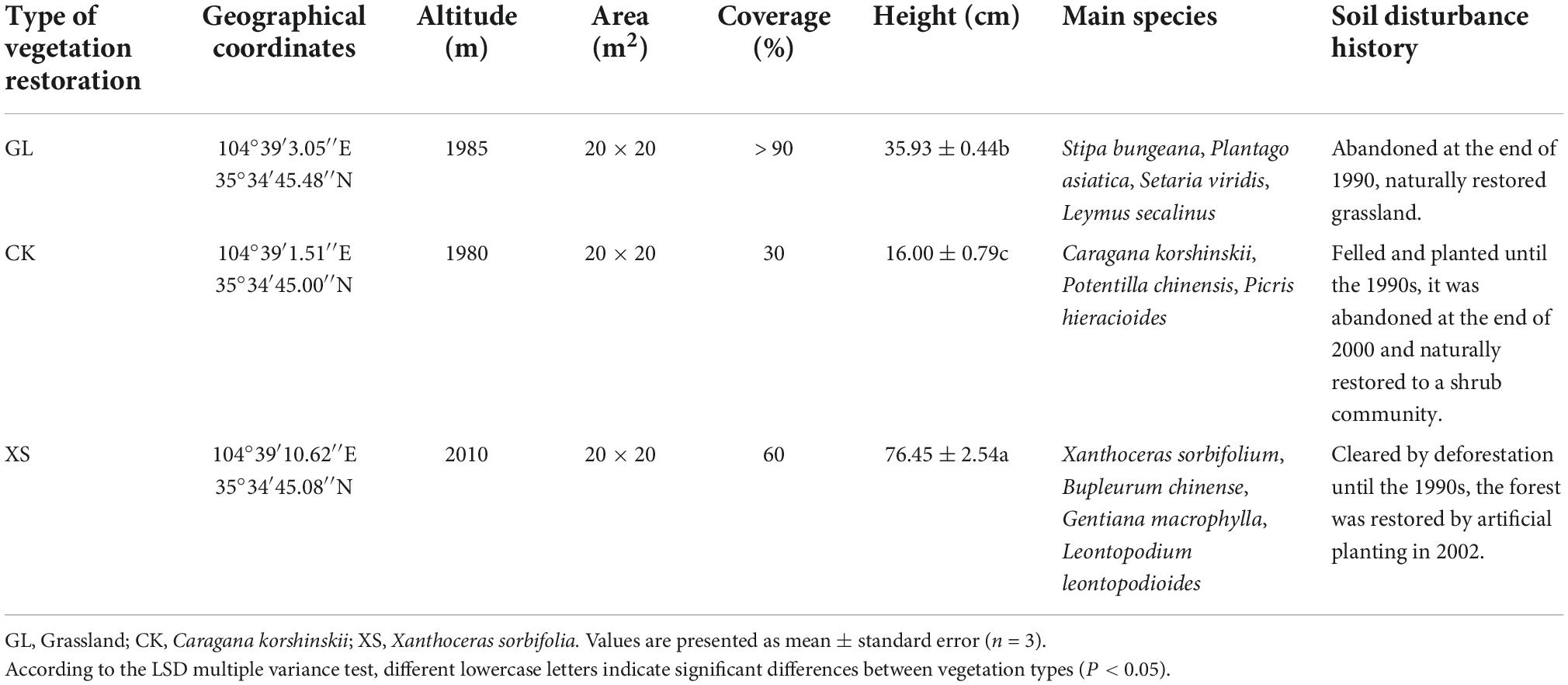
Table 1. Dominant species composition and land disturbance history of different vegetation restoration types.
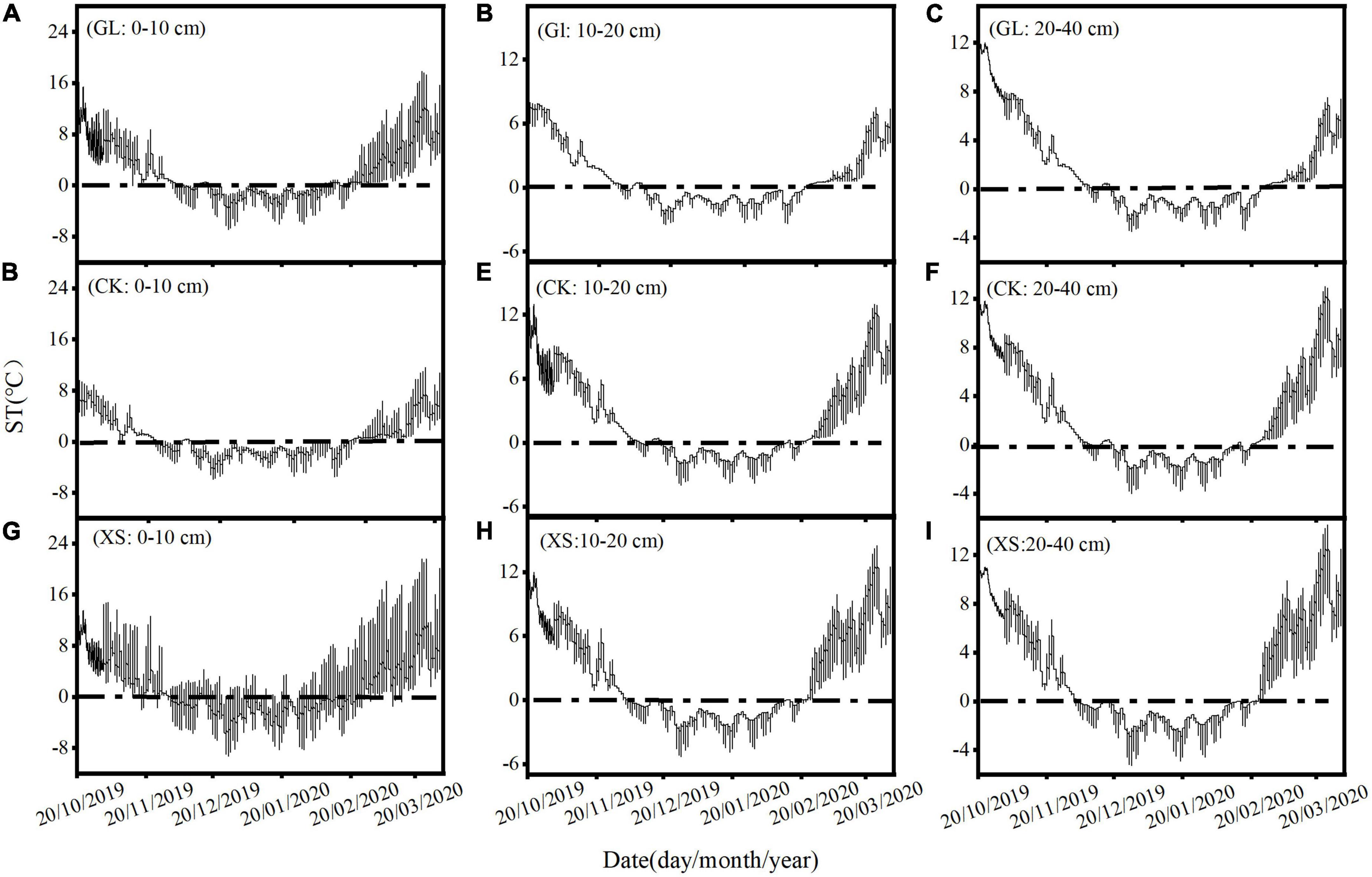
Figure 2. Soil temperature (ST) records for each soil layer from 0 to 40 cm below ground for GL [(A–C); Grassland], CK [(D–F); Caragana korshinskii], and XS [(G–I); Xanthoceras sorbifolia] experimental samples.
Soil sampling
We divided the FT periods and growing seasons based on the classification of FT periods and growing seasons by related scholars (Guo et al., 2010; Wei et al., 2011; Liu et al., 2013; Chen et al., 2020), and based on actual monitoring information of soil temperature at 0–40 cm in the experimental sample plots from 20 October 2019 to 31 March 2020 (Figure 2; Table 3). The sampling period covered the entire course of change at the sample site: the freezing phase: before freezing (BF, 27 October 2019), early stages of freezing (EF, 18 November 2019), stable freeze stage (SF, 12 January 2020); and the thawing phase: thawing stage (TS, 5 March 2020).
Soil freezing and thawing mainly affect changes in the moisture phase of the soil profile, and the 0–40 cm soil layer is often considered to be the active layer of moisture change (Yue et al., 2015). Also, factors such as temperature and light have a strong influence on the soil in the 0–40 cm depth range (Zhang et al., 2019a). Therefore, we sampled the 0–40 cm soil layer in layers (0–10, 10–20, and 20–40 cm) at each stage of seasonal FT through an SK-J petrol-powered soil sampler (50 mm diameter), selected at five sample points in each small sample plot according to the S-sampling method. After removing the debris from the soil samples, the same layer of soil from the same vegetation was mixed into one mixed soil sample and labeled to obtain a total of 36 (3 × 3 × 4) soil samples, which were taken back to the laboratory and stored in a refrigerator at 4°C. While collecting soil samples, soil temperature (ST) data were collected from 0 to 40 cm using a portable digital thermometer (JM624, Jinming Instrument Co., Tianjin, China) for monitoring.
Soil analysis
In July 2019 (before the experiment), we determined soil pH (soil-water ratio = 1:2.5) (Mao et al., 2016), soil bulk density, field capacity, and capillary porosity (Zhang et al., 2019b), respectively, according to the methods of previous studies. Moreover, at each seasonal FT stage, soil water content (SWC) was determined using the fresh soil drying method (Zhang et al., 2019b); soil total nitrogen (TN) was determined by the Kjeldahl method, and soil total phosphorus (TP) was determined by the molybdenum colorimetric method (Zhang et al., 2019b). We determined the sucrase and amylase activities by the 3, 5-dinitrosalicylic acid colorimetric method using sucrose solution and soluble starch as substrates, respectively (Wang et al., 2020a). SOC was determined by external heating with potassium dichromate (Zhang et al., 2019b). MBC was determined by chloroform fumigation extraction (Nie et al., 2016). DOC was determined by potassium sulfate extraction with external heating with potassium dichromate (Ma et al., 2020a). EOC was determined by oxidation with 333 mmol⋅L–1 KMnO4 solutions (Xu et al., 2021).
Sensitivity index value calculation
Using the content of SOC, MBC, DOC, and EOC for each vegetation restoration type at the BF stage as a reference, the sensitivity index (SI) for each indicator was calculated for each soil layer (0–10, 10–20, and 20–40 cm) during seasonal FT using the following equation (Dou et al., 2008; Priyanka and Anshumali, 2018):
where C is the content of SOC, MBC, DOC, and EOC during seasonal FT; CCK is the reference (BE stage) SOC, MBC, DOC, and EOC content.
Statistical analysis
Differences in soil physicochemical properties, soil carbon pools (i.e., SOC, MBC, DOC, and EOC), and SI values between vegetation restoration types, soil depths, and seasonal FT processes were analyzed by the LSD multiple variance test in SPSS 25.0 (n = 3; significance level of 95%, P < 0.05). The effects of the interaction of vegetation type, seasonal FT, and soil depth on SOC, MBC, DOC, and EOC were analyzed using the Two-Way ANOVA. The relationships between soil-related environmental factors (i.e., SWC, ST, TN, TP, C/N, C/P, sucrase, and amylase), and SOC, MBC, DOC, and EOC were also explored by redundancy analysis (RDA) and Pearson correlation analysis using Canoco 5.0 and Origin2022 software.
Results
Seasonal freeze-thaw dynamics of soil environmental factors under different vegetation restoration types
The seasonal FT dynamics of the 0–40 cm soil environmental factors (SWC, TN, TP, Sucrase, and Amylase) varied significantly under different vegetation restoration types (Table 4). Compared to the BF phase, the three vegetation species significantly reduced the TP content and increased the Sucrase content in the soil during seasonal FT (P < 0.05). As the seasonal FT process progressed, the soil TN and Amylase content reached a maximum in the SF stage, significantly higher than 50.45 and 27.27% in the BF stage, respectively (P < 0.05). Moreover, there was a significant increase in the soil SWC of the three vegetation types in the EF and TS stages, and a significant increase of 25.67 to 203.16% in the EF stage compared to the BF stage (P < 0.05).
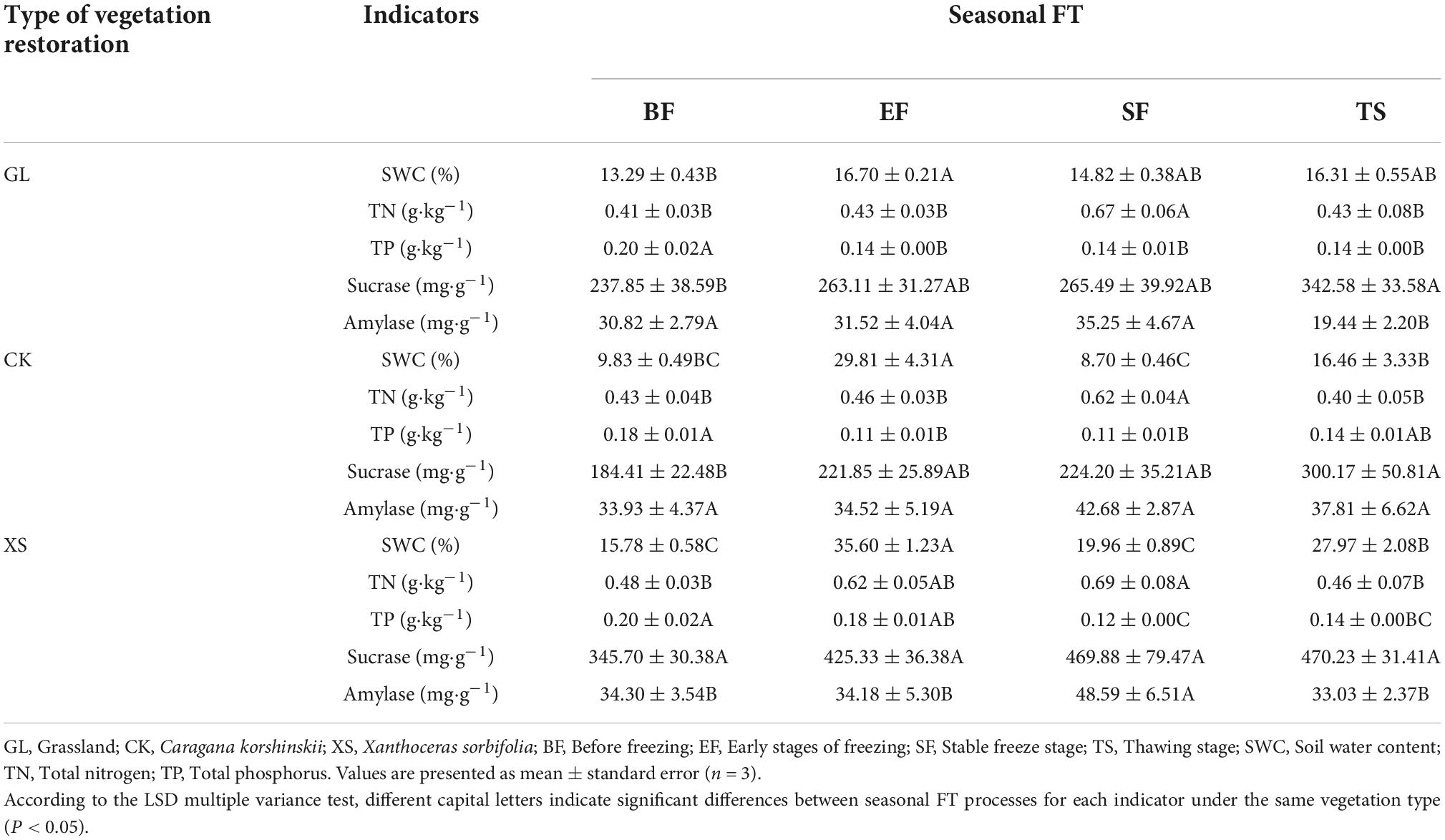
Table 4. Characteristics of changes in soil environmental factors in 0–40 cm under different vegetation restoration types during seasonal freeze-thaw (FT).
Seasonal freeze-thaw dynamics of soil carbon pools under different vegetation restoration types
The content of SOC, MBC, DOC, and EOC varied significantly (P < 0.05) during seasonal FT dynamics under different vegetation restoration types (Figure 3). In the 0–40 cm soil layer, the SOC, MBC, DOC, and EOC content of XS vegetation was overall higher than that of GL and CK vegetation during seasonal FT, and the dynamics of its SOC content were more stable (Figures 3A–D). Compared to the BF phase, the seasonal FT process significantly reduced the MBC content of the soil (P < 0.05) (Figure 3B), and increased the DOC content (Figure 3C). As the seasonal FT process progressed, the soil EOC content showed a trend of increasing and then decreasing (Figure 3D) and reached its highest value (mean = 3.80 g⋅kg–1) during the SF phase. A two-way ANOVA analysis showed significant interactions between seasonal FT processes and vegetation restoration type on SOC, MBC, DOC, and EOC in all the samples measured (Figure 3).
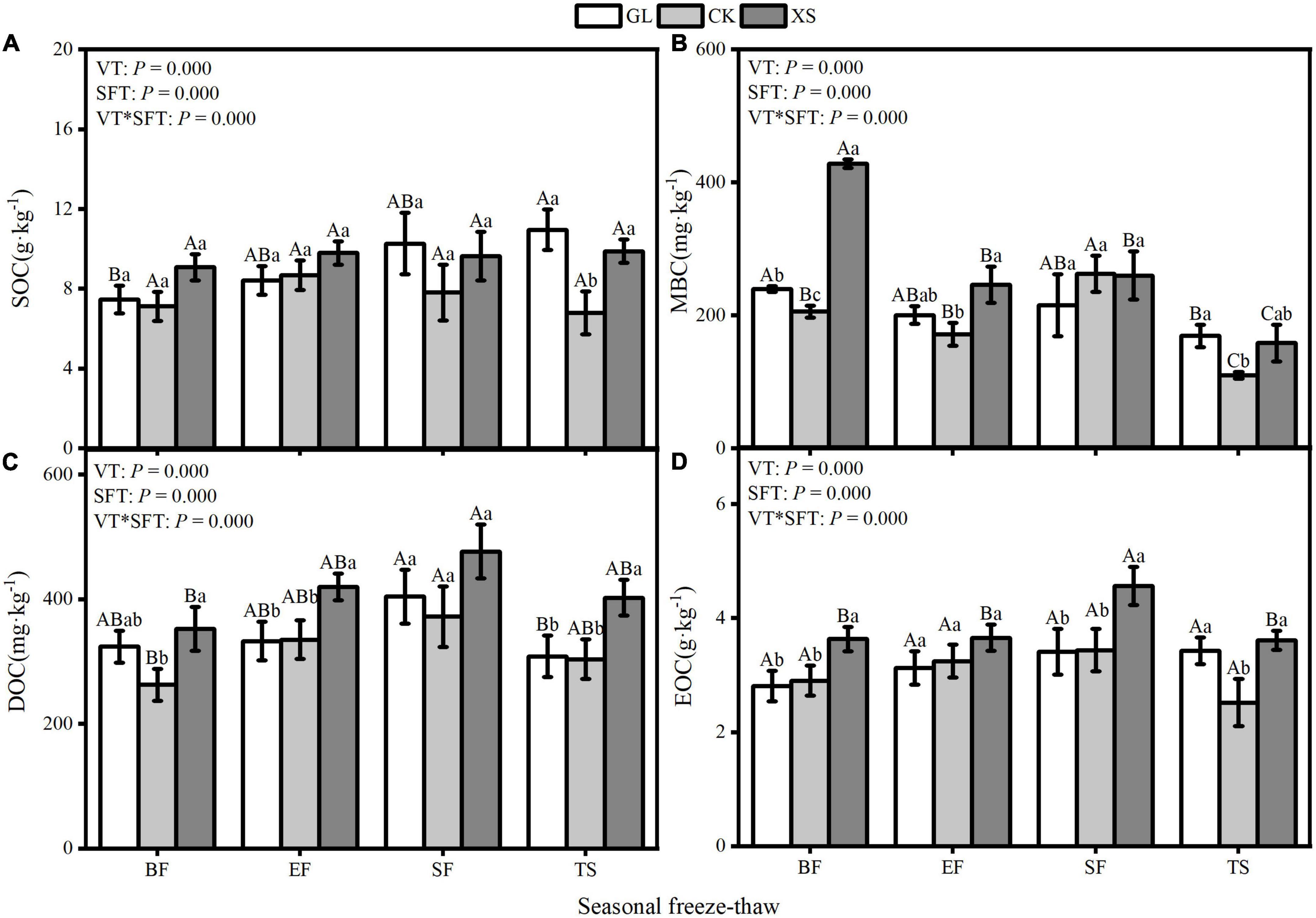
Figure 3. Seasonal freeze-thaw (FT) dynamics of SOC [(A); Soil organic carbon], MBC [(B); Microbial biomass carbon], DOC [(C); Dissolved organic carbon], and EOC [(D); Easily oxidized organic carbon] contents in 0–40 cm soil layers under different vegetation restoration types. GL, Grassland; CK, Caragana korshinskii; XS, Xanthoceras sorbifolia; BF, Before freezing; EF, Early stages of freezing; SF, Stable freeze stage; TS, Thawing stage. VT, SFT denote Type of vegetation restoration, Seasonal FT processes. Error bars indicate standard error of the mean (n = 3). According to the LSD multiple variance test, different capital letters indicate significant differences between seasonal FT processes for each indicator under the same vegetation type; different lowercase letters indicate significant differences between vegetation types under the same seasonal FT process (P < 0.05).
The SOC, MBC, EOC, and DOC content gradually decreased with increasing soil depth under all three vegetation types (Figure 4). Moreover, there were large spatial differences in soil profile variation in the content of SOC, MBC, DOC, and EOC during seasonal FT (Figure 4). In the 0–10 cm soil layer, the SOC, DOC, and EOC contents under all three vegetation types showed a rising and then falling trend during seasonal FT and reached a maximum during the SF phase [SOC (mean = 14.26 g⋅kg–1), DOC (mean = 575.31 mg⋅kg–1), EOC (mean = 5.13 g⋅kg–1)] (Figure 4). However, in the 10–20 cm and 20–40 cm soil layers, the pattern of change in SOC content during seasonal freezing and thawing was significantly different from that of the surface layer (0–10 cm) (Figure 4A). Also in the 20–40 cm soil layer, the patterns of change in soil MBC and DOC content during seasonal FT differed significantly from those in the 0–20 cm soil layer (Figures 4B,C). A two-way ANOVA analysis showed significant interactions between seasonal FT processes and soil depth on SOC, MBC, DOC, and EOC in all the samples measured (Figure 4).
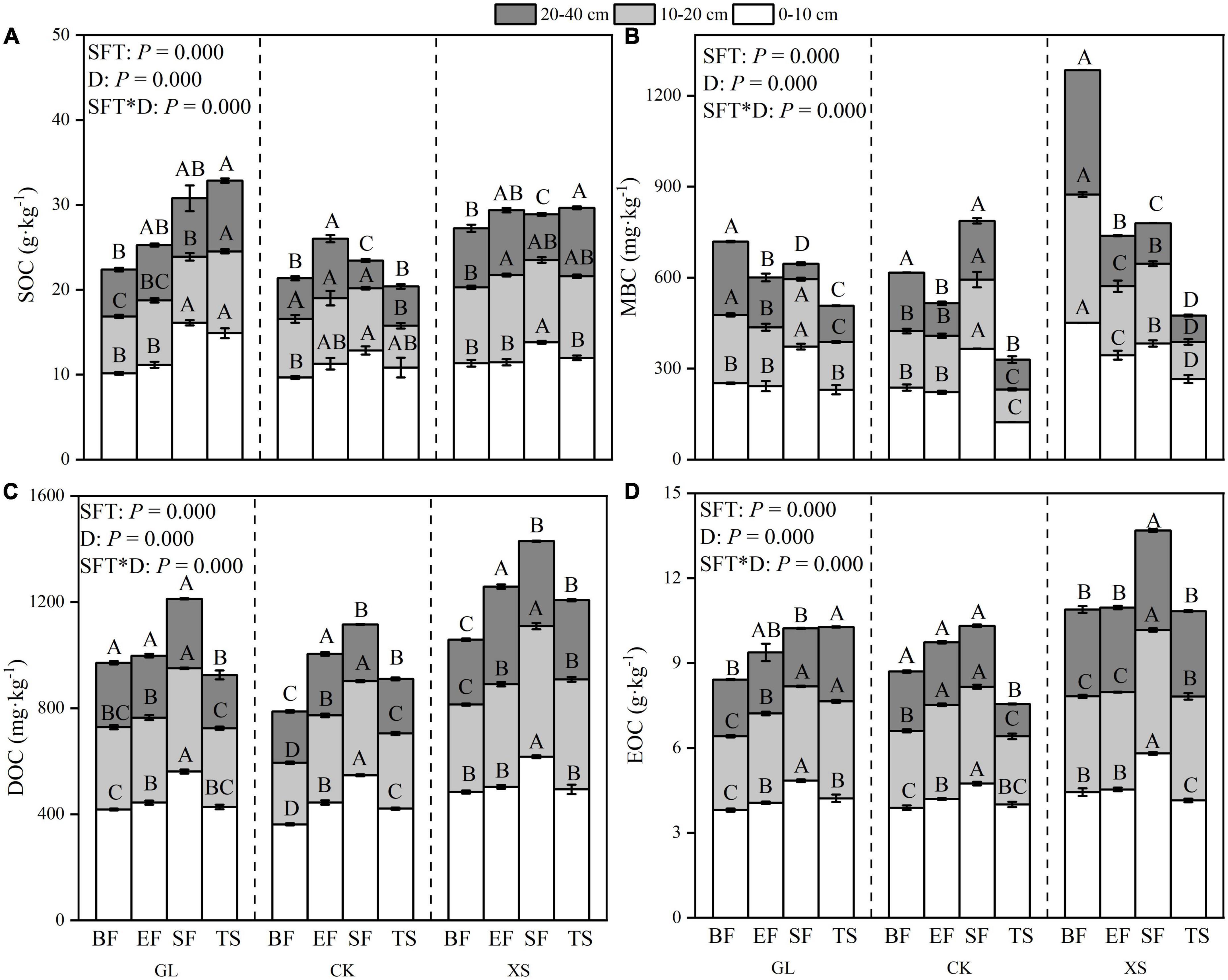
Figure 4. Seasonal freeze-thaw (FT) dynamics of SOC [(A), Soil organic carbon], MBC [(B), Microbial biomass carbon], DOC [(C), Dissolved organic carbon], and EOC [(D), Easily oxidized organic carbon] contents in each soil layer from 0 to 40 cm under different vegetation restoration types. GL, Grassland; CK, Caragana korshinskii; XS, Xanthoceras sorbifolia; BF, Before freezing; EF, Early stages of freezing; SF, Stable freeze stage; TS, Thawing stage. SFT, D denote Type of Seasonal FT processes, Depth of soil layer, respectively. Error bars indicate standard error of the mean (n = 3). According to the LSD multiple variance test, different capital letters indicate significant differences in the seasonal FT process for each indicator under the same vegetation type and soil layer (P < 0.05).
Sensitivity index of soil carbon pools during seasonal FT under different vegetation restoration types
The sensitivity of SOC, MBC, DOC, and EOC to seasonal FT processes varied considerably under different vegetation restoration types (Table 5). Using BF stage soils as a reference, the mean values of SOC, MBC, DOC, and EOC in 0–40 cm soils were ranked in order of absolute SI values: MBC (26.81) > DOC (19.54) > SOC (15.34) > EOC (10.22). The SI values of SOC in 0–40 cm soils of XS vegetation were not significantly different in seasonal FT processes (EF, SF, and TS) (P > 0.05), and its mean value (7.24) was lower than that of GL and CK vegetation. In addition, the sensitivity of SOC, MBC, DOC, and EOC to seasonal FT processes differed significantly between soil depths. Among them, the SI values of soil MBC and DOC under GL vegetation decreased significantly with increasing soil depth during the seasonal FT process. The SI values of DOC and EOC under CK vegetation were the lowest in the 20–40 cm soil layer during the seasonal FT process. At the same time, the SI values of MBC, DOC, and EOC in soils of 0–10 cm and 10–20 cm under all three vegetation restoration types were significantly higher in the SF stage than in the EF and TS stages (P < 0.05).
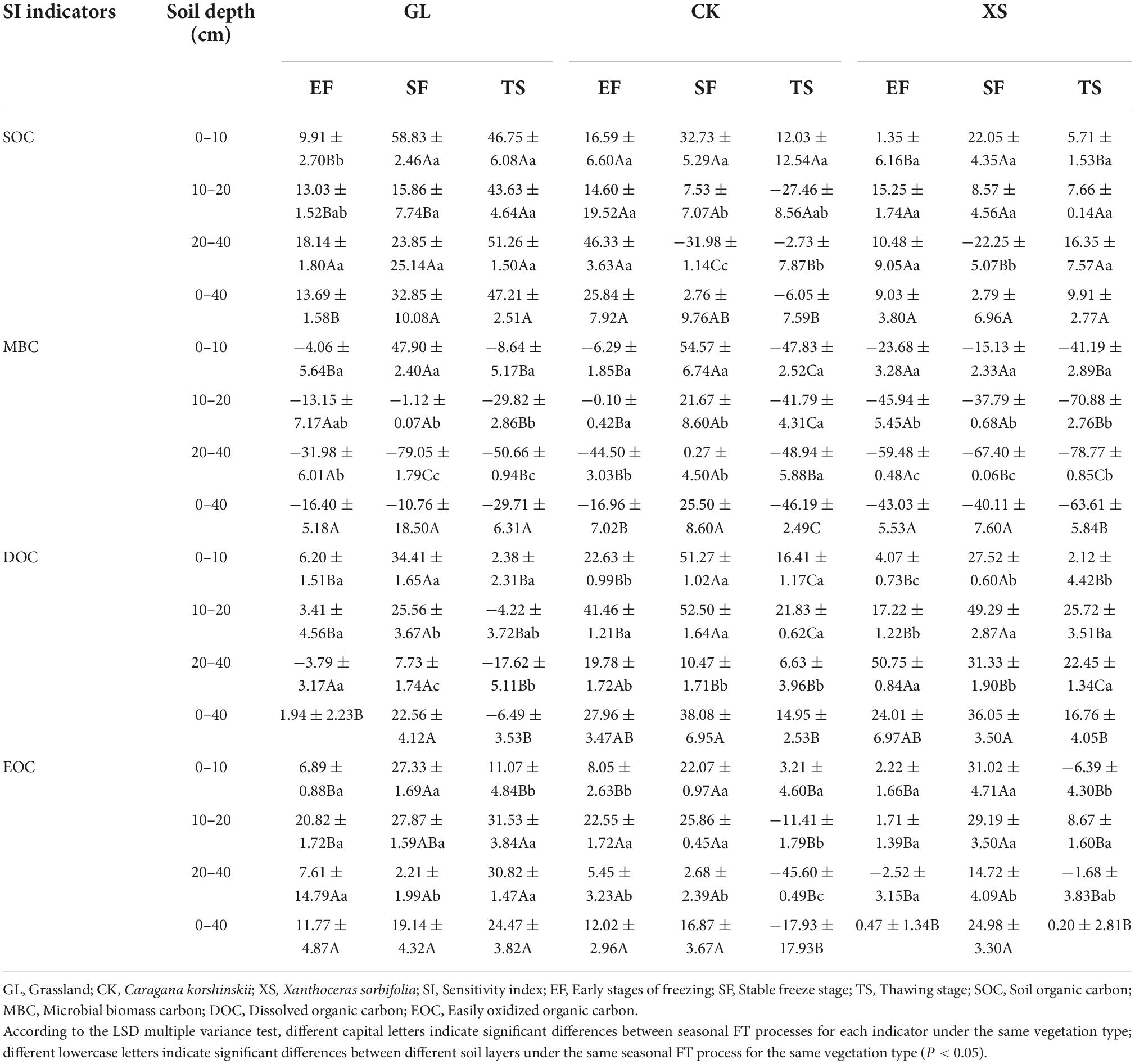
Table 5. Sensitivity index (SI) values of SOC, MBC, DOC, and EOC during seasonal FT under different vegetation restoration types.
Relationships between soil carbon pools and soil environmental factors
The results of the RDA test (Table 6) indicated that the RDA biaxial explained a total of 54.03% of the variation in soil carbon pools (SOC, MBC, DOC, and EOC) content during seasonal FT. Among the environmental factors affecting soil carbon pools, TN, ST, TP, and SWC had a better explanation for the variability in soil carbon pools (Table 6). Among them, TN explained the highest degree, up to 33.1% (P = 0.002) (Table 6), and all showed highly significant (P < 0.001) positive correlations between TN and carbon pools, with correlation coefficients of R = 0.74, 0.58, 0.85, and 0.81, respectively (Figure 5). Secondly, ST explained 15.4% (P = 0.002) of the carbon pools (Table 6) and there were highly significant negative correlations between ST and SOC, DOC, and EOC with correlation coefficients R = −0.32, −0.51, and −0.44 (Figure 5). Moreover, TP and SWC explained 2.4 and 1.9% of the soil carbon pools, respectively (P < 0.05) (Table 6), and there were different levels of significant positive correlations between TP and SWC and the soil carbon pools (Figure 5). Also, sucrase, amylase, and carbon pools all showed highly significant (P < 0.001) positive correlations with each other (Figure 5).
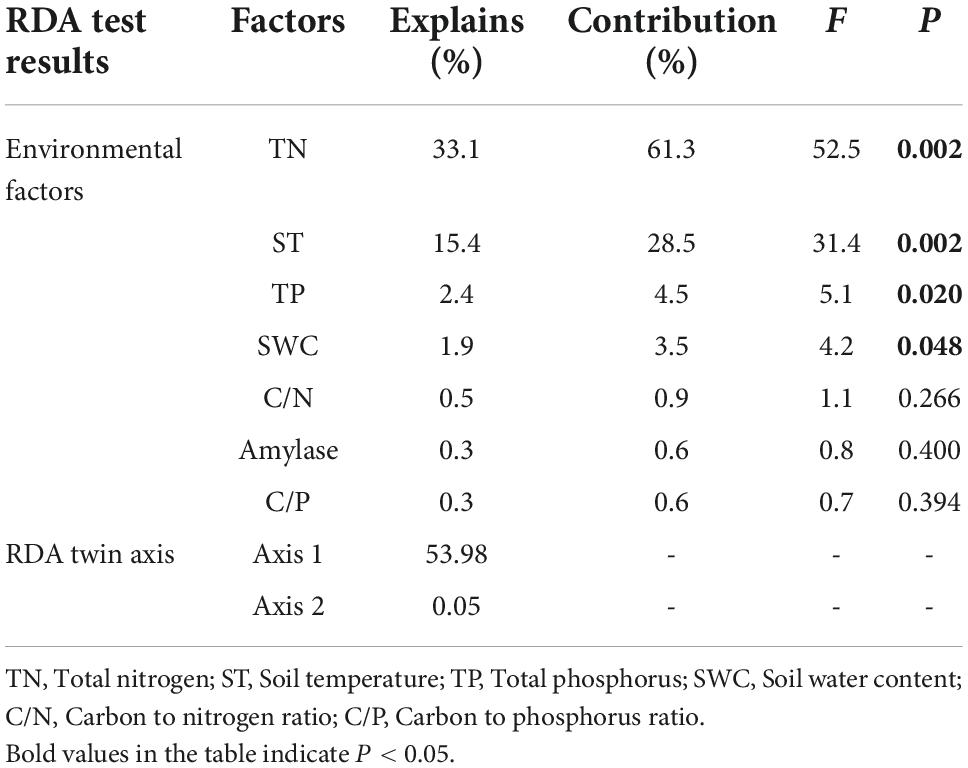
Table 6. Results of redundancy analysis (RDA) tests between soil carbon pools and environmental factors.
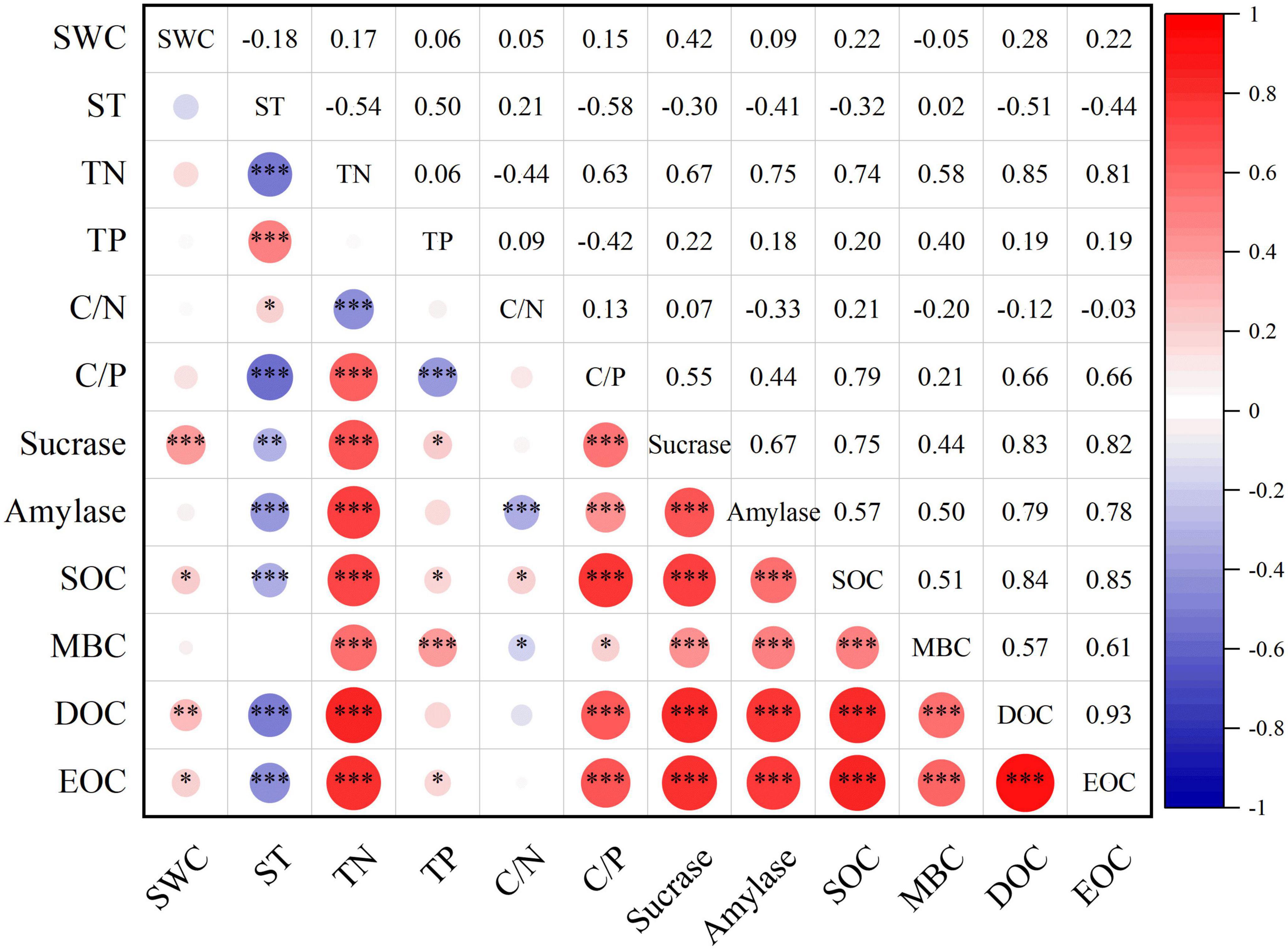
Figure 5. Heat map of correlation between soil carbon pools and environmental factors. *, **, and *** are significantly correlated at P < 0.05, P < 0.01, and P < 0.001 levels (bilateral), respectively. SWC, Soil water content; ST, Soil temperature; TN, Total nitrogen; TP, Total phosphorus; C/N, Carbon to nitrogen ratio; C/P, Carbon to phosphorus ratio; SOC, Soil organic carbon; MBC, Microbial biomass carbon; DOC, Dissolved organic carbon; EOC, Easily oxidized organic carbon.
Discussion
Differences in soil carbon pools between vegetation restoration types during seasonal freeze-thaw
Vegetation is an indispensable part of terrestrial ecosystems, and the frequent occurrence of seasonal freezing and thawing during vegetation restoration at high altitudes will greatly disturb regional terrestrial ecosystem carbon cycling processes (Xiao et al., 2019). This study demonstrated that the SOC, MBC, DOC, and EOC content of XS vegetation was higher than that of CK and GL vegetation during seasonal FT, which is consistent with the finding of Wang et al. (2020a). This is mainly because vegetation transports SOC and various organic carbon fractions to the soil through soil surface plant and animal residues, microbial content, and plant root exudates (Wang et al., 2020a). Studies have shown that during long-term vegetation restoration, differences in the selection of restored tree species have led to differences in the recovery of their above-ground components (biodiversity, richness) (Engel and Abella, 2011), resulting in large differences in surface plant community structure (Fu et al., 2020; Wang et al., 2020b). However, vegetation types with a more complex surface composition can provide a higher litter, cover area, and thickness, which are conducive to the accumulation of labile carbon pools in the soil (Wang et al., 2021). Moreover, the high species richness and vegetation cover of XS (Table 1), together with a large number of herbaceous plants, provide the soil with a more complex plant root system, a rich variety of litter, and plant and animal residues. This creates a more stable environment for the decomposition and release of soil organic matter (Sun et al., 2021), which in turn allows for increased input of soil carbon. Compared to XS vegetation, CK vegetation had a lower surface vegetation cover during revegetation (Table 1), making the litter sparse and the inter-root biomass low, hence the SOC pools of CK vegetation was relatively low. In addition, there was a highly significant positive correlation between organic carbon pools content and TN (Figure 5), and TN was one of the main environmental factors influencing changes in organic carbon pools (Table 6). Also, during seasonal FT, the TN content of XS vegetation was overall higher than that of GL and CK vegetation (Table 4), further confirming this reason.
This study demonstrated that SOC content varied significantly during seasonal FT, which is similar to the results of previous studies on SOC dynamics under plant growing and non-growing seasons (Boerner et al., 2005; Guo et al., 2018; Wu et al., 2021; Yang et al., 2022b). This may be due to the slow growth rate of vegetation before freezing, which requires fewer nutrients and provides the soil with abundant litter and root residues, facilitating the gradual accumulation of SOC (Wang et al., 2021). The freeze-thaw cycle breaks down the bonds between soil organic matter, fragmenting large agglomerates into smaller ones and releasing SOC encapsulated in soil aggregates (Herrmann and Witter, 2002; Larsen et al., 2002). Studies have shown that the microbial metabolic community in soils during the freeze-thaw cycle is dominated by fungi (Mannisto et al., 2009). Compared to bacterial communities, fungi have a higher carbon storage capacity, which makes the soil conducive to SOC storage during freeze-thaw (Mannisto et al., 2009). The freeze-thaw cycle destroys microbial cells, increases the decomposition mortality of fine roots, and releases small molecules of sugars and amino acids, increasing the content of organic matter in the soil, which in turn increases the SOC content of the soil (Fu et al., 2019). In addition, changes in soil moisture patterns during freeze-thaw leave the soil in a state of anoxia, which will inhibit the activity of hydrolytic enzymes in the soil, thus limiting the degradation of organic matter in the soil (Schindlbacher et al., 2011). Anoxic conditions will in turn limit the activity of aerobic microorganisms in the soil, reducing the production of CO2 in the soil and thus limiting the carbon export from the soil to the atmosphere (Koponen et al., 2006). At the same time, different vegetation restoration types will affect the sensitivity of their soils to external conditions such as water and temperature (Poulos et al., 2007), resulting in differences in the response of soil carbon pools to seasonal FT processes under different vegetation types. We found that the response of SOC to seasonal FT processes varied considerably between vegetation restorations types, with the SOC content of XS vegetation changing more consistently during seasonal FT, which is consistent with the finding of Bo et al. (2021). This is mainly due to the large differences in the buffering capacity of different surface vegetation types to seasonal FT events in the soil (Bo et al., 2021). Also, XS vegetation was taller, its surface vegetation species richness and the cover were higher, and its ability to hold and fix the water in the soil is greater (Figure 2, Tables 1, 4; Bo et al., 2021). However, an inconsistent correlation was shown between soil water temperature and SOC (Figure 5), allowing the XS vegetation SOC content to maintain a relatively stable equilibrium relationship during seasonal FT at higher soil moisture and temperature. Moreover, there was no significant difference (P > 0.05) in the SOC content and sensitivity index of the 0–40 cm layer under the XS vegetation restoration type during seasonal freeze-thaw (Figure 3, Table 5). The lower SI values (mean = 7.24) for XS vegetation SOC further illustrate the low response of XS vegetation SOC content to seasonal FT processes (Table 5).
We also found that the seasonal FT process reduced the MBC content and increased the DOC content in different vegetation soils compared to the BF and that there was a more pronounced accumulation of soil DOC and EOC during the SF and a significant decrease during the TS, which is consistent with the finding of Li et al. (2019). This is mainly because soil microbes maintain a relatively rich substrate content before freezing (Sorensen et al., 2018), and then successively experience freezing mortality and thawing period reproduction as the seasonal FT process progresses. However, microbial reproduction was limited by low-temperature activity and substrate constraints of pre-existing soil nutrients, which ultimately resulted in lower soil MBC content at all stages of seasonal FT than BF (Figure 3B). Moreover, soil DOC had a high sensitivity during seasonal FT processes (Table 5). Meanwhile, the changes in DOC content were mainly influenced by the relative combination of decomposition by dead soil microorganisms, the new release from the soil, and the amount of dissolved organic carbon that was broken down by mineralization (Herrmann and Witter, 2002). Seasonal FT disrupts the physical structure of the soil, causing hydrogen bonds in the organic matter with a large molecular weight bound to the soil to break, releasing organic matter with a small molecular weight (Larsen et al., 2002). FT processes also reduce the stability of soil aggregates, allowing the release of small molecules encapsulated by soil sorption (Herrmann and Witter, 2002), thus increasing the DOC content of the soil. Furthermore, under the SF stage, the low-temperature causes the death of some soil fungi and microorganisms, resulting in the outflow of large amounts of intracellular material (Fu et al., 2019), which leads to the release of small molecules of sugars and amino acids, thus increasing the content of organic carbon components in the soil. Also, sufficient nutrients such as nitrogen and phosphorus (Table 4) and decaying microbial residues in the soil provide a more than adequate and stable carbon source and microenvironment for the remaining cold-tolerant microorganisms (Zhu et al., 2020). This allowed soil enzyme activity to remain active during the SF period, which in turn contributed to the increase in soil carbon pools (Figure 5). Therefore, there was a significant increase in soil DOC and EOC content under the SF stage (Figures 3C,D). However, prolonged soil freezing gradually reduces the number of nutrients released by the disruption of soil aggregates (Fu et al., 2019). At the same time, anaerobic respiration by residual microorganisms in the soil was enhanced (Koponen et al., 2006), accelerating the depletion of existing soil nutrients. As a result, soil nutrient content decreases during the SF period toward thawing (Table 4). At this time, the soil temperature rises (Figure 2), and the free water content begins to increase (Table 4). The roots of plants that had not yet started to grow were unable to take up and use soil water and nutrients in time. Excessive and sudden increases in free soil water (Table 4) tend to leach labile nutrients from the soil to deeper layers of the soil (Ranger et al., 2007), resulting in lower soil DOC and EOC contents during the TS.
Differences in soil carbon pools at different soil depths during seasonal freeze-thaw
It was found that the organic carbon pools varied significantly between soil layers under different vegetation types during seasonal freezing and thawing and that the soil carbon pools contents were higher in the surface layer than in the lower layer (Figure 4). This is because the depth, density, and structure of the soil root system vary greatly in the vertical soil profile under different vegetation restoration types (Singh et al., 2011; Ma et al., 2022), which in turn greatly affects the transformation of soil organic carbon. In particular, plant root biomass decreases with increasing soil depth (Singh et al., 2011), and the root structure of different vegetation types differs significantly in the soil profile, with roots highly concentrated in the upper soil layer (Grant et al., 2012; Li et al., 2022). Studies have shown that the complex root structure of plants allows for a lag in the effect of external temperature on the soil, with the lag time being determined by a combination of soil depth and root structure (Schwieger et al., 2017; Tabbagh et al., 2017). However, the hysteresis effect and the thermal insulation effect of the complex root system (Guo et al., 2008) make the water temperature conditions in the lower soil layer more different from those in the upper soil layer. It has also been noted that the effect of external air temperature on soil temperature decreases with increasing soil depth (Tabbagh et al., 2017). During seasonal freezing and thawing, the temperature is transferred from the surface downward as the surface soil freezes and melts and is gradually stabilized with increasing soil depth (Tabbagh et al., 2017). Moreover, SOC is highly susceptible to the influence of external factors such as moisture and temperature (Figure 4), resulting in significant differences in the spatial distribution of the characteristics of soil organic carbon pools under different vegetation during seasonal FT, which also confirms our second hypothesis. Furthermore, during seasonal FT, the topsoil is the first to be affected by the external environment, producing FT and physically disrupting structures such as soil aggregates, releasing large amounts of soil nutrients (Liang et al., 2021). On the other hand, surface vegetation transports nutrients to the soil mainly through surface plant roots and plant and animal residues (Wang et al., 2020a). Also, the surface soil is better aerated and has better soil microenvironmental conditions than the underlying soil, thus facilitating the decomposition and transformation of dead leaves and microbial reproduction in the surface layer (Pahlavan-Rad and Pessarakli, 2009). At the same time, the effect of decomposition of dead litter and humus on soil nutrient accumulation decreases with increasing soil depth (Liu et al., 2021b). Moreover, there was a significant positive correlation between soil physicochemical properties, enzyme activity, and soil carbon pools to varying degrees (Figure 5). Therefore, the higher TN and enzyme activity in the surface layer will further contribute to the content of soil carbon pools fraction in the surface layer, making the soil carbon pools fraction in the lower soil layer lower than in the surface layer.
Conclusion
This study showed that the soil carbon pools (i.e., SOC, MBC, DOC, and EOC) under the restored type of Xanthoceras sorbifolia vegetation were higher than that of Caragana korshinskii vegetation and Grassland during seasonal freeze-thaw. Also, the changes in SOC of Xanthoceras sorbifolia vegetation were more stable during the seasonal freeze-thaw. The seasonal freeze-thaw process reduced the soil MBC content and increased the DOC content, and there was a more obvious accumulation of DOC and EOC content during the stable freeze period. Moreover, the SOC, MBC, DOC, and EOC contents under all three vegetation restoration types showed obvious surface aggregation, and the response of the soil carbon pools contents to the seasonal freeze-thaw process differed significantly among the different soil depths. Our results demonstrate that the seasonal freeze-thaw will affect the decomposition and transformation of soil carbon in vegetation systems, which in turn affects the regional soil carbon cycling processes. At the same time, the carbon sequestration function of the soil system under the Xanthoceras sorbifolia vegetation restoration type was stronger during the seasonal freeze-thaw process in the Longzhong Loess Plateau.
Data availability statement
The original contributions presented in this study are included in the article/Supplementary material, further inquiries can be directed to the corresponding author.
Author contributions
GL designed, managed, and supervised the whole project. SL analyzed the experimental data and wrote the manuscript. JW and JY helped in preparing the manuscript and in interpretation of the analyses during constructive. CY and MX carried out field sample collection and experimental operation. All authors read and approved the manuscript.
Funding
This study was supported by the Gansu Provincial Youth Science and Technology Program, China (22JR5RA877), the Excellent doctoral students’ Project of Gansu Province (22JR5RA843), the Higher Education industry support project of Gansu Province (2022CYZC-41), the Higher Education industry support project of Gansu Province (2021CYZC-15), the Key Research and Development Plan of Gansu Province (20YF8NA135), and the Finance Special Project of Gansu Province (GSCZZ 20160909).
Acknowledgments
We would like to thank all the people who were involved in the field and laboratory work.
Conflict of interest
The authors declare that the research was conducted in the absence of any commercial or financial relationships that could be construed as a potential conflict of interest.
Publisher’s note
All claims expressed in this article are solely those of the authors and do not necessarily represent those of their affiliated organizations, or those of the publisher, the editors and the reviewers. Any product that may be evaluated in this article, or claim that may be made by its manufacturer, is not guaranteed or endorsed by the publisher.
Supplementary material
The Supplementary Material for this article can be found online at: https://www.frontiersin.org/articles/10.3389/fevo.2022.1019627/full#supplementary-material
References
Bo, E., and Brandt, K. K. (2003). Uncoupling of microbial CO2 production and release in frozen soil and its implications for field studies of arctic C cycling. Soil Biol. Biochem. 35, 263–272. doi: 10.1016/S0038-0717(02)00258-4
Bo, L., Li, Z., Li, P., Xu, G., Xiao, L., and Ma, B. (2021). Soil freeze-thaw and water transport characteristics under different vegetation types in seasonal freeze-thaw areas of the loess plateau. Front. Earth Sci. 9:704901. doi: 10.3389/feart.2021.704901
Boerner, R. E. J., Brinkman, J. A., and Smith, A. (2005). Seasonal variations in enzyme activity and organic carbon in soil of a burned and unburned hardwood forest. Soil Biol. Biochem. 37, 1419–1426. doi: 10.1016/j.soilbio.2004.12.012
Buyer, J. S., Schmidt-Küntzel, A., Nghikembua, M., Maul, J. E., and Marker, L. (2016). Soil microbial communities following bush removal in a Namibian savanna. Soil 2, 101–110. doi: 10.5194/soil-2-101-2016
Carvalhais, N., Forkel, M., Khomik, M., Bellarby, J., Jung, M., Migliavacca, M., et al. (2014). Global covariation of carbon turnover times with climate in terrestrial ecosystems. Nature 514, 213–217. doi: 10.1038/nature13731
Chen, S., Li, X., Wu, T., Xue, K., Luo, D., Wang, X., et al. (2020). Soil thermal regime alteration under experimental warming in permafrost regions of the central Tibetan Plateau. Geoderma 372:114397. doi: 10.1016/j.geoderma.2020.114397
Cheng, G., and Wu, T. (2007). Responses of permafrost to climate change and their environmental significance, Qinghai-Tibet Plateau. J. Geophys. Res. 112, doi: 10.1029/2006jf000631
Chou, Y.-L., and Wang, L.-J. (2021). Seasonal freezing-thawing process and hydrothermal characteristics of soil on the Loess Plateau, China. J. Mountain Sci. 18, 3082–3098. doi: 10.1007/s11629-020-6599-9
Deng, J., Yin, Y., Luo, J., Zhu, W., and Zhou, Y. (2019). Different revegetation types alter soil physical-chemical characteristics and fungal community in the Baishilazi Nature Reserve. PeerJ 6:e6251. doi: 10.7717/peerj.6251
Deng, L., Wang, K., Zhu, G., Liu, Y., Chen, L., and Shangguan, Z. (2018). Changes of soil carbon in five land use stages following 10 years of vegetation succession on the Loess Plateau, China. Catena 171, 185–192. doi: 10.1016/j.catena.2018.07.014
Dou, F., Wright, A. L., and Hons, F. M. (2008). Sensitivity of labile soil organic carbon to tillage in wheat-based cropping systems. Soil Sci. Soc. Am. J. 72, 1445–1453. doi: 10.2136/sssaj2007.0230
Engel, E. C., and Abella, S. R. (2011). Vegetation recovery in a desert landscape after wildfires: influences of community type, time since fire and contingency effects. J. Appl. Ecol. 48, 1401–1410. doi: 10.1111/j.1365-2664.2011.02057.x
Feng, Y., Wang, J., Zhou, Q., Bai, M., Peng, P., Zhao, D., et al. (2022). Quantitative analysis of vegetation restoration and potential driving factors in a typical subalpine region of the Eastern Tibet Plateau. PeerJ 10:e13358. doi: 10.7717/peerj.13358
Fu, D., Wu, X., Duan, C., Chadwick, D. R., and Jones, D. L. (2020). Response of soil phosphorus fractions and fluxes to different vegetation restoration types in a subtropical mountain ecosystem. Catena 193:104663. doi: 10.1016/j.catena.2020.104663
Fu, Q., Yan, J., Li, H., Li, T., Hou, R., Liu, D., et al. (2019). Effects of biochar amendment on nitrogen mineralization in black soil with different moisture contents under freeze-thaw cycles. Geoderma 353, 459–467. doi: 10.1016/j.geoderma.2019.07.027
Grant, J. C., Nichols, J. D., Yao, R. L., Smith, R. G. B., Brennan, P. D., and Vanclay, J. K. (2012). Depth distribution of roots of Eucalyptus dunnii and Corymbia citriodora subsp. variegata in different soil conditions. Forest Ecol. Manag. 269, 249–258. doi: 10.1016/j.foreco.2011.12.033
Guo, D., Yang, M., and Wang, H. (2010). Sensible and latent heat flux response to diurnal variation in soil surface temperature and moisture under different freeze/thaw soil conditions in the seasonal frozen soil region of the central Tibetan Plateau. Environ. Earth Sci. 63, 97–107. doi: 10.1007/s12665-010-0672-6
Guo, J., Wang, B., Wang, G., Wu, Y., and Cao, F. (2018). Vertical and seasonal variations of soil carbon pools in ginkgo agroforestry systems in eastern China. Catena 171, 450–459. doi: 10.1016/j.catena.2018.07.032
Guo, X., Lu, X., Tong, S., and Guohua, D. (2008). Influence of environment and substrate quality on the decomposition of wetland plant root in the Sanjiang Plain, Northeast China. J. Environ. Sci. 20, 1445–1452. doi: 10.1016/S1001-0742(08)62547-4
Herrmann, A., and Witter, E. (2002). Sources of C and N contributing to the flush in mineralization upon freeze-thaw cycles in soils. Soil Biol. Biochem. 34, 1495–1505.
Huang, D., Zhou, L., Fan, H., Jia, Y., and Liu, M. (2021). Responses of aggregates and associated soil available phosphorus, and soil organic matter in different slope aspects, to seasonal freeze–thaw cycles in Northeast China. Geoderma 402:115184. doi: 10.1016/j.geoderma.2021.115184
Koponen, H. T., Jaakkola, T., Keinänen-Toivola, M. M., Kaipainen, S., Tuomainen, J., Servomaa, K., et al. (2006). Microbial communities, biomass, and activities in soils as affected by freeze thaw cycles. Soil Biol. Biochem. 38, 1861–1871. doi: 10.1016/j.soilbio.2005.12.010
Larsen, K. S., Jonasson, S., and Michelsen, A. (2002). Repeated freeze-thaw cycles and their effects on biological processes in two arctic ecosystem types. Appl. Soil Ecol. 21, 187–195.
Li, F., Zang, S., Liu, Y., Li, L., and Ni, H. (2019). Effect of freezing–thawing cycle on soil active organic carbon fractions and enzyme activities in the wetland of sanjiang plain, Northeast China. Wetlands 40, 167–177. doi: 10.1007/s13157-019-01164-9
Li, J., Wang, X., Jia, H., Liu, Y., Zhao, Y., Shi, C., et al. (2022). Effect of herbaceous plant root density on slope stability in a shallow landslide-prone area. Nat. Hazards 112, 2337–2360. doi: 10.1007/s11069-022-05268-0
Liang, Y., Deng, X., Song, T., Chen, G., Wang, Y., Zhang, Q., et al. (2021). Influences of seasonal freezing and thawing on soil water-stable aggregates in orchard in high cold region, Northeast China. Chinese Geograph. Sci. 31, 234–247. doi: 10.1007/s11769-021-1187-7
Liu, J., Wu, F., Yang, W., Shi, P., Wang, A., Yang, Y., et al. (2013). Effect of seasonal freeze–thaw cycle on net nitrogen mineralization of soil organic layer in the subalpine/alpine forests of western Sichuan, China. Acta Ecol. Sinica 33, 32–37. doi: 10.1016/j.chnaes.2012.12.005
Liu, K., Ye, W., Jing, H., and Kaneko, K. (2021a). Shear strength and microstructure of intact loess subjected to freeze-thaw cycling. Adv. Materials Sci. Eng. 2021, 1–15. doi: 10.1155/2021/1173603
Liu, R., Zhang, Y., Hu, X.-F., Wan, S., Wang, H., Liang, C., et al. (2021b). Litter manipulation effects on microbial communities and enzymatic activities vary with soil depth in a subtropical Chinese fir plantation. Forest Ecol. Manag. 480:118641. doi: 10.1016/j.foreco.2020.118641
Liu, M., Li, P., Liu, M., Wang, J., and Chang, Q. (2020). The trend of soil organic carbon fractions related to the successions of different vegetation types on the tableland of the Loess Plateau of China. J. Soils Sediments 21, 203–214. doi: 10.1007/s11368-020-02710-3
Luo, Z., Viscarra Rossel, R. A., and Shi, Z. (2020). Distinct controls over the temporal dynamics of soil carbon fractions after land use change. Glob. Chang Biol. 26, 4614–4625. doi: 10.1111/gcb.15157
Ma, J., Li, Z., Sun, B., and Ma, B. (2022). Mechanism and modeling of different plant root effects on soil detachment rate. Catena 212:106109. doi: 10.1016/j.catena.2022.106109
Ma, W., Li, G., Wu, J., Xu, G., and Wu, J. (2020a). Response of soil labile organic carbon fractions and carbon-cycle enzyme activities to vegetation degradation in a wet meadow on the Qinghai–Tibet Plateau. Geoderma 377:114565.
Ma, Y., Bi, Q., Li, G., Liu, X., Fu, G., Zhao, Y., et al. (2020b). Provenance variations in kernel oil content, fatty acid profile and biodiesel properties of Xanthoceras sorbifolium Bunge in northern China. Industrial Crops Products 151:112487. doi: 10.1016/j.indcrop.2020.112487
Malcolm, G. M., López-Gutiérrez, J. C., and Koide, R. T. (2009). Temperature sensitivity of respiration differs among forest floor layers in a Pinus resinosa plantation. Soil Biol. Biochem. 41, 1075–1079. doi: 10.1016/j.soilbio.2009.02.011
Man, W., Mao, D., Wang, Z., Li, L., Liu, M., Jia, M., et al. (2018). Spatial and vertical variations in the soil organic carbon concentration and its controlling factors in boreal wetlands in the Greater Khingan Mountains, China. J. Soils Sediments 19, 1201–1214. doi: 10.1007/s11368-018-2126-9
Mannisto, M. K., Tiirola, M., and Haggblom, M. M. (2009). Effect of freeze-thaw cycles on bacterial communities of arctic tundra soil. Microb Ecol. 58, 621–631. doi: 10.1007/s00248-009-9516-x
Mao, J., Nierop, K. G. J., Rietkerk, M., Sinninghe Damste, J. S., and Dekker, S. C. (2016). The influence of vegetation on soil water repellency-markers and soil hydrophobicity. Sci. Total Environ. 566-567, 608–620. doi: 10.1016/j.scitotenv.2016.05.077
Nie, X. J., Zhang, J. H., Cheng, J. X., Gao, H., and Guan, Z. M. (2016). Effect of soil redistribution on various organic carbons in a water- and tillage-eroded soil. Soil Tillage Res. 155, 1–8. doi: 10.1016/j.still.2015.07.003
Pahlavan-Rad, M. R., and Pessarakli, M. (2009). Response of Wheat Plants to Zinc, Iron, and manganese applications and uptake and concentration of Zinc, Iron, and Manganese in Wheat Grains. Commun. Soil Sci. Plant Anal. 40, 1322–1332. doi: 10.1080/00103620902761262
Poulos, H. M., Taylor, A. H., and Beaty, R. M. (2007). Environmental controls on dominance and diversity of woody plant species in a Madrean, Sky Island ecosystem, Arizona, USA. Plant Ecol. 193, 15–30. doi: 10.1007/s11258-006-9245-x
Priyanka, K., and Anshumali. (2018). Quantifying total and labile pools of soil organic carbon in cultivated and uncultivated soils in eastern India. Soil Res. 56, 413–420. doi: 10.1071/sr17188
Ranger, J., Loyer, S., Gelhaye, D., Pollier, B., and Bonnaud, P. (2007). Effects of the clear-cutting of a Douglas-fir plantation (Pseudotsuga menziesii F.) on the chemical composition of soil solutions and on the leaching of DOC and ions in drainage waters. Ann. Forest Sci. 64, 183–200. doi: 10.1051/forest:2006103
Schindlbacher, A., Rodler, A., Kuffner, M., Kitzler, B., Sessitsch, A., and Zechmeister-Boltenstern, S. (2011). Experimental warming effects on the microbial community of a temperate mountain forest soil. Soil Biol. Biochem. 43, 1417–1425. doi: 10.1016/j.soilbio.2011.03.005
Schwieger, S., Kreyling, J., Milbau, A., and Blume-Werry, G. (2017). Autumnal warming does not change root phenology in two contrasting vegetation types of subarctic tundra. Plant Soil 424, 145–156. doi: 10.1007/s11104-017-3343-5
Singh, B., Tripathi, K. P., and Singh, K. (2011). Community structure, diversity, biomass and net production in a rehabilitated subtropical forest in North India. Open J. Forestry 01, 11–26. doi: 10.4236/ojf.2011.12003
Sorensen, P. O., Finzi, A. C., Giasson, M.-A., Reinmann, A. B., Sanders-DeMott, R., and Templer, P. H. (2018). Winter soil freeze-thaw cycles lead to reductions in soil microbial biomass and activity not compensated for by soil warming. Soil Biol. Biochem. 116, 39–47. doi: 10.1016/j.soilbio.2017.09.026
Sui, X., Zeng, X., Li, M., Weng, X., Frey, B., Yang, L., et al. (2022). Influence of different vegetation types on soil physicochemical parameters and fungal communities. Microorganisms 10:829. doi: 10.3390/microorganisms10040829
Sun, X., Wang, G., Ye, Y., Ma, Q., Guan, Q., and Jones, D. L. (2021). Response of nitrogen fractions in the rhizosphere and bulk soil to organic mulching in an urban forest plantation. J. Forestry Res. 32, 2577–2588. doi: 10.1007/s11676-021-01310-2
Tabbagh, A., Cheviron, B., Henine, H., Guérin, R., and Bechkit, M. A. (2017). Numerical determination of vertical water flux based on soil temperature profiles. Adv. Water Resources 105, 217–226. doi: 10.1016/j.advwatres.2017.05.003
Wang, B., Liu, D., Yang, J., Zhu, Z., Darboux, F., Jiao, J., et al. (2021). Effects of forest floor characteristics on soil labile carbon as varied by topography and vegetation type in the Chinese Loess Plateau. Catena 196:104825. doi: 10.1016/j.catena.2020.104825
Wang, H., Wu, J., Li, G., and Yan, L. (2020a). Changes in soil carbon fractions and enzyme activities under different vegetation types of the northern Loess Plateau. Ecol. Evol. 10, 12211–12223. doi: 10.1002/ece3.6852
Wang, N., He, X., Zhao, F., Wang, D., and Jiao, J. (2020b). Soil seed bank in different vegetation types in the Loess Plateau region and its role in vegetation restoration. Restoration Ecol. 28, A5–A12. doi: 10.1111/rec.13169
Wang, T., Li, P., Li, Z., Hou, J., Xiao, L., Ren, Z., et al. (2019). The effects of freeze-thaw process on soil water migration in dam and slope farmland on the Loess Plateau, China. Sci. Total Environ. 666, 721–730. doi: 10.1016/j.scitotenv.2019.02.284
Wei, X., Shao, M., Fu, X., Ågren, G. I., and Yin, X. (2011). The effects of land use on soil N mineralization during the growing season on the northern Loess Plateau of China. Geoderma 160, 590–598. doi: 10.1016/j.geoderma.2010.11.007
Wilkinson, D. M., and Sherratt, T. N. (2016). Why is the world green? the interactions of top–down and bottom–up processes in terrestrial vegetation ecology. Plant Ecol. Diversity 9, 127–140. doi: 10.1080/17550874.2016.1178353
Williams-Linera, G., Bonilla-Moheno, M., López-Barrera, F., and Tolome, J. (2021). Litterfall, vegetation structure and tree composition as indicators of functional recovery in passive and active tropical cloud forest restoration. Forest Ecol. Manag. 493:119260. doi: 10.1016/j.foreco.2021.119260
Wu, J., Wang, H., Li, G., Ma, W., Wu, J., Gong, Y., et al. (2020). Vegetation degradation impacts soil nutrients and enzyme activities in wet meadow on the Qinghai-Tibet Plateau. Sci. Rep. 10:21271. doi: 10.1038/s41598-020-78182-9
Wu, J., Wang, H., Li, G., Wu, J., and Ma, W. (2021). Vertical and seasonal changes in soil carbon pools to vegetation degradation in a wet meadow on the Qinghai-Tibet Plateau. Sci. Rep. 11:12268. doi: 10.1038/s41598-021-90543-6
Xiao, L., Zhang, Y., Li, P., Xu, G., Shi, P., and Zhang, Y. (2019). Effects of freeze-thaw cycles on aggregate-associated organic carbon and glomalin-related soil protein in natural-succession grassland and Chinese pine forest on the Loess Plateau. Geoderma 334, 1–8. doi: 10.1016/j.geoderma.2018.07.043
Xu, C. Y., Du, C., Jian, J. S., Hou, L., Wang, Z. K., Wang, Q., et al. (2021). The interplay of labile organic carbon, enzyme activities and microbial communities of two forest soils across seasons. Sci. Rep. 11:5002. doi: 10.1038/s41598-021-84217-6
Yan, M., Cui, F., Liu, Y., Zhang, Z., Zhang, J., Ren, H., et al. (2019). Vegetation type and plant diversity affected soil carbon accumulation in a postmining area in Shanxi Province, China. Land Degradation Dev. 31, 181–189. doi: 10.1002/ldr.3438
Yang, C., Li, G., Yan, L., Ma, W., Wu, J., Tan, Y., et al. (2022a). Effects of plant community type on soil methane flux in semiarid loess hilly region, Central Gansu Province, China. Adv. Atmospheric Sci. 39, 1360–1374. doi: 10.1007/s00376-022-1169-4
Yang, Y., Shi, G., Liu, Y., Ma, L., Zhang, Z., Jiang, S., et al. (2022b). Experimental warming has not affected the changes in soil organic carbon during the growing season in an alpine meadow ecosystem on the qinghai-tibet plateau. Front. Plant Sci. 13:847680. doi: 10.3389/fpls.2022.847680
Yang, M., Guo, Q., Tong, T., Li, N., Xie, S., and Long, Y. (2017). Vegetation type and layer depth influence nitrite-dependent methane-oxidizing bacteria in constructed wetland. Arch. Microbiol. 199, 505–511. doi: 10.1007/s00203-016-1328-z
Ye, W., and Li, C. (2018). The consequences of changes in the structure of loess as a result of cyclic freezing and thawing. Bull. Eng. Geol. Environ. 78, 2125–2138. doi: 10.1007/s10064-018-1252-3
Yue, P., Zhang, Q., Zhao, W., Wang, R., Zhang, L., Wang, W., et al. (2015). Influence of environmental factors on land-surface water and heat exchange during dry and wet periods in the growing season of semiarid grassland on the Loess Plateau. Sci. China: Earth Sci. 58:13. doi: 10.1007/s11430-015-5133-3
Zhang, T., Shen, S., and Cheng, C. (2019a). Impact of radiations on the long-range correlation of soil moisture: a case study of the A’rou superstation in the Heihe River Basin. J. Geograph. Sci. 29, 1491–1506. doi: 10.1007/s11442-019-1673-3
Zhang, W., Liu, W., Xu, M., Deng, J., Han, X., Yang, G., et al. (2019b). Response of forest growth to C:N:P stoichiometry in plants and soils during Robinia pseudoacacia afforestation on the Loess Plateau. China. Geoderma 337, 280–289. doi: 10.1016/j.geoderma.2018.09.042
Zhao, W., Yin, Y., Li, S., Liu, J., Dong, Y., and Su, S. (2022). Soil microbial community varied with vegetation types on a small regional scale of the qilian mountains. Sustainability 14, 1–15. doi: 10.3390/su14137910
Keywords: Longzhong Loess Plateau, vegetation restoration type, seasonal freeze-thaw, soil carbon pools, redundancy analysis
Citation: Liu S, Wu J, Li G, Yang C, Yuan J and Xie M (2022) Seasonal freeze-thaw characteristics of soil carbon pools under different vegetation restoration types on the Longzhong Loess Plateau. Front. Ecol. Evol. 10:1019627. doi: 10.3389/fevo.2022.1019627
Received: 15 August 2022; Accepted: 31 October 2022;
Published: 17 November 2022.
Edited by:
Fujiang Hou, Lanzhou University, ChinaReviewed by:
Junyi Liang, China Agricultural University, ChinaMalak M. Tfaily, University of Arizona, United States
Copyright © 2022 Liu, Wu, Li, Yang, Yuan and Xie. This is an open-access article distributed under the terms of the Creative Commons Attribution License (CC BY). The use, distribution or reproduction in other forums is permitted, provided the original author(s) and the copyright owner(s) are credited and that the original publication in this journal is cited, in accordance with accepted academic practice. No use, distribution or reproduction is permitted which does not comply with these terms.
*Correspondence: Guang Li, bGlnQGdzYXUuZWR1LmNu