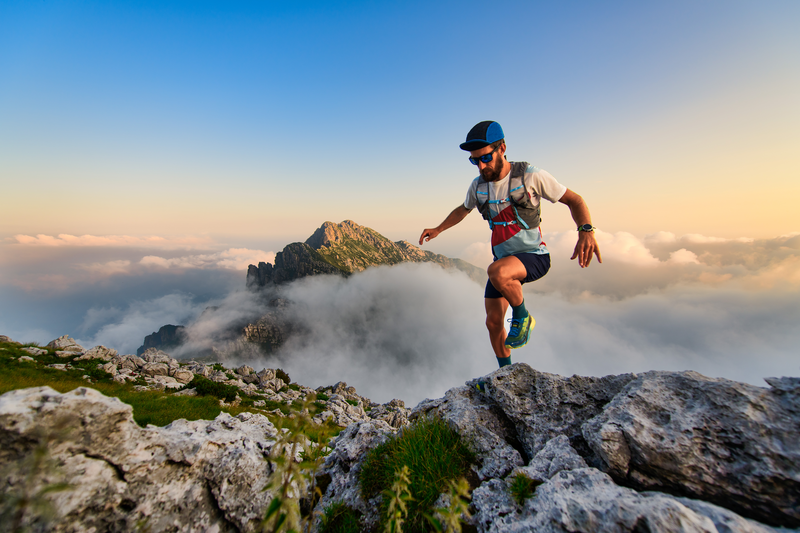
94% of researchers rate our articles as excellent or good
Learn more about the work of our research integrity team to safeguard the quality of each article we publish.
Find out more
ORIGINAL RESEARCH article
Front. Ecol. Evol. , 03 October 2022
Sec. Interdisciplinary Climate Studies
Volume 10 - 2022 | https://doi.org/10.3389/fevo.2022.1011495
This article is part of the Research Topic Plant-Soil-Microbe Interactions and Drivers in Ecosystem Development and Ecological Restoration View all 5 articles
Clarifying the soil microbial metabolism and resource limitations could help to understand the functions and processes of aboveground ecosystems, as well as to predict ecosystem stability under global climate change. Karst tiankeng is a kind of large-scale negative surface terrain on the surface which is similar to an oasis in degraded karst landscapes, but their soil microbial resource limitations still unclear. In this study, we evaluated and compared the soil microbial resource limitation in non-degraded tiankeng (NDT), moderately degraded tiankeng (MDT), heavily degraded tiankeng (HDT), and outside tiankeng (OT) by calculating soil ecoenzymatic stoichiometry. Overall, soil microbial communities were more limited by C and P in karst tiankeng ecosystem. The soil microbial C and P limitations significantly differed with the karst tiankeng degradation increased, and the lowest C and P limitations were observed in NDT. The higher microbial C and P limitations were observed in OT. Linear regression and redundancy analysis indicated that soil microbial C and P limitations were significantly influenced by soil nutrients. Karst tiankeng degradation influence the biogeochemical cycle and function of karst tiankeng systems. Our results highlight that karst tiankeng (especially the NDT) can provide a stable habitat for the survival of microorganisms in karst areas. Karst tiankeng is essential for regional ecological restoration and biodiversity conservation.
Biodiversity loss and global climate change are two serious problems facing different ecosystems in the world today (Liu and Ni, 2021). Karsts are a prime example of such a troubled ecosystem. The total area of global karst landforms is about 20 million km2 and about 12% of the world’s total area. It is one of the important carbon pools on the earth and a global biodiversity hotspot (Jiang et al., 2014; Li et al., 2017). With intensified human activities and climate change, karst areas have suffered severe biodiversity loss and environmental changes. Karst biodiversity is disappearing at an alarming rate, causing many endemic species to go extinct (Clements et al., 2006). Therefore, it is urgent to pay attention to ecological restoration and biodiversity conservation in karst areas.
As a special and large-scale negative surface terrain in karst areas, karst tiankeng is considered an oasis in degraded karst landscapes (Su et al., 2017). The underground forest nurtured by the unique geographical environment of karst tiankeng is of great significance for regional biodiversity conservation (Shui et al., 2015). Karst tiankeng is a special karst geological landscape first discovered in the karst area of southwest China and named by Zhu (2001). China is the “kingdom” of karst tiankeng resources, accounting for 70% of the world’s karst tiankengs, and preserving a systematic and complete chain of karst tiankeng evolution (Pu et al., 2021). Karst tiankeng has extraordinary spatial and morphological features such as huge volumes, steep and trapped rock walls, and deep well-shaped, or barrel-shaped profiles (Zhu and Waltham, 2005). Influenced by the vertical tiankeng walls of the tiankeng, the internal habitat of the tiankeng is relatively independent of the external environment, with unique hydrothermal conditions and microclimate. The unique closed ecosystem of karst tiankeng breeds unique flora, animal, and microbial resources (Pu et al., 2017; Jiang et al., 2021). Soil microorganisms are the most active components in terrestrial ecosystems, participating in almost all material transformation processes in the soil, and have a significant impact on soil fertility and vegetation communities (Blagodatskaya and Kuzyakov, 2013; Lagerlof et al., 2014). Thus, it is crucial to understand soil microbial processes in karst tiankeng ecosystems.
Soil microbial processes are controlled by microbial biomass and activity, which in turn are limited by the availability of nutrient (C, N, and P) resources, i.e., microbial resource limitation (Hill et al., 2012). Recently, ecoenzymatic stoichiometry has been widely used to characterize microbial resource limitations (Sinsabaugh et al., 2009). Ecoenzymatic stoichiometry is to calculate the ratio of different extracellular enzyme activities by using the combination of metrology and ecological metabolism theory (Sinsabaugh et al., 2008). Currently, the extracellular enzymes β-1,4-glucosidase (BG), leucine aminopeptidase (LAP), β-1,4-N-acetylglucosidase (NAG), and acid/Alkaline phosphatase (AP) is considered as the main biomarker for the microbial acquisition of C, N, and P (Sinsabaugh, 1994). Extracellular enzyme activity and enzymometry can reflect soil microbial metabolic characteristics and ecological responses to environmental changes (Sinsabaugh and Shah, 2011). Using this approach, microbial resource limitations in different ecosystems have been reported (Chen et al., 2019; Cui et al., 2019; Guan et al., 2020; Wang et al., 2020). However, our understanding of the microbial resource limitation of the karst tiankeng is still unclear. Understanding microbial resource limitations can identify the ability of microbes to utilize nutrient materials and estimate the balance between soil nutrient supply and microbial nutrient demand in ecosystems (Sinsabaugh et al., 2009). This information is useful for understanding soil nutrient cycling and energy flow processes in karst tiankeng, and predicting karst tiankeng ecosystem responses to global climate change.
Original tiankeng (non-degraded tiankeng) and degraded tiankeng are the two most representative types of karst tiankeng. The walls of the non-degraded tiankeng are upright and almost unaffected by human activities, maintaining a relatively independent habitat inside (Shui et al., 2022). Degraded tiankeng refers to the tiankeng that has lost the track of the underground river or the integrity of the vertical cliff wall has been severely damaged (Zhu and Waltham, 2005). The degraded tiankeng is greatly disturbed by the outside world, and the ecosystem and community distribution patterns in the pit are different from those of the non-degraded tiankeng (Jian et al., 2018). However, we currently do not know whether tiankeng degradation has an impact on soil properties, plant communities, and microbial communities, which in turn affects soil microbial processes in the karst tiankeng ecosystem.
In this study, microbial resource limitation studies were carried out in the karst tiankeng ecosystem. Three types of karst tiankengs (non-degraded tiankeng, moderately degraded tiankeng, and heavily degraded tiankeng) with different degrees of degradation were selected, they represent the basic types of karst tiankengs, and the outside karst tiankeng sites were selected as controls. Chen et al. (2019) study of microbial nutrient limitation in karst areas showed that karst areas were limited by C and P. Therefore, we hypothesized that the karst tiankeng ecosystem might follow a similar pattern of resource limitation. However, the karst tiankeng has unique habitats inside, so the microbial resource limitation may be lower, therefore, we hypothesize that (i) the microbial C and P resource limitation is lower in the karst tiankeng ecosystem; (ii) tiankengs with different degrees of degradation represent differences in environmental disturbances and microhabitats, and we hypothesize that as tiankengs degrade, the resource limitation of soil microbes will be more pronounced.
The study area is located in the Haifeng natural reserve, Zhanyi District, Qujing City, Yunnan Province, China (25°35′−25°57′N, 103°29′−103°39′E; Supplementary Figure 1). There has a typical subtropical plateau monsoon climate, with an average annual temperature of 14.5°C and average annual precipitation of 1,008 mm. Rainfall is mainly concentrated in summer and autumn. The soil is classified as red soils (rhodic nitisols in FAO classification). The study area is characterized by a typical karst landscape with severe soil erosion. The lithology in this area is mostly limestone. The natural reserve consists of dozens of karst tiankeng of varying sizes and degradation.
According to the sequence of the degradation degree of the tiankeng (Chen et al., 2009), three types of tiankeng were established: non-degraded tiankeng (NDT), moderately degraded tiankeng (MDT), and heavily degraded tiankeng (HDT). At the same time, the outside tiankeng sites (OT) were selected as a control. The morphological features of the three tiankengs were shown in Supplementary Table 1. Six plots (10 m × 10 m) were set for each tiankeng type, and the distance between each plot was >15 m. The original tiankeng is surrounded by vertical rock walls, and the six plots are located at the bottom of the tiankeng. The moderately and severely degraded tiankeng include two plots (tiankeng bottoms) and four plots (tiankeng slopes). Ten plots (10 m × 10 m) were randomized established at outside tiankeng sampling site with the distance of >15 m between the plots. Soil samples were collected in September 2021. In each plot, three quadrats (1 m × 1 m) were randomly established. In each quadrat, soil samples of 0–15 cm depth were collected in a five-point pattern and mixed as a composite sample. Finally, the three composite soil samples from each plot were mixed to form one sample as a single replicate for each type of tiankeng. This sampling method is widely used to eliminate errors caused by sampling plot soil heterogeneity (Veres et al., 2015). It means that six replicates of soil samples were obtained in each type of tiankeng, and 10 replicates of soil samples were obtained outside tiankeng sites. A total of 28 composite soil samples were collected. The collected soil sample was passed through a sieve (2 mm) and divided into two subsamples. One subsample was immediately stored at 4°C for measurement of enzymatic activities, and the other sample was air-dried for soil physicochemical analysis.
Soil bulk density was determined using ring sampler weighing (Zhou et al., 2019). The soil water content using the gravimetric method (Wang et al., 2016). Soil pH was estimated at a 1:2.5 soil: water (w/v) mixture using a glass electrode meter (InsMark™ IS126, Shanghai, China). The potassium dichromate oxidation method was used to measure the soil organic carbon (SOC). The soil total nitrogen (TN) was measured by the Kjeldahl method. Total phosphorus (TP) was detected using alkali fusion-Mo-Sb anti spectrophotometric method. The total phosphorus (TP) was determined by alkali fusion-Mo-Sb anti spectrophotometric. The total available phosphorus (AP) was determined by sodium hydrogen carbonate solution-Mo-Sb anti spectrophotometric method (Bao, 2000). Assays of soil enzyme activities involved in one C-acquiring enzyme (BG), two N-acquiring enzymes (NAG and LAP), and one P-acquiring enzyme (AP), were using the published microplate protocols according to the method of Saiya-Cork et al. (2002). The activity of the enzyme was defined as nmol g–1 SOC h–1.
Two methods were used to investigate microbial resource limitation status. First, using C:N as x-axis and N:P as y-axis to make a scatter plot of ecoenzymatic stoichiometry, reflecting different resource limitations (P, N, C and N, and C and P limited) (Hill et al., 2012; Schmidt et al., 2016). Second, vector length (L, unitless) and angle (A°) of enzymatic activity were calculated as follows (Moorhead et al., 2013). The vector length was used to quantified the microbial C limitation, and the longer vector length indicates greater C limitation. The vector angle was used to quantified the microbial N or P limitation, the vector angles <45° and >45° indicate N limitation and P limitation, respectively.
The differences in soil physicochemical properties, enzyme activities, and ecoenzymatic stoichiometry were examined by a one-way analysis of variance (ANOVA), followed by Duncan’s new multiple range method. Spearman coefficients were used to examine the relationship between soil enzyme activities and soil physicochemical properties using the R 4.1.2 software. Linear regressions analysis was used to analyze the relationships between soil nutrient content and soil nutrient stoichiometry vs. vector lengths and angles using the Origin 2019b (Cui et al., 2019). A redundancy analysis (RDA) was used after the Hellinger transformation of the data to examine the key environmental factors of changes in enzyme activities and ecoenzymatic stoichiometry using the “vegan” package in R 4.1.2 software. The statistical analyses were using the SPSS version 24 (SPSS Inc., Chicago, IL, USA). The scatter plot and bar graphs were drawn using Origin 2019b.
There were significant effects of tiankeng types on soil properties (Table 1). The TN, AP, and AN content was significantly higher in NDT compared to the other tiankeng types (P < 0.05). The SOC and TP content was significantly higher in NDT and MDT than in HDT. The C:N ratio had significantly higher values in HDT compared to the other tiankeng types (P < 0.05). The SWC was highest in NDT (P < 0.05). Soil pH had a narrow variation, with a range of 6.42–6.80. The significant differences in SOC, TN, TP, and AP were observed between the inside and outside the karst tiankeng (P < 0.05). The plant characteristics differed among the tiankeng types. The plant Shannon–Wiener was highest in MDT. The plant coverage was highest in NDT. As the karst tiankeng degradation level increased, the plant coverage decreased. Compared with the inside karst tiankeng sites, the plant Shannon–Wiener and coverage of OT sites were lower (Supplementary Table 2).
Extracellular enzyme activity varied among tiankeng types (Table 2). The activities of BG, NAG, and LAP enzyme activities were higher in the NDT than in other tiankeng types (P < 0.05). AP enzyme activity had not significantly differed amongst the types of tiankeng and was highest in the NDT. The significantly lowest activities of BG and LAP were founded in OT (P < 0.05). The activities of NAG, LAP, and AP did not show significant differences between HDT and OT. The enzymatic stoichiometry of the C:N ratio was significantly higher in the HDT than in other tiankeng types, while the N:P ratio was significantly higher in NDT and MDT (P < 0.05). The enzymatic stoichiometry of the N:P ratio has the highest values in the NDT. The HDT had no significant difference with OT in C:N and N:P ratios (P > 0.05).
Table 2. The selected soil extracellular enzyme activities and enzymatic stoichiometry for different types of karst tiankeng.
Different tiankeng types have different characteristics of ecoenzymatic stoichiometry. As shown in Figure 1, all sampling sites were located in P limited and C and P limited areas. The vector analysis of angle results confirmed that microbes resource limitation was P, not N (vector angle >45°) (Figure 2). The vector lengths differed significantly amongst the tiankeng types. The vector length was lowest in NDT, suggesting the lowest relative C limitation (P < 0.05; Figure 2A). The vector angle was highest in OT, indicating the highest relative P limitation (P < 0.05; Figure 2B).
Figure 1. The scatter plot of ecoenzymatic stoichiometry for different types of karst tiankeng. NDT, non-degraded tiankeng; MDT, moderately degraded tiankeng; HDT, heavily degraded tiankeng; OT, outside tiankeng.
Figure 2. The vector angles (A) and lengths (B) under different types of karst tiankeng. NDT, non-degraded tiankeng; MDT, moderately degraded tiankeng; HDT, heavily degraded tiankeng; OT, outside tiankeng. Different lowercase letters indicate significant differences between means for different types of karst tiankeng (P < 0.05).
The linear regression analysis revealed significant negative correlations between vector length and soil total P. The significantly negative correlations between vector angle and soil total N and soil total P, while there were significant positive correlations between C:N ratios (P < 0.05; Figure 3).
Figure 3. Relationships between vector angle and soil total N or soil total P or soil C: N (A–C), and between vector length and total P (D). All P-values of the regression analysis were <0.05.
The RDA ordination indicated that the soil properties explained approximately 46.00 and 41.17% of the variation in extracellular enzyme activities and their stoichiometry (Figure 4). BG activity was strongly positively correlated with C:P ratio. LAP and NAG activities were a strongly negative correlation with most soil nutrients. AP activity was positive correlation with available P, available N, and TP. In addition, enzyme C:N was strongly positive correlation with most soil nutrients, while the enzyme N:P was strongly negative correlation with most soil nutrients. The enzyme C:P was positive correlation with soil C:N and C:P ratio.
Figure 4. Redundancy analysis of the soil enzyme activities (A) and soil enzyme stoichiometry (B). NDT, non-degraded tiankeng; MDT, moderately degraded tiankeng; HDT, heavily degraded tiankeng; OT, outside tiankeng; BG, β-1,4-glucosidase (nmol g–1 SOC h–1); NAG, β-1,4-Nacetylglucosaminidase (nmol g–1 SOC h–1); LAP, L-leucine aminopeptidase (nmol g–1 SOC h–1); AP, acid phosphatase (nmol g–1 SOC h–1); eC:N, eC:P, and eN:P represent BG:(NAG + LAP), BG:AP, and (NAG + LAP):AP, respectively; BD, soil bulk density; SWC, soil water content; SOC, soil organic carbon; TN, total nitrogen; TP, total phosphorus; Available N, available nitrogen; Available P, available phosphorus.
In our study, the C and P limitations for soil microbial communities in karst tiankeng were found (Figures 1, 2). Microbial C limitation was thought to be ubiquitous, which was consistent with previous results (Chen et al., 2018, 2019). Previous studies have generally indicated that the most common limiting factor for soil microbes is C availability (Schimel and Weintraub, 2003). The strong combined effect between organic C and soil Ca results in the vast majority of organic C in karst soils being organic mineral combined C (Wen et al., 2017). Therefore, low available C content in karst soils is common. However, microbial N or P limitation in karst areas was controversial. Some studies indicate that soil microbial communities in karst areas were limited by P rather than N (Chen et al., 2018, 2019). Some studies have found that karst ecosystems have stronger microbial N limitations. Microbial resource limitations are often related to the availability of soil available resources (Castle et al., 2017). In karst calcareous soils, Ca easily combine with P to form an insoluble precipitate. The low available P (1.00–1.08 mg kg–1) in karst tiankeng soils (Table 1) may be responsible for microbial P limitation. In addition, microbial P limitation was a relative concept, and it also depends on the content of other nutrients (Chen et al., 2018). For example, when the soil was abundant in N content, soil P may become insufficient to provide for microbial growth, in other words, the microbial community was limited by P. According to previous studies (Wen et al., 2016), the soil total N (1.06–4.85 g kg–1) of karst tiankeng is at a high level. These results can support our explanation. In general, the general pattern of microbial resource limitation in karst tiankeng was limited by C and P. The above results are consistent with the first hypothesis of this study.
The vector analysis of ecoenzymatic stoichiometry indicated that relative degrees of microbial C and P limitation were the highest and lowest in the HDT and NDT, respectively (Figure 2A). As karst tiankeng degrades, microbial C and P limitation will be more pronounced, and supports the second hypothesis of this study. As one of the important ecosystem functions, the material cycle is mainly accomplished through the distribution and circulation of nutrients. Nutrient cycling is influenced by a variety of biological and abiotic factors such as climate, topography, soil microorganisms, and plants (Ma et al., 2015; Luo et al., 2017). The unique soil environment and microclimate within the NDT have bred an abundant underground forest (Shui et al., 2022). Increased aboveground vegetation biomass directly leads to increased litter and root turnover, thereby promoting the accumulation of soil organic matter and nutrients (Hu et al., 2006). As plant roots, microorganisms, and soil organic matter increase, soil aggregate stability also increases. The restoration of soil aggregates, in turn, can improve soil aeration and water retention, thereby helping to increase microbial activity (Wang et al., 2021). In addition, the decomposition of soil organic matter is usually easier to decompose in a humid and hot environment, while NDT has a significant “cold well effect” (Shui et al., 2018), which may weaken the decomposition process of soil organic matter and promote the accumulation of soil nutrients. The regional variation of microbial resource limitation was predominantly controlled by soil nutrients were supported by our results (Figures 3, 4). Karst tiankeng degradation is accompanied by decreased community stability, hydrothermal conditions, and climate fluctuations. Heavily degraded tiankeng, in particular, the collapse of vertical cliffs reshapes its internal hydrothermal conditions and microclimate, which is necessarily accompanied by changes in nutrient cycling processes. Slope runoff on degraded inverted slopes may exacerbate the leaching of karst soil nutrients (Jiang et al., 2021). Reduced plant diversity and coverage may lead to a reduction in nutrient input, which leads to a decrease in microbial metabolism and enzymatic synthesis capacity. Further in support of this, our results showed the lowest soil nutrient contents were detected in HDT (Table 1). Therefore, the microbial C and P limitations of HDT is higher than other types of karst tiankengs.
Our results also reflect higher microbial C and P limitations outside karst tiankeng. Outside the tiankeng is a typical karst landscape characterized by sparse vegetation, thin soil, and exposed rocks (Jiang et al., 2022). Low litter is not conducive to soil nutrient accumulation, and the highly developed karst system makes soil nutrient loss faster (Liu et al., 2020). Previous studies have indicated that in nutrient-poor soil environments, organic acids produced by plants accelerate the leaching of Ca and P in soil (Wei et al., 2010). Based on the previously mentioned, Ca form complexes with C and P, which may increase the microbial C and P limitations of OT. The microbial P limitation in HDT is higher than OT, which may be related to interference from grazing activities. Compared with other types of karst tiankengs, HDT has the strongest accessibility. Studies have indicated that soil nutrient loss is associated with grazing by native ungulates (Gass and Binkley, 2011). Our findings confirm that changes in the habitat within the tiankeng alter the rate of microbial-mediated ecosystem processes.
In the current study, using ecological enzyme measurement indicators, the general pattern of microbial resource limitation of karst tiankeng was investigated. The unique habitats inside karst tiankeng nurture high-quality soil environments and abundant vegetation that help to alleviate microbial resource limitations. With the degradation of karst tiankengs, the destruction of habitats inside the tiankengs, and the increase in external disturbances have exacerbated the resource limitation of soil microbes. From the perspective of alleviating microbial resource limitation, karst tiankeng (especially the non-degraded tiankeng) is essential for the ecological restoration and biodiversity conservation of karst ecosystems. Moreover, our study lacks monitoring of the microclimate of karst tiankengs, which limits the interpretation of habitat changes caused by karst tiankeng degradation, and we will focus on this part in future research. In addition, plant-soil-microbes as a continuum whose internal material and energy flow characteristics are critical to the food web and ecosystem function. It is not enough to only investigate microbial resource limitation, research with the eco-stoichiometric of plant-soil-microbial wholes should be investigated in the future.
By calculating soil extracellular enzymatic activities and ecoenzymatic stoichiometry, the microbial resource limitations of karst tiankeng were quantified. The results showed that the soil microbial communities of karst tiankeng were limited by C and P, and soil nutrients may be important factors limiting microbial metabolic activities and ecological functions in karst tiankeng. Among the three karst tiankeng types, the soil microbial communities of the non-degraded tiankeng were the lowest relative C and P limitations, owing to the abundant vegetation and soil nutrients in the unique habitat of non-degraded tiankeng that can alleviate the resource limitation of soil microbes. With the intensification of degradation, soil microbes are more resource limitation. Therefore, karst tiankeng (especially the non-degraded tiankeng) creates unique habitats for the survival of microorganisms and the maintenance of ecological functions in degraded karst areas and contributes to the conservation and biodiversity of species banks in the karst region. Our results provide useful knowledge for the management and ecological restoration of karst tiankeng ecosystems.
The original contributions presented in this study are included in the article/Supplementary material, further inquiries can be directed to the corresponding author.
CJ collected the soil samples, carried out the experiments, analyzed the data, and wrote the manuscript. HL collected the soil samples and performed the experiments. CJ and HZ designed the experimental plan. HZ edited the manuscript. All authors contributed to the article and approved the submitted version.
This work was supported by the Shenzhen Fundamental Research Program (GXWD20201231165807007-20200812142216001).
The authors declare that the research was conducted in the absence of any commercial or financial relationships that could be construed as a potential conflict of interest.
All claims expressed in this article are solely those of the authors and do not necessarily represent those of their affiliated organizations, or those of the publisher, the editors and the reviewers. Any product that may be evaluated in this article, or claim that may be made by its manufacturer, is not guaranteed or endorsed by the publisher.
The Supplementary Material for this article can be found online at: https://www.frontiersin.org/articles/10.3389/fevo.2022.1011495/full#supplementary-material
Blagodatskaya, E., and Kuzyakov, Y. (2013). Active microorganisms in soil: Critical review of estimation criteria and approaches. Soil Biol. Biochem. 67, 192–211. doi: 10.1016/j.soilbio.2013.08.024
Castle, S. C., Sullivan, B. W., Knelman, J., Hood, E., Nemergut, D. R., Schmidt, S. K., et al. (2017). Nutrient limitation of soil microbial activity during the earliest stages of ecosystem development. Oecologia 185, 513–524. doi: 10.1007/s00442-017-3965-6
Chen, H., Li, D. J., Mao, Q. G., Xiao, K. C., and Wang, K. L. (2019). Resource limitation of soil microbes in karst ecosystems. Sci. Total Environ. 650, 241–248. doi: 10.1016/j.scitotenv.2018.09.036
Chen, H., Li, D. J., Xiao, K. C., and Wang, K. L. (2018). Soil microbial processes and resource limitation in karst and non-karst forests. Funct. Ecol. 32, 1400–1409. doi: 10.1111/1365-2435.13069
Chen, S. X., Shui, W., He, J. F., and Zhang, Q. C. (2009). The construction of tiankeng landscape evaluation model and its index system. Acta Geol. Sich. 29, 28–34.
Clements, R., Sodhi, N. S., Schilthuizen, M., and Ng, P. K. L. (2006). Limestone karsts of southeast Asia: Imperiled arks of biodiversity. Bioscience 56, 733–742. doi: 10.1641/0006-3568200656
Cui, Y. X., Fang, L. C., Guo, X. B., Han, F., Ju, W. L., Ye, L. P., et al. (2019). Natural grassland as the optimal pattern of vegetation restoration in arid and semi-arid regions: Evidence from nutrient limitation of soil microbes. Sci. Total Environ. 648, 388–397. doi: 10.1016/j.scitotenv.2018.08.173
Gass, T. M., and Binkley, D. (2011). Soil nutrient losses in an altered ecosystem are associated with native ungulate grazing. J. Appl. Ecol. 48, 952–960. doi: 10.1111/j.1365-2664.2011.01996.x
Guan, P. T., Yang, J. J., Yang, Y. R., Wang, W., Zhang, P., and Wu, D. H. (2020). Land conversion from cropland to grassland alleviates climate warming effects on nutrient limitation: Evidence from soil enzymatic activity and stoichiometry. Glob. Ecol. Conserv. 24:e01328. doi: 10.1016/j.gecco.2020.e01328
Hill, B. H., Elonen, C. M., Seifert, L. R., May, A. A., and Tarquinio, E. (2012). Microbial enzyme stoichiometry and nutrient limitation in US streams and rivers. Ecol. Indic. 18, 540–551. doi: 10.1016/j.ecolind.2012.01.007
Hu, Y. L., Wang, S. L., and Zeng, D. H. (2006). Effects of single chinese fir and mixed leaf litters on soil chemical, microbial properties and soil enzyme activities. Plant Soil 282, 379–386. doi: 10.1007/s11104-006-0004-5
Jian, X. M., Shui, W., Wang, Y. N., Wang, Q. F., Chen, Y. P., Jiang, C., et al. (2018). Species diversity and stability of grassland plant community in heavily-degraded karst tiankeng: a case study of Zhanyi tiankeng in Yunnan, China. Acta Ecol. Sin. 38, 4704–4714. doi: 10.5846/stxb201706281163
Jiang, C., Feng, J., Zhu, S. F., and Shui, W. (2021). Characteristics of the Soil Microbial Communities in Different Slope Positions along an Inverted Stone Slope in a Degraded Karst Tiankeng. Biol. Basel 10:474. doi: 10.3390/biology10060474
Jiang, C., Sun, X.-R., Feng, J., Zhu, S.-F., and Shui, W. (2022). Metagenomic analysis reveals the different characteristics of microbial communities inside and outside the karst tiankeng. BMC Microbiol. 22:115. doi: 10.1186/s12866-022-02513-1
Jiang, Z. C., Lian, Y. Q., and Qin, X. Q. (2014). Rocky desertification in Southwest China: Impacts, causes, and restoration. Earth Sci. Rev. 132, 1–12. doi: 10.1016/j.earscirev.2014.01.005
Lagerlof, J., Adolfsson, L., Boerjesson, G., Ehlers, K., Vinyoles, G. P., and Sundh, I. (2014). Land-use intensification and agroforestry in the Kenyan highland: Impacts on soil microbial community composition and functional capacity. Appl. Soil Ecol. 82, 93–99. doi: 10.1016/j.apsoil.2014.05.015
Li, D. J., Wen, L., Yang, L. Q., Luo, P., Xiao, K. C., Chen, H., et al. (2017). Dynamics of soil organic carbon and nitrogen following agricultural abandonment in a karst region. J. Geophys. Res. Biogeosci. 122, 230–242. doi: 10.1002/2016jg003683
Liu, L. B., and Ni, J. (2021). Variations of the biodiversity and carbon functions of karst forests in two morphologically different sites in southwestern China. Israel J. Ecol. Evolut. 67, 9–16. doi: 10.1163/22244662-bja10006
Liu, Y., Liu, C., Rubinato, M., Guo, K., Zhou, J., and Cui, M. (2020). An Assessment of Soil’s Nutrient Deficiencies and Their Influence on the Restoration of Degraded Karst Vegetation in Southwest China. Forests 11:797. doi: 10.3390/f11080797
Luo, W., Li, M.-H., Sardans, J., Lu, X.-T., Wang, C., Penuelas, J., et al. (2017). Carbon and nitrogen allocation shifts in plants and soils along aridity and fertility gradients in grasslands of China. Ecol. Evolut. 7, 6927–6934. doi: 10.1002/ece3.3245
Ma, J., Bu, R., Liu, M., Chang, Y., Qin, Q., and Hu, Y. (2015). Ecosystem carbon storage distribution between plant and soil in different forest types in Northeastern China. Ecol. Engin. 81, 353–362. doi: 10.1016/j.ecoleng.2015.04.080
Moorhead, D. L., Rinkes, Z. L., Sinsabaugh, R. L., and Weintraub, M. N. (2013). Dynamic relationships between microbial biomass, respiration, inorganic nutrients and enzyme activities: informing enzyme-based decomposition models. Front. Microbiol. 4:223. doi: 10.3389/fmicb.2013.00223
Pu, G. Z., Lv, Y. N., Xu, G. P., Zeng, D. J., and Huang, Y. Q. (2017). Research Progress on Karst Tiankeng Ecosystems. Botan. Rev. 83, 5–37. doi: 10.1007/s12229-017-9179-0
Pu, G. Z., Wang, K. Y., Mo, L., Zeng, D. J., and Chen, X. X. (2021). Research progress on evolution and vegetation ecology of karst Tiankeng in China. Guihaia 41:12. doi: 10.11931/guihaia.gxzw202012032
Saiya-Cork, K. R., Sinsabaugh, R. L., and Zak, D. R. (2002). The effects of long term nitrogen deposition on extracellular enzyme activity in an Acer saccharum forest soil. Soil Biol. Biochem. 34, 1309–1315. doi: 10.1016/s0038-0717(02)00074-3
Schimel, J. P., and Weintraub, M. N. (2003). The implications of exoenzyme activity on microbial carbon and nitrogen limitation in soil: a theoretical model. Soil Biol. Biochem. 35, 549–563. doi: 10.1016/s0038-0717(03)00015-4
Schmidt, S. K., Porazinska, D., Concienne, B. L., Darcy, J. L., King, A. J., and Nemergut, D. R. (2016). Biogeochemical Stoichiometry Reveals P and N Limitation Across the Post-glacial Landscape of Denali National Park, Alaska. Ecosystems 19, 1164–1177. doi: 10.1007/s10021-016-9992-z
Shui, W., Chen, Y., Jian, X., Jiang, C., Wang, Q., and Guo, P. (2018). Spatial pattern of plant community in original karst tiankeng: A case study of Zhanyi tiankeng in Yunnan, China. J. Appl. Ecol. 29, 1725–1735. doi: 10.13287/j.1001-9332.201806.010
Shui, W., Chen, Y., Jian, X., Jiang, C., Wang, Q., Zeng, Y., et al. (2022). Original karst tiankeng with underground virgin forest as an inaccessible refugia originated from a degraded surface flora in Yunnan, China. Scientif. Rep. 12:78. doi: 10.1038/s41598-022-13678-0
Shui, W., Chen, Y. P., Wang, Y. W., Su, Z. A., and Zhang, S. (2015). Origination, study progress and prospect of karst tiankeng research in China. Acta Geogr. Sin. 70, 432–446. doi: 10.11821/dlxb201503007
Sinsabaugh, R. L. (1994). Enzymatic analysis of microbial pattern and process. Biol. Fertil. Soils 17, 69–74. doi: 10.1007/bf00418675
Sinsabaugh, R. L., Hill, B. H., and Shah, J. J. F. (2009). Ecoenzymatic stoichiometry of microbial organic nutrient acquisition in soil and sediment. Nature 462, 795–U117. doi: 10.1038/nature08632
Sinsabaugh, R. L., Lauber, C. L., Weintraub, M. N., Ahmed, B., Allison, S. D., Crenshaw, C., et al. (2008). Stoichiometry of soil enzyme activity at global scale. Ecol. Lett. 11, 1252–1264. doi: 10.1111/j.1461-0248.2008.01245.x
Sinsabaugh, R. L., and Shah, J. J. F. (2011). Ecoenzymatic stoichiometry of recalcitrant organic matter decomposition: the growth rate hypothesis in reverse. Biogeochemistry 102, 31–43. doi: 10.1007/s10533-010-9482-x
Su, Y. Q., Tang, Q. M., Mo, F. Y., and Xue, Y. G. (2017). Karst tiankengs as refugia for indigenous tree flora amidst a degraded landscape in southwestern China. Scientif. Rep. 7:4249. doi: 10.1038/s41598-017-04592-x
Veres, Z., Kotroczo, Z., Fekete, I., Toth, J. A., Lajtha, K., Townsend, K., et al. (2015). Soil extracellular enzyme activities are sensitive indicators of detrital inputs and carbon availability. Appl. Soil Ecol. 92, 18–23. doi: 10.1016/j.apsoil.2015.03.006
Wang, C., Wang, G., Wang, Y., Rafique, R., Ma, L., Hu, L., et al. (2016). Fire alters vegetation and soil microbial community in alpine meadow. Land Degradat. Devel. 27, 1379–1390. doi: 10.1002/ldr.2367
Wang, J., Wang, X. T., Liu, G. B., Wang, G. L., Wu, Y., and Zhang, C. (2020). Fencing as an effective approach for restoration of alpine meadows: Evidence from nutrient limitation of soil microbes. Geoderma 363:114148. doi: 10.1016/j.geoderma.2019.114148
Wang, Y., Shahbaz, M., Zhran, M., Chen, A., Zhu, Z., Galal, Y. G. M., et al. (2021). Microbial Resource Limitation in Aggregates in Karst and Non-Karst Soils. Agronomy Basel 11:1591. doi: 10.3390/agronomy11081591
Wei, L., Chen, C., and Xu, Z. (2010). Citric acid enhances the mobilization of organic phosphorus in subtropical and tropical forest soils. Biol. Fertil. Soils 46, 765–769. doi: 10.1007/s00374-010-0464-x
Wen, L., Li, D., Yang, L., Luo, P., Chen, H., Xiao, K., et al. (2016). Rapid recuperation of soil nitrogen following agricultural abandonment in a karst area, southwest China. Biogeochemistry 129, 341–354. doi: 10.1007/s10533-016-0235-3
Wen, L., Li, D. J., Chen, H., and Wang, K. L. (2017). Dynamics of soil organic carbon in density fractions during post-agricultural succession over two lithology types, southwest China. J. Environ. Manag. 201, 199–206. doi: 10.1016/j.jenvman.2017.06.048
Zhou, H., Zhang, D., Jiang, Z., Sun, P., Xiao, H., Wu, Y., et al. (2019). Changes in the soil microbial communities of alpine steppe at Qinghai-Tibetan Plateau under different degradation levels. Sci. Total Environ. 651, 2281–2291. doi: 10.1016/j.scitotenv.2018.09.336
Zhu, X. W. (2001). China’s karst tiankeng and its value for science and tourism. Sci. Technol. Rev. 60–63.
Keywords: karst tiankeng, fragile ecosystems, ecoenzymatic stoichiometry, special habitat, resource limitation
Citation: Jiang C, Li H and Zeng H (2022) Karst tiankeng create a unique habitat for the survival of soil microbes: Evidence from ecoenzymatic stoichiometry. Front. Ecol. Evol. 10:1011495. doi: 10.3389/fevo.2022.1011495
Received: 04 August 2022; Accepted: 08 September 2022;
Published: 03 October 2022.
Edited by:
Dingde Xu, Sichuan Agricultural University, ChinaReviewed by:
Hao Yang, Guangxi Normal University, ChinaCopyright © 2022 Jiang, Li and Zeng. This is an open-access article distributed under the terms of the Creative Commons Attribution License (CC BY). The use, distribution or reproduction in other forums is permitted, provided the original author(s) and the copyright owner(s) are credited and that the original publication in this journal is cited, in accordance with accepted academic practice. No use, distribution or reproduction is permitted which does not comply with these terms.
*Correspondence: Hui Zeng, emVuZ2hAcGt1c3ouZWR1LmNu
Disclaimer: All claims expressed in this article are solely those of the authors and do not necessarily represent those of their affiliated organizations, or those of the publisher, the editors and the reviewers. Any product that may be evaluated in this article or claim that may be made by its manufacturer is not guaranteed or endorsed by the publisher.
Research integrity at Frontiers
Learn more about the work of our research integrity team to safeguard the quality of each article we publish.