- 1Department of Anthropology, New York University, New York, NY, United States
- 2The New York Consortium in Evolutionary Primatology, New York, NY, United States
- 3Department of Biology, University of Washington, Seattle, WA, United States
- 4School of Life Sciences, Arizona State University, Tempe, AZ, United States
- 5Center for Evolution and Medicine, Arizona State University, Tempe, AZ, United States
- 6The Graduate Center of City University of New York, New York, NY, United States
- 7Department of Neuroscience, University of Pennsylvania, Philadelphia, PA, United States
- 8Centre for Research in Animal Behaviour, University of Exeter, Exeter, United Kingdom
- 9ASU-Banner Neurodegenerative Disease Research Center, Arizona State University, Tempe, AZ, United States
Identifying biomarkers of age-related changes in immune system functioning that can be measured non-invasively is a significant step in progressing research on immunosenescence and inflammaging in free-ranging and wild animal populations. In the present study, we aimed to investigate the suitability of two urinary compounds, neopterin and suPAR, as biomarkers of age-related changes in immune activation and inflammation in a free-ranging rhesus macaque (Macaca mulatta) population. We also investigated age-associated variation in gene transcription from blood samples to understand the underlying proximate mechanisms that drive age-related changes in urinary neopterin or suPAR. Neopterin was significantly positively correlated with age, and had a moderate within-individual repeatability, indicating it is applicable as a biomarker of age-related changes. The age-related changes in urinary neopterin are not apparently driven by an age-related increase in the primary signaler of neopterin, IFN-y, but may be driven instead by an age-related increase in both CD14+ and CD14− monocytes. suPAR was not correlated with age, and had low repeatability within-individuals, indicating that it is likely better suited to measure acute inflammation rather than chronic age-related increases in inflammation (i.e., “inflammaging”). Neopterin and suPAR had a correlation of 25%, indicating that they likely often signal different processes, which if disentangled could provide a nuanced picture of immune-system function and inflammation when measured in tandem.
Introduction
Age-related changes in immune system function are increasingly recognized as a key component in senescence and the resulting health decline seen with increasing age (Franceschi et al., 2000; Fulop et al., 2018). The terms “immunosenescence” and “inflammaging” have been coined to describe these late-life changes in immune system function. Immunosenescence refers to the decline in effectiveness of the immune system and inflammaging refers to the increase in inflammatory processes with age (Franceschi et al., 2000, 2018; Baylis et al., 2013). Understanding the characteristics of age-related changes in immune activation and inflammation is fundamental to understanding the proximate and ultimate mechanisms driving the phenomenon of aging. Much of our current understanding of immunosenescence and inflammaging comes from research on humans and laboratory animals (Franceschi et al., 2007; Gruver et al., 2007; Frasca and Blomberg, 2016; Min and Tatar, 2018). While valuable, this research comes with biases and limits to its applicability. For example, socioeconomic confounds in humans create challenges in disentangling the biological vs. societal forces driving aging. In laboratory animals, a lack of environmental and genetic diversity can sometimes limit the translational value of the research. The use of free-ranging animals living in naturalistic conditions provides the opportunity to investigate how immunosenescence and inflammaging are shaped by life-history, behavior, and ecology.
Identifying robust biomarkers of immune system function that can be feasibly measured in wild populations is key to extending laboratory and human research on age-related immune system function to wild animal populations. In human and model species, age-related changes in immune system function are typically measured through blood sampling to measure a range of physiological biomarkers [reviewed in Peters et al. (2019)]. However, these invasive measurement methods create both logistical and ethical challenges. This is especially true for large mammal populations, where invasive measurement often necessitates trapping and anesthetizing the animals (Sapolsky, 1983; Huber et al., 1984; Ezenwa et al., 2010; Hoffman et al., 2011). These challenges could at least be partially solved by switching to non-invasive methods of measuring health and aging in these populations. Urine and feces provide avenues to collect physiological markers of health non-invasively. These include urinary or fecal measurement of glucocorticoid metabolites (Ostrowski et al., 2006; Jaimez et al., 2012; Mohlman et al., 2020), urinary C-peptide of insulin (Emery Thompson and Knott, 2008; Higham et al., 2011; Sacco et al., 2021), isotopes (Deschner et al., 2012; Vogel et al., 2012; Rioux et al., 2019), fecal parasite load (Gillespie and Chapman, 2006; Nalubamba et al., 2012; Liu et al., 2022), and thyroid hormones (Gobush et al., 2014; Cristóbal-Azkarate et al., 2016). These measures are generally indicative of energy mobilization and allocation and may be linked to health, while some may also be indirectly related to immune system activation and function. However, none of these measures provide a direct quantification of immune system activation per se. The use of validated biomarkers of immune-system functioning that can be measured non-invasively in free-ranging animals is currently quite limited.
Urinary measurement of neopterin and the soluble urokinase Plasminogen Activator Receptor (suPAR), two compounds that are regularly used in clinical settings to assess disease progression (Eisenhut, 2013; Rasmussen et al., 2016), are excellent candidates as non-invasive biomarkers of immune system function due to their broad roles in cellular immune-system activation and inflammation. Neopterin is a nucleotide synthesized by macrophages in response to stimulation by inflammatory cytokines (Hamerlinck, 1999). The protein suPAR is the soluble version of the urokinase-type plasminogen activator receptor (uPAR) (Thunø et al., 2009). suPAR originates from the cleavage and release of membrane-bound uPAR following the binding of the protein urokinase. Higher circulating concentrations of both neopterin and suPAR in blood and urine are associated with higher levels of immune system activation (Reibnegger et al., 1988; Thunø et al., 2009). Both urinary suPAR and neopterin are used in clinical settings to evaluate disease prognosis, as higher circulating concentrations of both compounds are indicative of worse disease outcomes for a range of conditions, including viral infections (Fuchs et al., 1988; Andersen et al., 2008b), parasitic infections (Brown et al., 1990; Ostrowski et al., 2005), malignant tumors (Sucher et al., 2010; Liu et al., 2017), and autoimmune diseases (Reibnegger et al., 1986; Pliyev and Menshikov, 2010). Additionally, both neopterin and suPAR have been shown to be good indicators of the level of low-grade inflammation among otherwise healthy individuals (Thunø et al., 2009; Capuron et al., 2011). Increased levels of both circulating neopterin (Reibnegger et al., 1988; Diamondstone et al., 1994; Schennach et al., 2002; Wakabayashi et al., 2020) and suPAR (Haastrup et al., 2014; Rasmussen et al., 2016, 2020; Haupt et al., 2019) have been shown to be associated with age, suggesting they may hold promise as broad biomarkers related to patterns of inflammation and immunosenescence.
However, there are also notable differences between neopterin and suPAR in their molecular function and resulting clinical significance. Neopterin is indicative specifically of innate immune system activation and is exclusively sensitive to cell-mediated (Th1-1 type) responses (Hamerlinck, 1999), making it a better prognostic tool in monitoring HIV progression compared to suPAR (Nyamweya et al., 2012). Conversely, suPAR is particularly active in wound healing, and is likely a better prognostic indicator of tissue damage compared to neopterin (Higham et al., 2020). Correlations between circulating neopterin and suPAR in human clinical trials have been reported between 0.38 and 0.41 (Nyamweya et al., 2012; Tahar et al., 2016). As such, although both neopterin and suPAR hold promise as age-related biomarkers, they reflect different aspects of biology, and measuring both in tandem may provide a fuller and more nuanced picture of patterns of immune activation and inflammation.
Many characteristics inherent in neopterin and suPAR make them well-suited for use in field work settings. Both compounds are soluble in urine, and urinary concentrations, when adjusted according to urinary creatinine, correlate strongly with circulating blood plasma concentrations (Fuchs et al., 1994; Andersen et al., 2008a; Higham et al., 2015, 2020). This means that both compounds can be collected non-invasively, removing perhaps the largest logistical and ethical challenge associated with studies on wild or free-ranging animals. Previous validation studies have shown that both compounds are relatively robust to freeze-thaw cycles and prolonged periods above freezing temperature (Heistermann and Higham, 2015; Higham et al., 2020), making them suitable for use in the field. Additionally, individual fluctuations in suPAR (Sier et al., 1999) and neopterin (Auzeby et al., 1989) concentrations across daylight hours are negligible, such that measurements occurring at any time during the day are comparable to one another, and researchers need not restrict their sample collection to a narrow window of time. Validation studies in captive rhesus macaques have shown that urinary neopterin shows a sustained spike after an immune system challenge while urinary suPAR shows a sustained spike following tissue trauma, suggesting similar functions of these compounds to those seen in humans (Higham et al., 2015, 2020). Recent studies have begun to investigate the relationship between age and neopterin concentrations in wild and free-ranging primate populations, including studies amongst primarily juveniles (Behringer et al., 2019, 2021), and amongst adult individuals (Müller et al., 2017; Dibakou et al., 2020; González et al., 2020; Negrey et al., 2021). In studies of adults, some have found a significant increase in urinary neopterin with increasing age (Müller et al., 2017; Negrey et al., 2021), while others have not found associations between age and neopterin (Dibakou et al., 2020), or found a significant positive association in some conditions, but not others (González et al., 2020). This may be because of the greater variability inherent in wild and free-ranging populations, which leads to substantial sample sizes needed to detect the relationship between age and neopterin, rather than a lack of a consistent relationship. Additionally, it may be that age-related differences in neopterin can be observed only in populations in which large numbers of advanced aged individuals are found. To our knowledge, no studies have yet assessed the relationship between suPAR and age in wild populations. Further study into suitability of both neopterin and suPAR as biomarkers of aging and age-related changes in immune system function and inflammation is needed.
Immunosenescence is also reflected in transcriptional changes in the blood (Herrero et al., 2002; de Magalhães et al., 2009; Sidler et al., 2013). Circulating levels of suPAR or neopterin (i.e., the suPAR or neopterin phenotype) can be traced to a variety of genes which may both directly and indirectly influence their production. If these genes underlie the proximate mechanisms of age-related changes in urinary suPAR and neopterin concentrations, then we would expect to find age-associated gene expression changes in the respective transcriptomes of younger vs. older individuals. The suPAR phenotype is likely related to expression of at least two genes, plasminogen activator, urokinase receptor (PLAUR), and plasminogen activator, urokinase (PLAU). suPAR is formed by the binding of urokinase to uPAR, which are two proteins directly encoded by PLAU and PLAUR, respectively (Børglum et al., 1992; Thunø et al., 2009; Connolly et al., 2010). The neopterin phenotype is likely related to expression of IFNG (cytokine interferon-gamma [IFN-y]), as this cytokine primarily stimulates neopterin production (Huber et al., 1984; Henderson et al., 1991). Additionally, gene expression related to the production of both monocyte or monocyte-derived cells may be related to suPAR and neopterin production. suPAR is cleaved from innate immune cells including monocytes (Estreicher et al., 1990; Koch et al., 2014) and neopterin is synthesized by monocytes and monocyte-derived cells (Huber et al., 1984). Classical (i.e., CD14+) and non-classical (i.e., CD14−) monocytes are the cells most likely involved in the production of neopterin and suPAR (Hearps et al., 2012; Koch et al., 2014).
The free-ranging population of rhesus macaques on Cayo Santiago, Puerto Rico, provide an excellent opportunity to extend our current understanding of immunosenescence and inflammaging beyond humans and laboratory animals. This population lives in a naturalistic environment where they live and die with minimal intervention, form their own social groups and choose their own mates within a genetically diverse population (Rawlins and Kessler, 1986). Cayo Santiago is free of predators, and the rhesus macaques’ diet of wild-growing flora is supplemented by commercial monkey chow and ad libitum water to maintain their population level (Rawlins and Kessler, 1986). Consequently, the Cayo Santiago rhesus macaques live substantially longer than their wild counterparts (Cooper et al., 2022), and the primary causes of adult death on the island are age-related diseases and injury (Kessler et al., 2015). Although average lifespan for rhesus macaques that survive to reproductive maturity (age of 3 years) is 11 years for females and 10 years for males in this population (unpublished data), reproductive senescence does not begin until 18 years of age for the females in the population (Lee et al., 2021), and the maximum recorded age is 31 for females and 29 for males (unpublished data). These demographic conditions on Cayo Santiago provide a unique avenue to investigate changes in the immune system amongst older senescent individuals. Additionally, there is translational value of aging research on rhesus macaques given the phylogenetic and physiological similarities between rhesus macaques and humans (Chiou et al., 2020), which allow us to build upon and draw connections between the substantial human clinical research on neopterin and suPAR, and research on the free-ranging rhesus macaques.
In this study we investigate the suitability of neopterin and suPAR as non-invasive biomarkers of age-related changes in immune system function and inflammation in our study population. We aim to illuminate the age-related patterns of neopterin and suPAR both at the phenotypic and transcriptional level. In order to understand the compounds at the phenotypic level, we use urinary neopterin and suPAR concentrations generated from 354 urine samples from 165 individuals. Here we investigate: (1) associations between neopterin and suPAR concentrations and age; (2) repeatability of neopterin and suPAR within individuals; and the (3) correlation between suPAR and neopterin within individual samples. We then use gene expression data generated from a separate dataset of 505 blood samples from 406 individuals to investigate age-related changes in expression of genes that are either directly or indirectly related to neopterin and suPAR production. This gene expression dataset was produced as part of a separate study on primarily different individuals within the population. While there was an overlap of 48 individuals from whom we have both urine and blood samples, since the blood samples were taken between 2 and 7 years prior to when urine collection began, we are unable to investigate within-individual correlations between urinary phenotypes and gene expression. Using this gene expression dataset, we investigate (4) associations between age and neopterin- and suPAR-related gene expression.
Materials and methods
Study site and subjects
This study focuses on the free-ranging population of rhesus macaques located on Cayo Santiago, a 15.2 hectare island approximately 1 km off the southeastern coast of Puerto Rico. The population is composed of approximately 1,500 individuals that are all the descendants of 409 wild-caught Indian rhesus macaques that were brought to the island for research purposes in 1938. The population is minimally managed. There is minimal medical intervention on the island, monkeys freely roam and form their own social groups (Rawlins and Kessler, 1986), and there is no evidence of inbreeding depression (Widdig et al., 2017).
Urine sample collection
Since we aimed to identify aging phenotypes, we selected study subjects across the entirety of the adult life. Study subjects ranged in age from 3 to 28 years old (female average age = 13.0 years, male average age = 12.4 years). We sampled 165 unique individuals (67 male, 98 female) from two social groups (group V and group F). Between February 2020 and June 2021 we collected 354 samples, with an average of 2.1 ± 1.4 measurements per individual. Since the urinary assay for suPAR required a larger urine volume than neopterin, we were not able to assay suPAR for a subset of the samples. Consequently, the final sample size for suPAR measurements was 301 samples across 160 individuals.
Urine samples were collected by trained field technicians. No samples were collected during a period spanning October to part way through January, as during this period monkeys were being captured for routine measurements under anesthesia, and no other samples could be collected. During sample collection, between two and six technicians collected samples opportunistically from focal individuals 5 days per week, between the hours of 7:00 a.m. and 2:00 p.m. Immediately upon observation of a focal individual urinating, samples were collected from the ground or foliage using a disposable pipette, and then transferred to a vial and put on ice until transfer to a −80°C freezer at 2:30 p.m. Since soil and fecal matter may influence suPAR concentration (Higham et al., 2020), care was taken to exclude any such extraneous material at the time of sample collection by cleaning samples in the field. The cleaning of samples involves allowing any sediment to settle for several minutes in the collected sample, before siphoning off the liquid, insuring the exclusion of any form of debris. We shipped samples on dry ice to New York University to be assayed. Urine samples thawed during shipping but arrived cold with a small amount of dry ice still in the box. We compared concentrations of neopterin and suPAR between the thawed samples and samples which were taken from the same individuals but that did not thaw during transport, and for both neopterin values (t = −3.95, df = 454.41, p-value = 1), and suPAR values (t = 0.92, df = 647.53, p-value = 0.18), thawed samples did not have significantly lower concentrations than unthawed samples.
Urine measurement
We measured urinary neopterin and suPAR using commercial ELISA kits (neopterin: IBL International Cat. No. RE59321; suPAR: RayBiotech Cat. No. ELH-uPAR-1), performed according to the manufacturer’s instructions for each. Both the neopterin kit (Higham et al., 2015), and the suPAR kit (Higham et al., 2020) have been described and validated for use in rhesus macaques previously. Sample duplicates with a coefficient of variation (CV) greater than 15% were rerun. Samples which fell outside of the linear range of the assay were rerun at either a lower or higher dilution value to bring the sample within the linear range. Inter-assay variation, determined by the CVs calculated by high- and low-value quality controls, was 12% (high) and 9% (low) for neopterin, and 15% (high) and 12% (low) for suPAR. Intra-assay CVs were less than 11% for neopterin, and less than 10% for suPAR. We corrected each sample for urine concentration by indexing neopterin and suPAR values to urinary creatinine concentrations, measured using the Jaffe reaction, as described elsewhere (Bahr et al., 2000).
Blood collection, RNA extraction, and sequencing
In order to investigate how gene expression related to the neopterin and suPAR phenotypes changes across age, we used gene expression data collected as part of an ongoing study. The complete dataset has been published and described in detail elsewhere (Watowich et al., 2022). In brief, whole blood was drawn from sedated rhesus macaques by veterinary staff. A 2.5 mL blood sample was collected in a PAXgene Blood RNA Tube (PreAnalytiX GmbH) and stored at room temperature for 2–4 h before being transferred to 4°C for 24 h and finally to −80°C for long-term storage until RNA extraction. We included data from a total of 505 blood samples collected from 406 adults (221 male and 185 female) belonging to 9 different social groups between the years of 2013 to 2018. The ages of these individuals at the time of sampling ranged from 3 to 28 years, with an average age of 8.6 years. A detailed description of RNA extraction and sequencing protocols is provided in Watowich et al. (2022). In short, RNA from 457 samples was extracted using MagMAX for Stabilized Blood Tubes RNA Isolation Kits (Thermo Fisher; Cat. No. 4451893) and 48 samples using the PAXgene Blood RNA Kit IVD (Qiagen, Cat. No. 762164) following the standard protocols for each procedure. RNA sequencing libraries were prepared following the TM3′Seq protocol from Pallares et al. (2020) and sequenced on an Illumina NovaSeq S2 flowcell. cDNA reads were mapped to the Mmul_10 M. mulatta reference assembly.
This research was conducted following ASAB/ABS guidelines for the ethical treatment of animals. All data were collected following protocols approved by the University of Puerto Rico Institutional Animal Care and Use Committee (protocol number: A6850108).
Statistical analysis
We performed all statistical analyses using R software, version 4.2.0 (R Core Team, 2022). Both neopterin and suPAR (measured in ng/mg creatinine) were log-transformed for all analyses in order to satisfy normality assumptions (i.e., so that residual variance was normally distributed). To determine the association between age and urinary neopterin and suPAR, we ran linear mixed models for each biomarker separately. Both models included the same fixed and random effects. We included age (in years), sex, and social group (group F or group V) as fixed effects. We included individual ID as a random effect to control for the non-independence of the repeated measures of individuals. We included the month of collection as a random effect since it has been previously shown for neopterin that urinary markers of immune activation can vary at the population level between months, likely as a consequence of monthly metabolic differences related to the seasonality of reproduction (Petersen et al., 2021). To determine whether there may be any non-linear associations between age and either neopterin or suPAR, we also ran the same models as described above but with the inclusions of second order, and then third order polynomials of the “age” variable. For both neopterin and suPAR the models containing only a first order “age” term had the lowest AIC scores, and so we only present the results of these models.
To quantify the consistency of urinary neopterin and suPAR phenotypes within individuals, we calculated the repeatability (also known as the intra-class correlation coefficient) for each of the two compounds. Repeatability measures the proportion of total variance attributable to between-individual variance, with a higher repeatability indicative of lower phenotypic fluctuation within-individuals and larger differences in mean phenotype between individuals (Nakagawa and Schielzeth, 2010). To calculate repeatability for each compound, we used a simple random-effect regression model which included individual ID as the sole random effect, and then divided the residual variance by the total variance as estimated by this model. Using the rptR package in R, we calculated 95% confidence intervals using 1,000 parametric bootstrapped samples, for both neopterin and suPAR (Nakagawa and Schielzeth, 2010).
To determine if urinary neopterin and suPAR were correlated with each other, we calculated the repeated measures correlation (r) between the two compounds. The repeated measures correlation quantifies the correlation between paired measures (i.e., taken from the same individual urine sample) while simultaneously controlling for the non-independence of repeated sampling within-individuals (Bland and Altman, 1995). For this analysis we z-transformed concentrations (in ng/mg creatinine) of (log-transformed) neopterin and suPAR so that the scales of the measures were comparable. We used the r package rmcorr to calculate the repeated measures correlation and the 95% confidence interval for neopterin and suPAR using 1,000 parametric bootstrapped samples (Bakdash and Marusich, 2017).
We investigated age-related gene expression changes in IFNG (ENSMMUG00000056408), PLAUR (ENSMMUG 00000004616), and PLAU (ENSMMUG00000009906). We tested for differential gene expression across ages by modeling the effects of age and sex on normalized PLAUR gene expression using the R package EMMREML while controlling for effects of RNA quality and relatedness between individuals (Akdemir and Godfrey, 2015). Lastly, we characterized the extent to which monocyte-specific marker genes were associated with age to illuminate how neopterin and suPAR-producing cells may be affected by aging. We identified genes that are preferentially expressed in monocytes (i.e., “marker genes”) from single-cell RNA sequencing of peripheral blood mononuclear cells in rhesus macaques [details in Watowich et al. (2022)]. We then used resampling to test whether CD14+ and CD14− monocytes marker genes exhibited significantly stronger aging effect sizes compared to a random sample of the same number of genes.
Results
Aim 1: Associations between neopterin and suPAR concentrations and age
There was a significant positive relationship between age and urinary neopterin with the model predicting an average increase in neopterin of 38% over 10 years of aging (β = 0.034 ± 0.011; p < 0.01, Figure 1A and Table 1). There was no significant association between suPAR and age (β = 0.029 ± 0.024; p = 0.23, Figure 1B and Table 1). There were significantly higher neopterin concentrations in females than in males (β = −0.21 ± 0.10; p = 0.04, Figure 1A and Table 1), but there was no sex difference in suPAR concentrations (β = −0.15 ± 0.23; p = 0.53, Figure 1B and Table 1). We found no effect of social group on the urinary concentration of either compound (Table 1).
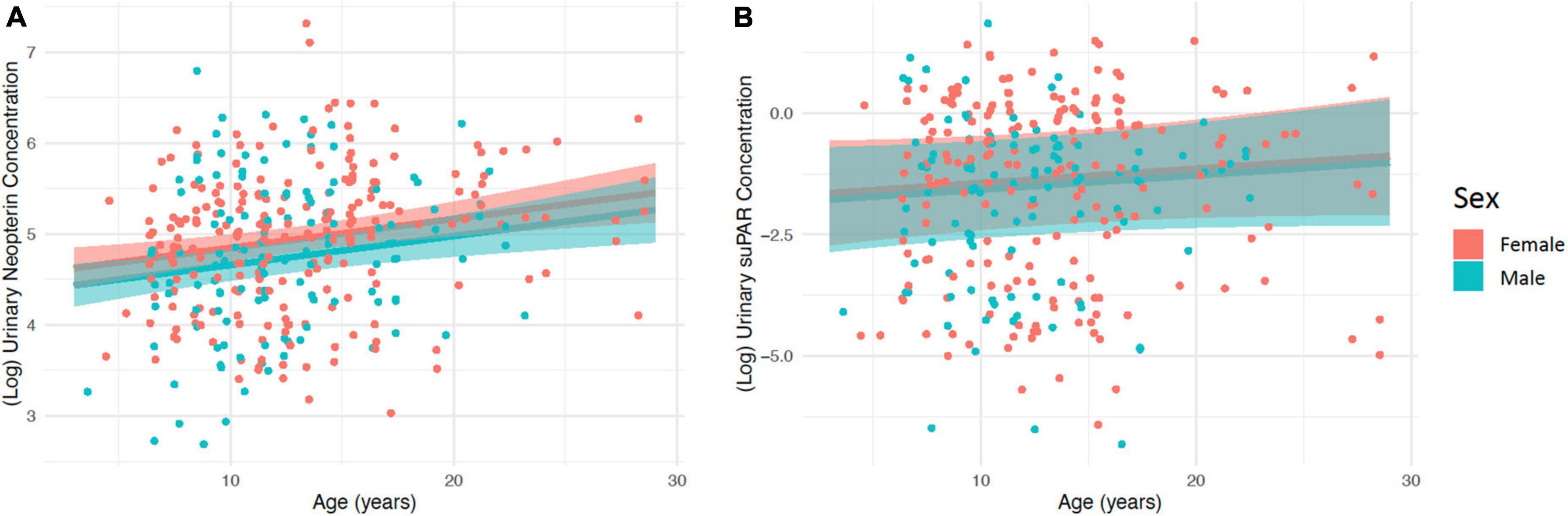
Figure 1. The relationship between age and (A) (log) urinary neopterin concentration (ng/mg creatinine), and (B) (log) urinary suPAR concentration (ng/mg creatinine), for both males and females. Predicted values are shown with 95% confidence intervals. Points represent the raw (log) urinary neopterin or suPAR concentration values.
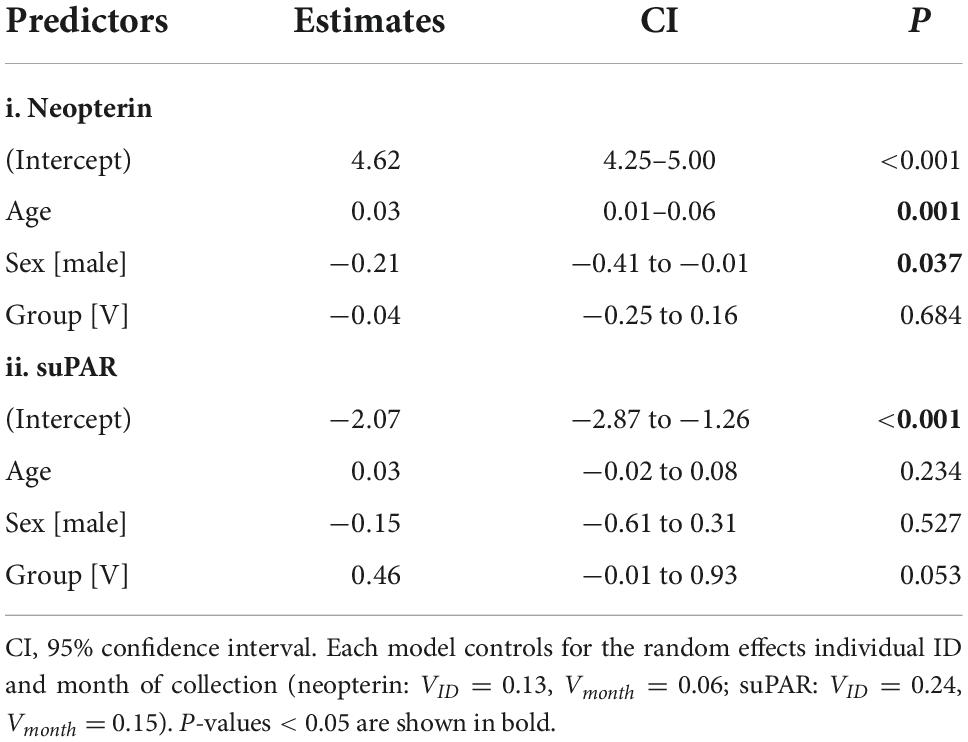
Table 1. Relationships between log-transformed values of urinary (i) neopterin and (ii) suPAR measured in ng/mg creatinine.
Aim 2: Repeatability of neopterin and suPAR within individuals
We calculated repeatability (R) for both neopterin and suPAR in order to quantify the phenotypic consistency of these urinary measures within-individuals, across the 15-month study period. We found moderate repeatability for neopterin (R = 0.27, 95% CI: [0.13–0.41]), and low repeatability for suPAR (R = 0.07, CI: 0–0.21). These results indicate that approximately 27% of the variance in neopterin measures was attributable to between-individual differences, while only approximately 7% of variance in suPAR measures was attributable to between-individual differences.
Aim 3: Correlation between suPAR and neopterin
We calculated the repeated measures correlation (r) between paired samples of neopterin and suPAR to determine the extent to which these compounds are indicative of the same health or environmental conditions within an individual monkey and timepoint. The magnitude of the repeated measures correlation was moderate (r = 0.25) and the bootstrapped confidence intervals indicated this correlation was significant (95% CI: [0.05, 0.43], p < 0.01, Figure 2).
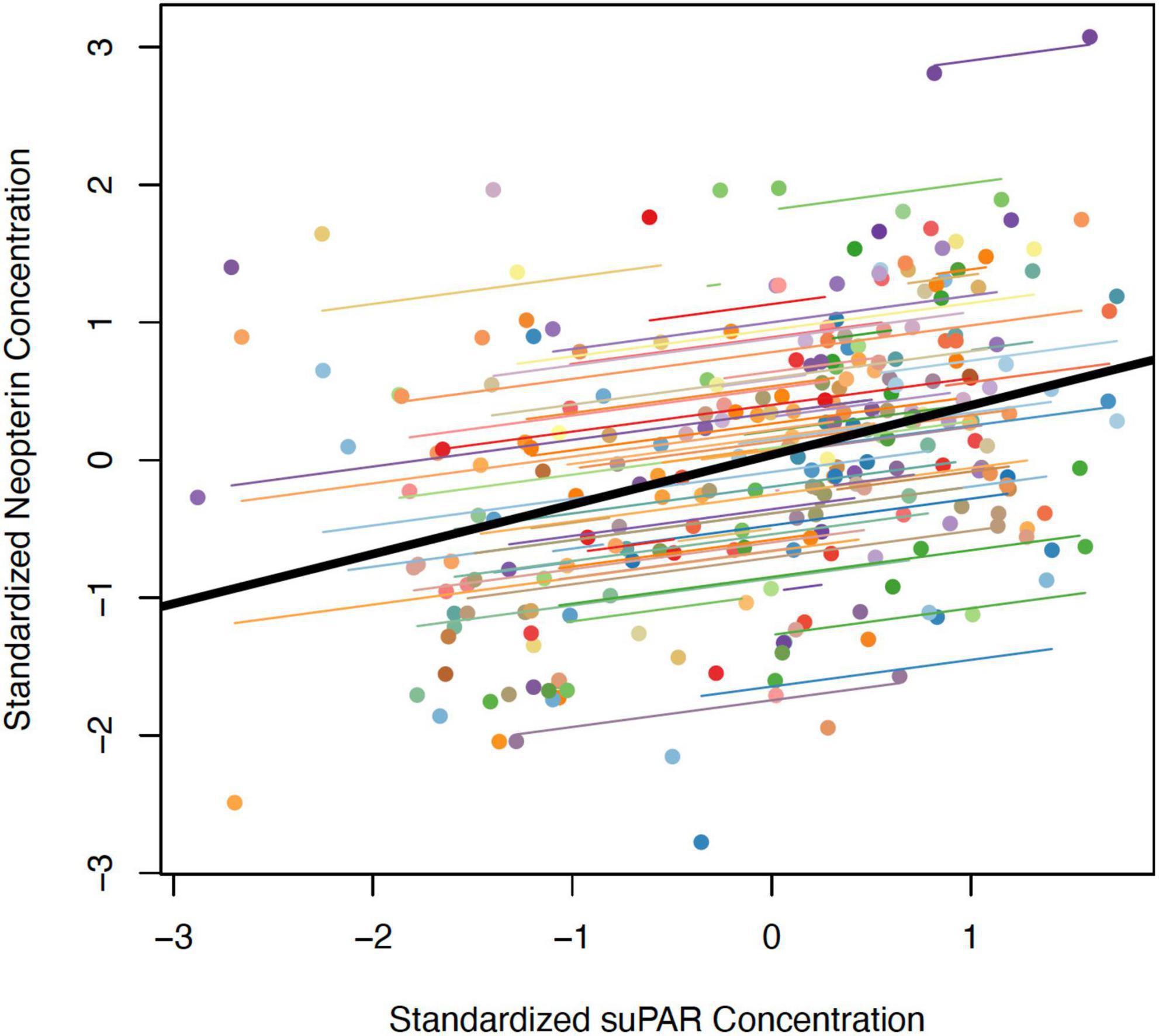
Figure 2. Correlation between z-transformed values of (log) urinary neopterin and suPAR (ng/mg creatinine) measured within the same urine samples. The thick black line represents the overall correlation. Each colored point represents a single urine sample from which both neopterin and suPAR were measured. Colored lines represent individual random slopes (i.e., an individual’s rate of change in neopterin following an increase in suPAR within that individual).
Aim 4: Associations between age and neopterin- and suPAR-related gene expression
Since circulating neopterin is likely to be directly influenced by IFNG and circulating suPAR is likely to be directly influenced by PLAUR and PLAU, we tested if each of these three genes were differentially expressed across age. Contrary to expectations, none of these three genes were significantly associated with age (IFNG: β = 0.013, p = 0.20; PLAUR: β = −0.004, p = 0.87; PLAU β = 0.022, p = 0.32). However, it is important to note that there were a substantial number of samples where these genes were not detectably expressed (i.e., there were no RNA-seq reads that mapped to the gene), indicating the genes were very lowly expressed. There were only 20 non-zeros for IFNG (4% of sample), 285 non-zeros for PLAUR (52% of sample), and 208 non-zeros for PLAU (38% of sample). This low level of expression likely reduced our power to detect a statistically significant association, especially in the case of the IFNG gene. Further, we tested whether genes that were markers of CD14+ and CD14− monocytes were more likely to be associated with age than expected by chance. We found that the median effect size of marker genes of both monocyte types were significantly more likely to be positively associated with age (CD14+: β = 2.85, p = 0; CD14−: β = 2.48, p = 0; Figure 3).
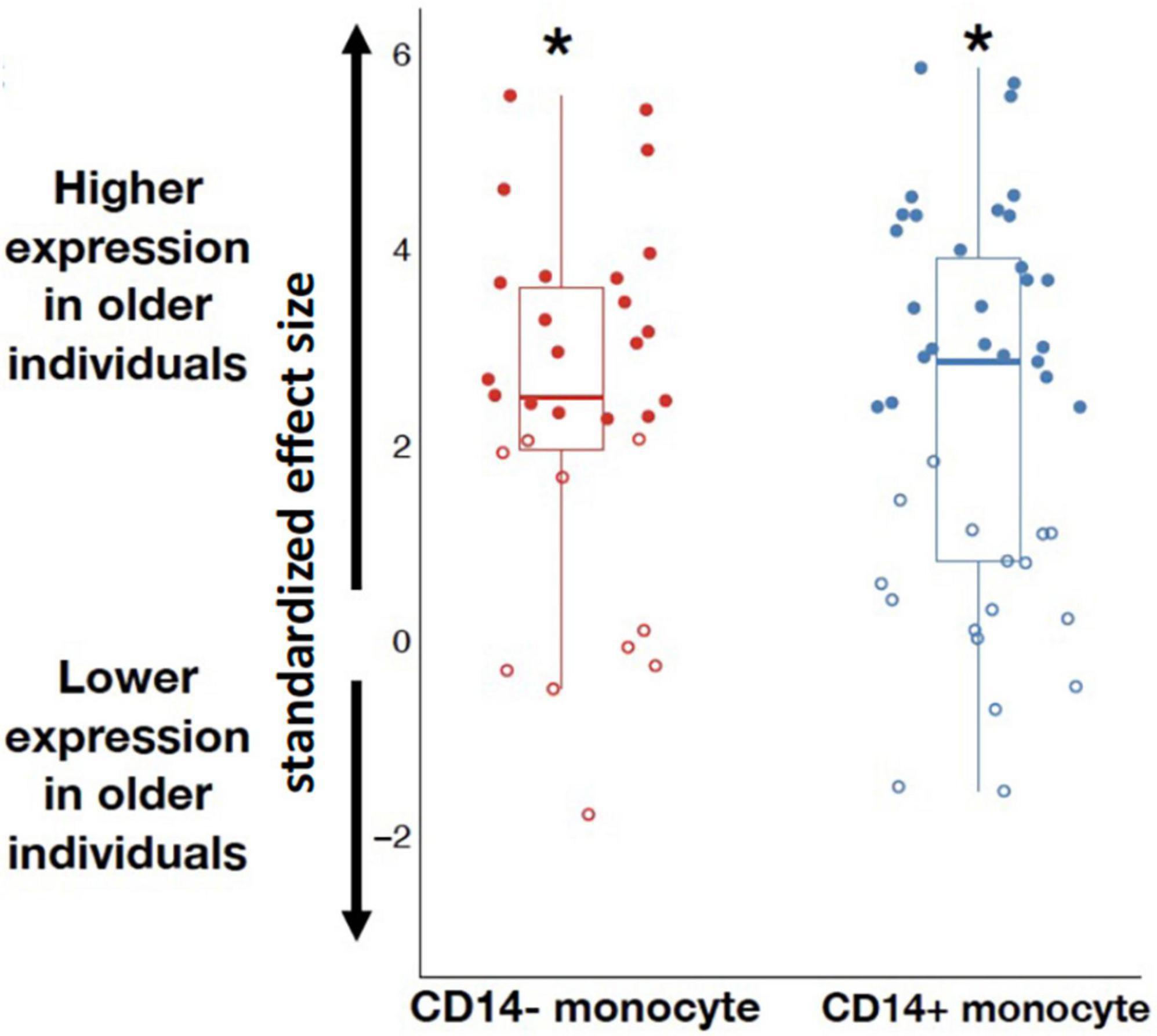
Figure 3. Marker genes for CD14– and CD14+ monocytes were more highly expressed among older individuals compared to younger individuals (p = 0; as indicated by the * symbol). Marker genes significantly associated with aging are fully colored, marker genes not significantly associated with aging at a 10% FDR are unfilled.
Discussion
In the present study, we aimed to investigate the suitability of two urinary compounds, neopterin and suPAR, as biomarkers of age-related changes in immune system function and inflammation in a free-ranging primate population. We found that while urinary neopterin and suPAR are moderately correlated with one another, only neopterin had a significant association with age. Neopterin had moderate repeatability within-individuals, and suPAR had lower repeatability, indicating that both are moderately to highly plastic within individuals. The investigation into age-related gene expression showed that while the expression of genes related to the signaling or activation of neopterin and suPAR did not change significantly with age, marker genes of two types of monocytes which produce neopterin and suPAR, CD14+ and CD14− monocytes, were more highly expressed in older individuals.
The results of this study indicate that urinary neopterin is a viable non-invasive biomarker of age-related changes in immune activation and function, as there was a strong correlation between neopterin and age. The increase in neopterin with age is indicative of higher levels of innate immune activity in these older individuals (Gieseg et al., 1995; Hoffmann et al., 1996; Schobersberger et al., 1996; Hamerlinck, 1999). This heightened immune activity in older age groups could be a consequence of many different factors. The age-related increase in urinary neopterin may be directly related to the phenomenon of immunosenescence, such that declines in some aspects of immune system function with age results in increasing susceptibility to degenerative diseases and infections, causing age-related differences in neopterin. Alternatively, but not mutually-exclusively, the association between neopterin and age may be driven by senescent processes indirectly related to the immune system, such as physical declines with age that increase the risk of injury and infection. It’s also possible that non-senescent processes that are simply associated with increased age drive the association between neopterin and age. For example, social behavioral shifts with age may influence an individual’s risk of communicable disease transmission (Siracusa et al., 2022), consequently causing an increase in neopterin with age. Since the present study only covered a period of 17 months and thus only tracks within-individual changes in neopterin over a relatively short time period compared to the full adult lifespan of rhesus macaques, it is also possible that the association between neopterin and age is a consequence of cohort-level differences in neopterin concentrations, rather than within-individual changes over age. However, given that a positive association between neopterin and age has been shown in other non-human primate populations (Müller et al., 2017; González et al., 2020; Negrey et al., 2021), as well as extensively in human populations (Reibnegger et al., 1988; Diamondstone et al., 1994; Schennach et al., 2002; Wakabayashi et al., 2020), the most parsimonious conclusion is that the association between neopterin and age is a consequence of within-individual senescent, or otherwise age-related, processes, rather than a unique cohort effect within the Cayo Santiago rhesus macaques.
The age-related increase of urinary neopterin in the Cayo Santiago rhesus macaques population over adulthood is comparable in magnitude to what has been found in other primate populations. The vast majority of neopterin research has been on humans. In humans, the rate of neopterin change with age, while significant, is typically small, with only a 20% increase in (log) neopterin reported over a cross-sectional 49 year period of adulthood in one study (Diamondstone et al., 1994). In free ranging Barbary macaques (log) neopterin increased by 4% over a comparable period of adult lifespan of 10 years in their cross-sectional sample (Müller et al., 2017). In our study, age-related differences are such that (log) neopterin is 6.6% higher per 10 years of adulthood (which reflects a 38% change in absolute neopterin concentrations). The similarities between these findings suggest that the physiological, physical, or behavioral factors influencing neopterin concentration differences across age may also be comparable and conserved across the primate order.
suPAR concentration was not significantly correlated with age in our study. While many human studies have found a positive correlation between suPAR and age (Haastrup et al., 2014; Rasmussen et al., 2016; Haupt et al., 2019), some studies have not found a significant correlation (Wittenhagen et al., 2004; Koch et al., 2011). It is not entirely clear what variables are determining why some studies have found a significant correlation between suPAR and age while others have not, although it is notable that studies with sample sizes in the hundreds typically do not find a significant correlation between suPAR and age, while studies with sample sizes in the thousands have found a significantly positive correlation. Our findings in this study, coupled with the considerably large number of subjects that are apparently needed in order to detect a correlation between suPAR and age in the human literature, suggest that urinary neopterin is likely a more informative biomarker of age-related changes in immune function than suPAR. However, suPAR still holds promise as a broad biomarker of inflammation, as it is commonly used in humans already (Thunø et al., 2009; Rasmussen et al., 2016), and has been validated to effectively indicate inflammation in rhesus macaques (Higham et al., 2020).
Similar to patterns observed in humans (Nyamweya et al., 2012; Tahar et al., 2016), we found that neopterin and suPAR measures were significantly correlated within-individuals within-timepoints in the rhesus macaques. This is unsurprising given that neopterin and suPAR are broad markers of changes in immune system activation and inflammation, and are thus likely to respond similarly to a range of stimuli. However, the magnitude of the correlation between neopterin and suPAR was only 25%, indicating that although neopterin and suPAR respond similarly to some environmental or physiological triggers, there are clearly many instances where they do not. The results of this study demonstrate that aging is one variable to which suPAR and neopterin do not respond in the same way. There is currently a dearth of empirical research on how different specific types of immune activation might influence neopterin and suPAR separately [but see Higham et al. (2020)]. A better understanding of the physiological and environmental drivers of these compounds individually will provide opportunities to use their measurement in tandem to illuminate and disentangle which physiological and environmental triggers an individual has been exposed to.
The repeatability of neopterin or suPAR is a measure of how consistent concentrations of the compound are on average within individuals across repeated measures. These repeated measures in this study were taken at different timepoints, typically several months apart. suPAR had a low repeatability of 7%, indicating that within-individuals suPAR concentrations are quite stochastic, and likely spike acutely and frequently to environmental stimuli. The repeatability of neopterin was 27%, indicating that neopterin levels are considerably plastic within-individuals, and may respond regularly to environmental stimuli with acute spikes, before returning to a relatively consistent within-individual baseline. For neopterin, the 95% confidence intervals of the repeatability estimate spanned a range from 13 to 41%, indicating that we have relatively low precision in this estimate, and the true estimate of repeatability may be substantially lower or higher than 27%. The repeatability estimate for suPAR also had a wide confidence interval, with the estimated repeatability falling between 0 and 21%, suggesting a range from moderately low to low repeatability. The low repeatability of suPAR may be indicative of why it is not a good biomarker of age-related changes. This is because the more stochastic variance there is in any biomarker, the more statistical power is needed to identify a relationship between that biomarker and a continuous within-individual physiological state, such as aging. Indeed, in our models the magnitude of the effect of age on neopterin (β = 0.034 ± 0.011) is approximately the same as the magnitude of the effect of age on suPAR (β = 0.029 ± 0.024). It is only a consequence of the larger standard error and resulting 95% confidence interval in the suPAR model that the age effect is non-significant. Thus, it is possible that with a larger sample size the precision of the model could improve and a positive association between suPAR and age may be revealed.
The gene expression analysis indicated that while genes that directly signal neopterin or form into suPAR did not differ across age, there was an increase in markers of monocyte expression, a primary producer of both compounds. The lack of age-related change in the two genes which encode the proteins which bind to form suPAR, the PLAUR and PLAU genes, is unsurprising given the lack of age-related change in urinary suPAR concentrations. We observed that expression of the gene that encodes the cytokine which primarily signals neopterin production, IFNG, trended toward increasing across aging, but did not reach significance, perhaps unsurprisingly as we only detected IFNG in 20 samples. However, this lack of association over aging could also indicate that the biochemical pathway driving increased neopterin with age is not an increase in IFN-y. It is also possible that IFN-y receptors become more sensitized to signaling neopterin with increasing age. Lastly, since both CD14+ and CD14− monocyte markers increase in expression with age, it may be that the increased availability of these types of cells which produce neopterin are the biochemical drivers of increasing neopterin with age.
To our knowledge, this is the first study to measure both urinary neopterin and suPAR simultaneously in a free-ranging animal population, as well as the first study to investigate the transcriptomic changes that may be driving any age-related change in neopterin or suPAR. Our findings clearly indicate that neopterin collected non-invasively through urine is a viable method for measuring age-related changes in immune system functioning. We found that these changes are not driven by an age-related increase in expression of the primary signaler of neopterin, IFN-y, but may be driven by an increase in both CD14+ and CD14− monocytes instead. suPAR was not correlated with age and had low repeatability within-individuals, indicating that it is likely better suited to measuring acute inflammation rather than chronic inflammaging. The continued sampling within-individuals across their entire adulthoods, as well as time-matched phenotypic and genomic date within-individuals, will provide opportunities to quantify variation of within-individual aging patterns. Further study is needed to elucidate the proximate and ultimate drivers of neopterin and suPAR beyond the effect of aging. Consideration of demographic and behavioral variables including reproduction, injury, social status, social connection, parturition, and death will all provide valuable avenues for understanding both the causes and consequences of elevated neopterin or suPAR. A more thorough understanding of the factors driving the concentrations of neopterin and suPAR within individuals will further open up opportunities to use these compounds to measure health, aging, and eco-evolutionary dynamics in free-ranging and wild populations non-invasively.
Data availability statement
The datasets presented in this study can be found in online repositories. The names of the repository/repositories and accession number(s) can be found below: BioProject, PRJNA715739.
Ethics statement
This animal study was reviewed and approved by University of Puerto Rico, Institutional Animal Care and Use Committee (IACUC).
Author contributions
JPH, NS-M, LB, MM, and EC conceived the ideas and designed the study and its methods. EC, NB, and CW conducted urinary immuno-assays. MW conducted RNA sequencing. EC and MW conducted statistical analysis and produced the figures. EC wrote the first draft of the manuscript. All authors contributed to the article and approved the submitted version.
Funding
This work was supported by the following grants from the National Institute of Health: grant nos. R01-AG060931 and R00-AG051764. Blood collection was supported by NIH grant R01-MH096875. The CPRC was supported by an Animal and Biological Material Resource Center Grant (P40OD012217) which was awarded to UPR from the Office of Research Infrastructure Programs (ORIP) and a Research Facilities Construction Grant (C06OD026690) was awarded for the renovation of CPRC facilities after Hurricane Maria. Infrastructure repair necessary for this research was also funded by NSF BCS-1800558 to JPH and Susan Antón.
Acknowledgments
We thank the many field technicians that helped with collection of urine and blood samples and the staff of the Caribbean Primate Research Center for access to the study population and assistance in blood sampling. We also thank Daniel Blumstein and two reviewers whose comments on a previous draft greatly improved the manuscript.
Conflict of interest
The authors declare that the research was conducted in the absence of any commercial or financial relationships that could be construed as a potential conflict of interest.
Publisher’s note
All claims expressed in this article are solely those of the authors and do not necessarily represent those of their affiliated organizations, or those of the publisher, the editors and the reviewers. Any product that may be evaluated in this article, or claim that may be made by its manufacturer, is not guaranteed or endorsed by the publisher.
References
Akdemir, D., and Godfrey, O. U. (2015). EMMREML: Fitting mixed models with known covariance structures (3.1) [R Package]. Available Online at: https://cran.r-project.org/package=EMMREML (accessed April 2022).
Andersen, O., Eugen-Olsen, J., Kofoed, K., Iversen, J., and Haugaard, S. B. (2008a). Soluble urokinase plasminogen activator receptor is a marker of dysmetabolism in HIV-infected patients receiving highly active antiretroviral therapy. J. Med. Virol. 80, 209–216. doi: 10.1002/jmv.21114
Andersen, O., Eugen-Olsen, J., Kofoed, K., Iversen, J., and Haugaard, S. B. (2008b). SuPAR associates to glucose metabolic aberration during glucose stimulation in HIV-infected patients on HAART. J. Infect. 57, 55–63. doi: 10.1016/j.jinf.2008.01.014
Auzeby, A., Bogdan, A., Krosi, Z., and Touitou, Y. (1989). Large-amplitude circadian variations of urinary neopterin in healthy man. Pteridines 1, 17–18. doi: 10.1515/pteridines.1989.1.1.17
Bahr, N. I., Palme, R., Möhle, U., Hodges, J. K., and Heistermann, M. (2000). Comparative aspects of the metabolism and excretion of cortisol in three individual nonhuman primates. Gen. Comp. Endocrinol. 117, 427–438. doi: 10.1006/gcen.1999.7431
Bakdash, J. Z., and Marusich, L. R. (2017). Repeated measures correlation. Front. Psychol. 8:456. doi: 10.3389/fpsyg.2017.00456
Baylis, D., Bartlett, D. B., Patel, H. P., and Roberts, H. C. (2013). Understanding how we age: Insights into inflammaging. Longev. Healthspan 2:8. doi: 10.1186/2046-2395-2-8
Behringer, V., Deimel, C., Stevens, J. M. G., Kreyer, M., Lee, S. M., Hohmann, G., et al. (2021). Cell-mediated immune ontogeny is affected by sex but not environmental context in a long-lived primate species. Front. Ecol. Evol. 9:629094. doi: 10.3389/FEVO.2021.629094
Behringer, V., Stevens, J. M. G., Wittig, R. M., Crockford, C., Zuberbuler, K., Leendertz, F. H., et al. (2019). Elevated neopterin levels in wild, healthy chimpanzees indicate constant investment in unspecific immune system. BMC Zool. 4:2. doi: 10.1186/s40850-019-0041-1
Bland, J. M., and Altman, D. G. (1995). Calculating correlation coefficients with repeated observations: Part 1–Correlation within subjects. BMJ 310:446. doi: 10.1136/bmj.310.6977.446
Børglum, A. D., Byskov, A., Ragno, P., Roldan, A. L., Tripputi, P., Cassani, G., et al. (1992). Assignment of the urokinase-type plasminogen activator receptor gene (PLAUR) to chromosome 19q13.1-q13.2. Am. J. Hum. Genet. 50, 492–497.
Brown, A. E., Webster, H. K., Teja-Isavadharm, P., and Keeratithakul, D. (1990). Macrophage activation in falciparum malaria as measured by neopterin and interferon-gamma. Clin. Exp. Immunol. 82, 97–101. doi: 10.1111/j.1365-2249.1990.tb05410.x
Capuron, L., Schroecksnadel, S., Féart, C., Aubert, A., Higueret, D., Barberger-Gateau, P., et al. (2011). Chronic low-grade inflammation in elderly persons is associated with altered tryptophan and tyrosine metabolism: Role in neuropsychiatric symptoms. Biol. Psychiatry 70, 175–182. doi: 10.1016/j.biopsych.2010.12.006
Chiou, K. L., Montague, M. J., Goldman, E. A., Watowich, M. M., Sams, S. N., Song, J., et al. (2020). Rhesus macaques as a tractable physiological model of human ageing. Philos. Trans. R. Soc. Lond., B, Biol. Sci. 375:20190612. doi: 10.1098/rstb.2019.0612
Connolly, B. M., Choi, E. Y., Gårdsvoll, H., Bey, A. L., Currie, B. M., Chavakis, T., et al. (2010). Selective abrogation of the uPA-uPAR interaction in vivo reveals a novel role in suppression of fibrin-associated inflammation. Blood 116, 1593–1603. doi: 10.1182/blood-2010-03-276642
Cooper, E. B., Brent, L. J. N., Snyder-Mackler, N., Singh, M., Sengupta, A., Khatiwada, K., et al. (2022). The natural history of model organisms: Rhesus macaques as an Anthropocene success story. eLife 11:e78169. doi: 10.7554/eLife.78169
Cristóbal-Azkarate, J., Maréchal, L., Semple, S., Majolo, B., and MacLarnon, A. (2016). Metabolic strategies in wild male Barbary macaques: Evidence from faecal measurement of thyroid hormone. Biol. Lett. 12:20160168. doi: 10.1098/rsbl.2016.0168
de Magalhães, J. P., Curado, J., and Church, G. M. (2009). Meta-analysis of age-related gene expression profiles identifies common signatures of aging. Bioinformatics 25, 875–881. doi: 10.1093/bioinformatics/btp073
Deschner, T., Fuller, B. T., Oelze, V. M., Boesch, C., Hublin, J.-J., Mundry, R., et al. (2012). Identification of energy consumption and nutritional stress by isotopic and elemental analysis of urine in bonobos (Pan paniscus). Rapid Commun. Mass Spectrome. 26, 69–77. doi: 10.1002/rcm.5312
Diamondstone, L. S., Tollerud, D. J., Fuchs, D., Wachter, H., Brown, L. M., Maloney, E., et al. (1994). Factors influencing serum neopterin and β2-microglobulin levels in a healthy diverse population. J. Clin. Immunol. 14, 368–374. doi: 10.1007/BF01546321
Dibakou, S. E., Souza, A., Boundenga, L., Givalois, L., Mercier-Delarue, S., Simon, F., et al. (2020). Ecological, parasitological and individual determinants of plasma neopterin levels in a natural mandrill population. Int. J. Parasitol. Parasites Wildl. 11, 198–206. doi: 10.1016/j.ijppaw.2020.02.009
Eisenhut, M. (2013). Neopterin in diagnosis and monitoring of infectious diseases. J. Biomark. 2013:196432. doi: 10.1155/2013/196432
Emery Thompson, M., and Knott, C. D. (2008). Urinary C-peptide of insulin as a non-invasive marker of energy balance in wild orangutans. Horm. Behav. 53, 526–535. doi: 10.1016/j.yhbeh.2007.12.005
Estreicher, A., Mühlhauser, J., Carpentier, J. L., Orci, L., and Vassalli, J. D. (1990). The receptor for urokinase type plasminogen activator polarizes expression of the protease to the leading edge of migrating monocytes and promotes degradation of enzyme inhibitor complexes. J. Cell Biol. 111, 783–792. doi: 10.1083/jcb.111.2.783
Ezenwa, V. O., Etienne, R. S., Luikart, G., Beja-Pereira, A., and Jolles, A. E. (2010). Hidden consequences of living in a wormy world: Nematode-induced immune suppression facilitates tuberculosis invasion in African buffalo. Am. Nat. 176, 613–624. doi: 10.1086/656496
Franceschi, C., Bonafè, M., Valensin, S., Olivieri, F., De Luca, M., Ottaviani, E., et al. (2000). Inflamm-aging. An evolutionary perspective on immunosenescence. Ann. N. Y. Acad. Sci. 908, 244–254. doi: 10.1111/j.1749-6632.2000.tb06651.x
Franceschi, C., Capri, M., Monti, D., Giunta, S., Olivieri, F., Sevini, F., et al. (2007). Inflammaging and anti-inflammaging: A systemic perspective on aging and longevity emerged from studies in humans. Mech. Ageing Dev. 128, 92–105. doi: 10.1016/j.mad.2006.11.016
Franceschi, C., Garagnani, P., Parini, P., Giuliani, C., and Santoro, A. (2018). Inflammaging: A new immune–metabolic viewpoint for age-related diseases. Nat. Rev. Endocrinol. 14, 576–590. doi: 10.1038/s41574-018-0059-4
Frasca, D., and Blomberg, B. B. (2016). Inflammaging decreases adaptive and innate immune responses in mice and humans. Biogerontology 17, 7–19. doi: 10.1007/s10522-015-9578-8
Fuchs, D., Hausen, A., Reibnegger, G., Werner, E. R., Dierich, M. P., and Wachter, H. (1988). Neopterin as a marker for activated cell-mediated immunity: Application in HIV infection. Immunol. Today 9, 150–155. doi: 10.1016/0167-5699(88)91203-0
Fuchs, D., Stahl-Hennig, C., Gruber, A., Murr, C., Hunsmann, G., and Wachter, H. (1994). Neopterin—Its clinical use in urinalysis. Kidney Int. Suppl. 47, S8–S11.
Fulop, T., Witkowski, J. M., Olivieri, F., and Larbi, A. (2018). The integration of inflammaging in age-related diseases. Semin. Immunol. 40, 17–35. doi: 10.1016/j.smim.2018.09.003
Gieseg, S. P., Reibnegger, G., Wachter, H., and Esterbauer, H. (1995). 7,8 Dihydroneopterin inhibits low density lipoprotein oxidation in vitro. Evidence that this macrophage secreted pteridine is an anti-oxidant. Free Radic. Res. 23, 123–136. doi: 10.3109/10715769509064027
Gillespie, T. R., and Chapman, C. A. (2006). Prediction of parasite infection dynamics in primate metapopulations based on attributes of forest fragmentation. Conserv. Biol. 20, 441–448. doi: 10.1111/j.1523-1739.2006.00290.x
Gobush, K. S., Booth, R. K., and Wasser, S. K. (2014). Validation and application of noninvasive glucocorticoid and thyroid hormone measures in free-ranging Hawaiian monk seals. Gen. Comp. Endocrinol. 195, 174–182. doi: 10.1016/j.ygcen.2013.10.020
González, N. T., Otali, E., Machanda, Z., Muller, M. N., Wrangham, R., and Thompson, M. E. (2020). Urinary markers of oxidative stress respond to infection and late-life in wild chimpanzees. PLoS One 15:e0238066. doi: 10.1371/journal.pone.0238066
Gruver, A., Hudson, L., and Sempowski, G. (2007). Immunosenescence of ageing. J. Pathol. 211, 144–156. doi: 10.1002/path.2104
Haastrup, E., Grau, K., Eugen-Olsen, J., Thorball, C., Kessing, L. V., and Ullum, H. (2014). Soluble urokinase plasminogen activator receptor as a marker for use of antidepressants. PLoS One 9:e110555. doi: 10.1371/journal.pone.0110555
Hamerlinck, F. F. V. (1999). Neopterin: A review. Exp. Dermatol. 8, 167–176. doi: 10.1111/j.1600-0625.1999.tb00367.x
Haupt, T. H., Rasmussen, L. J. H., Kallemose, T., Ladelund, S., Andersen, O., Pisinger, C., et al. (2019). Healthy lifestyles reduce suPAR and mortality in a Danish general population study. Immun. Ageing 16:1. doi: 10.1186/s12979-018-0141-8
Hearps, A. C., Martin, G. E., Angelovich, T. A., Cheng, W.-J., Maisa, A., Landay, A. L., et al. (2012). Aging is associated with chronic innate immune activation and dysregulation of monocyte phenotype and function. Aging Cell 11, 867–875. doi: 10.1111/j.1474-9726.2012.00851.x
Heistermann, M., and Higham, J. P. (2015). Urinary neopterin, a non-invasive marker of mammalian cellular immune activation, is highly stable under field conditions. Sci. Rep. 5:16308. doi: 10.1038/srep16308
Henderson, D. C., Sheldon, J., Riches, P., and Hobbs, J. R. (1991). Cytokine induction of neopterin production. Clin. Exp. Immunol. 83, 479–482. doi: 10.1111/j.1365-2249.1991.tb05664.x
Herrero, C., Sebastián, C., Marqués, L., Comalada, M., Xaus, J., Valledor, A. F., et al. (2002). Immunosenescence of macrophages: Reduced MHC class II gene expression. Exp. Gerontol. 37, 389–394. doi: 10.1016/S0531-5565(01)00205-4
Higham, J. P., Heistermann, M., and Maestripieri, D. (2011). The energetics of male–male endurance rivalry in free-ranging rhesus macaques, Macaca mulatta. Anim. Behav. 81, 1001–1007. doi: 10.1016/j.anbehav.2011.02.001
Higham, J. P., Kraus, C., Stahl-Hennig, C., Engelhardt, A., Fuchs, D., and Heistermann, M. (2015). Evaluating noninvasive markers of nonhuman primate immune activation and inflammation. Am. J. Phys. Anthropol. 158, 673–684. doi: 10.1002/ajpa.22821
Higham, J. P., Stahl-Hennig, C., and Heistermann, M. (2020). Urinary suPAR: A non-invasive biomarker of infection and tissue inflammation for use in studies of large free-ranging mammals. R. Soc. Open Sci. 7:191825. doi: 10.1098/rsos.191825
Hoffman, C. L., Higham, J. P., Heistermann, M., Coe, C. L., Prendergast, B. J., and Maestripieri, D. (2011). Immune function and HPA axis activity in free-ranging rhesus macaques. Physiol. Behav. 104, 507–514. doi: 10.1016/j.physbeh.2011.05.021
Hoffmann, G., Schobersberger, W., Frede, S., Pelzer, L., Fandrey, J., Wachter, H., et al. (1996). Neopterin activates transcription factor nuclear factor-kappa B in vascular smooth muscle cells. FEBS Lett. 391, 181–184. doi: 10.1016/0014-5793(96)00729-6
Huber, C., Batchelor, J. R., Fuchs, D., Hausen, A., Lang, A., Niederwieser, D., et al. (1984). Immune response-associated production of neopterin. Release from macrophages primarily under control of interferon-gamma. J. Exp. Med. 160, 310–316. doi: 10.1084/jem.160.1.310
Jaimez, N. A., Bribiescas, R. G., Aronsen, G. P., Anestis, S. A., and Watts, D. P. (2012). Urinary cortisol levels of gray-cheeked mangabeys are higher in disturbed compared to undisturbed forest areas in Kibale National Park, Uganda. Anim. Conserv. 15, 242–247. doi: 10.1111/j.1469-1795.2011.00508.x
Kessler, M. J., Hernández Pacheco, R., Rawlins, R. G., Ruiz-Lambides, A. V., Delgado, D. L., and Sabat, A. M. (2015). Long-term effects of tetanus toxoid inoculation on the demography and life expectancy of the Cayo Santiago rhesus macaques. Am. J. Primatol. 77, 211–221. doi: 10.1002/ajp.22323
Koch, A., Voigt, S., Kruschinski, C., Sanson, E., Dückers, H., Horn, A., et al. (2011). Circulating soluble urokinase plasminogen activator receptor is stably elevated during the first week of treatment in the intensive care unit and predicts mortality in critically ill patients. Crit. Care 15:R63. doi: 10.1186/cc10037
Koch, A., Zimmermann, H. W., Gassler, N., Jochum, C., Weiskirchen, R., Bruensing, J., et al. (2014). Clinical relevance and cellular source of elevated soluble urokinase plasminogen activator receptor (suPAR) in acute liver failure. Liver Int. 34, 1330–1339. doi: 10.1111/liv.12512
Lee, D. S., Kang, Y. H. R., Ruiz-Lambides, A. V., and Higham, J. P. (2021). The observed pattern and hidden process of female reproductive trajectories across the life span in a non-human primate. J. Anim. Ecol. 90, 2901–2914. doi: 10.1111/1365-2656.13590
Liu, D., Li, Z., Hou, Z., Bao, H., Luan, X., Zhang, P., et al. (2022). Ecological relationships among habitat type, food nutrients, parasites and hormones in wild boar Sus scrofa during winter. Wildl. Biol. 2022:e01020. doi: 10.1002/wlb3.01020
Liu, K. L., Fan, J. H., and Wu, J. (2017). Prognostic role of circulating soluble uPAR in various cancers: A systematic review and meta-analysis. Clin. Lab. 63, 871–880. doi: 10.7754/clin.lab.2017.170110
Min, K.-J., and Tatar, M. (2018). Unraveling the molecular mechanism of immunosenescence in Drosophila. Int. J. Mol. Sci. 19:2472. doi: 10.3390/ijms19092472
Mohlman, J. L., Navara, K. J., Sheriff, M. J., Terhune, T. M., and Martin, J. A. (2020). Validation of a noninvasive technique to quantify stress in northern bobwhite (Colinus virginianus). Conserv. Physiol. 8:coaa026. doi: 10.1093/conphys/coaa026
Müller, N., Heistermann, M., Strube, C., Schülke, O., and Ostner, J. (2017). Age, but not anthelmintic treatment, is associated with urinary neopterin levels in semi-free ranging Barbary macaques. Sci. Rep. 7:41973. doi: 10.1038/srep41973
Nakagawa, S., and Schielzeth, H. (2010). Repeatability for Gaussian and non-Gaussian data: A practical guide for biologists. Biol. Rev. 85, 935–956. doi: 10.1111/j.1469-185X.2010.00141.x
Nalubamba, K. S., Mudenda, N. B., and Malamo, M. R. (2012). A seasonal survey of gastrointestinal parasites in captive wild impala antelope on a game facility south of Lusaka, Zambia. J. Helminthol. 86, 418–425. doi: 10.1017/S0022149X11000617
Negrey, J. D., Behringer, V., Langergraber, K. E., and Deschner, T. (2021). Urinary neopterin of wild chimpanzees indicates that cell-mediated immune activity varies by age, sex, and female reproductive status. Sci. Rep. 11:9298. doi: 10.1038/s41598-021-88401-6
Nyamweya, S., Townend, J., Zaman, A., Steele, S. J., Jeffries, D., Rowland-Jones, S., et al. (2012). Are plasma biomarkers of immune activation predictive of HIV progression: A longitudinal comparison and analyses in HIV-1 and HIV-2 infections? PLoS One 7:e44411. doi: 10.1371/journal.pone.0044411
Ostrowski, S., Williams, J. B., Mésochina, P., and Sauerwein, H. (2006). Physiological acclimation of a desert antelope, Arabian oryx (Oryx leucoryx), to long-term food and water restriction. J. Comp. Physiol. B 176, 191–201. doi: 10.1007/s00360-005-0040-0
Ostrowski, S. R., Ullum, H., Goka, B. Q., Høyer-Hansen, G., Obeng-Adjei, G., Pedersen, B. K., et al. (2005). Plasma concentrations of soluble urokinase-type plasminogen activator receptor are increased in patients with malaria and are associated with a poor clinical or a fatal outcome. J. Infect. Dis. 191, 1331–1341. doi: 10.1086/428854
Pallares, L. F., Picard, S., and Ayroles, J. F. (2020). TM3’seq: A tagmentation-mediated 3’ sequencing approach for improving scalability of RNAseq experiments. G3 10, 143–150. doi: 10.1534/g3.119.400821
Peters, A., Delhey, K., Nakagawa, S., Aulsebrook, A., and Verhulst, S. (2019). Immunosenescence in wild animals: Meta-analysis and outlook. Ecol. Lett. 22, 1709–1722. doi: 10.1111/ele.13343
Petersen, R. M., Heistermann, M., and Higham, J. P. (2021). Social and sexual behaviors predict immune system activation, but not adrenocortical activation, in male rhesus macaques. Behav. Ecol. Sociobiol. 75:159. doi: 10.1007/s00265-021-03083-4
Pliyev, B. K., and Menshikov, M. Y. (2010). Release of the soluble urokinase-type plasminogen activator receptor (suPAR) by activated neutrophils in rheumatoid arthritis. Inflammation 33, 1–9. doi: 10.1007/s10753-009-9152-0
Rasmussen, L. J. H., Caspi, A., Ambler, A., Danese, A., Elliott, M., Eugen-Olsen, J., et al. (2020). Association between elevated suPAR, a new biomarker of inflammation, and accelerated aging. J. Gerontol. A Biol. Sci. Med. Sci. 76, 318–327. doi: 10.1093/gerona/glaa178
Rasmussen, L. J. H., Ladelund, S., Haupt, T. H., Ellekilde, G., Poulsen, J. H., Iversen, K., et al. (2016). Soluble urokinase plasminogen activator receptor (suPAR) in acute care: A strong marker of disease presence and severity, readmission and mortality. A retrospective cohort study. Emerg. Med. J. 33, 769–775. doi: 10.1136/emermed-2015-205444
Rawlins, R. G., and Kessler, M. J. (1986). “The history of the Cayo Santiago colony,” in The Cayo Santiago macaques: History, behavior, and biology, eds R. G. Rawlins and M. J. Kessler (Albany, NY: State University of New York Press), 13–46.
R Core Team (2022). R: A language and environment for statistical computing. Vienna: R Foundation for Statistical Computing.
Reibnegger, G., Egg, D., Fuchs, D., Günther, R., Hausen, A., Werner, E. R., et al. (1986). Urinary neopterin reflects clinical activity in patients with rheumatoid arthritis. Arthritis Rheum. 29, 1063–1070. doi: 10.1002/art.1780290902
Reibnegger, G., Huber, L. A., Jürgens, G., Schönitzer, D., Werner, E. R., Wachter, H., et al. (1988). Approach to define “normal aging” in man. Immune function, serum lipids, lipoproteins and neopterin levels. Mech. Ageing Dev. 46, 67–82. doi: 10.1016/0047-6374(88)90115-7
Rioux, E., Pelletier, F., and St-Laurent, M.-H. (2019). Influence of lipids on stable isotope ratios in mammal hair: Highlighting the importance of validation. Ecosphere 10:e02723. doi: 10.1002/ecs2.2723
Sacco, A. J., Granatosky, M. C., Laird, M. F., and Milich, K. M. (2021). Validation of a method for quantifying urinary C-peptide in platyrrhine monkeys. Gen. Comp. Endocrinol. 300:113644. doi: 10.1016/j.ygcen.2020.113644
Sapolsky, R. M. (1983). Endocrine aspects of social instability in the olive baboon (Papio anubis). Am. J. Primatol. 5, 365–379. doi: 10.1002/ajp.1350050406
Schennach, H., Murr, C., Gächter, E., Mayersbach, P., Schönitzer, D., and Fuchs, D. (2002). Factors influencing serum neopterin concentrations in a population of blood donors. Clin. Chem. 48, 643–645. doi: 10.1093/clinchem/48.4.643
Schobersberger, W., Hoffmann, G., Hobisch-Hagen, P., Bock, G., Volkl, H., Baier-Bitterlich, G., et al. (1996). Neopterin and 7,8-dihydroneopterin induce apoptosis in the rat alveolar epithelial cell line L2. FEBS Lett. 397, 263–268. doi: 10.1016/s0014-5793(96)01194-5
Sidler, C., Woycicki, R., Ilnytskyy, Y., Metz, G., Kovalchuk, I., and Kovalchuk, O. (2013). Immunosenescence is associated with altered gene expression and epigenetic regulation in primary and secondary immune organs. Front. Genet. 4:211. doi: 10.3389/fgene.2013.00211
Sier, C. F., Sidenius, N., Mariani, A., Aletti, G., Agape, V., Ferrari, A., et al. (1999). Presence of urokinase-type plasminogen activator receptor in urine of cancer patients and its possible clinical relevance. Lab. Invest. 79, 717–722.
Siracusa, E. R., Higham, J. P., Snyder-Mackler, N., and Brent, L. J. N. (2022). Social ageing: Exploring the drivers of late-life changes in social behaviour in mammals. Biol. Lett. 18:20210643. doi: 10.1098/rsbl.2021.0643
Sucher, R., Schroecksnadel, K., Weiss, G., Margreiter, R., Fuchs, D., and Brandacher, G. (2010). Neopterin, a prognostic marker in human malignancies. Cancer Lett. 287, 13–22. doi: 10.1016/j.canlet.2009.05.008
Tahar, R., Albergaria, C., Zeghidour, N., Ngane, V. F., Basco, L. K., and Roussilhon, C. (2016). Plasma levels of eight different mediators and their potential as biomarkers of various clinical malaria conditions in African children. Malar. J. 15:337. doi: 10.1186/s12936-016-1378-3
Thunø, M., Macho, B., and Eugen-Olsen, J. (2009). suPAR: The molecular crystal ball. Dis. Markers 27, 157–172. doi: 10.3233/DMA-2009-0657
Vogel, E. R., Crowley, B. E., Knott, C. D., Blakely, M. D., Larsen, M. D., and Dominy, N. J. (2012). A noninvasive method for estimating nitrogen balance in free-ranging primates. Int. J. Primatol. 33, 567–587. doi: 10.1007/s10764-011-9543-6
Wakabayashi, I., Nakanishi, M., Ohki, M., Suehiro, A., and Uchida, K. (2020). A simple and useful method for evaluation of oxidative stress in vivo by spectrofluorometric estimation of urinary pteridines. Sci. Rep. 10:11223. doi: 10.1038/s41598-020-67681-4
Watowich, M. M., Chiou, K. L., Montague, M. J. Cayo Biobank, Research Unit, Simons, N. D., et al. (2022). Natural disaster and immunological aging in a nonhuman primate. Proc. Natl. Acad. Sci. U.S.A. 119:e2121663119. doi: 10.1073/pnas.2121663119
Widdig, A., Muniz, L., Minkner, M., Barth, Y., Bley, S., Ruiz-Lambides, A., et al. (2017). Low incidence of inbreeding in a long-lived primate population isolated for 75 years. Behav. Ecol. Sociobiol. 71:18. doi: 10.1007/s00265-016-2236-6
Wittenhagen, P., Kronborg, G., Weis, N., Nielsen, H., Obel, N., Pedersen, S. S., et al. (2004). The plasma level of soluble urokinase receptor is elevated in patients with Streptococcus pneumoniae bacteraemia and predicts mortality. Clin. Microbiol. Infect. 10, 409–415. doi: 10.1111/j.1469-0691.2004.00850.x
Keywords: immunosenescence, inflammaging, neopterin, suPAR, gene expression, aging, transcriptomics, Macaca mulatta
Citation: Cooper EB, Watowich MM, Beeby N, Whalen C, Cayo Biobank Research Unit, Montague MJ, Brent LJN, Snyder-Mackler N and Higham JP (2022) Concentrations of urinary neopterin, but not suPAR, positively correlate with age in rhesus macaques. Front. Ecol. Evol. 10:1007052. doi: 10.3389/fevo.2022.1007052
Received: 29 July 2022; Accepted: 04 October 2022;
Published: 28 October 2022.
Edited by:
Daniel T. Blumstein, University of California, Los Angeles, United StatesReviewed by:
Markus Fendt, University Hospital Magdeburg, GermanyNadine Müller-Klein, University of Ulm, Germany
Copyright © 2022 Cooper, Watowich, Beeby, Whalen, Cayo Biobank Research Unit, Montague, Brent, Snyder-Mackler and Higham. This is an open-access article distributed under the terms of the Creative Commons Attribution License (CC BY). The use, distribution or reproduction in other forums is permitted, provided the original author(s) and the copyright owner(s) are credited and that the original publication in this journal is cited, in accordance with accepted academic practice. No use, distribution or reproduction is permitted which does not comply with these terms.
*Correspondence: Eve B. Cooper, ZXZlLmNvb3BlckBueXUuZWR1