- 1Inner Mongolia Key Laboratory of River and Lake Ecology, School of Ecology and Environment, Inner Mongolia University, Hohhot, China
- 2Key Laboratory of Mongolian Plateau Ecology and Resource Utilization, Ministry of Education, Hohhot, China
Understanding the water quality and its influencing factors of different water bodies is essential for managing water resources in closed inland lake basins in semi-arid regions. However, generally, groundwater or surface water is assessed separately, and the differences among different water bodies are neglected. This study assessed the water quality and its influencing factors of different water bodies in the Daihai Lake Basin (a closed inland lake basin in a semi-arid region) by analysing the hydrochemical data of groundwater, and spring, river, and lake waters in the dry and wet seasons. The dominant hydrochemical type of groundwater (81.48%), spring water (80%), and river water (83.33%) was HCO3–Ca•Mg, while that of lake water was Cl-Na (100%). Groundwater, spring water, and river water were suitable for drinking and agricultural irrigation; however, the groundwater quality was worse in the wet season than in the dry season. Na+ and Cl– majorly affected the lake water quality. The mean NO3– concentration in groundwater was 28.39 mg/L, and its non-carcinogenic hazard quotient indicated that high risk areas were mainly distributed in Tiancheng and northern Maihutu. The hydrochemical compositions of groundwater, spring water, and river water were mainly influenced by rock (silicate and carbonate) weathering and cation exchange, and agricultural activities were the main sources of groundwater NO3–. Moreover, the lake hydrochemical composition was mainly affected by evaporation and halite dissolution. Thus, groundwater NO3– pollution and lake water salinisation should be prioritised. These findings provide a more thorough understanding of water quality and its influencing factors in the closed inland lake basin in the semi-arid region, and can be used to develop the protection of ecosystems and water resources management strategies in the Daihai Lake Basin.
Introduction
Water resources are extremely important to promote socioeconomic development and maintain and conserve ecosystems, especially in semi-arid regions (Xiao et al., 2014; Abboud, 2018; Liu et al., 2022). In addition, the sustainable development of water resources plays an important role in the future social development, as water quality influences human health, quality of life, and crop growth (Wang and Li, 2022; Xiao et al., 2022). The concentration and occurrence of chemical components in water will have direct influence on the water quality (Talib et al., 2019; Sunkari et al., 2022). Hydrochemical components in natural water are the products of the interactions between water and the surrounding environmental factors during the water cycle. Processes, such as atmospheric precipitation, rock weathering, and evaporation, usually control the continuous changes in the hydrochemical components in natural water bodies (Zhou et al., 2016; Hua et al., 2020; Yan et al., 2021). In recent years, anthropogenic activities associated with rapid industrialisation, agricultural development, and urbanisation have continuously changed hydrochemical components, thereby constantly deteriorating the water resources (Armengol et al., 2017; Wang et al., 2022b). Therefore, prevention and mitigation of water pollution is necessary to ensure water security at a global scale.
Analysing the hydrochemistry and water quality cannot only identify the mechanisms of interaction between water and the surrounding environmental factors, but also reflect the status of regional water quality, thus, allowing for more rational protection and sustainable utilisation of water resources (Ghezzi et al., 2019; Xiao et al., 2021; Wang and Li, 2022). Under natural conditions, weathering of rocks due to water can lead to the dissolution of various minerals, which results in the gradual accumulation of chemical ions in water (Zhou et al., 2016; Ren et al., 2020). Contrastingly, pollutants originating from anthropogenic activities may occur in water within a relatively short period (Yan et al., 2021). The hydrochemistry of water bodies, particularly source and aggregation mechanisms of ions in water, can be assessed through methods, such as multivariate statistical analysis (Yidana et al., 2008; Mao et al., 2021), Piper diagram (Piper, 1944), Gibbs diagram (Gibbs, 1970), and ion ratios (Xiao et al., 2016; Ren et al., 2020). Additionally, water quality index (WQI) can effectively combine multiple physical and chemical parameters into a comprehensive value, and the method can reflect the overall status of water quality and is widely used in the water quality evaluation (Abbasnia et al., 2018; Rabeiy, 2018; Yan et al., 2021). Electrical conductivity (EC), sodium adsorption ratio (SAR), sodium percentage (Na%), and permeability index (PI) are effective indices for comprehensively evaluating the suitability of water for agricultural irrigation (Brindha and Kavitha, 2014; Liu et al., 2021). In addition, health risk assessment (HRA) can quantitatively describe the risks posed by water to human health, thereby providing essential information for water quality evaluation and water resource management (Su et al., 2017; Adimalla et al., 2020). These above-mentioned indices and HRAs, which can evaluate the suitability of water, have been widely applied previously in arid and semi-arid regions. For example, studies have been conducted on the Aksu River, Turkey (Sener et al., 2017), groundwater in Central Sindh, Pakistan (Talib et al., 2019), groundwater in the Ningxia, China (Wang et al., 2022b), and groundwater in the Upper Egypt area (Rabeiy, 2018). These studies separately assessed the water quality of either groundwater or surface water. However, while studying regional water quality, different water bodies and seasonal variations must be considered. Therefore, investigating the water quality and its influencing factors of different water bodies is significant to ensure the sustainable utilisation of water resources in a lake basin.
The Daihai Lake Basin is an important ecological area of the Inner Mongolia Autonomous Region. The water resources play critical roles in ensuring regional ecological environmental security and sustainable socioeconomic development in this basin. In recent years, the ecological environment of the Daihai Lake Basin has received widespread concern. Previous studies on the ecological environment of this area have mainly focused on climate change (Xu et al., 2004), ecological restoration (Wang et al., 2019), and lake water quality and quantity (Liang et al., 2021; Ma et al., 2022; Ren et al., 2022). However, comprehensive research on the water quality and its influencing factors in the Daihai Lake Basin is lacking. Being a semi-arid, closed inland lake basin, the shortage of water resources in the Daihai Lake Basin has hindered local economic development (Wang et al., 2022a,c). Additionally, this basin is a farming-pastoral ecotone, and thus, the excessive use of fertilisers and pesticides and irrational discharge of domestic wastewater can deteriorate the water quality (Liang et al., 2021; Sun et al., 2021). These adverse factors have been threatening the local population health, and agricultural development. Therefore, a comprehensive hydrogeochemical study is necessary to determine the water quality and its influencing factors of different water bodies in the region during the dry and wet seasons that can contribute to improving the understanding of the changes in the water quality of the regional water resources. Additionally, considering that the hydrochemistry of the lake basin is changing rapidly, up-to-date observational data must be used to comprehensively explore the water quality status and its influencing factors to formulate adaptive water resource management and pollution control strategies.
The main aims of the present study were to: (1) identify the hydrochemical characteristics of different water bodies in the Daihai Lake Basin, (2) assess the suitability of different water bodies for drinking and irrigation use and evaluate the potential human health risk of NO3– in groundwater, and (3) determine the main factors influencing the water quality of the different water bodies. The findings of this study can contribute to development and implementation of efficient strategies for water resource management and protection in the Daihai Lake Basin and provide a reference for water quality studies of water resources in other closed inland lake basins.
Study area
The Daihai Lake Basin (∼2341.67 km2; 40°11′–40°48′ N, 112°16′–112°59′ E) is a typical closed inland lake basin on the Inner Mongolia Plateau (Figure 1) with a temperate continental semi-arid monsoon climate. The average temperature is 6.09°C and multi-annual average precipitation is 415.55 mm. In the study area, the annual precipitation distribution is uneven, with significant dry and wet seasonal changes. It is mainly concentrated in June–September (wet season), accounting for 76.94% of the annual precipitation. The dry season is from October to May of the next year. Further, the multi-year average evaporation is 1795.96 mm.
Daihai Lake, a closed lake with an average water depth of ∼4 m and surface area of ∼55 km2, is the most important natural lake in this basin. The main sources of lake water are surface runoff, groundwater supply, and lake surface precipitation. Most rivers in the basin are seasonal rivers, and currently, only the Gongba, Tiancheng, and Suodaigou rivers are perennial rivers, which eventually flow into Daihai Lake. In recent years, the area of Daihai Lake has decreased sharply, water quality has deteriorated, and the flora and fauna in and around the lake have diminished on a large scale. Consequently, the lake basin ecosystem is on the verge of collapse, and thus, efforts to conserve the Daihai Lake ecosystem are urgently required.
Daihai Lake is located at the lowest elevation in the region. Furthermore, the Daihai Lake Basin is surrounded by Matou Mountain in the south, Manhan Mountain in the north, and a rifted basin in the centre. These mountainous ranges are inclined to the centre of the basin and are the main groundwater recharge areas consisting of harder gneisses and basalts. Cutting of mountain gullies exposes aquifers, thus, creating more springs in the mountains. The runoff area is majorly a slope plain near the foothills, mainly the middle and upper parts of the alluvial fan, and the aquifer comprises sand and gravel. Local groundwater is recharged by precipitation infiltration, lateral recharge in hilly areas, and infiltration recharge of surface water (floods and spring water). Groundwater movement changes according to the changes in lithology and hydraulic gradient. In the Daihai Lake shore area and the floodplain fan margin, the hydraulic gradient decreases. The aquifer mainly comprises Quaternary sandy soil, and the buried depth of the water level is relatively shallow; therefore, groundwater discharge is mainly through evaporation. Further, groundwater in this basin forms a complete hydrogeological unit from the mountain areas and piedmont gravel plains, which receive recharge from precipitation, to recharge runoff areas and the runoff discharge areas of the fine soil plains.
Materials and methods
Sample collection and analysis
In consideration of the regional hydrogeological condition, groundwater flow field, lake morphology, and river-lake link, we selected 27 groundwater sites, 5 spring water sites, 3 river water sites, and 5 lake water sites, respectively, to carry out the sampling during the dry season (April) and wet season (July) of 2021 (Figure 1). At each sampling sites, we used two clean polyethylene plastic bottles (500 mL) rinsed thrice by the source water to collect samples, and then sealed to avoid air bubbles and transported back to the laboratory for further testing and analysis. The groundwater samples mainly originated from the pore water of quaternary loose rocks were taken from the free surface of civilian wells ranging from 6 to 60 m depth. The collected water source was flowed for at least 10 min prior to water sampling to accurately reflect the groundwater at the position. Spring and river water samples were sampled at a depth of 0.2 m from the surface. Lake water were collected using 1.5 L plexiglass sampler at a depth of 0.5 m from the surface and at a depth of 0.5 m from the bottom to ensure reliability and validity of the data. During sampling, a global positioning system was used to accurately determine the geographical coordinates and elevation of the sampling site.
pH and total dissolved solids (TDS) of the different water samples were measured on site using a WTW Multi 3630 multimeter (Germany) with measurement accuracies of ± 0.004 and ± 0.5%, respectively. Ca2+, Mg2+, Na+, and K+ concentrations were measured by inductively coupled plasma optical emission spectrometry, SO42– concentration was determined by ion chromatography, and NO3– concentration was determined by UV–Vis spectrophotometry. Further, HCO3– concentration, Cl– concentration, and chemical oxygen demand (COD) were measured by acid-base indicator titration, silver nitrate titration, and potassium permanganate titration, respectively. The specifc analytical methods and their resources and equipments were listed in Supplementary Table 1 in Supplementary material. The same sampling methods and instruments were used for all samples. The accuracies of the hydrochemical data were verified using the charge balance error (). The CBE of all samples ranged from 0.04 to 5.59%, with a mean of 3.03%, which verified the reliability and accuracy of the data.
Data analysis
Data analysis process
The general hydrochemical characteristics and types of water resources were determined using descriptive statistics and the Piper diagram. WQI was used to evaluate the potability of water, while EC, SAR, Na%, and PI were used to comprehensively evaluate the suitability of water for agricultural irrigation. The model recommended by the United States Environmental Protection Agency (USEPA, 1989) was used to assess the risk posed by groundwater NO3– to human health through groundwater consumption. Further, Gibbs diagrams and ion ratios were used to determine the main influencing factors controlling the hydrochemistry, ion sources, and hydrogeochemical processes.
Evaluating the water quality for drinking
WQI was used to evaluate the potability of water resources in the Daihai Lake Basin. Eleven water quality parameters were selected, namely, pH, TDS, Ca2+, Mg2+, Na+, K+, HCO3–, SO42–, Cl–, NO3–, and COD to calculate the WQI, as follows:
where Wi is the relative weight and wi is the weight of each parameter. The weight range of water quality parameters was 2–5 (Supplementary Table 2 in Supplementary material), which has been verified in previous studies (Xiao et al., 2014; Yan et al., 2021). Further, n is the number of parameters, qi is the quality level of the ith parameter, Ci is the concentration of each parameter in each sample (mg/L, except for pH), and Si is the permissible value of each parameter. Considering the background conditions of water quality and the physical condition of the residents in China, this study adopted the guideline values of Drinking Water Quality of the People’s Republic of China (GB5749-2006) (Ministry of Health, 2006) as permissible values for drinking water parameters. The WQI values were divided into five classes: excellent (WQI < 25), good (25 < WQI < 50), moderate (50 < WQI < 100), poor (100 < WQI < 150), and very poor (150 < WQI).
Evaluating the water quality for irrigation
The Daihai Lake Basin includes a large farmland area (Supplementary Figure 1 in Supplementary material) (Liang et al., 2021), and the water resources in the basin play an important role in agricultural development. Excessive salinity in irrigation water can cause soil salinisation, whereas high Na concentrations can cause soil alkalinisation, compaction, and reduced permeability (Abbasnia et al., 2018; Bouimouass et al., 2022). Therefore, EC, SAR, Na%, and PI were used to comprehensively evaluate the suitability of water for agricultural irrigation. Each indicator was calculated as follows:
where the units of all cations and HCO3– are meq/L, and the units of EC, Na%, and PI are μs/cm, %, and %, respectively.
Human health risk assessment
HRA can quantitatively describe the impact of toxic substances in groundwater on human health (Xiao et al., 2022). The HRA model and criteria provided by the USEPA were used to assess the health risks of NO3– pollution in the groundwater of the Daihai Lake Basin to the local residents. As NO3– is a non-carcinogenic toxic substance and the risk assessment of toxic substances is based on the reference dose, this study adopted a non-carcinogenic HRA model, which can be calculated as follows:
where HQ is the non-carcinogenic risk index (dimensionless), ICD is the average daily exposure dose of a non-carcinogenic substance (mg/kg/d) and is calculated according to the Eq. 9, and DRf is the reference dose of a specific non-carcinogen in groundwater (mg/kg/d). The non-carcinogenic risk threshold HQ recommended by the USEPA is 1. HQ values of < 1 and > 1 indicate that the non-carcinogenic health risk to humans caused by nitrogen pollution is within acceptable and unacceptable levels, respectively; thus, higher the HQ value, greater the non-carcinogenic health risks.
NO3– in groundwater enters the human body mainly through drinking water. The average daily exposure dose (ICD) is calculated as follows (Qasemi et al., 2018; Liu et al., 2021):
where C is the measured concentration of NO3– in groundwater (mg/L), IR is the drinking water rate (L/d), EF is the exposure frequency (d/a), which is the time of exposure in a year, ED is the duration of exposure (a), indicating the number of years that the human has consumed the toxic substance throughout its life, BW is the average body weight of the inhabitants (kg), and AT is the average exposure time (d), that is, the average time of exposure. Supplementary Table 3 in Supplementary material lists the parameters of the HRA model for groundwater NO3– used in this study.
Results
Hydrochemical composition
The statistical results for the hydrochemical parameters are shown in the Table 1, Supplementary Table 4 in Supplementary material, and Figure 2. The average pH of the groundwater, spring water, river water, and lake water ranged from 8.03 to 9.19, indicating weakly alkaline water bodies in the Daihai Lake Basin. The average TDS values of groundwater, spring water, and river water were all less than 1000 mg/L, while the average TDS value of lake water was 23725 mg/L, indicating that the lake water was mainly salt water, whereas the other water bodies were fresh water. The orders of cation concentrations in groundwater, spring water, river water, and lake water were Ca2+ > Na+ > Mg2+ > K+, Na+ > Ca2+ > Mg2+ > K+, Na+ > Ca2+ > Mg2+ > K+, and Na+ > Mg2+ > K+ > Ca2+, respectively, indicating that the dominant cations were Ca2+ (44.04%), Na+ (54.32%), Na+ (45.15%), and Na+ (94.22%), respectively, in the four sample types; furthermore, the orders of anion concentrations were HCO3–> Cl– > NO3– > SO42–, HCO3–> SO42–> Cl– > NO3–, HCO3–> Cl– > SO42−[cpsbreak]> NO3–, and Cl– > HCO3–> SO42–> NO3–, respectively, indicating that the dominant anions were HCO3– (74.31%), HCO3– (74.04%), HCO3– (79.48%), and Cl– (84.88%), respectively, in the four sample types. Marginal differences were observed in the ion concentrations between the dry and wet seasons in groundwater, spring water, and lake water, whereas the ion concentrations in the river water were higher in the dry season than in the wet season (Figure 2 and Supplementary Table 4). Overall, the hydrochemical characteristics between the different water bodies showed some differences, with the exception of river water, which showed marginal seasonal variation.
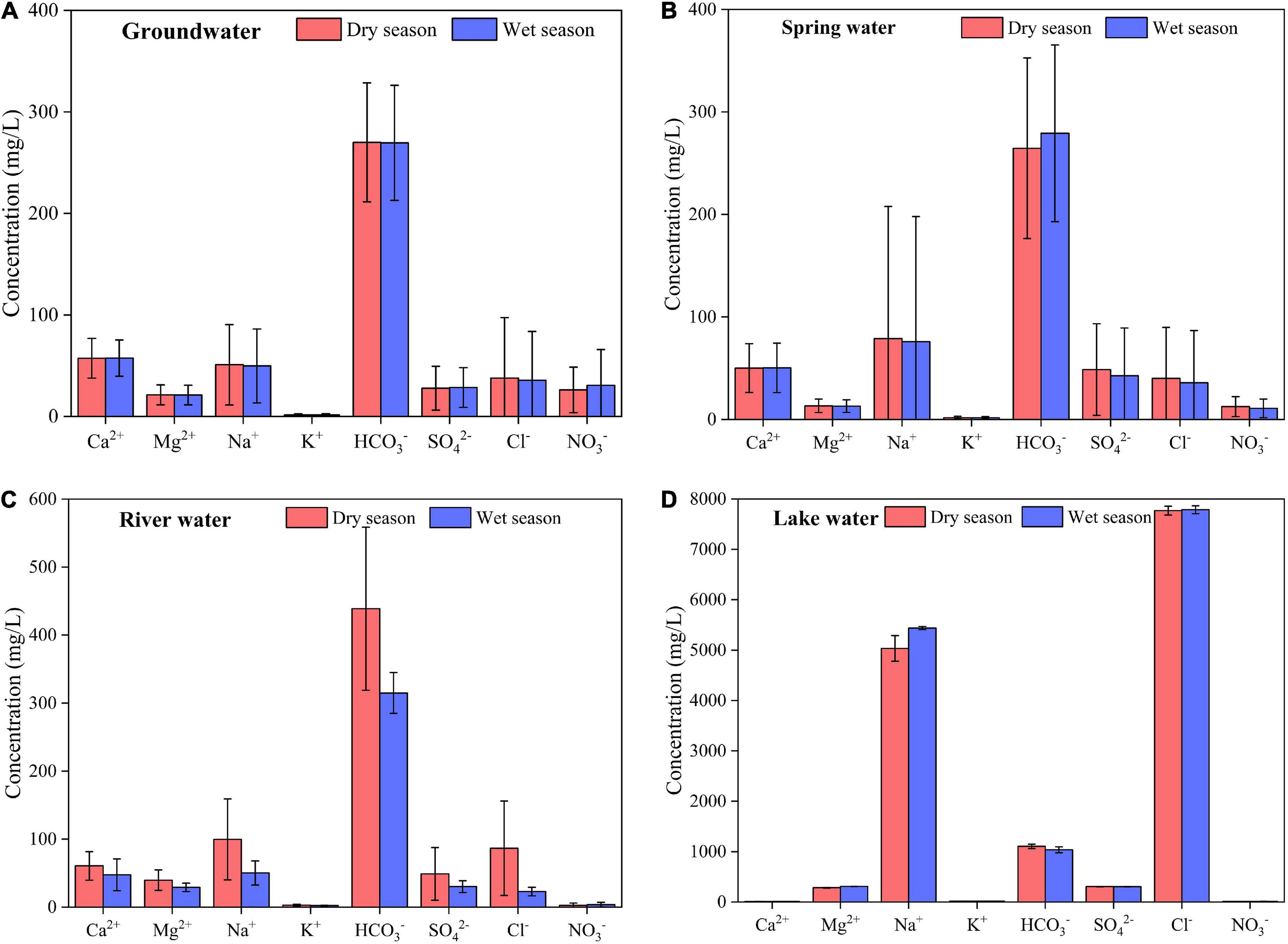
Figure 2. Ion concentrations of in groundwater (A), spring water (B), river water (C), and lake water (D).
The NO3– concentration in groundwater ranged from 2.25 to 167.83 mg/L, with an average of 28.39 mg/L, and it exceeded the permissible limit (44.29 mg/L) of the standards of Drinking Water Quality of the People’s Republic of China (GB5749-2006) (Ministry of Health, 2006) in 18.52% of the samples. However, the NO3– concentrations in the spring water, river water, and lake water were relatively low. The COD concentrations in groundwater, spring water, and river water were low, but they ranged from 19.8 to 24.75 mg/L, with an average of 22.05 mg/L, in lake water, thus, exceeding the permissible limit (3 mg/L) of the GB5749-2006 standards (Ministry of Health, 2006) and the Class V limits (10 mg/L < COD ≤ 15 mg/L) of the Environmental Quality Standards for Surface Water of the People’s Republic of China (GB3838-2002) (Ministry of Ecology and Environment, 2002). These results indicated severe organic pollution in Daihai Lake.
Hydrochemical types
RockWare AqQA software was applied to produce the Piper diagram for hydrochemistry during the dry and wet seasons (Figure 3). The groundwater, spring water, and river water samples were mainly distributed in Zone III, accounting for 81.48, 80, and 83.33% of the samples, respectively, indicating that the hydrochemical types of these water were mainly HCO3–Ca•Mg type; further, some groundwater, spring water, and river water samples were distributed in Zone II (HCO3–Na type), accounting for 14.81, 20, and 16.67% of the samples, respectively, and a small proportion of groundwater samples were distributed in Zone I (SO4•Cl-Ca•Mg type), accounting for 3.7% of the samples. All the lake water samples were distributed in Zone IV, indicating that the hydrochemical type of the lake was Cl-Na type.
Water quality evaluation
Drinking water quality assessment
WQI was used to evaluate the potability of the water resources in the Daihai Lake Basin. The average WQI of groundwater was higher in the wet season (50) than in the dry season (46.71). Figure 4 shows that the proportions of good and moderate water quality of the groundwater samples were 70.37 and 29.63% in the dry season, respectively, while they were 62.96 and 33.33%, respectively, in the wet season. Therefore, this indicates that the water quality was good and suitable for drinking in most parts of the Daihai Lake Basin. Notably, the proportion of good water quality of the groundwater samples decreased from the dry season (70.37%) to the wet season (62.96%), whereas an opposite trend was observed for the proportion of moderate water quality. In addition, during the wet season, groundwater samples were observed to have very poor quality. These results suggested that the groundwater quality decreased from the dry to wet season. The groundwater samples with poor water quality were distributed in Tiancheng. In addition, the good-quality groundwater samples were found in the recharge runoff areas in the piedmont, while the moderate-quality groundwater samples were scatted over the groundwater runoff discharge areas of the plain (Figure 4).
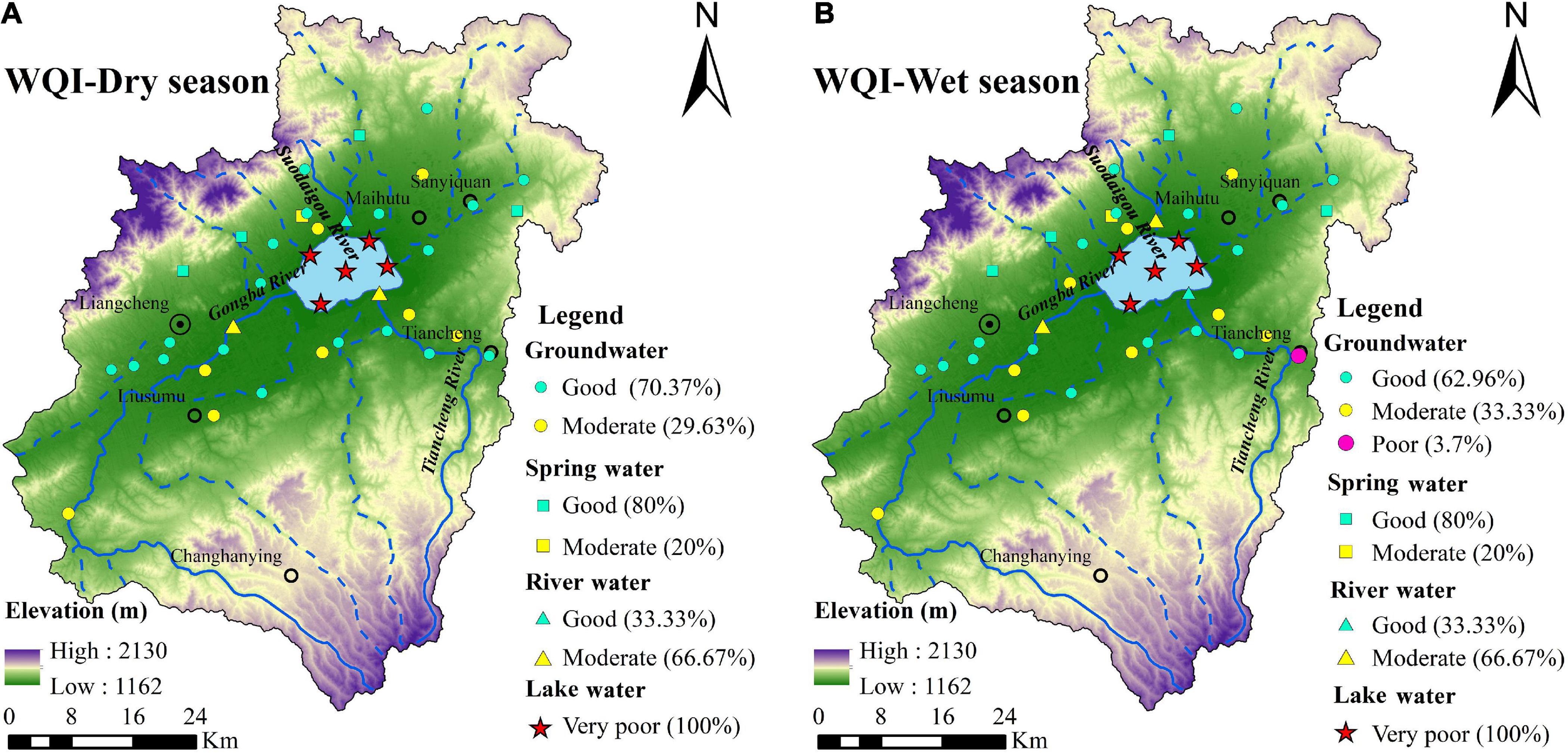
Figure 4. Spatial distribution of water quality assessment results in dry (A) and wet (B) seasons in the Daihai Lake Basin.
The spring water and river water samples in the dry and wet seasons showed good and moderate water quality, respectively, and the changes across the dry and wet seasons were stable, indicating that the spring water and river water qualities were good and suitable for drinking. Furthermore, all lake water samples in the dry and wet seasons showed very poor water quality, with an average WQI value of >800. Thus, the quality of the lake water was very poor, which made it unsuitable for drinking. The Na+ and Cl– concentrations in the lake water were 26.17 and 31.11 times higher than the standard values, respectively, and the concentrations of other ions were 0.12–9.87 times of the standard values, indicating that water quality in the lake was mainly affected by Na+ and Cl–.
Irrigation water quality evaluation
High ion concentrations in water affect the vegetation growth; therefore, estimating whether the water is suitable for irrigation, and ultimately crop growth, is necessary. The USSL diagram (USSL, 1954) was applied to analyse water salinity and determine whether the water was suitable for irrigation. Highly saline irrigation water is usually not conducive to water infiltration, and can consequently deteriorate soil quality. Figure 5A shows that most groundwater, spring water, and river water samples in the dry and wet seasons were distributed in the C2S1 and C3S1 zones, with the proportions of groundwater and spring water samples in the C2S1 zone being 20.37 and 30%, respectively, and the proportions of groundwater, spring water, and river water samples in the C3S1 zone being 79.63, 50, and 100%, respectively. All lake water samples were distributed in the C4S4 zone. Irrigation water with high Na+ content reduces soil fertility. Wilcox (1955) classified irrigation water quality based on Na% and EC. According to this classification, most groundwater, spring water, and river water samples in the dry and wet seasons were distributed in the excellent to good and good to permissible zones (Figure 5B). Specifically, the proportions of groundwater and spring water samples in the excellent to good zone were 20.37 and 30%, respectively, while the proportions of groundwater, spring water, and river water samples in the good to permissible zone were 62.96, 50, and 83.33%, respectively. However, all lake water samples were distributed in the unsuitable zone. Doneen (1964) classified water quality into three categories according to the PI. Accordingly, most groundwater, spring water, and river water samples, accounting for 75.93, 60, and 100%, respectively, in the dry season and wet season were distributed in the Class I zone, while all lake water samples were distributed in the Class II zone (Figure 5C). In summary, groundwater, spring water, and river water in the dry and wet seasons were suitable as water sources for agricultural irrigation, while lake water, which showed poor irrigation water quality, was extremely unsuitable for irrigation.
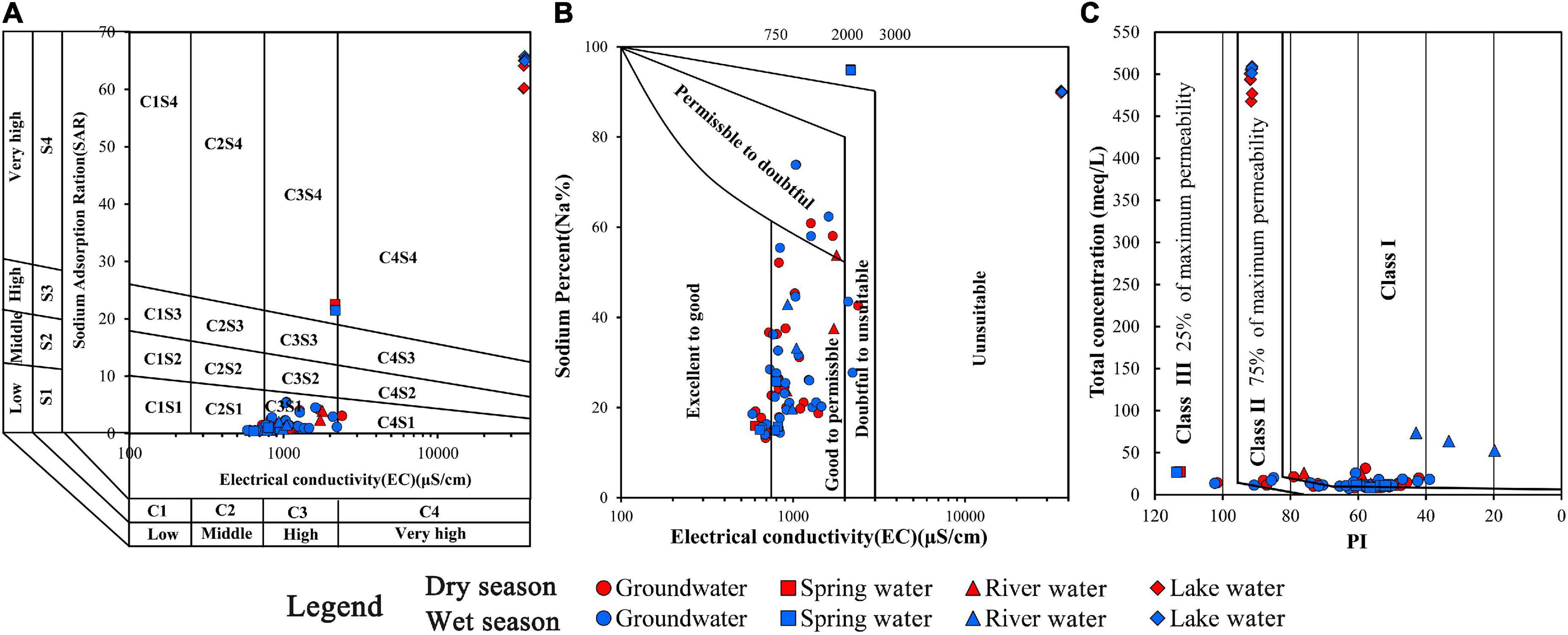
Figure 5. Classification of irrigation water quality in the Daihai Lake Basin according to USSL (A), Wilcox (B), and PI (C).
Health risk assessment of NO3–
NO3– pollution of groundwater has become an increasingly common phenomenon, especially in areas with developed agricultural and animal husbandry sectors (Liu et al., 2022). Long-term drinking of such NO3–-contaminated groundwater can lead to methemoglobinemia, cancer of the digestive system, and blue baby syndrome (Gao et al., 2020; Wang and Li, 2022). The results of this study showed high NO3– concentrations in the groundwater in the Daihai Lake Basin. Therefore, the HRA model and criteria provided by the USEPA were used to evaluate the health risks of NO3– in groundwater to local residents. The corresponding HRA results showed that the HQ of children and adults in the dry season ranged from 0.08 to 3.12 and 0.05 to 1.92, respectively, with mean values of 0.82 and 0.5, respectively, whereas in the wet season, it ranged from 0.07 to 5.24 and 0.04 to 3.23, respectively, with the mean values of 0.96 and 0.59, respectively. The proportion of groundwater samples with HQ <1 was 77.78 and 74.07% for children during the dry and wet seasons, respectively, whereas for adults, the proportion of groundwater samples with HQ <1 was 88.89 and 85.19%, respectively (Figure 6). This indicated that groundwater in most areas posed an acceptable health risk for children and adults. However, in the wet and dry seasons, areas with HQ >1 for children and adults were mainly distributed in Tiancheng and northern Maihutu, indicating that the groundwater in these areas posed an unacceptable risk to the health of children and adults. Notably, the mean HQ value and the proportion of groundwater samples with HQ >1 were higher for children and adults in the wet season than in the dry season, indicating that the risk of NO3– in the groundwater in the wet season was higher than that in the dry season. Additionally, NO3– in groundwater showed a significantly larger spatial distribution of health risks to children than to adults.
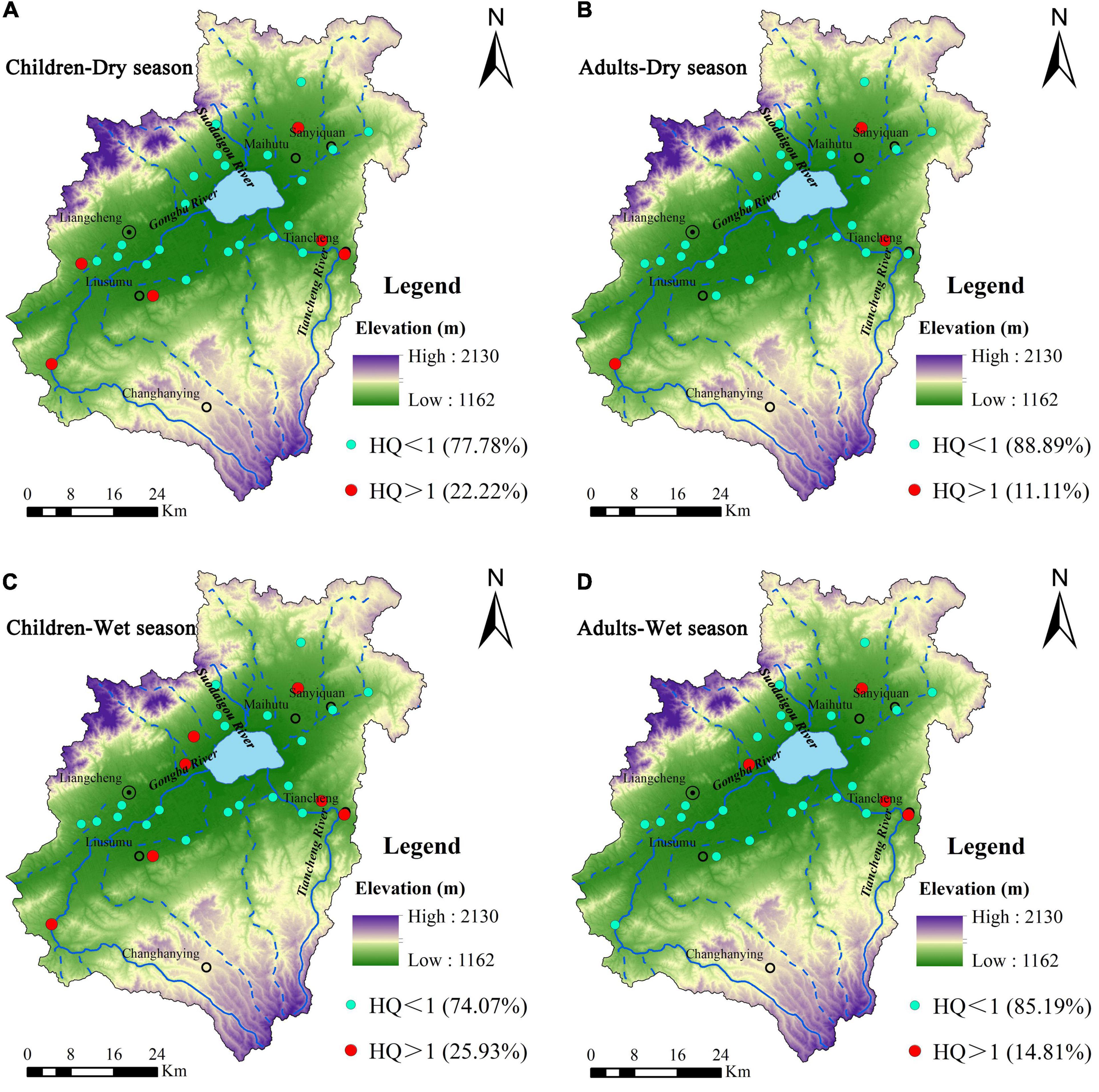
Figure 6. Spatial distribution of the results of health risk assessment of groundwater NO3– in the Daihai Lake Basin for children during the dry season (A), adults during the dry season (B), children during the wet season (C), and adults during the wet season (D).
Discussion
Natural factors influencing water quality
The Gibbs diagram is widely used to analyse the main factors controlling hydrochemical composition and evolution (Gibbs, 1970; Zhou et al., 2016; Ren et al., 2020). Figure 7 shows that all groundwater, spring water, and river water sampling points were located in the area of rock weathering dominance, indicating that rock weathering majorly controlled the water chemistry of these water bodies. Further, continuous leaching of groundwater, spring water, and river water during runoff caused the dissolution of minerals through water-rock interaction, thereby contributing to the chemical composition in the water. Abundant precipitation and increased leaching during the wet season raised the ion concentrations in the groundwater and the proportion of pollutants entering the groundwater through precipitation infiltration, consequently, resulting in relatively poor groundwater quality, especially in the Tiancheng. In addition, in the groundwater recharge runoff areas, where groundwater flow was faster and hydrodynamic conditions were stronger, continuous leaching resulted in the removal of salt ions and pollutants from the stratum, while the opposite was true in the groundwater runoff discharge areas. Therefore, the groundwater quality in the recharge runoff areas were better than that in the runoff discharge areas. Further, all lake water sampling points were located in the area of evaporation dominance, indicating that evaporation mainly influenced the lake water chemistry. Precipitation, groundwater, and river water were the main sources of water recharge to the lake. During groundwater and river water runoff, the hydrochemical ions from upstream continued to accumulate in the lake. In addition, because Daihai Lake is a closed inland lake in a semi-arid region, lake water was not leached throughout the year, but was lost through evaporation (Zhao et al., 2020). Therefore, continuous evaporation resulted in the saturation and eventually precipitation of the carbonate ions, and the concentrations of Na+ and Cl– gradually increased.
The main hydrochemical process can be further determined by calculating the ratio of cations to anions in water (Yang et al., 2016; Bouimouass et al., 2022). For example, during the dissolution of halite, the ratio of Na+ to Cl– is 1:1 (Xiao et al., 2014; Ren et al., 2020). Figure 8A shows that most groundwater, spring water, and river water samples fell above the y = x line, indicating that the Cl– was not available in sufficient proportion to balance Na+ possibly because of the weathering and dissolution of silicate rocks or cation exchange. Generally, halite dissolution causes water sampling points to be on the y = x line (Rajmohan and Elango, 2004; Xiao et al., 2021). Consistent with this previous finding, the lake water sampling points were distributed around the y = x line (Figure 8A), suggesting that Na+ and Cl– in the lake water were influenced by the dissolution of halite. Figure 8B shows that most groundwater, spring water, and river water sampling points were distributed below the y = x line, indicating that the weathering and dissolution of silicate rocks majorly affected the hydrochemical components of groundwater, spring water, and river water (Yang et al., 2016). However, the lake water sampling points were distributed on both sides of the y = x line. The ratio of (Ca2++ Mg2+) to HCO3– can reflect the influences of weathering and dissolution of carbonate rocks, such as calcite and dolomite, on the hydrochemistry (Zhou et al., 2016; Jia et al., 2020). Figure 8C shows that the groundwater, spring water, river water, and lake water sampling points were mainly distributed near the y = x line and between the y = x line and the 2y = x line, indicating that the weathering and dissolution of carbonate rocks were the main sources of Ca2+, Mg2+, and HCO3– in these water bodies. Moreover, the dissolution of gypsum can release equivalent amounts of Ca2+ and SO42– in water (Liu et al., 2021). Figure 8D shows that all groundwater, spring water, river water, and lake water sampling points deviated from the y = x line, indicating that Ca2+ and SO42– in these water bodies were not affected by the dissolution of gypsum.
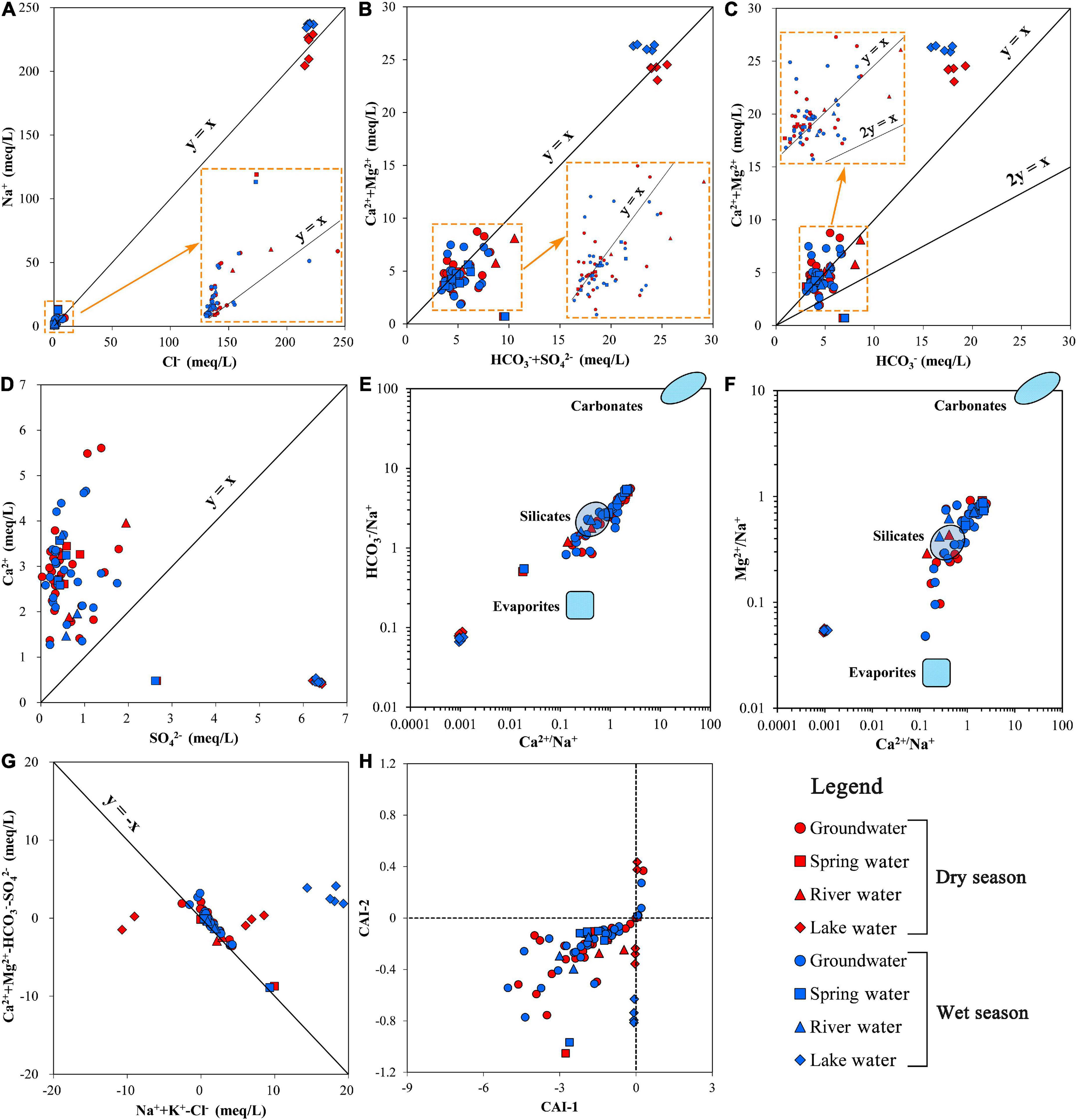
Figure 8. Ion ratios of the water bodies in Daihai Lake Basin: (A) Cl– vs. Na+, (B) ( + SO42–) vs. (Ca2+ + Mg2 +), (C) vs. (Ca2+ + Mg2 +), (D) SO42– vs. Ca2+, (E) Ca2+/Na+ vs. /Na+, (F) Ca2+/Na+ vs. Mg2+/Na+, (G) (Na+ + K+ – Cl–) vs. (Ca2+ + Mg2 + – – SO42–), and (H) CAI-1 vs. CAI-2.
In the Daihai Lake Basin, the groundwater is mainly contained in a Quaternary aquifer composed of sand pebbles and gravel and the lithology is mainly silicate and carbonate rocks. Further, the relationship between the molar ratios of Ca2+/Na+ versus Mg2+/Na+ and Ca2+/Na+ versus HCO3–/Na+ can be used to identify the effect of different types of the water-rock interactions on the hydrochemical components (Gaillardet et al., 1999). As shown in Figures 8E,F, most groundwater, spring water, and river water sampling points were located in the silicate control zone and between the silicate and carbonate control zones, indicating that the chemical components of these three water bodies were mainly affected by the weathering and dissolution of silicate and carbonate. Thus, the regional geological background considerably influenced the hydrochemical composition. Comparatively, the lake water sampling points deviated from the silicate and carbonate control zones and were relatively close to the evaporite control zone, indicating that the chemical components of the lake water were influenced by the weathering and dissolution of evaporites. In addition, as Daihai Lake is a closed inland lake in a semi-arid region, evaporation and concentration have influenced the chemical components of the lake water for long periods, which in turn has increased the concentration of salt ions in the lake water (Zhao et al., 2020).
Cation exchange has a major impact on the chemical composition of water. The relationship between Ca2++Mg2+-HCO3–-SO42– versus Na++K+-Cl– can be applied to identify whether cation exchange occurs in water. If it does, the ratio will show a negative correlation, with a slope of –1 (Su et al., 2017; Wang et al., 2022b). Moreover, the chlor-alkali index (CAI) can indicate whether positive or negative cation exchange occurs in water, as shown in Eqs. 10 and 11 (Abboud, 2018; Wang et al., 2022b). If CAI > 0, Na+ (K+) in the water is replaced by Ca2+ (Mg2+) in the aquifer, whereas if CAI < 0, Ca2+ (Mg2+) in the water is replaced by Na+ (K+) in the aquifer. As shown in the Figure 8G, most sampling points of groundwater, spring water, and river water, except lake water, were distributed on the y = –x line, indicating that cation exchange considerably impacts the hydrochemical composition of the corresponding water bodies. Figure 8H shows that most water sampling points of the groundwater, spring water, and river water were distributed in the zone where CAI < 0, indicating positive cation exchange (Eq. 12) in the corresponding water bodies, and when the Na+ content increased, Ca2+ and Mg2+ contents decreased.
Overall, the hydrochemical compositions of groundwater, spring water, and river water in the Daihai Lake Basin were mainly influenced by rock weathering and cation exchange, which is consistent with the results of Zhang (2020), indicating that natural conditions were the dominant factors in the hydrochemical compositions of these water bodies. Consequently, under the influence of natural factors, the groundwater in the Daihai Lake Basin was mainly weak alkaline with the major hydrochemical type of HCO3–Ca•Mg, which was in line with the results of Zhang et al. (2021) and Wang (2021). Furthermore, the hydrochemical compositions and chemical characteristics of spring water, river water, and groundwater and their influencing factors were almost consistent, suggesting a close hydraulic connection among three water bodies, which agreed with the statements of Wang et al. (2017) and Zhang (2020). Moreover, our evaluation results showed that the lake water quality was very poor, which is similar with the results of the water quality evaluation using nutrient parameters reported by Liang et al. (2021), Zhao et al. (2020), and Wang et al. (2019), indicating that Daihai Lake has been seriously polluted. Besides, we found that the chemical ions in the lake water were mainly influenced by natural factors like evaporation and evaporites, however, the nutrients such as nitrogen and phosphorus were largely affected by anthropogenic activities, suggesting the different pollution ways and mechanisms.
Anthropogenic factors influencing water quality
Rapid and continuous socioeconomic development and urbanisation, and population explosion negatively influence the aquatic environment (Gao et al., 2020; Yan et al., 2021). Anthropogenic activities usually result in the increase of concentration of chemical components in water; particularly, NO3– mainly originates from domestic sewage and agricultural production (Liu et al., 2021; Wang and Li, 2022). The molar ratios of NO3–/Na+ versus Cl–/Na+ can be used as an index to review the effect of anthropogenic activities on water bodies (Xiao et al., 2016; Mao et al., 2021). Generally, these molar ratios are high in polluted water. Figure 9 shows that agricultural activities were an important source of NO3– in groundwater. The Daihai Lake Basin includes large cultivated land and grassland areas, along with extensive animal husbandry sectors (Supplementary Figure 1). The total cumulative area of cultivated land and grassland accounts for 61.28–67.91% of the total basin area, of which the cultivated land accounts for the largest proportion (30.87–45.88%) (Liang et al., 2021), indicating intensive agricultural activities in the Daihai Lake Basin. Thus, excessive application of nitrogen fertilisers (e.g., NaNO3 and KNO3) and manure could increase the NO3– concentration in groundwater (Mao et al., 2021). Furthermore, widespread distribution of rural areas resulted in the high-density population (Supplementary Figure 1), producing a large amount of untreated domestic wastewater containing high concentration of nitrate nitrogen and affecting NO3– concentration directly or indirectly (Liu et al., 2021). In particular, attention should be paid to Tiancheng and areas to the north of Maihutu, where NO3– pollution was relatively serious. Spring water and river water were relatively less affected by agricultural activities, whereas lake water was affected by weathering and dissolution of evaporites.
Agricultural activities considerably influence NO3– concentration in the groundwater in the Daihai Lake Basin. Although the health risk of groundwater NO3– to humans was low in most areas, areas with high local HQ and seasonal changes were also observed. Therefore, prevention and risk management of groundwater pollution still need to be further strengthened.
Study limitations and future work
This study has addressed the water quality and its influencing factors of different water bodies in the Daihai Lake Basin during the dry and wet seasons up to certain extent. However, the formation of water chemical ions depends on hydrochemical evolution processes undergoing over space and time, which has an important impact on water quality (Yang et al., 2016; Ren et al., 2020). Future study could increase the numbers of monitoring sites and conduct the long-term monitoring to obtain more accurate data and reveal the hydrochemical evolution.
Our study showed that groundwater in some areas of the Daihai Lake Basin has been polluted. Therefore, the dynamic monitoring of groundwater quality should be strengthened in the future, with emphasis on the prevention and control of agricultural non-point sources of NO3– pollution and effective management of domestic sewage. Further, awareness among the citizens regarding the conservation of groundwater quality should be promoted to reduce the associated human health risk. In addition, replenishing water to Daihai Lake can improve the water quality to a certain extent due to the dilution of the pollutants, so the continuous hydrochemical researches should be conducted to ensure the efficiency of diversion engineering.
Conclusion
The present study highlighted the water quality and influencing factors of different water bodies in the Daihai Lake Basin using hydrochemical methods. The groundwater, spring water, and river water in the dry and wet seasons were mainly weakly alkaline fresh water, and their hydrochemical types were mainly HCO3–Ca•Mg, whereas the lake water was weakly alkaline salt water, with a hydrochemical type of Cl-Na. Except lake water, groundwater, spring water, and river water were suitable for drinking and agricultural irrigation applications, but the groundwater quality in the wet season was worse than that in the dry season. Further, high Na+ and Cl– concentrations were the main pollution factors affecting the lake water quality. Although 18.52% of the groundwater samples had high NO3– concentrations, the HRA showed that groundwater in most areas posed an acceptable risk to human health, whereas the areas with unacceptable risk were mainly located in Tiancheng and north Maihutu. Rock (silicate and carbonate rocks) weathering and cation exchange were the dominant factors in the hydrochemical compositions of groundwater, spring water, and river water, and agricultural activities were the main sources of NO3– in groundwater. Moreover, the hydrochemical composition of the lake water was mainly affected by evaporation and halite dissolution. These results indicated that the management of the water resources in the Daihai Lake Basin should be strengthened to control and mitigate the NO3– pollution of groundwater and salinisation of Daihai Lake.
Data availability statement
The original contributions presented in this study are included in the article/Supplementary material, further inquiries can be directed to the corresponding author.
Author contributions
XR: formal analysis, software, methodology, writing – original draft, and writing – review and editing. RY: supervision, conceptualization, and writing – review and editing. JK: data curation and resources. XL, RW, SZ, DW, and XZ: investigation and data curation. All authors contributed to the article and approved the submitted version.
Funding
This study was jointly funded by the National Key Research and Development Program of China (Grant No. 2021YFC3201203), National Natural Science Foundation of China (Grant No. 51869014), Major Science and Technology Projects of Inner Mongolia Autonomous Region (Grant No. 2020ZD0009), and Open Project Program of the Ministry of Education Key Laboratory of Ecology and Resources Use of the Mongolian Plateau (Grant No. KF2020006).
Acknowledgments
We thank our colleagues at the Inner Mongolia Key Laboratory of River and Lake Ecology for their help.
Conflict of interest
The authors declare that the research was conducted in the absence of any commercial or financial relationships that could be construed as a potential conflict of interest.
Publisher’s note
All claims expressed in this article are solely those of the authors and do not necessarily represent those of their affiliated organizations, or those of the publisher, the editors and the reviewers. Any product that may be evaluated in this article, or claim that may be made by its manufacturer, is not guaranteed or endorsed by the publisher.
Supplementary material
The Supplementary Material for this article can be found online at: https://www.frontiersin.org/articles/10.3389/fevo.2022.1005289/full#supplementary-material
References
Abbasnia, A., Yousefi, N., Mahvi, A. H., Nabizadeh, R., Radfard, M., Yousefi, M., et al. (2018). Evaluation of groundwater quality using water quality index and its suitability for assessing water for drinking and irrigation purposes: Case study of Sistan and Baluchistan province (Iran). Hum. Ecol. Risk Assess. 25, 988–1005. doi: 10.1080/10807039.2018.1458596
Abboud, I. A. (2018). Geochemistry and quality of groundwater of the Yarmouk basin aquifer, north Jordan. Environ. Geochem. Health 40, 1405–1435. doi: 10.1007/s10653-017-0064-x
Adimalla, N., Qian, H., and Nandan, M. J. (2020). Groundwater chemistry integrating the pollution index of groundwater and evaluation of potential human health risk: A case study from hard rock terrain of south India. Ecotoxicol. Environ. Safety 206:111217. doi: 10.1016/j.ecoenv.2020.111217
Armengol, S., Manzano, M., Bea, S. A., and Martinez, S. (2017). Identifying and quantifying geochemical and mixing processes in the Matanza-Riachuelo aquifer system, Argentina. Sci. Total Environ. 59, 1417–1432. doi: 10.1016/j.scitotenv.2017.05.046
Bouimouass, H., Fakir, Y., Tweed, S., Sahraoui, H., Leblanc, M., and Chehbouni, A. (2022). Traditional irrigation practices sustain groundwater quality in a semiarid piedmont. Catena 210:105923. doi: 10.1016/j.catena.2021.105923
Brindha, K., and Kavitha, R. (2014). Hydrochemical assessment of surface water and groundwater quality along Uyyakondan channel, south India. Environ. Earth Sci. 73, 5383–5393. doi: 10.1007/s12665-014-3793-5
Doneen, L. (1964). Water Quality for Agriculture, Department of Irrigation. Davis: University of California, 48.
Gaillardet, J., Dupre, B., Louvat, P., and Allegre, C. J. (1999). Global silicate weathering and CO consumption rates deduced from the chemistry of large rivers. Chem. Geol. 159, 3–30. doi: 10.1016/S0009-2541(99)00031-5
Gao, S., Li, C., Jia, C., Zhang, H., Guan, Q., Wu, X., et al. (2020). Health risk assessment of groundwater nitrate contamination: a case study of a typical karst hydrogeological unit in East China. Environ. Sci. Pollut. Res. 27, 9274–9287. doi: 10.1007/s11356-019-07075-w
Ghezzi, L., Iaccarino, S., Carosi, R., Montomoli, C., Simonetti, M., Paudyal, K. R., et al. (2019). Water quality and solute sources in the Marsyangdi River system of Higher Himalayan range (West-Central Nepal). Sci. Total Environ. 677, 580–589. doi: 10.1016/j.scitotenv.2019.04.363
Gibbs, R. (1970). Mechanisms controlling world water chemistry. Science 170:1088. doi: 10.1126/science.170.3962.1088
Hua, K., Xiao, J., Li, S., and Li, Z. (2020). Analysis of hydrochemical characteristics and their controlling factors in the Fen River of China. Sustain. Cities Soc. 52:101827. doi: 10.1016/j.scs.2019.101827
Jia, H., Qian, H., Zheng, L., Feng, W., Wang, H., and Gao, Y. (2020). Alterations to groundwater chemistry due to modern water transfer for irrigation over decades. Sci. Total Environ. 717:137170. doi: 10.1016/j.scitotenv.2020.137170
Liang, X., Liu, H. M., Ji, M. C., Chang, M., Wen, L., Yu, R. H., et al. (2021). Effects of land use/cover change on lake water quality in the semi-arid region of northern China: A case study in Lake Daihai Basin (2000-2018). J. Lake Sci. 33, 727–736. doi: 10.18307/2021.0309
Liu, F., Zhen, P., and Wang, S. (2022). Groundwater quality assessment and health risks from nitrate contamination in the Heilongdong Spring Basin, a typical headwater basin of the North China Plain. Environ. Sci. Pollut. Res. 29, 17655–17670. doi: 10.1007/s11356-021-17065-6
Liu, J., Peng, Y., Li, C., Gao, Z., and Chen, S. (2021). An investigation into the hydrochemistry, quality and risk to human health of groundwater in the central region of Shandong Province, North China. J. Clean. Product. 282:125416. doi: 10.1016/j.jclepro.2020.125416
Ma, J. L., Liu, D. W., Wang, J., Cheng, Y. N., Liu, H. M., and Wang, L. X. (2022). Dynamic ecological water demand based on long-term ecological water consumption in Lake Daihai, 1975 to 2020. J. Lake Sci. 34, 207–219. doi: 10.18307/2022.0117
Mao, H., Wang, G., Rao, Z., Liao, F., Shi, Z., Huang, X., et al. (2021). Deciphering spatial pattern of groundwater chemistry and nitrogen pollution in Poyang Lake Basin (eastern China) using self-organizing map and multivariate statistics. J. Clean. Product. 329:129697. doi: 10.1016/j.jclepro.2021.129697
Ministry of Ecology and Environment (2002). Environmental Quality Standards for Surface Water of the People’s Republic of China (GB3838-2002). Beijing: Ministry of Ecology and Environment of the People’s Republic of China.
Ministry of Health (2006). Ministry of Health: Standards for Drinking Water Quality (GB5749-2006). Beijing: Ministry of Health of the People’s Republic of China.
Piper, A. M. (1944). A graphic procedure in the geochemical interpretation of water-analyses. Eos Trans. Am. Geophys. Union 25, 914–928. doi: 10.1029/TR025i006p00914
Qasemi, M., Farhang, M., Biglari, H., Afsharnia, M., Ojrati, A., Khani, F., et al. (2018). Health risk assessments due to nitrate levels in drinking water in villages of Azadshahr, northeastern Iran. Environ. Earth Sci. 77:782. doi: 10.1007/s12665-018-7973-6
Rabeiy, R. E. (2018). Assessment and modeling of groundwater quality using WQI and GIS in Upper Egypt area. Environ. Sci. Pollut. Res. 25, 30808–30817. doi: 10.1007/s11356-017-8617-1
Rajmohan, N., and Elango, L. (2004). Identification and evolution of hydrogeochemical processes in the groundwater environment in an area of the Palar and Cheyyar River Basins, Southern India. Environ. Geol. 46, 47–46.
Ren, X., Gao, Z., An, Y., Liu, J., Wu, X., He, M., et al. (2020). Hydrochemical and isotopic characteristics of groundwater in the Jiuquan East Basin, China. Arab. J. Geosci. 13:545. doi: 10.1007/s12517-020-05573-7
Ren, X., Yu, R., Kang, J., Lü, C., Wang, R., Li, Y., et al. (2022). Water pollution characteristics and influencing factors of closed lake in a semiarid area: a case study of Daihai Lake, China. Environ. Earth Sci. 81:393. doi: 10.1007/s12665-022-10526-2
Sener, S., Sener, E., and Davraz, A. (2017). Evaluation of water quality using water quality index (WQI) method and GIS in Aksu River (SW-Turkey). Sci. Total Environ. 584-585, 131–144. doi: 10.1016/j.scitotenv.2017.01.102
Su, H., Kang, W., Xu, Y., and Wang, J. (2017). Assessing Groundwater Quality and Health Risks of Nitrogen Pollution in the Shenfu Mining Area of Shaanxi Province, Northwest China. Exposure Health 10, 77–97. doi: 10.1007/s12403-017-0247-9
Sun, W., Ni, Z., Meng, X., Jiang, Q., and Zhang, E. (2021). Environmental change recorded by radionuclides and organic geochemical signatures in a sediment core from Lake Daihai, North China. Catena 206:105564. doi: 10.1016/j.catena.2021.105564
Sunkari, E. D., Seidu, J., and Ewusi, A. (2022). Hydrogeochemical evolution and assessment of groundwater quality in the Togo and Dahomeyan aquifers, Greater Accra Region, Ghana. Environ. Res. 208:112679. doi: 10.1016/j.envres.2022.112679
Talib, M. A., Tang, Z., Shahab, A., Siddique, J., Faheem, M., and Fatima, M. (2019). Hydrogeochemical Characterization and Suitability Assessment of Groundwater: A Case Study in Central Sindh, Pakistan. Int. J. Environ. Res. Public Health 16:886. doi: 10.3390/ijerph16050886
USEPA (1989). Risk Assessment Guidance for Superfund Volume I: Human Health Evaluation Manual (Part A). Washington DC: Office of Emergency and Remedial Response.
USSL (1954). Agriculture Hand Book 60: Diagnosis and Improvement of Saline and Alkali Soils. Washington, DC: US Department of Agriculture (USDA), 69–81.
Wang, L. (2021). Characteristics of Groundwater in Daihai Basin and Its Influence on the Change of Seawater Balance in Daihai Basin. Hohhot: Inner Mongolia University.
Wang, S., Xu, C., Zhang, W., Chen, H., and Zhang, B. (2022a). Human-Induced water loss from closed inland Lakes: Hydrological simulations in China’s Daihai lake. J. Hydrol. 607:127552. doi: 10.1016/j.jhydrol.2022.127552
Wang, X., Zheng, W., Tian, W., Gao, Y., Wang, X., Tian, Y., et al. (2022b). Groundwater hydrogeochemical characterization and quality assessment based on integrated weight matter-element extension analysis in Ningxia, upper Yellow River, northwest China. Ecol. Indicat. 135:108525. doi: 10.1016/j.ecolind.2021.108525
Wang, Y., Shen, Y., Guo, Y., Li, B., Chen, X., Guo, X., et al. (2022c). Increasing shrinkage risk of endorheic lakes in the middle of farming-pastoral ecotone of Northern China. Ecol. Indicat. 135:108523. doi: 10.1016/j.ecolind.2021.108523
Wang, S. H., Bai, M. X., Chen, J. Y., Zhao, L., Zhang, B., Guo, Y. Y., et al. (2019). Research on the ecological protection and restoration of mountain-river-forest-farmland-lake-grassland system in typical farming-pastoral ecotone: taking Daihai Lake Basin in Inner Mongolia as an example. J. Environ. Eng. Technol. 9, 515–519.
Wang, T., Chen, J. S., Xu, Y., Zhan, L. C., and Huang, D. W. (2017). Isotopes and hydrochemistry of Daihai Lake recharging sources, Northern China. J. Radioanal. Nucl. Chem. 312, 615–629. doi: 10.1007/s10967-017-5241-y
Wang, Y., and Li, P. (2022). Appraisal of shallow groundwater quality with human health risk assessment in different seasons in rural areas of the Guanzhong Plain (China). Environ. Res. 207:112210. doi: 10.1016/j.envres.2021.112210
Wilcox, L. (1955). Classification and Use of Irrigation Waters. Washington, DC: US Department of Agriculture.
Xiao, J., Jin, Z., and Wang, J. (2014). Assessment of the hydrogeochemistry and groundwater quality of the Tarim River Basin in an extreme arid region, NW China. Environ. Manag. 53, 135–146. doi: 10.1007/s00267-013-0198-2
Xiao, J., Wang, L., Chai, N., Liu, T., Jin, Z., and Rinklebe, J. (2021). Groundwater hydrochemistry, source identification and pollution assessment in intensive industrial areas, eastern Chinese loess plateau. Environ. Pollut. 278:116930. doi: 10.1016/j.envpol.2021.116930
Xiao, J., Zhang, F., and Jin, Z. (2016). Spatial characteristics and controlling factors of chemical weathering of loess in the dry season in the middle Loess Plateau, China. Hydrol. Process. 30, 4855–4869. doi: 10.1002/hyp.10959
Xiao, Y., Liu, K., Hao, Q., Li, Y., Xiao, D., and Zhang, Y. (2022). Occurrence, Controlling Factors and Health Hazards of Fluoride-Enriched Groundwater in the Lower Flood Plain of Yellow River, Northern China. Exposure Health 14, 345–358. doi: 10.1007/s12403-021-00452-2
Xu, Q. H., Xiao, J. L., Zhongcun, J. F., Yang, X. L., Zheng, Z. H., Liang, W. D., et al. (2004). Pollen evidence of vegetation and climate changes in Daihai Lake area during the Holocene. J. Glaciol. Geocryol. 26, 73–80.
Yan, J., Chen, J., and Zhang, W. (2021). Study on the groundwater quality and its influencing factor in Songyuan City, Northeast China, using integrated hydrogeochemical method. Sci. Total Environ. 773:144958. doi: 10.1016/j.scitotenv.2021.144958
Yang, Q., Li, Z., Ma, H., Wang, L., and Martín, J. D. (2016). Identification of the hydrogeochemical processes and assessment of groundwater quality using classic integrated geochemical methods in the Southeastern part of Ordos basin, China. Environ. Pollut. 218, 879–888. doi: 10.1016/j.envpol.2016.08.017
Yidana, S. M., Ophori, D., and Banoeng-Yakubo, B. (2008). A multivariate statistical analysis of surface water chemistry data–the Ankobra Basin, Ghana. J. Environ. Manag. 86, 80–87. doi: 10.1016/j.jenvman.2006.11.023
Zhang, W. Q., Dong, S. G., Ma, M. Y., Zhao, Z., and Chen, Y. (2021). Chemical characteristics and origin of groundwater in the Daihai basin. Arid Zone Res. 38, 1546–1555.
Zhang, Z. (2020). Study on the relationship between groundwater and surface water in Daihai Basin. Shijiazhuang: Hebei GEO University.
Zhao, L., Chen, J. Y., Jiang, X., Zheng, S. F., and Wang, S. H. (2020). Temporal and Spatial Distribution Characteristics and Difference Analysis of Nitrogen and Phosphorus in Daihai Lake. Environ. Sci. 41, 1676–1683.
Keywords: water body hydrochemistry, water quality, human health risk, influencing factors, Daihai Lake Basin
Citation: Ren X, Yu R, Kang J, Li X, Wang R, Zhuang S, Wang D and Zhang X (2022) Hydrochemical evaluation of water quality and its influencing factors in a closed inland lake basin of Northern China. Front. Ecol. Evol. 10:1005289. doi: 10.3389/fevo.2022.1005289
Received: 28 July 2022; Accepted: 29 August 2022;
Published: 16 September 2022.
Edited by:
Chengcheng Xia, Sichuan University, ChinaReviewed by:
Hongbo Zhang, Chang’an University, ChinaLiana Vuta, Politehnica University of Bucharest, Romania
Copyright © 2022 Ren, Yu, Kang, Li, Wang, Zhuang, Wang and Zhang. This is an open-access article distributed under the terms of the Creative Commons Attribution License (CC BY). The use, distribution or reproduction in other forums is permitted, provided the original author(s) and the copyright owner(s) are credited and that the original publication in this journal is cited, in accordance with accepted academic practice. No use, distribution or reproduction is permitted which does not comply with these terms.
*Correspondence: Ruihong Yu, rhyu@imu.edu.cn